- 1LBE, INRA, Narbonne, France
- 2UR OPAALE, Irstea, Rennes, France
- 3Univ Bretagne Loire, Rennes, France
Despite the development of on-farm anaerobic digestion as a process for making profitable use of animal by-products, factors leading to the inactivation of pathogenic bacteria during storage of digestates remain poorly described. Here, a microcosm approach was used to evaluate the persistence of three pathogenic bacteria (Salmonella enterica Derby, Campylobacter coli and Listeria monocytogenes) in digestates from farms, stored for later land spreading. Nine samples, including raw digestates, liquid fractions of digestate and composted digestates, were inoculated with each pathogen and maintained for 40 days at 24∘C. Concentrations of pathogens were monitored using culture and qPCR methods. The persistence of L. monocytogenes, detected up to 20 days after inoculation, was higher than that of Salmonella Derby, detected for 7–20 days, and of C. coli (not detected after 7 days). In some digestates, the concentration of the pathogens by qPCR assay was several orders of magnitude higher than the concentration of culturable cells, suggesting a potential loss of culturability and induction of Viable but Non-Culturable (VBNC) state. The potential VBNC state which was generally not observed in the same digestate for the three pathogens, occurred more frequently for C. coli and L. monocytogenes than for Salmonella Derby. Composting a digestate reduced the persistence of seeded L. monocytogenes but promoted the maintenance of Salmonella Derby. The effect of NH/NH3 on the culturability of C. coli and Salmonella Derby was also shown. The loss of culturability may be the underlying mechanism for the regrowth of pathogens. We have also demonstrated the importance of using molecular tools to monitor pathogens in environmental samples since culture methods may underestimate cell concentration. Our results underline the importance of considering VBNC cells when evaluating the sanitary effect of an anaerobic digestion process and the persistence of pathogens during the storage of digestates and subsequent land spreading.
Introduction
Anaerobic digestion (AD) is one option for managing organic waste originating from agriculture, industry or wastewater treatment. By converting wastes, AD generates both methane for use as energy and the residual digestate as a fertilizer which appears to be a very good candidate to replace inorganic fertilizers (Tambone et al., 2010). However, the use of digestate as fertilizer inevitably involves the issue of health risks associated with pathogenic microorganisms present in waste of fecal origin (such as manure, slurry or sludge): such pathogens may be spread along with the digestate on agricultural soils (Smith et al., 2004; Goberna et al., 2011; Johansen et al., 2013), with consequent contamination of the food chain. Among them, these pathogens number a variety of viruses, including swine hepatitis E virus, bacteria including Salmonella sp., Listeria monocytogenes, Mycobacterium paratuberculosis, thermotolerant Campylobacter and parasites including Cryptosporidium parvum and Giardia sp. (Avery et al., 2012). Among bacteria, the most studied pathogens belong to the genera Salmonella, Campylobacter and Listeria. According to the report of the European Food Safety Authority (EFSA, 2015), the numbers of human listeriosis further increase since 2008 (increase of 16% from 2013 to 2014). Although the number of cases is relatively low, the death rate is higher than other foodborne diseases. Campylobacteriosis was the most commonly reported zoonosis with an increase in confirmed human cases in the European Union since 2008 (increase of 10% from 2013 to 2014). Salmonellosis cases increase for the first time since 2008. From 2008 to 2014, salmonellosis decreased by 44%, mainly due to the successful Salmonella control programs.
The behavior of pathogens during AD has been studied by several authors (Smith et al., 2005; Wagner et al., 2009; Avery et al., 2014; Fu et al., 2015). Temperature appears to be the main factor affecting pathogen viability during AD (Wagner et al., 2009). Thermophilic AD reduces the quantity of pathogens more efficiently than mesophilic AD which only reduces the concentration of pathogens by 1–2 log units (Avery et al., 2012). Due to their insufficient elimination during the AD process (Pietronave et al., 2004), pathogens may be still present in low concentrations in the digestate. Many confined-livestock farms store their waste or final products for several months prior to use as fertilizer (Himathongkham et al., 1999). However, very little information about the viability of pathogens during the storage of digestates has been reported. Furthermore, some studies have shown potential regrowth of coliforms and pathogens during the storage of compost (Sidhu et al., 2001; Pietronave et al., 2004), biowaste (Gibbs et al., 1995; Bagge et al., 2005) or digestate (Fu et al., 2015; Orzi et al., 2015). Sidhu et al. (2001) did not observe a decline of Salmonella sp. when the compost was stored. These authors highlighted a strong negative correlation between the Salmonella inactivation rate and the maturity of the compost. They suggested that long-term storage could facilitate the regrowth of pathogens due to a decline in the antagonist effect of indigenous microorganisms.
Another issue concerns the loss of culturability of bacteria during waste treatment. In fact, several studies have demonstrated that pathogens can enter into a Viable but Non-Culturable (VBNC) state in biosolids or animal by-products after various treatments, including the AD process (Higgins et al., 2007; Qi et al., 2008; Viau and Peccia, 2009; Fu et al., 2014; Desneux et al., 2016). Fu et al. (2015) demonstrated the induction of a VBNC state of Salmonella sp. and Shigella sp. during the thermophilic AD of biosolids but followed during cake storage with a subsequent regaining of culturability. Exposure to various stresses can induce the VBNC state and VBNC cells may be reactivated back to a culturable state under suitable stimuli (Li et al., 2014). The presence of VBNC bacteria in the environment constitutes a health risk for humans since the cells may retain their potential to infect (Besnard et al., 2001).
In order to improve risk assessment of animal by-products when re-used in agriculture, we investigated the persistence during the storage of digestates of three pathogenic bacteria found in animal feces: Salmonella Derby (Huong et al., 2014), Campylobacter coli (Schweitzer et al., 2011; Egger et al., 2012) and Listeria monocytogenes (Desneux et al., 2016). The persistence of pathogens in nine digestates from farms practicing land spreading (raw digestates, liquid fractions of digestate after separation and dried or composted solid fractions of digestate) was studied via microcosm assays lasting 40 days. More especially, the goals were to: (i) test different digestates for inactivation of pathogenic bacteria and to identify the characteristics of digestates affecting their persistence; and (ii) study the occurrence of a VBNC state in digestates using both the quantitative PCR (qPCR) assay and culture methods.
Materials and Methods
Sampling of Digestates
Nine digestates (named Dig1–9) originating from wet mesophilic AD were sampled from seven agricultural sites in France, gathering different types of digestates that can be spread on soil: three raw digestates (Dig1–3), three liquid fractions of digestate (Dig4–6) obtained after digestate separation, one composted and dried fraction of digestate (Dig7) and two composted solid fractions of digestate (Dig8–9). Digestate designation and their origin are presented in Table 1. To evaluate the variability due to sampling and the analytical variability, two independent samples were collected for digestates Dig2 and Dig4, on the same day following the same sampling protocol (named R1 and R2). All the collected samples (about 50 kg each) were homogenized for further analyses.
Physical and Chemical Characterization of Digestates
Representative aliquots of digestate (10–50 g for composted digestates and 10–50 mL for raw digestates and liquid fractions) were used in carrying out all the analytical tests. For each samples, the moisture content was determined by drying the sample at 105°C for 48 h. The volatile matter (VM) was measured by calcination of this dried sample at 550°C for 3 h. The pH was measured in accordance with the AFNOR standard NF EN 15933: for solid digestates (<60% moisture content, Dig7–9), in the aqueous extract obtained after adding distilled water (1:5, w/v), or directly for liquid digestates (>88% moisture content, Dig1–6). Total organic carbon (TOC) was analyzed using a liquid-phase carbon analyzer (TOC-V CHS/CSN, Shimadzu Corporation). Total nitrogen was determined using the Total Kjeldahl Nitrogen (TKN) method (Rodier, 1975). Ammonia (NH) concentration was determined by high-performance liquid chromatography (HPLC) using the manufacturer’s protocol (ICS-3000 Ion Chromatography System). Total Phosphorus (P2O5) content was measured by colorimetric determination, in accordance with Fiske and Subbarow (1925). Total Potassium (K2O) content was measured in accordance with the AFNOR standard NF EN ISO 11885. TOC, NH, TKN, P2O5 were analyzed in fresh samples whereas K2O was analyzed in dried samples. All the analyses were performed in triplicate and showed variability below 10%. All analyses were expressed as g of raw matter.
Bacterial Strains and Culture Conditions
The bacterial strains used were L. monocytogenes strain CIP110870 and Salmonella enterica subsp. enterica serovar Derby strain CIP110872 (Salmonella Derby), both originating from pig manure, and a gentamicin-resistant Campylobacter coli strain UB14-031/1 of clinical origin (provided by the French National Reference Center for Campylobacter and Helicobacter, Bordeaux). To facilitate L. monocytogenes and Salmonella enumeration on plate agar, two rifampicin-resistant (Rifr) mutants of L. monocytogenes and Salmonella Derby were generated from the two selected strains, as described in Lung et al. (2001). The Rifr strains were cultivated at 37°C in nutritive broth (ThermoFisher Scientific, France) supplemented with 3 g L-1 of glucose. C. coli was cultivated under microaerobic conditions at 42°C in nutrient Broth No.2 (ThermoFisher Scientific), supplemented with 5% of horse blood (v:v) and Campylobacter growth supplements (ThermoFisher Scientific). The plates were placed in a microaerobic environment using CampyGen (ThermoFisher Scientific) which produces 6–15% O2 and 3–9% CO2 (Macé et al., 2015). The broths inoculated with L. monocytogenes, Salmonella Derby and C. coli were incubated for, respectively 18, 17, and 28 h, to reach the beginning of the stationary phase.
