- 1Department of Anaesthetics, Glan Clwyd Hospital, Bodelwyddan, Wales
- 2Public Health Wales, Temple of Peace and Health, Cardiff, Wales
- 3Adult Critical Care Services, University Hospital Wales, Cardiff, Wales
- 4Cardiff Institute of Infection and Immunity, Cardiff University, Cardiff, Wales
- 5Directorate of Critical Care, Royal Gwent Hospital, Newport, Wales
Introduction: The reported incidence of ventilator-associated pneumonia (VAP) in Wales is low compared with surveillance data from other European regions. It is unclear whether this reflects success of the Welsh healthcare-associated infection prevention measures or limitations in the application of European VAP surveillance methods. Our primary aim was to investigate episodes of ventilator-associated respiratory tract infection (VARTI), to identify episodes that met established criteria for VAP, and to explore reasons why others did not, according to the Hospitals in Europe Link for Infection Control through Surveillance (HELICS) definitions.
Materials and Methods: During two 14-day study periods 2012–2014, investigators reviewed all invasively ventilated patients in all 14 Welsh Intensive Care Units (ICUs). Episodes were identified in which the clinical team had commenced antibiotic therapy because of suspected VARTI. Probability of pneumonia was estimated using a modified Clinical Pulmonary Infection Score (mCPIS). Episodes meeting HELICS definitions of VAP were identified, and reasons for other episodes not meeting definitions examined. In the second period, each patient was also assessed with regards to the development of a ventilator-associated event (VAE), according to recent US definitions.
Results: The study included 306 invasively ventilated patients; 282 were admitted to ICU for 48 h or more. 32 (11.3%) patients were commenced on antibiotics for suspected VARTI. Ten of these episodes met HELICS definitions of VAP, an incidence of 4.2 per 1000 intubation days. In 48% VARTI episodes, concurrent chest radiography was not performed, precluding the diagnosis of VAP. Mechanical ventilation (16.0 vs. 8.0 days; p = 0.01) and ICU stay (25.0 vs. 11.0 days; p = 0.01) were significantly longer in patients treated for VARTI compared to those not treated. There was no overlap between episodes of VARTI and of VAE.
Discussion: HELICS VAP surveillance definitions identified less than one-third of cases in which antibiotics were commenced for suspected ventilator-associated RTI. Lack of chest radiography precluded nearly 50% cases from meeting the surveillance definition of VAP, and as a consequence we are almost certainly underestimating the incidence of VAP in Wales.
Introduction
Pneumonia is the major cause of ICU-acquired infection in Europe and the United States (Vincent et al., 1995; Richards et al., 2000; Burgmann et al., 2010), the vast majority of episodes occurring in intubated and mechanically-ventilated patients (ventilator-associated pneumonia, VAP; Kohlenberg et al., 2010). VAP tends to occur in the sickest of critically ill patients (Chastre and Fagon, 2002; Blot et al., 2014) and is associated with appreciable morbidity, mortality, and financial cost (Safdar et al., 2005; Bekaert et al., 2011; Melsen et al., 2013; Muscedere et al., 2013). Given that some factors associated with development of VAP appear modifiable (Barbier et al., 2013), attention has been focused on regional and national healthcare-associated infection (HAI) surveillance indices to guide local quality improvement and wider bench-marking.
The Welsh Healthcare-Associated Infection Programme (WHAIP) has monitored episodes of VAP through the National Mandatory Healthcare Associated Infection Surveillance Programme for Wales since 2008. Using European HELICS surveillance definitions (Table 1; HELICS, 2004) clinical staff in each ICU have reported VAP episodes equivalent to an incidence density of 1.2–2.2 per 1000 intubation days for 2009–2013 (WHAIP, 2014). These rates are low when compared to data from other areas of the UK (Scotland, 6.5 per 1000 intubation days; HPS/SICSAG, 2013) and Europe (12.2 per 1000 intubation days; ECDC, 2008). This may reflect the success of national healthcare improvement initiatives in Wales (e.g., the 1000 Lives Campaign), but could feasibly underestimate the true incidence of ventilator-associated pneumonia (VAP) given how subjective the clinical and radiological features of VAP can be (Klompas, 2012). In the US, loss of confidence has led to the recent revision of ICU surveillance methodology and the introduction of alternate ventilator-associated event (VAE) surveillance definitions by the Centers for Disease Control and Prevention National Healthcare Safety Network (CDC NHSN). The primary focus of these definitions is now an objective deterioration in oxygenation (Figure 1; Magill et al., 2013). Such “Ventilator-Associated Conditions” (VACs) may then potentially be sub-classified according to other unambiguous features (temperature, white cell count, and initiation of antibiotic therapy) as an Infection-related Ventilator-Associated Complication (I-VAC) for mandatory surveillance purposes.
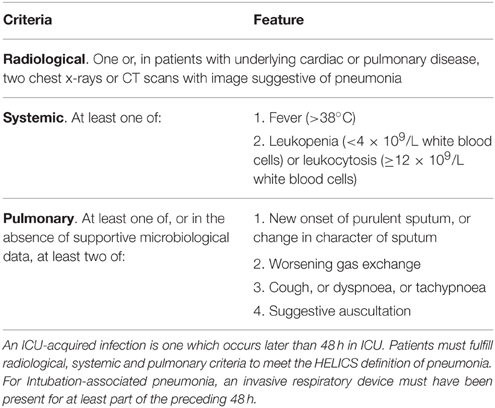
Table 1. HELICS case definition of intubation-associated pneumonia (HELICS, 2004).
