- 1Guangdong Key Laboratory for Veterinary Drug Development and Safety Evaluation, South China Agricultural University, Guangzhou, China
- 2Technical Center for Inspection and Quarantine, Zhuhai Entry-Exit Inspection and Quarantine Bureau, Zhuhai, China
Mycoplasma gallisepticum is the causative agent of chronic respiratory disease (CRD), a prevalent disease of poultry, which is responsible for significant economic losses in farms. Although several antimicrobial agents are currently recommended for the treatment and prevention of M. gallisepticum infections, investigations of M. gallisepticum have been hampered by their fastidious growth requirements and slow growth rate. As such, little work has been conducted concerning the PK/PD relationship and mechanisms of antibiotic resistance between antimicrobials against M. gallisepticum. In the present study, danofloxacin was orally administrated to the infected chickens once daily for 3 days by an established in vivo M. gallisepticum infection model. Not only the concentrations of danofloxacin in plasma and lung tissues were analyzed, but also the counting of viable cells and changes in antimicrobial susceptibility in air sac and lung were determined. The PK and PD data were fitted by WinNonlin to evaluate the PK/PD interactions of danofloxacin against M. gallisepticum. PCR amplification of quinolone resistance-determining regions (QRDRs) and DNA sequencing were performed to identify point mutations in gyrA, gyrB, parC, and parE of the selected resistant mutant strains. In addition, susceptibility of enrofloxacin, ofloxacin, levofloxacin, gatifloxacin, and norfloxacin against these mutant strains were also determined. The PK profiles indicated that danofloxacin concentration in the lung tissues was higher than plasma. Mycoplasmacidal activity was achieved when infected chickens were exposed to danofloxacin at the dose group above 2.5 mg/kg. The ratios of AUC24/MIC (the area under the concentration-time curve over 24 h divided by the MIC) for 2 log10 (CFU) and 3 log10 (CFU) reduction were 31.97 and 97.98 L h/kg, respectively. Substitutions of Ser-83→Arg or Glu-87→Gly in gyrA; Glu-84→Lys in parC were observed in the resistant mutant strains that were selected from the dose group of 1 and 2.5 mg/kg. MICs of danofloxacin, enrofloxacin, ofloxacin, levofloxacin, gatifloxacin, and norfloxacin against the resistant mutant strains with a single mutation in position-83 were higher than that with a single mutation in position-87. These findings suggested that danofloxacin may be therapeutically effective to treat M. gallisepticum infection in chickens if administered at a dosage of 5.5 mg/kg once daily for 3 days.
Introduction
Mycoplasma gallisepticum is a primary pathogen that is responsible for chronic respiratory disease (CRD) in chickens as well as sinusitis in turkeys. The primary signs of M. gallisepticum infections include nasal discharge, keratoconjunctivitis, air sacculitis, and depression (Levisohn and Kleven, 2000). M. gallisepticum infections cause considerable economic losses in poultry industry as a result of increased embryo mortality, reduced egg production and decreased weight gain (Kleven, 1990). M. gallisepticum can be transmitted horizontally from infected individuals to healthy individuals by aerosol route or vertically via eggs. Control of M. gallisepticum infections with antimicrobial therapy is usually effective and economically feasible. M. gallisepticum is susceptible to some antibacterial agents in vitro, such as quinolones, tetracyclines, macrolides, and pleuromutilins. However, increasing drug resistance in M. gallisepticum has been discovered in the past decades (Pakpinyo and Sasipreeyajan, 2007; Gerchman et al., 2008). Danofloxacin, one of the synthetic quinolones, has play a significant role in treatment of M. gallisepticum infections (Jordan et al., 1993).
Mycoplasma gallisepticum should be cultivated on specially formulated media, for the reason that Mycoplasma is dependent on outside sources of precursor molecules for macromolecular syntheses (Razin and Tully, 1970; Greenberg-Ofrath et al., 1993). Moreover, viable count estimation (CFU determination) is difficult by the strict nutritional conditions for growth. Therefore, there were a few reports concerning the PK/PD relationship and mechanisms of antibiotic resistance between antimicrobials against M. gallisepticum. In the past years, in vitro models, ex vivo models, and in vivo models have been established to evaluate the characteristics of antibacterial agents against M. gallisepticum (Xiao et al., 2015a,b; Zhang et al., 2016). Antimicrobial chemotherapy with an optimal dose-response outcome may be based on the relationship of PK/PD in vivo model (Vinks et al., 2014).
Most of the published articles indicated that the substitutions in quinolone resistance-determining region (QRDR) were generally induced by point mutations in topoisomerase and gyrase. The mutations in QRDR of gyrA, gyrB, parC, and parE were correlated with the development of quinolone resistance in M. gallisepticum (Reinhardt et al., 2002a,b; Lysnyansky et al., 2008, 2012). After different dosage regimens, the resistant mutant strains were selected from the tissues. Then mutations in the QRDR of gyrA, gyrB, parC, and parE were identified in the current study. The purpose of this study is to characterize the PK–PD relationship and resistance development of danofloxacin against M. gallisepticum in an in vivo infection model where viable cells count estimation was performed by isolating M. gallisepticum from the infected chickens.
Materials and Methods
Organisms and Chemicals
Mycoplasma gallisepticum strain S6 was obtained from the Chinese Veterinary Microorganism Culture Collection Center (Beijing, China). It was initially isolated from Nippon Institute for biological science in 1958 (Adler et al., 1958). Moreover, S6 is a recognized common clinical isolate in China. Danofloxacin mesylate standard (>99%), enrofloxacin standard (98%), ofloxacin standard (97%), levofloxacin standard (97%), gatifloxacin standard (99%), norfloxacin standard (98%), and penicillin sodium were kindly supplied by Guangdong Dahuanong Animal Health Products.
