- 1Laboratoire de Biotechnologies Végétale et Microbienne, Unité Mixte de Recherche et d'Innovation en Sciences Agronomiques et Génie Rural, Institut National Polytechnique Felix Houphouët-Boigny, Yamoussoukro, Côte d'Ivoire
- 2Laboratoire Central de Biotechnologies, Centre National de la Recherche Agronomique, Abidjan, Côte d'Ivoire
- 3Unité de Formation et de Recherche en Agroforesterie, Université Jean Lorougnon Guédé, Daloa, Côte d'Ivoire
The use of arbuscular mycorrhizal fungal (AMF) inoculation in sustainable agriculture is now widespread worldwide. Although the use of inoculants consisting of native AMF is highly recommended as an alternative to commercial ones, there is no strategy to allow the selection of efficient fungal species from natural communities. The objective of this study was (i) to select efficient native AMF species (ii) evaluate their impact on nematode and water stresses, and (iii) evaluate their impact on cassava yield, an important food security crop in tropical and subtropical regions. Firstly, native AMF communities associated with cassava rhizospheres in fields were collected from different areas and 7 AMF species were selected, based upon their ubiquity and abundance. Using these criteria, two morphotypes (LBVM01 and LBVM02) out of the seven AMF species selected were persistently dominant when cassava was used as a trap plant. LBVM01 and LBVM02 were identified as Acaulospora colombiana (most abundant) and Ambispora appendicula, respectively, after phylogenetic analyses of LSU-ITS-SSU PCR amplified products. Secondly, the potential of these two native AMF species to promote growth and enhance tolerance to root-knot nematode and water stresses of cassava (Yavo variety) was evaluated using single and dual inoculation in greenhouse conditions. Of the two AMF species, it was shown that A. colombiana significantly improved the growth of the cassava and enhanced tolerance to water stress. However, both A. colombiana and A. appendicula conferred bioprotective effects to cassava plants against the nematode Meloidogyne spp., ranging from resistance (suppression or reduction of the nematode reproduction) or tolerance (low or no suppression in cassava growth). Thirdly, the potential of these selected native AMF to improve cassava growth and yield was evaluated under field conditions, compared to a commercial inoculant. In these conditions, the A. colombiana single inoculation and the dual inoculation significantly improved cassava yield compared to the commercial inoculant. This is the first report on native AMF species exhibiting multiple benefits for cassava crop productivity, namely improved plant growth and yield, water stress tolerance and nematode resistance.
Introduction
Cassava (Manihot esculenta Crantz) is a Central and South American native plant with tuberous roots rich in starch. It is a staple food for over 800 million people living in developing countries (Burns et al., 2010). In Côte d'Ivoire, it is the second most important food crop with an estimated annual production in 2013 of 2.5 million tons (FAO, 2014). This crop plays an important role in food security and income generation for many smallholder families. Despite its importance, cassava productivity is low in Côte d'Ivoire where yields are around 6 to 8t/ha compared to a global average level of 13t/ha (FAO, 2014). This low yield appears to be due to several factors. Firstly cassava cropping systems in Côte d'Ivoire are intensive and result in a rapid loss of soil fertility usually requiring long fallow periods (up to 7 years) to restore phosphorus and nitrogen levels. Secondly, cassava productivity is affected by pests, with root-knot nematodes being of major importance (Caveness, 1982; McSorley et al., 1983; Jatala and Bridge, 1990). Cassava yield losses due to nematode damage can be up to 87% (Caveness, 1982; IITA, 1990). Root-knot nematode damage can now be considered as a threat to the production of this major crop plant in Côte d'Ivoire where producers are mainly small farmers who cannot afford to buy nematicides. A third problem is the effect of climate change on crop productivity; notably the impact of drought, as unreliability of rainfall is a factor limiting cassava cultivation in tropical and subtropical areas (N'Guettia and Bernard, 1986). Consequently, although known for its ability to withstand drought, the net biomass production of cassava is reduced in times of water stress, irrespective of variety (Connor et al., 1981). Therefore, in order to sustain cassava productivity for farmers in tropical regions, it is important to develop a technology that can confer simultaneously on this plant (i) better growth and yield, (ii) a better tolerance to nematodes and (iii) a better tolerance to water deficit. Compounding the effects of disease and water stress is the increased vulnerability of rural families and smallholder cassava producers who often do not have access to appropriate technologies, services and markets. In developed countries, farmers rely extensively on industrial fertilizers to maximize crop productivity. Unfortunately, because of their financial and environmental costs, industrial fertilizers are not a solution for underdeveloped countries (Sanchez, 2002).
Cassava farmers could benefit from the multiple services offered by soil microorganisms such as arbuscular mycorrhizal fungi (AMF). Indeed, AMF belonging to the phylum Glomeromycota (Schüßler et al., 2001), constitute a multifunctional partner in the mutualistic interaction they develop with most land plants. The major function of AMF is to provide the mycorrhizal plant with water and essential nutrients such as phosphorus and nitrogen (He et al., 2003; Smith and Read, 2008). In addition to this nutritional function they provide, AMF can enhance plant tolerance to both biotic and abiotic stresses (Augé, 2001, 2004; Ortas et al., 2001; Plenchette et al., 2005; Al-karaki, 2006; Pozo and Azcón-Aguilar, 2007; Porcel et al., 2011; Augé et al., 2015). This multifunctional ability of the partner fungi has led to the development of mycorrhizal inoculants as biofertilizers in agriculture. Mycorrhizal inoculation has been applied for decades to promote better plant growth for various crop plants (Osonubi et al., 1995; Carretero et al., 2009). Cassava is highly mycorrhizal (Sieverding, 1989; Oyetunji and Osonubi, 2007) and there is evidence that AMF play an important role in increasing the productivity of cassava (Sieverding, 1989; Cardoso and Kuyper, 2006; Ceballos et al., 2013). Despite this positive impact of AMF inoculation on cassava productivity, and the known positive impact of mycorrhizal inoculation on root-knot nematode infection in crops such as yam and grapevine (St-Arnaud and Vujanovic, 2007; Tchabi, 2008; Hao et al., 2012; Veresoglou and Rillig, 2012), studies of AMF effects on root-knot nematode and water deficit in cassava remain scarce. In other studies, the impact of AMF on water stress has been documented for several crop plants, including cassava (Augé, 2001; Oyetunji et al., 2007). Although these studies point out the importance of AMF, there is no report of an AMF species that can (i) promote cassava growth and yield (ii) alleviate root-knot nematode damage and (iii) alleviate water stress. Therefore, the identification of AMF exhibiting these three traits could be a step forward to sustain cassava productivity in tropical regions.
The objective of this work was to recover native AMF species from smallholder farms and evaluate their potential to promote cassava growth and enhance resistance to root-knot nematode and water stress. Criteria such as ubiquity and relative abundance in field soils and baited soils were used to select native AMF species that were subsequently further evaluated for plant growth promotion in greenhouse and field conditions.
Materials and Methods
Plant Material
The improved cassava variety TME 7 “Yavo” provided by the National Agency for Rural Development Support (ANADER) in Yamoussoukro was used for the experiment. This variety has an 8-month cycle and is known to be resistant to the African cassava mosaic virus. In general, cassava leaves and roots are well developed after 4 months. At this stage, biotic and abiotic stresses can affect cassava growth parameters (Connor et al., 1981). Therefore all the experiments in greenhouse were run on 4-month-old cassava plants.
Methods
Selecting Potential Arbuscular Mycorrhizal Fungi for Inoculum Development
Recovery of AMF species from field soils
Soil samples were collected from cassava fields during the dry period in December 2012 in three agro-ecological zones (Azaguié, Yamoussoukro and Abengourou), which are considered important cassava production areas in Côte d'Ivoire (Chaleard, 1988; Kouadio et al., 2010). Twelve soil samples (1 kg each) were collected at a depth of 0–20 cm from cassava plant rhizospheres, using the sampling method of Huang and Cares (2004), from four fields in each agro-ecological zone (Table 1). AMF were extracted from 50 g of field soils by wet sieving (Gerdemann and Nicolson, 1963) using 4 sieves (45, 90, 125, and 500 μm). AMF species were identified as described below, and selected according to abundance, occurrence and ubiquity. Species occurrence was determined as the number of fields in which a particular species was found divided by the total number of fields. Each morphotype was maintained in monoculture using variety “Yavo” as a host, in 2-L pots containing soil + sand (3:1 v/v) sterilized by autoclaving.
