- 1Department of Animal and Avian Sciences, University of Maryland, College Park, MD, USA
- 2Biological Sciences Program – Molecular and Cellular Biology, University of Maryland, College Park, MD, USA
- 3Center for Food Safety and Security Systems, University of Maryland, College Park, MD, USA
The therapeutic roles of phenolic blueberry (Vaccinium corymbosum) and blackberry (Rubus fruticosus) pomace (commercial byproduct) extracts (BPE) and their mechanism of actions were evaluated against methicillin resistant Staphylococcus aureus (MRSA). Five major phenolic acids of BPE, e.g., protocatechuic, p. coumaric, vanillic, caffeic, and gallic acids, as well as crude BPE completely inhibited the growth of vegetative MRSA in vitro while BPE+methicillin significantly reduced MRSA biofilm formation on plastic surface. In addition, BPE restored the effectiveness of methicillin against MRSA by down-regulating the expression of methicillin resistance (mecA) and efflux pump (norA, norB, norC, mdeA, sdrM, and sepA) genes. Antibiogram with broth microdilution method showed that MIC of methicillin reduced from 512 μg/mL to 4 μg/mL when combined with only 200 μg Gallic Acid Equivalent (GAE)/mL of BPE. Significant reduction in MRSA adherence to and invasion into human skin keratinocyte Hek001 cells were also noticed in the presence of BPE. BPE induced anti-apoptosis and anti-autophagy pathways through overexpression of Bcl-2 gene and down-regulation of TRADD and Bax genes (inducers of apoptosis pathway) in Hek001 cells. In summary, novel and sustainable prophylactic therapy can be developed with BPE in combination with currently available antibiotics, especially methicillin, against skin and soft tissue infections with MRSA.
Introduction
Staphylococcus aureus has historically been a tenacious human pathogen; in recent decades it has become an even more serious threat due to its acquisition of multiple antibiotic resistances (AR) (Wilkinson et al., 2005; Novick, 2008). AR is growing worldwide, and the discovery of new antibiotics is not keeping pace with evolving bacterial resistance; alternative therapeutics is urgently required. Moreover, certain pathogenic S. aureus strains known as “superbugs” are exceptionally rigid and quickly develop protective mechanisms against synthetic antibiotics. One common superbug increasingly seen in hospitals and community is methicillin-resistant Staphylococcus aureus (MRSA). MRSA is resistant to most antibiotics currently available (Fair and Tor, 2014).
Tang et al. (2011) has reported that MRSA accounts for more than half of all skin and soft tissue infections (SSTIs). In addition, community-acquired-MRSA (CA-MRSA) has also been found in livestock animals, veterinary hospital, and workers (Wulf et al., 2007, 2008). CA-MRSA are recognized as one of common superbugs by multi-locus sequence typing (Ekkelenkamp et al., 2006; Wulf et al., 2008). Though infection with MRSA in hospital setting is being reduced in recent years, the Centers for Disease Control and Prevention (CDC) estimated that the number of MRSA infections in the US exceeds 94,000 per year, and causes more deaths in the US than human immunodeficiency virus and Acquired Immune Deficiency Syndrome (Bancroft, 2007). In addition to hospital settings, CA-MRSA has become a global public health concern (Gardam, 2000; Le Loir et al., 2003; Do Carmo et al., 2004; Gustafson and Wilkinson, 2005; Kennedy et al., 2008; Pu et al., 2009). Because of these emergent issues, development of alternative strategies to combat MRSA infection is of pressing need.
The seeds and skins of berries contain polyphenols, phytosterols, tocopherols, fiber, protein, and biotin (Puupponen-Pimiä et al., 2005, 2008; Salaheen et al., 2014, 2015). Major components of polyphenols include anthocyanins, procyanidins, and hydroxycinnamates. Procyanidins are a class of polymeric polyphenolics and can contain catechin or epicatechin (Djilas et al., 2009). Our recent HPLC/high mass accuracy TOF mass spectrometry analysis indicated that major phenolic compounds in berry pomace (byproduct) include but are not limited to flavan, glucuronides, quinolones, catechol, coumarin, phenols, tannins, quercetin, vanillic acid, caffeic acid, ellagic acid, and gallic acid (Salaheen et al., 2016). Flavans are a type of anthocyanidin pigments with antimicrobial activity against several pathogenic bacteria (Sasaki et al., 2007; Yao et al., 2011). Antimicrobial properties in glucuronides, catechol, and phenols have also been reported (Salem and Saleh, 2002; Puupponen-Pimiä et al., 2008). Quinolones have antimicrobial activity and anti-quorum sensing activity against Pseudomonas (Rasmussen and Givskov, 2006). Alves et al. (2013) has reported that acid derivatives including vanillic acid, caffeic acid, ellagic acid, and gallic acid from mushrooms were able to synergistically inhibit MRSA.
In this study, we aim to evaluate the function of phenolic blueberry and blackberry pomace extracts (BPE) in restoring the antibacterial activity of methicillin by complete inhibition of MRSA cells both vegetative and biofilm formation through alteration of physical, biochemical, and transcriptome profile.
Materials and Methods
Preparation of Pomace Extracts
Bioactive phenolic extracts from blackberry (Rubus fruticosus) and blueberry (Vaccinium corymbosum) pomaces were prepared according to the protocol previously described (Salaheen et al., 2014). Total phenolic contents in each extract was determined using spectrophotometric method (Singleton et al., 1999). Total phenolic content was expressed as Gallic Acid Equivalent (GAE). Berry Pomace Extract (BPE) was prepared by combining blackberry and blueberry pomace extract at 1:1 ratio. The pH ranges of the crude extracts were 4.5–5 and pH of the extracts varied with the methods of treatment and extraction.
Bacterial Strains, Growth Condition, Antimicrobial Susceptibility, and Methicillin Rescue Assay
Methicillin resistant Staphylococcus aureus COL (SCCmec type I; gift from Dr. Steven Ricke, University of Arkansas; complete genome available from http://www.genome.jp/dbget-bin/www_bget?gn:T00225) was used in the current study for in vitro assays. Strain COL is resistant to oxacillin, methicillin, and tetracycline, possesses a non-functional mecI-mecR1 system, and β-lactamase deficient (De Lencastre and Tomasz, 1994; De Lencastre et al., 1999). Bacterial cells were grown in Cation Adjusted Muller Hinton Broth (CAMHB; Clinical and Laboratory Standards Institute, [CLSI], 2013) at 37°C for 24 h with shaking at 120 rpm.
Minimum Inhibitory Concentrations (MIC) and Minimum Bactericidal Concentration (MBC) of BPE, and other purified phenolic compounds including Protocatechuic acid, Taxifolin, Myricetin, Quercetin, P. coumaric acid, Gallic acid, Vanillic acid, and Caffeic acid were determined using broth micro-dilution method described previously with some modifications (Salaheen et al., 2015). In brief, BPE concentration starting from 4092 μg GAE/mL to 0.0 μg GAE/mL with a twofold dilution were added to CAMHB to a final volume of 990 μL. Ten microliter bacterial suspension containing 5 × 105 CFU/mL was added to 24-wells plates followed by incubation at 37°C with shaking at 120 rpm for 24 h. After incubation, bacterial suspensions were serially diluted in PBS and plated on Baird–Parker agar medium (BP, Himedia, India). Growth inhibition and all other assays were repeated at least three times with three biological replicates.