Media
Mycoplasma gallisepticum growth medium base was provided by Qingdao Hope Biological Technology. Sterile pig serum was purchased from Guangzhou Ruite Biological Technology. Nicotinamide adenine dinucleotide (NADH) and cysteine were obtained from Guangzhou Prob Information Technology. The M. gallisepticum growth medium base was dissolved with water and autoclaved at 115°C for 30 min. NADH and Cysteine were sterilized by 0.22 μm filtration and then added to the M. gallisepticum medium base at a final concentration of 0.2%. Inactivated pig serum was further supplemented at the final concentration of 10%. Eighty (80) million IU of penicillin was also added into the prepared medium. Finally, its pH was adjusted to 7.8 ± 0.1 with 1 mol/L NaOH and kept at 4°C for use within 2 weeks. Solid medium was prepared by adding 1% agar into the growth medium. The culture of M. gallisepticum was diluted with the growth medium by 10-fold serial dilutions, then 10 μL of each M. gallisepticum dilutions were transferred to the surface of M. gallisepticum agar plates. After being sealed with gas permeable film, the plates were incubated for at least 7 days in a 37°C, 5% CO2 humidified incubator and colonies of M. gallisepticum were counted by the inverted microscope (Leica Microsystems DM1L, Germany).
Susceptibility Determination
The standard of 0.33 g danofloxacin mesylate, 0.261 g enrofloxacin, 0.264 g ofloxacin, 0.264 g levofloxacin, 0.259 g gatifloxacin, and 0.261 g norfloxacin were dissolved in 100 mL sterile distilled water to get the initial concentration of 2560 mg/L, respectively. The final drug concentration of agar plates ranged from 0.019 to 38.40 mg/L in double dilution.
The in vitro susceptibility of danofloxacin, enrofloxacin, ofloxacin, levofloxacin, gatifloxacin, and norfloxacin against M. gallisepticum strain S6 as well as danofloxacin resistant mutant strains isolated from chickens were determined by the agar dilution methods as described by Hannan (2000). The single colony was selected and cultured in drug-free growth medium for 5 days. When color of the medium changed from red to orange or yellow (M. gallisepticum was in logarithmic phase), the concentration of M. gallisepticum was 108 CFU/mL. The culture was diluted to 107 CFU/mL by the blank growth medium. Then a 10 μL sample of M. gallisepticum culture (107 CFU/mL) was inoculated onto agar plates containing two-fold serial dilutions of quinolones. Growth control (M. gallisepticum inoculum without quinolones) and sterility control (blank medium) were also included in the MIC determination. E. coli ATCC 25922 was used for quality control strain. The MIC was determined as the minimal concentration of antibacterial agent that resulted in no growth on the agar plate after 7 days.
Animals and Inoculation
All in vivo experiments were approved by the animal research committees of South China Agriculture University Animal Ethics Committee (Approve number: 2015 A027). One-day-old chickens were provided from Guangdong Academy of Agricultural Sciences (Guangzhou, Guangdong, China). The chickens were M. gallisepticum free and fed with antimicrobial-free feed.
In preliminary experiments, the chickens were inoculated with a 0.2-mL aliquot solution containing 107, 108, and 109 CFU/mL of M. gallisepticum (twice a day) via intratracheal injection for 3 days (n = 30/group). After inoculation, the infected chickens were anatomized. The signs of disease and pathological changes were observed to validate the success of the experimental infection. M. gallisepticum ELISA kit were purchased from Shenzhen Lvshiyuan Biotechnology Technology. Serum was also collected from the infected chickens and the antibody of M. gallisepticum was measured by the commercially available ELISA kits according to the supplier’s instructions. The optical density at 450 nm (OD450) was recorded using a universal Microplate Reader ELx800 (Bio-Tek Instruments, United States). Besides, the load of M. gallisepticum in air sac and lung were monitored to confirm infection. To ensure the accuracy of the results, this experimentation was performed for three times. All chickens were housed in the same animal room and received identical daily care. Moreover, the feeding environment was controlled rigidly in order to prevent other M. gallisepticum infection.
Evaluation of the M. gallisepticum infection model was mainly based on the clinical signs and colonization of M. gallisepticum. The infected chicken exhibit mostly respiratory presentations including ocular and nasal discharge, sneezing, cough, breathing difficulty/mouth breathing, and moist rales. Catarrhal inflammation (sinuses, trachea, and bronchi), the thickened and opaque of air sacs and lung enlargement were found in most of M. gallisepticum infected chickens. Additionally, M. gallisepticum specific antibody was observed in the infected chickens.
A total of 1140 chickens were randomly divided into five groups. (1) A group of 840 chickens were used to study the pharmacokinetics of danofloxacin in infected chickens; (2) A group of 210 chickens were used to study the pharmacodynamics of danofloxacin in infected chickens; (3) 40 chickens were used as growth control group to observe the load of M. gallisepticum in infected chickens; (4) 40 chickens were used as negative control group to observe the load of M. gallisepticum in healthy chickens; (5) The remaining 10 health chickens were used as blank control to obtain blank plasma and lung tissue. The chickens in growth and negative control group were administrated by oral gavage with 0.85% NaCl once daily for 3 days. The chickens in blank group were untreated and the blank samples (plasma and lung tissues) were collected before the experiment.