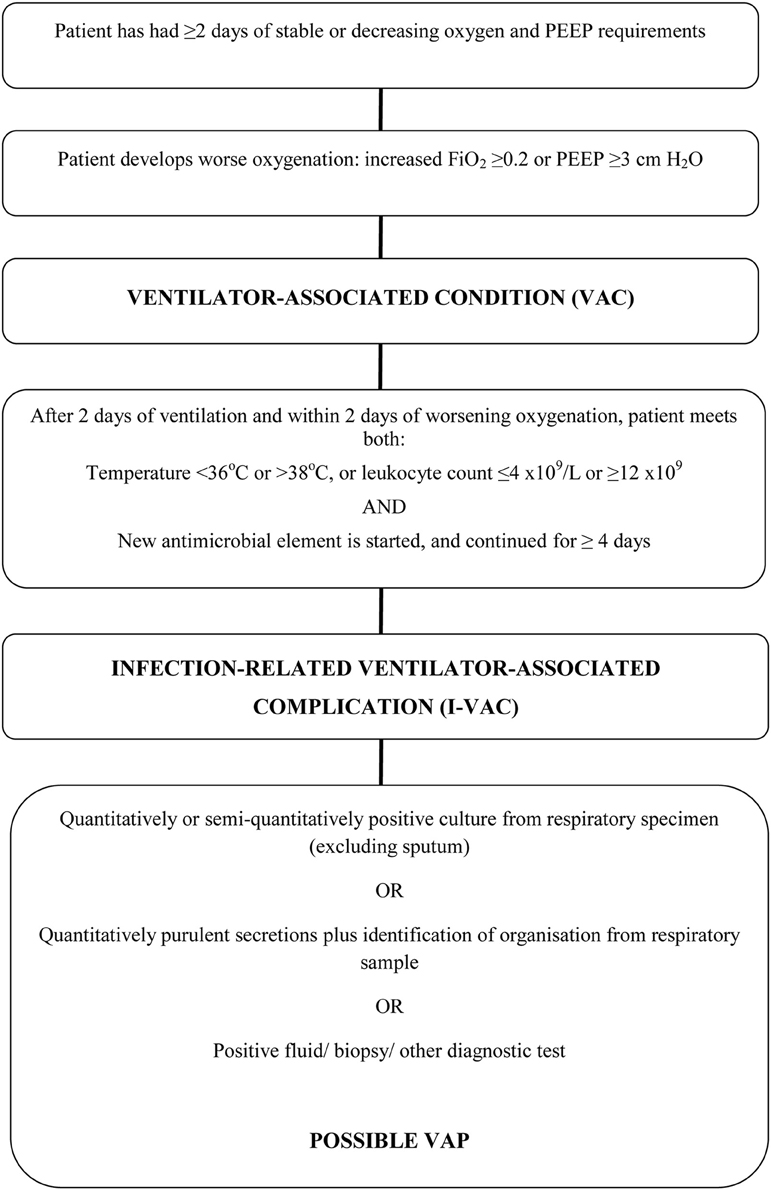
Figure 1. Ventilator-associated events surveillance algorithm. Adapted from Magill (Klompas, 2012).
Our primary aim was to identify episodes of VAP according to the HELICS definition, and to explore reasons why other episodes of ventilator-associated respiratory tract infection (VARTI) did not meet the criteria. Our secondary aims were to investigate clinical outcomes among the wider group of patients being treated for suspected VARTI, and to explore the feasibility of using alternative methods to identify infective ventilator-associated morbidity for surveillance purposes in Wales.
Materials and Methods
A prospective evaluation of current clinical practice by individual Health Boards was conducted within the mandated critical care surveillance programme in Wales. For this reason formal ethical approval was not necessary, however the Clinical Audit departments at each center approved participation.
The study was conducted in two stages. During the initial 14-day study period (November 2012), local investigators identified through the Welsh Intensive Care Society Audit and Research Group (WICSARG) were asked to complete a daily standard data collection form for each intubated and mechanically ventilated patient either already present on or admitted to their ICU. A VARTI was considered to have occurred when the clinical team commenced an invasively ventilated patient on systemic antibiotic therapy for suspected respiratory infection 48 h or more after ICU admission. For each episode, clinical and radiological features were recorded in order to make a diagnosis of pneumonia according to the HELICS definition (Table 1). The modified Clinical Pulmonary Infection Score (mCPIS) was also calculated (Table 2; Fartoukh et al., 2003) and used to give an indication of likelihood of pneumonia, with a score of 6 or more interpreted as suggesting a high probability. Microbiology results from contemporaneous respiratory specimens were also collected.