Antibiotic (methicillin) rescue assay was also carried out in CAMHB with 2% NaCl. BPE and/or methicillin containing broth was added to 96-well culture plates (Greiner Bio-One CellStar, NC) with concentration ranging from 0 to 400 μg GAE/mL of BPE and concentration ranging from 0 to 512 μg/mL of methicillin. Plates were incubated for 24 h at 37°C followed by drop-plating on BP.
The dose dependent responses of BPE and/or methicillin were analyzed using the Nonlinear Curve Fitting Function of Microcal Origin 7.5 (Microcal Software Inc., Northampton, MA). The sub-lethal dose (SLT2LOG) at which microbial cell numbers were reduced by ∼2 log compared with the control was determined following the method described by Ahn et al. (2014). The SLT2LOG was determined as combination of 25 μg GAE/mL BPE with 4.0 μg/mL of methicillin in CAMHB against MRSA strain.
Physicochemical and Virulence Properties of MRSA Treated with Sub-lethal Dose of BPE (SLT2LOG)
Physicochemical properties of bacterial cells, e.g., cell surface hydrophobicity and auto-aggregation, were evaluated following the methodologies previously described (Rosenberg, 1984; Del Re et al., 2000; Peng et al., 2015c). Virulence properties of MRSA strains, e.g., serum agglutination and hemolysis activity on sheep red blood cells (RBC) (Lampire, Everett, PA, USA) and single human donor RBC (Innovative Research, MI) were determined following the methods described previously by Jiang et al. (2007). MRSA was grown in CAMHB with 2% NaCl in either presence of only 4.0 μg/mL of methicillin or presence of predetermined SLT2LOG of inhibitors (25 μg GAE/mL BPE and 4.0 μg/mL of methicillin) at 37°C for 18 h. The harvested MRSA cells were used to extract RNA with ZR Bacterial RNA MiniPrep kit (Zymo Research, San Diego, CA, USA) for quantitative RT-PCR assay. Serum agglutination test was carried out with BactiStaph Latex Test Kit (Ref: R21144, Remel, Lenexa, KS, USA) and the time required for complete agglutination was measured with stopwatch (VWR, Radnor, PA, USA). Hemolysis activity of MRSA was determined according to the protocol described by Jiang et al. (2007).
Adhesion and Invasiveness Assay
Adhesion and invasion ability of MRSA strains were determined under two conditions: induction of several concentrations of BPE and methicillin combination treatments during infection (bacterial inoculum and treatments concurrently added on host cell monolayer; representing preventive state) and the same range of treatments after infection (treatments added after 1 h infection of host cell monolayer with bacterial inoculum; representing therapeutic state). A concentration of 4 μg/mL methicillin was used in combination with 0, 50, 100, 200, 400, and 800 μg GAE/mL of BPE as the various treatment groups. The adhesion and invasion of human keratinocyte HEK001 (ATCC CRL 2404TM) cells by MRSA strains was carried out following the method previously described (Salaheen et al., 2014; Peng et al., 2015b). To simulate the therapeutic state, treatments containing 4 μg/mL methicillin in conjunction with 0, 50, 100, 200, 400, and 800 μg GAE/mL of BPE were used on infected cell monolayer at 37°C for 1 h.
Effects of BPE on Cultured HEK001 Cells
Viability of cultured HEK001 cells in the presence of various concentration of BPE was measured following the method described previously with modifications (Strober, 2001). HEK001 cell monolayers were washed and 1 mL of fresh Keratinocyte-SFM medium containing 5 ng/mL epidermal growth factor (EGF) and various concentrations of BPE (0, 50, 100, 200, 400, and 800 μg GAE/mL) were placed in triplicate wells followed by incubation for 2 h at 37°C and viability assay was carried out with 0.4% Trypan Blue solution (Sigma, MO). Treated HEK001 cell monolayers were also imaged with Nexus6 (Google Play Store) and the images were processed in Microsoft Office Picture Manager (Version 2010). Treated HEK001 cell monolayers were also used to extract RNA for quantitative RT-PCR assay.
Synergistic Effect of BPE and Methicillin on MRSA Growth and Biofilm Formation
To prepared biofilms, MRSA was inoculated at approximately 5 × 105 CFU/mL in 6-well plates (Corning, New York, NY, USA) containing 22 mm × 22 mm plastic slides (VWR) and CAMHB with 2% NaCl at 37°C for 96 h without shaking. CAMHB containing 2% NaCl with 4 μg/mL methicillin was combined with MIC or double MIC of BPE and incubated at 37°C under shaking at 120 rpm for 1 and 24 h. For numerical analysis, MRSA cells were recovered with sterile cell scraper (VWR, PA) from the plastic surface and plated on BP.
For visualization, treated biofilms on plastic slides were stained with FilmTracerTM LIVE/DEAD Biofilm Viability Kit (Life Technologies, OR). Slides were viewed at room temperature using a Zeiss AxioObserver. Z1 inverted microscope, and images were acquired using the Zeiss Axiovision Rel 4.6 software with the Zeiss Axiocam HRC camera. All exported images were processed in Microsoft Office Picture Manager (Version 2010). RNA was also extracted from the bacterial cells of treated MRSA biofilms on plastic slides and used for quantitative RT-PCR assay.
Measurement of Transcriptomic Changes of MRSA and HEK001 Cells Treated with BPE and Methicillin
To measure the expression of MRSA and HEK001cell genes in the presence of BPE, quantitative RT-PCR assay was carried out in three different sections of this study: (i) expression of virulence and antimicrobial resistance mediatory genes of MRSA, (ii) expression of genes involved in biofilm formation of MRSA, and (iii) expression of the genes related to cellular growth and apoptosis of host HEK001 cells. RNA extraction procedures have been described above. QScript cDNA Supermix (QuantaBio, Beverly, MA, USA) was used from cDNA synthesis and qRT-PCR was performed in EcoTM (Illumina, San Deigo, CA, USA) according to the protocol previously described (Peng et al., 2015c).
Statistical Analysis
All data were analyzed using the Statistical Analysis System software (SAS, Institute Inc., Cary, NC, USA). One-way analysis of variance (ANOVA) was used, followed by Tukey’s test to determine significant differences among treatments at p < 0.05.
Results
Inhibition of MRSA Growth with Phenolics
The inhibitory effects of various phenolics and their respective MBC have been listed in Table 1. We found that the MBC of the tested phenolic acids including Protocatechuic acid, P. coumaric acid, Gallic acid, Vanillic acid, and Caffeic acid inhibited the growth of MRSA in CAMHB liquid culture at concentrations ranging from 0.512 to 2.046 mg GAE/mL with P. coumaric acid being the most effective>1.028 mg GAE/mL of combination of these five phenolic acids were required for inhibiting the growth of MRSA. Flavonoids, e.g., Taxifolin, Myricetin, and Quercetin, failed to demonstrate any growth inhibitory effect up to 4.092 mg GAE/mL. Crude BPE exhibited the highest inhibitory effect against MRSA, the MBC and MIC were observed 0.512 mg GAE/mL and 0.400 mg GAE/mL, respectively.
