- 1School of Pharmacy and Biomolecular Sciences, Brighton University, Brighton, UK
- 2Department of Molecular Biosciences, The Wenner-Gren Institute, Stockholm University, Stockholm, Sweden
- 3Cardiff School of Pharmacy and Pharmaceutical Sciences, Cardiff University, Cardiff, UK
A surge in the level and scale of antibiotic resistance has prompted renewed interest in the application of bacteriophages to treat bacterial infections. However, concerns still exist over their efficacy and safety. Acinetobacter baumannii phage BS46, a member of the family Myoviridae, has previously been shown to be effective in murine models. The cytotoxic effect of this phage was evaluated in mouse fibroblast 3T3 cells using four different assays: trypan blue; staining with Hoechst and propidium iodide; lactate dehydrogenase release; and the MTS assay. The addition of phage concentrations up to 2 × 109 pfu/mL showed little to no impact on the viability of 3T3 cells after 24 h exposure using the different assays. This study demonstrates that phage BS46 is non-cytotoxic to 3T3 cells using four different assays and that appropriate quality assurance protocols for phage therapeutics are required.
Introduction
There is a resurgence of interest in phage to treat multidrug resistant infections in humans, with some bacteriophage (phage) products entering clinical trials (Rhoads et al., 2009; Wright et al., 2009) or in animal testing (Biswas et al., 2002; Morello et al., 2011). Although the use of phages within a clinical setting dates back to the early part of the 20th Century (Kutter et al., 2010), their use has been primarily confined to the former Soviet Union and Eastern Europe, in part due to concerns about safety and efficacy (Hanlon, 2007). Small numbers of phage preparations have been approved by the U.S. Food & Drug Administration for use as food additives within the food industry for the control of Listeria monocytogenes (Bren, 2007) and within the European Union (Anonymous, 2012). The small amount of human clinical trial data that exists in currently available literature has not demonstrated toxicity issues (Merabishvili et al., 2009). However, the potential to induce an immune response regardless of the level of preparation purity is of major concern to those seeking to utilize phage therapy clinically. There are a number of assays available to measure cell cytotoxicity. These often differ in the parameters they measure and have been shown to exhibit different levels of sensitivity (Fotakis and Timbrell, 2006). No standard assay for determining the cytotoxic effect of phage has been reported.
Acinetobacter baumannii is an opportunistic human pathogen causing a wide range of infections including wound, urinary tract infections (Antunes et al., 2014) and ventilator-acquired pneumonia (Qureshi et al., 2015). A. baumannii is an important multidrug resistant microorganism (Cai et al., 2012; Zarrilli et al., 2013; Antunes et al., 2014), with resistance to colistin being recently reported (Qureshi et al., 2015). The use of phages against this important pathogen is an exciting prospect, but for application in humans, particularly for wound infections, their cytotoxicity needs to be evaluated. Here the A. baumannii phage BS46, a member of the Myoviridae family was used. It has previously been shown to provide a protective effect in mice when challenged with up to five times the LD50 (108 cfu) of A. baumannii (Soothill, 1992).
The current investigation sought to determine the cytotoxic effects of a single A. baumannii phage and to evaluate different methods of determining cytotoxic effect following the addition of bacteriophage.
Materials and Methods
All chemicals and reagents were obtained from Fisher Scientific (Loughborough, UK) or Sigma–Aldrich (Gillingham, UK) unless otherwise stated in the text.
Sterile Lambda (λ) buffer was prepared by the addition of 2.5 mL 2% (w/v) gelatin to 6 mL 1 M Tris base at pH 7.2. To this 2.5 g MgSO4.7H20 was added and the resulting suspension made up to 1 L with dH2O. The resulting solution was then autoclaved at 121°C for 15 min.
Routine Culture of Bacterial and Bacteriophage Strains
Acinetobacter baumannii HER1401 and phage BS46 (Soothill, 1992; Ackermann et al., 1994) were obtained from The Felix d’Hérelle Reference Center for Bacterial Viruses, GREB, Faculté de Médecine Dentaire, Université Laval, Canada.
Acinetobacter baumannii HER1401 cultured in Tryptone Soy Broth (TSB; Oxoid, Basingstoke, UK) for 18 h at 37°C under 120 rpm agitation was used to inoculate 100 mL TSB. That was then incubated for 3–5 h at 37°C under 120 rpm agitation until an OD600 of 0.5 (∼108 cfu/mL) was reached. Bacterial suspensions were then infected with 1 mL phage suspension (∼106 pfu/mL), statically incubated at 37°C for 15 min and then incubated for a further 18 h at 37°C and 120 rpm.