Danofloxacin Pharmacokinetics in In Vivo Infection Model
The chickens were inoculated with a 0.2-mL aliquot solution containing 109 CFU/mL of M. gallisepticum (twice a day) via intratracheal injection for 3 days. Groups of infected chickens were orally administrated by oral gavage with danofloxacin at a dose of 1, 2.5, 5, 10, or 20 mg/kg once daily for 3 days, and they were euthanized at 0.25, 0.5, 1, 2, 4, 6, 8, 12, 24, 25, 26, 28, 32, 36, 48, 49, 50, 52, 56, 60, and 72 h after the first oral gavage of danofloxacin. Blood and lung tissues were collected from eight chickens at each sampling time point per treatment group. Blood samples were centrifuged at 3,000 × g for 10 min at 4°C, and then plasma was collected. The samples of plasma and lung tissues were stored at -20 until analyzed by HPLC within 2 weeks.
Danofloxacin concentrations in plasma and lung tissues were determined by high performance liquid chromatography with fluorescence detection (HPLC-FD) (Agilent Technologies, United States). The HPLC was equipped with an Agilent TC-C18 column (250 mm × 4.6 mm, 5 μm) using a mobile phase of triethylamine phosphate (pH 2.4): acetonitrile (19:81, v/v) and a flow rate of 0.8 mL/min. Injection volume was 20 μL. A calibration curve was established with seven danofloxacin concentrations (0.001–0.1 μg/mL).
Plasma sample (0.5 mL) was added to 0. 5 mL of acetonitrile, vortexed for 1 min and then incubated in a 45°C water bath for 10 min to precipitate proteins. After centrifugation at 10,000 × g at 4°C for 5 min, 0.5 mL of supernatant were transferred to a centrifuge tube containing 0.5 mL of ultra-pure water. Finally, the mixture was vortexed for 30 s and filtered through a 0.22 μm syringe filter prior to HPLC analysis.
Lung tissue sample (0.15 g) was homogenized and then 1.5 mL of Mcllvaine–Na2EDTA buffer (PH 4.0 ± 0.5) was added to extract danofloxacin. The samples were vortexed for 1 min and incubated in a 45°C water bath for 10 min to precipitate proteins. After centrifugation at 10,000 × g at 4°C for 5 min, the supernatant was filtered through a 0.22 μm syringe filter prior to HPLC analysis.
Pharmacokinetic parameters, including elimination half-life (t1/2), the area under the concentration-time curve over 24 h (AUC24), and maximum concentration of drug in samples (Cmax), the time of peak concentration (Tmax) were calculated by the non-compartmental model using the WinNonlin software (version 6.1, Pharsight).
Statistical Analysis
T-test was used for statistical analysis. The differences of morbidity and mortality of chickens in different inoculum sizes as well as Tmax and t1/2 of danofloxacin in plasma and lung were calculated. P < 0.05 was considered to be statistically significant.
Efficacy of Danofloxacin against M. gallisepticum in Chicken Infection Model and Changes in Susceptibility
The chickens were inoculated with a 0.2-mL aliquot solution containing 109 CFU/mL of M. gallisepticum (twice a day) via intratracheal injection for 3 days. Then the infected chickens were administrated by oral gavage with danofloxacin at the dose of 1, 2.5, 5, 7.5, 10, 15, or 20 mg/kg once daily for 3 days. At 24 h after each drug administration, the chickens were euthanized. The air sacs and lung tissues were aseptically collected and homogenized in 1 mL of medium. Viable cell numbers were determined via 10-fold serial dilutions and plating 10 μL of each diluted culture onto agar plates without danofloxacin. The samples (air sacs and lung tissues) were obtained from 10 chickens at each sampling time point per treatment group. Values for reduction less than 3 log10 CFU denoted mycoplasmastasis activity; whereas values more than or equal to 3 log10 CFU indicated mycoplasmacidal.
When the chickens were euthanized at 72 h following the initial administration, the samples were homogenized and vortexed in 1 mL of medium, then 50 μL samples were incubated in 10 mL drug-free growth medium. When color of the medium changed to yellow (M. gallisepticum was cultured in logarithmic phase), the MIC was determined by the agar dilution method using the agar plates containing serial danofloxacin concentrations. A two-fold increase of danofloxacin concentrations were used in susceptibility determination, which ranged from 1XMIC to 128XMIC. The resistant mutants could be selected when danofloxacin concentrations of the agar plates were above MIC.
PK–PD Analysis
Pharmacokinetic profiles of danofloxacin in lung tissues were analyzed by non-compartmental model using WinNonlin software (version 6.1; Pharsight, CA, United States). The pharmacokinetic–pharmacodynamic index of AUC24/MIC was calculated using in vitro MIC values and PK parameters derived from danofloxacin concentration in lung tissues samples. Effectiveness of danofloxacin was reflected as the reduction in viable cells of M. gallisepticum in lung tissues after each treatment compared to the control group. The PK/PD analysis was performed by using a sigmoid maximum effect (Emax) model. This model is described by the following equation:
Where E is the antibacterial effect that was assessed as the reduction in log10 CFU/lung after each administration of danofloxacin, compared to the log10 CFU/lung in untreated control group; Emax is the reduction in log10 CFU/lung for the untreated control chickens; E0 is the maximum reduction after administration that represents the maximum antibacterial effect; Ce is the AUC24/MIC parameter; EC50 is the AUC24/MIC value required to achieve 50% of the maximal antibacterial effect; and N is the Hill coefficient that describes the steepness of the AUC24/MIC and effect curve.
Dosage Calculation
For the purpose of deducing a optimal regimen, the dose required for different magnitudes of efficiency is provided by the following equation described by Toutain et al. (2007):
Where dose (per day) is the optimal dose (mg/kg⋅bw); AUC/MIC is the targeted end point for the desired effect (L⋅h/kg); MIC90 is the 90% of the MIC distribution (mg/L); and clearance is the lung clearance expressed as kg/kg/h (CLperhour was 0.373 kg/kg/h). fu is the free drug fraction, and F is the bioavailability.