Isolation of abundant AMF species by trapping
Field soils were used to trap AMF species using the cassava variety “Yavo.” The collected soils were mixed with a substrate composed of a mixture of soil and sand (3:1,v/v) sterilized at 120°C and 2 bars for 1 h on two successive days (Bâ et al., 2000) in a 1:1 ratio (v/v). Soils were placed in 10-L pots. The pots were watered every other day with 400 ml of water without fertilizer. After 4 months, cassava plants showed good physiological development. Soils were carefully recovered using a spatula after 4 months of cultivation. 50 g of soils were used to identify abundant and ubiquitous AMF morphotypes.
Morphological Identification of Selected AMF Spores
Spores were extracted by wet sieving and mounted between slide and coverslip in polyvinyl-lacto-glycerol and Melzer's solution (Morton et al., 1993). They were observed under a microscope and morphologically identified based on their color, shape, and composition of their walls (Schenck and Perez, 1990; see http://invam.wvu.edu/, http://www.zor.zut.edu.pl/ collection websites). Spores were photographed using a Motic BA310 Trinocular compound microscope.
Molecular Identification of Selected AMF Morphotypes
In order to confirm the morphological identification of the selected AMF species, PCR amplification was performed using primers LR1-LSUmAr/LR1- LSUmBr. For each selected species, 10 spores were collected in a 1.5 ml microfuge tube for DNA extraction using the DNeasy Plant Mini Kit (Qiagen). A first PCR amplification using primers LR1 and LSUmAr (van Tuinen et al., 1998; Stockinger et al., 2009) and a nested PCR using LR1 and LSUmBr primers (Krüger et al., 2009; Stockinger et al., 2010) were performed in 30 cycles (95°C 5 min; 94°C 1 min; 58°C 30 s; 72°C 45 s; 72°C 5 min; 25°C 1 s). The size of PCR products were checked on 1% agarose gels. For sequencing, the amplified PCR products were purified using a commercial kit (Nucleospin Extract II) and cloned using the TOPO TA Cloning® Kit (Invitrogen) according to the manufacturer's instructions. Three positive clones were selected for sequencing by GATC Biotech (Konstanz, Germany) using the directional Sanger method. Sequence analyses were done by Blast with NCBI and MAARJAM databases and phylogenetic analyses were performed using the software MEGA 6.06 and the neighbor-joining method (Saitou and Nei, 1987).
Mycorrhizal Inoculum Production
To produce inoculum, the selected strains were grown individually in the greenhouse in a sterile substrate containing cassava plants. For single inoculation, the inoculum (S1: A. colombiana or S2: A. appendicula) was in the form of 50 g of sterile substrate (soil + sand; 3:1, v/v) containing pieces of mycorrhizal roots, hyphae and about 350 AMF spores. The soil characteristics were (pH = 7.1; organic matter = 2.81%; total nitrogen = 0.15%; available phosphorus = 55 mg/kg) and for the sand (pH = 6.7; organic matter = 0.17%; total nitrogen = 0.01%; available phosphorus = 2 mg/kg). For dual inoculation, the two inocula (25 g each) were mixed to make 50 g.
Evaluation of the Impact of Selected AMF on Cassava Growth and Phosphorus Status in Greenhouse
Experimental design and culture condition
The greenhouse experiment was conducted comparing three AMF combinations (S1, S2, and S1S2) plus the control S0, and 6 replicates (completely randomized blocks) over 4 months. Pots were filled with 8 kg of substrate (soil + sand; 3:1, v/v). Each pot contained one cassava plant that was watered every other day with 400 ml of water without fertilizer.
Assessment of mycorrhizal development
For assessment of root colonization by AMF, fine cassava roots were sampled 4 months after planting, with three replicates per treatment. Each treatment contained three plants. Roots were rinsed and cut into 1–2 cm fragments. These roots fragments were cleared by boiling in 10% (w/v) KOH and stained with 0.05% (v/v) trypan blue in lactoglycerol according to the method of Phillips and Haymann (1970). Ten pieces of roots per plant were placed in glycerol (50%) between slide and coverslip (Kormanik and McGraw, 1982) and observed under an optical microscope. The colonized roots were observed and evaluated according to Trouvelot et al. (1986).
Assessment of the Mycorrhizal Inoculation on Cassava Growth and Phosphorus Levels in Greenhouse
Plant growth was assessed by measuring plant height and foliar surface area using Connor's et al. methods (Connor et al., 1981), and total fresh and dry matter. Plant total fresh matter was determined using an OHAUS balance and the dry matter after oven drying at 80°C for 48 h. Eight young cassava leaves were analyzed for P content after 4 months by the mineralization and calcination method using a Tecator model 40 instrument (Sidney, 1984). All measurements were done in triplicate.
Evaluation of the Impact of Selected AMF Species on Cassava Tolerance and Resistance to Root-Knot Nematode Meloidogyne spp. in Greenhouse
Preparation of nematode inoculum
The nematode inoculum was made using a population of Meloidogyne spp., isolated from tomato galls grown in a greenhouse. The inoculum was prepared by finely cutting infected tomato roots that were soaked in a jar containing NaClO (0.25%) and shaken for 2 min (Hussey and Barker, 1973). Nematode eggs and juveniles were collected on a 25 μm sieve, rinsed in sterile water and counted under a 40x binocular magnifier. A suspension of 1000 nematodes (juveniles + eggs) was added to each cassava plant (each pot).
Experimental design and culture condition
A 4 × 2 factorial experiment with three replicates and completely randomized design was carried out in the greenhouse over a 4-month period. One factor was the AMF treatment: each selected AMF strain was used either in single inoculation (S1, S2) or dual inoculation (S1S2) and a non-inoculated control (S0). The other factor was inoculation with root knot nematodes, either at the same time as the AMF inoculation (I2), or 1 month after AMF inoculation (I4). Inoculation with nematodes was achieved by loading aliquots of 1000 freshly hatched juveniles and eggs suspended in distilled water into 5-cm-deep holes equidistant around each plant. Pots were filled with 8 kg of substrate (soil + sand; 3:1, v/v). Each pot contained one cassava plant that was watered every other day with 400 ml of water without fertilizer.
Assessment of the mycorrhizal inoculation impact on nematode population
At the end of the experiment, nematodes and eggs were counted according to Daykin and Hussey (1985). The total content of phenols, which are an indicator of plant defense compounds against nematode attack, in roots was estimated using a colorimetric method (Singleton et al., 1999). Total phenol content was measured after 2 h at room temperature incubation by absorbance at 765 nm, measured in a Jenway 7315 Spectrophotometer. The quantification was done using a gallic acid calibration curve. Biomass (total fresh matter, total dry matter) and phosphorus in cassava leaves were also determined as described above.
Impact of the Selected AMF Inoculation on Cassava Resistance to Water Stress in Greenhouse
Experimental design and culture condition
Before the main experiment, a pot containing 8 kg of soil was filled with water until saturation. Excess water was then allowed to drain over 2 days and field capacity (FC) was measured according to Colombani et al. (1973). A 4 × 2 factorial experiment with three replicates and a completely randomized design was carried out in a greenhouse over a 4-month period. One factor was the AMF treatment: each selected AMF strain applied either in single inoculation (S1, S2) or dual inoculation (S1S2), and an non-inoculated control (S0). The other factor was water regime. All plants were watered to 100% of FC for 2 months after planting. They were then divided in two groups for the remaining 2 months. One group was regularly watered to 100% FC while the other was watered to 10% of FC. That watering regime corresponded to 400 mm of water/year, which can be considered a severe water stress to cassava (FAO, 2013). The total number of plants for the experiment was 48. Mycorrhizal abundance was estimated on roots harvested monthly using the Trouvelot et al. (1986) method. Foliar surface areas were measured on the 3rd and 4th month, and biomass was determined after 4 months, as described previously. The chlorophyll a content of young plant leaves was determined using the method of Arnon (1949). Soluble sugar content (TS) of young leaves, a measure of osmoprotection during water stress, was determined according to Dubois et al. (1956) using the Jenway 7315 spectrophotometer.
