- 1UMR 1014 SECALIM, Oniris, Nantes, France
- 2Institute of Chemical Technology, Faculty of Food and Biochemical Technology, Department of Biochemistry and Microbiology, Prague, Czech Republic
- 3CNRS-UMR 8030 and Commissariat à l’Energie Atomique et aux Energies Alternatives CEA/DRF/IG/Genoscope LABGeM, Evry, France
Campylobacter jejuni is the leading cause of bacterial enteritis in Europe. Human campylobacteriosis cases are frequently associated to the consumption of contaminated poultry meat. To survive under environmental conditions encountered along the food chain, i.e., from poultry digestive tract its natural reservoir to the consumer’s plate, this pathogen has developed adaptation mechanisms. Among those, biofilm lifestyle has been suggested as a strategy to survive in the food environment and under atmospheric conditions. Recently, the clinical isolate C. jejuni Bf has been shown to survive and grow under aerobic conditions, a property that may help this strain to better survive along the food chain. The aim of this study was to evaluate the adhesion capacity of C. jejuni Bf and its ability to develop a biofilm. C. jejuni Bf can adhere to abiotic surfaces and to human epithelial cells, and can develop biofilm under both microaerobiosis and aerobiosis. These two conditions have no influence on this strain, unlike results obtained with the reference strain C. jejuni 81-176, which harbors only planktonic cells under aerobic conditions. Compared to 81-176, the biofilm of C. jejuni Bf is more homogenous and cell motility at the bottom of biofilm was not modified whatever the atmosphere used. C. jejuni Bf whole genome sequence did not reveal any gene unique to this strain, suggesting that its unusual property does not result from acquisition of new genetic material. Nevertheless some genetic particularities seem to be shared only between Bf and few others strains. Among the main features of C. jejuni Bf genome we noticed (i) a complete type VI secretion system important in pathogenicity and environmental adaptation; (ii) a mutation in the oorD gene involved in oxygen metabolism; and (iii) the presence of an uncommon insertion of a 72 amino acid coding sequence upstream from dnaK, which is involved in stress resistance. Therefore, the atypical behavior of this strain under aerobic atmosphere may result from the combination of insertions and mutations. In addition, the comparison of mRNA transcript levels of several genes targeted through genome analysis suggests the modification of regulatory processes in this strain.
Introduction
Campylobacter is a Gram-negative bacterium, spiral-shaped and motile. This human pathogen lives as commensal of the gastrointestinal tract of most warm-blooded animals, especially poultry but also mammals (Park, 2002). Human infection by Campylobacter is commonly associated to the consumption of contaminated poultry meat. The genus Campylobacter includes very heterogeneous species that are present in a variety of environments but more than 80% of confirmed cases of campylobacteriosis were reported to be associated to Campylobacter jejuni (EFSA and ECDC, 2016).
The clinical manifestation of campylobacteriosis is severe gastro enteritis. However, Campylobacter infection is occasionally a precursor of serious post-infectious illness, including immune-reactive complications such as Guillain Barré and Miller Fisher Syndromes, two chronic and potentially fatal forms of paralysis (WHO, 2013). Since 2005, Campylobacter has been the most commonly reported human gastrointestinal bacterial pathogen in the European Union (EFSA and ECDC, 2016). In 2014, 236,851 cases of human campylobacteriosis were reported in EU. This zoonosis represents an incidence rate of 71 per 100,000 population exceeding the number of salmonellosis, which has a notification rate of 23.4 cases per 100,000 population. In addition, the cost of campylobacteriosis to public health systems and the loss of individual health and productivity were evaluated around 2.4 billion Euros per year in Europe (EFSA and ECDC, 2016) and between 1.2 and 4 billion $ for the US (Eberle and Kiess, 2012; Batz et al., 2014). The need for controlling this pathogen along the food chain explains the numerous studies reported in the literature that aimed at understanding its metabolism and virulence.
Campylobacter jejuni presents specific growth requirements, as it is thermotolerant with an optimal growth temperature of 40–42°C, microaerophilic (optimal O2 concentration of 5%), and capnophilic requiring 10% CO2 for an optimal growth. However, C. jejuni is able to persist in different environmental stress conditions explaining its high prevalence around the world. This food-borne pathogen has indeed developed adaptation mechanisms to survive under various harsh conditions it can encounter, from poultry gastrointestinal tract to the consumer’s plate. One of the most important characteristics of this bacterium is its ability to survive in aerobic environments despite its microaerophilic nature. This suggests an ability to cope with oxidative stress mediated by environmental oxygen tension and reactive oxygen species. To survive against such stresses, biofilm formation has been suggested to be one of the strategies used by this pathogen to persist in the environment (Buswell et al., 1998; Nguyen et al., 2012; Turonova et al., 2015). Commonly, biofilms are defined as multicellular layers of bacteria embedded within a matrix of extracellular polymeric substances (EPSs; Costerton, 1995; Costerton et al., 1995; Donlan, 2002; Donlan and Costerton, 2002). C. jejuni strains have been reported to be able to form different types of biofilm characterized as a structure attached to a surface, a pellicle formed at the surface of the liquid, or aggregates floating in the liquid culture (Joshua et al., 2006). Recently, we have reported the atypical property of C. jejuni Bf, a strain able to grow on plates under aerobic atmosphere, thus with a very low concentration of CO2 (0.035%), but with 21% O2 (Rodrigues et al., 2015). The possible growth of C. jejuni strains under aerobiosis and after various oxidative stresses was previously reported (Chynoweth et al., 1998; Garénaux et al., 2008b; Hinton, 2016). The aim of this study was to investigate the ability of C. jejuni Bf to adhere to biotic and abiotic surfaces and to form biofilm. We compared the behavior of this strain under both microaerobiosis and aerobiosis to determine a possible increased capacity to resist to the presence of high level of O2, which can be encountered during meat products processing and storage. Finally, genome comparison was also performed in order to detect genetic elements putatively involved in the phenotype of this strain. For that purpose, the draft genome (Bronnec et al., 2016) was completed and the gene and metabolic repertoires of C. jejuni Bf were compared to those of other complete or draft genomes.
Materials and Methods
Bacterial Strain and Culture Conditions
Stains used in this study are presented Table 1. C. jejuni strains were stored at -80°C in Brain Heart Infusion broth (BHI) containing 20% (vol/vol) glycerol. Prior to each experiment frozen cells were streaked on Karmali agar plates (Oxoid Limited, UK), incubated at 42°C for 24 h under microaerobic conditions in CampyGen sachet (Oxoid Limited, UK): 5% oxygen, 10% carbon dioxide, and 85% nitrogen.
As described previously by Rodrigues et al. (2015), C. jejuni Bf cells can be acclimated to aerobic conditions (namely AAC cells for aerobically acclimated cells). This was performed by sub-culturing three times (once for 48 h and then twice 24 h) on Karmali agar plates under aerobiosis (air; Rodrigues et al., 2015). In order to maintain the same conditions for all samples, cultures under microaerobiosis were identically performed three times under microaerobiosis (MAC cells for microaerobic conditions).
Adhesion to Inert Surfaces
The adhesion capability was evaluated using BioFilm Ring Test® (BioFilm Control, France) as described by Sulaeman et al. (2010), with several modifications. Briefly, the experiments were performed using the kit commercialized by BioFilm Control (KITC004) including polystyrene Costar plates with flat bottom (Corning, USA), magnetic beads solution (TON004) and contrast liquid (LIC0001). Two conditions were tested for adhesion assay, microaerobiosis and aerobiosis. Grown cells were recovered from Karmali agar plates and suspended at 108 CFU/mL (OD610 nm = 0.5 ± 0.1) in filtered BHI (provided with the kit). C. jejuni suspensions (200 μL), containing magnetic beads at 1% (vol/vol), were inoculated in Costar plate wells. After 2 h of incubation at 42°C, the adhesion capability of strains was evaluated by measuring a biofilm formation index (BFI) with the BFC Element 3 software (BioFilm Control, France). Assays were repeated at least three times with three technical replicates.
Confocal Laser Scanning Microscopy (CLSM)
Static Biofilm Formation Assay
Campylobacter jejuni Bf and C. jejuni 81-176 cells were recovered from Karmali agar plates and suspended in BHI at 108 CFU/mL (OD610 nm = 0.5 ± 0.1). Two hundred microliters of bacterial suspension were inoculated in sterile 96-well polystyrene microtiter plates with a micro-clear® bottom 190 ± 5 μm (Greiner Bio One, Germany). Several incubation times (30 min, 1, 2, 4 h) at 42°C were tested to evaluate the minimum time required for adhesion of the cells at the bottom of the well. Adhesion was performed under microaerobiosis (with bacteria first grown under microaerobiosis) and under aerobiosis (with C. jejuni Bf grown under aerobiosis and C. jejuni 81-176 grown under microaerobiosis). Then, the bacterial suspension in the microtiter plate was carefully replaced with 200 μl of sterile BHI. Plates were then incubated at 42°C for 24 and 48 h under microaerobic or aerobic conditions. At least 1 h before the biofilm observation, the cells were stained by adding Syto 9 at 0.01 mM final concentration (LIVE/DEAD® Kit, Life Technologies, USA) directly into the wells, following the method of Turonova et al. (2015). Experiments were performed using three biological replicates. For each condition, three technical replicates were performed, and two acquisitions in each of them.
Confocal Laser Scanning Microscopy
After staining, image acquisition was performed using a spinning disk confocal microscope (Andor, UK; Olympus, Japan). The entire wells were first inspected to see biofilm formation and its global structure. Two different locations of each well were scanned using a 10X objective lens with the signal recorded in the green channel (excitation 488 nm, emission 500–525 nm). The chosen place for the acquisition was representative of the whole structure and a stack of horizontal planar images with a size of x = 670.8 μm and y = 897.84 μm (e.g., 1040 × 1392 pixels) was scanned with a z-step of 1 μm. Video acquisitions were performed in a selected layer of the same size as described before using a 40X NA 1.4 oil immersion objective lens with an exposure time of 100 ms. Acquisitions were achieved in three distinct positions in the biofilm structure: the bottom, middle and top of the biofilm.
Image Processing
Confocal laser scanning microscopy (CLSM) images from top to bottom were processed using IMARIS software (v 7.6, Bitplane AG, Switzerland). For visualization of the biofilm, shadow projections and three-dimensional structures were generated. Beside the biofilm appearance, quantitative structural parameters of biofilms were calculated. Biofilm volume and thickness were the selected parameters used to compare the architectural differences of the biofilms formed. The bio-volume corresponds to the total volume of cells in the acquired field (x × y × z = μm3) and the thickness is the maximum height reached by the biofilm (μm).