PCR Amplification of Quinolone Resistance-Determining Regions (QRDRs) and DNA Sequencing
The components used for PCR (Polymerase Chain Reaction) were obtained from Takara Bio. The resistant mutant strains were conserved firstly when selected from the agar plates containing serial danofloxacin concentrations. In this stage, three individual mutant strain of each sample with increased MICs were selected and conserved. Single colony of these mutants was passaged for five generations independently. The mutations in gyrA, gyrB, parC, and parE were identified before and after their respective passages to determine whether the mutations could be inherited steadily. DNA extraction of the selected mutants and PCR amplification were performed using a previously described protocol (Ley et al., 1997). gyrA, gyrB, parC, and parE were amplified by the specific primers designed from strain S6 of M. gallisepticum (Table 1). Amplify reaction was achieved as following: PCR mixture was consisted of 2.5 μL 10 × EX Taq buffer (Mg2+ plus), 2.5 μL dNTP, 0.5 μL primer F (10 μmol/mL), 0.5 μL primer R (10 μmol/mL), 1 μL of extracted M. gallisepticum DNA, 0.25 μL EX Taq and 17.75 μL distilled water. Positive control (M. gallisepticum strain S6) and blank control (distilled water) were also performed concurrently for each reaction. The amplification conditions were at 94°C for 5 min, 94°C for 45 s, 55°C for 45 s, 72°C for 1 min for 30 cycles. The final extension cycles were at 72°C for 5 min. After amplification, the sequencing reaction was performed by Beijing Genomics Institute using Sanger sequencing.
Results
Susceptibility Determination
The MICs of danofloxacin, enrofloxacin, ofloxacin, levofloxacin, gatifloxacin, norfloxacin against M. gallisepticum S6 parental and its mutant strains are shown in Table 2. For culture 1, mutant strains selected from the dose group of 1 mg/kg had a (Ser-83→Arg) substitution at the position corresponding to amino acid 83 in gyrA of E. coli. For culture 2, mutant strains selected from the dose group of 1 mg/kg carried a (Glu-87→Gly) substitution at the position of 87 in gyrA of E. coli. For culture 3, mutant strains selected from the dose group of 2.5 mg/kg had a (Ser-83→Arg) substitution at the position corresponding to amino acid 83 in gyrA of E. coli. For culture 4, mutant strains selected from the dose group of 2.5 mg/kg and had a (Ser-83→Arg) substitution at the position corresponding to amino acid 83 in gyrA of E. coli and an additional (Glu-84→Lys) substitution at the position corresponding to amino acid 84 in parC of E. coli. Gatifloxacin was found to exhibit marginally superior activity against M. gallisepticum and its MIC was 0.038 mg/L for S6; 0.6 mg/L for resistant mutant culture 1, 3, and 4; 0.15 mg/L for mutant culture 2, respectively. The mutant culture 1, 3, 4 harboring a (Ser-83→Arg) substitution in gyrA had an increased MIC to danofloxacin, enrofloxacin, ofloxacin, levofloxacin, gatifloxacin, norfloxacin. This value was 4- to 16-fold higher than culture 2 containing a (Glu-87→Gly) substitution in gyrA.
M. gallisepticum Infection Model
The inoculum size, morbidity, mortality, M. gallisepticum load in air sac and lung are presented in Table 3. When the inoculum size was 7.26 log10 CFU/mL, the morbidity and mortality were far lower than that of 8.33 and 9.31 log10 CFU/mL. There were no significant differences in morbidity (p = 0.12) and mortality (p = 0.58) between the inoculum size of 8.33 and 9.31 log10 CFU/mL, but different number of colonies was observed in air sac and lung. M. gallisepticum load in air sac and lung appeared to always depend on the inoculum size. As the inoculum size increased, the viable count of M. gallisepticum in air sac and lung increased evidently. When the inoculum size was 9.31 log10 CFU/mL, M. gallisepticum load in air sac and lung were approximately 0.5 log10 CFU/mL more than that of 8.33 log10 CFU/mL. Based on the morbidity, mortality, M. gallisepticum load in air sac and lung, the best inoculum size for the infection model was 109 CFU/mL. Neither clinical signs of disease nor antibody of M. gallisepticum were observed in negative control group. When the viable cells of air sac and lung from the negative control group were quantitated, no M. gallisepticum was found from the agar plates.
PK Profiles of Danofloxacin in Infection Model
The time-concentration curves of danofloxacin in plasma and lung tissues after three oral administrations at doses of 1, 2.5, 5, 10, and 20 mg/kg are shown in Figures 1, 2, respectively. The main PK parameters obtained from plasma and lung tissues are presented in Tables 4, 5, respectively. The mean Tmax was 2.20 ± 0.3 h in plasma and 2.40 ± 1.01 h in lung tissues for all five different doses. There were no significant differences in Tmax between lung and plasma (p = 0.68). The mean half-life (T1/2) was 11.5 ± 1.00 h in plasma and 9.2 ± 1.34 h in lung tissues. There were significant differences in t1/2 between lung and plasma (p = 0.0092). Besides, the PK parameters were dose-dependent, where the AUC24 values for the escalating doses ranged from 1.49 to 14.54 (μg⋅h/mL) in plasma and 2.72 to 58.58 (μg⋅h/g) in lung tissues, respectively. A significant correlation (R2> 0.96) was found between dose and AUC24 according to the linear relationship for each day after three oral administrations doses ranged from 1 to 20 mg/kg.