Cassava Plant Inoculation under Field Conditions
Study area
An experimental area of about 2500 m2 was set up in Duokro, 15 km from Yamoussoukro in Côte d'Ivoire, to test the effect of local and commercial strains of mycorrhizae on colonization, and cassava yield during the 2015–2016 season. The average temperature in this region over the season was 32 ± 2°C, average total annual rainfall is 1495 mm and average annual humidity is 79 ± 12%.
Experimental design
The field experiment was established using a randomized complete block design with five inoculation treatments: Ambispora appendicula (T1), Acaulospora colombiana (T2), the dual inoculant A. colombiana–A. appendicula (T3), a commercial inoculum Mykepro P501 produced by PremierTech biotechnologies (T4), and a non-inoculated control (T5). The commercial inoculant is composed of a single species Rhizophagus intraradices. Mineral fertilizer (30 kg N/ha, 20 kg P/ha and 50 kg K/ha) was applied to the non-inoculated control plots. For each treatment, there were three replicates, resulting in a total of 18 plots. Cassava was planted in ridges 80 cm wide and 20 cm high, separated by 20-cm wide furrows, following the contour. The blocks were arranged perpendicular to the slope. Each plot contained 40 plants, including 16 inoculated plants and 2 lines of 24 plants curbs to limit edge effects. The planting density was 10,000 plants/ha. 25-cm long cassava cuttings, 1.5–2.5 cm thick, with 5–6 nodes were planted in 20-cm deep holes. Cuttings were inserted diagonally in order to promote sprouting. No irrigation water or pesticides were applied. Cassava plant inoculation was done on farm. For the native inoculum, each plant was inoculated with 100 g of inoculums containing 1000 spores + mycorrhizal roots. For the commercial inoculum 6 g containing 3000 spores + mycorrhizal roots was added to each plant, corresponding to triple the dose applied in temperate zones.
Assessment of cassava tuber yield
Tubers were harvested on March 10, 2016. Fresh tubers were weighed and the yield converted to t/ha. When a significant difference was observed in yield compared to plots without AMF, the gain (G) in yield was calculated according to the formula:
Statistical Analyses
All experimental data in greenhouse were subjected to statistical analyses by performing either one or two-way analysis of variance (ANOVA) using Statistica 7.1. The significance of the treatment effects was determined using LSD Fisher test with P = 0.05.
All field experiment data were analyzed by ANOVA. Fisher's LSD test was also used to determine whether or not treatments were different from each other at P < 0.05.
Results
Selection and Identification of Potential Useful AMF Species for Cassava Crop Inoculation
Using spore characteristics, several AMF species were identified (Table 2) in the three agricultural zones. The species Acaulospora scrobiculata, A. colombiana, A. appendicula, Claroideoglomus etunicatum, Glomus glomerulatum and an unidentified species Glomus Sp2 were abundant at various levels in all three zones. However, when the cassava cultivar Yavo was inoculated with field soils in greenhouse, only A. colombiana, A. appendicula were confirmed in all soils, with A. appendicula having low abundance (Table 2). These two morphotypes (LBVM01 and LBVM02), which were present in all soils and also abundant in trapped communities, were considered as good candidates for cassava inoculation. They were initially identified based on morphological criteria using PVLG and Melzer's reagent as Acaulospora sp. and Ambispora sp. (Figure 1). An expected 700 bp fragment was amplified from each morphotype. Both BLAST and phylogenetic analyses allowed the identification of the morphotype LBVM01 as A. colombiana (Genbank accession number KX168435) and the other LBVM02 as A. appendicula (Genbank accession number KX168436) (Table 3; Figure 2).
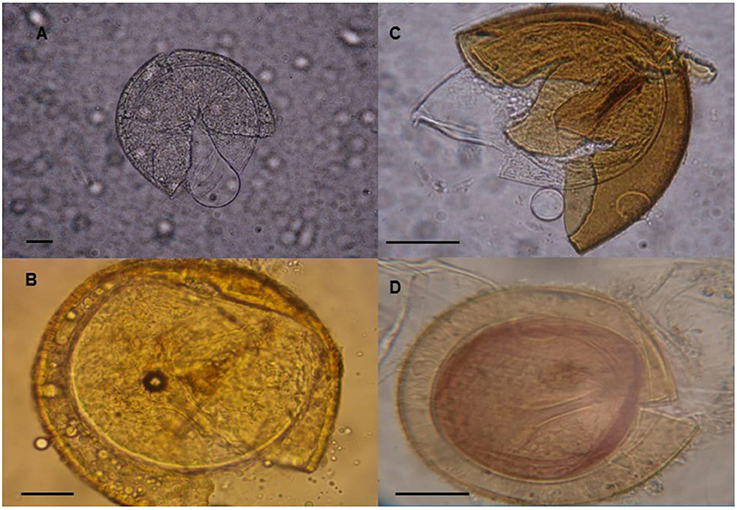
Figure 1. Spores of arbuscular mycorrhizal fungi used in this study. (A) Acaulospora colombiana and (C) Ambispora appendicula stained with lactoglycerol polyvinyl. (B) Acaulospora colombiana and (D) Ambispora appendicula stained with Melzer's reagent. Scale bar = 1/25 μm.
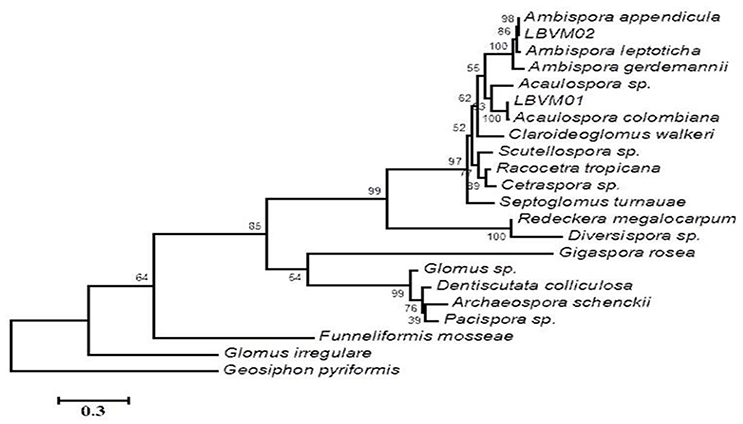
Figure 2. Phylogenetic identification of LBVOl and LBVM02 isolated from field soils. SSU-ITS-LSU gene sequences from AMFs species Ambispora appendicula (FN547527), Ambispora leptoticha (KC166277), Ambispora gerdemanii (KC166282), Acaulospora sp. (HF56794), Acaulospora colombiana (FR750063), Scutellospora sp. (AF396818), Racocetra tropicana (GU385898), Cetraspora sp. (HM565946), Septoglomus tumauae (KF060327), Redeckera megalocarpum (NR121478), Diversispora sp. (KJ850185), Gigaspora rosea (U60451), Glomus sp. (AB326023), Dentiscutata colliculosa (GQ376067), Archaeospora schenckii (KP144303), Claroideoglomus walker (KP191492); Pacispora sp. (JQ182768), Funneliforrnis mossae (KM360085), Glomus irregulare (GU585513) and Geosiphon pyriformis (JX535577) were used for comparison. The tree was constructed by the neighbor-joining method using Mega version 6.
Effect of A. colombiana and A. appendicula Single and Dual Inoculation on Cassava Growth and P Uptake
After 4 months of culture in greenhouse conditions (Table 4), all cassava plants inoculated (singly or dually) with A. colombiana and A. appendicula were mycorrhizal. Frequencies and intensities of mycorrhization did not differ significantly between single and dual inoculation (frequency of 26.7 and 48.3%, and intensity of 14.5 and 38.7%, respectively). No mycorrhizal structures were observed in cassava control plants. The foliar P content of cassava plants inoculated with A. colombiana was significantly (p = 0.002) improved (1.3-fold) compared to the non-inoculated control, whereas the A. appendicula single inoculation had no impact. The foliar P content of dual inoculated plants was significantly improved (1.5-fold) compared to the control. The growth parameters (plant height, foliar surface area, total dry and total fresh matter) of A. colombiana singly inoculated cassava plants were significantly improved (p = 0.040; p = 0.008; p = 0.000; p = 0.001, respectively) after 4 months in the greenhouse. The A. appendicula single inoculation had significant impact only on total fresh matter. However, dual inoculation significantly improved all parameters.