Microcosm Assay Inoculated with Live Cells
Each 120 mL of the pathogen culture suspension in its stationary phase was centrifuged at 5000 g for 10 min. The pellet was washed in 30 mL of 0.8% of sterilized NaCl solution. Following a second step of centrifugation, the pellet was resuspended in 10–12 mL of NaCl solution prior to inoculation in the digestates. Microcosms, established in 500 mL flasks with plastic screw cap lids, contained 125 mL or 100 g of digestate. Each microcosm was inoculated with 1 mL of L. monocytogenes, Salmonella Derby or C. coli culture suspension to reach an initial concentration of 106–107 CFU mL-1 or g-1. The inoculated flasks were stored in the dark at 24°C for 40 days. There were two replicates per digestate. According to the nature of the digestate (liquid or solid), the microcosms were prepared in accordance with the two following protocols: for liquid digestates (Dig1–6), a volume of 125 mL was transferred into a 500 mL flask, and for solid digestates (Dig7–9), 100 g were transferred into five 500 mL-flasks and at each sampling one flask was sacrificed by adding 300 mL of peptone water. At each sampling time, aliquots for cultural (1 mL) and qPCR (500 μL) quantification were collected immediately after inoculation of the strains (t0) and then after 3, 7, 20, and 40 days of incubation. Aliquots for qPCR were stored at -20°C before analysis.
Control Assay Inoculated with Killed Cells
A second series of microcosms was prepared in the same way with the bacterial suspensions in a NaCl solution after the cells were lysed by ultrasound for 8 min at 360 W and then heated to 85°C and maintained here for 10 min. The absence of culturable cells was checked by inoculation onto a culture medium as described above. Before inoculation in the flasks, the suspensions of killed cells were stored at -20°C. Each microcosm was performed in duplicate. The microcosms inoculated with killed cells were prepared and incubated in the same way as for the previous microcosms. The concentration of DNA of each pathogen was measured by qPCR at the beginning of the experiment (t0) and thereafter 1, 2, 5, 7, 20, and 40 days of incubation.
Enumeration of Pathogens and Endogenous Escherichia coli by Culture Method
Serial 10-fold dilutions were prepared in peptone water (ThermoFisher Scientific). A volume of 0.1 mL of each dilution was surface plated on the specific medium. When the level of bacteria was expected to be low, 0.1 mL of the digestate was surface plated on one agar plate. E. coli was enumerated on Tryptone Bile X-Glucuronide (TBX, ThermoFisher Scientific) agar plates. The plates were incubated at 44°C for 24 h. After incubation, blue colonies (glucuronidase positives) were counted. L. monocytogenes and Salmonella Derby strains were enumerated on Palcam agar (ThermoFisher Scientific) and on XLD agar (ThermoFisher Scientific), respectively, supplemented with 50 mg L-1 of cycloheximide and 100 mg L-1 of rifampicin (Sigma Aldrich, France). The plates were then incubated 48 h at 37°C. For C. coli, the cells were enumerated on Karmali-Agar (ThermoFisher Scientific), supplemented with Karmali selective supplement and 32 mg L-1 of gentamicin, then incubated for 72 h at 42°C under microaerobic conditions using CampyGen (ThermoFisher Scientific). Results are expressed as CFU mL-1 or g-1. The limit of detection was 25 CFU mL-1 or g-1.
Quantification of Pathogens by qPCR
DNA extraction was performed on 500 μL of digestate using the FastDNA SPIN Kit for soil, in accordance with the manufacturer’s protocol (MP Bio, Solon, OH, USA). The quantity and quality of the DNA were checked by spectrophotometry (Infinite NanoQuant M200, Tecan, Austria). All the DNA was stored at -20°C until further use.
Reactions took place using the concentrations of oligomers and the PCR programs described in Table 2. Reactions were prepared in 25 μL, using 96-well optical reaction plates (Applied Biosystems, Foster City, CA, USA). The following components were added: 12.5 μL of 2X SsoAdvanced universal supermix (Bio-Rad, Hercules, CA, USA), 5 μL of diluted DNA extracts, the forward primer, reverse primer and the TaqManTM probe labeled with FAM as a reporter and TAMRA as a quencher. Amplification reactions were run on the CFX96 Real-Time system (Bio-Rad). All tests were performed in triplicate. Data analysis was carried out with the Bio-Rad CFX Manager software, version 3:0. The cycle threshold (Ct), which corresponds to the cycle number at which the reaction became exponential, was then compared to a standard curve and used to calculate the number of target per PCR.
One standard curve was generated for each assay, using plasmid containing the target gene (MWG Operon Eurofin, Ebersberg, Germany). The standard curves designed by the Bio-Rad CFX Manager software were then generated by the amplification of serial 10-fold dilutions of the plasmid solutions in sterilized water. Slopes of standard curves were between -3.1 and -3.5, corresponding to an efficiency of the PCR reaction between 90 and 110%. The regression coefficient values (R2) were always above 0.95.
No background signal for any of the qPCR systems was detected in the control which was obtained by replacing DNA by sterilized water. To validate the absence of PCR inhibition, internal control tests were performed. One microliter of 20X PrimePCR positive-control probe assay (labeled with HEX as a reporter and lowa Black as a quencher) (Bio-Rad) was added to wells containing 5 μL of DNA extracts as well as to wells containing DNA free water. All were done in triplicate. The Ct obtained with and without DNA extracts were compared and a different Ct (±0.5) indicated an inhibition of the PCR reaction.
The level of background signal and the efficiency of the PCR were checked for each assay. The PCR results were then converted to target gene copy numbers per g of raw matter. Average values and standard deviation were obtained using at least three values. The detection limit was determined for each sample since the quantity of sample used for DNA extraction varied from one sample to another. The detection limits were between 1.9 × 103 and 1.0 × 104 copies g-1.
Analysis of Pathogen Quantifications by qPCR and Culture Method
Quantification of DNA or enumeration of CFU recovered from each sampling day and for each pathogen was normalized by dividing the mean values by the mean obtained at day 0 (C/C0). We performed a Log transformation of the ratio Log(C/C0) and plotted these values against the sampling day for each pathogen and each digestate. Two parameters describing the persistence of pathogen were calculated: the decimal reduction at 7 days and the inactivation rate (d-1) corresponding to the slope of the inactivation curve and expressed in Log.
The number of culturable cells was estimated by plate count and the number of total cells was estimated by qPCR. Given that the target genes amplified by qPCR (hlyA, invA and glyA) are present as a single copy in the genome for the three pathogenic bacteria (Table 2), results can be expressed as a genome equivalent. The difference between the concentration of total cells measured by qPCR (CqPCR) and the concentration of culturable cells (Cculture), expressed in Log, (Log CqPCR – Log Cculture) was calculated at 7 days for C. coli and Salmonella Derby and at 20 days for L. monocytogenes (before the initial lag phase), with both CqPCR and Cculture, expressed as genome equivalent mL-1 or g-1. The presence of potential VBNC cells was assumed when (Log CqPCR – Log Cculture) was significant, taking into account the coefficient of variation (CV) of each quantification method. Moreover, killed cells control was used to confirm that the qPCR signal of microcosms inoculated with killed cells decreased at a higher rate than the qPCR signal of microcosms inoculated with live cells. Potential VBNC state was validated if the qPCR signal from killed cells was significantly below the qPCR signal of live cells.
Statistical Analysis
The mean, standard deviation and CV of physical and chemical analysis and pathogen quantification in Log at 7 days were calculated for the two digestates sampled twice (Dig2 and Dig4). A Pearson correlation matrix was performed in order to test for correlations between the physical and chemical characteristics of a digestate (pH, moisture content, VM, TOC, NTK, NH, K2O and P2O5) and the persistence of pathogens (decimal reduction and inactivation rate). Statistical analyses were carried out using the Rcmdr package of R-Software (R Foundation for Statistical Computing, Vienna, Austria).
Results
Comparison of the Persistence of the Three Pathogenic Bacteria in Digestate Microcosms
The nine digestates spiked with cells of L. monocytogenes, Salmonella Derby and C. coli were monitored for 40 days by both the culture method and qPCR assay. The variability of microbial, physical and chemical analyses was tested on two independent samples R1 and R2, collected from different digestors (Dig2 and Dig4). Taking into account the two samples and the two replicates per sample, the CV was below 12% (Supplementary Table S1) indicating data’s good reproducibility. Furthermore, the inactivation curves show good reproducibility between duplicates (Supplementary Figure S1). The behavior of each pathogen in each digestate is presented in Figure 1. Generally, concentrations declined from t0 exponentially. In some cases, the inactivation curve appears to have two parts, the first with a lag phase during which concentrations remained stable until day 3 or 7, followed by a second phase of sharp decline. Two parameters were chosen to characterize all inactivation curves: decimal reduction at 7 days and the inactivation rate (Supplementary Table S2). This profile with the lag phase was observed for samples Dig3–4 of C. coli, and for half the samples of L. monocytogenes. For these digestates, the inactivation rate was calculated after the lag phase.