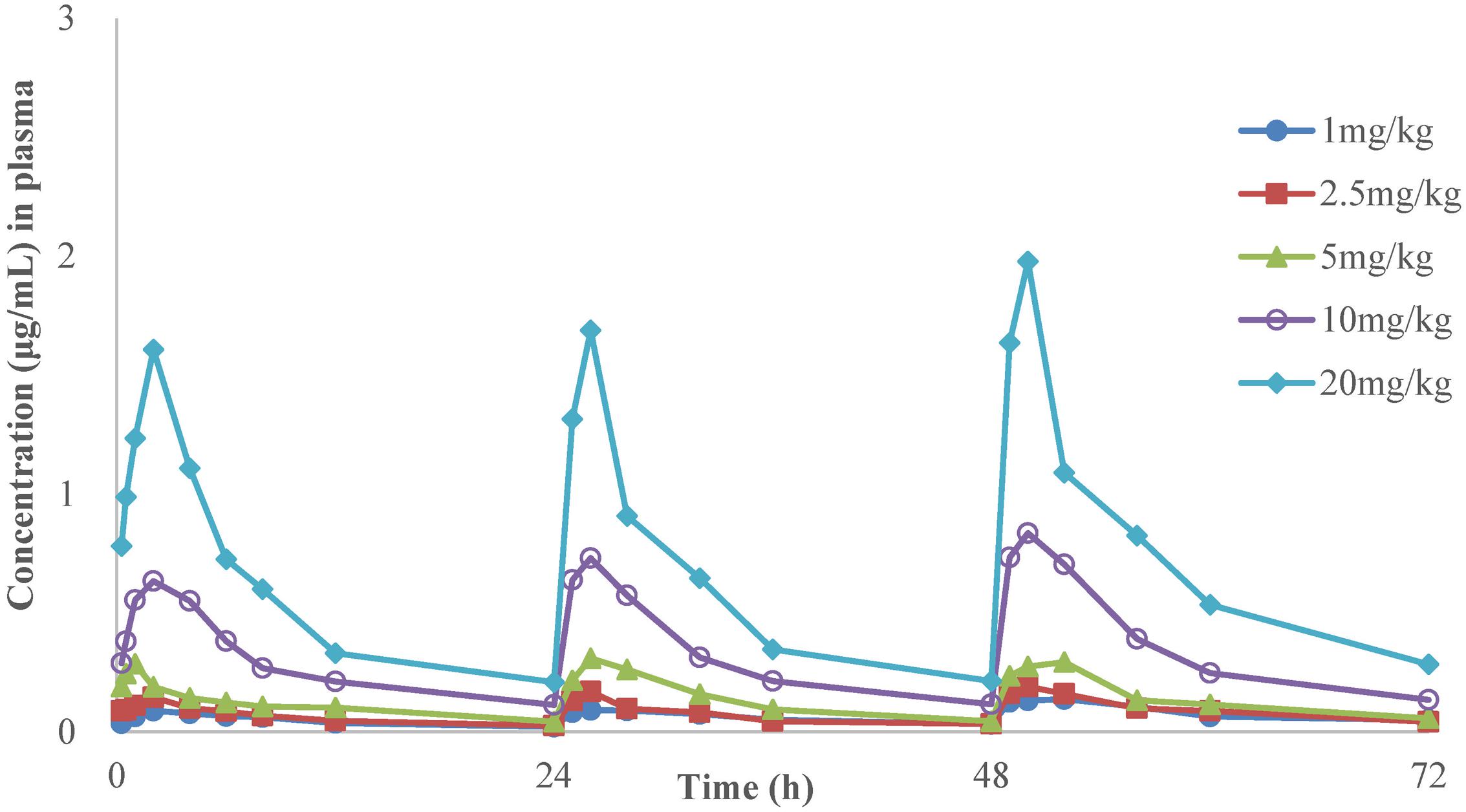
FIGURE 1. The time-concentration curves of danofloxacin in plasma after three oral administrations doses of 1, 2.5, 5, 10, and 20 mg/kg in M. gallisepticum infection model (n = 8/time point).
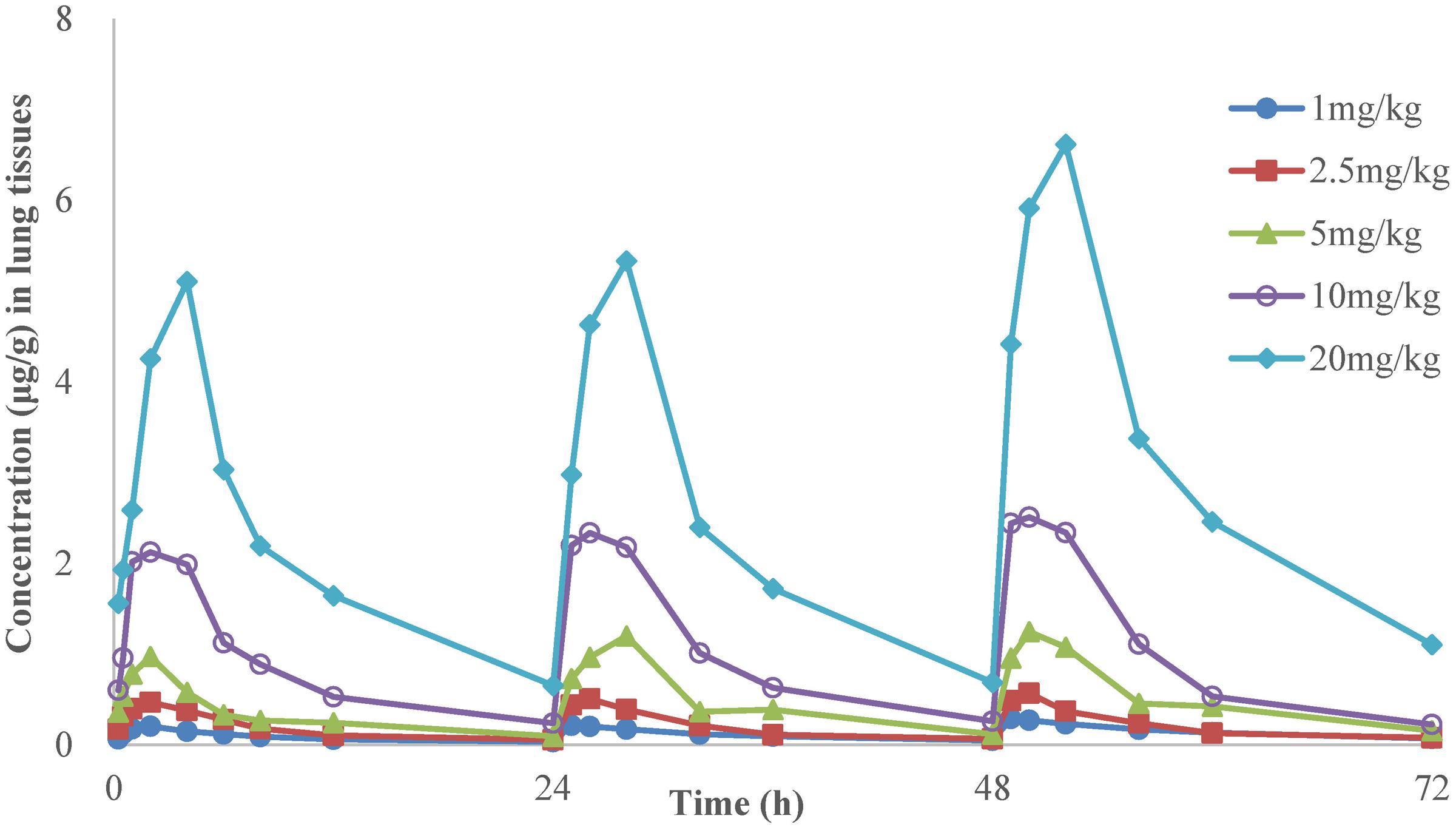
FIGURE 2. The time-concentration curves of danofloxacin in