Synergistic Effect of BPE with Methicillin against MRSA
The synergistic inhibitory effects of BPE and methicillin against the MRSA have been illustrated in Table 2. MBC of methicillin against MRSA was >512 μg/mL, whereas conjunction with various concentrations of BPE minimized the MBC of methicillin to as low as 4 μg/mL which is below the concentration range suggested by the CLSI for MRSA. We determined the SLT2LOG (treatment at which MRSA numbers were reduced by 2 logs compared with control group) to be as the combination of 25 μg GAE/mL BPE with 4 μg/mL of methicillin and used this treatment for further studies. We observed that the combination of 200 μg GAE/mL BPE with 4 μg/mL of methicillin in CAMHB broth reduced MRSA by >4 logs compared to the treatments of 200 μg GAE/mL BPE or 4 μg/mL of methicillin in CAMHB individually. To determine the reactivation of effect of methicillin through efflux pump genes alteration, we checked the differential expression levels in the multi-drug resistance (MDR) efflux pump genes in MRSA in the presence of SLT2LOG (Figure 1). For example, the expression of MDR efflux pump genes, e.g., norA, norB, norC, mdeA, sdrM, and sepA were significantly down-regulated. More specifically, SLT2LOG also reduced the expression of methicillin resistance gene, mecA. However, SLT2LOG did not alter the expression of qacA/B gene, and stimulated gtf gene.
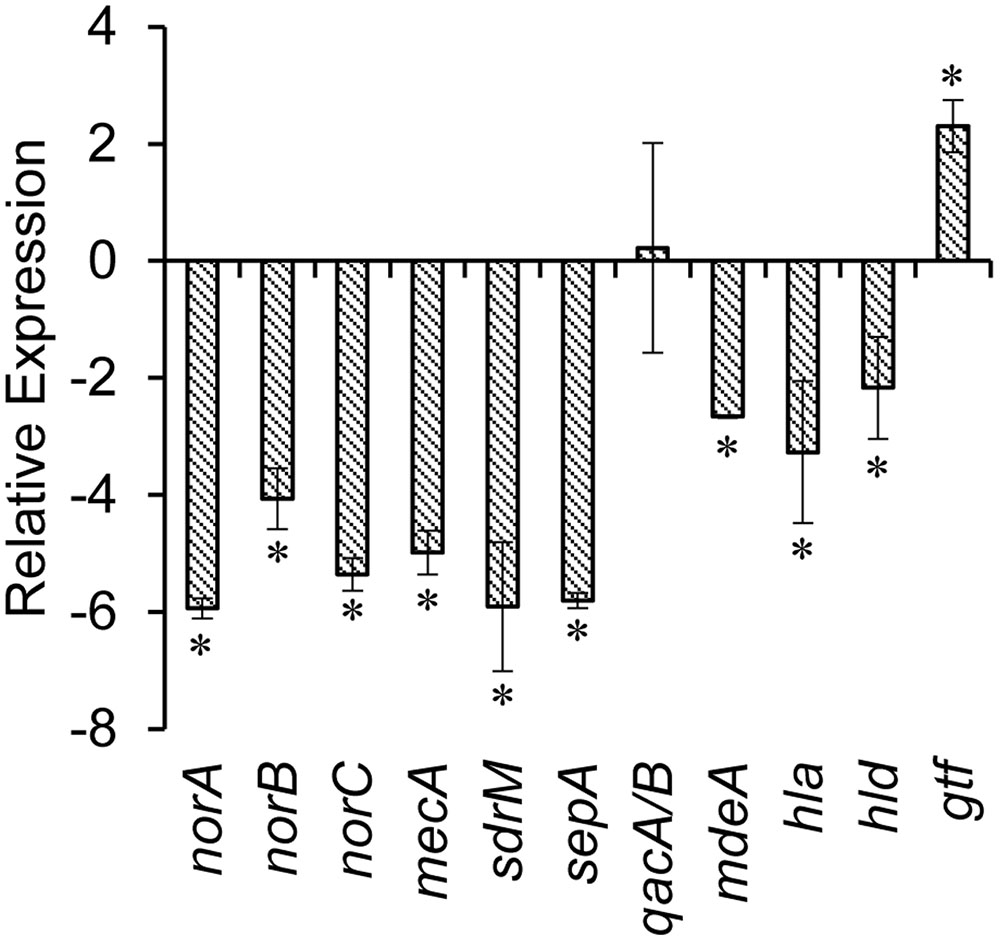
FIGURE 1. Relative expression of virulence genes of methicillin resistant Staphylococcus aureus (MRSA) treated with SLT2LOG of BPE and methicillin. ∗indicates significantly increased or decreased relative expression of genes at p < 0.05.
Alteration of Physicochemical Properties and Expression of Virulence Genes of MRSA in the Presence of Sub-lethal Treatment (SLT2LOG) of BPE and Methicillin Combination
The effects of the SLT2LOG on the physicochemical properties and virulence factors of MRSA have been shown in Table 3. In the presence of SLT2LOG BPE and methicillin, auto-aggregation capability of MRSA increased significantly. Untreated bacterial cells showed lower auto-aggregation, 8.84 ± 1.64% whereas in presence of SLT2LOG the value was increased to 15.18 ± 1.27% (p < 0.05). A co-occurrence was noticed between the phenomenon of increased auto-aggregation in MRSA to enhanced expression of gtf gene which codes for polysaccharide synthase playing important roles in exopolysaccharide formation (Deutsch et al., 2008). SLT2LOG of BPE and methicillin combination also reduced the hemolytic property of MRSA; hemolysis of sheep and human RBCs by MRSA were reduced to 59.50 ± 8.36%, and 73.50 ± 26.07%, respectively, in the presence of SLT2LOG. The expression of α-hemolysin (hla) and δ-hemolysin (hld) genes were also significantly down-regulated with SLT2LOG (Figure 1). However, cell surface hydrophobicity and serum agglutination capability of MRSA were not altered significantly with SLT2LOG.

TABLE 3. Physicochemical properties and mechanical behaviors of S. aureus COL treated with sub-lethal concentration (SLC2LOG) of berry pomace extract (BPE) and methicillin.
Role of SLT2LOG Dose of BPE and Methicillin Combination on Human Keratinocyte Cells-MRSA Interactions
Adherence and invasion of human keratinocyte HEK001 cells with MRSA were performed under several concentrations of BPE in combination with methicillin during treatment and post-infection phase (Figure 2). In the presence of BPE, adherence of MRSA to HEK001 cells were decreased at a dose-dependent manner. Significant reduction in the adherence of MRSA to HEK001 was observed at the concentration from 400 μg GAE/mL of BPE and beyond (p < 0.05). Numerical reduction in MRSA adherence to HEK001 cells was noticed with the lowest concentration, e.g., 100 μg GAE/mL of BPE (Figure 2). Invasion of MRSA into HEK001 cells was also plummeted at a dose-dependent manner (Figure 2A). Significant difference in the invasion of MRSA into HEK001 was observed at the concentration of BPE starting from 100 μg GAE/mL (Figure 2A).