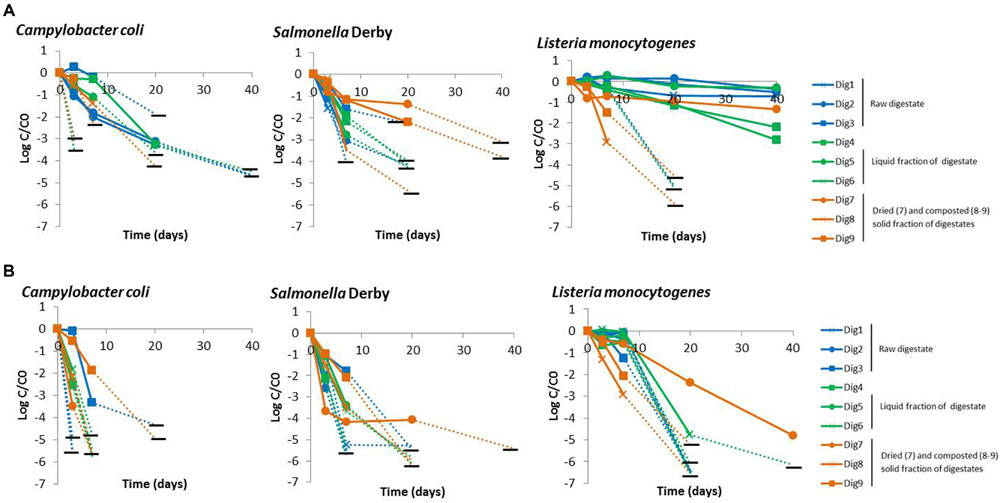
FIGURE 1. Persistence of C. coli, Salmonella Derby and L. monocytogenes measured by qPCR assay (A) and the culture method (B). Only one microcosm duplicate is presented. Blue, green and orange curves represent respectively, raw digestates, liquid fraction of digestate and composted solid fractions of digestate. Horizontal black lines represent the detection limits. The last point connected by a dotted line represents the first point below the detection limit.
The three pathogens survived differently in the digestates (Figure 1). Overall, L. monocytogenes showed the highest persistence. It was detected by qPCR in all digestates after 20 days and was still present after 40 days in 5 of the 9 digestates (Dig1–2, Dig4–5, and Dig7). Furthermore, the concentration measured by qPCR in 3 of the 9 digestates (Dig1–2 and Dig5) did not decrease over time. A high persistence during the first days of storage was also observed using the culture method with no decay at 7 days for five digestates. In contrast, C. coli was not detected by the culture method in most digestates (6 of the 9 digestates) after 7 days and the detection limit was also rapidly reached for quantification using qPCR. Salmonella Derby showed an intermediate persistence. It was quantified during 7–20 days and was still detected by qPCR up to 7 days in most of the digestates (7 of the 9 digestates).
Potential Induction of VBNC State
To test whether the culturability was lost faster than viability and if the induction of VBNC state was occurring, decimal reduction by qPCR and the culture method were compared for each pathogen after 7 days of incubation (Figure 2). As shown in the boxplots of Figure 2, high variability was observed, both by culture or qPCR, for C. coli with values ranging between 1.7 and 5.8 for culture and between 0.1 and 3.5 for qPCR. For Salmonella Derby, concentration varied from 1.7 to 5.8 for culture and from 0.7 to 4.1 for qPCR. The variability was lower for L. monocytogenes: from 0 to 3.3 for culture and from -0.3 to 2 for qPCR. Different behavior was observed depending on the digestate and the pathogen.
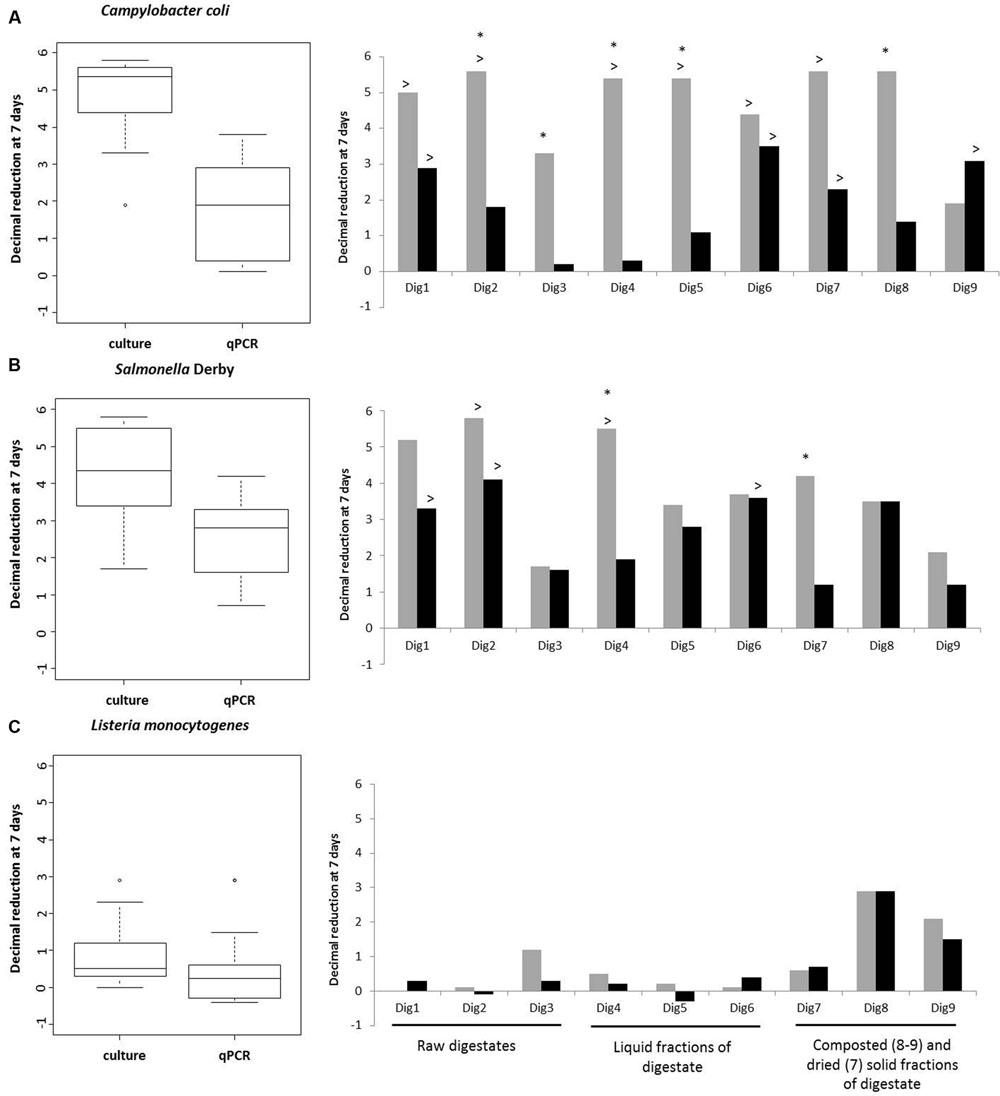
FIGURE 2. Distribution of the decimal reductions among digestates in left panel and decimal reduction for each digestate in right panel, for C. coli (A), Salmonella Derby (B) and L. monocytogenes (C), measured by qPCR assay (black bars) and the culture method (gray bars). The symbol > significates that the decimal reduction is calculated using the detection limit. The symbol ∗ shows digestates with a potential induced VBNC state.
For C. coli, very different inactivation curves were obtained depending on the digestate. The detection limit of both methods was reached in three digestates (Dig1 and Dig6–7). A reduction of more than 5 Log of culturable cells associated to a relatively low reduction of gene copies (0.1–3.8 Log) was observed in four digestates (Dig2, Dig4–5 and Dig8), suggesting a potential induction of a VBNC state. In two digestates (Dig3 and Dig9), the culturable cells were less affected by the 7 days of storage in so far as their concentrations decreased by only 1.9 (for Dig9) to 3.3 Log (for Dig3). It is noteworthy that the decimal reduction measured by qPCR was 0.2 in Dig3 but reached more than 3 Log in Dig9. These results suggested a drastic effect of the digestate on C. coli persistence.
The behavior of Salmonella Derby also differed from one digestate to another. The highest decimal reductions of culturable cells (more than 5 Log) were observed in digestates Dig1-2 and Dig4. In some digestates, the decimal reduction observed by both techniques was similar (around 2 Log for Dig3 and around 3 Log for Dig5–6 and Dig8). In others, the difference between the culture and qPCR methods could not be calculated because the concentration was below the detection limit (for Dig1-2). For Dig4 and Dig9, the difference was above 1.9 Log. It is noteworthy that this difference increased with time, and at 20 days, significant differences were also observed for Dig9 (Figure 1).
For L. monocytogenes, the decimal reduction after 7 days was less than 0.5 Log in 7 of the 9 digestates. However, two composted digestates (Dig8–9) led to 2 decimal reductions of the culturable cells and 1 (for Dig9) to 2 (Dig8) decimal reductions of gene copies. After the lag phase, the concentrations of culturable cells of L. monocytogenes decreased by at least 5 Log, regardless of the digestate (Figure 3). Two types of persistence behaviors as measured by qPCR were observed. In the first type of behavior, the qPCR signal either remained stable (Dig1–2 and Dig5) or decreased slightly (Dig4 and Dig7) (Figure 3A), suggesting that L. monocytogenes remained viable, with no drastic loss of viable cells, over 40 days but lost its culturability after 7 days, possibly entering into a VBNC state. In contrast, in four digestates (Dig3, Dig6, Dig8–9) the inactivation curves given by qPCR and the culture method were close to each other throughout the experiment (Figure 3B).
