- 1Institute of Antibiotics, Huashan Hospital, Fudan University, Shanghai, China
- 2Key Laboratory of Clinical Pharmacology of Antibiotics, Ministry of Health, Shanghai, China
Objectives: Current worldwide spread of carbapenem resistance in Enterobacteriaceae constitutes a critical public health threat. This study aims to investigate how carbapenem resistance is acquired in Enterobacteriaceae in patients during antimicrobial therapy.
Methods: Clinical strains from the same anatomical site of the same patients that converted from carbapenem-susceptible to resistant during antimicrobial therapy and showed identical or similar PFGE patterns were identified. The similarly sized plasmids carried by the susceptible and resistant strains, the latter containing the carbapenemase genes, were sequenced and analyzed.
Results: Paired strains were identified from four patients: three had neurosurgical conditions while the other had acute exacerbation of COPD. Two pairs of Klebsiella pneumoniae (KP1-S/R and KP2-S/R, S and R indicating susceptible and resistant strains, respectively), one pair of Morganella morganii (MM-S/R) and one pair of Enterobacter aerogenes (EA-S/R) were collected. All four carbapenem-resistant strains carried plasmids harboring blaKPC−2. Compared with the similarly sized plasmids in KP1-S and KP2-S, an insertion sequence that includes ISKpn6-like, blaKPC−2 and ISKpn8 was noted in pKP1-R and pKP2-R. Strains MM-R and EA-R had blaKPC−2-carrying plasmids not resembling plasmids in strains MM-S and EA-S suggesting their new acquisition while on therapy.
Conclusions: Enterobacteriaceae can acquire carbapenem resistance during antimicrobial therapy through horizontal transfer of an insertion sequence or plasmid.
Introduction
Carbapenems are the most effective antibiotics for the treatment of refractory Gram-negative bacterial infections. And yet, carbapenem-resistant Enterobacteriaceae (CRE) are increasingly isolated from clinical samples globally (Nordmann et al., 2012). According to the CHINET (a national antimicrobial resistance surveillance project in China), the rates of carbapenem-resistant K. pneumoniae was 2.4% in 2005 and increased to approximately 13.4% in 2014 (Hu et al., 2016). The treatment options for CRE are limited and these infections are associated with poor clinical outcome (Schwaber et al., 2008). Understanding how CRE spreads is crucial for timely implementation of infection control measures.
The most common mechanism of carbapenem resistance in Enterobacteriaceae is the production of plasmid-mediated carbapenemases, and KPCs (Klebsiella pneumoniae carbapenemases) is currently the most prevalent in many countries including China (Tzouvelekis et al., 2012). Horizontal transfer through transposons and integrons may account for the dissemination of carbapenemase genes carried by CRE strains (Munoz-Price and Quinn, 2009). Interspecies transfer of the gene encoding carbapenemase OXA-48 has been demonstrated in the gut using animal models, suggesting that horizontal transfer of carbapenemase genes may be an important mode of spread of CRE (Göttig et al., 2015).
Previously, we have reported the transfer of NDM-1 gene in E. coli in a patient: a clonally related carbapenem-resistant E. coli strain was isolated 1 month later after the isolation of a carbapenem-susceptible E. coli (Ding et al., 2015). A similar observation has also been made with K. pneumoniae in a patient with pneumonia (Elliott et al., 2006). It is also reported that, in some patients with Gram-negative bacterial infections, bacterial strains belonging to same species from the same anatomical site might lose susceptibility to the agents that are being used for treatment (Lee et al., 2011; Solé et al., 2015).
Numerous studies have addressed epidemiology, risk factors and treatment outcome of CRE infections (Tzouvelekis et al., 2012), but relatively little attention has been paid to the genetic process of conversion from carbapenem-susceptible to resistant of a bacterial strain. In this study, we retrospectively surveyed the clinical microbiology data of Enterobacteriaceae isolates and the clinical data of patients in a tertiary hospital in Shanghai, China from January 2011 to December 2012. As a result, four patients who had Enterobacteriaceae strains with identical or similar PFGE patterns and conversion of carbapenem susceptibility from susceptible to resistant during antimicrobial therapy were identified. We revealed that horizontal transfer of an insertion sequence or plasmid carrying blaKPC−2 was responsible for the in vivo development of carbapenem resistance.
Methods
Patients
The study was conducted at Huashan Hospital, Fudan University, a 1300-bed tertiary care hospital in Shanghai, China. The clinical microbiology data of Enterobacteriaceae strains from inpatients between January 2011 and December 2012 were retrospectively reviewed. Two or more strains of identical or similar species isolated from the same anatomical site of a patient were collected if their carbapenem susceptibility converted from susceptible to resistant during antimicrobial therapy. Isolates from the same patients with identical or similar PFGE patterns were then further studied. The clinical data were collected, including general demographics, such as age and sex; the ward to which the patient was assigned after admission; previous use of antimicrobial agents, particularly carbapenems, extended spectrum cephalosporins and fluoroquinolones. This study was approved by the Institutional Review Board of Huashan Hospital and was exempted for obtaining written informed consents from patients (number: KY2014-233). Patient records were anonymized and de-identified prior to analysis.