Following incubation 10 mL chloroform was added and then the suspensions were incubated at 37°C, 120 rpm for 10 min prior to centrifugation at 2500 × g for 10 min. The resulting supernatant was then passed through a 0.45 μm syringe filter (Millipore, UK) to produce a crude lysate.
Production of Concentrated Purified Phage
Prior to use, crude lysates were enumerated and assessed for viability using the agar overlay method (Adams, 1959). To each crude lysate, sodium chloride was added to give a 1 M final concentration, stored on ice for 1 h and centrifuged at 11000 × g for 10 min at 4°C. Following centrifugation, 10% (w/v) polyethylene glycol 8000 (PEG 8000) was added to the supernatant and stored at 4°C for 18 h.
Suspensions were centrifuged at 11000 × g for 10 min at 4°C, the supernatant discarded, the pellet re-suspended in 11 mL λ buffer and 1 mL chloroform. The organic and aqueous phases were separated by centrifugation at 3000 × g for 15 min at 4°C. The organic phase was discarded and the aqueous phase made up to 50 mL with λ buffer and passed twice through 0.45 μm filters. Purified phage preparations were diluted in λ buffer to provide a working suspension of 8 × 109 pfu/mL and enumerated using the agar overlay method as previously described (Adams, 1959) and stored in sterile glass containers at 2–8°C.
Prior to use in cytotoxicity experiments, phages were diluted in λ buffer to yield 2 × 109, 2 × 108, and 2 × 107 pfu/mL and suspensions equilibrated to room temperature.
Cell Culture
Acinetobacter baumannii is a known bacterial pathogen associated with wounds. The embryonic Swiss albino mouse fibroblast cell line ‘3T3(+3)’ ECACC No. 89022402 was used here as a model since fibroblasts are associated with wound healing. 3T3 cells were grown in Dulbecco’s Modified Eagle’s Medium (DMEM) supplemented with 1 g/L glucose, L-glutamine and sodium bicarbonate, 10% (v/v) fetal calf serum (FCS) and 1% (v/v) penicillin and streptomycin. All cells were incubated at 37°C with 5% CO2 in a humidified atmosphere.
Analysis of Bacteriophage Cytotoxicity in 3T3 Cells
Preparation of Cells for Use in Cytotoxicity Experiments
Working culture plates were produced by the addition of 5000, 10000, 15000, or 2 × 105 3T3 cells (depending on the assay type) to wells of sterile Nunclon surface 24-well cell culture plates (Nunc Denmark) into a total volume of 1 mL pre-warmed supplemented DMEM and incubated for 24 h at 37°C in 95% air: 5% CO2 in a humidified atmosphere.
In order to remove mouse fibroblast 3T3 cells from 24 well plates, DMEM was removed, 200 μL of trypsin added to each well and plates incubated for 3–5 min at 37°C in a 95% air: 5% CO2 atmosphere. Cell suspensions were centrifuged at 400 × g for 5 min, the supernatant discarded and the pellet re-suspended in 1 mL fresh DMEM.
Trypan Blue Exclusion Assay
3T3 cell suspensions and 50 μL purified phage in experimental wells (n = 6) were prepared and incubated for 24, 48, and 72 h as before. λ buffer was used as a negative control.
Following trypsinisation, cell viability was assessed using a Trypan blue assay (Strober, 2001). In brief, 20 μL of cell suspension was added to 4 μL 0.4% (w/v) trypan blue solution in phosphate buffered saline (Sigma–Aldrich, Gillingham, UK) and the total number of cells immediately quantified from five squares of a haemocytometer at 100 × magnification using an inverted light microscope (Wilovert standard; Hund Wetzlar, Germany). If the cell density was too high then cells were diluted 1:10 in DMEM prior to imaging. Unstained cells were considered to be alive while blue stained cells were considered to be dead. In all cases, samples from each well were read in quintuplicate on two different occasions.
Hoechst and Propidium Iodide Staining for Cell Apoptosis
BS46 phages were inoculated to growing 3T3 cells as described above. λ buffer was used as a negative control.
Viable and apoptotic cells were quantified at multiple time points (t = 24, 48, 72 h) after phage addition using a modified propidium iodide (PI) staining method (Belloc et al., 1994). In brief, 900 μL of DMEM was mixed with 50 μL of a 1 mg/mL (in 10 mL demineralized water) PI solution and 50 μL of bisbenzimide. Wells were stained with PI and six random fields of view counted using an Axiovert 25 inverted fluorescence microscope (Karl Zeiss Ltd., Welwyn Garden City, UK) with a 420 nm filter. Viable cells were identified by uniform blue fluorescence and apoptotic cells by their fragmented nuclei with either blue or pink fluorescence.