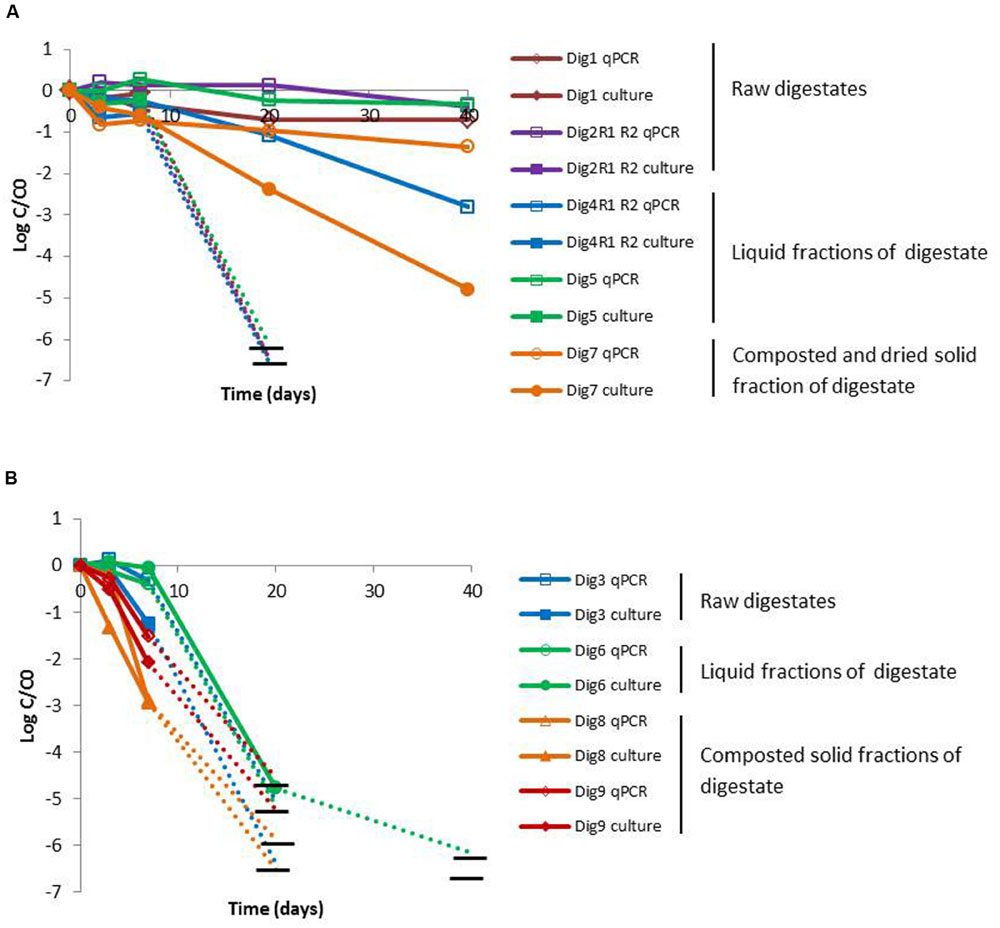
FIGURE 3. Persistence of L. monocytogenes according to the difference between the quantification of cells by qPCR assay and the culture method: significant difference between the two methods ( > 1 log) after 20 days of storage (A) and no difference (B). Empty and full symbols represent quantification by qPCR assay and the culture method, respectively. Horizontal black lines represent the detection limit. The last point connected by a dotted line represents the first point below the detection limit.
Overall, a potential VBNC state was observed for the three pathogenic bacteria but it was not systematic. The loss of culturability of C. coli and Salmonella Derby, which appeared in the first days of incubation, appeared later for L. monocytogenes. Although the loss of culturability depended on the digestates, the type of digestate (raw, liquid fraction or composted) alone cannot explained the loss of culturability. According to the coefficient of variation (CV) of the culture and PCR methods, the difference in decimal reduction above 1 Log was considered as indicator of a potential VBNC state. Potential loss of culturability was thus observed in five digestates (Dig2–5 and Dig8) in the case of C. coli, in three digestates (Dig4, Dig7, and Dig9) for Salmonella Derby and in five digestates (Dig1–2, Dig4–5, and Dig7) for L. monocytogenes.
To check if the higher values of qPCR could be due to the presence of no-degraded DNA from non-viable cells, the decrease of the qPCR signal obtained from killed cells of the three pathogens spiked in the nine digestates was determined and compared to the decrease obtained with live cells. Table 3 presents the concentrations of pathogens measured in microcosms spiked with live cells by using qPCR assays and the culture method and in microcosms spiked with killed cells by using qPCR. A potential VBNC state was validated by a qPCR signal from killed cells significantly lower than the qPCR signal of live cells. The level of significance was given by the CV of each qPCR signal: 0.5 Log for C. coli, 1 Log for Salmonella Derby and 0.2 Log for L. monocytogenes (Supplementary Table S1). A potential VBNC state was validated for most of the digestates inoculated with killed cells. The validation could not be made for the composted and dried sample Dig7 which presented a very low decrease in the qPCR signal from killed cells that could be explained by the very low moisture content which prevents microbial and DNAse activity. Apart from the two microcosms performed with this digestate, a potential VBNC state was demonstrated for all the remaining 11 microcosms spiked with killed cells: 5 of the 9 digestates for C. coli, 2 for Salmonella Derby and 4 for L. monocytogenes.
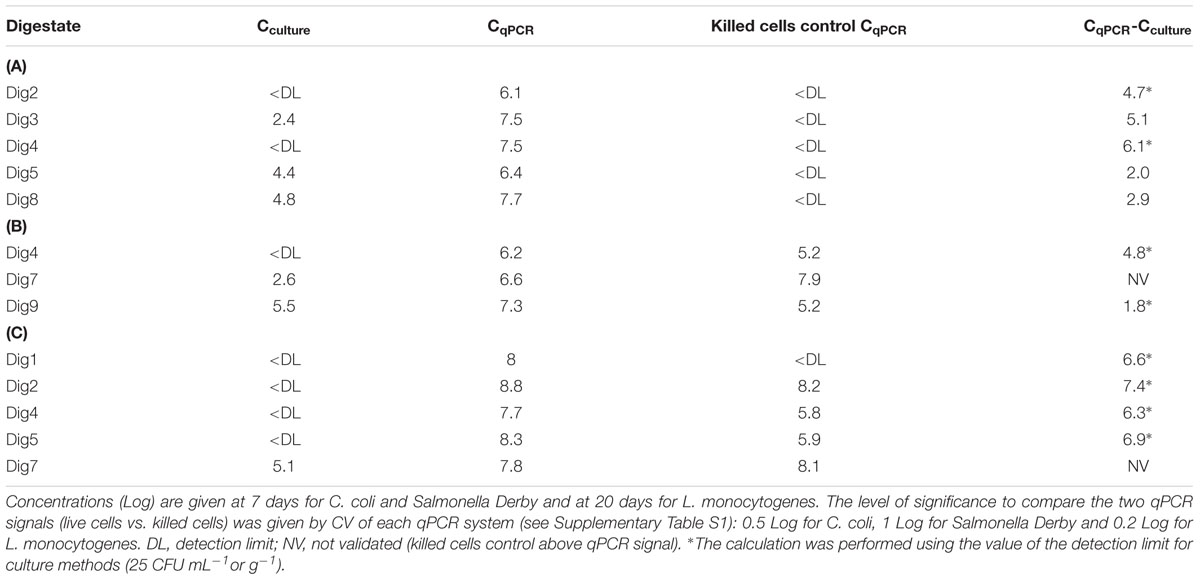
TABLE 3. Validation of the degradation of DNA from non-viable cells for digestates presenting significant differences between qPCR and culture values (>1 Log) for C. coli (A), Salmonella Derby (B) and L. monocytogenes (C).
Correlation between Physical and Chemical Characteristics of Digestates and Persistence of Pathogens and the Potential Induction of VBNC State
Table 4 summarizes the physical and chemical characteristics of the digestates. The pH of all the digestates was alkaline, in a range of 7.3–8.8. The moisture content ranged between 25.4 and 59.1% for composted or dried solid fractions of the digestate (Dig7–9) and between 88.6 and 95.8% for raw digestates and liquid fractions of digestate (Dig1–6). These ranges of pH and moisture content were theoretically favorable to microbial activity, except for Dig7 which presented the lowest moisture content (25.4%). In addition to the moisture content, composted and dried digestates presented higher concentrations of VM (19.2–45.7%), TOC (94.8–235.8 gC kg-1), TKN (8.4–32.5 gN kg-1), K2O (7.3–12.8 g kg-1) and P2O5 (16.2–88.8 g kg-1) than liquid fractions and raw digestates. Finally, the NH content was between 0.1 and 3.3 g kg-1 for all digestates, except for Dig4 which contained a particularly high concentration of NH (7.9 g kg-1).