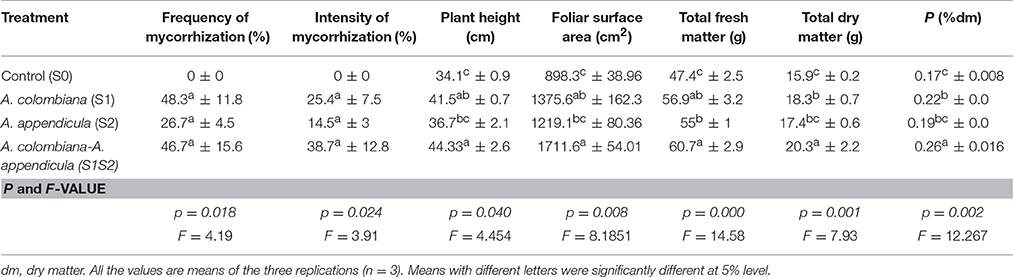
Table 4. Impact of A. colombiana and A. appendicula single and dual inoculation on phosphorus nutrition and cassava growth after 4 months.
Susceptibility of A. colombiana and A. appendicula Inoculated Cassava Plants to Root-Knot Nematode Meloidogyne spp
Four months after single or dual inoculation with A. colombiana or A. appendicula, in the presence of the nematode Meloidogyne spp., all treated cassava plants were mycorrhizal (Table 5). However, the frequencies and intensities of mycorrhization were significantly lower (p = 0.002 for both) in the roots of cassava plants that were co-inoculated with the nematode Meloidogyne spp. In this condition, the presence of A. colombiana and A. appendicula as single or dual inoculant significantly reduced nematode egg and population densities. In this experiment, none of the three mycorrhizal inoculation methods significantly affected foliar P contents. However, the single inoculation using A. appendicula and the dual inoculation significantly increased cassava total fresh biomass. When the nematodes were added 1 month after AMF inoculation, mycorrhizal root colonization levels were still high after 4 months (frequency 20 and 36.7%, and intensity 15 and 21.5% for single and dual inoculation, respectively). In this case, only the single inoculation with A. colombiana significantly (p = 0.006) reduced the number of nematode eggs in the cassava roots. Also, only A. colombiana as a sole inoculants significantly increased the foliar P content. However, only the dual inoculation increased cassava plant fresh and dry biomass. Phenol contents of AMF pre-inoculated cassava roots were significantly higher than the controls (not inoculated with AMF).
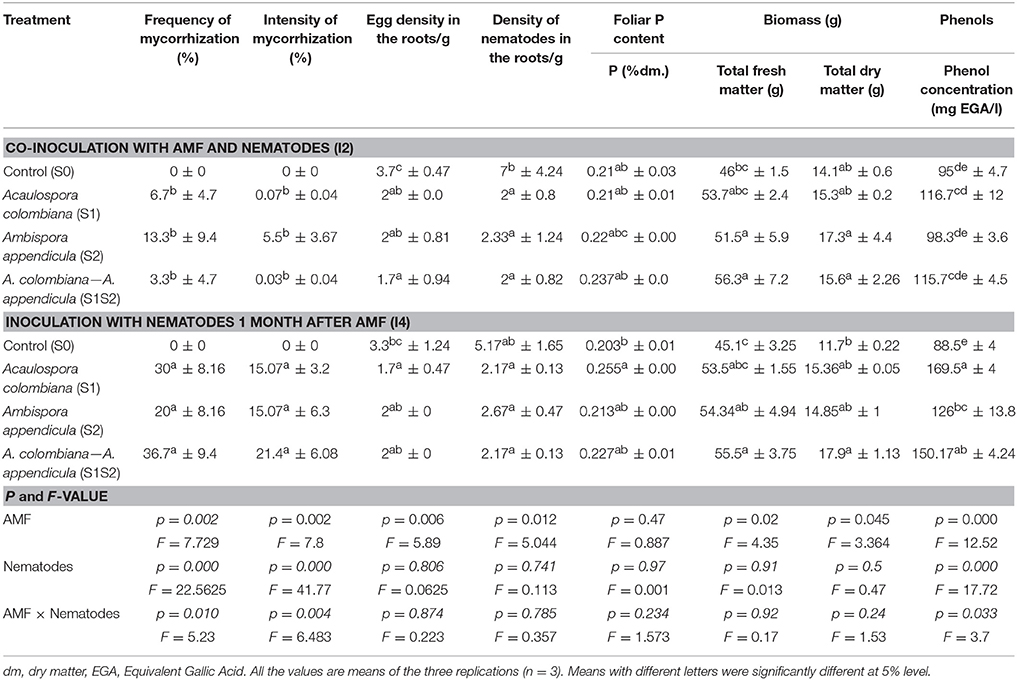
Table 5. Impact of A. colombiana and A. appendicula single and dual inoculation on nematode communities and cassava plant growth.
Development of Single and Dual A. colombiana and A. appendicula Inoculated Plants during Water Stress
When cassava plants were well watered (100% FC), mycorrhizal colonization significantly increased during the first 2 months when inoculated with A. colombiana (from 23 to 46.7%) and with the dual inoculation (10 to 23.7%), but not with A. appendicula (constant at 10%) (Table 6). One month after water stress was initiated (10% FC), mycorrhizal colonization declined significantly in the cassava roots for all mycorrhizal treatments (Table 6). After 2 months of water stress the same trend was observed for all mycorrhizal treatments. However, A. colombiana colonization of cassava roots remained stable at 26.7% in the fourth month and this was significantly higher (p = 0.049) than A. appendicula. Under water stress, the presence of A. colombiana had a significant positive impact on the growth parameters (total dry matter, fresh matter and foliar surface area) (Table 7). In comparison, neither the single inoculation with A. appendicula nor the dual inoculation positively impacted these growth parameters. Moreover, under this severe water stress, the cassava plants inoculated with A. colombiana significantly improved all functional traits measured, including chlorophyll a (0.209 mg/g FM) and total sugar content (496 μmol/mg FM), compared to non-inoculated plants (Table 7).
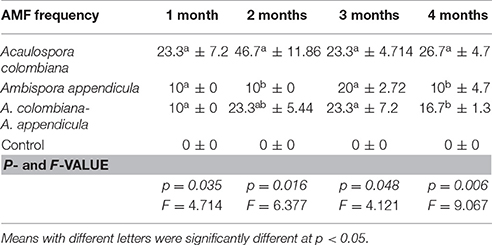
Table 6. Mycorrhizal frequency evolution from well-watered regime (100% of field capacity) at 1 to 2 months, to drought (10% of field capacity) at 2–4 months.
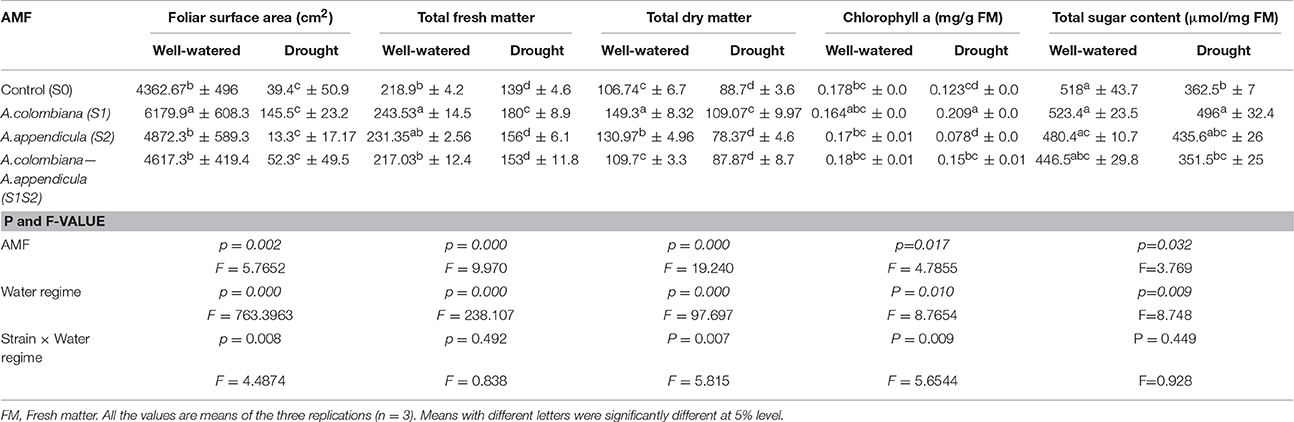
Table 7. Impact of A. colombiana and A. appendicula single and dual inoculation on cassava plant growth and physiological traits 2 months after initiation of water stress.