Adhesion Assay to Epithelial Intestinal Cells In vitro
Human intestinal cell lines HT29 and HT29-MTX were used to compare adhesion abilities of C. jejuni NCTC 11168, C. jejuni 81-176 and C. jejuni Bf under microaerobic conditions. In addition, adhesion capabilities of C. jejuni Bf acclimated to ambient air were also assessed. Maintenance of cells and adhesion assays were performed according to Haddad et al. (2010). Briefly, intestinal cells were grown in Dulbecco’s minimum essential medium (DMEM) supplemented with 10% fetal bovine serum (FBS), containing 200 mM L-glutamine, 250 μg/mL gentamicin (Sigma-Aldrich, USA) and 2.5 μg/mL amphotericin B (Sigma-Aldrich, USA). The cells were grown routinely in tissue culture flasks at 37°C in a 5% CO2-humidified atmosphere.
For experimental assays, cultured cells were dissociated from plastic flasks using trypsin-EDTA solution (Invitrogen, USA) and approximately 105 eukaryotic cells were seeded into each well of 24-well tissues culture tray and incubated for 5 days at 37°C in humidified atmosphere at 5% of CO2. The cells were washed with DMEM and each well was inoculated with a suspension of approximately 107 CFU of bacteria. To evaluate the number of adhered bacterial cells, the infected monolayers were incubated for 1 h at 37°C in a humidified 5% CO2 incubator and rinsed five times with phosphate buffered-saline (PBS, Eurobio, France). The cell monolayer was lysed by addition of 0.5 mL of Triton X-100 0.1% (Labo-Si, France) at room temperature for 30 min. C. jejuni cells were enumerated from the lysate on Karmali agar plates after 48 h incubation at 42°C under microaerobic condition. Experiments were performed using three biological replicates, and for each two technical replicates.
Genome Sequence Completion and Comparative Genomic Analysis
To complete the draft genome sequence of C. jejuni Bf (Bronnec et al., 2016), PCR amplifications were performed on regions presenting uncertainties and for gap-filling purpose on contig extremities with primers designed in the flanking regions of each gap and PCR products were sequenced (Biofidal, France). As genome comparison showed that C. jejuni Bf was closer to other genomes than that of the reference genome of C. jejuni NCTC 11168 a new mapping was performed on the closest complete genome available (C. jejuni ATCC 32488 SRZ049709). Automatic annotation was performed on the MicroScope platform (MaGe; Vallenet et al., 2006, 2013) and manually checked.
Nucleotide sequence accession number: this whole genome project has been deposited in ENA under the accession no. FCEZ01000001-FCEZ01000095. The version described in this paper is the second version, FCEZ01000001-FCEZ01000095.
Using the tools available on the MicroScope platform, genomic comparisons were conducted between C. jejuni Bf genome and other C. jejuni genomes listed in Supplementary Table S1. A total of 33 complete and 19 draft C. jejuni genomes were used. “PkGDB Synteny Statistics” tool was used to perform similarity analysis between C. jejuni Bf and all C. jejuni genomes available to date on the PkGDB database. “Gene phyloprofile” tool has enabled the genomic comparison by searching specific genes of C. jejuni Bf in comparison with the other genomes, with the following homology constraints: minLrap ≥ 0.8, maxLrap ≥ 0 and identity ≥ 30%.
RNA Isolation and Reverse Transcription
After growth AAC or MAC C. jejuni cells were recovered from Karmali plates and suspended in BHI at 108 CFU/mL (OD610 nm = 0.5 ± 0.1). RNA isolation, control and reverse transcription were performed according to Haddad et al. (2012) with some modifications. Briefly, one milliliter of this suspension was centrifuged at 3,300 g for 6 min at 4°C, and then resuspended in 1 mL of Extract-All (Eurobio, France) and mixed with 0.2 mL of chloroform. After a centrifugation at 12,000 g during 15 min at 4°C, RNAs from the aqueous phase were precipitated with isopropanol, washed twice in cold 75% ethanol and then solubilized in 50 μL of RNase-free water. Samples were then treated with TurboDNase (Life Technologies, France) to remove potential DNA contamination. The integrity of RNA was verified using 1% agarose gel and quantified using a NanoDrop spectrophotometer (Thermo Scientific, France). Absence of DNA contamination was validated by PCR. RNA was isolated from three biological replicates. Reverse transcription was performed on 100 ng of RNA using the RevertAid H Minus First-Strand cDNA synthesis kit (Euromedex, France) using random hexamer primers according to the manufacturer’s instructions.
Quantitative Real-Time PCR
The quantitative real-time PCR assay was performed using SYBR Green I (Applied Biosystems, USA) and MJ Research PTC-200 Thermal Cycler (GMI, USA). The chosen internal control was rrs (Hyytiäinen et al., 2012) with primers rrs_F AAGGGCCATGATGACTTGACG and rrs_R AGCGCAACCCACGTATTTAG. The studied genes were cosR (with primers cosR_F TTTGAAAGCTGGAGCTGATG and cosR_R GGTTCCGCCAAGTCTTAGTC) and dnaK (DnaK)_F AAACGCCAAGCGGTAACTAA and DnaK_R TTCTTTAGCCGCGTCTTCAT). The operon oorDABC (with primers oorD2_F TGCGGTTTTAGGACAAATGA and oorD2_R TTCATCTCTTTTTGCCACCA, oorA2_F GCGGCAATGAGTGGAGTAAA and oorA2_R TTGGAAGACCTGTTGAAGGA, oorB2_F TGGTAAGTGGAGATGGGGATA and oorB2_R GTTGGGCTTGTTTGGGAAT, oorC_F GTGGTGGCCCTACTAAGGTG and oorC_R AACCCTTATCTGCAGTCGAAA) was also studied. Finally, a CDS of unknown function (u30002_F TTCAGAACCTACGAGGATGGA and u30002_R TTCAATCCTCCAAGCACACA) located upstream from dnaK was also investigated. The PCR mix was prepared as follows: 100 ng to 1 μg of cDNA (for cosR expression or oorDABC, dnaK, and u30002_F), 1 μM of each primers and 12.5 μL of SYBR Green I Master Mix. The amplification program included an initial denaturing step of 10 min at 95°C followed by 40 cycles of 15 s at 95°C and 1 min at 60°C. A negative control was included in each run. Relative quantification of gene expression was calculated according to the 2-ΔΔCt method (Livak and Schmittgen, 2001). Results were normalized to the gene transcription of the reference strain C. jejuni 81-176 in microaerobic conditions. The experiments were performed in triplicate from three independent cultures. For each experiment, at least three technical replicates were realized.
Statistical Analysis
Adhesion results from Biofilm Ring Test were analyzed using Statgraphics Centurion software version 17.1.06 (Statpoint Technologies, USA). An analysis of variance (ANOVA) was assessed to determine the individual effect of each variable (species and atmosphere). Statistical data were completed using the Fisher LSD (least significant difference) technique for multiple comparisons with a significance level at 95%.
Numerical data on biofilm formation obtained from IMARIS were also assessed for an ANOVA. The two variables identified were the maximum height of biofilm and the biomass volume. The two factors considered were the time of biofilm formation (24 or 48 h) and the combination strain/atmosphere, e.g., C. jejuni 81-176 grown under microaerobiosis (81-176μO2), C. jejuni Bf under microaerobiosis (BfμO2) and C. jejuni Bf under aerobiosis (BfO2). This procedure allows the analysis of variance at several factors for each variable. Significant effects were considered when p-value < 0.05.
Results obtained for the adhesion assay to epithelial intestinal cells in vitro and from RT-qPCR were analyzed using Student’s t-test. p-value < 0.05 were considered statistically significant.
Results
Adhesion Capability and Biofilm Ultrastructure to Abiotic Surfaces
Ability to Adhere to Abiotic Surface
Adhesion assays using BioFilm Ring Test® method were conducted under microaerobic and aerobic conditions with an initial bacterial concentration of 5 × 106 CFU/well. According to the biofilm formation index measured with the BFC Element 3 software all strains showed adhesion capacity and could be classified into four groups: strains with strong (0 ≤ BFI < 4), delayed (4 ≤ BFI < 7), or weak adhesion (7 ≤ BFI < 16), and those showing no adhesion capacity (BFI ≥ 16; Figure 1).
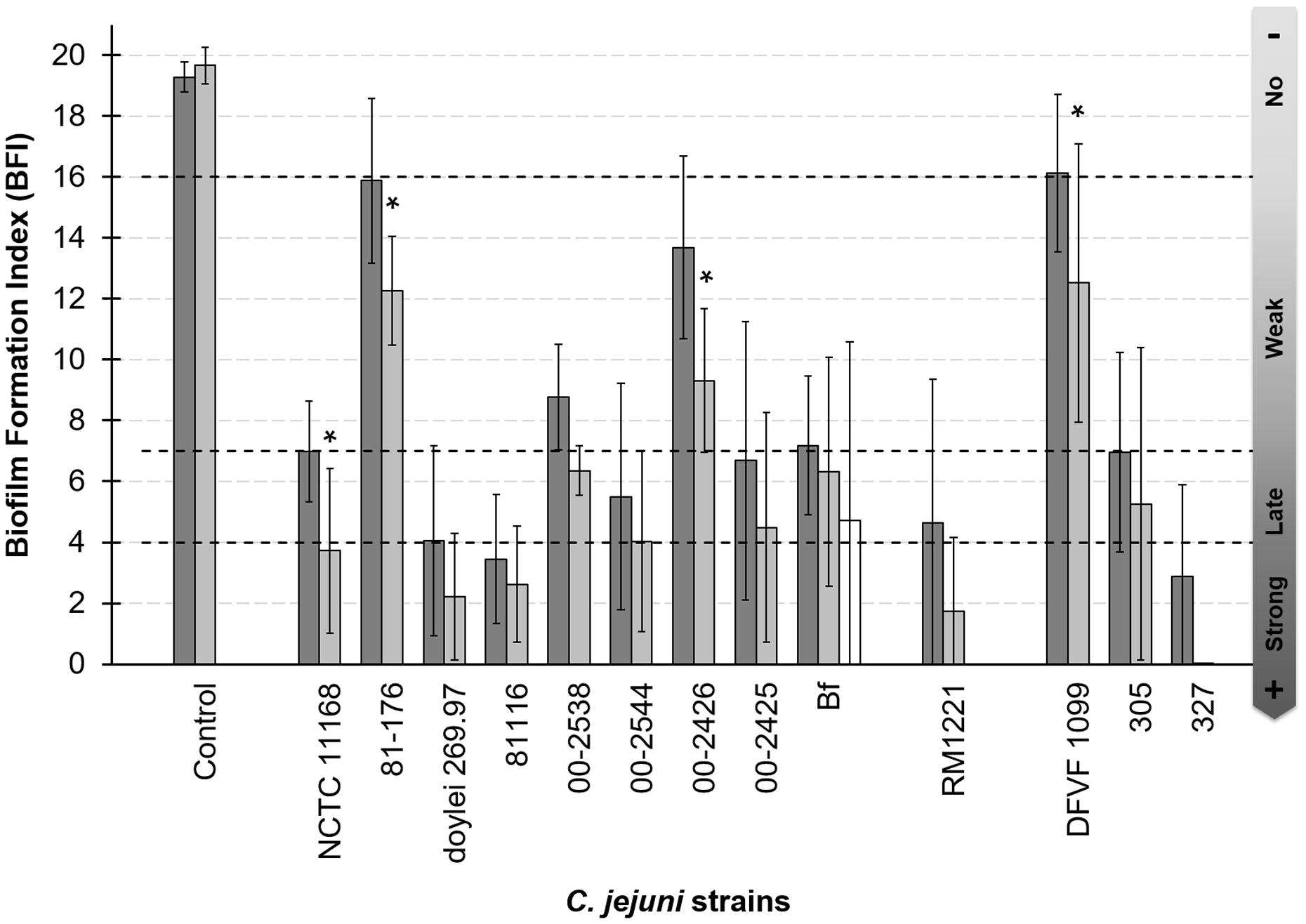
FIGURE 1. Adhesion capability to polystyrene of Campylobacter jejuni is strain dependent. Adhesion capability was measured after 2 h of incubation at 42°C under microaerobiosis with MAC grown cells (dark bars) and under aerobiosis with MAC grown cells (gray bars). White bar indicate biofilm formation index under aerobiosis of C. jejuni Bf AAC cells. The nine first strains were clinical isolates, the 10th was isolated from meat and three last strains were isolated from poultry. Four biological replicates were performed in microerobiosis and three in aerobiosis. Three technical replicate were realized each time. For the control and each strain, the mean values of BFI between O2 and μO2 conditions were compared. Asterisks show when these values were statistically different between the two conditions (p-value < 0.05).