Clinical Strains and Susceptibility Testing
All the strains were identified by the Vitek 2 automated system (BioMerieux, France). Antimicrobial susceptibility testing was performed by the agar dilution method as recommended by the Clinical and Laboratory Standards Institute (CLSI, 2014). Isolates with nonsusceptibility to either of the two carbapenems (imipenem and meropenem) tested were classified as carbapenem-resistant.
Carbapenemase Phenotypic Detection
Modified Hodge Test (MHT) was used for carbapenemase detection, and the positive result has a clover leaf-like indentation of the E. coli 25922 growing along the test organism growth streak within the disk diffusion zone. The phenotypic detection of carbapenemase was also evaluated with boronic acid disk tests, the test was considered positive for class A carbapenemase production when the diameter of the growth-inhibitory zone around imipenem disk with boronic acid was ≥5 mm larger than that around a disk containing imipenem alone.
PFGE Analysis
PFGE was employed to determine the genetic relationship among the clinical isolates of the same species from the same patients. Genomic DNA was digested with XbaI for 4 h at 37°C. The digested DNAs were then separated in 1% agarose gel using a CHEF-DRIII system (Bio-Rad Laboratories), with a linear pulse time ramped from 2 to 70 s for 24 h at 14°C and constant voltage of 6 V/cm. Similarity between PFGE patterns was determined according to the criteria proposed by Tenover et al. and with Bionumerics software using the Dice coefficient. Strains sharing the restriction patterns of less than or equal to three-band differences (Tenover et al., 1995) and similarity coefficients >80% were considered to be closely related strain type (Harris et al., 2007; Wu et al., 2014).
PCR Detection of β-Lactamase Genes
PCR detection of β-lactamase genes encoding carbapenemases (blaAIM, blaBIC, blaGIM, blaIMP, blaKPC, blaNDM, blaOXA−48, blaSIM,blaSPM, and blaVIM) (Poirel et al., 2011), extended-spectrum β-lactamases (blaCTX−M) (Woodford et al., 2006), plasmid-mediated AmpC β-lactamase (blaACC, blaCIT, blaDHA, blaEBC, blaFOX, and blaMOX) (Pérez-Pérez and Hanson, 2002) was performed as previously described. PCR amplicons were sequenced and compared with sequences available in the GenBank database using BLAST searches.
Plasmid Analysis
Plasmid DNA was extracted using a Plasmid Midi Kit (QIAGEN, Germany) according to the manufacturer's instructions. The DNA was electrophoresed in 0.8% agarose for 1.5 h. Three reference strains carrying different sizes of plasmids, with several plasmids in E. coli V517 (size, 54, 5.6, 5.1, 3.9, 3.0, 2.7, and 2.1 kb), R1 (92 kb) in E. coli J53 and R27 (182 kb) in E. coli J53. The plasmids of clinical strains were introduced by electroporation into E. coli DH10B using a Gene Pulser II system (Bio-Rad Laboratories). Putative transformants were selected through PCR screening for blaKPC from the colonies growing on Mueller-Hinton agar plates containing ampicillin (50 μg/mL). Transformants harboring a single plasmid with blaKPC were selected. For carbapenem susceptible strains, transformants with plasmids of similar sizes with the corresponding blaKPC-positive plasmids were selected for further investigation. PCR-based replicon typing (Carattoli et al., 2005) was performed on the plasmid of these transformants. Each plasmid was then subjected to EcoRI digestion for 2 h at 37°C and electrophoresed on a 0.8% agarose gel for 1.5 h.
Southern blot analysis was performed with DIG High Prime DNA Labeling and Detection Starter Kit II (F. Hoffmann-La Roche Ltd., Basel, Swiss). Plasmids were separated on a 0.8% agarose gel and transferred to a nylon membrane as described previously (Southern, 1975). The probe for blaKPC−2 was amplified using KPC-Fm/KPC-Rm primers (Poirel et al., 2011) using KP1-R as a template. Following gel-purification, the 800-bp product was randomly primed with DIG-11-dUTP using the DIG-High Prime DNA Labeling and Detection Starter Kit II.
High-Throughput Sequencing
Plasmid DNA concentration was assessed using Qubit 2.0 Fluorometer (Life Technologies, Carlsbad, CA, USA). Ten nanograms of DNA was employed to prepare barcoded libraries with the Ion Plus Fragment Library kit (Life Technologies). Template preparation and enrichment was performed with the Ion OneTouchTM 2 System (Life Technologies). Finally, sequencing was carried out using Ion 318TM chips on the Ion PGM System (PGMTM, Life Technologies) and with the Ion PGMTM Sequencing 400 kit. Sequence gaps were filled by PCR and sequenced using primer walking. Lasergene sequence analysis software system was used to align the sequence.
Nucleotide Sequence Accession Numbers
The annotated sequences of pKP1-S, pKP1-R, pKP2-S, and pKP2-R have been submitted to GenBank nucleotide sequence database under accession numbers KT922275, KT922274, KT922273, and KT922272, respectively.