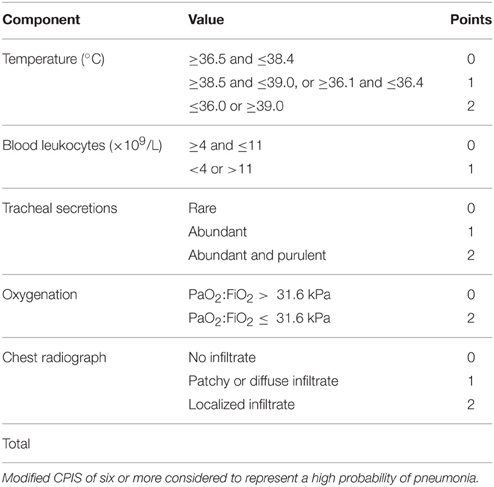
Table 2. Modified clinical pulmonary score (mCPIS; Fartoukh et al., 2003).
The second stage of the study was undertaken between March and April 2014. During this period, ventilator settings and physiological measurements were also collected daily to enable additional identification of VAE according to NHSN CDC definitions, (see Figure 1; Magill et al., 2013). Episodes of VARTI and VAP according to the HELICS definition were identified as previously. For this stage of the study, investigators were also asked to submit 90-day outcome data (duration of mechanical ventilation and ICU length of stay, ICU, and hospital mortality) from their local WardWatcher (Critical Care Audit Ltd., UK) database.
Statistical Analysis
Our primary outcomes were: episodes of VARTI (as defined above) and of VAP, according to the HELICS definition. Secondary outcomes were: probability of pneumonia in all cases of VARTI according to a mCPIS, and episodes of VAE and 90-day outcomes (for patients in the second stage of the study). Data was collected using Microsoft Access and analyzed using MINITAB 13.32 (Minitab Inc., UK). T-tests were used to analyze normally-distributed data and Mann–Whitney U-test for non-parametric data. Proportions were compared using Fisher's exact test.
Results
We collected data on 306 invasively ventilated ICU patients; of these, 282 were admitted to ICU for 48 h or more. VARTI developed in 32 (11.3%) patients (one patient developed two episodes of VARTI), a median period of nine (IQR 5–14) days after intubation and initiation of mechanical ventilation. There were no significant differences in baseline characteristics between patients developing VARTI vs. other patients (Table 3). The commonest features present when antibiotics were commenced were: abnormal white cell count and offensive and/or purulent sputum (48 and 45%, episodes, respectively), whereas deterioration in gas exchange was relatively uncommon (24% episodes; Table 4).
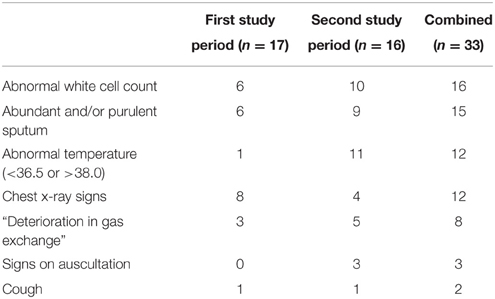
Table 4. Clinical and radiological features present at initiation of antibiotics for suspected VARTI.
Among those with VARTI, only 10 episodes met the HELICS definition for VAP (Figure 2 and Table 5). The total number of intubation days (for ventilated patients admitted to ICU for 48 h or more) was 2381, giving a VAP rate of 4.2 per 1000 intubation days. In total 3.5% of all patients admitted to ICU for 48 h or more developed a HELICS-defined VAP. Six of the 10 HELICS-defined cases of VAP were “PN1,” i.e., on the basis of a minimally-contaminated invasive respiratory sample (e.g., BAL; Table 6). The main reasons that episodes of VARTI failed to meet HELICS definitions are presented in Table 7. Most strikingly, in 16 (48.4%) cases, chest radiography was not performed on the day antibiotics were prescribed for new onset respiratory tract infection precluding diagnosis according to HELICS definition.
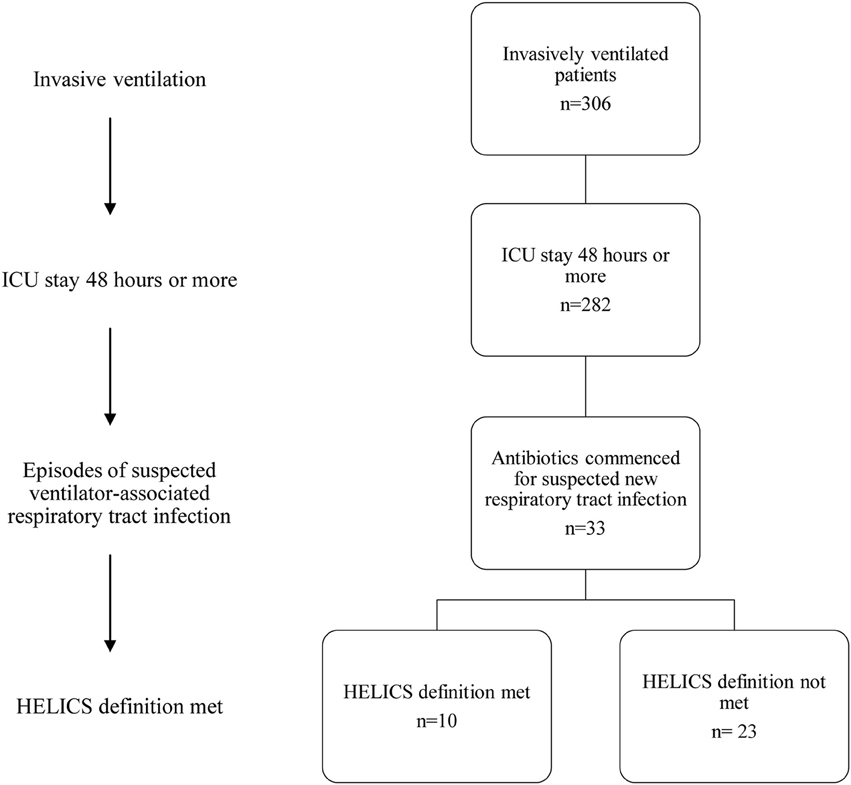
Figure 2. Flowchart demonstrating numbers of patients with suspected ICU-acquired respiratory tract infection.