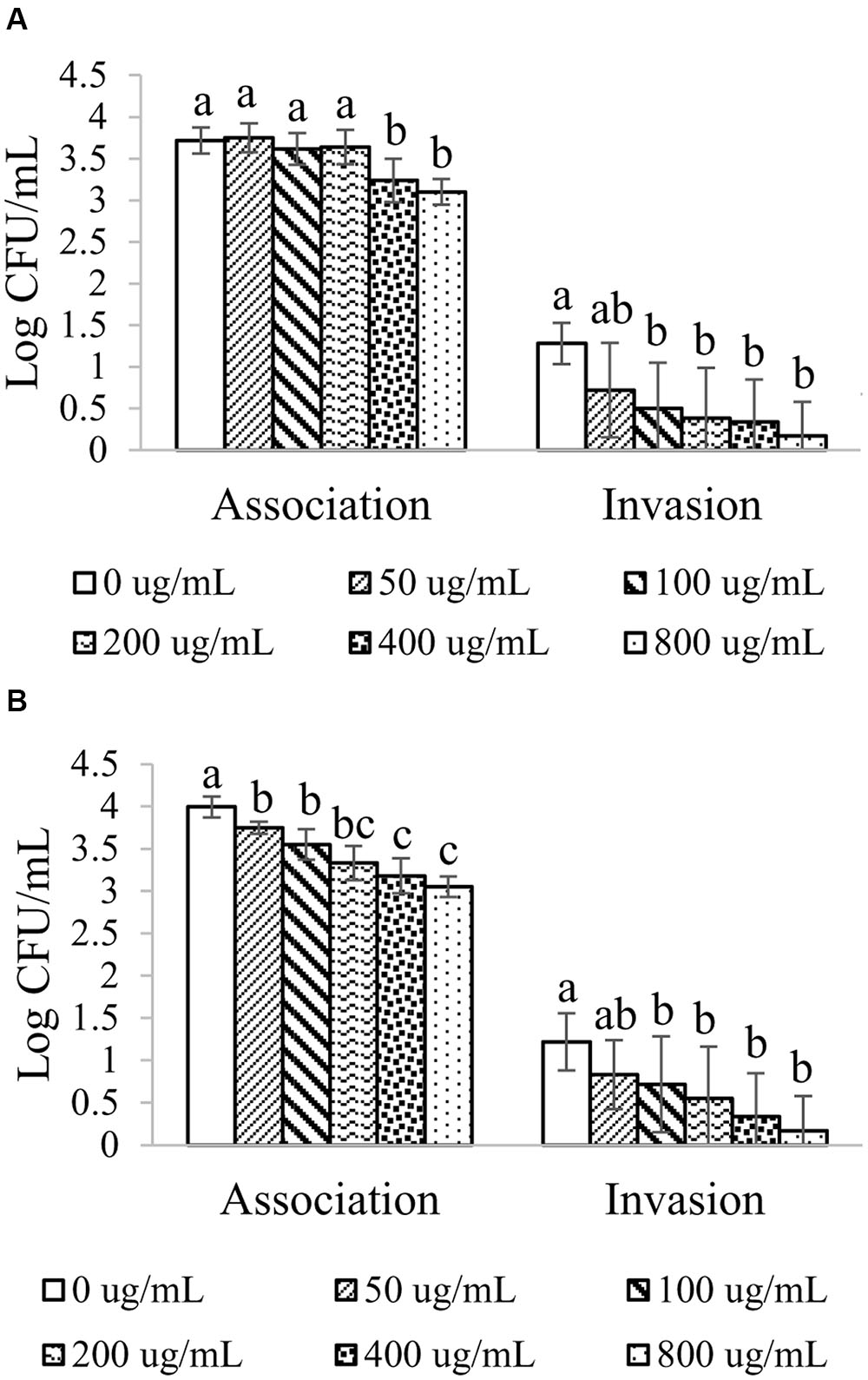
FIGURE 2. Adhesion and invasion ability of MRSA to HEK001 in the presence of various concentrations of BPE and BPE combined with 4 μg/mL of methicillin during infection (A) and post infection (B). Means with different letters (A–D) are significantly different (p < 0.05).
A dose-dependent decrease in the adherence of MRSA to HEK001 cells was also observed when infected HEK001 cells were treated with BPE (Figure 2B). Significant difference in the adherence of MRSA to HEK001 (0.3–1.5 logs) was observed at the concentration of BPE from 50 μg GAE/mL and beyond (p < 0.05). Invasion of MRSA into HEK001 cells was reduced by 0.3–1 log depending on the concentration of BPE; significant difference in the invasion of MRSA into HEK001 cells was observed at the concentration of BPE starting from 100 μg GAE/mL.
Effect of BPE on Human Keratinocyte (HEK001) Cell Viability
Trypan blue exclusion assay indicated a dose-dependent reduction in the viability of adherent HEK001 cell (Figure 3A). The percentages of 96.06, 92.72, 92.42, 91.51, 90.61, and 87.88 of adherent HEK001 cells to the plastic surfaces were remained viable after 2 hr treatment with 0, 50, 100, 200, 400, and 800 μg GAE/mL of BPE, respectively (Figure 3A). Usually, human cultured keratinocytes are large, epithelial, round, adherent cells growing as a confluent monolayer and after 2 h exposure to BPE, neither substantial changes in cell morphology, nor any detachment from the surface were noticed microscopically (Figure 3B). However, a slight dehydration was observed with increased concentrations of BPE. Investigation into the expression of HEK001 genes active in necrosis, apoptosis, and autophagy pathways indicated that treatment with BPE only induced the expression of Bcl-2 (inhibitor to apoptosis and autophagy pathways) by ∼ 2 while down-regulating TRADD and Bax genes (inducers of apoptosis pathway) by two- to threefold (Figure 3C).
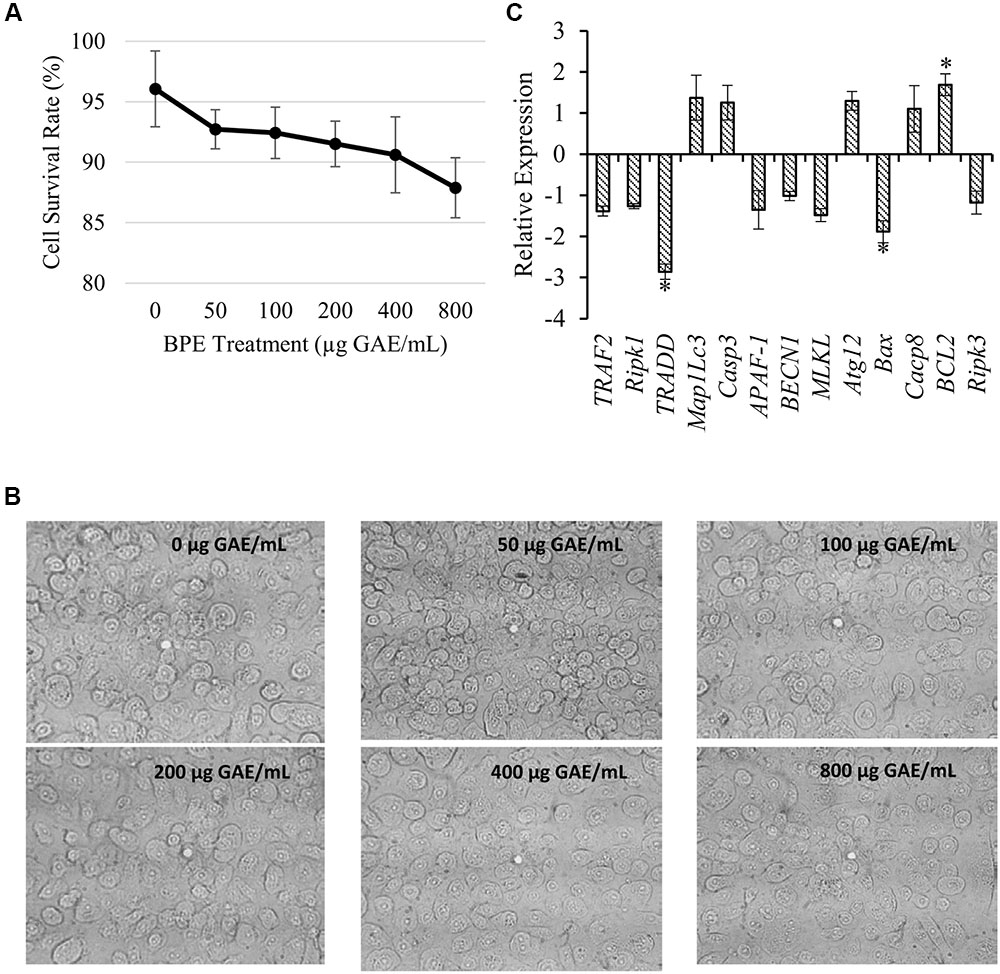
FIGURE 3. Effect of various concentrations of BPE combined with 4 μg/mL of methicillin on MRSA-cultured HEK001 cell interactions. (A) Cell survival rate at various concentrations of BPE; (B) Visualization of cell morphology in the presence of BPE; (C) Expression of the genes related to HEK001 cell growth and apoptosis after treatment with 200 μg GAE/mL of BPE combined with 4 μg/mL of methicillin. ∗indicates significant differential value compared to the BPE (–) Methicillin 4 μg/mL (+) group at p < 0.05.