Results
Clinical Cases
From January 2011 to December 2012, four pairs of Enterobacteriaceae from four patients with identical species, identical or similar PFGE patterns and discordant carbapenem susceptibility were identified. Of these four patients, two had a pair of K. pneumoniae, one a pair of M. morganii and the other a pair of E. aerogenes (Table 1).
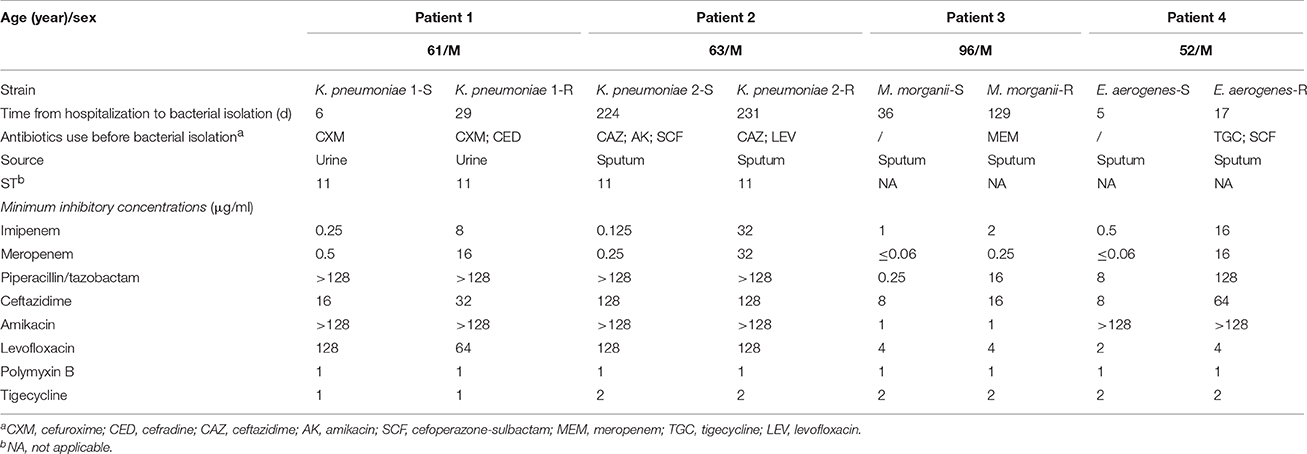
Table 1. Clinical and microbiological characteristics of four pairs of isolates with conversion of carbapenem susceptibility.
Patient 1. A 61-year-old male with cerebral hemorrhage presented to the emergency department in March 2011. After hematoma cleaning, he developed catheter-associated urinary tract infection. The patient was empirically treated with intravenous cefuroxime for 2 weeks with resolution of fever, then switched to oral cefradine. Urine culture on hospital day 6 grew carbapenem-susceptible K. pneumoniae (KP1-S). Carbapenem-resistant K. pneumoniae (KP1-R) was isolated from the urine on day 30. No other multidrug-resistant isolates were isolated after this.
Patient 2. A 63-year-old male was hospitalized with meningioma in October 2010. After resection of meningioma and placement of tracheostomy, he was diagnosed with esophageal injury and possible tracheoesophageal fistula. He had pneumonia 1 month later, and carbapenem-susceptible Pseudomonas aeruginosa and MDR Acinetobacter baumannii were isolated from sputum. The patient was treated with ceftazidime, amikacin and cefoperazone-sulbactam. Seven and half months later, carbapenem-susceptible K. pneumoniae (KP2-S) was isolated from the sputum, then he received ceftazidime and levofloxacin. One week after the therapy, carbapenem-resistant K. pneumoniae (KP2-R) grew from the sputum.
Patient 3. A 96-year-old male was admitted to the ICU in March 2011 because of acute exacerbation of chronic obstructive pulmonary disease (COPD). He had been colonized with multiple MDR organisms in the respiratory tract, including carbapenem-resistant P. aeruginosa and carbapenem-resistant K. pneumoniae. A carbapenem-susceptible M. morganii (MM-S) was isolated from the sputum a month later. Hospital-acquired pneumonia was presumed and treated with meropenem for 2 months. Four months after admission, a carbapenem-resistant M. morganii (MM-R) was detected in the sputum.
Patient 4. A 52-year-old male was admitted after sustaining craniocerebral injuries from a fall in November 2012. He required evacuation of intracranial hematoma and decompressive craniotomy. Five days after admission, a carbapenem-susceptible E. aerogenes (EA-S) was isolated from the sputum. During the first 2 weeks of hospitalization, a variety of MDR organisms were isolated, including MDR A. baumannii (respiratory tract), carbapenem-resistant P. aeruginosa (respiratory tract) and carbapenem-resistant K. pneumoniae (blood). He had intermittent fever during hospitalization. The patient was treated with tigecycline and cefoperazone-sulbactam. Seventeen days after admission, a carbapenem-resistant E. aerogenes (EA-R) strain was isolated from the sputum.
Characterization of Clinical Strains
Antimicrobial susceptibility testing revealed that strains KP1-R, KP2-R, MM-R, EA-R were carbapenem-non-susceptible with imipenem MICs of 8, 32, 2, and 16 mg/L, respectively (Table 1). The modified Hodge test was positive for carbapenemase production and synergy between boronic acid and imipenem was observed, suggesting the presence of KPC carbapenemase. The blaKPC−2 gene was detected by PCR in all the four carbapenem non-susceptible strains. No other carbapenemase genes were detected (Table 2).