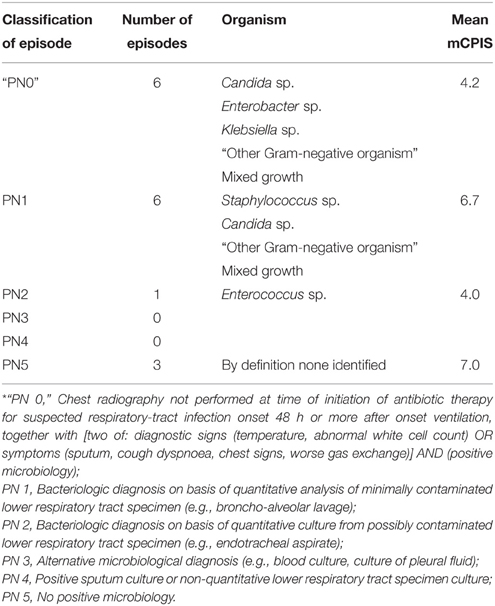
Table 6. PN classification of HELICS-defined episodes of VAP (HELICS, 2004) and “non-HELICS” episodes referred to as “PN 0”*.
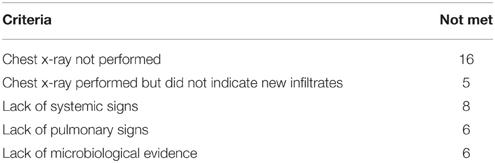
Table 7. Reasons that episodes of VARTI did not meet HELICS definition (HELICS definition not met in 23 out of 33 VARTI episodes).
Of the 10 HELICS-defined cases of VAP, seven had mCPIS of six or more (suggesting high probability of pneumonia). In the 23 episodes in which HELICS criteria were not fulfilled only two had a mCPIS score of six or more; mean mCPIS was significantly lower among this cohort of patients [3.8 vs. 6.5 (p = 0.01); Table 5], though positive microbiology was subsequently identified in approximately two-thirds of cases. In six of these 23 non-HELICS episodes, there were two or more signs and symptoms suggestive of pneumonia as well as subsequent positive microbiology in the absence of chest x-ray examination, we have referred to these episodes as “PN0” (Table 6).
Applying new CDC NHSN criteria, we identified four cases of ventilator-associated complication (VAC) among the 113 patients ventilated for 48 h or more in the second cohort: three on the basis of as a result of sustained increase in PEEP application and one because of sustained increase in inspired oxygen concentration (Table 8). In none of these cases could an episode of VAC be further sub-classified as an IVAC. There was no overlap between cases of VARTI and VAC (see Euler diagram, Figure 3).
With regards to clinical outcomes (measured only in this second stage), initiation of antibiotic therapy for VARTI 48 h or more after onset of mechanical ventilation was associated with significantly prolonged mechanical ventilation and ICU stay [median 16.0 vs. 8.0 days (p = 0.01) and 25.0 vs. 11.0 days (p = 0.01), respectively], though not a significant increase in ICU or hospital mortality (Tables 9, 10). Sample sizes were too small to compare outcome data between patients who did and did not develop VAP or VAC.
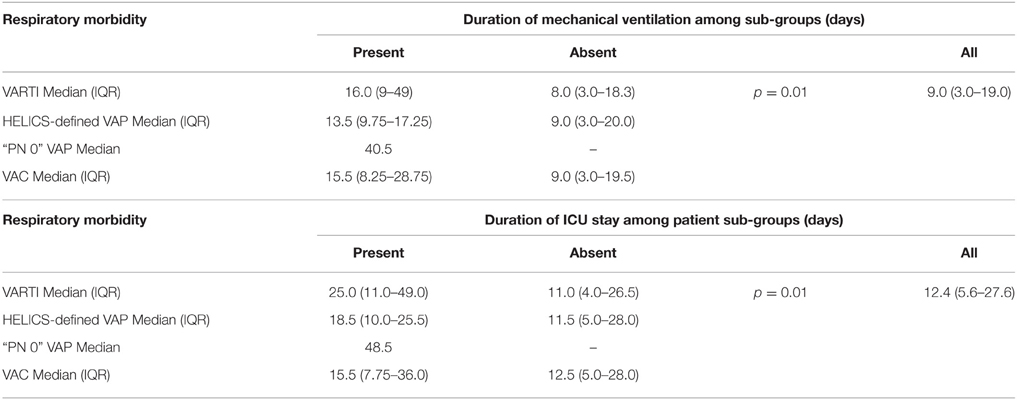
Table 9. Outcomes: durations of mechanical ventilation and ICU length of stay, according to ICU-acquired respiratory morbidity.