Among the 13 strains tested the ability to adhere to polystyrene varied independently from their clinical, animal, or food origin. Three strains were considered as strongly adherent (C. jejuni subsp. jejuni 81116, 327 and C. jejuni subsp. doylei 269.97), six showed a delayed adhesion (C. jejuni Bf, NCTC 11168, RM1221, 00-2544, 00-2425, and 305), and three presented a weak adhesion (C. jejuni 00-2538, 00-2426, 81-176). C. jejuni DFVF1099 appeared non-adherent under microaerobiosis. Although, the BFI values did not significantly differ between microaerobiosis and aerobiosis. Aerobiosis improved adhesion of C. jejuni NCTC 11168, 81-176, 00-2425 and DFVF (p < 0.05), and only a statistically non-significant tendency to better adhere was observed for the other strains. As among these strains, C. jejuni Bf is the only one able to grow on plate under aerobic condition (Rodrigues et al., 2015), the adhesion capability of cells grown under aerobiosis was also tested. As shown Figure 1 C. jejuni Bf grown aerobically was able to adhere to inert surface as well as cells grown microaerobically, and the BFI did not statistically differed between these two conditions. Although, our adhesion results seemed contradictory with previous studies (Gunther and Chen, 2009; Sulaeman et al., 2010; Turonova et al., 2015), we chose to explore the capacity of biofilm formation of C. jejuni Bf in comparison to C. jejuni 81-176 because this virulent strain is consistently capable of producing mature biofilm (Gunther and Chen, 2009) and often considered as the reference. In addition, this strain could be used as a positive control for biofilm formation by CLSM and its well annotated genome was available.
Biofilm Development and Three-Dimensional Structure
We determined that a period of 2 h of adhesion to the polystyrene resulted in optimal initiation of biofilm formation for the two strains (data not shown).
After 24 h at 42°C under microaerobiosis, C. jejuni 81-176 developed a compact and highly structured biofilm strongly condensed at well center (Figure 2A, Supplementary Figure S1A). After 48 h of incubation the biofilm observed was quite similar with thick and dense structures (data not shown). Under the same conditions, C. jejuni Bf was also capable of forming biofilm but its structure seemed more expanded in the well and more flat in comparison with that of C. jejuni 81-176 (Figure 2B, Supplementary Figure S1B). The structure was less compact with a patchy coverage of the surface and composed by few large and compact structures and several microcolonies (Figure 2B, Supplementary Figure S1B).
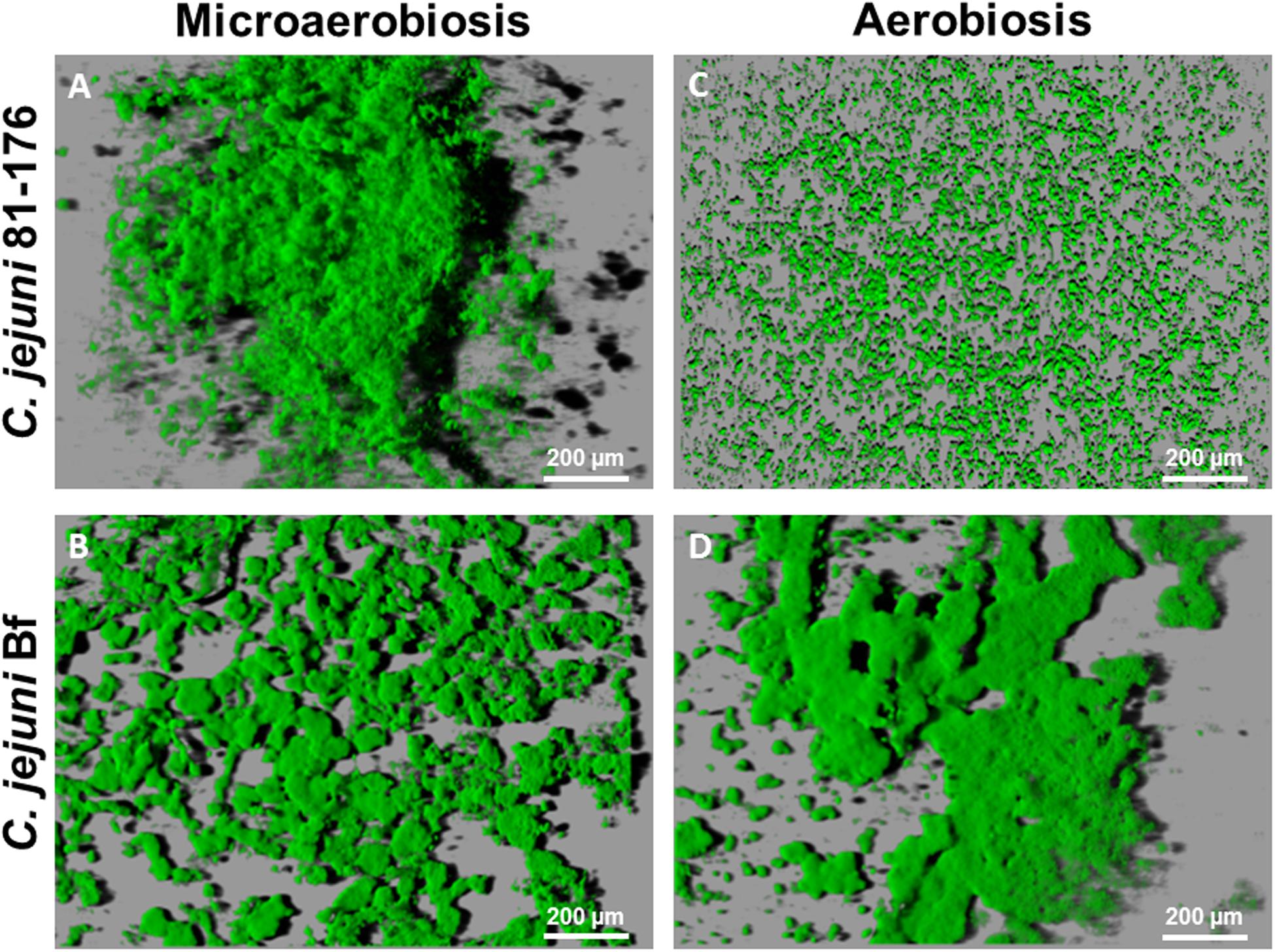
FIGURE 2. Campylobacter jejuni biofilm architecture after IMARIS processing of CLSM images from top to bottom. Shadows projections were acquired for the biofilm developed after 24 h incubation at 42°C. Under microaerobiosis C. jejuni 81-176 (A) and C. jejuni Bf (B) established a biofilm. Under aerobiosis C. jejuni Bf (D) was able to develop a biofilm and C. jejuni 81-176 (C) persisted in its planktonic state.
During incubation under aerobiosis C. jejuni 81-176 did not develop any biofilm but rather, harbored microcolonies of surface attached cells (Figure 2C). In contrast C. jejuni Bf biofilm appeared more compact and structured under aerobic condition, as compared to the one formed in microaerobiosis (Figure 2D, Supplementary Figure S1C). After 48 h of cultivation at 42°C, biofilm formed by C. jejuni Bf was more compact with micro colonies less spread around the surface of the well (data not shown).
Quantification and Comparison of Biofilm Structures
The quantity of biofilm was characterized using two variables: bio-volume and maximum thickness. The individual effect of different factors (duration of cultivation, strain, atmosphere) on the two variables were considered (Figure 3). For each variable, the period of biofilm cultivation (24 or 48 h) had no significant effect. Multiple-comparison procedure was used to determine the significantly different means (Supplementary Table S2). For maximum thickness the Fisher’s LSD method revealed two significantly different groups T1 and T2. The first group (T1) encompasses biofilm structure formed by C. jejuni 81-176 and the second group (T2) is composed by biofilms formed by C. jejuni Bf under both microaerobic and aerobic conditions. Conversely, a unique homogeneous group (V) was obtained when considering biofilm volume, independently from the strain or the conditions tested.