Anti-biofilm Formation Effect of BPE in Combination with Methicillin
Effect of BPE (MIC and 2x MIC) in combination with 4 μg/mL methicillin on preformed MRSA biofilms has been documented (Figure 4). Treatment with only 2x MIC of BPE (but not MIC of BPE) combined with 4 μg/mL methicillin for 1 h treatment significantly reduced the number of MRSA cells (∼1 log) in preformed biofilm on plastic surface (Figure 4A). We also observed in the same assay that the longer period of treatments with both MIC and 2x MIC of BPE (24 hr) caused significant reductions (1.5–2.0 logs) in the viability of biofilmed MRSA. Microscopic observation using SYTO 9 green fluorescence dye (stains live biofilm cells) and propidium iodide (stains cells with injured outer membrane) also confirmed these findings (Figure 4B). Highest inhibition of MRSA biofilm was observed on treatment with 2x MIC of BPE combined with 4 μg/mL methicillin after 24 h. MIC of BPE with 4 μg/mL methicillin resulted in MRSA membrane injury (visible red and orange MRSA cells) but no significant alteration in the MRSA viability on agar medium. The expressions of 13 genes of MRSA that are involved in biofilm formation were compared and the annotations of these genes are described in Supplementary Table S1. Differential expression was observed with treatment among 10 of these genes: significant up-regulation in seven (clfA, fib, cna, agrA, capA, kdpA, and sarA) and down-regulation in three (ebps, eno, and saeR) compared to non-treatment group. Expression of cna, clfA, fib, agrA, capA, kdpA, and sarA was 3–15 fold up-regulated at 24 h of treatment, with cna being the most up-regulated gene (Figure 4C). On the other hand, ebps, eno, and saeR exhibited 3–5 fold down-regulations at 24 h of treatment. No significant differential expression of any gene was observed after 1 h treatment with up to 2x MIC concentration of BPE.
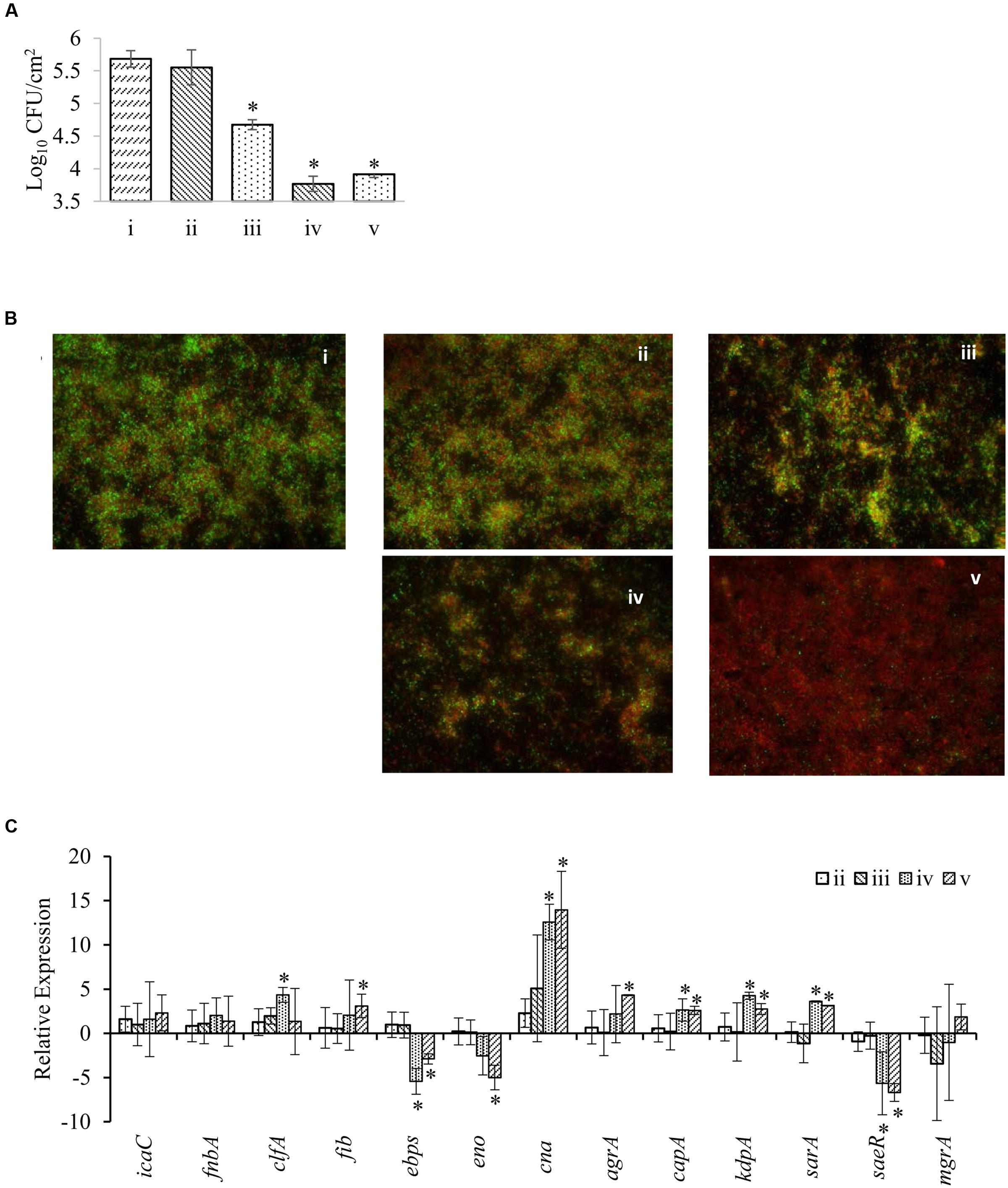
FIGURE 4. Effect of BPE combined with 4 μg/mL of methicillin on MRSA biofilm formation. (A) Inhibition of MRSA cells forming biofilm on plastic surface; (B) fluorescence microscopy of MRSA biofilms in the presence of BPE; (C) Expression of the genes related to biofilm formation in MRSA. Treatments: (i) BPE (–) Methicillin 4 μg/mL (+); ii, BPE (MIC) Methicillin 4 μg/mL (+) 1 h; (iii) BPE (2x MIC) Methicillin 4 μg/mL (+) 1 h; (iv) BPE (MIC) Methicillin 4 μg/mL (+) 24 h; (v) BPE (2x MIC) Methicillin 4 μg/mL (+) 24 h. ∗indicates significant differential value compared to the BPE (–) group at p < 0.05.
