- 1Public Health Research Institute Center, New Jersey Medical School, Rutgers University, Newark, NJ, USA
- 2Laboratoire de Microbiologie et Génétique Moléculaires, Université de Toulouse, Toulouse, France
The K-state in the model bacterium Bacillus subtilis is associated with transformability (competence) as well as with growth arrest and tolerance for antibiotics. Entry into the K-state is determined by the stochastic activation of the transcription factor ComK and occurs in about ∼15% of the population in domesticated strains. Although the upstream mechanisms that regulate the K-state have been intensively studied and are well understood, it has remained unexplained why undomesticated isolates of B. subtilis are poorly transformable compared to their domesticated counterparts. We show here that this is because fewer cells enter the K-state, suggesting that a regulatory pathway limiting entry to the K-state is missing in domesticated strains. We find that loss of this limitation is largely due to an inactivating point mutation in the promoter of degQ. The resulting low level of DegQ decreases the concentration of phosphorylated DegU, which leads to the de-repression of the srfA operon and ultimately to the stabilization of ComK. As a result, more cells reach the threshold concentration of ComK needed to activate the auto-regulatory loop at the comK promoter. In addition, we demonstrate that the activation of srfA transcription in undomesticated strains is transient, turning off abruptly as cells enter the stationary phase. Thus, the K-state and transformability are more transient and less frequently expressed in the undomesticated strains. This limitation is more extreme than appreciated from studies of domesticated strains. Selection has apparently limited both the frequency and the duration of the bistably expressed K-state in wild strains, likely because of the high cost of growth arrest associated with the K-state. Future modeling of K-state regulation and of the fitness advantages and costs of the K-state must take these features into account.
Introduction
The transcription factor ComK (van Sinderen et al., 1995) directly activates more than 100 genes (Berka et al., 2002; Hamoen et al., 2002; Ogura et al., 2002). While about 20 of these mediate the uptake, processing and integration of exogenous DNA resulting in transformation (Burton and Dubnau, 2010), the roles of the remaining ∼80 genes are poorly understood. Because these genes are not needed for transformation (J. Hahn, unpublished) the expression of ComK must result in phenotypes beyond competence that presumably enhance fitness. In fact, ComK also induces a period of growth arrest during which the cells that express ComK exhibit antibiotic tolerance (Nester and Stocker, 1963; Haijema et al., 2001; Johnsen et al., 2009; Briley et al., 2011; Hahn et al., 2015; Yuksel et al., 2016). This persistent state has been called the K-state, to emphasize that ComK regulates more than competence for transformation (Berka et al., 2002).
A long recognized and remarkable feature of the K-state is that it is expressed in a minor fraction of a clonal population, in a more or less all or nothing fashion (Nester and Stocker, 1963; Singh and Pitale, 1967; Hadden and Nester, 1968; Haseltine-Cahn and Fox, 1968; Maamar and Dubnau, 2005; Smits et al., 2005). Entry into the K-state is determined stochastically and studies in domesticated strains derived from the indole-requiring auxotrophic strain 168 (Spizizen, 1958) have ascribed this stochastic determination to noise in the basal expression of the comK promoter (Maamar et al., 2007; Süel et al., 2007). When the noisy expression of comK causes a subpopulation of cells to exceed a threshold level of ComK, two dimers of this protein bind cooperatively to the comK promoter (van Sinderen and Venema, 1994; Hamoen et al., 1998), activating a positive feedback loop and the rapid transition of these cells into the K-state, where ComK activates downstream genes.
The frequency of these activation events is extremely low during exponential growth and then rises as a culture approaches the stationary phase of growth. This temporal control has two principal causes. First, growing cultures secrete ComX, a quorum sensing pheromone, which accumulates and ultimately causes the phosphorylation of the response-regulator protein ComA (Magnuson et al., 1994). ComA-P then binds to and activates the promoter of the srfA operon, which encodes the small protein ComS (Nakano and Zuber, 1991; Nakano et al., 1991; Roggiani and Dubnau, 1993; Hamoen et al., 1995). ComS in turn competes with ComK for binding to the MecA-ClpC-ClpP protease, which rapidly degrades ComK during growth (Turgay et al., 1997, 1998; Prepiak and Dubnau, 2007). Stabilization occurs toward the end of exponential growth when high cell densities have produced sufficient ComX levels. A second cause of temporal regulation derives from the exquisitely controlled kinetics of comK basal expression (Leisner et al., 2007; Mirouze et al., 2012). Ensemble measurements show that the average basal rate of comK transcription increases gradually during growth, reaches a maximum as cells depart from exponential growth (T0), and then declines. This uptick in basal expression is due to a gradual increase in the phosphorylated form of the master regulator Spo0A as cells approach stationary phase (Mirouze et al., 2012). Spo0A is phosphorylated as a consequence of a phosphorylation cascade in which several kinases transfer phosphoryl groups to Spo0F (Burbulys et al., 1991). These groups are then passed to the phosphotransfer protein Spo0B and finally to Spo0A. Low levels of Spo0A-P directly activate the basal expression of comK, while higher levels bind to repressive operator sites so that the rate of comK expression decreases (Mirouze et al., 2012). Thus, Spo0A-P opens and then closes a temporal gate for transitions to the K-state.
Although K-state regulation has been well characterized, there was reason to believe that our understanding was lacking. It has been observed that the transformability of undomesticated isolates of Bacillus subtilis and its close relatives is much lower than that of the domesticated derivatives of 168 (Cohan et al., 1991). In fact the model undomesticated isolate NCIB3610 (hereafter 3610), is poorly transformable, although it is very closely related to the wild parent of 168. The poor transformability is due in part to comI, a gene that is absent in 168-derivatives. Interestingly, the ComI protein appears to decrease the uptake of DNA, without affecting K-state expression (Konkol et al., 2013). Together, these observations suggest that some regulatory feature has been lost in the domesticated strains and that our appreciation of K-state regulation is consequently incomplete.
In the present study, we have shown that a known promoter mutation in degQ in domesticated strains (Yang et al., 1986; McLoon et al., 2011) is primarily responsible for this difference in transformability. It is of interest that this mutation also contributes to the failure of strain 168 derivatives to form robust biofilms (McLoon et al., 2011). A consequence of this mutation is that the response regulator DegU is poorly phosphorylated in domesticated strains (Stanley and Lazazzera, 2005; Kobayashi, 2007). This deficit in DegU-P derepresses PsrfA. This causes ComK to be stabilized, allowing more cells to pass the threshold for comK auto-activation, thereby increasing the fraction of K-state cells. It is known that unphosphorylated DegU is required for K-state expression because it helps ComK bind to its own promoter, thus acting as a priming protein when the ComK concentration is low (Hamoen et al., 2000). Thus, our present results show that the regulation of the K-state in undomesticated strains requires the proper ratio of phosphorylated and unphosphorylated DegU, in accordance with the view that this protein acts as a rheostat for development (Verhamme et al., 2007).
Materials and Methods
Microbiological Methods
Bacterial strains are listed in Supplementary Table S1. The backgrounds used for all experiments were either IS75, a derivative of strain 168, PS216 (an undomesticated strain of B. subtilis isolated in Slovenia and kindly provided by Inés Mandic-Mulec) (Durrett et al., 2013), 3610△comI or 3610 comIQ12L (both gifts from Dan Kearns). The comIQ12L mutation abolishes ComI activity (Konkol et al., 2013), removing a block in DNA uptake. Constructs were introduced into IS75 by transformation (Albano et al., 1987) and into 3610△comI and PS216 by transduction using bacteriophage SPP1 (Cozy and Kearns, 2010). An exception was for the swapping of the degQ alleles, which was carried out by transformation, as described below. Bacterial growth was at 37°C in competence medium (Albano et al., 1987) unless otherwise specified. Antibiotic selections were carried out on Lysogeny Broth (LB) agar plates (Cozy and Kearns, 2010) containing ampicillin (100 μg ml-1), spectinomycin (100 μg ml-1), erythromycin (5 μg ml-1), kanamycin (Kan) (5 μg ml-1) or chloramphenicol (5 μg ml-1). In some cases selection was for erythromycin (Ery) (1 μg ml-1) plus lincomycin (20 μg ml-1). Solid media were solidified by the addition of 1.5% agar. Transformation frequencies were determined using genomic DNA isolated from a leucine prototroph.
Luciferase Assays
Light output from luciferase reporter constructs was measured as previously described (Mirouze et al., 2011). Briefly, strains were first grown in LB medium for 2 h. Cells were then centrifuged and resuspended in fresh competence medium (Albano et al., 1987), adjusting all the cultures to an OD600 of 2. These pre-cultures were then diluted 20-fold in fresh competence medium and 200 μl was distributed in duplicate in the wells of a 96-well black plate (Corning Incorporated Costar). Ten microliters of luciferin was added to each well to reach a final concentration of 1.5 mg/ml (4.7 mM). The cultures were incubated at 37°C with agitation in a PerkinElmer Envision 2104 Multilabel Reader equipped with an enhanced sensitivity photomultiplier for luminometry. The temperature of the clear plastic lid was maintained at 38°C to avoid condensation. Relative Luminescence Units (RLU) and OD600 were measured at 1 min intervals after two 30-s shaking steps. The data were processed using a script written in MATLAB, exported to Excel and plotted as RLU/OD versus time from the beginning of growth.