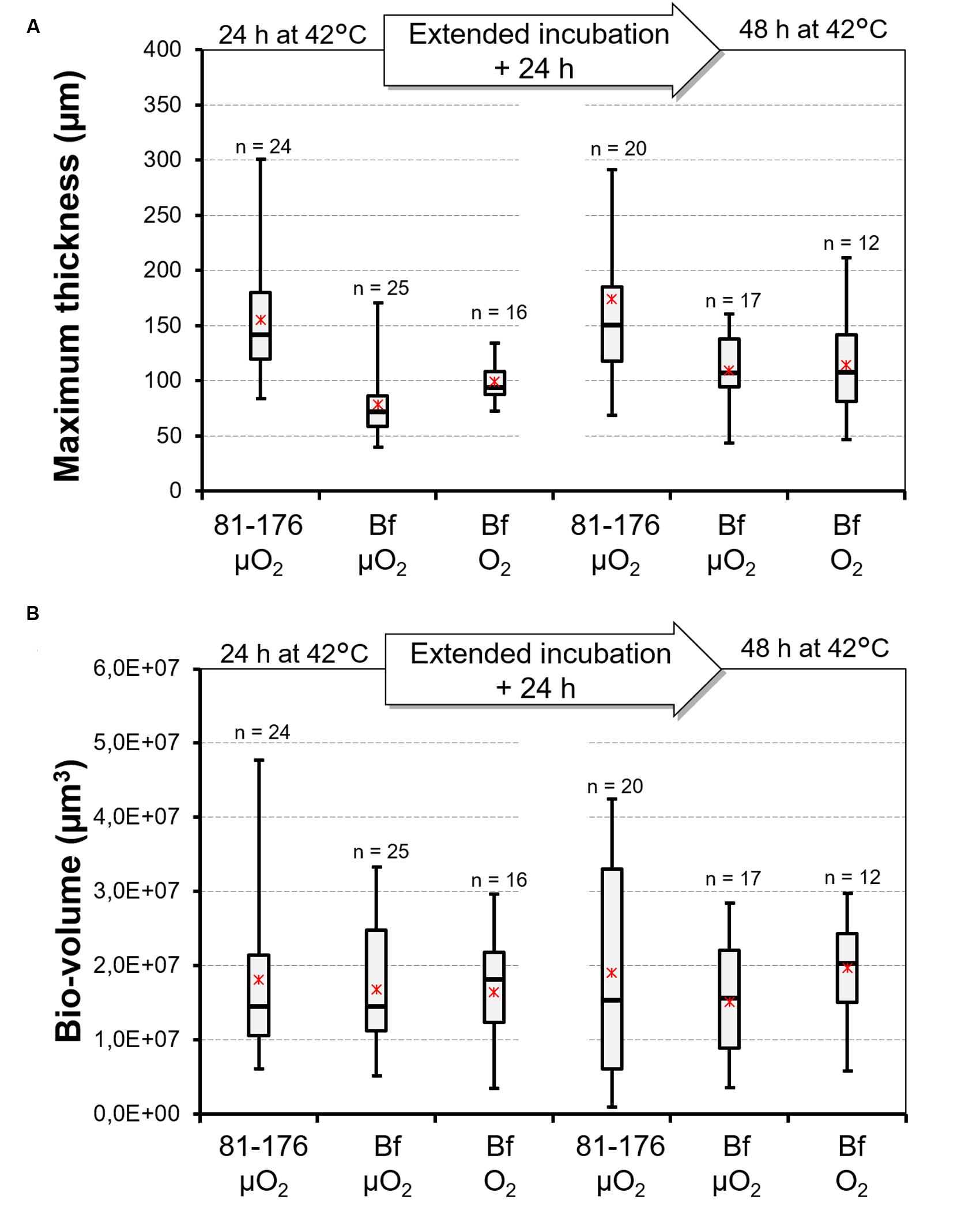
FIGURE 3. Distribution of data describing the biofilm architecture of C. jejuni 81-176 and C. jejuni Bf. Box plot representing the distribution of maximum thickness (A) and bio-volume (B) values observed for the biofilms developed by C. jejuni 81-176 and C. jejuni Bf under microaerobiosis (μO2) and under aerobiosis (O2) after 24 and 48 h of incubation at 42°C. Minimum and maximum values are reported. Asterisks indicate the mean and dashes the median. The number of repeats was added above each box plot.
Cell Motility Observation
As reported previously (Turonova et al., 2015), we observed motile C. jejuni 81-176 cells at different locations of the biofilm structure (e.g., at the bottom, middle, and top) after 24 and 48 h of biofilm formation. Similarly, a subpopulation of C. jejuni Bf also showed the capacity to move within the biofilm structure in the two conditions tested (Supplementary files S1 and S2). A better motility was detected at the bottom of the biofilm where the structure is more dispersed. No obvious difference was observed in the motility of C. jejuni Bf under microaerobiosis or aerobiosis.
C. jejuni Bf Adhesion to Epithelial Intestinal Cells In vitro
In addition to interaction with abiotic surfaces, we also determined the ability of C. jejuni Bf to adhere to biotic surfaces. For that purpose, the adhesion of C. jejuni Bf to HT29 and HT29-MTX cells was compared to those of C. jejuni 81-176 and NCTC 11168. The presence or absence of mucus did not significantly affect the adhesion of C. jejuni Bf and C. jejuni NCTC 11168 strains to intestinal cells (p-value < 0.05), whereas C. jejuni 81-176 adhered better to mucus producing cells (Figure 4).
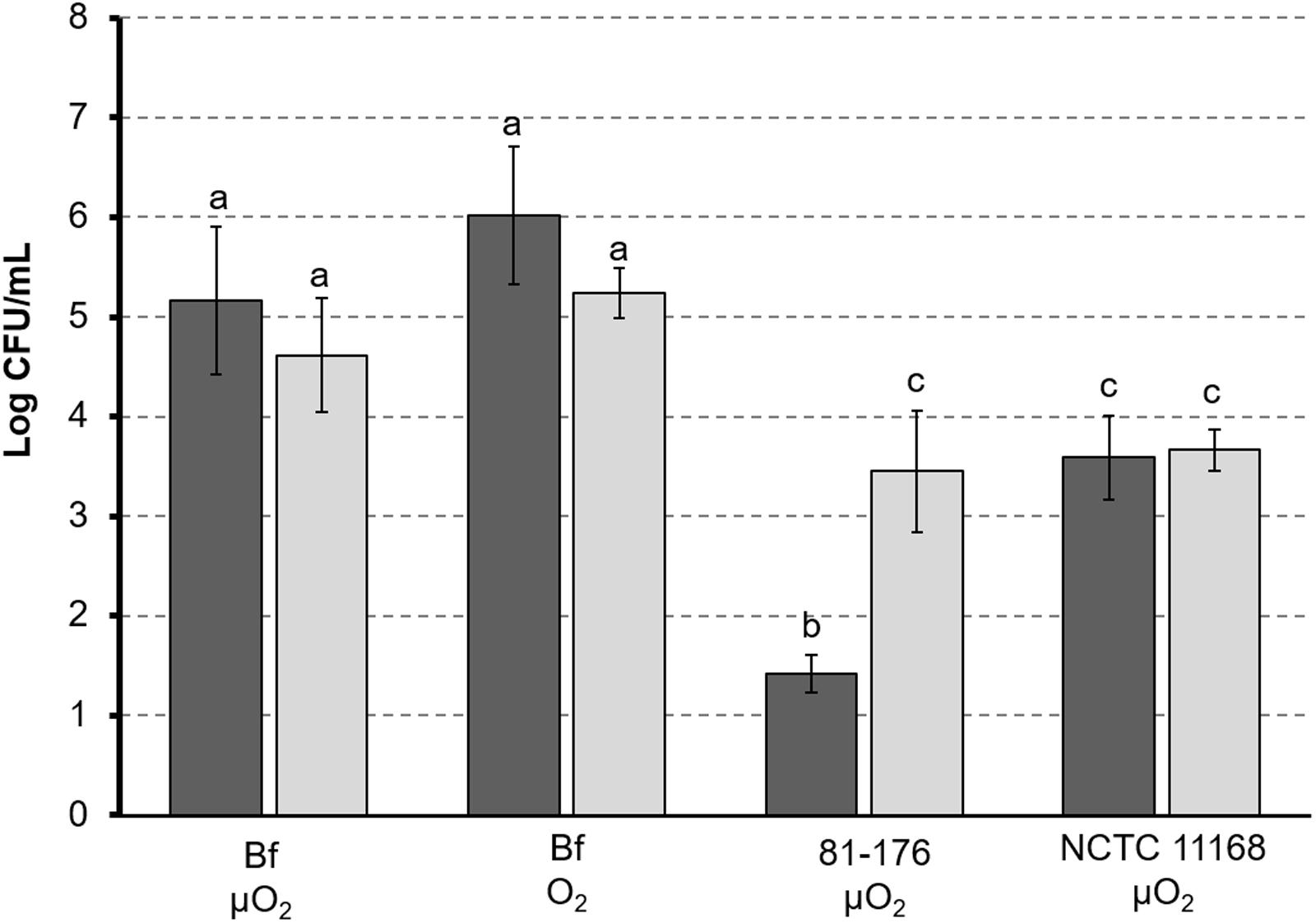
FIGURE 4. Adhesion of C. jejuni to intestinal cells. Campylobacter jejuni 81-176 and C. jejuni NCTC 11168 were grown under microaerobic conditions (μO2) and C. jejuni was cultured under microaerobic conditions (μO2) or ambient atmosphere (O2). Cells lines HT29 (black) and HT29-MTX producing mucus (gray) were used for adhesion tests. Adhesion of C. jejuni is expressed as amount of bacterial cells (expressed as CFU/mL) released from lysed eukaryotic cells after 1 h adhesion. Letters indicate values statistically similar (p-value < 0.05).
Under microaerobic conditions, C. jejuni Bf exhibited a significantly (p-value < 0.05) higher adhesion capability than the two reference strains, independently on the cell line used for experiment (Figure 4). In addition, after growth under ambient atmosphere C. jejuni Bf showed the same adhesion properties than after growth under microaerobiosis (Figure 4).
Genome Analysis
The analysis of the draft genome of C. jejuni Bf did not reveal any clear gene acquisition or deletion which could explain its ability to grow under aerobiosis (Bronnec et al., 2016). In the present study we completed the genome sequence and a deeper analysis of the gene repertoire of this strain was conducted. We first searched in the genome of C. jejuni Bf for functions that could potentially be involved in the singular phenotype of this strain: ability to grow, to adhere and to form biofilm independently from aeration conditions. A list of 165 C. jejuni genes reported in the literature as important for biofilm formation, adhesion, and oxygen metabolism was established (Supplementary Table S3) and their presence was searched in C. jejuni Bf genome. Some of these genes were putatively involved in several functions, also involved in adhesion to eukaryotic cells, or were reported to be affected by oxidative stress. Therefore, we considered them as significant for our study. Most of the literature dedicated to stress resistance and biofilm formation by C. jejuni focused on reference strains such as NCTC 11168, 81-176, and 81116. However, this species presents an important genomic diversity (Jeon et al., 2010; Zeng et al., 2013a). Therefore, we also compared the C. jejuni Bf genome sequence to 52 (complete or draft) C. jejuni genomes to search for genes that could be mutated or specific of C. jejuni Bf.
Gene Repertoire of C. jejuni Bf Related to Biofilm Formation and Adhesion
Many genes have been reported as directly or indirectly related to the biofilm development although the molecular mechanisms of their involvement are not clearly understood in C. jejuni. From various studies on C. jejuni we have selected 64 genes potentially required for strong biofilm formation and searched for their presence/absence in the genome of C. jejuni Bf. The results are presented Supplementary Table S3. Only four out of the 64 genes were missing in C. jejuni Bf. These correspond to CDS tagged as cj0628, cj0755, cj1564, and cj1725 in C. jejuni NCTC 11168. The gene cj0628 encodes CapA (Campylobacter adhesion protein A) an auto-transporter which was considered as an adhesin necessary for adhesion to Caco-2 cells and chicken colonization (Ashgar et al., 2007). The gene cj0755 encodes the ferric enterobactin receptor CfrA and is overexpressed in C. jejuni NCTC 11168 biofilm cells but its absence has already been reported in other C. jejuni strains (Kalmokoff et al., 2006; Zeng et al., 2013a,b; Sung and Khan, 2015). Tlp3, a transducer-like protein recently renamed CcmL (Rahman et al., 2014) for Campylobacter chemoreceptor for multiple ligands is encoded by cj1564. A mutation of ccmL reduce motility and enhance biofilm formation in C. jejuni 11168-O (Rahman et al., 2014). These three genes and the putative periplasmic protein cj1725; also overexpressed in C. jejuni NCTC 11168 biofilm cells (Kalmokoff et al., 2006); are absent from C. jejuni Bf as previously reported for other C. jejuni genomes (Pearson et al., 2007; Hepworth et al., 2011).