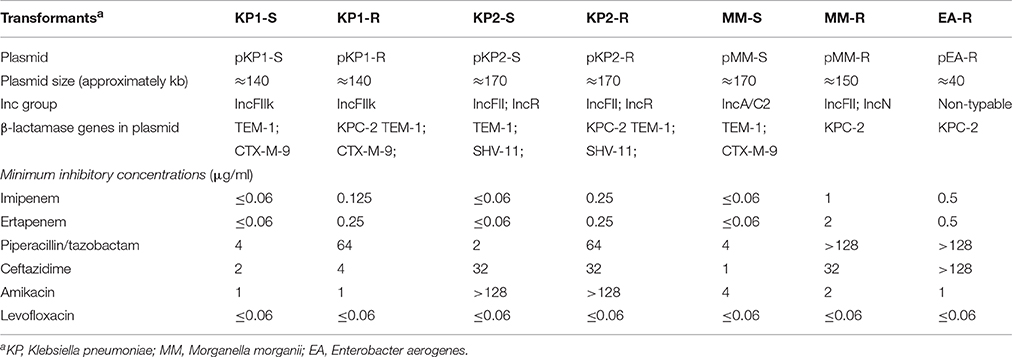
Table 2. Comparison of KPC-2 plasmid and plasmid from corresponding carbapenem-susceptible strain and of minimal inhibitory concentration of transformants.
Three pairs of Enterobacteriaceae (KP2-S and KP2-R, MM-S and MM-R, EA-S, and EA-R) showed identical PFGE patterns, suggesting that the two strains from each patient belonged to the same clone. The pair of K. pneumoniae 1 (KP1-S and KP1-R) strains had >90% similarity of PFGE profiles, indicating they were highly related (Figure 1). Plasmid DNA analysis revealed that similar plasmid profiles were found for the pairs of K. pneumoniae isolates (KP1-S and KP1-R, KP2-S, and KP2-R). The plasmid profile was identical between E. aerogenes strains EA-S and EA-R except that EA-R had one extra plasmid. Similarly sized plasmids also existed in M. morganii strains MM-S and MM-R (Supplementary Figure 1). Southern blot revealed that blaKPC−2 was located on plasmids in all four carbapenem-non-susceptible strains (KP1-R, KP2-R, MM-R, and EA-R) (Supplementary Figure 1).
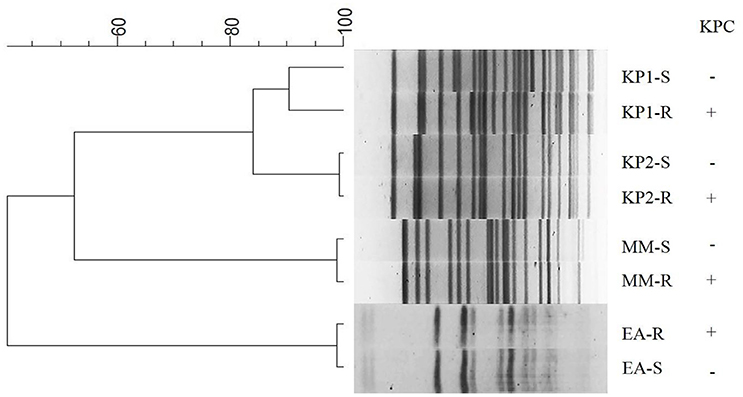
Figure 1. Dendrogram of XbaI-digested genomic DNA of clinical isolates. The dendrogram was constructed using BioNumerics software version 6.6 (Applied Maths, St-Martens-Latem, Belgium). KP, Klebsiella pneumoniae; MM, Morganella morganii; EA, Enterobacter aerogenes.
Comparison of Plasmids between Carbapenem-Resistant and -Susceptible Strains
In order to compare the plasmids harboring blaKPC−2 in carbapenem-resistant isolates, they were isolated through electroporation experiment and designated pKP1-S, pKP1-R, pKP2-S, pKP2-R, pMM-S, pMM-R, and pEA-R.
The screening of β-lactamase genes revealed that the pair of plasmids pKP1-S and pKP1-R, shared the same resistance genes blaTEM−1 and blaCTX−M−9, except that blaKPC−2 was detected in pKP1-R. pKP2-S harbored blaTEM−1 and blaSHV−11, while blaKPC−2, blaTEM−1, and blaSHV−11 were carried by pKP2-R. pMM-S harbored blaTEM−1 and blaCTX−M−9, while only blaKPC−2 was detected by pMM-R. blaKPC−2 was detected in pEA-R. (Table 2).
Plasmids from two pairs of transformants (pKP1-S and pKP1-R; pKP2-S and pKP2-R) yielded similar EcoRI digestion patterns. EcoRI digestion patterns of pMM-S and pMM-R were inconsistent (Supplementary Figure 1, Table 2).