Pearson’s r correlations were calculated between physical and chemical parameters and the persistence of pathogens (inactivation rate and decimal reduction at 7 days) (Table 5). The persistence of the three pathogens did not correlate to the same parameters. The decimal reduction of C. coli was only significantly correlated to NH content (positively for culturable cells and negatively for gene copies), suggesting that NH /NH3 could have a negative effect on culturability but not on the viability of the bacteria. The decimal reduction of culturable cells of Salmonella Derby was also correlated only to NH content. However, significant correlation was found between the inactivation rate of the gene copies of this pathogen and several parameters: positively correlated to the moisture content and negatively to the nutrient content (TOC, TKN, P2O5) and VM. Composted digestates were characterized by low moisture content, high nutrient contents and VM. The correlation observed for Salmonella Derby thus indicated a higher persistence in composted digestates than in raw digestates and in liquid fractions. The decimal reduction at 7 days or the inactivation rate of L. monocytogenes measured by both methods was positively correlated to the nutrient content and VM and negatively correlated to the moisture content, thus indicating a lower persistence of this bacterium in composted digestates than in raw digestates and the liquid fraction of digestates.
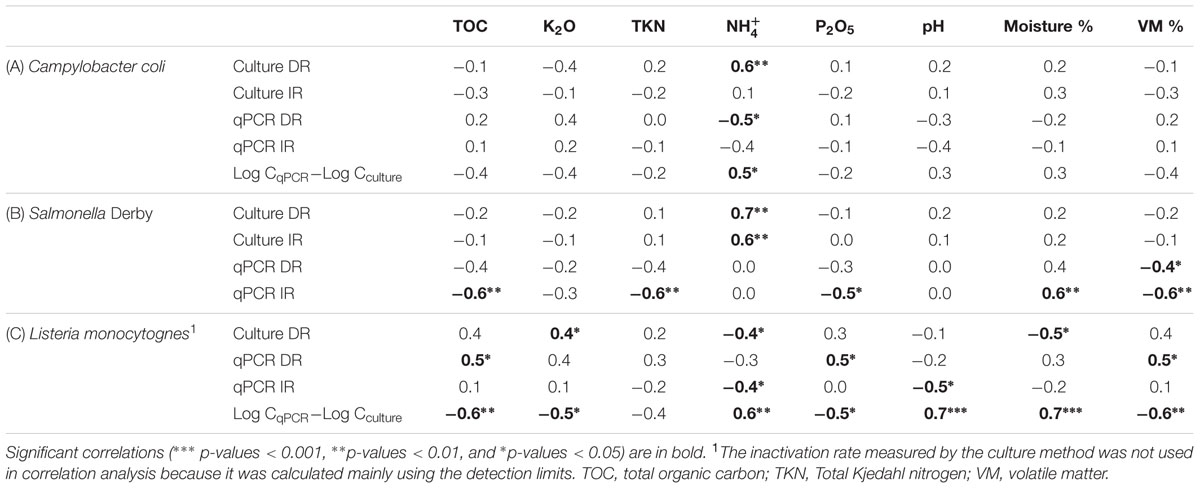
TABLE 5. Pearson’s r correlation analysis between physical and chemical characteristics of digestates and pathogen inactivation rate (IR) and decimal reduction at 7 days (DR) calculated by both qPCR or the culture method and the difference in concentrations between qPCR assay and the culture method (data from Table 3) for C. coli (A), Salmonella Derby (B) and L. monocytogenes (C).
For C. coli and L. monocytogenes, correlations were also investigated between the potential VBNC concentration (estimated by the difference of concentrations between qPCR and culture), and the physical and chemical characteristics of the digestates (Table 5). This was not investigated for Salmonella Derby since potential induction of a VBNC state was shown for only two digestates. The difference in L. monocytogenes concentration between the two methods was positively correlated to NH content. This difference was also positively correlated to the pH and the moisture content but negatively correlated to the nutrient content and VM. This result indicated a loss of culturability in digestates characterized by high pH and moisture content and low nutrient content and VM (characterizing raw digestates and liquid fractions). Indeed, where the pH and moisture content were lowest, i.e., in composted digestates, in one raw digestate and in one liquid fraction, no loss of culturability was observed.
Discussion
The fate of three pathogenic bacteria found in animal feces was investigated in microcosms containing nine digestates sampled on farms (raw digestates, liquid fractions and composted solid fractions). As indicated by the low level of endogenous E. coli (Supplementary Table S3), we hypothesized that the concentration of the three pathogens in the digestates was low. Exogenous cells of each target pathogen were thus inoculated into the digestates.
Different kinds of behavior were observed for the three pathogens. Considering quantification by the culture method and qPCR assay, L. monocytogenes survived much longer than Salmonella Derby and C. coli. Using culture, Nicholson et al. (2005) reported that L. monocytogenes in stored slurry and sewage survived longer (up to 6 months) than E. coli O157, Salmonella and Campylobacter (up to 3 months). The higher persistence of L. monocytogenes had previously been reported by Hutchison et al. (2005) in studying livestock waste samples. In this paper, L. monocytogenes was detected up to 40 days in 6 of the 9 digestates using qPCR assays which is in agreement with the results of Desneux et al. (2016) who using qPCR, detected up to 63 days two strains of L. monocytogenes inoculated in pig manures and stored at 20°C. L. monocytogenes is known to be particularly resistant to environmental factors (Avery et al., 2014) and to grow over a wide range of temperature and pH conditions (Bille and Doyle, 1991). Its genome contains an extensive repertoire of genes encoding transport proteins and regulators, a characteristic of the genome of ubiquitous bacteria (Vivant et al., 2013). Interestingly, in the present study, culturable L. monocytogenes was detected after 40 days only in one digestate (Dig7), highlighting a relatively low persistence of the culturable cells in digestates whereas Desneux et al. (2016) detected a longer persistence of culturable L. monocytogenes in manure. This suggests that digestates may represent a less favorable environment than manure for the survival of L. monocytogenes. C. coli were rapidly reduced in the nine digestates, especially when it was quantified by culture methods. Some studies have shown that Campylobacter sp. is a fragile bacterium and appears to be rapidly inactivated in animal by-products (Nicholson et al., 2005; Sinton et al., 2007). But these results were based on enumeration using culture methods, not taking into account a potential VBNC state. In our analysis, the reduction of C. coli was lower when it was measured by qPCR than by the culture method (Figure 2). This result is in accordance with previous studies that showed good persistence of Campylobacter sp. in digested sludge or compost when molecular methods were used (Horan et al., 2004; Wagner et al., 2009; Inglis et al., 2010). McAllister and Topp (2014) reported a long persistence of C. coli, above 112 days, in manure samples. Inglis et al. (2010) demonstrated that C. coli can survived over 10 months in manure compost. As observed for L. monocytogenes, our results seemed to indicate a low persistence of C. coli in digestates compared to manure samples. It is noteworthy that the temperature of storage of the microcosms (24°C) could be unfavorable for the persistence of C. coli as reported by Bui et al. (2011). These authors investigated the effect of temperature on the survival of C. coli in stored swine manure and demonstrated that this bacterium survived only 6 days at 22°C. The persistence of Salmonella Derby was intermediate between those of L. monocytogenes and C. coli.
Several abiotic factors can affect the persistence of pathogenic bacteria. The characteristics of manure samples, including temperature, moisture content and particle size, may contribute to the persistence of pathogens (Ahmed et al., 1995; Garcia et al., 2010; Diao et al., 2015). In this study, the temperature was maintained at 24°C and we focused on such characteristics of the digestates as moisture content, nutrient content, VM and pH. Such physical and chemical characteristics measured in digestates differentiated the three composted or dried digestates (Dig7–9) from the others (raw digestates and liquid fractions). In fact, composted digestates in contrast to raw digestates and liquid fractions were characterized by lower moisture content and higher nutrient contents and VM. The persistence of Salmonella Derby was positively correlated with the nutrient content and VM and negatively correlated with the moisture content (Table 5), indicating a better persistence of Salmonella Derby in composted digestates. Results of Pietronave et al. (2004) confirmed that, under proper storage conditions, finished compost can still provide bio-available nutrients to support Salmonella sp. growth. Lemunier et al. (2005) demonstrated the long-term survival of Salmonella Enteridis in stabilized compost compared to less mature ones. In contrast to Salmonella Derby, we observed a negative correlation between the persistence of L. monocytogenes and the pH, nutrient content and VM and a positive correlation with the moisture content (Table 5), indicating a lower persistence of L. monocytogenes in composted digestates. Klein et al. (2011) had previously reported a higher inactivation rate of L. monocytogenes in manure compost than in a manure stockpile. However, a lower persistence was also observed in non-composted digestates (raw digestate Dig3 and liquid fraction Dig6), suggesting that the composting treatment did not alone explain the behavior of L. monocytogenes. It is noteworthy that Dig3 and Dig6 were intermediate between the two composted digestates and the other five digestates in terms of nutrient content, pH range and VM. The different behavior between Salmonella Derby and L. monocytogenes in composts has also been observed by Lemunier et al. (2005). The persistence of seeded Salmonella Enteritidis was demonstrated in all composts irrespective of their maturity, whereas the persistence of L. monocytogenes was associated to the degree of maturity. Lemunier et al. (2005) explained this result by an increase in the antagonistic effects of the indigenous microflora. Paniel et al. (2010) suggested also that in compost samples, the indigenous microflora appeared to play a significant role in suppression of L. monocytogenes, Salmonella Infantis and Enterococcus faecalis. Some authors have shown that indigenous microflora may limit the persistence of pathogens by limiting availability of nutrients and by indigenous antimicrobial activity (Sidhu et al., 2001; Erickson et al., 2014b). Furthermore, it has been reported that composts contain fungi and Actinomycetes which produce substances inhibiting bacterial pathogens (Franke-Whittle et al., 2014). The higher resistance of Salmonella to antimicrobial compounds in comparison to L. monocytogenes (Patel et al., 2014), may explain that, in our study, the persistence of L. monocytogenes was more impacted by the compost than Salmonella. A significant correlation with the persistence of C. coli was found only with the ammonia (NH /NH3) content. NH, which was measured in this study, was in equilibrium with NH3, a toxic compound. Depending on the conditions of AD, including temperature and pH, the equilibrium between NH and NH3 can be modified. Given the pH values of the nine digestates (ranging between 7.0 and 8.8), the NH3 form is well represented (1–26%, data not shown) suggesting that its toxic effect is not negligible. In digestate samples, Orzi et al. (2015) reported a reduction of pathogen concentrations because of NH3 production. Interestingly, in our study, NH /NH3 content negatively correlated with the persistence of C. coli when measured by the culture method, though not by qPCR, suggesting that NH /NH3 may have a negative effect only on the culturability of C. coli but not on its viability.