A number of Campylobacter genes have been previously described as mediating in vitro adhesion to human cells. Most of these genes were present in C. jejuni Bf genome (Supplementary Table S3). Among those, genes encoding the fibronectin binding proteins CadF (Konkel et al., 1997; Ziprin et al., 1999; Monteville et al., 2003) and FlpA (Flanagan et al., 2009; Konkel et al., 2010), the adhesins PEB 1, PEB 4 (Kervella et al., 1993; Pei et al., 1998; Del Rocio Leon-Kempis et al., 2006; Asakura et al., 2007), and JlpA (Jin et al., 2001) were recorded in C. jejuni Bf. Moreover, the membrane proteins known to be involved in adhesion step, such as the major outer membrane protein MOMP, a porin (Moser et al., 1997), and KpsE involved in the export of the capsular polysaccharide (Bachtiar et al., 2007) were found on C. jejuni Bf genome. As well, the lipooligosaccharide (LOS) biosynthesis gene cluster composed of 14 genes flanked by waaC-htrB and waaV-waaF was also present. Moreover, the genes cstII and neuBCA responsible for the sialylation of LOS (Parker et al., 2005, 2008) were observed in the genome of C. jejuni Bf. Interestingly, C. jejuni Bf possesses the 13 genes encoding an entire type VI secretion system (T6SS; Bleumink-Pluym et al., 2013) firstly described in C. jejuni by Lertpiriyapong et al. (2012), including hcp and icmF1 genes.
Although C. jejuni Bf possesses a large repertoire for adhesion and biofilm formation, some genes previously described as related to adhesion were absent from its genome. As mentioned above, the gene encoding the autotransporter protein CapA (Ashgar et al., 2007) is absent from C. jejuni Bf genome. In addition, the γ-glutamyltranspeptidase (GGT) involved in colonization of chicken is also absent from this strain. These genes are also absent in many C. jejuni isolates (Flanagan et al., 2009; Floch et al., 2014), for which the biofilm forming ability is yet unknown.
Gene Repertoire to Cope with Oxygen
Various enzymes and proteins are thought or known to protect bacteria against oxidative stress. Among them seven main enzymes/proteins and few regulators are well-documented in C. jejuni (Pesci et al., 1994; Grant and Park, 1995; Baillon et al., 1999; Ishikawa et al., 2003; Atack et al., 2008; Butcher et al., 2010; Hwang et al., 2011; Flint et al., 2014; Kim et al., 2015). These proteins involved in peroxide or superoxide detoxification include the alkyl hydroxyperoxide reductase (AhpC), the superoxide dismutase (SodB), the catalase (KatA) and Cj1386, the thiol peroxydase (Tpx), the bacterioferritin co-migratory protein (Bcp), and the bacterioferritin (Dps). The regulators Fur, PerR, and CosR have been reported to be involved in oxidative stress response. All the genes encoding enzymes or regulators involved in oxidative stress response are present in the genome of C. jejuni Bf (Supplementary Table S3).
A complete aerobic respiration pathway was detected with ccoNOQP, petABC, cydAB nuoABCDEFGHIJKLMN, and sdhBC gene clusters encoding cytochrome c oxidase, cytochrome bc and cytochrome bd complexes, NADH quinone oxidoreductase, and succinate dehydrogenase, respectively. As previously reported (Bronnec et al., 2016) the gene oorD, from the gene cluster oorDABC encoding 2-oxoglutarate oxidoreductase – a component of tricarboxylic acid (TCA) cycle – harbors a point mutation that may affect its activity. Since TCA cycle serves as electron donor for oxidative phosphorylation, we also search for genes involved in this metabolic route in C. jejuni Bf genome but did not notice any difference with other C. jejuni genomes (data not shown).
Comparative Genomics of C. jejuni Bf vs. Other Genomes
Comparing the gene repertoire of C. jejuni Bf with that of other strains, on the basis of the functions putatively involved in oxygen metabolism, biofilm formation and adhesion did not reveal any obvious missing gene in this strain. Therefore, we performed genome comparison without focusing on functions but rather to detect which strains were the closest, to narrow our analysis.
The genome similarity analysis was based on the number and percentage of identity of genes and on synteny groups. The comparison was realized using 52 genomes available (32 complete and 19 draft). We observed that C. jejuni Bf was divergent from the well-studied reference genomes (C. jejuni NCTC 11168 and C. jejuni 81-176). Among the other genomes included in our genomic comparison, C. jejuni ATCC 33560 draft genome was the closest. Interestingly, both strains belong to the same MLST group (Rodrigues et al., 2015; MLST database http://pubmlst.org/campylobacter). More than 98% of the CDS of C. jejuni Bf were in bidirectional best hits (BBHs) with the CDS of C. jejuni ATCC 33560 draft genome (34 contigs). Such a similarity between the two strains prompted us to compare their phenotype. C. jejuni ATCC 33560 was not aerotolerant (data not shown). Consequently, we focused on the differences between the genome sequences of these two strains. Thirty eight CDS were unique to the two strains compared to the 51 others strains, most of them considered as encoding peptides of unknown function (Supplementary Table S4). Among those we noticed a small CDS inserted in the cluster hcrA/grpE/dnaK, directly upstream of dnaK. This gene, of unknown function, encodes a protein of 72 amino acids that may potentially affect the expression of dnaK. Among the 37 remaining unique CDS, many were of small size and could be considered as false or doubtful CDS or resulting from fragmented genes. None could be associated to functions related to oxygen metabolism.
Comparison of Gene Transcription in C. jejuni Bf under Different Atmospheres
The phenotype of C. jejuni Bf regarding growth, adhesion to biotic and abiotic surfaces and biofilm formation suggested that this strain behaves similarly under air or under atmosphere conditions described as optimal (low O2 concentration and high CO2 concentration). Since only few genome features specific to this strain were observed, we hypothesized that a subtle change in gene expression may be involved. According to the literature, CosR is involved in oxidative stress response but also in biofilm maturation in C. jejuni (Hwang et al., 2011, 2012, 2014; Oh and Jeon, 2014; Turonova et al., 2015). The expression of cosR from cells grown under microaerobic or aerobic condition was measured. As well we determined the expression of several genes that were pointed out during genome analysis: oorDABC genes, dnaK and its upstream CDS. C. jejuni 81-176 grown was used as a control. Under microaerobiosis, cosR and oorDABC gene expression levels in C. jejuni Bf were not statistically different from those of C. jejuni 81-176 whereas we noticed an 8-fold increase of dnaK expression in C. jejuni Bf.
After aerobic growth of C. jejuni Bf, the relative expression of cosR and oorDABC were strongly increased in comparison with C. jejuni Bf grown in microaerobiosis. Indeed, cosR expression level was 12-times higher in aerobiosis. As well, oorD, oorA, oorB, and oorC were expressed 22, 19, 18, and 12 times more, respectively. The expression of dnaK and its upstream CDS were constitutive in C. jejuni Bf whatever the conditions tested.
We searched for the presence of the CosR box previously reported in C. jejuni NCTC 11168 by Hwang et al. (2011, 2012) upstream from these genes. We observed a motif similar to the CosR box upstream from oorD with only 14 out of the 21 bp consensus sequence conserved. Interestingly, a similar box was also present upstream from dnaK due to the insertion of a small CDS. Although, the motif was moderately conserved (14 out of 21 bp) we cannot exclude that such an insertion in C. jejuni Bf may modify dnaK expression or regulation by comparison to C. jejuni 81-176.
Discussion
During the last decade, C. jejuni has been regularly reported as the leading cause of bacterial foodborne infection in Europe. Given the public health significance of this zoonosis it is relevant to understand the survival mechanisms adopted by this pathogen. Indeed, passage through the food chain exposes this microaerophilic pathogen to various harsh environmental conditions including oxidative stress. Among the strategies to resist, biofilm is a life-style known to protect bacteria from various environmental stresses, antimicrobial agents and also increased bacterial resistance to host immune response (Gilbert et al., 1993; Donlan and Costerton, 2002; Chmielewski and Frank, 2003). Recently described, C. jejuni Bf presents a higher ability to survive against oxidative stress and this clinical strain also presents the particularity to grow under aerobic conditions (Rodrigues et al., 2015). In this report, we studied the ability of this strain to adhere and develop biofilms. We also evaluated the influence of aerobiosis on adhesion properties. Finally, we searched for genomic features that may explain the atypical phenotype of the strain.
Biofilm formation is a succession of several steps beginning with initial attachment. Therefore, we have investigated the capacity of C. jejuni to adhere to an inert surface in order to evaluate subsequently its ability to initiate and develop a biofilm. The adhesion capability was variable between the 13 strains we tested. C. jejuni Bf showed a delayed adhesion, suggesting that a longer contact period with the polystyrene may lead to a stronger adhesion. Surprisingly, C. jejuni 81-176 strain showed a low adhesion capacity, even after several verification tests, although, this strain was previously reported to adhere and develop biofilm (Gunther and Chen, 2009; Sulaeman et al., 2010; Turonova et al., 2015). The main differences between the current study and previous ones rely on the experimental design, especially the media used for growth. These have been already reported to influence C. jejuni adhesion to inert surface (Reeser et al., 2007).
We have also investigated the capacity of C. jejuni to adhere and form biofilm under aerobiosis. Interestingly, cultivation of C. jejuni Bf under aerobiosis enhanced its adhesion to polystyrene. Few studies have been conducted to evaluate the ability of C. jejuni to form biofilm aerobically (Asakura et al., 2007; Reuter et al., 2010; Turonova et al., 2015). As raised by Turonova et al. (2015, 2016) the use of CLSM allows observation of structural changes in the biofilm formed by C. jejuni. Subsequently to our adhesion assay, the capacity of C. jejuni Bf and C. jejuni 81-176 to produce biofilm under aerobiosis were also evaluated and observed using CLSM. The ultrastructure of the biofilm formed by C. jejuni 81-176 being well-characterized (Gunther and Chen, 2009; Turonova et al., 2015), we chose this stain as a reference. In optimal growth conditions (e.g., under microaerobiosis and at 42°C), C. jejuni Bf is also able to develop a structured biofilm as previously described for several C. jejuni strains (Asakura et al., 2007; Gunther and Chen, 2009; Reuter et al., 2010; Turonova et al., 2015). Comparison of bio-volume and thickness of the biofilm formed by the two strains cultivated in microaerobiosis revealed structural differences. Indeed, the biofilm developed by C. jejuni 81-176 appeared thick with heterogeneous structures, whereas the one formed by C. jejuni Bf was more homogeneous, flatter and spread in the well. Statistical analysis confirmed that C. jejuni 81-176 developed a biofilm 1.7 fold higher than C. jejuni Bf but with a non-significant difference in volume level. The microaerophilic strain C. jejuni 81-176 was unable to develop a biofilm in ambient atmosphere at 42°C even after 48 h of incubation. This apparent contradiction with other studies reporting that aerobiosis enhances biofilm formation may rely on differences in experimental conditions and on the strain that were used. Indeed most studies focused on C. jejuni NCTC 11168. These were performed under different growth conditions with the use of Brucella (Reuter et al., 2010) or Muller-Hinton broths (Asakura et al., 2007) and an incubation temperature of 37°C. The study including C. jejuni 81-176 was performed to compare only the influence of oxygen using O2 and CO2-enriched conditions, e.g., 19% O2, 10% CO2, 71% N2 (Turonova et al., 2015) which are different from the gaseous conditions we used (ambiant air). In addition, incubation temperature was 37°C and adhesion duration was longer (4–5 h; Turonova et al., 2015) vs. 42°C and 2 h in the present study.