Sequence Analysis
Three pairs of plasmids (pKP1-S, pKP1-R, pKP2-S, pKP2-R, pMM-S, and pMM-R) were sequenced to reveal the critical processes involved in the acquisition of carbapenemases. The preliminary replicon types of plasmids were determined with the replicon-specific primers, but pMM-R and pEA-R could not be typed. Thus, these six plasmids were further typed with sequence alignment based on the plasmid sequencing data.
IncFIIk replicon was detected in plasmid pKP1-S and pKP1-R. The skeleton of plasmid pKP1-R was similar with that of pKP1-S. A 2.6 kb scaffold in pKP1-S was not present in pKP1-R, while two scaffolds about 2.3 and 2.4 kb in pKP1-R were not found in pKP1-S. Plasmid pKP1-R was very similar to two blaKPC−2-carrying plasmids, pKP048 (GenBank accession no. FJ628167.2) and pKPC-LK30 (GenBank accession no. KC405622.1). Analysis on an 11,954-bp scaffold carrying blaKPC−2 gene in pKP1-R (it is about 6 kb larger than the corresponding scaffold in pKP1-S, GenBank accession no. KT922274), we found that a 6625-bp DNA fragment inserted at the upstream end of tnpA had taken place on pKP1-S (GenBank accession no. KT922275). The blaKPC−2 gene was located on an insertion sequence which included an ISKpn6-like element followed by blaKPC−2 and interrupted ISKpn8 (Figure 2).
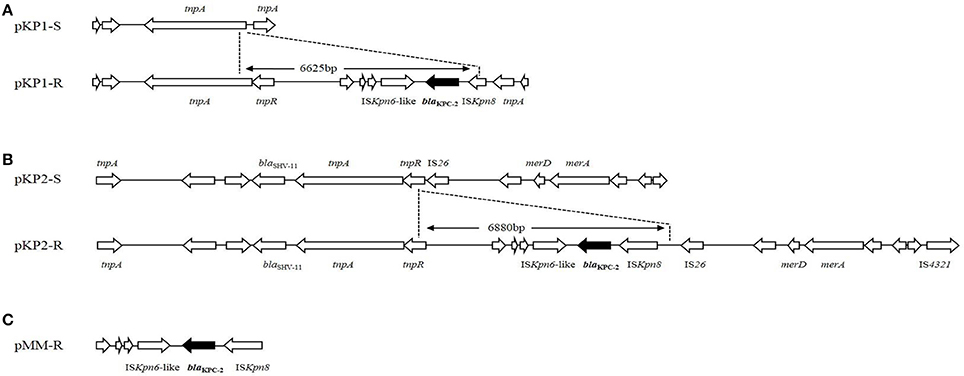
Figure 2. Plasmid structures surrounding blaKPC−2 gene in K.pneumoniae and M. morganii. (A) Comparison between plasmids pKP1-S and pKP1-R. (B) Comparison between plasmids pKP2-S and pKP2-R. (C) blaKPC−2 and its surrounding genetic elements of plasmid pMM-R. The differences between the plasmids are shown, and the genes are depicted as arrows according to the direction of transcription. The dots indicated the inserted DNA sequences in pKP1-R and pKP2-R. The blaKPC−2 genes are shown in black.
Both IncFII and IncR replicons were present in pKP2-S and pKP2-R. The scaffold of plasmid pKP2-R was also similar with that of pKP2-S. Two fragments about 4.2 and 1.3 kb in pKP2-S were not present in pKP2-R. Over 80% sequence of plasmid pKP2-R is identical to pKPC-LK30 (GenBank accession no. KC405622.1). For pKP2-R, a nucleotide sequence of 25,428 bp surrounding blaKPC−2 gene was obtained (GenBank accession no. KT922272). Compared with the scaffold obtained from pKP2-S (GenBank accession no. KT922273), a DNA fragment insertion of 6880 bp was found. Like pKP1-R, the insertion sequence including the ISKpn6-like element, blaKPC−2 and ISKpn8 was inserted at the upstream end of tnpR and downstream of IS26 in pKP2-S (Figure 2). A 5819-bp common region in the inserted DNA sequences from pKP1-R and pKP2-R is identical to Tn1721 that includes ISKpn6-like, blaKPC−2 and interrupted ISKpn8. The blaKPC−2 was also carried by the same insertion sequence including ISKpn6-like, blaKPC−2 and ISKpn8 in plasmid pMM-R (Figure 2).
IncA/C2 replicon was detected in plasmid pMM-S. A 120-kb region in pMM-S was similar to pVC1447 (GenBank accession no: KJ817377), a plasmid originally isolated from Vibrio cholerae. Both IncFII and IncN replicons were present in pMM-R. Plasmid pMM-R was 150 kb in length and had a 45-kb region identical to pKP1034 isolated from K. pneumoniae sequence type 11, which co-harbored blaKPC−2, fosA3, rmtB and blaCTX−M−65 (GenBank accession no: KP893385). No similar sequence was found between the scaffold of pMM-R and pMM-S.