Lactate Dehydrogenase (LDH) Release
Lactate Dehydrogenase (LDH) values were determined using the CytoTox 96 Non-Radioactive cytotoxicity assay (Promega, Southampton, UK) according to the manufacturer’s instructions. Absorbance at 492 nm was determined in an automatic Titertek Multiskan Plus MKII plate reader (LabX, Midland, ON, Canada), at 492 nm. Absorbance values were adjusted to compensate for the contribution of DMEM and the percentage cytotoxicity calculated as below;
% Cytotoxicity = [Experimental LDH release/Maximum LDH release] × 100.
Cell Viability Using an MTS Assay
Fifty micro liter of purified phage suspension were added to the experimental wells and incubated for 24, 48, and 72 h as described above. Negative controls containing 50 μL λ buffer and untreated cells were also performed. The number of viable cells was determined using the CellTiter 96 aqueous One Solution Cell Proliferation Assay (MTS; Promega, Madison, WI, USA) according to the manufacturer’s instructions. Absorbance at 492 nm was determined on an automatic Titertek Multiskan Plus MKII plate reader (LabX, Midland, Canada) and expressed as a percentage of non-treated controls to calculate the percentage proliferation status (Carmichael et al., 1987).
Statistical Analysis
All experiments were performed in triplicate on different days unless otherwise stated in the text.
Trypan Blue Exclusion Test
Data were statistically analyzed using a Kruskal–Wallis One way analysis of variance due to the data being non-normally distributed.
Apoptotic Assay
Data were statistically treated using a Univariate General linear model and Levene’s test.
MTS Assay
One-way between groups ANOVA with Tukey’s post hoc test was used to compare cell viability as percentage of controls.
Results
Trypan Blue Assay
The initial concentration of phage showed a non-significant impact (p > 0.05) on the viability of 3T3 cells after 24 h exposure (Figure 1) with 2 × 108 pfu/mL exhibiting the highest reduction in viability (∼10%). Seeding density (5 × 103 or 2 × 105 cells/well) also had no significant effect on the viability of cells following 24 h exposure to phage (p > 0.1 Tukey’s and Kruskal–Wallis).
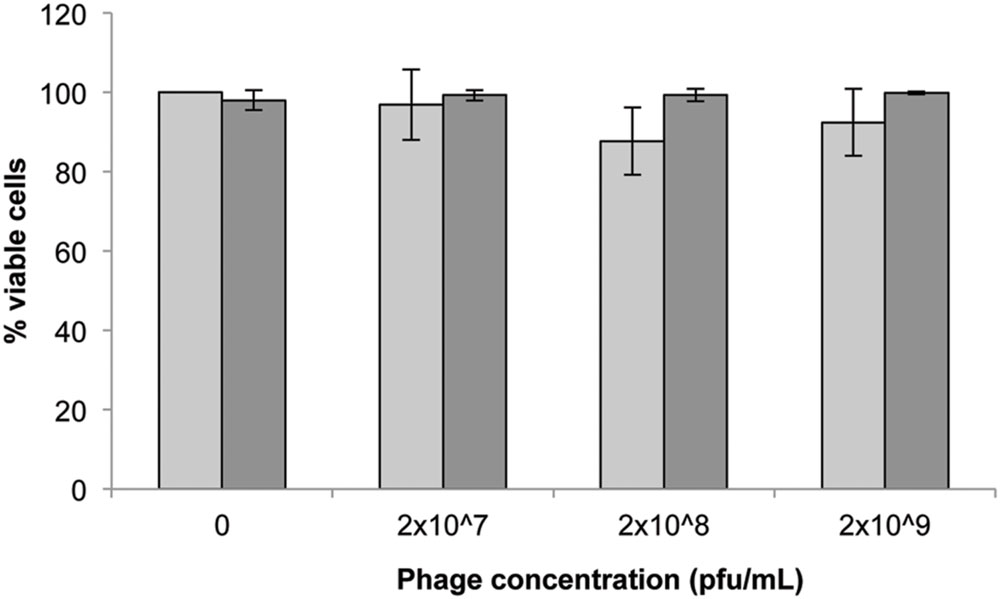
FIGURE 1. 3T3 mouse fibroblast cell viability following 24 h exposure to Acinetobacter phage BS46 in DMEM by trypan blue exclusion assay. : 5 × 103 cells/well;
: 2 × 105 cells/well. Data are the mean of 3 replicates ± SD.
Hoechst and Propidium Iodide Staining
Statistically significant reductions in the number of viable cells were observed following 72 h incubation with either 2 × 107 and 2 × 108 pfu/mL compared to untreated controls (Figure 2; p < 0.001). Incubation time had no significant impact on cell viability (p = 0.418).