lung tissues after three oral administrations doses of 1, 2.5, 5, 10, and 20 mg/kg in M. gallisepticum infection model (n = 8/time point).
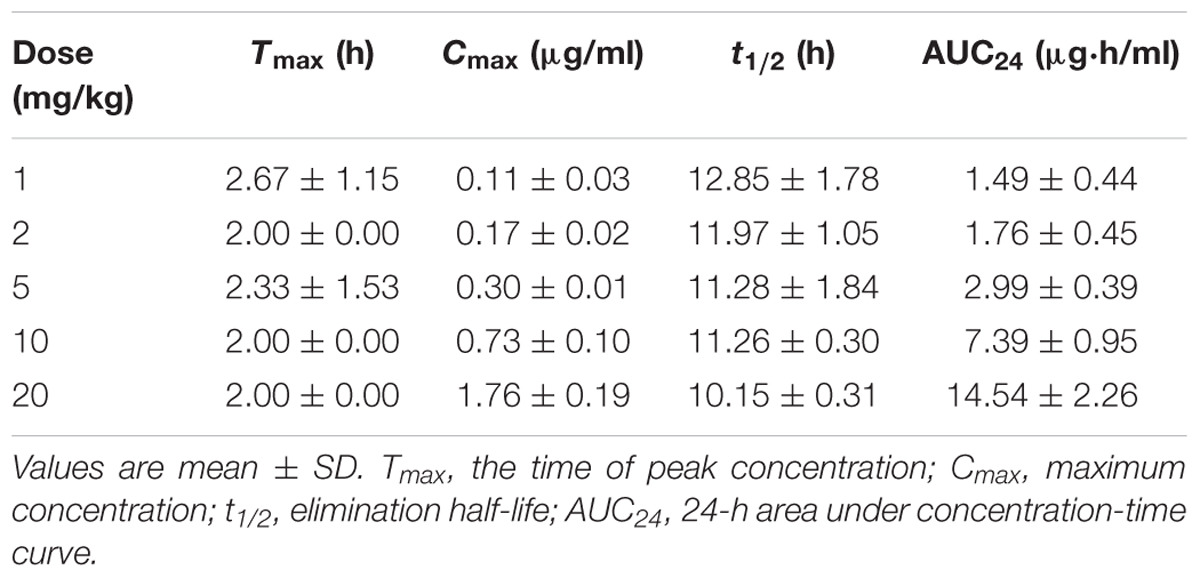
TABLE 4. Pharmacokinetic parameters of danofloxacin in plasma following three oral administrations of various doses in M. gallisepticum infected chickens.