Discussion
In this study, we identified four instances where patients were initially colonized or infected with a blaKPC-negative strain, which later evolved to acquire blaKPC. These patients shared in common critical illness and recent receipt of β-lactam agents. However, only one patient received carbapenem therapy before the carbapenem-resistant clinical strains were isolated. As previously reported, underlying medical conditions and antimicrobial exposure (fluoroquinolones and antipseudomonal penicillins in particular) are important risk factors for CRE acquisition (Falagas et al., 2007; Schwaber et al., 2008). This study also underscores that β-lactam agents other than carbapenems may also exert selective pressure for acquisition of blaKPC thus evolution to CRE.
In all four patients studied here, carbapenem resistance was associated with in vivo acquisition of an insertion sequence that carries blaKPC−2 or a plasmid harboring blaKPC−2 in Enterobacteriaceae. The most prevalent carbapenemase is KPC in China (Hu et al., 2012). The two pairs of K. pneumoniae belonged to ST11 and the four plasmids pKP1/2-R/S from these isolates were all classified as IncF family. ST11 was previously reported as the dominant clone in the region (Qi et al., 2011), and IncF family is the most predominant blaKPC−2-carrying plasmid, which is particularly well-suited for K. pneumoniae and for sustaining the presence of blaKPC−2 through multiple independent insertion and transposition events (Mathers et al., 2015).
Plasmids analysis provides insights into the mechanism of acquisition of genetic determinants carrying carbapenemase genes. Sequencing data revealed that pKP1-R and pKP2-R acquired mobile genetic elements containing blaKPC−2 gene. The two insertion sequences were parts of Tn1721 as previously described (Shen et al., 2009). We also found that tnpA was located upstream of blaKPC−2 in pKP1-R and pKP2-R, suggesting the transposition might involve mobilization of the insertion sequence. Interestingly, the insertion sequences, together with the flanking regions of blaKPC−2 in plasmids pKP1-R and pKP2-R, are identical with other blaKPC−2 harboring plasmids pKP048 (Shen et al., 2009) and pKPC-LK30 (Chen et al., 2014), respectively. This finding suggests that insertion of the blaKPC−2 region into IncF family plasmids is likely to be site-specific. The differences of scaffolds between pKP1-R and pKP1-S, pKP2-R and pKP2-S indicate that DNA fragments exchange may take place. Further studies are still needed to elucidate the mechanisms of the DNA fragment mobilization. To the best of our knowledge, this is the first report of insertion of blaKPC−2-carrying genetic element to plasmids in clinical isolates of K. pneumoniae in vivo.
The sizes of plasmids pMM-S and pMM-R were similar but they had different restriction patterns (data not shown). pMM-S was belonged to plasmid type of IncA/C2, while pMM-R was IncFII and IncN. Plasmid sequencing data showed that no similar sequence was observed between the scaffold of pMM-S and pMM-R. A possible explanation is that the carbapenem-susceptible M. morganii acquired a phylogenetically distinct IncFII and IncN plasmid carrying blaKPC−2 while lost the IncA/C2 plasmid. The blaKPC−2-carrying plasmid pMM-R may have been acquired from another MDR organism that was not cultured. The horizontal transfer of plasmids containing resistance genes is an essential mechanism for the dispersion of antimicrobial resistance (Mathers et al., 2015). Sidjabat et al. (2009) also revealed that blaKPC−2 was likely transferred in vivo from E. coli to Serratia marcescens by conjugation of the plasmid harboring blaKPC−2.
Co-infections with carbapenem-resistant strains of Gram-negative species are a point of interest in medical microbiology (Marchaim et al., 2012). All patients except patient 1 had co-infection or co-colonization with carbapenem-resistant A. baumannii (patient 2), P. aeruginosa and K. pneumoniae (patient 3), P. aeruginosa, A. baumannii and K. pneumoniae (patient 4), respectively. Under antimicrobial selection pressure, transposition of insertion sequences or movement of plasmid from these co-infected strains may emerge as responses to environment changes (Sidjabat et al., 2009). Those co-infecting carbapenem-resistant strains were investigated to track the potential sources of blaKPC−2 genetic elements and plasmids. Clinical strains of K. pneumoniae from patients 3 and 4 in fact harbored blaKPC−2; however, the sizes of the plasmids harboring blaKPC−2 were not identical to the plasmids from pMM-R and pEA-R, respectively (data not shown). Although the source of plasmids harboring blaKPC−2 could not be identified, we assume that blaKPC−2 was acquired from the co-infecting or co-colonizing strains. The mobility of blaKPC−2 genetic elements may be responsible for the formation of plasmids pMM-R and pEA-R.
In conclusion, our results suggest that, in addition to clonal expansion, mobile genetic elements including insertion sequences and plasmids can play a significant role in the acquisition of carbapenem resistance in vivo, adding further complexity to the already complicated molecular epidemiology.
Author Contributions
BD and ZS involved acquisition of data (laboratory and clinical), data analysis and interpretation, drafting of manuscript and critical revision. They contributed equally for this work. FH, MY, XX, and QG participated this work for acquisition of data, discussion, and manuscript revision. MW designed this work and had critical manuscript revision.
Conflict of Interest Statement
The authors declare that the research was conducted in the absence of any commercial or financial relationships that could be construed as a potential conflict of interest.
Acknowledgments
We thank Dr. Yohei Doi for his critical review of this manuscript. This work was supported by National Natural Science Foundation of China (grant numbers 81120108024, 81273559, and 81473250) and Shanghai Municipal Science and Technology Commission (grant number 12JC1401700).