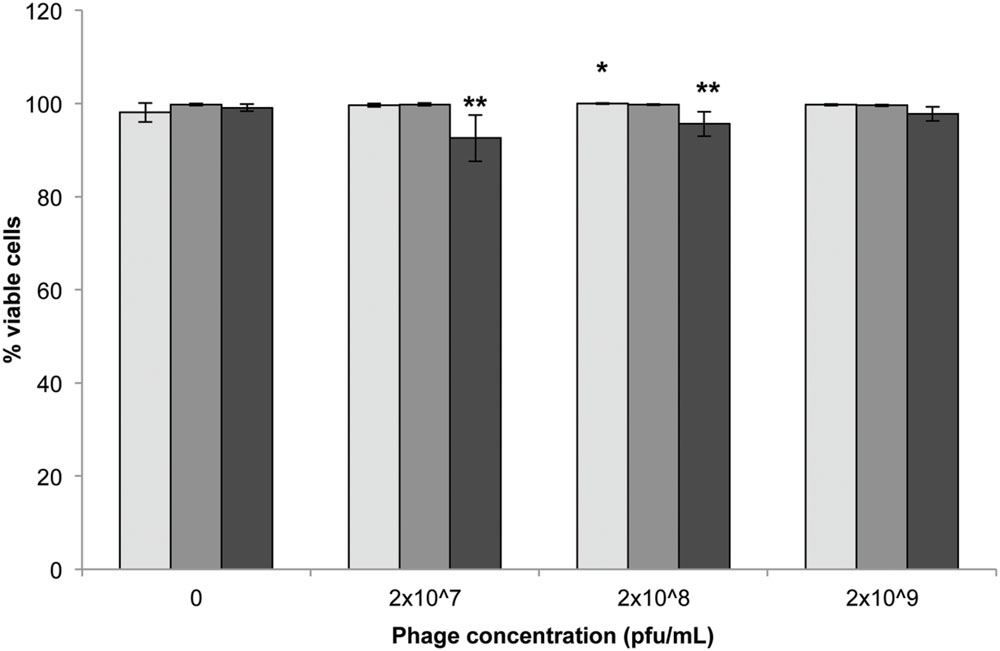
FIGURE 2. 3T3 mouse fibroblast cell viability following exposure to Acinetobacter phage BS46 by Hoechst propidium iodide staining. : 24,
: 48 and
: 72 h. Data shown are the mean of 3 replicates ± SD. ∗p < 0.05 compared to control, ∗∗p < 0.001 compared to controls and highest phage concentration (2 × 109 pfu/mL) at 72 h.
LDH Release
Phage addition at varying concentrations resulted in small but not statistically significant increases in cell death compared to untreated samples (between 5 and 10% reduction; Figure 3) with the exception of 2 × 109 pfu/mL at 24 h (p < 0.001).
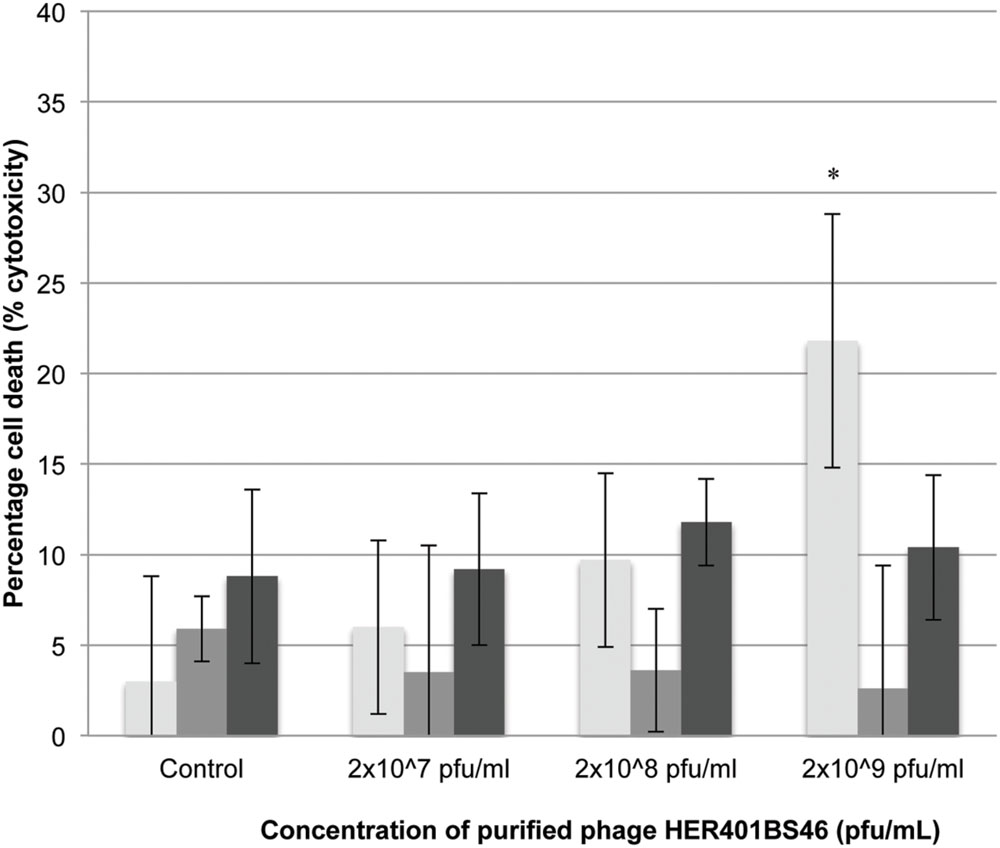
FIGURE 3. LDH release of 3T3 mouse fibroblast cells following exposure to Acinetobacter phage BS46. ∗p < 0.001 compared to the control. : 24,
: 48, and
: 72 h. Data shown are the mean of 3 replicates ± SD.