SDS-PAGE and Immunoblotting
Cell pellets were resuspended in STM buffer (50 mM Tris pH 8.0, 25% sucrose, 50 mM NaCl, 5 mM MgCl2) containing 300 μg ml-1 lysozyme and incubated at 37°C for 5 min. The volume of STM was normalized to the turbidity measurement of the culture (determined in a Klett colorimeter) when the sample was collected. Sample buffer (final concentration of 20 mM Tris HCl pH 6.8, 10% glycerol, 1% SDS, 0.01% bromophenol blue, 2% 2-mercaptoethanol) was added to the samples, which were then incubated at 100°C for 5 min. Samples were separated by electrophoresis in 12% Tris-tricine SDS polyacrylamide gels (Schägger and von Jagow, 1987). The proteins were transferred to nitrocellulose membranes (Millipore) using a Trans Blot Turbo semidry transfer apparatus (Bio-Rad). Primary antiserum raised in rabbits against ComK was used at a dilution of 1:5000. Signal was detected using a secondary dilution of 1:10,000 goat anti-rabbit antiserum conjugated to horseradish-peroxidase (HRP) followed by visualization using Enhanced Chemiluminescence (ECL) Prime Western Blot Detection Reagent (GE Healthcare) according to the manufacturers’ instructions. Images were recorded by using a Thermo Scientific MyECL Imager and band intensities were measured using ImageJ software (Schneider et al., 2012).
degQ Allele Swapping with pMiniMAD2
To swap the degQ promoter between IS75 and 3610 at the native loci, we used the pMiniMAD2 cloning strategy as described (Cozy and Kearns, 2010; Mukherjee et al., 2013). A 2 kb fragment containing degQ from strain 168 or 3610 was amplified using primers (HinDIII-degQ9: 5′-GCAGCAAAGCTTCTGCGATTTCCGGATAAAAGAACATAATAATCCCAG-3′ and BamHI-degQ10: 5′-GCAGCAGGATCCGCAGCCTGCTTCTTATATGCTGATCG-3′). The amplicons, which carried the degQ wild and mutant alleles near their centers, were cloned into the HinDIII and BamHI sites on pMiniMAD2, to produce the plasmids pED1932 and pED1931, which carried the wild-type and mutant degQ alleles, respectively. These plasmids were used to transform IS75 (with pED1932) and 3610 (with pED1931) where they integrated by single crossover events. Plasmid-free strains carrying the swapped degQ promoters were isolated (Cozy and Kearns, 2010; Mukherjee et al., 2013) to create 168 degQ3610 (BD7454) and 3610 degQIS75 (BD7445). The presence of the swapped promoters was confirmed by sequencing of PCR products amplified from the chromosome, carried out by Eton Biosciences (Union, NJ, USA).
The Phyper-spank-degQ Construction
The primers degQ15 (5′-TTAGTCGACAGCTAGCCACCATACACAATTCATTGATCTTTCA-3′) and degQ16 (5′-CTTGCATGCGGCTAGCTACTCGTTAATCCTACTGTATACAAGGA-3′) were used to amplify a 676 bp sequence containing the degQ gene without its promoter. The amplicon was inserted into the Phyper-spank vector pED1870 that had been cut with NheI, using the In-Fusion HD cloning kit (Clontech, Inc.), as per the manufacturer’s instructions. The resulting plasmid was integrated into the desired host strains by transformation with single crossover events, by selection for Kan resistance. pED1870 carries the Phyper-spank, lacI and lacO sequences. This and all other constructs were verified by sequencing. The resulting plasmid creates a strain in which degQ is under Phyper-spank at its native locus.
Microscopy
Cells were harvested, diluted into PBS (81 mM Na2HPO4 + 24.6 mM NaH2PO4 + 100 mM NaCl) and 1 μl of each culture was placed on a pad of 1% agarose made up in 0.5X TAE buffer. Images were collected using a Nikon Eclipse Ti inverted microscope equipped with an Orca Flash 4.0 digital camera (Hamamatsu), with a Nikon TIRF 1.45 NA Plan Neoflur 100 oil immersion objective. NIS-Elements AR (v 4.40, Nikon) software was used to collect and analyze images, which were then imported into Photoshop to configure the images for publication. Fluorescence intensities were determined using the automated General Analysis tool of NIS-Elements.
Results
Undomesticated Bacillus subtilis Strains Express the K-state in Few Cells
It has been observed that the transformability of undomesticated B. subtilis strains is generally lower than that of the domesticated strain 168 and its derivatives (Cohan et al., 1991). Because the K-state is expressed bistably, this difference may reflect differing efficiencies of transformation per cell or differing frequencies of K-state cells in the population. We therefore determined the percentage of competence-expressing cells during growth in five randomly chosen natural isolates: the B. subtilis subsp. subtilis RO-OO-2 and RO-FF-1 strains, the B. subtilis subsp. spizizenii RO-E-2 [all three isolated in the Mojave desert (Cohan et al., 1991)], the commonly used model strain 3610 (Branda et al., 2006; McLoon et al., 2011) and B. subtilis PS216, isolated in Slovenia (Durrett et al., 2013). Throughout this study we have used comI mutants of 3610. ComI does not affect the expression of comK but does inhibit the uptake of transforming DNA (Konkol et al., 2013). In each case, after verifying that the regulatory sequences upstream of comK were the same in all the strains (not shown), comK promoter (PcomK) fusions to the genes encoding Cyan Fluorescent Protein (CFP) or Green Fluorescent Protein (GFP) were integrated by single reciprocal recombination and the percentages of comK-expressing cells were enumerated microscopically and compared to that of the 168-derivative IS75, which is the reference domesticated strain used throughout this study. We determined that the time of maximal transformability for PS216 and 3610 was attained after 240 min of growth and after 315 min for IS75 (Supplementary Figure S1). The results in Figure 1 were therefore obtained with samples taken at those times, which correspond to about T0 and T2 for the undomesticated and domesticated strains, respectively. The growth curves for all the strains under these conditions were similar (not shown). The results summarized in Figure 1 show that the samples from the undomesticated isolates contain significantly fewer competence-expressing cells (0.2–5%) than the domesticated strain sample (15.4%).
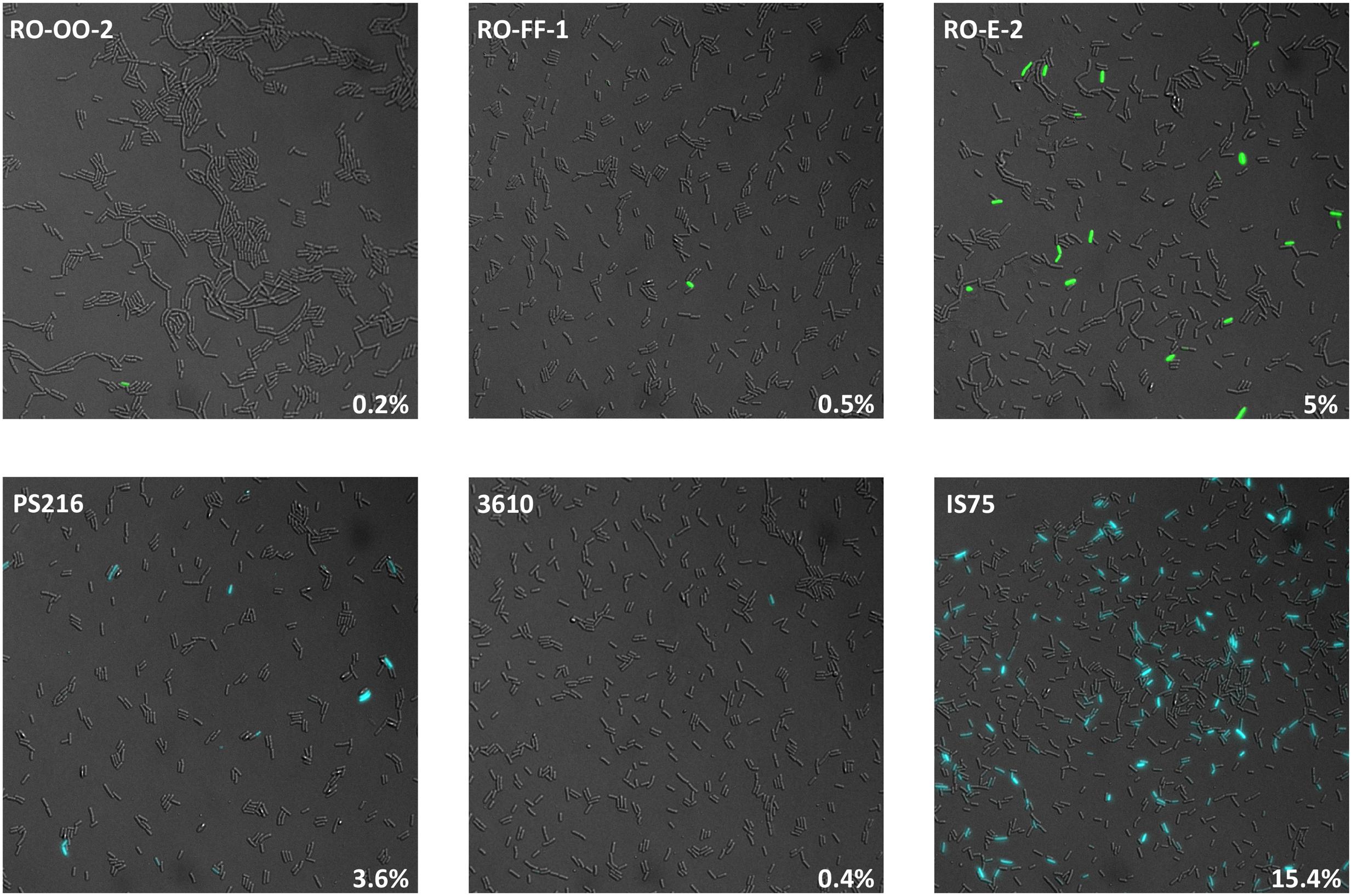
FIGURE 1. Undomesticated strains express the K-state with low frequency. Shown are representative images of the indicated strains expressing PcomK fusions to the genes encoding GFP (top row) or CFP (bottom row). The fields were selected to show at least one expressing cell. The measured frequencies of K-state cells are indicated in the lower right of each panel. As explained in the text, the domesticated strain (IS75) was imaged at T2 and the undomesticated strains at T0. Strain numbers are presented in Supplementary Table S1.
To compare the transcription rates from PcomK in strains 3610 and PS216 to that of the domesticated strain, we utilized promoter fusions to firefly luciferase, which reports transcription rates rather than the accumulation of a gene product (Mirouze et al., 2011). The fusions were integrated by single-reciprocal recombination placing the reporter gene under control of the normal comK regulatory sequences. The peak rates of transcription from PcomK in the domesticated strain and in both 3610 and PS216 approximately reflect the frequencies of K-state cells in each population (Figure 2). The peak transcription rates in the undomesticated strains are about 9.6- and 5.7-fold lower than in the IS75 background, while the fractions of competence-expressing cells differ by 38- and 4.2-fold, respectively. We do not expect an exact correspondence between the peak rates of transcription and transformation frequencies; light output from luciferase does not inform us about the amount of ComK synthesized, the activation of the downstream genes needed for transformation and the assembly of the transformation machinery. In the domesticated strain, the transcription rate remains elevated even as the cultures enter stationary phase (Figure 2A). In 3610 and PS216, the rates decline rapidly from a maximum reached at about T0 (Figures 2B,C). These data demonstrate that unknown mechanisms limit the probability of transitions to the K-state and cause the expression of comK to cease abruptly. Interestingly, these mechanisms have been lost in the domesticated strains.