Discussion
Due to the lack of success in developing new antibiotics by pharmaceutical companies and the common trend of bacterial mechanism in gaining resistance against currently available antibiotics through natural selection, the World Health Organization has warned that we are heading toward a “post-antibiotic era” (Aryee and Price, 2015). Therefore, recent researches have been directed towards finding new antimicrobials and novel strategies to combat multi-drug resistant bacterial pathogens. In this study, we have tested the function and mechanism of BPE in restoring the antibacterial activity of methicillin against MRSA.
Polyphenols, secondary metabolites in higher plants, are abundantly found in plant tissues mainly as glycosides and/or complex polymerized compounds (Puupponen-Pimiä et al., 2005, 2008; Peng et al., 2015a). The seeds and skins of fruits, particularly berries, contain polyphenols, phytosterols, tocopherols, fiber, protein, and biotin (Salaheen et al., 2014, 2015, 2016). Our recent HPLC/high mass accuracy TOF mass spectrometry analysis indicated that major phenolic compounds in blackberry and blueberry pomace, include but are not limited to flavan, glucuronides, quinolones, catechol, coumarin, phenols, tannins, quercetin, vanillic acid, caffeic acid, ellagic acid, and gallic acid and most of them are antibacterial (Alves et al., 2013; Salaheen et al., 2016). In this study, we confirmed that BPE from both blueberry and blackberry pomace are capable of complete inhibition of MRSA. In addition, findings from this study indicate that BPE compounds perform better synergistically which opens further research options to determine appropriate ratios of these compounds to be used against MRSA. Altered auto-aggregation of MRSA after treatment with BPE and methicillin indicated alteration of cell membrane and consequent rupture of cells followed by cell-death (Salaheen et al., 2014).
Our results indicated that BPE possess the capability to restore the effective antibacterial activity of currently ineffective antibiotics, especially methicillin against MRSA. Though actual mechanism of reactivation of antibiotics have not been documented but our data agreed with previous findings (Stapleton et al., 2004; Aiyegoro and Okoh, 2009; Chung et al., 2011). We found significant down-regulation of MDR efflux pump genes, e.g., norA, norB, norC, sdrM and sepA, and more specifically methicillin resistance mediatory mecA gene in the presence of BPE. Exposure to methicillin inactivates the high-binding-affinity Penicillin Binding Proteins (PBP) that are normally present in S. aureus. However, a novel PBP2a protein, encoded by mecA gene and normally present in MRSA, displaying a low affinity for methicillin takes over the synthesis of peptidoglycan which permits the MRSA to grow (Chambers, 2001). We hypothesize that the differential expression of mecA and MDR efflux pump genes in the presence of BPE is possible mechanism of methicillin reactivation against MRSA. Though sufficient reports are unavailable on the direct implication of efflux pump genes on methicillin resistance in MRSA, these genes are highly expressed in MRSA isolates (Jang, 2016). So, in this study, we included efflux pump genes in addition to the major methicillin resistance gene, macA.
In addition to the inhibition of the growth of vegetative MRSA cells, BPE alone and BPE in combination with methicillin significantly reduced the biofilmed MRSA. Biofilm formation behavior of S. aureus that are responsible for a variety of diseases in humans (Kskiwiecin et al., 2015). Up-regulation of several genes involved in biofilm formation was observed in the presence of BPE and methicillin. These include major component of quorum sensing in S. aureus: accessory gene regulator (agrA), capsular polysaccharide regulatory elements (capA, sarA, kdpA), and microbial surface components recognizing adhesive matrix molecules (cna, fib, and clfA). These findings indicate compensatory and stress response in MRSA biofilm in presence of BPE and methicillin. Membrane and slime layer disruption can be considered the major mechanism of BPE to reduce MRSA biofilm on plastic surface (Federman et al., 2016). Further, alteration of other virulence properties, e.g., reduction of RBC hemolysis and reduced infection rate to HEK001 cells were also observed in the presence of BPE.
Skin infections with MRSA often induce excess host inflammation due to cytokines produced by host and exotoxins secreted by the bacterial cells, which in turn aggravate the pathogenesis of the disease (Montgomery et al., 2013; Sharma-Kuinkel et al., 2013). Anti-inflammatory effects of phenolics from BPE would potentially be able to limit the inflammatory process induced by MRSA infection (Lechner et al., 2004). We observed induction of anti-apoptosis and anti-autophagy pathways in the presence of BPE through over-expression of Bcl-2 gene while down-regulation of TRADD and Bax genes in HEK001 cells. Interesting to notice that HEK001 cells survival rate in the presence of various concentrations of BPE is not consistent with anti-apoptosis and anti-autophagy genes’ expression. This indicates apoptosis and autophagy independent pathways are responsible for the reduced survival of HEK001 in the presence of BPE. Anti-apoptosis and anti-autophagy pathways in MRSA infected HEK001 cells followed by BPE treatment may play important prophylactic roles in skin and soft tissue infections. At a first glance the MBC value of BPE against MRSA seems high. However, in conditions like skin and soft tissue infections with MRSA, BPE can be used in dressing materials and in that case, the effective concentration will be feasible to use (Muthaiyan et al., 2012).
Conclusion
Our findings suggest that phenolic extracts of blackberry and blueberry pomaces have high potential to enhance activity of current antibiotic regime, especially methicillin against MRSA. In addition, novel prophylactic substitutes can be developed from the crude extracts of blackberry and blueberry pomaces/fruits for targeting the altered pathogenicity and inhibit the growth of MRSA in both vegetative cells as well as in preformed biofilm and control infection with S. aureus regardless its antibiotic resistance patterns.
Author Contributions
SS contributed to design of the work, performing experiments, interpretation of data for the work, responsible for the integrity of the work as a whole, drafting the manuscript, and revising the work. MP contributed in conducting part of the experiments, responsible for the accuracy of the work, revising the manuscript, and the final approval of the version to be published. JJ contributed to design of the work, performing part of the experiments, interpretation of data for the work, and ensuring the integrity of the work. HT contributed in performing part of the experiments, and the acquisition and analysis of data for the work. DB contributed to the conception and design of the work, ensuring that questions related to the accuracy or integrity of any part of the work are appropriately investigated and resolved, critically revising the final approval of the version to be published, and responsibility for the integrity of the work as a whole.
Funding
This work was supported by the UME (#1108200) and MAES grants awarded to DB.
Conflict of Interest Statement
The authors declare that the research was conducted in the absence of any commercial or financial relationships that could be construed as a potential conflict of interest.
Acknowledgment
We acknowledge the collaborative of all the undergraduate interns from our laboratory.
Supplementary Material
The Supplementary Material for this article can be found online at: http://journal.frontiersin.org/article/10.3389/fmicb.2017.00253/full#supplementary-material
TABLE S1 | Biofilm formation genes and primers for MRSA.