Our study clearly demonstrated that composting and NH /NH3 content affected the persistence of seeded pathogens. However, the differences between non-composted digestates may be explained by other factors than those measured here. Among such physical and chemical properties, volatile acids were not tested in our study, but this factor has been shown to affect the persistence of L. monocytogenes in manure-based compost mixtures of animal origin (Erickson et al., 2014a). Characteristics of environmental matrices can thus contribute to the persistence of pathogens, as suggested by Diao et al. (2015). In particular, the effect on the persistence of pathogenic bacteria of biotic factors such as microbial diversity and activity still remains to be explored in treated waste (Sidhu et al., 2001; Paniel et al., 2010; Klein et al., 2011; Orzi et al., 2015). It is noteworthy that the best persistence of Salmonella derby and L. monocytogenes estimated by culture was observed for Dig7 which had lowest moisture content (25%), unfavorable to microbial activity (Tate and Terry, 1980).
The persistence of pathogens in environmental matrices (such as soil, manure or compost) has been estimated in the literature mainly by culture methods (Nicholson et al., 2005; Paniel et al., 2010; Locatelli et al., 2013). In environmental conditions, bacteria are submitted to various stresses and lose their culturability, what has been called a Viable but Non-culturable (VBNC) state (Oliver, 2010; Li et al., 2014). To circumvent their loss of culturability, several authors have used DNA-based quantification (qPCR) to study bacteria in environmental samples such as soil, wastewater, sludge, manure or compost (Wéry et al., 2008; Klein et al., 2011; Fu et al., 2015). One of the main criticisms of the use of qPCR for quantifying live bacteria in environmental samples is that it may overestimate the concentration of viable bacteria since extracellular DNA or DNA from non-viable cells can also produce a PCR signal (Nocker and Camper, 2006). However, it was also assumed that extracellular DNA from non-viable cells in manure harboring an abundant and active microflora is quickly degraded and recycled by the microbial populations (Lebuhn et al., 2005; Klein et al., 2011). Furthermore, Fu et al. (2015) demonstrated that the persistence of Salmonella sp. and Shigella sp. during AD or sludge storage was very similar as indicated by qPCR or reverse transcription qPCR which are both indicators of bacterial cell viability. Desneux et al. (2016) reported that in pig manure or storage lagoons inoculated with L. monocytogenes, the quantification of DNA by qPCR gave similar results to those obtained by qPCR combined with propidium monoazide treatment, indicating that the concentration of viable cells was not overestimated by qPCR. In our study, for the three pathogenic species, the decimal reduction was lower in qPCR assays than by the culture method in several digestates, suggesting potential induction of a VBNC state. For all digestates presenting comparatively higher values, whether obtained by qPCR or culture methods, there was at least one of the qPCR signals (i.e., gene copies of C. coli, L. monocytogenes or Salmonella Derby) which decreased very fast (>2 Log in 7 days) confirming that DNA from non-viable cells was rapidly degraded. Additionally, experiments in microcosms inoculated with killed cells of the three pathogens were carried out and the qPCR values were compared with those obtained in microcosms inoculated with live cells. In all but one digestate, the qPCR signal obtained from DNA extracted from microcosms inoculated with killed cells was below those obtained from DNA extracted from microcosms inoculated with live cells. This validated that DNA released by non-viable cells was rapidly degraded. In Dig7, the PCR signals from killed cells was not below those from live cells (Table 3), suggesting that DNA from killed cells was not degraded but persisted over time. Dig7 was significantly drier (25% moisture content) than the other digestates (56–97% moisture content). Such low moisture content could thus explain the persistence of DNA observed in the microcosm inoculated with killed cells. In fact, low humidity is known to affect DNAse activity (Blum et al., 1997). At the end, except for Dig7, we assume that the concentrations of gene copies measured by qPCR in the microcosms inoculated with live bacteria represented viable cells. The potential presence of VBNC of C. coli, Salmonella Derby and L. monocytogenes in matrices such as digestates is not surprising in so far as studies have reported a VBNC state for all the three bacteria in environmental matrices (Oliver, 2010). Using ethidium monoazide and the qPCR technique, Inglis et al. (2010) quantified VBNC of C. jejuni in bovine manure compost and Desneux et al. (2016) showed a VBNC state of L. monocytogenes in pig manure.
According to some authors, the VBNC state is a survival strategy adopted by many bacteria in response to adverse environmental conditions (Li et al., 2014). Bacteria in a VBNC state lose their culturability on microbial media but retain their cellular integrity and such viability as respiration, enzyme activity and gene expression. The induction of a VBNC state has been already reported in some studies dealing with the treatment of organic waste (Bui et al., 2011; Klein et al., 2011; Leblanc-Maridor et al., 2011; Desneux et al., 2016) and, more specifically, after an AD process (Qi et al., 2008; Viau and Peccia, 2009). Regrowth observed during the storage of digestate, compost or organic waste could be due to a regain of pathogen culturability under favorable conditions after a state of a VBNC induced by an AD process. A recent study by Fu et al. (2015) demonstrated that Salmonella sp. and Shigella sp. did not actually regrow (no increase of DNA content or cell division) but were reactivated from a VBNC state to a culturable state. Bacteria are capable of regain their culturability under favorable conditions and thus, in the case of pathogenic bacteria pose potential health risks. Hence, culture techniques appear to be ill-suited to evaluate (i) the real efficiency of biological treatment such as AD; and (ii) the biological safety of a digestate intended for spreading on agricultural land. In our experiments, the occurrence of a potential VBNC state was more frequent for C. coli (5 of the 9 digestats) and L. monocytogenes (4 of the 9 digestates) than for Salmonella Derby (Table 3). Although VBNC cells of Salmonella have been evidenced in manured soil using RT-PCR (Garcia et al., 2010), the majority of digestates (7 of 9) showed no loss of culturability of Salmonella Derby. On the other hand, in the case of C. coli, 5 of the 9 digestates showed a potential VBNC state and the underestimation by the culture method was of several orders of magnitude after only 7 days. Health risks linked to Campylobacter sp. may thus significantly be underestimated when evaluated only with culture-dependent techniques. Interestingly, for L. monocytogenes, a potential VBNC state was detected only after 7 days of storage, suggesting that a VBNC state can be acquired only after a relatively long period. Finally, the fact that the pathogenic bacteria did not lose their culturability in all digestates showed that the entry into a VBNC state does not depend only on the species but also on environmental conditions (Desneux et al., 2016). The temperature used (24°C) which is unfavorable to the survival of C. coli (Bui et al., 2011) could be a factor of induction of VBNC state and explain why VBNC state was prevalent for this pathogen in our experimental conditions. In our study, we observed that each of the three pathogenic bacteria did not enter into a VBNC state in the same digestates, showing that factors inducing loss of culturability are different between the three species: in the case of L. monocytogenes, a potential VBNC state was induced in raw digestates and liquid fractions (except Dig3 and 6) but not in composted digestates. Also, a positive correlation between NH /NH3 content and the potential inducing of a VBNC was found in C. coli and L. monocytogenes microcosms, suggesting that the negative effect of NH /NH3 on bacterial culturability could represent a stress leading to a VBNC state. The link between the nature of the digestates and the induction of VBNC state needs further investigation.
Conclusion
This study has demonstrated that L. monocytogenes showed greater persistence in digestates than both C. coli and Salmonella Derby, probably due to its ubiquitous nature. For three pathogens, a potential VBNC state was induced in digestates, especially for C. coli and L. monocytogenes. There is a concern for the possibility of regrowth by these two pathogens during the storage of digestate. We have demonstrated the importance of the use of molecular tools such as qPCR for monitoring pathogenic bacteria in environmental samples since culture methods may underestimate bacterial cell concentration. The potential regrowth observed in some final products used for land spreading could be due to a regain to culturability of bacteria fallen into a VBNC state. This occurrence of VBNC cells caused difficulty in evaluating the real efficacy from a sanitary perspective of an AD process and, also, the biological safety of digestates. The persistence of pathogens and the potential induction of a VBNC state were digestate-dependent. We were able to demonstrate: (i) the effect of composting a digestate, which reduced the persistence of seeded L. monocytogenes but promoted the maintenance of Salmonella Derby; and (ii) the effect of NH /NH3 on the culturability of C. coli and Salmonella Derby. There is a need to investigate other factors, including the influence of biological factors such as the activity and diversity of endogenous microflora and biochemical factors such as the bio-availability of organic matter. In fact, improved knowledge of such parameters may enable us to understand microbial regrowth and/or recontamination as observed in stored digestates in order to improve the management of products from AD processes, thus enhancing their profitable use in agriculture.