Supplementary Material
The Supplementary Material for this article can be found online at: http://journal.frontiersin.org/article/10.3389/fmicb.2016.01651
References
Carattoli, A., Bertini, A., Villa, L., Falbo, V., Hopkins, K. L., and Threlfall, E. J. (2005). Identification of plasmids by PCR-based replicon typing. J. Microbiol. Methods 63, 219–228. doi: 10.1016/j.mimet.2005.03.018
Chen, Y. T., Lin, J. C., Fung, C. P., Lu, P. L., Chuang, Y. C., Wu, T. L., et al. (2014). KPC-2-encoding plasmids from Escherichia coli and Klebsiella pneumoniae in Taiwan. J. Antimicrob. Chemother. 69, 628–631. doi: 10.1093/jac/dkt409
Clinical Laboratory Standards Institute (2014). Performance Standards for Antimicrobial Susceptibility Testing; 24th Informational Supplement (M100–S24). Wayne, PA: CLSI.
Ding, B., Hu, F., Yang, Y., Guo, Q., Huang, J., and Wang, M. (2015). Four carbapenem-resistant gram-negative species carrying distinct carbapenemases in a single patient. J. Clin. Microbiol. 53, 1031–1033. doi: 10.1128/JCM.03623-14
Elliott, E., Brink, A. J., van Greune, J., Els, Z., Woodford, N., Turton, J., et al. (2006). In vivo development of ertapenem resistance in a patient with pneumonia caused by Klebsiella pneumoniae with an extended-spectrum beta-lactamase. Clin. Infect. Dis. 42, E95–E98. doi: 10.1086/503264
Falagas, M. E., Rafailidis, P. I., Kofteridis, D., Virtzili, S., Chelvatzoglou, F. C., Papaioannou, V., et al. (2007). Risk factors of carbapenem-resistant Klebsiella pneumoniae infections: a matched case control study. J. Antimicrob. Chemother. 60, 1124–1130. doi: 10.1093/jac/dkm356
Göttig, S., Gruber, T. M., Stecher, B., Wichelhaus, T. A., and Kempf, V. A. J. (2015). In vivo horizontal gene transfer of the carbapenemase OXA-48 during a nosocomial outbreak. Clin. Infect. Dis. 60, 1808–1815. doi: 10.1093/cid/civ191
Harris, A. D., Perencevich, E. N., Johnson, J. K., Paterson, D. L., Morris, J. G., Strauss, S. M., et al. (2007). Patient-to-patient transmission is important in extended-spectrum beta-lactamase-producing Klebsiella pneumoniae acquisition. Clin. Infect. Dis. 45, 1347–1350. doi: 10.1086/522657
Hu, F., Chen, S., Xu, X., Guo, Y., Liu, Y., Zhu, D., et al. (2012). Emergence of carbapenem-resistant clinical Enterobacteriaceae isolates from a teaching hospital in Shanghai, China. J. Med. Microbiol. 61, 132–136. doi: 10.1099/jmm.0.036483-0
Hu, F. P., Guo, Y., Zhu, D. M., Wang, F., Jiang, X. F., Xu, Y. C., et al. (2016). Resistance trends among clinical isolates in China reported from CHINET surveillance of bacterial resistance, 2005–2014. Clin. Microbiol. Infect. 22, S9–S14. doi: 10.1016/j.cmi.2016.01.001
Lee, H. Y., Chen, C. L., Wang, S. B., Su, L. H., Chen, S. H., Liu, S. Y., et al. (2011). Imipenem heteroresistance induced by imipenem in multidrug-resistant Acinetobacter baumannii: mechanism and clinical implications. Int. J. Antimicrob. Agents 37, 302–308. doi: 10.1016/j.ijantimicag.2010.12.015
Marchaim, D., Perez, F., Lee, J., Bheemreddy, S., Hujer, A. M., Rudin, S., et al. (2012). “Swimming in resistance”: co-colonization with carbapenem-resistant Enterobacteriaceae and Acinetobacter baumannii or Pseudomonas aeruginosa. Am. J. Infect. Control 40, 830–835. doi: 10.1016/j.ajic.2011.10.013
Mathers, A. J., Peirano, G., and Pitout, J. D. (2015). The role of epidemic resistance plasmids and international high-risk clones in the spread of multidrug-resistant Enterobacteriaceae. Clin. Microbiol. Rev. 28, 565–591. doi: 10.1128/CMR.00116-14
Munoz-Price, L. S., and Quinn, J. P. (2009). The spread of Klebsiella pneumoniae carbapenemases: a tale of strains, plasmids, and transposons. Clin. Infect. Dis. 49, 1739–1741. doi: 10.1086/648078
Nordmann, P., Dortet, L., and Poirel, L. (2012). Carbapenem resistance in Enterobacteriaceae: here is the storm! Trends Mol. Med. 18, 263–272. doi: 10.1016/j.molmed.2012.03.003
Pérez-Pérez, F. J., and Hanson, N. D. (2002). Detection of plasmid-mediated AmpC beta-lactamase genes in clinical isolates by using multiplex PCR. J. Clin. Microbiol. 40, 2153–2162. doi: 10.1128/JCM.40.6.2153-2162.2002