Campylobacter jejuni Bf is able to develop biofilms under both microaerobiosis and aerobiosis, with no significant modification in terms of bio-volume and thickness. We can hypothesize that under aerobiosis C. jejuni Bf develops a more structured biofilm resulting in a microaerobic local environment more adequate for its growth, as was proposed for NCTC 11168 (Stewart and Franklin, 2008; Reuter et al., 2010; Turonova et al., 2015). Nevertheless, this study is the first report on the capacity of a C. jejuni strain to form biofilm after growth under aerobiosis.
Adhesion to surface is clearly a preliminary step to biofilm formation and some proteins involved in adhesion to inert surfaces are also important for interaction with epithelial cells. Compared to C. jejuni 81-176 and NCTC 11168, C. jejuni Bf presents a higher ability to adhere to human intestinal cells after growth in either microaerobiosis or aerobiosis. Mucus production did not modify adhesion capability of C. jejuni Bf and NCTC 11168, but enhanced that of C. jejuni 81-176. The better ability of the clinical strain C. jejuni Bf to adhere to human intestinal cells might be explained by the presence of a complete T6SS as reported in few other strains (Lertpiriyapong et al., 2012; Harrison et al., 2014; Corcionivoschi et al., 2015). This structure is absent from C. jejuni NCTC 11168 and 81-176.
Once the phenotype characterization performed, we focused on comparative genomics to point out genes specific of C. jejuni Bf. The genome analysis revealed that this strain possesses the genes necessary to develop a biofilm. Among all of the genes identified in the literature related to biofilm formation only four were absent, which is not particularly relevant since these genes are also absent from several C. jejuni genomes (Hofreuter et al., 2006; Rahman et al., 2014). In addition, we cannot totally exclude that their absence could result from sequencing errors or sequence misassembly. The gene repertoire of C. jejuni Bf necessary to resist to oxidative stress revealed no difference with that of other strains. In C. jejuni the CosR regulator has been reported as responsible for the regulation of genes participating to oxidative stress response but also to biofilm formation (Kalmokoff et al., 2006; Svensson et al., 2009; Garénaux et al., 2008a; Hwang et al., 2011, 2012; Oh and Jeon, 2014; Turonova et al., 2015). We have shown that C. jejuni Bf cosR was 12-fold over-expressed in aerobiosis, suggesting that the regulation of genes involved in oxidative stress response and biofilm formation might be modified in this strain. We highlighted two genetic modifications in C. jejuni Bf that may rely on its behavior: a point mutation in oorD (Bronnec et al., 2016) and an insertion upstream from dnaK. The oorD mutation may result in a different phenotype toward oxygen metabolism since in Helicobacter pylori, the 2-oxoglutarate oxidoreductase encoded by oorDABC, was reported as important for the microaerophilic phenotype of this species (Hughes et al., 1998). In addition, we showed that C. jejuni Bf oorDABC operon is up-regulated under aerobiosis. Conversely, dnaK transcription was constitutive in C. jejuni Bf regarding atmosphere used for growth. However, this gene is up-regulated in C. jejuni Bf, in comparison with 81-176. This may be the consequence of the insertion just upstream from dnaK which may result in a modification of its transcription. Furthermore, DnaK belongs to a protein family involved in general stress response. Its high level of expression in C. jejuni Bf might explain a better resistance to oxidative stress of this stain compared to that of C. jejuni 81-176. Comparing the resistance of the two strains to other stresses would be necessary to confirm this hypothesis. In addition, DnaK has also been described as moonlighting in several bacteria, i.e., harboring a different function when expressed on the cell surface (Amblee and Jeffery, 2015 and references therein). Indeed, DnaK from several Gram+ and Gram- species has been shown to bind plasminogen or eukaryotic cell surfaces when present on bacterial surface. We have no evidence of such a location in C. jejuni Bf, but this should be considered to search for a potential role of this protein, which gene is highly express in a clinical strain capable of adhering to surfaces and developing biofilm.
Conclusion
The ability of C. jejuni to develop a structured biofilm is highly variable depending on the surface, the environmental conditions but also the strain. C. jejuni Bf has the particularity to multiply under aerobiosis, but we also have shown that this strain is able to form a structured biofilm when cultured in aerobic condition. Further experiments could be conducted at environmental temperatures (vs. optimal one, 42°C) to investigate C. jejuni Bf ability to form biofilm under aerobiosis. Genome analysis did not highlight any obvious acquisition of functions in this strain. Its atypical behavior apparently results from a modification in the regulation of several genes involved in oxidative stress response, oxygen metabolism, adhesion, and biofilm formation.
Author Contributions
Conceived and designed the experiments: VB, NH, OT and MZ; performed the experiments: VB, HT, RR, AB and SC; analyzed the data: VB, MZ, NH, OT and SC; wrote the paper: VB, NH and MZ; corrected the paper: VB, NH, HT, OT, SC and MZ.
Funding
VB was supported by a Ph.D. grant from the Institut National de la Recherche Agronomique (INRA, MICA division) and from the Région des Pays de la Loire. The study was financially supported by Secalim (INRA/Oniris, Nantes, France), and the Cost BacFoodNet FA1202 through a short term scientific mission, PHC Barrande 2015 (PROJET N° 34004QB), and the Pays de la Loire regional project GRIOTTE.
Conflict of Interest Statement
The authors declare that the research was conducted in the absence of any commercial or financial relationships that could be construed as a potential conflict of interest.
Acknowledgments
We are grateful to Petra Grznarova (Department of Biochemistry and Microbiology, University of Chemistry and Technology, Prague, Czech Republic) for material support and for her assistance with the CLSM.
The LABGeM (CEA/IG/Genoscope & CNRS UMR8030) and the France Génomique National infrastructure (funded as part of Investissement d’avenir program managed by Agence Nationale pour la Recherche, contract ANR-10-INBS-09) are acknowledged for support within the MicroScope annotation platform.
Campylobacter jejuni strains were kindly provided by Clifford Clark (National Laboratory for Enteric Pathogens, Winnipeg, MB, Canada), Susanne Knøchel (University of Copenhagen), and Muriel Guyard (French Agency for Food, Environmental and Occupational Health & Safety, Ploufragan, France).
We thank BioFilm Control SAS (Saint-Beauzire, France), particularly, Thierry Bernardi for kindly provided facilities for the BioFilm Ring Test and Arnaud Clement for his technical assistance.
We thank Dr. Thécla Lesuffleur (INSERM UMR S938, Paris France) for providing HT29-MTX cells.
We thank Philippe Courcoux (Applied Statistics Laboratory, Oniris, Nantes, France) for his assistance and his advices for the statistical analysis of data.
Supplementary Material
The Supplementary Material for this article can be found online at: http://journal.frontiersin.org/article/10.3389/fmicb.2016.01002
References
Amblee, V., and Jeffery, C. J. (2015). Physical features of intracellular proteins that moonlight on the cell surface. PLoS ONE 10:e0130575. doi: 10.1371/journal.pone.0130575
Asakura, H., Yamasaki, M., Yamamoto, S., and Igimi, S. (2007). Deletion of peb4 gene impairs cell adhesion and biofilm formation in Campylobacter jejuni. FEMS Microbiol. Lett. 275, 278–285. doi: 10.1111/j.1574-6968.2007.00893.x
Ashgar, S. S., Oldfield, N. J., Wooldridge, K. G., Jones, M. A., Irving, G. J., Turner, D. P., et al. (2007). CapA, an autotransporter protein of Campylobacter jejuni, mediates association with human epithelial cells and colonization of the chicken gut. J. Bacteriol. 189, 1856–1865. doi: 10.1128/JB.01427-06
Atack, J. M., Harvey, P., Jones, M. A., and Kelly, D. J. (2008). The Campylobacter jejuni thiol peroxidases Tpx and Bcp both contribute to aerotolerance and peroxide-mediated stress resistance but have distinct substrate specificities. J. Bacteriol. 190, 5279–5290. doi: 10.1128/JB.00100-08
Bachtiar, B. M., Coloe, P. J., and Fry, B. N. (2007). Knockout mutagenesis of the kpsE gene of Campylobacter jejuni 81116 and its involvement in bacterium-host interactions. FEMS Immunol. Med. Microbiol. 49, 149–154. doi: 10.1111/j.1574-695X.2006.00182.x
Baillon, M.-L. A., van Vliet, A. H., Ketley, J. M., Constantinidou, C., and Penn, C. W. (1999). An iron-regulated alkyl hydroperoxide reductase (AhpC) confers aerotolerance and oxidative stress resistance to the microaerophilic pathogen Campylobacter jejuni. J. Bacteriol. 181, 4798–4804.
Batz, M., Hoffmann, S., and Morris, J. G. Jr. (2014). Disease-outcome trees, EQ-5D scores, and estimated annual losses of quality-adjusted life years (QALYs) for 14 foodborne pathogens in the United States. Foodborne Pathog. Dis. 11, 395–402. doi: 10.1089/fpd.2013.1658
Bleumink-Pluym, N. M., van Alphen, L. B., Bouwman, L. I., Wosten, M. M., and van Putten, J. P. (2013). Identification of a functional type VI secretion system in Campylobacter jejuni conferring capsule polysaccharide sensitive cytotoxicity. PLoS Pathog. 9:e1003393. doi: 10.1371/journal.ppat.1003393
Bronnec, V., Haddad, N., Cruveiller, S., Hernould, M., Tresse, O., and Zagorec, M. (2016). Draft genome sequence of Campylobacter jejuni Bf, an atypical strain able to grow under aerobiosis Genome Announc. 4:e00120-16. doi: 10.1128/genomeA.00120-16
Buswell, C. M., Herlihy, Y. M., Lawrence, L. M., McGuiggan, J. T., Marsh, P. D., Keevil, C. W., et al. (1998). Extended survival and persistence of Campylobacter spp. in water and aquatic biofilms and their detection by immunofluorescent-antibody and -rRNA staining. Appl. Environ. Microbiol. 64, 733–741.