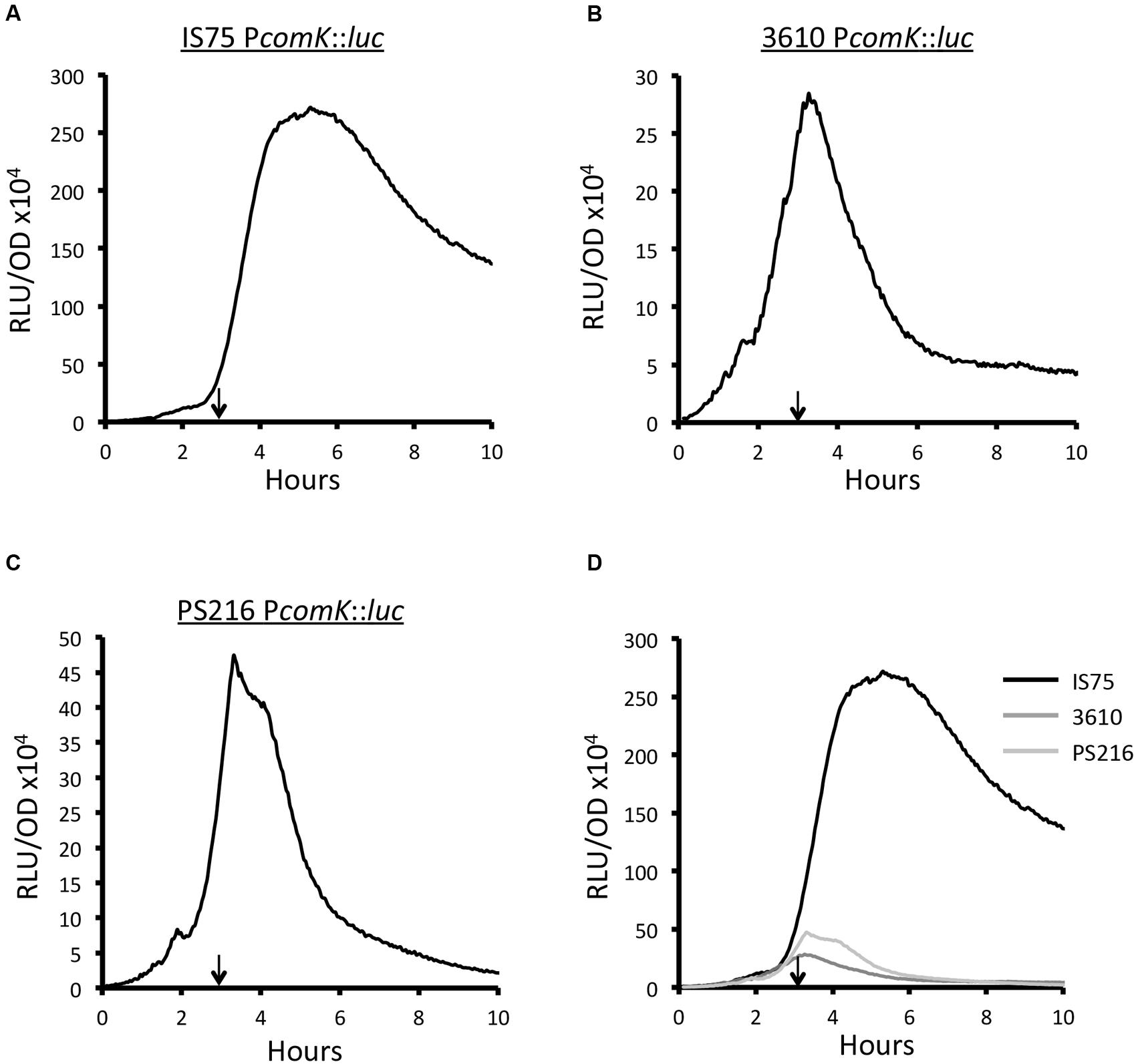
FIGURE 2. Expression from PcomK is higher in IS75 (A) than in the undomesticated strains 3610 (B) and PS216 (C). (D) The three curves plotted on the same scale. The vertical arrows on panels (A–C) point to T0. Strain numbers are presented in Supplementary Table S1.
A degQ Mutation in the Domesticated Strains Causes Increased comK Expression
To identify gene(s) that limit transitions to the K-state in undomesticated strains, we transformed PS216 with DNA from a domesticated strain that carries a fusion of the comG promoter to lacZ, linked to a kanamycin (Kan) resistance determinant. It was reasoned that selection for this marker on plates containing Kan and 5-bromo-4-chloro-3-indolyl-β-D-galactopyranoside (X-gal) would select for transformable cells and might transfer an unlinked mutation from the domesticated strain, capable of conferring increased expression from PcomG. Indeed, dark blue colonies were observed, representing about 1% of the total number of KanR colonies, a frequency consistent with “congression,” the simultaneous transformation of B. subtilis by unlinked markers. Although PS216 colonies are normally mucoid, all of these blue colonies were not. Mucoidy in B. subtilis indicates the production of poly-γ-glutamic acid, due to the expression of the pgs operon and is dependent on the presence of phosphorylated DegU and the small protein, DegQ (Stanley and Lazazzera, 2005). DegQ increases the net transfer of a phosphoryl group from the histidine kinase DegS to its cognate response regulator protein DegU (Kobayashi, 2007). Because derivatives of strain 168 carry a promoter mutation that markedly decreases the expression of degQ (Yang et al., 1986; McLoon et al., 2011), we reasoned that the blue colonies might have inherited this mutation. The degQ promoters of several blue transformants were sequenced and indeed all had the T→C promoter mutation at position -10 that exists in 168 derivatives.
To determine whether this mutation was responsible for the high comK expression of domesticated strains, we swapped the wild-type and mutant degQ alleles between the domesticated and undomesticated strains, using pMiniMAD2. For this, and for all subsequent experiments reported here, we have used 3610, which has been widely adopted as a model undomesticated B. subtilis, rather than the less intensively studied PS216. Figure 3A shows the expression profiles of PsrfA, PcomK, and PcomG luciferase fusions in the IS75 background, for strains carrying the indigenous mutant (degQIS75) and wild-type (degQ3610) alleles. Clearly the introduction of the wild-type degQ allele resulted in a marked diminution of the transcription rates of each reporter so that the peak levels were similar to those of 3610 (compare to Figure 3B). The converse was also true (Figure 3B); introduction of the degQIS75 allele into the 3610 background increased the maximum transcription rates of all three promoters to approximately the levels measured in IS75 (Figure 3A). A degQ knockout was also tested (Supplementary Figure S2) using a PcomG-luc reporter in the 3610 background. As expected, a large increase in comG transcription was noted. The effects of degQ allele swapping shown in Figure 3 can thus be explained most economically by a repressing effect of DegU-P on PsrfA, which would decrease the amount of ComS, destabilizing ComK. In fact it has been shown that mutations resulting in an elevated level of DegU-P do indeed depress the transcription of srfA in the domesticated background (Hahn and Dubnau, 1991).
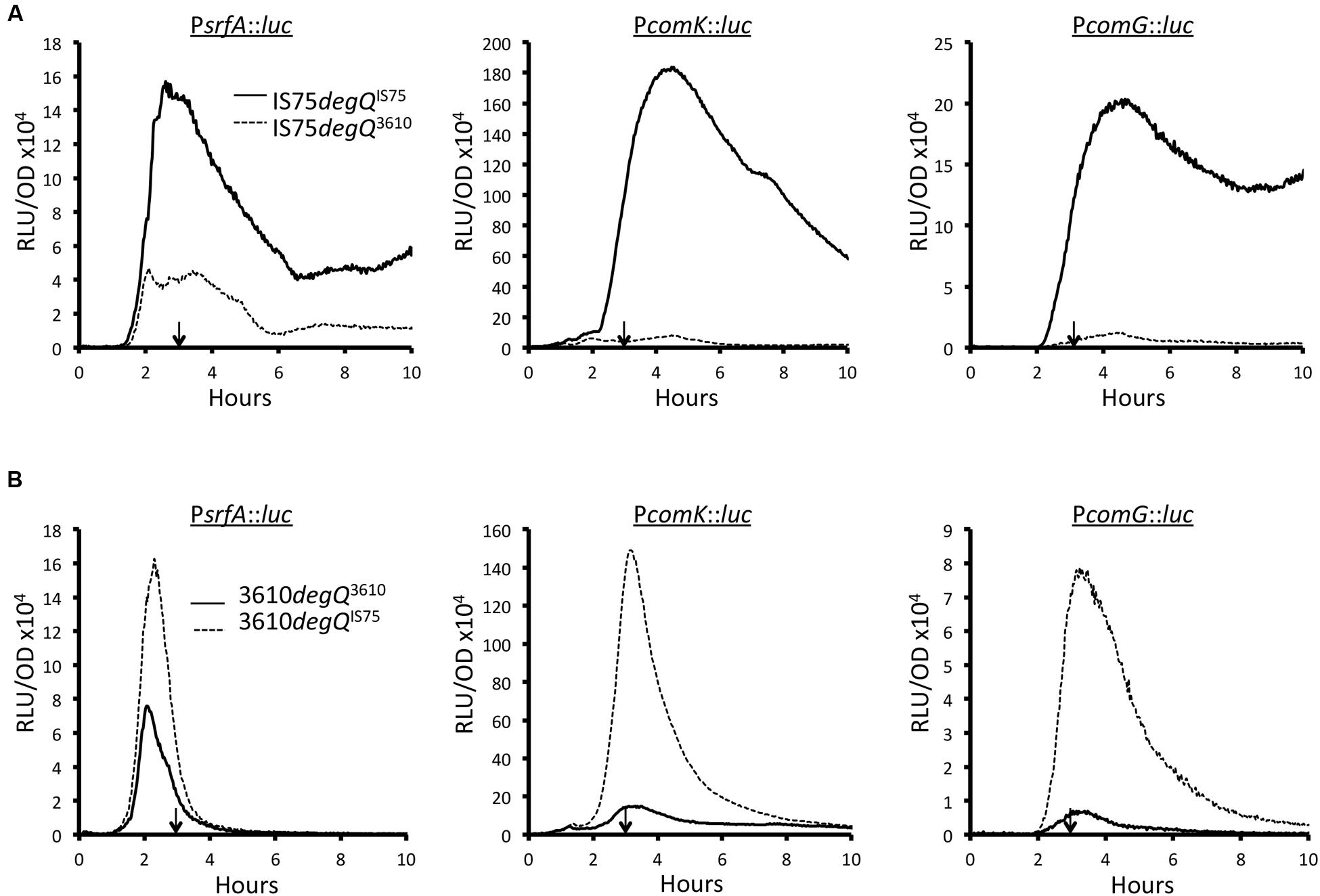
FIGURE 3. Effect of the mutant (degQIS75) and wild-type (degQ3610) degQ alleles on expression of srfA, comK, and comG in IS75 (A) and 3610 (B) backgrounds. In each panel, the data from strains with indigenous and swapped alleles are indicated by solid and dashed lines, respectively. The vertical arrows in each panel point to T0. Strain numbers are presented in Supplementary Table S1.