MTS Assay
Following 24 h exposure to different concentrations of phages (2 × 109, 2 × 108, or 2 × 107 pfu/mL) the number of viable cells appeared to increase at higher initial seeding densities (10000 and 15000 cells/well) compared to controls treated with λ buffer only (Figure 4A). At 48 h incubation there was no significant difference between phage samples and λ buffer (Figure 4B; p > 0.5). After 72 h cells exposed to phages cell viability increased significantly compared to the controls. Cells seeded at 10000 cells/well remained more viable (Figure 4C; p < 0.05) when exposed to 2 × 109 and 2 × 108 pfu/mL phage, compared to λ buffer and untreated (DMEM only) cells.
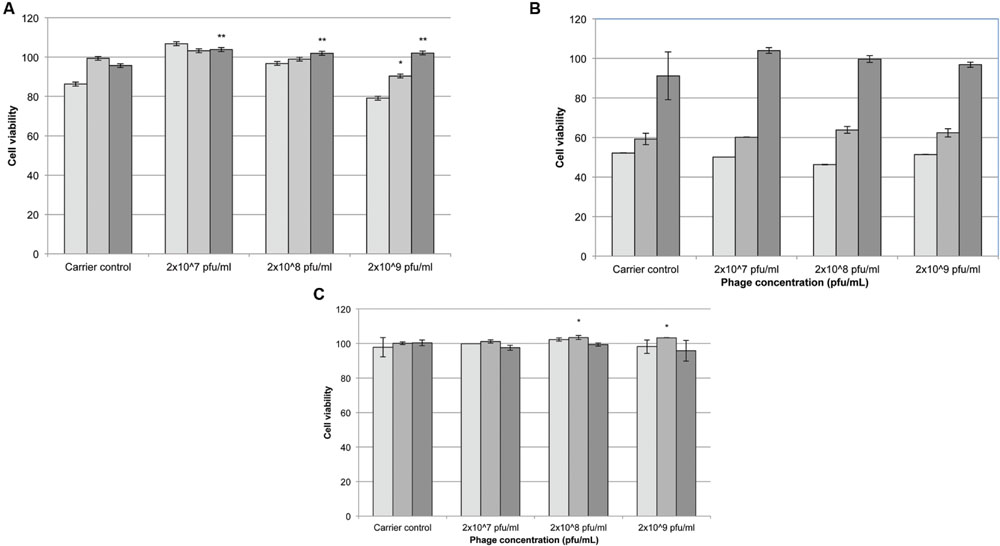
FIGURE 4. The effect of Acinetobacter phage BS46 on MTS formation of 3T3 cells. Percentage cell viability was calculated from the untreated cells which represent 100% viability. Carrier control corresponds to cells treated with λ buffer. (A): 24 h, (B): 48 h, and (C): 72 h incubation. 5000 cells/well,
10000 cells/well and
15000 cells/well. ∗p < 0.05, compared to 2 × 107 pfu/mL; ∗∗p < 0.05 compared to λ buffer. Data are the mean of 3 replicates ± SD.
Discussion
Bacterial cell products can have both direct and indirect cytotoxic effects on cells in the stationary phase (Perfetto et al., 2003), particularly through the action of bacterial endotoxins which affect the expression of adhesion molecules, inflammatory responses (Tang et al., 2011), cytokine release (Perfetto et al., 2003; Tardif et al., 2004), or the release of bacterial toxins (Los et al., 2013). However, little information exists in the current literature on the effects of the direct addition of therapeutic bacteriophages on immortalized cell lines such as 3T3 fibroblasts.