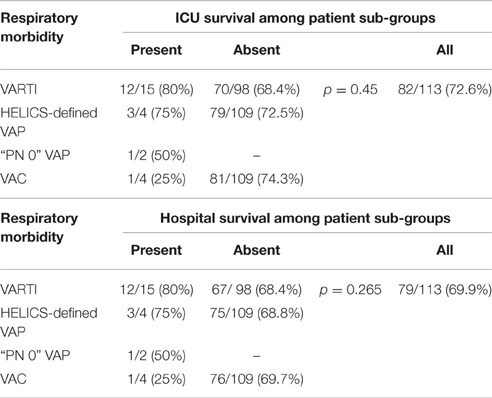
Table 10. Outcomes: survival to ICU and hospital discharge, according to ICU-acquired respiratory morbidity.
Discussion
This study sheds significant light on the diagnostic processes undertaken by ICU clinicians in Wales prior to commencing antibiotics for suspected VARTI and provides new insight into the utility of the currently adopted HELICS criteria used for surveillance.
Our study investigated 282 invasively ventilated patients admitted to ICU for 48 h or more, and in this group antibiotics were commenced for suspected VARTI in 32 (11.3%) patients. The most common clinical features present when clinicians commenced antibiotic therapy were abnormal white cell count and abundant and/or purulent sputum, rather than deterioration in gas exchange which has been the focus of new CDC NHSN surveillance methods. Initiation of antibiotic therapy for suspected VARTI was associated with an approximate doubling of duration of mechanical ventilation and ICU stay.
There were 10 episodes of VAP according to HELICS definitions, equating to 4.2 episodes per 1000 intubation days. Appreciating the relatively small scale of the study, that a “Hawthorne effect” may have influenced the reporting practices of clinical staff, and that inter-rater variation in the interpretation of data will inevitably lead to variation in reported incidence (Thomas et al., 2011; Stevens et al., 2014), the VAP incidence reported during this period of enhanced surveillance is closer to the incidence reported by colleagues in Scottish and European centers than previous Welsh surveillance program estimates. It seems possible that episodes of VAP have been historically under-reported in Wales.
The most striking finding of our study is the lack of consistent approach to chest radiography, though. Clinicians did not perform chest radiography in nearly half of the cases treated for suspected VARTI, and this is important since chest radiography is used to define VAP for purposes of clinical therapy as well as for surveillance. Antibiotic stewardship principles indicate that the minimum effective duration of antibiotic therapy should be used (Kollef and Micek, 2012). If ventilator-associated tracheobronchitis (VAT) is to be treated with antibiotic therapy—and recent evidence seems to suggest that this may prevent progression to VAP and improve clinical outcomes (Nseir et al., 2005, 2008, 2014; Shahin et al., 2013; Martin-Loeches et al., 2015)—clinical resolution may occur earlier with treatment of VAT than with VAP (Martin-Loeches et al., 2015). The distinction between episodes of VARTI with and without radiographic evidence of consolidation (i.e., between episodes of VAP and VAT) is therefore likely to be important clinically.
However, for surveillance purposes (according to HELIS), chest radiography features are essential for defining an episode of VAP. Given the variability in chest x-ray performance, it is perhaps unsurprising that HELICS-defined episodes of VAP represented less than one-third of cases of the total actually treated for VARTIs in our study. Subjectivity and inter-observer variability with respect to chest radiography interpretation have been highlighted as a potential contribution to differences in reported VAP rates in the US (Klompas, 2012) but we are unaware of previous reports which describe the non-performance of chest radiography as being such a potentially important confounder of surveillance data.
In the US, attempts to focus on “hard” objective data and to exclude more subjective elements (including chest radiography; Klompas, 2010) recently led to complete revision of ICU surveillance methodology and the introduction of the concept of a “Ventilator-Associated Event,” defined on the basis of a deterioration in oxygenation as a “Ventilator-Associated Complication” or “VAC” (Magill et al., 2013). An episode of VAC correlates with poorer clinical outcome (Klompas et al., 2011), but a number of authors have observed that such episodes represent a heterogeneous group of pulmonary conditions which include VAP but also atelectasis, pulmonary edema, and thrombo-embolism (Lilly et al., 2014; Stoeppel et al., 2014; Bouadma et al., 2015; Chang et al., 2015). In our cohort, only a very small number of cases of VAC were identified. Mortality was high in the group of patients with VAC but we found no overlap with our VARTI cohort. Deterioration in oxygenation does not appear to be a frequent reason for commencing antibiotic therapy in our patients. Furthermore, in ICUs which predominantly use paper-based rather than electronic patient records, daily collection of data for VAC analysis was reported by our data collectors to be rather difficult and time-consuming.