The Sharp Decrease in Transcription in 3610 is Not Due to DegQ
Somewhat surprisingly, the characteristic sharp decreases in the transcription rates of srfA and of comK in the 3610 background are not affected by the introduction of the mutant degQ promoter (Figure 3). Nor are the sustained high transcription rates from these promoters in the domesticated background obliterated when the wild-type degQ allele is introduced. This is seen clearly when the peak values of the swapped and un-swapped strains are normalized (Supplementary Figure S3). The comK transcription patterns can be explained by differences in srfA transcription if we make the simplifying assumption that ComS is unstable. Thus, when the rate of comS transcription decreases sharply, ComK would no longer be protected from degradation, and the transcription of comK and of comG would decrease. In accord with this reasoning, the transcription rates of srfA, comK and comG begin to drop after 2.3, 3.1, and 3.1 h of growth, respectively (Figure 3). The converse would apply in the domesticated strains; sustained transcription from the srfA promoter would provide a steady supply of ComS, stabilizing ComK. To test this idea, we measured the transcription rates of srfA in three different backgrounds. As noted above, PS216 is intermediate between 3610 and IS75 in transformability, in comK expression and in the percentage of cells that enter the K-state. Supplementary Figure S4 shows that while transcription from PsrfA drops to zero in 3610, it decreases to an intermediate level in PS216. This can be seen clearly when the three curves are normalized to the same peak value (Supplementary Figure S4B). These comparisons are in agreement with the hypothesis that the kinetics of srfA transcription underlie the strain differences in transformability.
The DegQ Effect on K-state Expression is Mediated by Increased DegU-P
If the presence of the mutant degQ allele affects competence expression because of its depressing effect on DegU phosphorylation, it should be mimicked, at least in 3610, by inactivation of degS, which encodes the DegU-cognate histidine kinase. The results obtained in the 3610 background support a role for DegU-P as a regulator of srfA and hence of comK and comG expression (Figure 4). The degS knockout strain reaches a higher rate of srfA transcription than the wild-type and presumably produces more ComS (Figure 4B). Consequently ComK is stabilized, more comK transcription takes place and comG transcription is actually increased (Figure 4B). In contrast with this straightforward result, Figure 4A reveals that the inactivation of degS has no effect on srfA transcription in the IS75 background. This is not unexpected, because in this background, which is naturally lacking in DegQ, the amount of DegU-P is low and does not limit srfA transcription. However, the expression from comK is affected, decreasing in peak value about twofold. This relatively minor effect of degS inactivation can be explained as follows. Unphosphorylated DegU is known to increase the affinity of ComK for its own promoter (Hamoen et al., 2000). Because DegU-P activates one of the promoters that drive degU expression (Ogura and Tsukahara, 2010), it is likely that the inactivation of degS decreases the amount of DegU and thus compromises ComK binding to PcomK. The expression from PcomG is also affected (Figure 4A) but less than that from PcomK, suggesting that ComK is normally produced in excess.
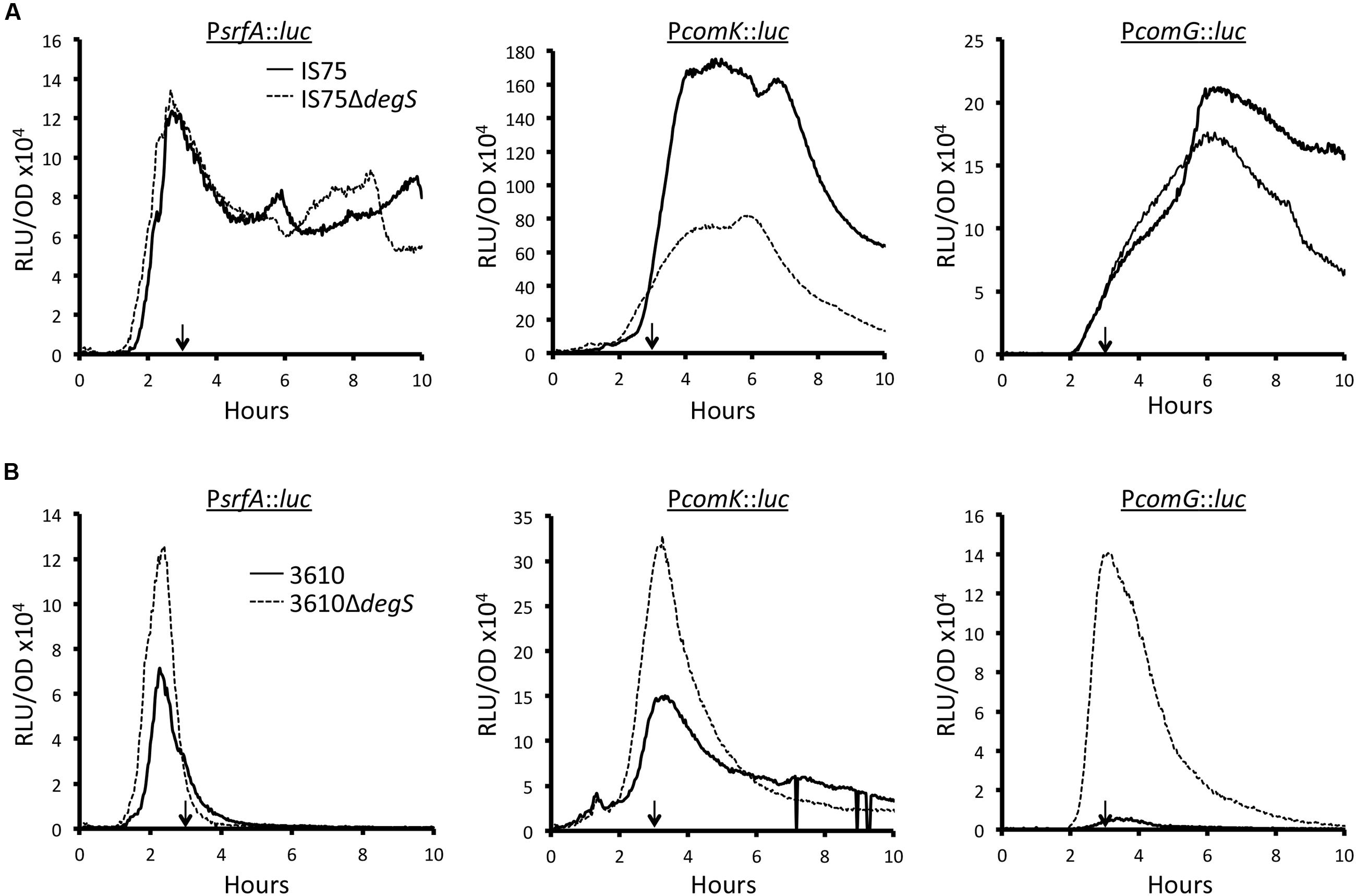
FIGURE 4. Effect of ΔdegS on expression of srfA, comK, and comG in IS75 (A) and 3610 (B) backgrounds. The vertical arrows in each panel point to T0. Strain numbers are presented in Supplementary Table S1.
Further evidence that the depressing effect on srfA expression in 3610 is due to phosphorylated DegU was obtained using a degUD56N allele. The DegUD56N protein cannot be phosphorylated. Supplementary Figure S5 shows that the DegUD56N mutant strain is de-repressed for srfA transcription similarly to the degS inactivated strain (compare to Figure 4B). As observed when the degQ alleles were swapped (Figure 3; Supplementary Figure S3), no effect of either the D56N or degS knockout mutations was observed on the sharp downturn in srfA transcription in the 3610 background.
DegQ Regulates the Stability of ComK
If the effects of DegU-P on PsrfA, and hence on comS transcription underlie the differences in K-state expression, we would expect to see this reflected in measurements of ComK stability. Because the amount of ComK in the presence of elevated DegQ is quite low, we resorted to the following strategy to investigate its stability. A strain was constructed that carries a copy of comK under control of the xylose-inducible Pxyl promoter inserted in the ectopic amyE locus of IS75. The native comK gene was left intact so that the effects of induction due to the addition of xylose would be amplified by the auto-activation of comK transcription. Also present was a copy of degQ under control of the isopropyl-β-D-thiogalactoside (IPTG)-inducible Phyper-spank promoter, located in the chromosome at the degQ locus. This strain was grown in the continuous presence of xylose, with and without IPTG. When late log phase was reached, rifampicin and puromycin were added to terminate mRNA and protein synthesis, samples were collected thereafter at regular intervals, and Western blotting was used to monitor the decay of ComK (Figure 5). As predicted, the stability of ComK was markedly reduced when degQ was induced. In the uninduced culture the half-life of ComK is well in excess of 20 min; extrapolation would suggest it to be at least 60 min. The half-life of ComK in the presence of induced DegQ is 7–8 min. As expected, the initial amount of ComK was also reduced in the induced culture. These data confirm that the enhanced transformability of the domesticated strain can be ascribed to the increased stability of ComK, caused by the de-repression of srfA (comS).