Butcher, J., Flint, A., Stahl, M., and Stintzi, A. (2010). “Campylobacter Fur and PerR regulons,” in Iron uptake and homeostasis in microorganisms, eds P. Cornelis and S. C. Andrews (Haverhill: Caister Academic Press), 168–202.
Chmielewski, R., and Frank, J. (2003). Biofilm formation and control in food processing facilities. Compr. Rev. Food Sci. Food Saf. 2, 22–32. doi: 10.1111/j.1541-4337.2003.tb00012.x
Chynoweth, R. W., Hudson, J. A., and Thom, K. (1998). Aerobic growth and survival of Campylobacter jejuni in food and stream water. Lett. Appl. Microbiol. 27, 341–344. doi: 10.1046/j.1472-765X.1998.00453.x
Corcionivoschi, N., Gundogdu, O., Moran, L., Kelly, C., Scates, P., Stef, L., et al. (2015). Virulence characteristics of hcp+ Campylobacter jejuni and Campylobacter coli isolates from retail chicken. Gut Pathog. 7:1. doi: 10.1186/s13099-015-0067-z
Costerton, J. (1995). Overview of microbial biofilms. J. Ind. Microbiol. 15, 137–140. doi: 10.1007/BF01569816
Costerton, J. W., Lewandowski, Z., Caldwell, D. E., Korber, D. R., and Lappin-Scott, H. M. (1995). Microbial biofilms. Ann. Rev. Microbiol. 49, 711–745. doi: 10.1146/annurev.mi.49.100195.003431
Del Rocio Leon-Kempis, M., Guccione, E., Mulholland, F., Williamson, M. P., and Kelly, D. J. (2006). The Campylobacter jejuni PEB1a adhesin is an aspartate/glutamate-binding protein of an ABC transporter essential for microaerobic growth on dicarboxylic amino acids. Mol. Microbiol. 60, 1262–1275. doi: 10.1111/j.1365-2958.2006.05168.x
Donlan, R. M. (2002). Biofilms: microbial life on surfaces. Emerg. Infect. Dis. 8, 881–890. doi: 10.3201/eid0809.020063
Donlan, R. M., and Costerton, J. W. (2002). Biofilms: survival mechanisms of clinically relevant microorganisms. Clin. Microbiol. Rev. 15, 167–193. doi: 10.1128/CMR.15.2.167-193.2002
Eberle, K. N., and Kiess, A. S. (2012). Phenotypic and genotypic methods for typing Campylobacter jejuni and Campylobacter coli in poultry. Poult. Sci. 91, 255–264. doi: 10.3382/ps.2011-01414
EFSA and ECDC (2016). The European Union summary report on trends and sources of zoonoses, zoonotic agents and food-borne outbreaks in 2014. EFSA J. 2015. 13:4329. doi: 10.2903/j.efsa.2015.4329
Floch, P., Pey, V., Castroviejo, M., Dupuy, J. W., Bonneu, M., de La Guardia, A. H., et al. (2014). Role of Campylobacter jejuni gamma-glutamyl transpeptidase on epithelial cell apoptosis and lymphocyte proliferation. Gut Pathog. 6:1. doi: 10.1186/1757-4749-6-20
Flanagan, R. C., Neal-McKinney, J. M., Dhillon, A. S., Miller, W. G., and Konkel, M. E. (2009). Examination of Campylobacter jejuni putative adhesins leads to the identification of a new protein, designated FlpA, required for chicken colonization. Infect. Immun. 77, 2399–2407. doi: 10.1128/IAI.01266-08
Flint, A., Sun, Y.-Q., Butcher, J., Stahl, M., Huang, H., and Stintzi, A. (2014). Phenotypic screening of a targeted mutant library reveals Campylobacter jejuni defenses against oxidative stress. Infect. Immun. 82, 2266–2275. doi: 10.1128/IAI.01528-13
Fouts, D. E., Mongodin, E. F., Mandrell, R. E., Miller, W. G., Rasko, D. A., Ravel, J., et al. (2005). Major structural differences and novel potential virulence mechanisms from the genomes of multiple Campylobacter species. PLoS Biol. 3:e15. doi: 10.1371/journal.pbio.0030015
Garénaux, A., Guillou, S., Ermel, G., Wren, B., Federighi, M., and Ritz, M. (2008a). Role of the Cj1371 periplasmic protein and the Cj0355c two-component regulator in the Campylobacter jejuni NCTC 11168 response to oxidative stress caused by paraquat. Res Microbiol., 159, 718–726. doi: 10.1016/j.resmic.2008.08.001
Garénaux, A., Jugiau, F., Rama, F., de Jonge, R., Denis, M., Federighi, M., et al. (2008b). Survival of Campylobacter jejuni strains from different origins under oxidative stress conditions: effect of temperature. Curr. Microbiol. 56, 293–297. doi: 10.1007/s00284-007-9082-8
Gilbert, P., Evans, D. J., and Brown, M. R. (1993). Formation and dispersal of bacterial biofilms in vivo and in situ. J. Appl. Bacteriol. 74(Suppl.), 67S–78S. doi: 10.1111/j.1365-2672.1993.tb04343.x
Grant, K. A., and Park, S. F. (1995). Molecular characterization of katA from Campylobacter jejuni and generation of a catalase-deficient mutant of Campylobacter coli by interspecific allelic exchange. Microbiology 141, 1369–1376. doi: 10.1099/13500872-141-6-1369
Gundogdu, O., Bentley, S. D., Holden, M. T., Parkhill, J., Dorrell, N., and Wren, B. W. (2007). Re-annotation and re-analysis of the Campylobacter jejuni NCTC11168 genome sequence. BMC Genomics 8:162. doi: 10.1186/1471-2164-8-162
Gunther, N. W., and Chen, C. Y. (2009). The biofilm forming potential of bacterial species in the genus Campylobacter. Food Microbiol. 26, 44–51. doi: 10.1016/j.fm.2008.07.012
Haddad, N., Maillart, G., Garénaux, A., Jugiau, F., Federighi, M., and Cappelier, J. M. (2010). Adhesion ability of Campylobacter jejuni to Ht-29 cells increases with the augmentation of oxidant agent concentration. Curr. Microbiol. 61, 500–505. doi: 10.1007/s00284-010-9644-z
Haddad, N., Tresse, O., Rivoal, K., Chevret, D., Nonglaton, Q., Burns, C. M., et al. (2012). Polynucleotide phosphorylase has an impact on cell biology of Campylobacter jejuni. Front. Cell. Infect. Microbiol. 2:30. doi: 10.3389/fcimb.2012.00030
Harrison, J. W., Dung, T. T. N., Siddiqui, F., Korbrisate, S., Bukhari, H., Tra, M. P. V., et al. (2014). Identification of possible virulence marker from Campylobacter jejuni isolates. Emerg. Infect. Dis. 20:1026. doi: 10.3201/eid2006.130635
Hepworth, P. J., Ashelford, K. E., Hinds, J., Gould, K. A., Witney, A. A., Williams, N. J., et al. (2011). Genomic variations define divergence of water/wildlife-associated Campylobacter jejuni niche specialists from common clonal complexes. Environ. Microbiol. 13, 1549–1560. doi: 10.1111/j.1462-2920.2011.02461.x
Hinton, A. Jr. (2016). Growth of Campylobacter incubated aerobically in fumarate-pyruvate media or media supplemented with dairy, meat, or soy extracts and peptones. Food Microbiol. 58, 23–28. doi: 10.1016/j.fm.2016.03.010
Hofreuter, D., Tsai, J., Watson, R. O., Novik, V., Altman, B., Benitez, M., et al. (2006). Unique features of a highly pathogenic Campylobacter jejuni strain. Infect. Immun. 74, 4694–4707. doi: 10.1128/IAI.00210-06
Hughes, N. J., Clayton, C. L., Chalk, P. A., and Kelly, D. J. (1998). Helicobacter pylori porCDAB and oorDABC genes encode distinct pyruvate: flavodoxin and 2-Oxoglutarate: acceptor oxidoreductases which mediate electron transport to NADP. J. Bacteriol. 180, 1119–1128.
Hwang, S., Kim, M., Ryu, S., and Jeon, B. (2011). Regulation of oxidative stress response by CosR, an essential response regulator in Campylobacter jejuni. PLoS ONE 6:e22300. doi: 10.1371/journal.pone.0022300
Hwang, S., Miller, W. G., Ryu, S., and Jeon, B. (2014). Divergent distribution of the sensor kinase CosS in non-thermotolerant Campylobacter species and its functional incompatibility with the response regulator CosR of Campylobacter jejuni. PLoS ONE 9:e89774. doi: 10.1371/journal.pone.0089774
Hwang, S., Zhang, Q., Ryu, S., and Jeon, B. (2012). Transcriptional regulation of the CmeABC multidrug efflux pump and the KatA catalase by CosR in Campylobacter jejuni. J. Bacteriol 194, 6883–6891. doi: 10.1128/JB.01636-12
Hyytiäinen, H., Juntunen, P., Akrenius, N., and Hänninen, M.-L. (2012). Importance of RNA stabilization: evaluation of ansB, ggt, and rpoA transcripts in microaerophilic Campylobacter jejuni 81176. Arch. Microbiol. 194, 803–808. doi: 10.1007/s00203-012-0820-3
Ishikawa, T., Mizunoe, Y., Kawabata, S.-I., Takade, A., Harada, M., Wai, S. N., et al. (2003). The iron-binding protein Dps confers hydrogen peroxide stress resistance to Campylobacter jejuni. J. Bacteriol. 185, 1010–1017. doi: 10.1128/JB.185.3.1010-1017.2003
Jeon, B., Muraoka, W. T., and Zhang, Q. (2010). Advances in Campylobacter biology and implications for biotechnological applications. Microb. Biotechnol. 3, 242–258. doi: 10.1111/j.1751-7915.2009.00118.x
Jin, S., Joe, A., Lynett, J., Hani, E. K., Sherman, P., and Chan, V. L. (2001). JlpA, a novel surface-exposed lipoprotein specific to Campylobacter jejuni, mediates adherence to host epithelial cells. Mol. Microbiol. 39, 1225–1236. doi: 10.1111/j.1365-2958.2001.02294.x
Joshua, G. P., Guthrie-Irons, C., Karlyshev, A., and Wren, B. (2006). Biofilm formation in Campylobacter jejuni. Microbiology 152, 387–396. doi: 10.1099/mic.0.28358-0
Kalmokoff, M., Lanthier, P., Tremblay, T. L., Foss, M., Lau, P. C., Sanders, G., et al. (2006). Proteomic analysis of Campylobacter jejuni 11168 biofilms reveals a role for the motility complex in biofilm formation. J. Bacteriol. 188, 4312–4320. doi: 10.1128/JB.01975-05
Kervella, M., Pagès, J.-M., Pei, Z., Grollier, G., Blaser, M. J., and Fauchere, J. (1993). Isolation and characterization of two Campylobacter glycine-extracted proteins that bind to HeLa cell membranes. Infect. Immun. 61, 3440–3448.