In the current investigation, a partially purified A. baumannii phage preparation was not cytotoxic to 3T3 mouse fibroblast cell line, although some assay dependent differences in viability were observed. No statistically significant differences were seen between 3T3 cells treated with phage and untreated controls, corresponding with similar investigations performed by Merabishvili et al. (2009) against human neonatal foreskin keratinocytes when assessed by trypan blue exclusion. While patient derived cells will offer the closest analog to whole organism testing in vitro, the use of such cell types comes with associated cost and ethical considerations as well as increased variation between multiple donors. Although no substitute for in vivo testing, the use of immortalized cell lines would decrease the overall research cost of preclinical studies and enable standardization between laboratories, allowing for better comparative testing to be performed. In addition fibroblasts were used in an attempt to understand the effect of phage on skin or wounds, recognizing that A. baumannii is an important wound pathogen.
Although no cytotoxic effect was observed with all the assays tested, the assessment of cellular viability with the MTS assay suggested that phages provided a positive effect on cell viability. This observation supports that of Chung et al. (2010) who reported that genetically engineered M13 bacteriophage improved cell attachment under specific experimental conditions. Although no direct cytotoxic effect was observed, the addition of phages has been shown to induce pro-inflammatory cytokines such as IL-10 and IFN-γ (Dąbrowska et al., 2014; Park et al., 2014). Majewska et al. (2015) reported a weak antibody production in mice following T4 phages ingestion. However, this is dependent on phage type and the protein composition of individual phages (Dąbrowska et al., 2014) and would be of particular importance in non-topical applications that would result in the increased exposure of phages to the immune system (Hodyra-Stefaniak et al., 2015). Here we have used a partially purified phage and it is possible that bacterial debris were present in the preparation. However, the phage preparation used here did not show any cytotoxicity overall. The endotoxin level of any phage product would need to be measured for regulatory purposes before commercialization. A number of methods to reduce endotoxin levels have been described: a single round of PEG precipitation and centrifugation has been shown to remove up to 88% of endotoxins from a bacteriophage preparation (Branston et al., 2015). However, additional purification stages such as ultracentrifugation in a CsCl gradient or chromatographic methods will further reduce impurities (Boratyński et al., 2004).
The current investigation sought to assess the cytotoxicity of a purified preparation of A. baumannii phage BS46 using four separate methods. Some discrepancies in the results were observed, notably at high phage concentrations, with the PI and MTS assay showing some small but statistically significant reduction in cell number and the LDH assay showing no significant differences in cell viability. The LDH assay presented the highest variability in results as indicated by the large error bars. It measures the activity of the oxidoreductase of LDH, a stable enzyme, released from damaged cells in the culture medium. Variability in activity, which has been reported in other applications (Bopp and Lettieri, 2008), may be cause by differences in cell seeding. Such variability in results was not noted with the other assays performed. Both the Trypan blue exclusion assay and the Hoechst and PI assays require microscope based analysis which may limit their usefulness although high throughput protocol could potentially be increased using flow cytometry. The MTS assay gives indirect measurement of viability [requires metabolism of MTS (3-(4,5-dimethylthiazol-2-yl)-5-(3-carboxymethoxyphenyl)-2-(4-sulfophenyl)-2H-tetrazolium] to formazan and not a measurement of cell damage. This indirect measurement based on cell metabolism may explain why the MTS assay was the only one that showed an increased in cell viability. For developing a product for phage therapy, for which a high number of samples need to be processed, the high throughput trypan blue assay may be the most practical protocol to use. It is thus important to consider the method used to assess the cytotoxic effects of phage preparations and highlights the pressing need for standardized phage testing protocols.
The purified phage suspension exhibited little toxicity and this result is encouraging for the treatment of topical infections with phages. For non-topical applications, the assessment of cytotoxicity could be complemented with the determination of immune-stimulatory capacity (Merabishvili et al., 2009; Szermer-Olearnik and Boratyński, 2015).
Author Contributions
Conceived and designed experiments: AH, GH, SD, J-YM. Performed experiments AH. Analyzed data: AH, GH, SD, J-YM. Wrote the manuscript: CC, J-YM, GH, SD.
Funding
This study resulted from a Ph.D. studentship funded by the University of Brighton and the Royal Pharmaceutical Society of Great Britain.
Conflict of Interest Statement
The authors declare that the research was conducted in the absence of any commercial or financial relationships that could be construed as a potential conflict of interest.
References
Ackermann, H. W., Brochu, G., and Konjin, H. P. E. (1994). Classification of Acinetobacter phages. Arch. Virol. 135, 345–54. doi: 10.1007/BF01310019
Anonymous (2012). Scientific opinion on the evaluation of the safety and efficacy of listextm P100 for the removal of listeria monocytogenes surface contamination of raw fish. EFSA J. 10:2615.