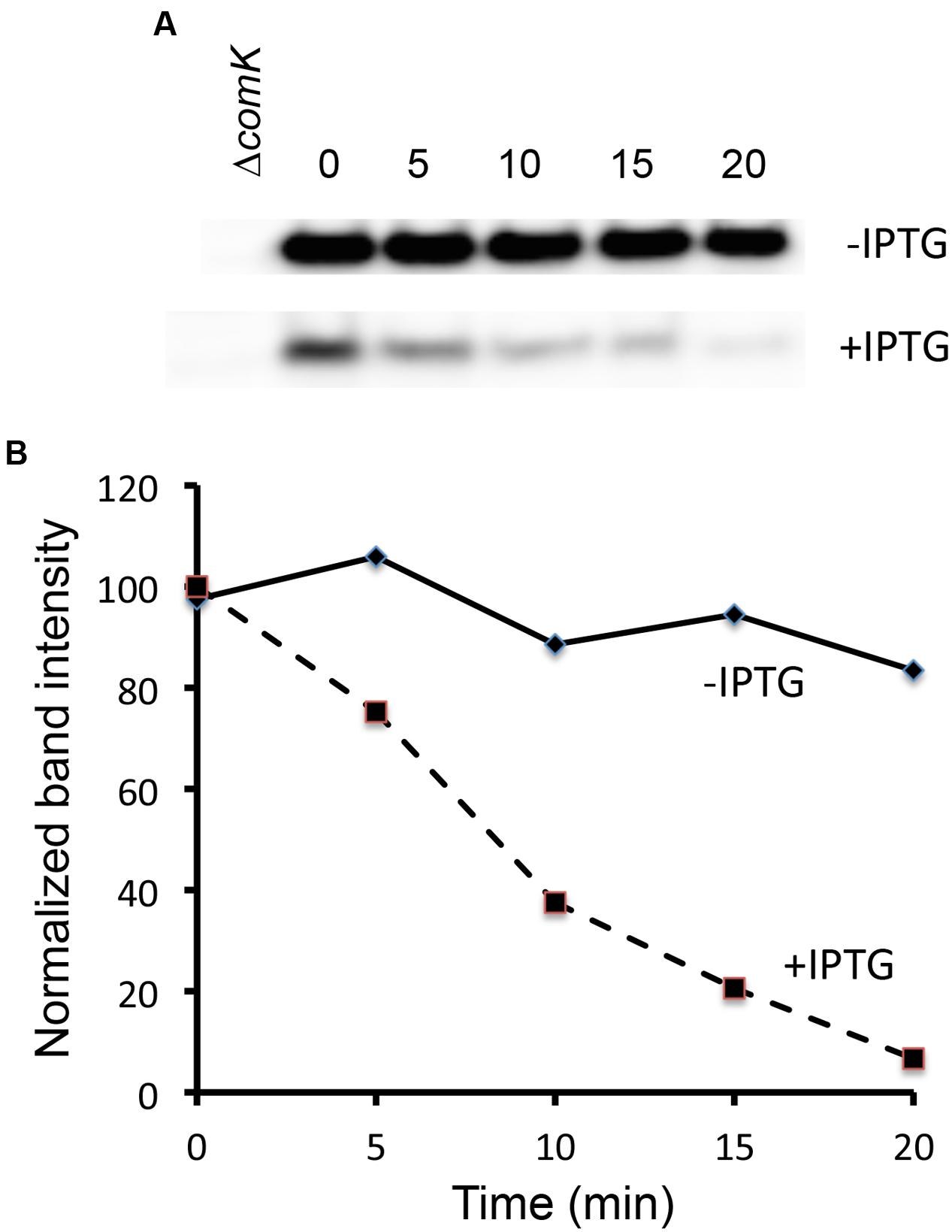
FIGURE 5. DegQ lowers ComK stability. A strain carrying Pxyl-comK and Phyper-spank-degQ (BD8288) was grown in the presence of xylose and with and without IPTG. At T-1, puromycin and rifampicin, each at 200 μg/ml were added. At the indicated times, samples were withdrawn for Western blotting with anti-ComK antiserum. (A) The -IPTG gel was exposed for a shorter time to compensate for its higher initial intensity of the ComK band. Extracts from a ΔcomK strain were included to assist in the identification of the correct band. The ±IPTG images were from the same gel and placed one above the other (B) Bands intensities were quantified from digitized images using ImageJ software and plotted against time after the addition of the inhibitors.
Single Cell Expression in degQ-Swapped Strains
Ensemble measurements of srfA and comG transcription show that their expression is correlated, and influenced by the levels of DegU-P. Figure 6 shows this behavior on a single cell level. For these experiments, cells expressing promoter fusions of genes encoding mCherry and GFP to PsrfA and PcomG, respectively, were examined microscopically. Unlike firefly luciferase these reporters reflect the accumulation of gene products. These images were collected for the wild-type IS75 and 3610 strains as well as for strains in which the degQ alleles had been swapped. It is obvious that the GFP expressed from PcomG accumulated in more cells in the IS75 background than in 3610, as expected. Also as expected, the intensity of the mCherry fluorescence expressed from PsrfA was much higher in IS75 than in the undomesticated strain. When the degQIS75 allele was introduced into 3610, the expression from PsrfA increased and the frequency of PcomG-expressing cells also increased. Nevertheless, the mCherry fluorescence in IS75 is still more intense than that of the 3610 degQIS75 strain, despite the fact that the maximum rates of srfA expression are the same in both strains (Figure 3). This probably reflects the sustained srfA transcription rate in IS75 and the sharp decrease in the 3610 background. As expected, when degQ3610 was swapped into IS75 the mCherry fluorescence decreased to a level lower than that of wild-type IS75 and even lower than that of 3610 degQIS75, consistent with the fourfold higher amplitude of the PsrfA transcription rate curve in 3610 degQIS75 than in IS75 degQ3610 (Figure 3). These data demonstrate that the results presented above for promoter transcription rates were reflected on the single-cell level.
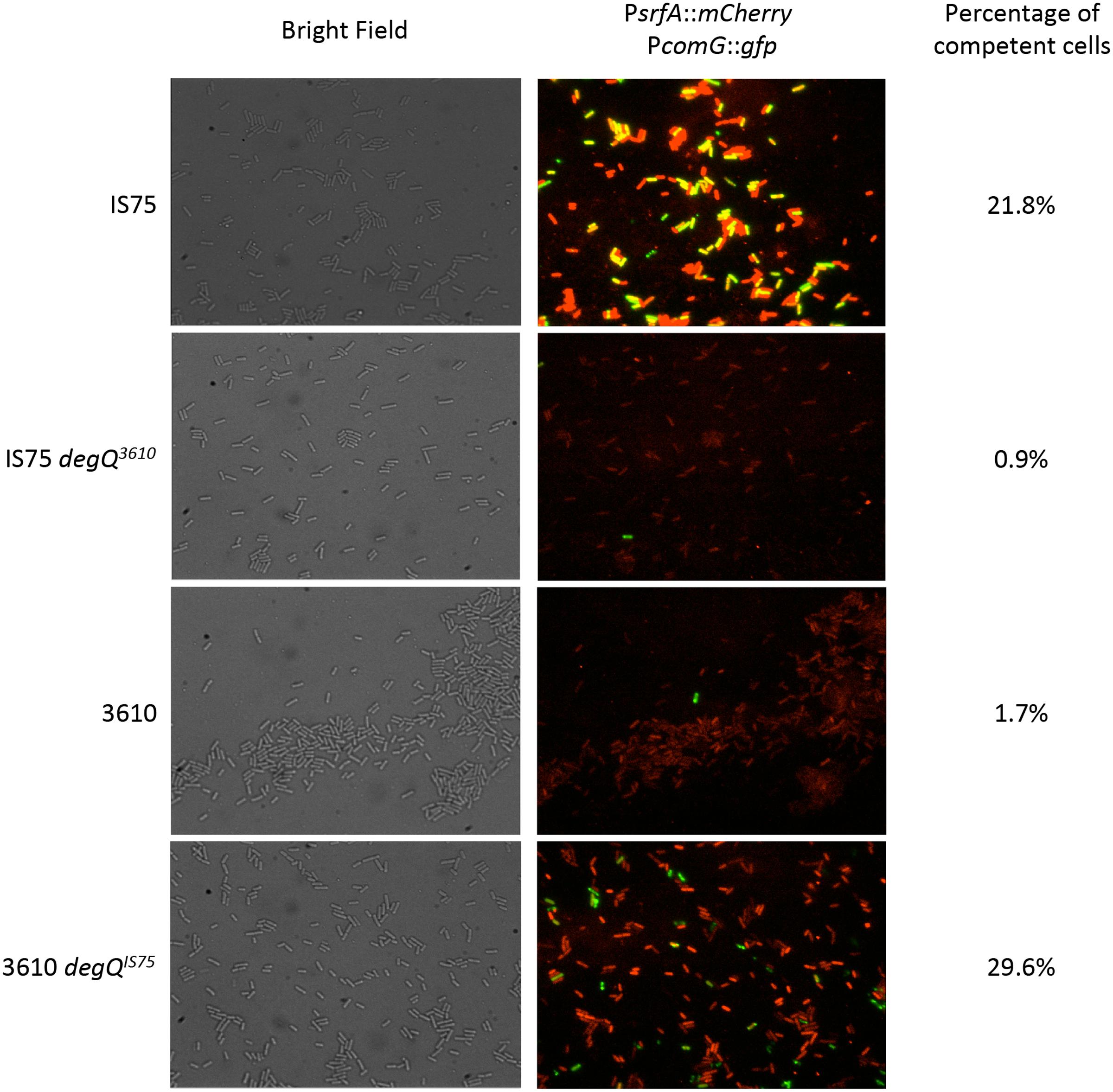
FIGURE 6. Single-cell expression of PsrfA-mCherry and PcomG-gfp. The indicated strains, all of which carried these two fusion constructs, were grown to the time of maximum K-state expression and samples were taken for microscopy. Representative images are shown. The 3610 and IS75 degQ3610 images were selected to include at least one K-state cell each. On the right are the percentages of K-state cells determined by examining at least 1200 cells for each strain. Strain numbers are presented in Supplementary Table S1.
We next sought to determine whether the K-state was expressed preferentially in cells that had accumulated more mCherry, expressed from PsrfA. For this, we measured the average pixel intensities of mCherry in K-state cells, identified by their GFP signals, compared to the intensities of non-K-state cells. Equal numbers of K-state and non-K-state cells were selected from each microscope field to minimize inter-field bias. Table 1 shows that at the time of these measurements, K-state cells in both the 3610 and IS75 backgrounds do not appear to exhibit a noticeably different mCherry signal than the non-K-state cells. This would suggest that variation in transcription from PsrfA may not be an important determinant of K-state transitions. However, this conclusion must be tempered by two considerations. First, the decision to enter the K-state was made, on the average, before these measurements were made. Second, the mCherry signal does not necessarily reflect the concentration of ComS.
RapP Influences the Sharp Downturn in srfA Expression in 3610
As noted above (Figure 3), the sharp downturn in srfA, and hence in comK and comG transcription, is clearly not dependent on DegQ and remains unexplained. We have considered the possibility that the differing kinetics of srfA transcription in 3610 and IS75 are influenced by the absence of a functional copy of swrA in 168-derivatives, because SwrA has been reported to modulate the binding of DegU-P to some promoters (Ogura and Tsukahara, 2012). To test this we used pMiniMAD2 to swap the functional and mutant swrA alleles between the two strains. No effect on srfA transcription was observed (not shown). Similarly, we wondered whether surfactin, the product of the srfA operon, would exert an effect on the kinetics of srfA transcription. Accordingly, we inactivated the srfA operon in the 3610 strain and no effect on PsrfA transcription was observed (not shown). Another strain difference is that 3610 carries a large plasmid, which encodes RapP, a phosphatase that acts on Spo0F-P (Parashar et al., 2013). It has been reported that the inactivation of rapP mitigates the sharp downturn in srfA transcription (Parashar et al., 2013; Omer Bendori et al., 2015). This result has been verified in our hands; we have consistently observed a shoulder in the srfA transcription rate curves in the rapP knockout strain, and we have found that the transcription rate does not drop to zero as it does in the rapP+ parent (Supplementary Figure S6A). Although the inactivation of rapP mitigates the downturn in srfA transcription in 3610, it does not phenocopy the sustained transcription observed in IS75 (compare Supplementary Figure S6A with Figure 4A). Clearly other genes must be involved that differ between the domesticated and undomesticated strains.