Kim, J. -C., Oh, E., Hwang, S., Ryu, S., and Jeon, B. (2015). Non-selective regulation of peroxide and superoxide resistance genes by PerR in Campylobacter jejuni. Front. Microbiol. 6:126. doi: 10.3389/fmicb.2015.00126
Konkel, M. E., Garvis, S. G., Tipton, S. L., Anderson, D. E. Jr., and Cieplak, W. Jr. (1997). Identification and molecular cloning of a gene encoding a fibronectin-binding protein (CadF) from Campylobacter jejuni. Mol. Microbiol. 24, 953–963. doi: 10.1046/j.1365-2958.1997.4031771.x
Konkel, M. E., Larson, C. L., and Flanagan, R. C. (2010). Campylobacter jejuni FlpA binds fibronectin and is required for maximal host cell adherence. J. Bacteriol. 192, 68–76. doi: 10.1128/JB.00969-09
Lertpiriyapong, K., Gamazon, E. R., Feng, Y., Park, D. S., Pang, J., Botka, G., et al. (2012). Campylobacter jejuni type VI secretion system: roles in adaptation to deoxycholic acid, host cell adherence, invasion, and in vivo colonization. PLoS ONE 7:e42842. doi: 10.1371/journal.pone.0042842
Livak, K. J., and Schmittgen, T. D. (2001). Analysis of relative gene expression data using real-time quantitative PCR and the 2- ΔΔCT method. Methods 25, 402–408. doi: 10.1006/meth.2001.1262
Monteville, M. R., Yoon, J. E., and Konkel, M. E. (2003). Maximal adherence and invasion of INT 407 cells by Campylobacter jejuni requires the CadF outer-membrane protein and microfilament reorganization. Microbiology 149, 153–165. doi: 10.1099/mic.0.25820-0
Moser, I., Schroeder, W., and Salnikow, J. (1997). Campylobacter jejuni major outer membrane protein and a 59-kDa protein are involved in binding to fibronectin and INT 407 cell membranes. FEMS Microbiol. Lett. 157, 233–238. doi: 10.1111/j.1574-6968.1997.tb12778.x
Nguyen, V. T., Fegan, N., Turner, M. S., and Dykes, G. A. (2012). Role of attachment to surfaces on the prevalence and survival of Campylobacter through food systems. J. Food Prot. 75, 195–206. doi: 10.4315/0362-028X.JFP-11-012
Oh, E., and Jeon, B. (2014). Role of alkyl hydroperoxide reductase (AhpC) in the biofilm formation of Campylobacter jejuni. PLoS ONE 9:e87312. doi: 10.1371/journal.pone.0087312
Park, S. F. (2002). The physiology of Campylobacter species and its relevance to their role as foodborne pathogens. Int. J. Food. Microbiol. 74, 177–188. doi: 10.1016/S0168-1605(01)00678-X
Parker, C. T., Gilbert, M., Yuki, N., Endtz, H. P., and Mandrell, R. E. (2008). Characterization of lipooligosaccharide-biosynthetic loci of Campylobacter jejuni reveals new lipooligosaccharide classes: evidence of mosaic organizations. J. Bacteriol. 190, 5681–5689. doi: 10.1128/JB.00254-08
Parker, C. T., Horn, S. T., Gilbert, M., Miller, W. G., Woodward, D. L., and Mandrell, R. E. (2005). Comparison of Campylobacter jejuni lipooligosaccharide biosynthesis loci from a variety of sources. J. Clin. Microbiol. 43, 2771–2781. doi: 10.1128/JCM.43.6.2771-2781.2005
Parkhill, J., Wren, B. W., Mungall, K., Ketley, J. M., Churcher, C., Basham, D., et al. (2000). The genome sequence of the food-borne pathogen Campylobacter jejuni reveals hypervariable sequences. Nature 403, 665–668. doi: 10.1038/35001088
Pearson, B. M., Gaskin, D. J., Segers, R. P., Wells, J. M., Nuijten, P. J., and van Vliet, A. H. (2007). The complete genome sequence of Campylobacter jejuni strain 81116 (NCTC11828). J. Bacteriol. 189, 8402–8403. doi: 10.1128/JB.01404-07
Pei, Z., Burucoa, C., Grignon, B., Baqar, S., Huang, X.-Z., Kopecko, D. J., et al. (1998). Mutation in the peb1A locus of Campylobacter jejuni reduces interactions with epithelial cells and intestinal colonization of mice. Infect. Immun. 66, 938–943.
Pesci, E. C., Cottle, D. L., and Pickett, C. L. (1994). Genetic, enzymatic, and pathogenic studies of the iron superoxide dismutase of Campylobacter jejuni. Infect. Immun. 62, 2687–2694.
Rahman, H., King, R. M., Shewell, L. K., Semchenko, E. A., Hartley-Tassell, L. E., Wilson, J. C., et al. (2014). Characterisation of a multi-ligand binding chemoreceptor CcmL (Tlp3) of Campylobacter jejuni. PLoS Pathog. 10:e1003822. doi: 10.1371/journal.ppat.1003822
Reeser, R. J., Medler, R. T., Billington, S. J., Jost, B. H., and Joens, L. A. (2007). Characterization of Campylobacter jejuni biofilms under defined growth conditions. Appl. Environ. Microbiol. 73, 1908–1913. doi: 10.1128/AEM.00740-06
Reuter, M., Mallett, A., Pearson, B. M., and van Vliet, A. H. (2010). Biofilm formation by Campylobacter jejuni is increased under aerobic conditions. Appl. Environ. Microbiol. 76, 2122–2128. doi: 10.1128/AEM.01878-09
Rodrigues, R. C., Pocheron, A.-L., Hernould, M., Haddad, N., Tresse, O., and Cappelier, J.-M. (2015). Description of Campylobacter jejuni Bf, an atypical aero-tolerant strain. Gut Pathog. 7:1. doi: 10.1186/s13099-015-0077-x
Stewart, P. S., and Franklin, M. J. (2008). Physiological heterogeneity in biofilms. Nat. Rev. Microbiol. 6, 199–210. doi: 10.1038/nrmicro1838
Sulaeman, S., Le Bihan, G., Rossero, A., Federighi, M., De, E., and Tresse, O. (2010). Comparison between the biofilm initiation of Campylobacter jejuni and Campylobacter coli strains to an inert surface using BioFilm Ring Test. J. Appl. Microbiol. 108, 1303–1312. doi: 10.1111/j.1365-2672.2009.04534.x
Sung, K., and Khan, S. (2015). “Biofilm development by Campylobacter jejuni,” in Biofilms in the Food Environment, 2nd Edn, eds A. L. Pometto and A. Demirci, 29–50. doi: 10.1002/9781118864036.ch2
Svensson, S. L., Davis, L. M., MacKichan, J. K., Allan, B. J., Pajaniappan, M., Thompson, S. A., et al. (2009). The CprS sensor kinase of the zoonotic pathogen Campylobacter jejuni influences biofilm formation and is required for optimal chick colonization. Mol. Microbiol. 71, 253–272. doi: 10.1111/j.1365-2958.2008.06534.x
Takamiya, M., Ozen, A., Rasmussen, M., Alter, T., Gilbert, T., Ussery, D. W., et al. (2011). Genome Sequence of Campylobacter jejuni strain 327, a strain isolated from a turkey slaughterhouse. Stand. Genomic Sci. 4, 113–122. doi: 10.4056/sigs.1313504
Turonova, H., Briandet, R., Rodrigues, R., Hernould, M., Hayek, N., Stintzi, A., et al. (2015). Biofilm spatial organization by the emerging pathogen Campylobacter jejuni: comparison between NCTC 11168 and 81-176 strains under microaerobic and oxygen-enriched conditions. Front. Microbiol. 6:709. doi: 10.3389/fmicb.2015.00709
Turonova, H., Neu, T., Ulbrich, P., Pazlarova, J. and Tresse, O. (2016). The biofilm matrix of Campylobacter jejuni determined by fluorescence lectin-binding analysis. Biofouling 32, 597–608. doi: 10.1080/08927014.2016.1169402
Vallenet, D., Belda, E., Calteau, A., Cruveiller, S., Engelen, S., Lajus, A., et al. (2013). MicroScope - an integrated microbial resource for the curation and comparative analysis of genomic and metabolic data. Nucleic Acids Res. 41, D636–D647. doi: 10.1093/nar/gks1194
Vallenet, D., Labarre, L., Rouy, Z., Barbe, V., Bocs, S., Cruveiller, S., et al. (2006). MaGe: a microbial genome annotation system supported by synteny results. Nucleic Acids Res. 34, 53–65. doi: 10.1093/nar/gkj406
WHO (2013). The Global View of Campylobacteriosis: Report of an Expert Consultation. Geneva: WHO Document Production Services, 57.
Zeng, X., Mo, Y., Xu, F., and Lin, J. (2013a). Identification and characterization of a periplasmic trilactone esterase, Cee, revealed unique features of ferric enterobactin acquisition in Campylobacter. Mol. Microbiol. 87, 594–608. doi: 10.1111/mmi.12118
Zeng, X., Xu, F., and Lin, J. (2013b). Specific TonB-ExbB-ExbD energy transduction systems required for ferric enterobactin acquisition in Campylobacter. FEMS Microbiol. Lett. 347, 83–91. doi: 10.1111/1574-6968.12221
Keywords: food borne pathogen, biofilm, confocal microscopy, oxidative stress, genome sequence
Citation: Bronnec V, Turoňová H, Bouju A, Cruveiller S, Rodrigues R, Demnerova K, Tresse O, Haddad N and Zagorec M (2016) Adhesion, Biofilm Formation, and Genomic Features of Campylobacter jejuni Bf, an Atypical Strain Able to Grow under Aerobic Conditions. Front. Microbiol. 7:1002. doi: 10.3389/fmicb.2016.01002
Received: 21 March 2016; Accepted: 13 June 2016;
Published: 30 June 2016.
Edited by:
Avelino Alvarez-Ordóñez, Teagasc Food Research Centre, IrelandReviewed by:
Helen Louise Brown, Cardiff Metropolitan University, UKGary Dykes, Curtin University, Australia
Copyright © 2016 Bronnec, Turoňová, Bouju, Cruveiller, Rodrigues, Demnerova, Tresse, Haddad and Zagorec. This is an open-access article distributed under the terms of the Creative Commons Attribution License (CC BY). The use, distribution or reproduction in other forums is permitted, provided the original author(s) or licensor are credited and that the original publication in this journal is cited, in accordance with accepted academic practice. No use, distribution or reproduction is permitted which does not comply with these terms.
*Correspondence: Monique Zagorec, bW9uaXF1ZS56YWdvcmVjQG9uaXJpcy1uYW50ZXMuZnI=