Impact of Mycorrhizal Inoculation on Cassava Yield under Field Conditions
The impact on cassava yields of the native AMF in single and dual inoculation was assessed in comparison to the commercial inoculant MykePro and the standard chemical fertilizer application (Figure 3). The results showed that the chemical fertilizer NPK significantly improved cassava yield (11.38 t/ha) compared to non-inoculated control (8.21 t/ha). This represents a yield gain of 38.5%. Of the AMF treatments, only A. colombiana single inoculation and the dual inoculation significantly (p = 0.003) improved cassava yield (9.58 and 9.81 t/ha, respectively) compared to non-inoculated control (8.21 t/ha). This represents a yield gain of 19.4% for the dual inoculation and 16.6% for A. colombiana. A. appendicula and the commercial inoculant had no significant impact on cassava yield compared to the non-inoculated control.
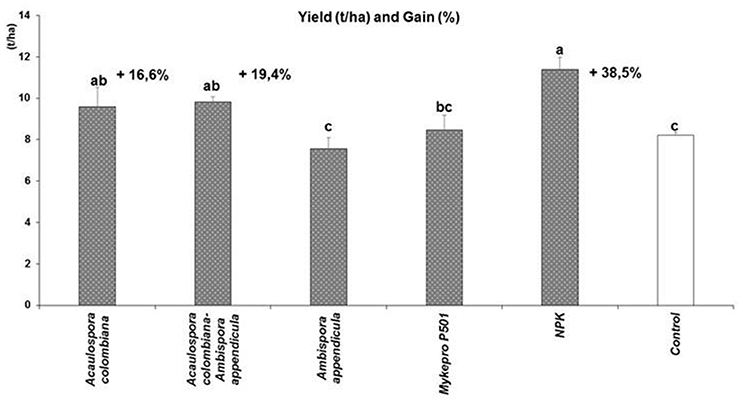
Figure 3. Yield and yield gain of fresh cassava tubers as affected by inoculation with arbuscular mycorrhizal fungi or application of chemical fertilizer, under field conditions. Columns with the same letter are not significantly different at P = 0.05.
Discussion
This work aimed to select an abundant native AMF capable of improving cassava crop productivity via several mechanisms, namely improved plant growth, water stress tolerance and nematode resistance. This is an improvement on previous studies, which tended to focus on one aspect affecting cassava yield, without studying the possible interactions with nematodes and drought.
During this study, there was a difference in the way the two native AMF species impacted cassava plant growth in greenhouse conditions. It was shown that only A. colombiana significantly increased the plant growth parameters, such as foliar surface area, plant height and biomass (Table 3). It has been reported that several factors, such as environmental conditions and functional diversity, can affect nutrient exchange between the fungi and plant partners (Walder and van der Heijden, 2015). The experimental conditions used in this work might have been favorable to A. colombiana, which significantly improved P uptake compared to A. appendicula. Variable effects among endogenous single species due to the use of different culture media were also observed in other studies (Williams et al., 2012; Ortas and Ustuner, 2014). Also, the two native AMF species may differ in terms of regulation of genes involved in P uptake. Such observations were made when maize plants were individually inoculated with different AMF species (Tian et al., 2013). In our study, single inoculation with A. appendicula had no effect. Meanwhile dual inoculation with both species positively improved P uptake and cassava plant growth. Similar observations were made when citrus was treated with different AMF species using a dual inoculation approach (Ortas and Ustuner, 2014). It could mean that when used together as dual inoculants, the two native AMF species induce phosphate transporters in cassava plants, as reported for different AMF species used to inoculate maize plants (Tian et al., 2013).
The study on the interaction between the two native AMF and Meloidogyene spp. in greenhouse revealed that negative effects of the AMF against the nematode (reduction of egg and nematode densities) were clearly observed, whether or not AMF and nematodes were co- or post-inoculated (1 month later). Interestingly, the presence of the nematode exerted a negative effect on the AMF, by reducing mycorrhizal intensities and frequencies in the case of simultaneous inoculation. Both types of interactions between nematode and AMF have already been reported. These mutual negative effects occur when fungi and nematodes are competing for space and nutrients (Schouteden et al., 2015). For example, the fungus Scutellospora heterogama exerted a biocontrol effect on the sedentary endoparasitic nematode Meloidogyne incognita (reproduction was reduced) only when it was pre-inoculated whereas co-inoculation had no effect (Dos Anjos et al., 2010). Such observations have also been made for migratory endoparasitic nematodes. For example, it was shown that Radopholus similis and Pratylencus coffeae affected the frequency of Funneliformis mosseae colonization in banana, but not the intensity (Elsen et al., 2003a,b). In contrast, root colonization by R. irregularis in vitro banana plantlets was not affected either by R. similis (Koffi et al., 2013) or by P. coffeae in transformed carrot roots (Elsen et al., 2003c).
Overall, in the presence of these native AMF, cassava plants continued to grow even though nematodes were present. It appears that the mycorrhizal cassava plants were either resistant (e.g., suppression or reduction of the nematode reproduction) or tolerant (low or no suppression in cassava plant growth) to nematodes, as reported in other studies (Hussey and Roncadori, 1982; Affokpon et al., 2011). However, the mechanism of the bioprotection conferred to cassava plants by the native AMF against the root-knot nematode Meloidogyne spp. is not yet understood. It may be due to the production of phytochemical inhibitors of nematodes, as was observed elsewhere. Indeed, in this work it was observed that phenolic compounds were significantly increased in cassava plant roots when nematodes were post-inoculated. Previous work has shown production of phenolic compounds to be a plant defense mechanism against nematode attacks (Zhu and Yao, 2004; Xu et al., 2008). Elsewhere, accumulation of phenolic compounds has been observed in mycorrhizal Impatiens balsamina, an ornamental plant, in presence of M. incognita (Banuelos et al., 2014). Singh et al. (1990) concluded that the pre-inoculation of plants, coupled with biochemical changes are responsible for resistance to nematodes. In contrast, when the cassava plants were co-inoculated with the AMF and the nematodes, there was no significant increase in phenolic compounds. Obviously there may be another mechanism involved in the inhibition of Meloidogyne spp. activity. For example, there was an up regulation of mycorrhiza-induced plant defense genes against the ectoparasitic nematode Xiphinema index in grapevine plants pre-inoculated with R. intraradices (Hao et al., 2012).
Besides its capacity to promote cassava growth and enhance resistance and tolerance to the root-knot nematode, A. colombiana also conferred water stress tolerance to cassava plants under severe drought condition. This AMF species significantly improved cassava plant growth under water stress. It was observed that mycorrhizal frequencies decreased gradually during the period of drought for all treatments, compared to the 100% FC water regime. However, despite severe water stress, the mycorrhizal colonization frequencies of A. colombiana remained higher than the control and stable over time. This water stress tolerance could be the result of A. colombiana promoting specific plant stress resistance response during the drought period, as suggested by others (Augé, 2001). For example the presence of this AMF may enhance photosynthetic activity due to the high levels of chlorophyll a and total sugars in A. colombiana colonized cassava plants compared to non-mycorrhizal plants (Mathur and Vyas, 1995).