Given the more sustained transcription of srfA in the 3610 ΔrapP strain, we would expect the transcription of comK to increase due to stabilization of ComK. Instead, the transcription of comK was reduced (Supplementary Figure S6B). This unexpected effect was caused by a depressing effect of rapP inactivation on the basal level of comK transcription. This is shown by the expression of PcomK when auto-regulation is abrogated due to the absence of a functional copy of comK (Supplementary Figure S6C). In the comK rapP background, the basal expression of comK first increases as in the rapP+ strain but then abruptly declines.
These data have an interesting implication; although PS216, like IS75, lacks a rapP ortholog, the basal level expression of comK is similar in amplitude and overall kinetics in IS75, 3610, and PS216 (Supplementary Figure S7). It appears that selection has used both RapP-dependent and independent mechanisms to maintain the balanced increase and decrease in basal comK expression.
Discussion
The first important conclusion of this study is that the frequency of K-state cells in the population is controlled in natural isolates by a pathway that regulates the amount of DegU-P, providing one more illustration of the importance of using undomesticated strains as a way to approximate real-life biology (McLoon et al., 2011). This previously unrecognized pathway for K-state regulation, summarized in Figure 7, acts by controlling the transcription of the srfA operon, which in turn affects the stability of ComK. Although noise in the basal expression of comK selects cells for competence, it appears that the instability of ComK in undomesticated strains effectively decreases the fraction of cells that exceed the threshold level of ComK needed to activate the auto-induction of comK transcription. This pathway is lost in 168-derivatives due to a promoter mutation that reduces the transcription of degQ. Since this mutation is present in all of the sequenced 168 derivatives, it must have been present in 168 itself, and is responsible for the choice of the indole-requiring 168 strain as a highly transformable subject for further investigation (Spizizen, 1958). We do not know how the elevated level of DegU-P that is present in 3610 acts to decrease the transcription from PsrfA, nor do we know whether DegU-P acts directly on this promoter. Although degS and degU mutations that cause the accumulation of very high levels of DegU-P were known to inhibit the K-state (Msadek et al., 1990; Hahn and Dubnau, 1991), we show here for the first time that this mechanism is biologically relevant; DegU plays both positive and negative roles in regulation of the K-state in undomesticated strains. It is conceivable that the mucoidy, which can accompany increased DegU-P may itself compromise the binding of DNA to K-state cells, thereby contributing to the low transformability of undomesticated strains apart from the effect of DegU-P on PsrfA.
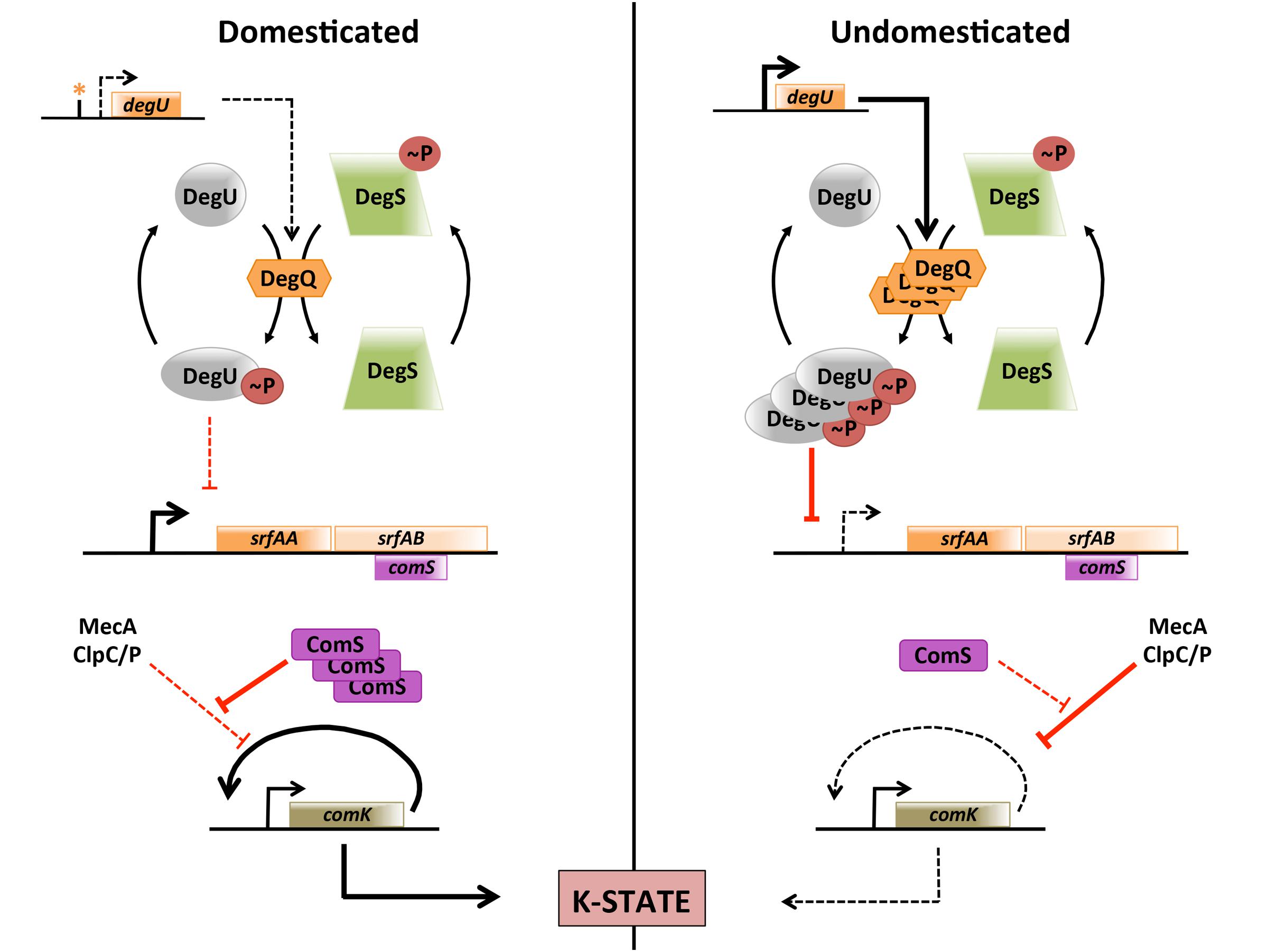
FIGURE 7. K-state regulation in domesticated and undomesticated strains. Dotted lines indicate weak or absent processes. In domesticated B. subtilis a promoter mutation decreases the rate of degQ transcription. The resulting low level of DegQ decreases DegU phosphorylation, relieving repression at the srfA promoter. As a result more ComS is synthesized and the degradation of ComK by MecA/ClpCP is decreased. It is not known whether the effect of DegU-P on srfA transcription is mediated by direct binding to the srfA promoter.
Interestingly, PdegQ is activated by ComA-P (Msadek et al., 1991), suggesting the following attractive mechanism. As the pheromone ComX accumulates, both comS and degQ would be transcribed. ComS would then stabilize ComK, helping to activate the auto-regulation of comK transcription, while DegQ would accumulate, increasing the concentration of DegU-P and eventually shutting down srfA transcription. However, as shown above (Figure 3B), the sharp down turn in srfA transcription that occurs in 3610 is not dependent on DegQ. Instead it is partly dependent on RapP, a phosphatase that acts on Spo0F-P, presumably restricting the rate of Spo0A-P accumulation. We conclude that this pleasing model does not seem to be true, at least under laboratory conditions.
Unphosphorylated DegU binds to sequences upstream from comK, helping ComK bind to its own promoter (Hamoen et al., 2000). We have shown here that DegU-P plays an important role in 3610, PS216 and presumably in other natural isolates, restricting the expression of srfA and hence of comK. Because DegU and DegU-P have respective positive and negative effects on K-state expression, the ratio of their concentrations must be critically controlled. Because srfA is essential for biofilm formation (Lopez et al., 2009), swarming motility (Kearns et al., 2004) and surface spreading (Kinsinger et al., 2003), the balance of phosphorylated and unphosphorylated DegU is also important for these forms of development, beyond the role of DegU-P in activating the expression of the hydrophobin BslA for biofilms (Hobley et al., 2013). In particular, swarming requires DegU-P (Verhamme et al., 2007). Biofilm formation is likewise inhibited when the amount of DegU-P is too high (Kobayashi, 2007; Verhamme et al., 2007). The mechanisms and upstream signals that control the level of DegU phosphorylation are not clear, but are certainly complex (Jers et al., 2011; Cairns et al., 2015) and important to elucidate.
As described above, Spo0A phosphorylation sets a temporal gate, opening and closing a window of opportunity for transitions to the K-state. Although phosphorylated and unphosphorylated DegU also play both positive and negative roles in the K-state, they may not control a temporal gate, because neither the inactivation of degS (Figure 4B) nor introduction of the non-phosphorylatable mutant form of DegU (D56N) (Supplementary Figure S5) affect the sharp downturn in srfA transcription in 3610. Perhaps instead, the ratio of DegU and DegU-P is simply adjusted in response to upstream signals to help set the probability of K-state development.
The inactivation of the RapP phosphatase in 3610 markedly decreases the basal expression from PcomK, thus reducing transitions to the K-state (Supplementary Figure S6). It is possible that RapP has an activity aside from its ability to dephosphorylate Spo0F-P and that an unknown gene in this strain down-regulates the basal transcription of comK in the absence of RapP. However, because Spo0A-P is known to repress the basal expression of comK, it seems more likely that in the absence of RapP, excess phosphorelay activity increases the production of Spo0A-P and represses PcomK. This is consistent with the kinetics of the basal expression shown in Supplementary Figure S7, which displays an initial rise identical to that in the rap+ strain, followed by sharp repression. However, regardless of the presence or absence of rapP, the basal expression of comK in IS75, PS216, and 3610 are similar (Supplementary Figure S7). Apparently, additional factors in IS75 and PS216 must serve to control the phosphorelay in the absence of RapP. Selection, whether in the laboratory or in nature, precisely modulates the formation of Spo0A-P, probably because of the multiple and dramatic consequences imposed by the presence of too much or too little of this key transcription factor. We have shown elsewhere that 168-derivatives carry a mutation in sigH, which decreases the activity of the phosphorelay compared to that of 3610 (Dubnau et al., 2016). Using pMiniMAD2, we have swapped sigH168 into a 3610 derivative lacking rapP and found that the basal level of comK transcription is still reduced (not shown). We suggest that some other difference between IS75 and 3610 is responsible for retarding Spo0A-P accumulation under K-state conditions.