References
Ahn, J., Almario, J. A., Salaheen, S., and Biswas, D. (2014). Physicochemical, mechanical, and molecular properties of nonlysogenic and P22-lysogenic Salmonella Typhimurium treated with citrus oil. J. Food Prot. 77, 758–764. doi: 10.4315/0362-028X.JFP-13-449
Aiyegoro, O., and Okoh, I. (2009). Use of bioactive plant products in combination with standard antibiotics: implications in antimicrobial chemotherapy. J. Med. Plants Res. 3, 1147–1152.
Alves, M. J., Ferreira, I. C., Froufe, H. J., Abreu, R. M., Martins, A., and Pintado, M. (2013). Antimicrobial activity of phenolic compounds identified in wild mushrooms, SAR analysis and docking studies. J. Appl. Microbiol. 115, 346–357. doi: 10.1111/jam.12196
Aryee, A., and Price, N. (2015). Antimicrobial stewardship–can we afford to do without it? Br. J. Clin. Pharmacol. 79, 173–181. doi: 10.1111/bcp.12417
Bancroft, E. A. (2007). Antimicrobial resistance: it’s not just for hospitals. JAMA 298, 1803–1804. doi: 10.1001/jama.298.15.1803
Clinical and Laboratory Standards Institute [CLSI] (2013). M100-S23. Performance Standards for Antimicrobial Susceptibility Testing; Twenty-Third Informational Supplement, Vol. 33. Wayne, PA: Clinical and Laboratory Standards Institute. Available at: http://reflab.yums.ac.ir/uploads/clsi_m100-s23-2013.pdf
Chambers, H. F. (2001). Methicillin-resistant Staphylococcus aureus. Mechanisms of resistance and implications for treatment. Postgrad. Med. 109(Suppl. 2), 43–50. doi: 10.3810/pgm.02.2001.suppl12.65
Chung, P. Y., Navaratnam, P., and Chung, L. Y. (2011). Synergistic antimicrobial activity between pentacyclic triterpenoids and antibiotics against Staphylococcus aureus strains. Ann. Clin. Microbiol. Antimicrob. 10:25. doi: 10.1186/1476-0711-10-25
De Lencastre, H., and Tomasz, A. (1994). Reassessment of the number of auxiliary genes essential for expression of high-level methicillin resistance in Staphylococcus aureus. Antimicrob. Agents Chemother. 38, 2590–2598. doi: 10.1128/AAC.38.11.2590
De Lencastre H., Wu, S. W., Pinho, M. G., Ludovice, A. M., Filipe, S., Gardete, S., et al. (1999). Antibiotic resistance as a stress response: complete sequencing of a large number of chromosomal loci in Staphylococcus aureus strain COL that impact on the expre ssion of resistance to methicillin. Microb. Drug Resist. 5, 163–175. doi: 10.1089/mdr.1999.5.163
Del Re, B., Sgorbati, B., Miglioli, M., and Palenzona, D. (2000). Adhesion, autoaggregation and hydrophobicity of 13 strains of Bifidobacterium longum. Lett. Appl. Microbiol. 31, 438–442. doi: 10.1046/j.1365-2672.2000.00845.x
Deutsch, S. M., Falentin, H., Dols-Lafargue, M., Lapointe, G., and Roy, D. (2008). Capsular exopolysaccharide biosynthesis gene of Propionibacterium freudenreichii subsp. shermanii. Int. J. Food Microbiol. 31, 252–258. doi: 10.1016/j.ijfoodmicro.2008.04.006
Djilas, S., Canadanovic-Brunet, J., and Cetkovic, G. (2009). By-products of fruits processing as a source of phytochemicals. Chem. Ind. Chem. Eng. Q. 15, 191–202. doi: 10.1016/j.foodchem.2016.04.011
Do Carmo, L. S., Cummings, C., Linardi, V. R., Dias, R. S., De Souza, J. M., De Sena, M. J., et al. (2004). A cause of massive staphylococcal food poisoning incident. Foodborne Pathog. Dis. 1, 241–246. doi: 10.1089/fpd.2004.1.241
Ekkelenkamp, M. B., Sekkat, M., Carpaij, N., Troelstra, A., and Bonten, M. J. (2006). Endocarditis due to meticillin-resistant Staphylococcus aureus originating from pigs. Ned. Tijdschr. Geneeskd. 150, 2442–2447.
Fair, R. J., and Tor, Y. (2014). Antibiotics and bacterial resistance in 21st century. Perspect. Med. Chem. 6, 25–64.
Federman, C., Joo, J., Almario, J. A., Salaheen, S., and Biswas, D. (2016). Citrus-derived oil inhibits Staphylococcus aureus growth and alters its interactions with bovine mammary cells. J. Dairy Sci. 99, 3667–3674. doi: 10.3168/jds.2015-10538
Gardam, M. A. (2000). Is methicillin-resistant Staphylococcus aureus an emerging community pathogens? A review of the literature. Can. J. Infect. Dis. 11, 202–211. doi: 10.1155/2000/424359
Gustafson, J. E., and Wilkinson, B. J. (2005). “Staphylococcus aureus as a food pathogen: the staphylococcal enterotoxins and stress response systems,” in Understanding Pathogen Behaviour; Virulence Stress Response and Resistance, ed. M. Griffiths (Cambridge: Woodhead Publishing Limited), 331–357.
Jang, S. (2016). Multidrug efflux pumps in Staphylococcus aureus and their clinical implications. J. Microbiol. 54, 1–8. doi: 10.1007/s12275-016-5159-z
Jiang, N., Tan, N. S., Ho, B., and Ding, J. L. (2007). Respiratory protein–generated reactive oxygen species as an antimicrobial strategy. Nat. immunol. 8, 1114–1122. doi: 10.1038/ni1501
Kennedy, A. D., Otto, M., Braughton, K. R., Whitney, A. R., Chen, L., Mathema, B., et al. (2008). Epidemic community-associated methicillin-resistant Staphylococcus aureus: recent clonal expansion and diversification. Proc. Natl. Acad. Sci. U.S.A. 105, 1327–1332. doi: 10.1073/pnas.0710217105
Kskiwiecin, J., Kahlmeter, G., and Jin, T. (2015). Biofilm formation by Staphylococcus aureus isolates from skin and soft tissue infections. Curr. Microbiol. 70, 698–703. doi: 10.1007/s00284-014-0770-x
Le Loir, Y., Baron, F., and Gautier, M. (2003). Staphylococcus aureus and food poisoning. Genet. Mol. Res. 2, 63–76.