Overall, this study clearly showed the multiple functions of the native AMF species A. colombiana. Importantly, A. colombiana was dominant in all three study areas and was persistently found and easily produced in trap culture. Abundance and persistence of AMF species are very important for efficient AMF species selection to ensure potential inocula are not lost during trap culture propagation (Trejo-Aguilar et al., 2013). This is essential, as the most widespread method for inoculum propagation is the use of trap plants (Berruti et al., 2016).
Under field conditions, A. colombiana showed a good potential for improving cassava productivity. The dual inoculation using the two native AMF species also increased cassava yield under field conditions. This opens up the possibility of using single and dual inoculation of these two native AMF species to improve cassava productivity in the field. During this study the native inoculants performed better than the commercial inoculant. Indeed, the origin and the composition of AMF are very important factors to take into account for inoculum development (Berruti et al., 2016). It has been shown that native AMF have higher efficiency in terms of plant protection against nematode (Affokpon et al., 2011) and stress tolerance (Ruiz-Lozano and Azcón, 2000) than commercial inoculants generally used in the field. Commercial inoculants are generally comprised of AMF species that can be considered as exotic species in tropical and subtropical regions (Oliveira et al., 2005; Schreiner, 2007). One main drawback in the use of commercial inoculants is the fact that the species used might not survive the competition with local AMF communities. Rodriguez and Sanders (2015), who discussed this issue, recommended research to understand local communities through metagenomics and genetic studies. The use of native inoculants comprised of native AMF like A. colombiana is highly recommended as an alternative to exotic species (Oliveira et al., 2005). As a persistent and abundant generalist, A. colombiana may have been a good competitor under field conditions, as in the greenhouse. Moreover, since commercial inoculants can be either ineffective (Faye et al., 2013) or badly formulated (Corkidi et al., 2004), the use of A. colombiana is more likely to be affordable and effective for cassava farmers in tropical and subtropical regions.
In conclusion, this study clearly points out the potential of A. colombiana as a native AM fungus suitable for inoculating cassava. The process developed in this study to select the multipurpose (plant growth improvement, water stress tolerance and nematode resistance) AMF species A. colombiana for cassava could be applied to efficiently select effective AMF inocula for other crops.
Author Contributions
This work is done is the scope of a project in the Laboratoire de Biotechnologie Végétale et Microbienne under the supervision of ZA. SJ designed and run all the experiments as a Ph.D. student. KC helped in designing the PCR amplification protocols and sequence analyses. VR was a cosupervisor of this work as a collaborator on this project. ZA is the coordinator of the project and SJ supervisor.
Funding
We are grateful to the West African Agricultural Productivity Program (WAAPP) for funding the Project IVO-RHIZE (Projects 047/PPAAO/2012 and 028/CS/PPAAO/2015), within which this study was conducted. We are also grateful to this program for funding SJ scholarship and the Agence Universitaire de la Francophonie (AUF) for awarding him a biotechnology mobility program.
Conflict of Interest Statement
The authors declare that the research was conducted in the absence of any commercial or financial relationships that could be construed as a potential conflict of interest.
Acknowledgments
We would like to thank Dr. Diederik van Tuinen for hosting SJ at the Agroécologie, AgroSup Dijon, CNRS, INRA, Univ. Bourgogne Franche-Comté 17 rue Sully BP 86510 21065 Dijon Cedex, France.
References
Affokpon, A., Coyne, D. L., Lawouin, L., Tossou, C., Agbèdè, R. D., and Coosemans, J. (2011). Effectiveness of native West African arbuscular mycorrhizal fungi in protecting vegetable crops against root-knot nematodes. Biol. Fertil. Soils. 47, 207–217. doi: 10.1007/s00374-010-0525-1
Al-karaki, G. N. (2006). Nursery inoculation of tomato with arbuscular mycorrhizal fungi and subsequent performance under irrigation with saline water. Sci. Horticult. 109, 1–7. doi: 10.1016/j.scienta.2006.02.019
Arnon, D. L. (1949). Copper enzymes in isolated chloroplasts. Polyphenoloxidase in Beta vulgaries. Plant. Physiol. 24, 1–15.
Augé, R. M. (2001). Water relations, drought and vesicular-arbuscular mycorrhizal symbiosis. Mycorrhiza 11, 3–42. doi: 10.1007/s005720100097
Augé, R. M. (2004). Arbuscular mycorrhizae and soil/plant water relations. Can. J. Soil Sci. 84, 373–381. doi: 10.4141/S04-002
Augé, R. M., Toler, H. D., and Saxton, A. M. (2015). Arbuscular mycorrhizal symbiosis alters stomatal conductance of host plants more under drought than under amply watered conditions: a meta-analysis. Mycorrhiza 25, 13–24. doi: 10.1007/s00572-014-0585-4
Bâ, A. M., Plenchette, C., Danthu, P., Duponnois, R., and Guissou, T. (2000). Functional compatibility of two arbuscular mycorrhizae with thirteen fruit trees in Senegal. Agroforestry Syst. 50, 95–105. doi: 10.1023/A:1006482904452
Banuelos, J., Alarcón, A., Larsen, J., Cruz-Sánchez, S., and Trejo, D. (2014). Interactions between arbuscular mycorrhizal fungi and Meloidogyne incognita in the ornamental plant Impatiens balsamina. J. Soil Sci. Plant Nutr. 14, 63–74. doi: 10.4067/S0718-95162014005000005
Berruti, A., Lumini, E., Balestrini, R., and Bianciotto, V. (2016). Arbuscular mycorrhizal fungi as natural biofertilizers: let's benefit from past successes. Front. Microbiol. 6:1559. doi: 10.3389/fmicb.2015.01559
Burns, A., Gleadow, R., Cliff, J., Zacarias, A., and Cavagnaro, T. (2010). Cassava: the drought, war and famine crop in a changing world. Sustainability 2, 3572–3607. doi: 10.3390/su2113572
Cardoso, I. M., and Kuyper, T. W. (2006). Mycorrhizas and tropical soil fertility. Agric. Ecosyst. Environ. 116, 72–84. doi: 10.1016/j.agee.2006.03.011
Carretero, C. L., Cantos, M., Garcia, J. L., Azcon, R., and Troncoso, A. (2009). Growth response of micropropagated cassava clones as affected by Glomus intraradices colonization. J. Plant Nutr. 32, 261–273. doi: 10.1080/01904160802608601
Caveness, F. E. (1982). Root-Knot Nematodes as Parasites of Cassava. International Institute of Tropical Agriculture, Research Briefs, Vol. 3. Ibadan: International Institute of Tropical Agriculture.
Ceballos, I., Ruiz, M., Fernandez, C., Pena, R., Rodriguez, A., and Sanders, I. R. (2013). The in vitro mass-produced model mycorrhizal fungus, Rhizophagus irregularis, significantly increases yields of the globally important food security crop Cassava. PLoS ONE 8:e70633. doi: 10.1371/journal.pone.0070633
Chaleard, J. L. (1988). Le manioc, la ville et le paysan Approvisionnement urbain et mutations rurales dans la région de Bouaké (Côte d'Ivoire). Cahier Sci. Hum. 24, 333–348.
Colombani, J., Lamagat, J. P., and Thiebaux, J. (1973). Mesure de la perméabilité des sols en place: un nouve1 apparei1 pour la méthode Muntz, une extension de la méthode Porchet aux sols hétérogènes. Bull. Sci. Hydrologiques 18, 197–235.
Connor, D. J., Cock, J. H., and Parra, G. E. (1981). The response of cassava to water shortage, growth and yield. Field Crop Res. 4, 181–200.
Corkidi, L., Allen, E. B., Merhaut, D., Allen, M. F., Downer, J., Bohn, J., et al. (2004). Assessing the infectivity of commercial mycorrhizal inoculants in plant nursery conditions. J. Environ. Horticult. 22, 149–154.
Daykin, M. E., and Hussey, R. S. (1985). “Staining and histo-pathological techniques in nematology,” in An Advanced Treatise on Meloidogyne, Vol. II, eds K. R. Barker, C. C. Carter, and J. N. Sasser (Raleigh, NC: North Carolina State University Graphics), 39–48.