We calculated a modified CPIS when antibiotics were commenced for suspected respiratory tract infection as an index of the probability of pneumonia. A mCPIS of six or greater was significantly more likely to occur with HELICS-defined episodes of VAP and for other episodes of RTI. However, as chest x-ray features contribute up to two points to this score, and are also an essential criterion for HELICS-confirmed episode, this is an expected association. Without reducing the variability with which chest radiography is performed, CPIS is unlikely to provide a reliable tool for our surveillance program.
Initiation of antibiotic therapy for suspected VARTI was associated with a significant increase in duration of mechanical ventilation and ICU stay. Among this group of patients were those with HELICS-defined episodes of VAP, for whom longer durations of ventilation and ICU stay are consistent with previous work (Safdar et al., 2005; Bekaert et al., 2011; Melsen et al., 2013; Muscedere et al., 2013). However, among this group were also cases in which there was positive respiratory microbiology and two or more diagnostic signs or symptoms of pneumonia, but in whom chest radiography had not been performed. Not having met established HELICS definitions, we have referred to these as “PN0” episodes (Tables 6, 9, 10). Our present limited data suggests that these patients also have relatively prolonged duration of mechanical ventilation and ICU stay and suggests to us that the application of HELICS surveillance definition is failing to detect episodes of VARTI with appreciable morbidity.
Our study has a number of limitations. Firstly, data collection was restricted to two 14-day periods. Infectious episodes occurring outside the primary data collection period may have been missed, and as a result we may have under-estimated the number of infections occurring on a per patient basis, rather than per intubation days. Despite this, our estimate of VARTI (11.3%) correlates very closely with estimates from a previous UK antimicrobial point prevalence study (12%; Coello et al., 2011). Secondly, with the exception of the VAE data during the latter phase of the study, data collection was triggered by initiation of antibiotic therapy. Significant respiratory tract infection could have been present, but our ability to capture an episode relied on the clinical team initiating treatment. However, using the initiation of antibiotics to trigger ICU surveillance data capture has previously been described (Kaiser et al., 2014) and is recognized in the VAE surveillance methodology as an unambiguous factor within the definition of an “I-VAC.” A major strength of our study is that all acute ICUs in Wales contributed and we believe our findings accurately reflect current intensive care practice.
Conclusions
Our data suggest that established measurements of VAP rates in Wales have unfortunately under-estimated the true incidence of VAP. The higher rate suggested by our enhanced surveillance study is more in keeping with rates published for comparable geographical regions. However, even this higher rate may underestimate the true incidence given the marked variability in performance of chest x-ray at time of diagnosis of respiratory tract infection. The absence of radiographic data is a limitation to a surveillance mechanism which uses the HELICS definition of VAP. This is a novel finding and a concern which may be relevant outside our country.
Ensuring a mechanism for identifying the wider burden of VARTI in Wales while enabling us to contribute to a Europe-wide surveillance programme will be a challenge, particularly in the context of current diagnostic practices. The use of mCPIS for surveillance is hampered by inconsistent radiographic performance and our study indicates that mCPIS could not reliably replace the HELICS definition of VAP. Our results also confirm that adopting the CSC NHSN VAE surveillance methodology in Wales may not provide a solution. The low degree of agreement between episodes of clinician-perceived respiratory tract infection and episodes of VAC among our patient population—as well as logistical problems of data collection in “paper based” ICUs—raises doubt over the suitability of this methodology in Wales at present. However, a sensible step may be the further characterization of episodes of VARTI—diagnosed according to clinical and microbiological features, but without the benefit of radiographic data. This approach including the “PN0” definition is being piloted in the Welsh ICUs and the validity of this approach should be subject to further collaborative study.
WICSARG and WHAIP Members
On behalf of the Welsh Intensive Care Society Audit and Research Group (WICSARG) and Welsh Healthcare-Associated Infection Programme (WHAIP): Ceri Battle, Ceri Brown, Edward Curtis, Eloise Dawe, Campbell Edmonson, Peter Havalda, Maria Hobrok, Alison Ingham, Sylvia Ireland, Karen James, Chris Littler, Michael Martin, Nick Mason, Anthony Osborne, Igor Otahal, Ilona Schmidt, Richard Self, Chris Subbe, Chris Thorpe, Piroska Toth-Tarsoly.
Author Contributions
RP, WH, SH, and TS co-designed the study, coordinated data collection, performed data analysis, and contributed to this draft. HR and GS co-designed the study, coordinated data collection, and contributed to this draft. All authors have agreed to be considered accountable for this work and approve the submitted draft.
Conflict of Interest Statement
The authors declare that the research was conducted in the absence of any commercial or financial relationships that could be construed as a potential conflict of interest.
Acknowledgments
We thank members of WICSARG and WHAIP for their involvement as well as other clinical and administrative colleagues in participating centers.