Bacillus subtilis K-state cells are growth-arrested (Nester and Stocker, 1963; Haijema et al., 2001). The K-state appears to be a persistent state in which a trade off between the costs of growth-arrest and the expression of the competence machinery are balanced by fitness benefits due to tolerance in the face of environmental insults, e.g., exposure to antibiotics (Nester and Stocker, 1963; Johnsen et al., 2009; Hahn et al., 2015; Yuksel et al., 2016), as well as the ability to acquire useful genetic information. In the face of this trade-off, bet-hedging due to the bistable expression of the K-state, presumably helps to maximize fitness (Dubnau and Losick, 2006; Veening et al., 2008). It seems reasonable to assume that the frequency of K-state expression must be adjusted to obtain the biggest fitness advantage. Selection appears to have set the basal expression of comK and the kinetics of srfA expression to keep the frequency and timing of transitions to the K-state near an optimum. As noted above it has done this by at least two distinct mechanisms; RapP-dependent and RapP-independent. What appear to be important for fitness are the final kinetic behaviors of srfA and of comK basal expression. Although the transition frequency varies among undomesticated strains, it appears to be lower than in 168-derivatives, suggesting that the optimal bet-hedging frequency lies in a range below ∼15% (Figure 1).
Not only do the expressions of srfA, comK, and comG decline abruptly in PS216 and 3610, but so does transformability (Supplementary Figure S1). In other words competence, as traditionally defined, is restricted in these two strains to the time of entry to stationary phase, reaching a maximum at about T0 and decreasing thereafter. Perhaps this rapid decrease is due to the turnover of competence proteins, which might provide resources for the eventual growth of cells that are hunkered down in a persistent state. ComK expression is not terminated abruptly in IS75, so this turnover might have been overlooked. This would imply the existence of an additional K-state-related mechanism that has been lost during domestication, masking the natural transience of transformability.
Author Contributions
Both MM and DD contributed to the planning and interpretation of experiments and to the writing of this manuscript. MM carried out the experimental work.
Funding
This work was supported by NIH grant GM057720.
Conflict of Interest Statement
The authors declare that the research was conducted in the absence of any commercial or financial relationships that could be construed as a potential conflict of interest.
Acknowledgments
We thank all the members of our laboratory for constant discussions, suggestions and advice. We thank Dan Kearns and Inés Mandic-Mulec for their kind gifts of strains.
Supplementary Material
The Supplementary Material for this article can be found online at: http://journal.frontiersin.org/article/10.3389/fmicb.2016.01868/full#supplementary-material
References
Albano, M., Hahn, J., and Dubnau, D. (1987). Expression of competence genes in Bacillus subtilis. J. Bacteriol. 169, 3110–3117. doi: 10.1128/jb.169.7.3110-3117.1987
Berka, R. M., Hahn, J., Albano, M., Draskovic, I., Persuh, M., Cui, X., et al. (2002). Microarray analysis of the Bacillus subtilis K-state: genome-wide expression changes dependent on ComK. Mol. Microbiol. 43, 1331–1345. doi: 10.1046/j.1365-2958.2002.02833.x
Branda, S. S., Chu, F., Kearns, D. B., Losick, R., and Kolter, R. (2006). A major protein component of the Bacillus subtilis biofilm matrix. Mol. Microbiol. 59, 1229–1238. doi: 10.1111/j.1365-2958.2005.05020.x
Briley, K. Jr., Prepiak, P., Dias, M. J., Hahn, J., and Dubnau, D. (2011). Maf acts downstream of ComGA to arrest cell division in competent cells of B. subtilis. Mol. Microbiol. 81, 23–39. doi: 10.1111/j.1365-2958.2011.07695.x
Burbulys, D., Trach, K. A., and Hoch, J. A. (1991). Initiation of sporulation in B. subtilis is controlled by a multicomponent phosphorelay. Cell 64, 545–552. doi: 10.1016/0092-8674(91)90238-T
Burton, B., and Dubnau, D. (2010). Membrane-associated DNA transport machines. Cold Spring Harb. Perspect. Biol. 2:a000406. doi: 10.1101/cshperspect.a000406
Cairns, L. S., Martyn, J. E., Bromley, K., and Stanley-Wall, N. R. (2015). An alternate route to phosphorylating DegU of Bacillus subtilis using acetyl phosphate. BMC Microbiol. 15:78. doi: 10.1186/s12866-015-0410-z
Cohan, F. M., Roberts, M. S., and King, E. C. (1991). The potential for genetic exchange by transformation within a natural population of Bacillus subtilis. Evolution 45, 1383–1421. doi: 10.2307/2409888
Cozy, L. M., and Kearns, D. B. (2010). Gene position in a long operon governs motility development in Bacillus subtilis. Mol. Microbiol. 76, 273–285. doi: 10.1111/j.1365-2958.2010.07112.x
Dubnau, D., and Losick, R. (2006). Bistability in bacteria. Mol. Microbiol. 61, 564–572. doi: 10.1111/j.1365-2958.2006.05249.x
Dubnau, E. J., Carabetta, V. J., Tanner, A. W., Miras, M., Diethmaier, C., and Dubnau, D. (2016). A protein complex supports the production of Spo0A-P and plays additional roles for biofilms and the K-state in Bacillus subtilis. Mol. Microbiol. 101, 606–624. doi: 10.1111/mmi.13411
Durrett, R., Miras, M., Mirouze, N., Narechania, A., Mandic-Mulec, I., and Dubnau, D. (2013). Genome Sequence of the Bacillus subtilis biofilm-forming transformable strain PS216. Genome Announc. 1:e288-13. doi: 10.1128/genomeA.00288-13
Hadden, C., and Nester, E. W. (1968). Purification of competent cells in the Bacillus subtilis transformation system. J. Bacteriol. 95, 876–885.
Hahn, J., and Dubnau, D. (1991). Growth stage signal transduction and the requirements for srfA induction in development of competence. J. Bacteriol. 173, 7275–7282. doi: 10.1128/jb.173.22.7275-7282.1991
Hahn, J., Tanner, A. W., Carabetta, V. J. I., Cristea, M., and Dubnau, D. (2015). ComGA-RelA interaction and persistence in the Bacillus subtilis K-state. Mol. Microbiol. 97, 454–471. doi: 10.1111/mmi.13040
Haijema, B. J., Hahn, J., Haynes, J., and Dubnau, D. (2001). A ComGA-dependent checkpoint limits growth during the escape from competence. Mol. Microbiol. 40, 52–64. doi: 10.1046/j.1365-2958.2001.02363.x
Hamoen, L. W., Eshuis, H., Jongbloed, J., Venema, G., and van Sinderen, D. (1995). A small gene, designated comS, located within the coding region of the fourth amino acid-activation domain of srfA, is required for competence development in Bacillus subtilis. Mol. Microbiol. 15, 55–63. doi: 10.1111/j.1365-2958.1995.tb02220.x
Hamoen, L. W., Smits, W. K., de Jong, A., Holsappel, S., and Kuipers, O. P. (2002). Improving the predictive value of the competence transcription factor (ComK) binding site in Bacillus subtilis using a genomic approach. Nucleic Acids Res. 30, 5517–5528. doi: 10.1093/nar/gkf698
Hamoen, L. W., Van Werkhoven, A. F., Bijlsma, J. J., Dubnau, D., and Venema, G. (1998). The competence transcription factor of Bacillus subtilis recognizes short A/T-rich sequences arranged in a unique, flexible pattern along the DNA helix. Genes Dev. 12, 1539–1550. doi: 10.1101/gad.12.10.1539
Hamoen, L. W., Van Werkhoven, A. F., Venema, G., and Dubnau, D. (2000). The pleiotropic response regulator DegU functions as a priming protein in competence development in Bacillus subtilis. Proc. Natl. Acad. Sci. U.S.A. 97, 9246–9251. doi: 10.1073/pnas.160010597
Haseltine-Cahn, F., and Fox, M. S. (1968). Fractionation of transformable bacteria from competent cultures of Bacillus subtilis on renografin gradients. J. Bacteriol. 95, 867–875.