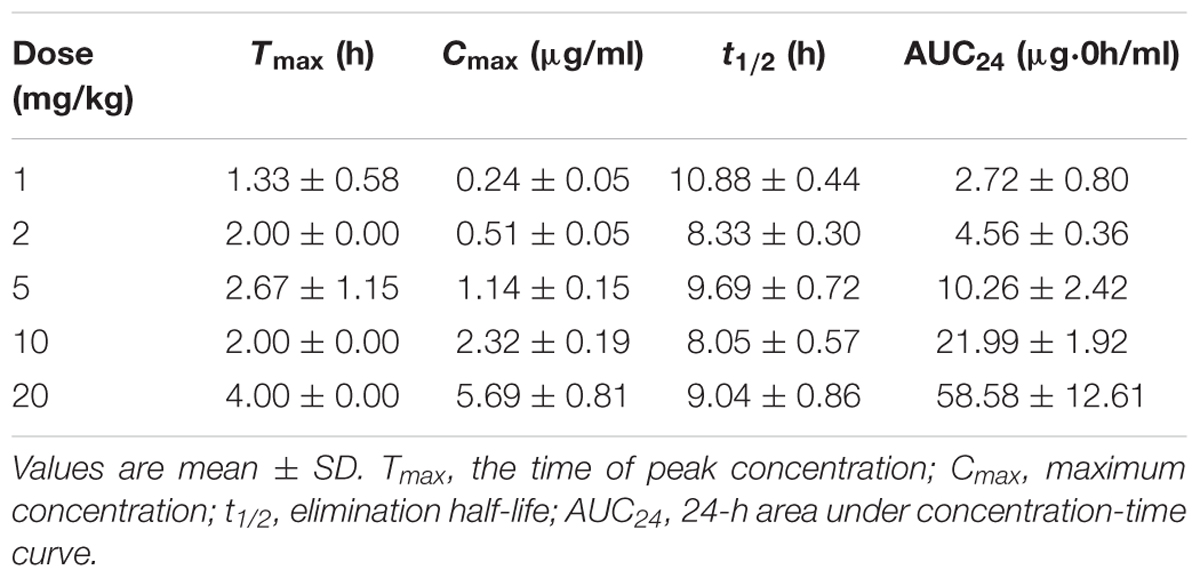
TABLE 5. Pharmacokinetic parameters of danofloxacin in lung tissues following three oral administrations of various doses in M. gallisepticum infected chickens.
PD of Danofloxacin in Chicken Infection Model and Susceptibility Changes
The effects of danofloxacin against M. gallisepticum in air sac and lung tissues with different regimens are shown in Figures 3, 4, respectively. As danofloxacin concentration increased, antimycoplasmal activity gradually increased. Both in air sac and lung, mycoplasmacidal activity was observed when M. gallisepticum was exposed to concentration of danofloxacin at a dose above 2.5 mg/kg. When the dose was at 1 mg/kg, the MICs increased to 0.6 mg/L in 4 out of 20 samples and the MICs increased to 4.8 mg/L in the remaining 16 samples. The mutants were selected from the agar plates whose danofloxacin concentrations were 0.3 or 2.4 mg/L, respectively. When the dose was 2.5 mg/kg, a decreased susceptibility was observed in all of the samples, and the MICs increased prominently to 4.8 mg/L. The mutants were selected from the agar plates whose danofloxacin concentration was 2.4 mg/L. Three individual mutant strain of each sample with increased MICs were passaged for five times. When the doses were at 5 and 7.5 mg/kg, the MIC of danofloxacin against the survival cells was determined. The danofloxacin MIC of the parental strain M. gallisepticum was 0.15 and no mutants were observed in the agar plate containing danofloxacin ≥0.15 mg/L. When the doses were at 10, 15, and 20 mg/kg, no survival cells were recovered in the treatment groups at 72 h.
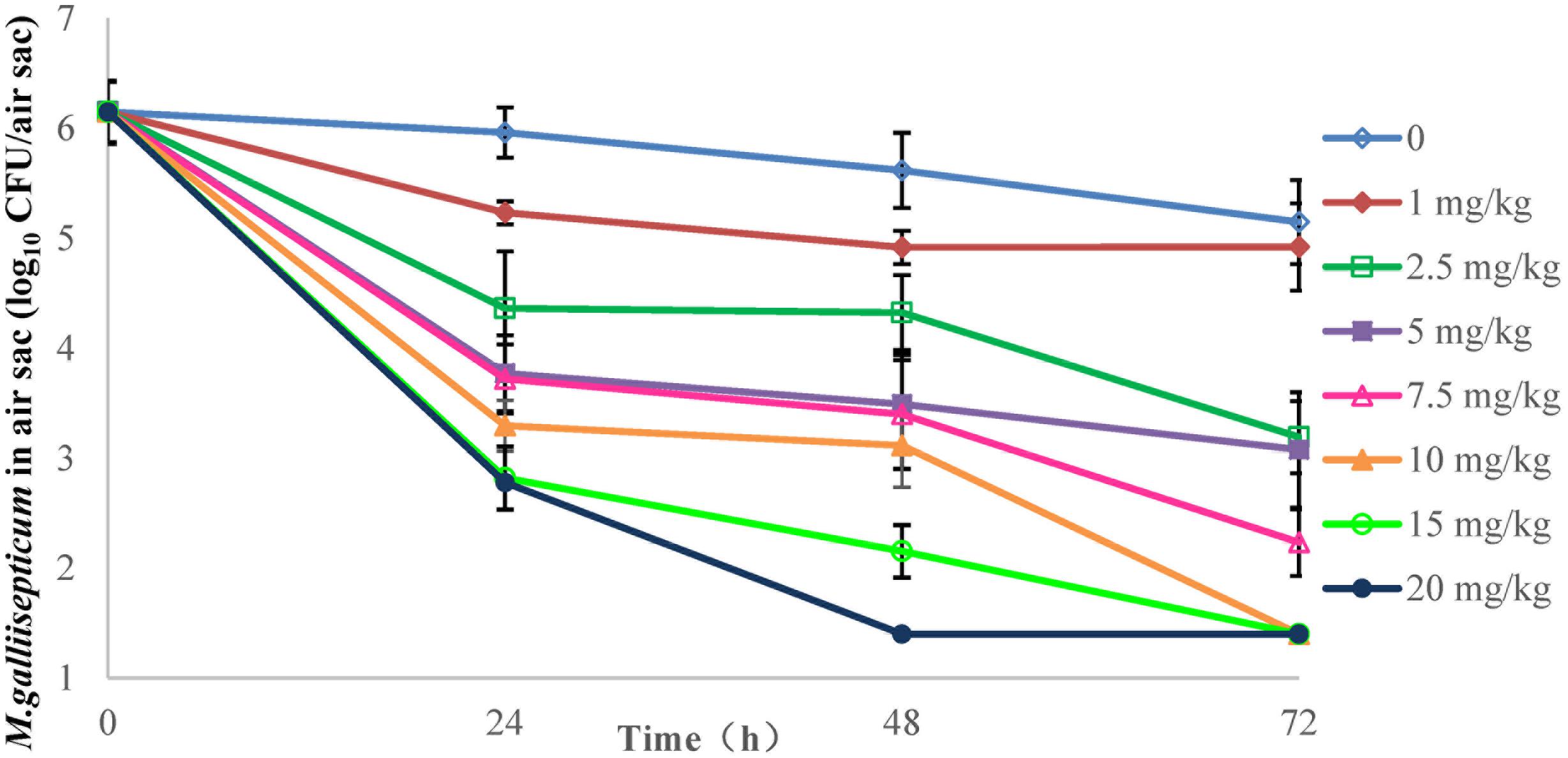
FIGURE 3. Viable counts of M. gallisepticum in air sac after three oral administrations of danofloxacin (n = 10/time point).