Author Contributions
GM, A-MP, and NW designed laboratory work. GM, CD, and A-MP sampled digestates. GM and CZ performed laboratory work. AC participated in part of the laboratory work and the sampling. GM, A-MP, and NW analyzed data. GM drafted the manuscript. A-MP, NW, and CD finalized the manuscript. All the authors read and approved the final manuscript.
Funding
This research was supported financially by the Agence de l’Environnement et de la Maitrise de l’Energie (ADEME) (agreement number: 1306C0032).
Conflict of Interest Statement
The authors declare that the research was conducted in the absence of any commercial or financial relationships that could be construed as a potential conflict of interest.
Acknowledgment
We thank Eric Latrille for advice regarding appropriate methods of statistical analysis.
Supplementary Material
The Supplementary Material for this article can be found online at: http://journal.frontiersin.org/article/10.3389/fmicb.2016.01469
References
Ahmed, U., Mundt, C. C., and Coakley, S. M. (1995). Host-pathogen relationship of geographically diverse iolates of Spetoria tritici and wheat cultivars. Plant Path. 44, 838–847. doi: 10.1111/j.1365-3059.1995.tb02743.x
Avery, L. M., Anchang, K. Y., Tumwesige, V., Strachan, N., and Goude, P. J. (2014). Potential for pathogen reduction in anaerobic digestion and biogas generation in Sub-Saharan Africa. Biomass Bioenergy 70, 112–124. doi: 10.1016/j.biombioe.2014.01.053
Avery, L. M., Booth, P., Campbell, C., Tompkins, D., and Hough, R. L. (2012). Prevalence and survival of potential pathogens in source-segregated green waste compost. Sci. Total Environ. 431, 128–138. doi: 10.1016/j.scitotenv.2012.05.020
Bagge, E., Sahlström, L., and Albihn, A. (2005). The effect of hygienic treatment on the microbial flora of biowaste at biogas plants. Water Res. 39, 4879–4886. doi: 10.1016/j.watres.2005.03.016
Besnard, V., Federighi, M., Declerq, E., Jugiau, F., and Cappelier, J.-M. (2001). Environmental and physico-chemical factors induce VBNC state in Listeria monocytogenes. Vet. Res. 33, 359–370. doi: 10.1051/vetres:2002022
Bille, J., and Doyle, M. P. (1991). Listeria and Erysipelothrix. Manual of Clinical Microbiology, 5th Edn. Washington, DC: American Society for Microbiology.
Blum, S. E., Lorenz, M. G., and Wackernagel, W. (1997). Mechanism of retarded DNA degradation and prokaryotic origin of DNases in nonsterile soils. Syst. Appl. Microbiol. 20, 513–521. doi: 10.1016/S0723-2020(97)80021-5
Bui, X. T., Wolff, A., Madsen, M., and Bang, D. D. (2011). Fate and survival of Campylobacter coli in swine manure at various temperatures. Front. Microbiol. 2:262. doi: 10.3389/fmicb.2011.00262
Desneux, J., Biscuit, A., Picard, S., and Pourcher, A.-M. (2016). Fate of viable but non-culturable listeria monocytogenes in pig manure microcosms. Front. Microbiol. 7:245. doi: 10.3389/fmicb.2016.00245
Diao, J., Chen, Z., Gong, C., and Jiang, X. (2015). Factors affecting pathogen survival in finished dairy compost with different particle sizes under greenhouse conditions. Foodborne Pathog. Dis. 12, 749–758. doi: 10.1089/fpd.2014.1912
EFSA (2015). The European Union summary report on trends and sources of zoonoses. Zoonotic agents and food-borne outbreaks in 2014. EFSA J. 13:4329. doi: 10.2903/j.efsa.2015.4329
Egger, R., Korczak, B. M., Niederer, L., Overesch, G., and Kuhnert, P. (2012). Genotypes and antibiotic resistance of Campylobacter coli in fattening pigs. Vet. Microbiol. 155, 272–278. doi: 10.1016/j.vetmic.2011.08.012
Erickson, M. C., Liao, J., Ma, L., Jiang, X., and Doyle, M. P. (2014a). Thermal and nonthermal factors affecting survival of Salmonella and Listeria monocytogenes in animal manure–based compost mixtures. J. Food Prot. 77, 1512–1518. doi: 10.4315/0362-028X.JFP-14-111
Erickson, M. C., Habteselassie, M. Y., Liao, J., Webb, C. C., Mantripragada, V., Davey, L. E., et al. (2014b). Examination of factors for use as potential predictors of human enteric pathogen survival in soil. J. Appl. Microbiol. 116, 335–349. doi: 10.1111/jam.12373
Fiske, C. H., and Subbarow, Y. (1925). The colorimetric determination of phosphorus. J. Biol. Chem. 66, 375–400.
Franke-Whittle, I. H., Confalonieri, A., Insam, H., Schlegelmilch, M., and Körner, I. (2014). Changes in the microbial communities during co-composting of digestates. Waste Manage. 34, 632–641. doi: 10.1016/j.wasman.2013.12.009
Fu, B., Jiang, Q., Liu, H., and Liu, H. (2014). Occurrence and reactivation of viable but non-culturable E. coli in sewage sludge after mesophilic and thermophilic anaerobic digestion. Biotechnol. Lett. 36, 273–279. doi: 10.1007/s10529-013-1361-9
Fu, B., Jiang, Q., Liu, H. B., and Liu, H. (2015). Quantification of viable but nonculturable Salmonella spp. and Shigella spp. during sludge anaerobic digestion and their reactivation during cake storage. J. Appl. Microbiol. 119, 1138–1147. doi: 10.1111/jam.12887
Garcia, R., Baelum, J., Fredslund, L., Santorum, P., and Jacobsen, C. S. (2010). Influence of temperature and predation on survival of Salmonella enterica serovar Typhimurium and expression of invA in soil and manure-amended soil. Appl. Environ. Microbiol. 76, 5025–5031. doi: 10.1128/AEM.00628-10
Gibbs, R., Hu, C. J., Ho, G. E., Philips, P. A., and Unkovich, I. (1995). Pathogen die-off in stored wastewater sludge. Water Sci. Technol. 31, 91–95. doi: 10.1016/0273-1223(95)00247-K
Goberna, M., Podmirseg, S. M., Waldhuber, S., Knapp, B. A., Garcia, C., and Insam, H. (2011). Pathogenic bacteria and mineral N in soils following the land spreading of biogas digestates and fresh manure. Appl. Soil Ecol. 49, 18–25. doi: 10.1016/j.apsoil.2011.07.007
Higgins, M. J., Chen, Y. C., Murthy, S. N., Hendrickson, D., Farrel, J., and Schafer, P. (2007). Reactivation and growth of non-culturable indicator bacteria in anaerobically digested biosolids after centrifuge dewatering. Water Res. 41, 665–673. doi: 10.1016/j.watres.2006.09.017
Himathongkham, S., Bahari, S., Riemann, H., and Cliver, D. (1999). Survival of Escherichia coli O157: H7 and Salmonella typhimurium in cow manure and cow manure slurry. FEMS Microbiol. Lett. 178, 251–257. doi: 10.1111/j.1574-6968.1999.tb08684.x
Hoorfar, J., Ahrens, P., and Radstrom, P. (2000). Automated 5’ nuclease PCR assay for identification of Salmonella enterica. J. Clin. Microbiol. 38, 3429–3435.
Horan, N. J., Fletcher, L., Betmal, S. M., Wilks, S., and Keevil, C. W. (2004). Die-off of enteric bacterial pathogens during mesophilic anaerobic digestion. Water Res. 38, 1113–1120. doi: 10.1016/j.watres.2003.12.004
Huong, L. Q., Forslund, A., Madsen, H., and Dalsgaard, A. (2014). Survival of Salmonella spp. and fecal indicator bacteria in Vietnamese biogas digesters receiving pig slurry. Int. J. Hyg Environ. Health 217, 785–795. doi: 10.1016/j.ijheh.2014.04.004
Hutchison, M. L., Walters, L. D., Avery, S. M., Munro, F., and Moore, A. (2005). Analyses of livestock production, waste storage, and pathogen levels and prevalences in farm manures. Appl. Environ. Microbiol. 71, 1231–1236. doi: 10.1128/AEM.71.3.1231-1236.2005
Inglis, G. D., McAllister, T., Larney, F. J., and Topp, E. (2010). Prolonged survival of Campylobacter species in bovine manure compost. Appl. Environ. Microbiol. 76, 1110–1119. doi: 10.1128/AEM.01902-09
Johansen, A., Carter, M. S., Jensen, E. S., Hauggard-Nielsen, H., and Ambus, P. (2013). Effects of digestate from anaerobically digested cattle slurry and plant materials on soil microbial community and emission of CO2 and N2O. Appl. Soil Ecol. 63, 36–44. doi: 10.1016/j.apsoil.2012.09.003
Klein, M., Brown, L., Ashbolt, N. J., Stuetz, R. M., and Roser, D. J. (2011). Inactivation of indicators and pathogens in cattle feedlot manures and compost as determined by molecular and culture assays. FEMS Microbiol. Ecol. 77, 200–210. doi: 10.1111/j.1574-6941.2011.01098.x
Leblanc-Maridor, M., Beaudeau, F., Seegers, H., Denis, M., and Belloc, C. (2011). Rapid identification and quantification of Campylobacter coli and Campylobacter jejuni by real-time PCR in pure cultures and in complex samples. BMC Microbiol. 11:113. doi: 10.1186/1471-2180-11-113
Lebuhn, M., Effenberger, M., Garcés, G., Gronauer, A., and Wilderer, P. A. (2005). Hygienization by anaerobic digestion: comparison between evaluation by cultivation and quantitative real-time PCR. Water Sci. Technol. 52, 93–99.