References
Barbier, F., Andremont, A., Wolff, M., and Bouadma, L. (2013). Hospital-acquired pneumonia and ventilator-associated pneumonia: recent advances in epidemiology and management. Curr. Opin. Pulm. Med. 19, 216–228. doi: 10.1097/MCP.0b013e32835f27be
Bekaert, M., Timsit, J. F., Vansteelandt, S., Depuydt, P., Vésin, A., Garrouste-Orgeas, M., et al. (2011). Attributable mortality of ventilator-associated pneumonia: a reappraisal using causal analysis. Am. J. Respir. Crit. Care Med. 184, 1133–1139. doi: 10.1164/rccm.201105-0867OC
Blot, S., Koulenti, D., Dimopoulos, G., Martin, C., Komnos, A., Krueger, W. A., et al. (2014). Prevalence, risk factors, and mortality for ventilator-associated pneumonia in middle-aged, old, and very old critically ill patients*. Crit. Care Med. 42, 601–609. doi: 10.1097/01.ccm.0000435665.07446.50
Bouadma, L., Sonneville, R., Garrouste-Orgeas, M., Darmon, M., Souweine, B., Voiriot, G., et al. (2015). Ventilator-associated events: prevalence, outcome, and relationship with ventilator-associated pneumonia. Crit. Care Med. 43, 1798–1806. doi: 10.1097/CCM.0000000000001091
Burgmann, H., Hiesmayr, J. M., Savey, A., Bauer, P., Metnitz, B., and Metnitz, P. G. H. (2010). Impact of nosocomial infections on clinical outcome and resource consumption in critically ill patients. Intensive Care Med. 36, 1597–1601. doi: 10.1007/s00134-010-1941-2
Chang, H.-C., Chen, C.-M., Kung, S.-C., Wang, C.-M., Liu, W.-L., and Lai, C.-C. (2015). Differences between novel and conventional surveillance paradigms of ventilator-associated pneumonia. Am. J. Infect. Control 43, 133–136. doi: 10.1016/j.ajic.2014.10.029
Chastre, J., and Fagon, J.-Y. (2002). Ventilator-associated pneumonia. Am. J. Respir. Crit. Care Med. 165, 867–903. doi: 10.1164/ajrccm.165.7.2105078
Coello, R., Brannigan, E., Lawson, W., Wickens, H., and Holmes, A. (2011). Prevalence of healthcare device-associated infection using point prevalence surveys of antimicrobial prescribing and existing electronic data. J. Hosp. Infect. 78, 264–268. doi: 10.1016/j.jhin.2011.01.028
ECDC (2008). European Center for Disease Control and Prevention: Annual Epidemiological Report on Communicable Diseases in Europe.
Fartoukh, M., Maître, B., Honoré, S., Cerf, C., Zahar, J. R., and Brun-Buisson, C. (2003). Diagnosing pneumonia during mechanical ventilation: the clinical pulmonary infection score revisited. Am. J. Respir. Crit. Care Med. 168, 173–179. doi: 10.1164/rccm.200212-1449OC
HELICS (2004). Hospital in Europe Link for Infection Control through Surveillance (HELICS): Surveillance of Nosocomial Infections in Intensive Care Units, Protocol Version 6.1.2004.
HPS/SICSAG (2013). Health Protection Scotland/ Scottish Intensive Care Society Audit Group: Surveillance of Healthcare Associated Infections in Scottish Intensive Care Units. Annual Report of Data from January–December 2012.
Kaiser, A. M., de Jong, E., Evelein-Brugman, S. F., Peppink, J. M., Vandenbroucke-Grauls, C. M., and Girbes, A. R. (2014). Development of trigger-based semi-automated surveillance of ventilator-associated pneumonia and central line-associated bloodstream infections in a Dutch intensive care. Ann. Intensive Care 4:40. doi: 10.1186/s13613-014-0040-x
Klompas, M. (2010). Interobserver variability in ventilator-associated pneumonia surveillance. Am. J. Infect. Control 38, 237–239. doi: 10.1016/j.ajic.2009.10.003
Klompas, M. (2012). Is a ventilator-associated pneumonia rate of zero really possible? Curr. Opin. Infect. Dis. 25, 176–182. doi: 10.1097/QCO.0b013e3283502437
Klompas, M., Khan, Y., Kleinman, K., Evans, R. S., Lloyd, J. F., Stevenson, K., et al. (2011). Multicenter evaluation of a novel surveillance paradigm for complications of mechanical ventilation. PLoS ONE 6:e18062. doi: 10.1371/journal.pone.0018062
Kohlenberg, A., Schwab, F., Behnke, M., Geffers, C., and Gastmeier, P. (2010). Pneumonia associated with invasive and noninvasive ventilation: an analysis of the German nosocomial infection surveillance system database. Intensive Care Med. 36, 971–978. doi: 10.1007/s00134-010-1863-z
Kollef, M. H., and Micek, S. T. (2012). Antimicrobial stewardship programs: mandatory for all ICUs. Crit. Care 16, 179. doi: 10.1186/cc11853
Lilly, C. M., Landry, K. E., Sood, R. N., Dunnington, C. H., Ellison, R. T. III., Bagley, P. H., et al. (2014). Prevalence and test characteristics of national health safety network ventilator-associated events. Crit. Care Med. 42, 2019–2028. doi: 10.1097/CCM.0000000000000396
Magill, S. S., Klompas, M., Balk, R., Burns, S. M., Deutschman, C. S., Diekema, D., et al. (2013). Developing a new, national approach to surveillance for ventilator-associated events. Crit. Care Med. 41, 2467–2475. doi: 10.1097/CCM.0b013e3182a262db