Poirel, L., Walsh, T. R., Cuvillier, V., and Nordmann, P. (2011). Multiplex PCR for detection of acquired carbapenemase genes. Diagn. Microbiol. Infect. Dis. 70, 119–123. doi: 10.1016/j.diagmicrobio.2010.12.002
Qi, Y., Wei, Z., Ji, S., Du, X., Shen, P., and Yu, Y. (2011). ST11, the dominant clone of KPC-producing Klebsiella pneumoniae in China. J. Antimicrob. Chemother. 66, 307–312. doi: 10.1093/jac/dkq431
Schwaber, M. J., Klarfeld-Lidji, S., Navon-Venezia, S., Schwartz, D., Leavitt, A., and Carmeli, Y. (2008). Predictors of carbapenem-resistant Klebsiella pneumoniae acquisition among hospitalized adults and effect of acquisition on mortality. Antimicrob. Agents Chemother. 52, 1028–1033. doi: 10.1128/AAC.01020-07
Shen, P., Wei, Z., Jiang, Y., Du, X., Ji, S., Yu, Y., et al. (2009). Novel genetic environment of the carbapenem-hydrolyzing beta-lactamase KPC-2 among Enterobacteriaceae in China. Antimicrob. Agents Chemother. 53, 4333–4338. doi: 10.1128/aac.00260-09
Sidjabat, H. E., Silveira, F. P., Potoski, B. A., Abu-Elmagd, K. M., Adams-Haduch, J. M., Paterson, D. L., et al. (2009). Interspecies spread of Klebsiella pneumoniae carbapenemase gene in a single patient. Clin. Infect. Dis. 49, 1736–1738. doi: 10.1086/648077
Solé, M., Fàbrega, A., Cobos-Trigueros, N., Zamorano, L., Ferrer-Navarro, M., Ballesté-Delpierre, C., et al. (2015). In vivo evolution of resistance of Pseudomonas aeruginosa strains isolated from patients admitted to an intensive care unit: mechanisms of resistance and antimicrobial exposure. J. Antimicrob. Chemother. 70, 3004–3013. doi: 10.1093/jac/dkv228
Southern, E. M. (1975). Detection of specific sequences among DNA fragments separated by gel electrophoresis. J. Mol. Biol. 98, 503–517.
Tenover, F. C., Arbeit, R. D., Goering, R. V., Mickelsen, P. A., Murray, B. E., Persing, D. H., et al. (1995). Interpreting chromosomal DNA restriction patterns produced by pulsed-field gel electrophoresis: criteria for bacterial strain typing. J. Clin. Microbiol. 33, 2233–2239.
Tzouvelekis, L. S., Markogiannakis, A., Psichogiou, M., Tassios, P. T., and Daikos, G. L. (2012). Carbapenemases in Klebsiella pneumoniae and other Enterobacteriaceae: an evolving crisis of global dimensions. Clin. Microbiol. Rev. 25, 682–707. doi: 10.1128/CMR.05035-11
Woodford, N., Fagan, E. J., and Ellington, M. J. (2006). Multiplex PCR for rapid detection of genes encoding CTX-M extended-spectrum (beta)-lactamases. J. Antimicrob. Chemother. 57, 154–155. doi: 10.1093/jac/dki412
Wu, P. C., Lo, W. T., Chen, S. J., and Wang, C. C. (2014). Molecular characterization of Group A streptococcal isolates causing scarlet fever and pharyngitis among young children: a retrospective study from a northern Taiwan medical center. J. Microbiol. Immunol. Infect. 47, 304–310. doi: 10.1016/j.jmii.2013.02.007
Keywords: Enterobacteriaceae, carbapenem resistance, insertion sequence, plasmid, KPC-2
Citation: Ding B, Shen Z, Hu F, Ye M, Xu X, Guo Q and Wang M (2016) In vivo Acquisition of Carbapenemase Gene blaKPC-2 in Multiple Species of Enterobacteriaceae through Horizontal Transfer of Insertion Sequence or Plasmid. Front. Microbiol. 7:1651. doi: 10.3389/fmicb.2016.01651
Received: 03 February 2016; Accepted: 04 October 2016;
Published: 21 October 2016.
Edited by:
Manuela Caniça, National Institute of Health Dr. Ricardo Jorge, PortugalReviewed by:
Christopher Morton Thomas, University of Birmingham, UKSebastian Guenther, Free University of Berlin, Germany
Andrea Endimiani, University of Bern, Switzerland
Copyright © 2016 Ding, Shen, Hu, Ye, Xu, Guo and Wang. This is an open-access article distributed under the terms of the Creative Commons Attribution License (CC BY). The use, distribution or reproduction in other forums is permitted, provided the original author(s) or licensor are credited and that the original publication in this journal is cited, in accordance with accepted academic practice. No use, distribution or reproduction is permitted which does not comply with these terms.
*Correspondence: Minggui Wang, bWd3YW5nQGZ1ZGFuLmVkdS5jbg==
†These authors have contributed equally to this work.