Lemunier, M., Francou, C., Rousseaux, S., Houot, S., Dantigny, P., Piveteau, P., et al. (2005). Long-term survival of pathogenic and sanitation indicator bacteria in experimental biowaste composts. Appl. Environ. Microbiol. 71, 5779–5786. doi: 10.1128/AEM.71.10.5779
Li, D., Tong, T., Zeng, S., Lin, Y., Wu, S., and He, M. (2014). Quantification of viable bacteria in wastewater treatment plants by using propidium monoazide combined with quantitative PCR (PMA-qPCR). J. Environ. Sci. 26, 299–306. doi: 10.1016/S1001-0742(13)60425-8
Locatelli, A., Spor, A., Jolivet, C., Piveteau, P., and Hartmann, A. (2013). Biotic and abiotic soil properties influence survival of Listeria monocytogenes in soil. PLoS ONE 8:e75969. doi: 10.1371/journal.pone.0075969
Lung, A. J., Lin, C.-M., Kim, J. M., Marshall, M. R., Nordstedt, R., Thompson, N. P., et al. (2001). Destruction of Escherichia coli O157:H7 and Salmonella enteritidis in cow manure composting. J. Food Prot. 64, 1309–1314.
Macé, S., Haddad, N., Zagorec, M., and Tresse, O. (2015). Influence of measurement and control of microaerobic gaseous atmospheres in methods for Campylobacter growth studies. Food Microbiol. 52, 169–176. doi: 10.1016/j.fm.2015.07.014
McAllister, T. A., and Topp, E. (2014). Role of livestock in microbiological contamination of water: commonly the blame, but not always the source. Anim. Front. 2, 17–27. doi: 10.2527/af.2012-0039
Nicholson, F., Groves, S. J., and Chambers, B. J. (2005). Pathogen survival during livestock manure storage and following land application. Bioresour. Technol. 96, 135–143. doi: 10.1016/j.biortech.2004.02.030
Nocker, A., and Camper, A. K. (2006). Selective removal of DNA from dead cells of mixed bacterial communities by use of ethidium monoazide. Appl. Environ. Microbiol. 72, 1997–2004. doi: 10.1128/AEM.72.3.1997-2004.2006
Nogva, H. K., Rudi, K., Naterstad, K., Holck, A., and Lillehaug, D. A. G. (2000). Application of 5 J -nuclease PCR for quantitative detection of Listeria monocytogenes in pure cultures. water, skim milk, and unpasteurized whole milk. Appl. Environ. Microbiol. 66, 4266–4271.
Oliver, J. D. (2010). Recent findings on the viable but nonculturable state in pathogenic bacteria. FEMS Microbiol. Rev. 34, 415–425. doi: 10.1111/j.1574-6976.2009.00200.x
Orzi, V., Scaglia, B., Lonati, S., Riva, C., Boccasile, G., Alborali, G. L., et al. (2015). The role of biological processes in reducing both odor impact and pathogen content during mesophilic anaerobic digestion. Sci. Total Environ. 526, 116–126. doi: 10.1016/j.scitotenv.2015.04.038
Paniel, N., Rousseaux, S., Gourland, P., Poitrenaud, M., and Guzzo, J. (2010). Assessment of survival of Listeria monocytogenes. Salmonella infantis and Enterococcus faecalis artificially inoculated into experimental waste or compost. J. Appl. Microbiol. 108, 1797–1809. doi: 10.1111/j.1365-2672.2009.04584.x
Patel, J. D., Parmar, M., Patel, P., Rohit, P., and Taviyad, R. (2014). Dynamism of antimicrobial activity of actinomycetes — a case study from undisturbed microbial niche. Adv. Microbiol. 4, 324–334. doi: 10.4236/aim.2014.46039
Pietronave, S., Fracchia, L., Rinaldi, M., and Martinotti, M. G. (2004). Influence of biotic and abiotic factors on human pathogens in a finished compost. Water Res. 38, 1963–1970. doi: 10.1016/j.watres.2004.01.027
Qi, Y., Dentel, S. K., and Herson, D. S. (2008). Effect of total solids on fecal coliform regrowth in anaerobically digested biosolids. Water Res. 42, 3817–3825. doi: 10.1016/j.watres.2008.06.001
Rodier, J. (1975). L’analyse de L’eau : Eaux Naturelles, Eaux Résiduaires, Eau de Mer. Paris: Dunod.
Schweitzer, N., Dán,Á, Kaszanyitzky, É, Samu, P., Tóth,ÁG., Varga, J., et al. (2011). Molecular epidemiology and antimicrobial susceptibility of Campylobacter jejuni and Campylobacter coli isolates of poultry, swine, and cattle origin collected from slaughterhouses in hungary. J. Food Prot. 74, 905–911. doi: 10.4315/0362-028X.JFP-10-376
Sidhu, J., Gibbs, R. A., Ho, G. E., and Unkovich, I. (2001). The role of indigenous microorganisms in suppression of Salmonella regrowth in composted biosolids. Water Res. 35, 913–920. doi: 10.1016/S0043-1354(00)00352-3
Sinton, L., Hall, C., and Braithwaite, R. (2007). Sunlight inactivation of Campylobacter jejuni and Salmonella enterica, compared with Escherichia coli, in seawater and river water. J. Water Health 5, 357–365. doi: 10.2166/wh.2007.031
Smith, K. E., Stenzel, S., Bender, J. B., Wagstrom, E., Soderlund, D., Leano, F. T., et al. (2004). Outbreaks of enteric infections caused by multiple pathogens associated with calves at a farm day camp. Pediatr. Infect. Dis. J. 23, 1098–1104. doi: 10.1097/01.inf.0000145409.74116.e5
Smith, S. R., Lang, N. L., Cheung, K. H. M., and Spanoudaki, K. (2005). Factors controlling pathogen destruction during anaerobic digestion of biowastes. Waste Manag. 25, 417–425. doi: 10.1016/j.wasman.2005.02.010
Tambone, F., Scaglia, B., D’Imporzano, G., Schievano, A., Orzi, V., Salati, S., et al. (2010). Assessing amendment and fertilizing properties of digestates from anaerobic digestion through a comparative study with digested sludge and compost. Chemosphere 81, 577–583. doi: 10.1016/j.chemosphere.2010.08.034
Tate, R. L., and Terry, R. E. (1980). Variation in microbial activity in histols and its relationship to soil moisture. Appl. Environ. Microbiol. 40, 313–317.
Viau, E., and Peccia, J. (2009). Survey of wastewater indicators and human pathogen genomes in biosolids produced by class A and class B stabilization treatments. Appl. Environ. Microbiol. 75, 164–174. doi: 10.1128/AEM.01331-08
Vivant, A. L., Garmyn, D., Maron, P. A., Nowak, V., and Piveteau, P. (2013). Microbial Diversity and structure are drivers of the biological barrier effect against Listeria monocytogenes in Soil. PLoS ONE 8:e76991. doi: 10.1371/journal.pone.0076991
Wagner, A. O., Malin, C., Gstraunthaler, G., and Illmer, P. (2009). Survival of selected pathogens in diluted sludge of a thermophilic waste treatment plant and in NaCl-solution under aerobic and anaerobic conditions. Waste Manag. 29, 425–429. doi: 10.1016/j.wasman.2008.03.003
Keywords: anaerobic digestion, Listeria monocytogenes, Salmonella, Campylobacter, digestate, persistence, VBNC state
Citation: Maynaud G, Pourcher A-M, Ziebal C, Cuny A, Druilhe C, Steyer J-P and Wéry N (2016) Persistence and Potential Viable but Non-culturable State of Pathogenic Bacteria during Storage of Digestates from Agricultural Biogas Plants. Front. Microbiol. 7:1469. doi: 10.3389/fmicb.2016.01469
Received: 01 July 2016; Accepted: 02 September 2016;
Published: 14 September 2016.
Edited by:
Avelino Alvarez-Ordóñez, Teagasc Food Research Centre, IrelandCopyright © 2016 Maynaud, Pourcher, Ziebal, Cuny, Druilhe, Steyer and Wéry. This is an open-access article distributed under the terms of the Creative Commons Attribution License (CC BY). The use, distribution or reproduction in other forums is permitted, provided the original author(s) or licensor are credited and that the original publication in this journal is cited, in accordance with accepted academic practice. No use, distribution or reproduction is permitted which does not comply with these terms.
*Correspondence: Nathalie Wéry, bmF0aGFsaWUud2VyeUBzdXBhZ3JvLmlucmEuZnI=