Martin-Loeches, I., Povoa, P., Rodríguez, A., Curcio, D., Suarez, D., Mira, J. P., et al. (2015). Incidence and prognosis of ventilator-associated tracheobronchitis (TAVeM): a multicentre, prospective, observational study. Lancet Respir. Med. 3, 859–868. doi: 10.1016/S2213-2600(15)00326-4
Melsen, W. G., Rovers, M. M., Groenwold, R. H., Bergmans, D. C., Camus, C., Bauer, T. T., et al. (2013). Attributable mortality of ventilator-associated pneumonia: a meta-analysis of individual patient data from randomised prevention studies. Lancet Infect. Dis. 13, 665–671. doi: 10.1016/S1473-3099(13)70081-1
Muscedere, J., Sinuff, T., Heyland, D. K., Dodek, P. M., Keenan, S. P., Wood, G., et al. (2013). The clinical impact and preventability of ventilator-associated conditions in critically ill patients who are mechanically ventilated. Chest 144, 1453–1460. doi: 10.1378/chest.13-0853
Nseir, S., Di Pompeo, C., Soubrier, S., Lenci, H., Delour, P., Onimus, T., et al. (2005). Effect of ventilator-associated tracheobronchitis on outcome in patients without chronic respiratory failure: a case-control study. Crit. Care 9, R238–R245. doi: 10.1186/cc3508
Nseir, S., Favory, R., Jozefowicz, E., Decamps, F., Dewavrin, F., Brunin, G., et al. (2008). Antimicrobial treatment for ventilator-associated tracheobronchitis: a randomized, controlled, multicenter study. Crit. Care 12, R62. doi: 10.1186/cc6890
Nseir, S., Martin-Loeches, I., Makris, D., Jaillette, E., Karvouniaris, M., Valles, J., et al. (2014). Impact of appropriate antimicrobial treatment on transition from ventilator-associated tracheobronchitis to ventilator-associated pneumonia. Crit. Care 18, R129. doi: 10.1186/cc13940
Richards, M. J., Edwards, J. R., Culver, D. H., and Gaynes, R. P. (2000). Nosocomial infections in combined medical-surgical intensive care units in the United States. Infect. Control Hosp. Epidemiol. 21, 510–515. doi: 10.1086/501795
Safdar, N., Dezfulian, C., Collard, H. R., and Saint, S. (2005). Clinical and economic consequences of ventilator-associated pneumonia: a systematic review. Crit. Care Med. 33, 2184–2193. doi: 10.1097/01.CCM.0000181731.53912.D9
Shahin, J., Bielinski, M., Guichon, C., Flemming, C., and Land Kristof, A. S. (2013). Suspected ventilator-associated respiratory infection in severely ill patients: a prospective observational study. Crit. Care 17:R251. doi: 10.1186/cc13077
Stevens, J. P., Kachniarz, B., Wright, S. B., Gillis, J., Talmor, D., Clardy, P., et al. (2014). When policy gets it right: variability in U.S. Hospitals' diagnosis of ventilator-associated pneumonia*. Crit. Care Med. 42, 497–503. doi: 10.1097/ccm.0b013e3182a66903
Stoeppel, C. M., Eriksson, E. A., Hawkins, K., Eastman, A., Wolf, S., Minei, J., et al. (2014). Applicability of the National Healthcare Safety Network' s surgical intensive care unit : a 1-year review. J. Trauma Acute Care Surg. 77, 934–937. doi: 10.1097/TA.0000000000000425
Thomas, B. W., Maxwell, R. A., Dart, B. W., Hartmann, E. H., Bates, D. L., Mejia, V. A., et al. (2011). Errors in administrative-reported ventilator-associated pneumonia rates: are never events really so? Am. Surg. 77, 998–1002.
Keywords: ventilator-associated pneumonia, ventilator-associated tracheobronchitis, respiratory tract infection, ventilator-associated event, ventilator-associated complication, surveillance
Citation: Pugh R, Harrison W, Harris S, Roberts H, Scholey G, Szakmany T and the WICSARG Investigators and WHAIP (2016) Is HELICS the Right Way? Lack of Chest Radiography Limits Ventilator-Associated Pneumonia Surveillance in Wales. Front. Microbiol. 7:1271. doi: 10.3389/fmicb.2016.01271
Received: 25 March 2016; Accepted: 02 August 2016;
Published: 18 August 2016.
Edited by:
Tim Eckmanns, Robert Koch Institute, GermanyReviewed by:
Haider Abdul-Lateef Mousa, University of Basrah, IraqParas Jain, Albert Einstein College of Medicine, USA
Copyright © 2016 Pugh, Harrison, Harris, Roberts, Scholey, Szakmany and the WICSARG Investigators and WHAIP. This is an open-access article distributed under the terms of the Creative Commons Attribution License (CC BY). The use, distribution or reproduction in other forums is permitted, provided the original author(s) or licensor are credited and that the original publication in this journal is cited, in accordance with accepted academic practice. No use, distribution or reproduction is permitted which does not comply with these terms.
*Correspondence: Richard Pugh, pughr@hotmail.com
†The collaborative authors are listed at the end of the article.