Lechner, D., Stavri, M., Oluwatuyi, M., Pereda-Miranda, R., and Gibbons, S. (2004). The anti-staphylococcal activity of Angelica dahurica (Bai Zhi). Phytochemistry 65, 331–335. doi: 10.1016/j.phytochem.2003.11.010
Montgomery, C. P., Daniels, M. D., Zhao, F., Spellberg, B., Chong, A. S., and Daum, R. S. (2013). Local inflammation exacerbates the severity of Staphylococcus aureus skin infection. PLoS ONE 8:e69508. doi: 10.1371/journal.pone.0069508
Muthaiyan, A., Biswas, D., Crandall, P. G., Wilkinson, B. J., and Ricke, S. C. (2012). Application of orange essential oil as an antistaphylococcal agent in a dressing model. BMC Complement. Altern. Med. 12:125. doi: 10.1186/1472-6882-12-125
Peng, M., Aryal, U., Cooper, B., and Biswas, D. (2015a). Metabolites produced during the growth of probiotics in cocoa supplementation and the limited role of cocoa in host-enteric bacterial pathogen interactions. Food Control 53, 124–133. doi: 10.1016/j.foodcont.2015.01.014
Peng, M., Bitsko, E., and Biswas, D. (2015b). Functional properties of peanut fractions on the growth of probiotics and foodborne bacterial pathogens. J. Food Sci. 80, M635–M641. doi: 10.1111/1750-3841.12785
Peng, M., Reichmann, G., and Biswas, D. (2015c). Lactobacillus casei and its byproducts alter the virulence factors of foodborne bacterial pathogens. J. Funct. Foods 15, 418–428. doi: 10.1016/j.jff.2015.03.055
Pu, S., Han, F., and Ge, B. (2009). Isolation and characterization of methicillin-resistant Staphylococcus aureus strains from Louisiana retail meats. Appl. Environ. Microbiol. 75, 265–267. doi: 10.1128/AEM.01110-08
Puupponen-Pimiä, R., Nohynek, L., Ammann, S., Oksman-Caldentey, K. M., and Buchert, J. (2008). Enzyme-assisted processing increases antimicrobial and antioxidant Activity of bilberry. J. Agric. Food Chem. 56, 681–688. doi: 10.1021/jf072001h
Puupponen-Pimiä, R., Nohynek, L., Hartmann-Schmidlin, S., Kähkönen, M., Heinonen, M., Määttä-Riihinen, K., et al. (2005). Berry phenolics selectively inhibit the growth of intestinal pathogens. J. Appl. Microbiol. 98, 991–1000. doi: 10.1111/j.1365-2672.2005.02547.x
Rasmussen, T. B., and Givskov, M. (2006). Quorum-sensing inhibitors as anti-pathogenic drugs. Intern. J. Med. Microbiol. 296, 149–161. doi: 10.1016/j.ijmm.2006.02.005
Rosenberg, M. (1984). Bacterial adherence to hydrocarbons: a useful technique for studying cell surface hydrophobicity. FEMS Microbiol. Lett. 22, 289–295. doi: 10.1111/j.1574-6968.1984.tb00743.x
Salaheen, S., Jaiswal, E., Joo, J., Peng, M., Ho, R., O’Connor, D., et al. (2016). Bioactive extracts from berry byproducts on the pathogenicity of Salmonella Typhimurium. Int. J. Food Microbiol. 237, 128–135. doi: 10.1016/j.ijfoodmicro.2016.08.027
Salaheen, S., Nguyen, C., Hewes, D., and Biswas, D. (2014). Cheap extraction of antibacterial compounds of berry pomace and their mode of action against the pathogen Campylobacter jejuni. Food Control 46, 174–181. doi: 10.1016/j.foodcont.2014.05.026
Salaheen, S., Nguyen, C., Mui, C., and Biswas, D. (2015). Bioactive berry juice byproducts as alternative and natural inhibitors for Salmonella Gallinarum and Salmonella Pullorum. J. Appl. Poult. Res. 24, 186–197. doi: 10.3382/japr/pfv021
Salem, H., and Saleh, G. (2002). Selective spectrophotometric determination of phenolic beta-lactam antibiotics. J. Pharm. Biomed. Anal. 28, 1205–1213. doi: 10.1016/S0731-7085(02)00027-4
Sasaki, R., Nishimura, N., Hoshino, H., Isa, Y., Kadowaki, M., Ichi, T., et al. (2007). Cyanidin 3-glucoside ameliorates hyperglycemia and insulin sensitivity due to downregulation of retinol binding protein 4 expression in diabetic mice. Biochem. Pharmacol. 74, 1619–1627. doi: 10.1016/j.bcp.2007.08.008
Sharma-Kuinkel, B. K., Zhang, Y., Yan, Q., Ahn, S. H., and Fowler, V. G. Jr. (2013). Host gene expression profiling and in vivo cytokine studies to characterize the role of linezolid and vancomycin in methicillin-resistant Staphylococcus aureus (MRSA) murine sepsis model. PLoS ONE 8:e60463. doi: 10.1371/journal.pone.0060463
Singleton, V. L., Orthofer, R., and Lamuela-Raventos, R. M. (1999). Analysis of total phenols and other oxidation substrates and antioxidants by means of Folin-Ciocalteu reagent. Methods Enzymol. 299, 152–178. doi: 10.1016/S0076-6879(99)99017-1
Stapleton, P. D., Shah, S., Anderson, J. C., Hara, Y., Hamilton-Miller, J. M., and Taylor, P. W. (2004). Modulation of beta-lactam resistance in Staphylococcus aureus by catechins and gallates. Int. J. Antimicrob. Agents 23, 462–467. doi: 10.1016/j.ijantimicag.2003.09.027
Strober, W. (2001). Trypan blue exclusion test of cell viability. Curr. Protoc. Immunol. Appendix 3:Aendix3B. doi: 10.1002/0471142735.ima03bs21
Tang, C. S., Wang, C. C., Huang, C. F., Chen, S. J., Tseng, M. H., and Lo, W. T. (2011). Antimicrobial susceptibility of Staphylococcus aureus in children with atopic dermatitis. Pediatr. Int. 53, 363–367. doi: 10.1111/j.1442-200X.2010.03227.x
Wilkinson, B. J., Muthaiyan, A., and Jayaswal, R. K. (2005). The cell wall stress stimulon of Staphylococcus aureus and other Gram-positive bacteria. Curr. Med. Chem. Antiinfect. Agents 4, 259–276. doi: 10.2174/1568012054368119
Wulf, M. W., Markestein, A., van der Linden, F. T., Voss, A., Klaassen, C., and Verduin, C. M. (2007). First outbreak of methicillin-resistant Staphylococcus aureus ST398 in a Dutch hospital, June 2007. Euro. Surveill 13:8051.
Wulf, M. W., Sørum, M., van Nes, A., Skov, R., Melchers, W. J., Klaassen, C. H., et al. (2008). Prevalence of methicillin-resistant Staphylococcus aureus among veterinarians: an international study. Clin. Microbiol. Infect. 14, 29–34. doi: 10.1111/j.1469-0691.2007.01873.x
Keywords: Staphylococcus aureus, phenolic compounds, antibiotic resistance, antimicrobials, gene expression
Citation: Salaheen S, Peng M, Joo J, Teramoto H and Biswas D (2017) Eradication and Sensitization of Methicillin Resistant Staphylococcus aureus to Methicillin with Bioactive Extracts of Berry Pomace. Front. Microbiol. 8:253. doi: 10.3389/fmicb.2017.00253
Received: 10 November 2016; Accepted: 07 February 2017;
Published: 21 February 2017.
Edited by:
Patrick Rik Butaye, Ghent University, BelgiumReviewed by:
Liang Li, Los Angeles Biomedical Research Institute at Harbor-UCLA Medical Center, USALeila Vali, Kuwait University, Kuwait
Pramod Kumar, National Centre for Disease Control, India
Copyright © 2017 Salaheen, Peng, Joo, Teramoto and Biswas. This is an open-access article distributed under the terms of the Creative Commons Attribution License (CC BY). The use, distribution or reproduction in other forums is permitted, provided the original author(s) or licensor are credited and that the original publication in this journal is cited, in accordance with accepted academic practice. No use, distribution or reproduction is permitted which does not comply with these terms.
*Correspondence: Debabrata Biswas, ZGJpc3dhc0B1bWQuZWR1
†These authors have contributed equally to this work.