Dos Anjos, É. C. T., Cavalcante, U. M. T., Gonçalves, D. M. C., Pedrosa, E. M. R., dos Santos, V. F., and Maia, L. C. (2010). Interactions between an arbuscular mycorrhizal fungus (Scutellospora heterogama) and the root-knot nematode (Meloidogyne incognita) on sweet passion fruit (Passiflora alata). Braz. Arch. Biol. Technol. 53, 801–809. doi: 10.1590/S1516-89132010000400008
Dubois, M., Gilles, K. A., Hamilton, P. A., Robeg, A., and Smith, F. (1956). Colometric method for determination of suggars and related substances. Anal. Chem. 28, 350–356.
Elsen, A., Baimey, H., Swennen, R., and De Waele, D. (2003b). Relative mycorrhizal dependency and mycorrhiza-nematode interaction in banana cultivars (Musa spp.) differing in nematode susceptibility. Plant Soil. 256, 303–313. doi: 10.1023/A:1026150917522
Elsen, A., Beeterens, R., Swennen, R., and De Waele, D. (2003a). Effects of an arbuscular mycorrhizal fungus and two plant-parasitic nematodes on Musa genotypes differing in root morphology. Biol. Fertil. Soils 38, 367–376. doi: 10.1007/s00374-003-0669-3
Elsen, A., Declerck, S., and De Waele, D. (2003c). Use of root organ cultures to investigate the interaction between Glomus intraradices and Pratylenchus coffeae. Appl. Environ. Microbiol. 69, 4308–4311. doi: 10.1128/AEM.69.7.4308-4311.2003
FAO (2014). Faostat Agricultural Database-Agricultural Production. Available online at: http://www.fao.org
Faye, A., Dalpé, Y., Ndung'u-Magiroi, K., Jefwa, J., Ndoye, I., Diouf, M., et al. (2013). Evaluation of commercial arbuscular mycorrhizal inoculants. Can. J. Plant Sci. 93, 1201–1208. doi: 10.4141/cjps2013-326
Gerdemann, J. W., and Nicolson, T. H. (1963). Spores of endogone species from soil by wet sieving and decanting. Trans. Br. Mycol. Soc. 46, 235–244.
Hao, Z., Fayolle, L., van Tuinen, D., Chatagnier, O., Li, X., Gianinazzi, S., et al. (2012). Local and systemic mycorrhiza-induced protection against the ectoparasitic nematode Xiphinema index involves priming of defence gene responses in grapevine. J. Exp. Bot. 63, 3657–3672. doi: 10.1093/jxb/ers046
He, X. H., Critchley, C., and Bledsoe, C. (2003). Nitrogen transfer within and between plants through common mycorrhizal networks (CMNs). Crit. Rev. Plant Sci. 22, 531–567. doi: 10.1080/713608315
Huang, S. P., and Cares, J. E. (2004). “Nematodes,” in Anonyme, Echantillonnage (Méthodes), Restitution du séminaire de Embu 23 au 27 février 2004, Nairobi. Doc. 4/CSM-BGBD CI, 7.
Hussey, R. S., and Barker, K. R. (1973). A comparison of methods of collecting inocula for Meloidogyne spp., including a new technique. Plant Dis. Rep. 57, 1025–1028.
Hussey, R. S., and Roncadori, R. W. (1982). Vesicular arbuscular mycorrhizae may limit nematode activity and improve plant growth. Plant Dis. 66, 9–14.
Jatala, P. J. Bridge (1990). “Nematode parasites of root and tuber crops,” in Plant-Parasitic Nematodes in Subtropical and Tropical Agriculture, eds M. R. Luc., A. Sikora, and J. Bridge (Wallingford: CAB International), 137–180.
Koffi, M. C., Vos, C., Draye, X., and Declerck, S. (2013). Effects of Rhizophagus irregularis MUCL 41833 on the reproduction of Radopholus similis in banana plantlets grown under in vitro culture conditions. Mycorrhiza 23, 279–288. doi: 10.1007/s00572-012-0467-6
Kormanik, P. P., and McGraw, A. C. (1982). “‘Quantification of vesicular arbuscular mycorrhizae in plants roots,” in Methods and Principles of Mycorrhizal Research, ed N. C. Schenck (Minnesota, MN: American Phytopatological Society), 37–45.
Kouadio, K. K. H., Dao, D., Tschannen, A., and et Girardin, O. (2010). Rentabilité comparative des systèmes de culture à base de manioc à l'Est de la Côte d'Ivoire. J. Anim. Plant Sci. 9, 1094–1103.
Krüger, M., Stockinger, H., Krüger, C., and Schüßler, A. (2009). DNA-based species level detection of Glomeromycota: one PCR primer set for all arbuscular mycorrhizal fungi. New Phytol. 183, 212–223. doi: 10.1111/j.1469-8137.2009.02835.x
Mathur, N., and Vyas, A. (1995). Influence of VA mycorrhizae on net photosynthesis and transpiration of Ziziphus mauritiana. J. Plant Physiol. 147, 328–330. doi: 10.1016/S0176-1617(11)82161-9
McSorley, S. K., O'Hair, J. L., and Parrado, J. L. (1983). Nematodes of cassava, Manihot esculenta Crantz. Nematropica 13, 261–287.
Morton, J. B., Bentivenga, S. P., and Wheeler, W. W. (1993). Germ plasma in the International collection of arbuscular and vesicular arbuscular mycorrhizal fungi (INVAM) and procedures for culture development, documentation and storage. Mycotaxon 48, 491–528.
N'Guettia, R., and Bernard, G. (1986). “Besoin en eau et production du manioc,” in Seminar of A.N.A.M., 25. Bouaké (CIV).
Oliveira, R. S., Vosatka, M., Dodd, J. C., and Castro, P. M. (2005). Studies on the diversity of arbuscular mycorrhizal fungi and the efficacy of two native isolates in a highly alkaline anthropogenic sediment. Mycorrhiza 16, 23–31. doi: 10.1007/s00572-005-0010-0
Ortas, I., Kaya, Z., and Çakmak, I. (2001). “Influence of VA-mycorrhiza inoculation on growth of maize and green pepper plants in phosphorus and zinc deficient soils,” in Plant Nutrition - Food Security and Sustainability of Agro-Ecosystems, eds W. J. Horst, M. K. Schenk, A. Burkert, N. Claassen, H. Flessa, W. B. Frommer, H. E. Goldbach, H. W. Olfs, V. Romheld, B. Sattelmacher, U. Schmidhalter, S. Schubert, N. von Wiren, and L. Wittenmayer (Dordrecht: Kluwer Academic Publication), 632–633.
Ortas, O., and Ustuner, O. (2014). The effects of single species, dual species and indigenous mycorrhiza inoculation on citrus growth and nutrient uptake. Eur. J. Soil Biol. 63, 64–69. doi: 10.1016/j.ejsobi.2014.05.007
Osonubi, O., Atayese, M. O., and Mulongoy, K. (1995). The effect of vesicular–arbuscular mycorrhizal inoculation on nutrient uptake and yield of alley cropped cassava in a degraded alfisol of south western Nigeria. Biol. Fert. Soils. 20, 70–76.
Oyetunji, O. J., Ekanayake, I. J., and Osonubi, O. (2007). Chlorophyll fluorescence analysis for assessing water deficit and Arbuscular Mycorrhizal Fungi (AMF) inoculation in cassava (Manihot esculenta Crantz). Adv. Biol. Res. 1, 108–117.
Oyetunji, O. J., and Osonubi, O. (2007). Assessment of influence of alley cropping system and arbuscular mycorrhizal (AM) fungi on cassava productivity in derived savanna zone of Nigeria. World J. Agric. Sci. 3, 489–495.
Phillips, J. M., and Haymann, D. S. (1970). Improved proceeding for clearing roots and staining parasitic and vesicular arbuscular mycorrhizal fungi for rapid assessment of infection. T. Brit. Mycol. Soc. 69, 275–280.
Plenchette, C., Clermont-dauphin, C., Meynard, J. M., and Fortin, J. A. (2005). Managing arbuscular mycorrhizal fungi in cropping systems. Can. J. Plant Sci. 85, 31–40.