Antunes, L. C., Visca, P., and Towner, K. J. (2014). Acinetobacter baumannii: evolution of a global pathogen. Pathog. Dis. 71, 292–301. doi: 10.1111/2049-632X.12125
Belloc, F., Dumain, P., Boisseau, M. R., Jalloustre, C., Reiffers, J., Bernard, P., et al. (1994). A flow cytometric method using Hoechst 33342 and propidium iodide for simultaneous cell cycle analysis and apoptosis determination in unfixed cells. Cytometry 17, 59–65. doi: 10.1002/cyto.990170108
Biswas, B., Adhya, S., Washart, P., Paul, B., Trostel, A. N., Powell, B., et al. (2002). Bacteriophage therapy rescues mice bacteremic from a clinical isolate of vancomycin-resistant Enterococcus faecium. Infect. Immun. 70, 204–210. doi: 10.1128/IAI.70.1.204-210.2002
Boratyński, J., Syper, D., Weber-Dabrowska, B., Łusiak-Szelachowska, M., Poźniak, G., and Górski, A. (2004). Preparation of endotoxin-free bacteriophages. Cell. Mol. Biol. Lett. 9, 253–259.
Bopp, S. K., and Lettieri, L. (2008). Comparison of four different colorimetric and fluorometric cytotoxicity assays in a zebrafish liver cell line. BMC Pharmacol. 8:8. doi: 10.1186/1471-2210-8-8
Branston, S. D., Wright, J., and Keshavarz-Moore, E. (2015). A non-chromatographic method for the removal of endotoxins from bacteriophages. Biotechnol. Bioeng. 112, 1714–1719. doi: 10.1002/bit.25571
Cai, Y., Chai, D., Wang, R., Liang, B., and Bai, N. (2012). Colistin resistance of Acinetobacter baumannii: clinical reports, mechanisms and antimicrobial strategies. J. Antimicrob. Chemother. 67, 1607–1615. doi: 10.1093/jac/dks084
Carmichael, J., DeGraff, W. G., Gazdar, A. F., Minna, J. D., and Mitchell, J. B. (1987). Evaluation of a tetrazolium-based semiautomated colorimetric assay: assessment of radiosensitivity. Cancer Res. 47, 943–946.
Chung, W. J., Merzlyak, A., and Lee, S. W. (2010). Fabrication of engineered M13 bacteriophages into liquid crystalline films and fibers for directional growth and encapsulation of fibroblasts. Soft Matter 6, 4454–4459. doi: 10.1039/c0sm00199f
Dąbrowska, K., Miernikiewicz, P., Piotrowicz, A., Hodyra, K., Owczarek, B., Lecion, D., et al. (2014). Immunogenicity studies of proteins forming the T4 phage head surface. J. Virol. 88, 12551–12557. doi: 10.1128/JVI.02043-14
Fotakis, G., and Timbrell, J. A. (2006). In vitro cytotoxicity assays: comparison of LDH, neutral red, MTT and protein assay in hepatoma cell lines following exposure to cadmium chloride. Toxicol. Lett. 160, 171–177. doi: 10.1016/j.toxlet.2005.07.001
Hanlon, G. W. (2007). Bacteriophages: an appraisal of their role in the treatment of bacterial infections. Int. J. Antimicrob. Agents 30, 118–128. doi: 10.1016/j.ijantimicag.2007.04.006
Hodyra-Stefaniak, K., Miernikiewicz, P., Drapała, J., Drab, M., Jończyk-Matysiak, E., Lecion, D., et al. (2015). Mammalian host-versus-phage immune response determines phage fate in vivo. Sci. Rep. 5:14802. doi: 10.1038/srep14802
Kutter, E., De Vos, D., Gvasalia, G., Alavidze, Z., Gogokhia, L., Kuhl, S., et al. (2010). Phage therapy in clinical practice: treatment of human infections. Curr. Pharm. Biotechnol. 11, 69–86. doi: 10.2174/138920110790725401
Los, F. C., Randis, T. M., Aroian, R. V., and Ratner, A. J. (2013). Role of pore-forming toxins in bacterial infectious diseases. Microbiol. Rev. 77, 173–207. doi: 10.1128/mmbr.00052-12
Majewska, J., Beta, W., Lecion, D., Hodyra-Stefaniak, K., Klopot, A., Kazmierczak, Z., et al. (2015). Oral application of T4 phage induces weak antibody production in the gut and in the blood. Viruses 7, 4783–4799. doi: 10.3390/v7082845
Merabishvili, M., Pirnay, J.-P., Verbeken, G., Chanishvili, N., Tediashvili, M., Lashkhi, N., et al. (2009). Quality-controlled small-scale production of a well-defined bacteriophage cocktail for use in human clinical trials. PLoS ONE 4:e4944. doi: 10.1371/journal.pone.0004944