Hobley, L., Ostrowski, A., Rao, F. V., Bromley, K. M., Porter, M., Prescott, A. R., et al. (2013). BslA is a self-assembling bacterial hydrophobin that coats the Bacillus subtilis biofilm. Proc. Natl. Acad. Sci. U.S.A. 110, 13600–13605. doi: 10.1073/pnas.1306390110
Jers, C., Kobir, A., Sondergaard, E. O., Jensen, P. R., and Mijakovic, I. (2011). Bacillus subtilis two-component system sensory kinase DegS is regulated by serine phosphorylation in its input domain. PLoS ONE 6:e14653. doi: 10.1371/journal.pone.0014653
Johnsen, P. J., Dubnau, D., and Levin, B. R. (2009). Episodic selection and the maintenance of competence and natural transformation in Bacillus subtilis. Genetics 181, 1521–1533. doi: 10.1534/genetics.108.099523
Kearns, D. B., Chu, F., Rudner, R., and Losick, R. (2004). Genes governing swarming in Bacillus subtilis and evidence for a phase variation mechanism controlling surface motility. Mol. Microbiol. 52, 357–369. doi: 10.1111/j.1365-2958.2004.03996.x
Kinsinger, R. F., Shirk, M. C., and Fall, R. (2003). Rapid surface motility in Bacillus subtilis is dependent on extracellular surfactin and potassium ion. J. Bacteriol. 185, 5627–5631. doi: 10.1128/JB.185.18.5627-5631.2003
Kobayashi, K. (2007). Gradual activation of the response regulator DegU controls serial expression of genes for flagellum formation and biofilm formation in Bacillus subtilis. Mol. Microbiol. 66, 395–409. doi: 10.1111/j.1365-2958.2007.05923.x
Konkol, M. A., Blair, K. M., and Kearns, D. B. (2013). Plasmid-encoded ComI Inhibits competence in the ancestral 3610 strain of Bacillus subtilis. J. Bacteriol. 195, 4085–4093. doi: 10.1128/JB.00696-13
Leisner, M., Stingl, K., Radler, J. O., and Maier, B. (2007). Basal expression rate of comK sets a ‘switching-window’ into the K-state of Bacillus subtilis. Mol. Microbiol. 63, 1806–1816. doi: 10.1111/j.1365-2958.2007.05628.x
Lopez, D., Vlamakis, H., Losick, R., and Kolter, R. (2009). Paracrine signaling in a bacterium. Genes Dev. 23, 1631–1638. doi: 10.1101/gad.1813709
Maamar, H., and Dubnau, D. (2005). Bistability in the Bacillus subtilis K-state (competence) system requires a positive feedback loop. Mol. Microbiol. 56, 615–624. doi: 10.1111/j.1365-2958.2005.04592.x
Maamar, H., Raj, A., and Dubnau, D. (2007). Noise in gene expression determines cell fate in Bacillus subtilis. Science 317, 526–529. doi: 10.1126/science.1140818
Magnuson, R., Solomon, J., and Grossman, A. D. (1994). Biochemical and genetic characterization of a competence pheromone from B. subtilis. Cell 77, 207–216. doi: 10.1016/0092-8674(94)90313-1
McLoon, A. L., Guttenplan, S. B., Kearns, D. B., Kolter, R., and Losick, R. (2011). Tracing the domestication of a biofilm-forming bacterium. J. Bacteriol. 193, 2027–2034. doi: 10.1128/JB.01542-10
Mirouze, N., Desai, Y., Raj, A., and Dubnau, D. (2012). Spo0A∼P imposes a temporal gate for the bimodal expression of competence in Bacillus subtilis. PLoS Genet. 8:e1002586. doi: 10.1371/journal.pgen.1002586
Mirouze, N., Prepiak, P., and Dubnau, D. (2011). Fluctuations in spo0A transcription control rare developmental transitions in Bacillus subtilis. PLoS Genet. 7:e1002048. doi: 10.1371/journal.pgen.1002048
Msadek, T., Kunst, F., Henner, D., Klier, A., Rapoport, G., and Dedonder, R. (1990). Signal transduction pathway controlling the synthesis of a class of degradative enzymes in Bacillus subtilis: expression of the regulatory genes and analysis of mutations in degS and degU. J. Bacteriol. 172, 824–834.
Msadek, T., Kunst, F., Klier, A., and Rapoport, G. (1991). DegS-DegU and ComP-ComA modulator-effector pairs control expression of the Bacillus subtilis pleiotropic regulatory gene degQ. J. Bacteriol. 173, 2366–2377. doi: 10.1128/jb.173.7.2366-2377.1991
Mukherjee, S., Babitzke, P., and Kearns, D. B. (2013). FliW and FliS function independently to control cytoplasmic flagellin levels in Bacillus subtilis. J. Bacteriol. 195, 297–306. doi: 10.1128/JB.01654-12
Nakano, M. M., Magnuson, R., Myers, A., Curry, J., Grossman, A. D., and Zuber, P. (1991). srfA is an operon required for surfactin production, competence development, and efficient sporulation in Bacillus subtilis. J. Bacteriol. 173, 1770–1778. doi: 10.1128/jb.173.5.1770-1778.1991
Nakano, M. M., and Zuber, P. (1991). The primary role of ComA in establishment of the competent state in Bacillus subtilis is to activate the expression of srfA. J. Bacteriol. 173, 7269–7274. doi: 10.1128/jb.173.22.7269-7274.1991
Nester, E. W., and Stocker, B. A. D. (1963). Biosynthetic latency in early stages of deoxyribonucleic acid transformation in Bacillus subtilis. J. Bacteriol. 86, 785–796.
Ogura, M., and Tsukahara, K. (2010). Autoregulation of the Bacillus subtilis response regulator gene degU is coupled with the proteolysis of DegU-P by ClpCP. Mol. Microbiol. 75, 1244–1259. doi: 10.1111/j.1365-2958.2010.07047.x
Ogura, M., and Tsukahara, K. (2012). SwrA regulates assembly of Bacillus subtilis DegU via its interaction with N-terminal domain of DegU. J. Biochem. 151, 643–655. doi: 10.1093/jb/mvs036
Ogura, M., Yamaguchi, H., Kobayashi, K., Ogasawara, N., Fujita, Y., and Tanaka, T. (2002). Whole-genome analysis of genes regulated by the Bacillus subtilis competence transcription factor ComK. J. Bacteriol. 184, 2344–2351. doi: 10.1128/JB.184.9.2344-2351.2002
Omer Bendori, S., Pollak, S., Hizi, D., and Eldar, A. (2015). The RapP-PhrP quorum-sensing system of Bacillus subtilis strain NCIB3610 affects biofilm formation through multiple targets, due to an atypical signal-insensitive allele of RapP. J. Bacteriol. 197, 592–602. doi: 10.1128/JB.02382-14
Parashar, V., Konkol, M. A., Kearns, D. B., and Neiditch, M. B. (2013). A plasmid-encoded phosphatase regulates Bacillus subtilis biofilm architecture, sporulation, and genetic competence. J. Bacteriol. 195, 2437–2448. doi: 10.1128/JB.02030-12
Prepiak, P., and Dubnau, D. (2007). A peptide signal for adapter protein-mediated degradation by the AAA+ protease ClpCP. Mol. Cell 26, 639–647. doi: 10.1016/j.molcel.2007.05.011
Roggiani, M., and Dubnau, D. (1993). ComA, a phosphorylated response regulator protein of Bacillus subtilis, binds to the promoter region of srfA. J. Bacteriol. 175, 3182–3187. doi: 10.1128/jb.175.10.3182-3187.1993
Schägger, H., and von Jagow, G. (1987). Tricine-sodium dodecyl sulfate-polyacrylamide gel electrophoresis for the separation of proteins in the range from 1 to 100 kDa. Anal. Biochem. 166, 368–379. doi: 10.1016/0003-2697(87)90587-2
Schneider, C. A., Rasband, W. S., and Eliceiri, K. W. (2012). NIH Image to ImageJ: 25 years of image analysis. Nat. Methods 9, 671–675. doi: 10.1038/nmeth.2089
Singh, R. N., and Pitale, M. P. (1967). Enrichment of Bacillus subtilis transformants by zonal centrifugation. Nature 213, 1262–1263. doi: 10.1038/2131262a0
Smits, W. K., Eschevins, C. C., Susanna, K. A., Bron, S., Kuipers, O. P., and Hamoen, L. W. (2005). Stripping Bacillus: ComK auto-stimulation is responsible for the bistable response in competence development. Mol. Microbiol. 56, 604–614. doi: 10.1111/j.1365-2958.2005.04488.x
Spizizen, J. (1958). Transformation of biochemically deficient strains of Bacillus subtilis by deoxyribonucleate. Proc. Natl. Acad. Sci. U.S.A. 44, 1072–1078. doi: 10.1073/pnas.44.10.1072
Stanley, N. R., and Lazazzera, B. A. (2005). Defining the genetic differences between wild and domestic strains of Bacillus subtilis that affect poly-gamma-dl-glutamic acid production and biofilm formation. Mol. Microbiol. 57, 1143–1158. doi: 10.1111/j.1365-2958.2005.04746.x
Süel, G. M., Kulkarni, R. P., Dworkin, J., Garcia-Ojalvo, J., and Elowitz, M. B. (2007). Tunability and noise dependence in differentiation dynamics. Science 315, 1716–1719. doi: 10.1126/science.1137455
Turgay, K., Hahn, J., Burghoorn, J., and Dubnau, D. (1998). Competence in Bacillus subtilis is controlled by regulated proteolysis of a transcription factor. EMBO J. 17, 6730–6738. doi: 10.1093/emboj/17.22.6730
Turgay, K., Hamoen, L. W., Venema, G., and Dubnau, D. (1997). Biochemical characterization of a molecular switch involving the heat shock protein ClpC, which controls the activity of ComK, the competence transcription factor of Bacillus subtilis. Genes Dev. 11, 119–128. doi: 10.1101/gad.11.1.119
van Sinderen, D., Luttinger, A., Kong, L., Dubnau, D., Venema, G., and Hamoen, L. (1995). comK encodes the competence transcription factor, the key regulatory protein for competence development in Bacillus subtilis. Mol. Microbiol. 15, 455–462. doi: 10.1111/j.1365-2958.1995.tb02259.x
van Sinderen, D., and Venema, G. (1994). comK acts as an autoregulatory control switch in the signal transduction route to competence in Bacillus subtilis. J. Bacteriol. 176, 5762–5770. doi: 10.1128/jb.176.18.5762-5770.1994
Veening, J. W., Smits, W. K., and Kuipers, O. P. (2008). Bistability, epigenetics, and bet-hedging in bacteria. Annu. Rev. Microbiol. 62, 193–210. doi: 10.1146/annurev.micro.62.081307.163002
Verhamme, D. T., Kiley, T. B., and Stanley-Wall, N. R. (2007). DegU co-ordinates multicellular behaviour exhibited by Bacillus subtilis. Mol. Microbiol. 65,554–568. doi: 10.1111/j.1365-2958.2007.05810.x
Yang, M., Ferrari, E., Chen, E., and Henner, D. J. (1986). Identification of the pleiotropic sacQ gene of Bacillus subtilis. J. Bacteriol. 166, 113–119. doi: 10.1128/jb.166.1.113-119.1986
Keywords: K-state, competence, bistability, DegU, DegQ, ComK, strain domestication, Bacillus subtilis
Citation: Miras M and Dubnau D (2016) A DegU-P and DegQ-Dependent Regulatory Pathway for the K-state in Bacillus subtilis. Front. Microbiol. 7:1868. doi: 10.3389/fmicb.2016.01868
Received: 30 September 2016; Accepted: 07 November 2016;
Published: 22 November 2016.
Edited by:
José Eduardo González-Pastor, Centro de Astrobiología – Consejo Superior de Investigaciones Científicas, SpainReviewed by:
Kürşad Turgay, Leibniz University of Hanover, GermanyJonathan Dworkin, Columbia University, USA
Copyright © 2016 Miras and Dubnau. This is an open-access article distributed under the terms of the Creative Commons Attribution License (CC BY). The use, distribution or reproduction in other forums is permitted, provided the original author(s) or licensor are credited and that the original publication in this journal is cited, in accordance with accepted academic practice. No use, distribution or reproduction is permitted which does not comply with these terms.
*Correspondence: David Dubnau, ZHVibmF1ZGFAbmptcy5ydXRnZXJzLmVkdQ==