Porcel, R., Aroca, R., and Ruiz-Lozano, J. M. (2011). Salinity stress alleviation using arbuscular mycorrhizal fungi. A review. Agron. Sustain. Dev. 32, 181–200. doi: 10.1007/s13593-011-0029-x
Pozo, M. J., and Azcón-Aguilar, C. (2007). Unraveling mycorrhiza-induced resistance. Curr. Opin. Plant Biol. 10, 393–398. doi: 10.1016/j.pbi.2007.05.00
Rodriguez, A., and Sanders, I. R. (2015). The role of community and population ecology in applying mycorrhizal fungi for improved food security. ISME J. 9, 1053–1061. doi: 10.1038/ismej.2014.207
Ruiz-Lozano, J. M., and Azcón, R. (2000). Symbiotic effciency and infectivity of an autochthonous arbuscular mycorrhizal Glomus sp. from saline soils and Glomus deserticola under salinity. Mycorrhiza 10, 137–143. doi: 10.1007/s005720000075
Saitou, N., and Nei, M. (1987). The neighbor-joining method for reconstructing phylogenetic trees. Mol. Biol. Evol. 4, 406–425.
Schenck, N. C., and Perez, Y. (1990). Manual for the Identification of VA Mycorrhizal Fungi. Gainesville: Synergistic Publications.
Schouteden, N., De Waele, D., Panis, B., and Vos, C. M. (2015). Arbuscular mycorrhizal fungi for the biocontrol of plant-parasitic nematodes: a review of the mechanisms involved. Front. Microbiol. 6:1280. doi: 10.3389/fmicb.2015.01280
Schreiner, R. P. (2007). Effects of native and non-native arbuscular mycorrhizal fungi on growth and nutrient uptake of ‘Pinot noir’ (Vitis vinifera L.) in two soils with contrasting levels of phosphorus. Appl. Soil Ecol. 36, 205–215. doi: 10.1016/j.apsoil.2007.03.002
Schüßler, A. H., Gehrig, H., Schwarzott, D., and Walker, C. (2001). Analysis of partial Glomales SSU rRNA gene sequences: implications for primer design and phylogeny. Mycol. Res. 105, 5–15. doi: 10.1017/S0953756200003725
Sidney, W. (1984). “Methods of soil analysis,” in Official Methods of Analysis of the Association of Official Analytic Chemist, 14th Edn., ed S. W. Arlington. (Virginia: AOAC), 8–37.
Sieverding, E. (1989). Ecology of VAM fungi in tropical agrosystems. Agricult. Ecosys. Environ. 29, 369–390.
Singh, Y. P., Singh, R. S., and Sitaramalah, K. (1990). “Mechanism of resistance of mycorrhizal tomato against root-knot nematodes in Trends in Mycorhizal Research,” in Proceedings of the National Conference on Mycorrhiza, eds B. L. Jalali and H. Chand (Karnal: Haryana Agriculture University), 96.
Singleton, V. L., Ortofer, R., and Lamuela-Raventos, R. M. (1999). “Analysis of total phenols and other oxidation substrates and antioxidants by means of Folin Ciocalteu reagent,” in Methods in Enzymology, ed L. Packer (Orlando: Academic Press), 152–178.
Smith, S. E., and Read, D. J. (2008). “Mineral nutrition, toxic element accumulation and water relations of arbuscular mycorrhizal plants,” in Mycorrhizal Symbiosis, 3rd Edn., eds S. E. Smith and D. J. Read (London: Academic Press), 145–148.
St-Arnaud, M., and Vujanovic, V. (2007). “Effect of the arbuscular mycorrhizal symbiosis on plant diseases and pests,” in Mycorrhizae in Crop Production: Applying Knowledge, eds C. Hamel and C. Plenchette (Binghampton, NY: Haworth), 188.
Stockinger, H., Krüger, M., and Schüssler, A. (2010). DNA barcoding of arbuscular mycorrhizal fungi. New Phytol. 187, 461–474. doi: 10.1111/j.1469-8137.2010.03262.x
Stockinger, H., Walker, C., and Schüßler, A. (2009). ‘Glomus intraradices DAOM197198’, a model fungus in arbuscular mycorrhiza research, is not Glomus intraradices. New Phytol. 183, 1176–1187. doi: 10.1111/j.1469-8137.2009.02874.x
Tchabi, A. (2008). Arbuscular Mycorrhizal Fungi in the Sub-Saharan Savannas of Benin and their Association with Yam (Dioscorea spp.): Potential of Yam Growth Promotion and Deduction of Nematode Infestation. Thèse de doctorat. Université de Basel.
Tian, H., Drijber, R. A., Li, X., Miller, D. N., and Wienhold, B. J. (2013). Arbuscular mycorrhizal fungi differ in their ability to regulate the expression of phosphate transporters in maize (Zeamays L.). Mycorrhiza 23, 507–514. doi: 10.1007/s00572-013-0491-1
Trejo-Aguilar, D., Lara-Capistrán, L., Maldonado-Mendoza, I. E., Zulueta-Rodríguez, R., Sangabrie-Conde, W., Mancera-López, M. E., et al. (2013). Loss of arbuscular mycorrhizal fungal diversity in trap cultures during long-term subculturing. IMA Fungus 4, 161–167. doi: 10.5598/imafungus.2013.04.02.01
Trouvelot, A., Kough, J. L., and Gianinazzi-Pearson, V. (1986). “Mesure du taux de mycorrhization VA d'un système radiculaire. Recherche de methods d'estimation ayant une signification fonctionnelle,” in Physiological and Genetical Aspects of Mycorrhizae, eds V. Gianinazzi-Pearson and S. Gianinazzi (France: INRA Paris), 217–221.
van Tuinen, D., Zhao, B., and Gianinazzi-Pearson, V. (1998). “PCR in studies of AM fungi: from primers to application,” in Mycorrhiza Manual, eds A. K. Varma (Heidelberg: Springer-Verlag), 387–399.
Veresoglou, S. D., and Rillig, M. C. (2012). Suppression of fungal and nematode plant pathogens through arbuscular mycorrhizal fungi. Biol. Lett. 8, 214–216. doi: 10.1098/rsbl.2011.0874
Walder, F., and van der Heijden, M. G. A. (2015). Regulation of resource exchange in the arbuscular mycorrhizal symbiosis. Nat. Plants 1, 15159. doi: 10.1038/nplants.2015.159
Williams, A., Ridgway, H., and Norton, D. (2012). Different arbuscular mycorrhizae and competition with an exotic grass affect the growth of Podocarpus cunninghamii Colenso cuttings. New For. 44, 183–195. doi: 10.1007/s11056-012-9309-9
Xu, X., Qin, G., and Tian, S. (2008). Effect of microbial biocontrol agents on alleviating oxidative damage of peach fruit subjected to fungal pathogen. Int. J. Food Microbiol. 126, 153–158. doi: 10.1016/j.ijfoodmicro.2008.05.019
Keywords: AMF, ecological engineering, cassava yield, tolerance, Meloidogyne, drought
Citation: Séry DJ-M, Kouadjo ZGC, Voko BRR and Zézé A (2016) Selecting Native Arbuscular Mycorrhizal Fungi to Promote Cassava Growth and Increase Yield under Field Conditions. Front. Microbiol. 7:2063. doi: 10.3389/fmicb.2016.02063
Received: 29 July 2016; Accepted: 07 December 2016;
Published: 22 December 2016.
Edited by:
Mohamed Hijri, Université de Montréal, CanadaReviewed by:
Erik Limpens, Wageningen University and Research Centre, NetherlandsRaffaella Balestrini, National Research Council, Italy
Copyright © 2016 Séry, Kouadjo, Voko and Zézé. This is an open-access article distributed under the terms of the Creative Commons Attribution License (CC BY). The use, distribution or reproduction in other forums is permitted, provided the original author(s) or licensor are credited and that the original publication in this journal is cited, in accordance with accepted academic practice. No use, distribution or reproduction is permitted which does not comply with these terms.
*Correspondence: Zézé Adolphe, eW91aGUuZGViYUBnbWFpbC5jb20=