Morello, E., Saussereau, E., Maura, D., Huerre, M., Touqui, L., and Debarbieux, L. (2011). Pulmonary bacteriophage therapy on Pseudomonas aeruginosa cystic fibrosis strains: first steps towards treatment and prevention. PLoS ONE 6:e16963. doi: 10.1371/journal.pone.0016963
Park, K., Cha, K. E., and Myung, H. (2014). Observation of inflammatory responses in mice orally fed with bacteriophage T7. J. Appl. Microbiol. 117, 627–633. doi: 10.1111/jam.12565
Perfetto, B., Donnarumma, G., Criscuolo, D., Paoletti, I., Grimaldi, E., Tufano, M. A., et al. (2003). Bacterial components induce cytokine and intercellular adhesion molecules-1 and activate transcription factors in dermal fibroblasts. Res. Microbiol. 154, 337–344. doi: 10.1016/S0923-2508(03)00084-6
Qureshi, Z. A., Hittle, L. E., O’Hara, J. A., Rivera, J. I., Syed, A., Shields, R. K., et al. (2015). Colistin-resistant Acinetobacter baumannii: beyond carbapenem resistance. Clin. Infect. Dis. 60, 1295–1303. doi: 10.1093/cid/civ048
Rhoads, D. D., Wolcott, R. D., Kuskowski, M. A., Wolcott, B. M., Ward, L. S., and Sulakveldze, A. (2009). Bacteriophage therapy of venous leg ulcers in humans: results of a phase i safety trial. J. Wound Care 18, 237–243. doi: 10.12968/jowc.2009.18.6.42801
Soothill, J. S. (1992). Treatment of experimental infections of mice with bacteriophages. J. Med. Microbiol. 37, 258–261. doi: 10.1099/00222615-37-4-258
Strober, W. (2001). Trypan blue exclusion test of cell viability. Curr. Prot. Immunol. 3(Suppl. 21), A.3B.1–A.3B.2. doi: 10.1002/0471142735.ima03bs21
Szermer-Olearnik, B., and Boratyński, J. (2015). Removal of endotoxins from bacteriophage preparations by extraction with organic solvents. PLoS ONE 10:e0122672. doi: 10.1371/journal.pone.0122672
Tang, Y., Sun, F., Li, X., Zhou, Y., Yin, S., and Zhou, X. (2011). Porphyromonas endodontalis lipopolysaccharides induce RANKL by mouse osteoblast in a way different from that of Escherichia coli lipopolysaccharide. J. Endod. 37, 1653–1658. doi: 10.1016/j.joen.2011.08.015
Tardif, F., Ross, G., and Rouabhia, M. (2004). Gingival and dermal fibroblasts produce interleukin-1 beta converting enzyme and interleukin-1 beta but not interleukin-18 even after stimulation with lipopolysaccharide. J. Cell. Physiol. 198, 125–132. doi: 10.1002/jcp.10400
Wright, A., Hawkins, C. H., Änggård, E. E., and Harper, D. R. (2009). A controlled clinical trial of a therapeutic bacteriophage preparation in chronic otitis due to antibiotic-resistant Pseudomonas aeruginosa; a preliminary report of efficacy. Clin. Otolaryngol. 34, 349–357. doi: 10.1111/j.1749-4486.2009.01973.x
Keywords: bacteriophage, cytotoxicity, Acinetobacter baumannii, lactate dehydrogenase, MTS assay, trypan blue, hoechst stain, propidium iodide
Citation: Henein AE, Hanlon GW, Cooper CJ, Denyer SP and Maillard J -Y (2016) A Partially Purified Acinetobacter baumannii Phage Preparation Exhibits no Cytotoxicity in 3T3 Mouse Fibroblast Cells. Front. Microbiol. 7:1198. doi: 10.3389/fmicb.2016.01198
Received: 06 June 2016; Accepted: 19 July 2016;
Published: 03 August 2016.
Edited by:
Peter Mullany, University College London, UKReviewed by:
Eric Christopher Keen, Washington University, USABenjamin Andrew Evans, Anglia Ruskin University, UK
D. Ipek Kurtboke, University of the Sunshine Coast, Australia
Copyright © 2016 Henein, Hanlon, Cooper, Denyer and Maillard. This is an open-access article distributed under the terms of the Creative Commons Attribution License (CC BY). The use, distribution or reproduction in other forums is permitted, provided the original author(s) or licensor are credited and that the original publication in this journal is cited, in accordance with accepted academic practice. No use, distribution or reproduction is permitted which does not comply with these terms.
*Correspondence: Jean-Yves Maillard, bWFpbGxhcmRqQGNhcmRpZmYuYWMudWs=
†Deceased