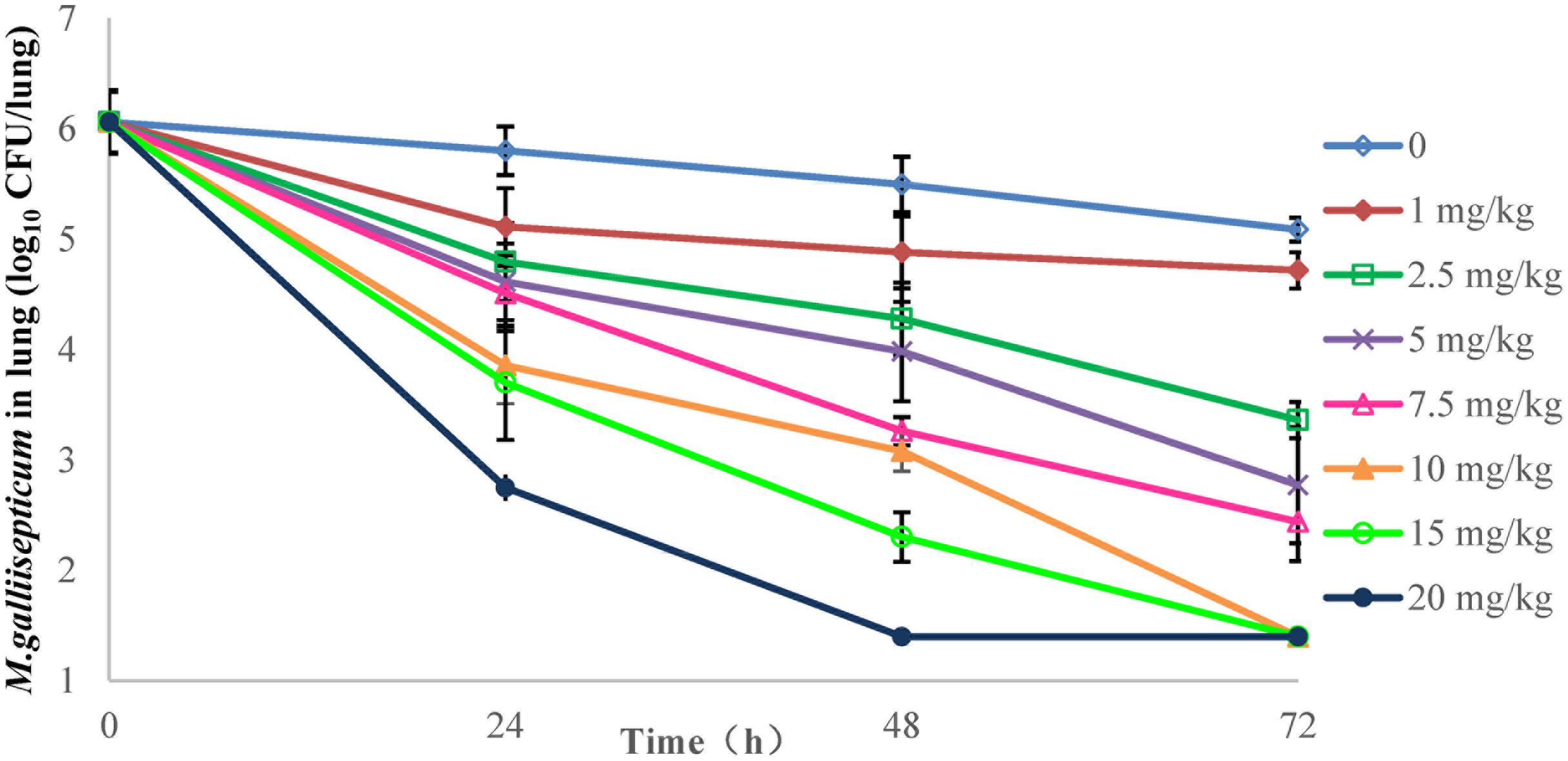
FIGURE 4. Viable counts of M. gallisepticum in lung tissues after three oral administrations of danofloxacin (n = 10/time point).
PK and PD Analysis
The PK/PD parameters (AUC24/MIC) derived from in vivo infection model and anti-mycoplasmal effects are presented in Figure 5. The PK–PD parameters are described in Table 6. AUC24/MIC correlated well with anti-mycoplasmal efficacy (R2 = 0.8901). The dose–response relationships with danofloxacin against M. gallisepticum were evaluated using Sigmoid Emax model. The ratios of AUC24/MIC for 2 log10 CFU/lung reduction and 3 log10 CFU/lung reduction were 31.97 and 97.98 L⋅h/kg, respectively.
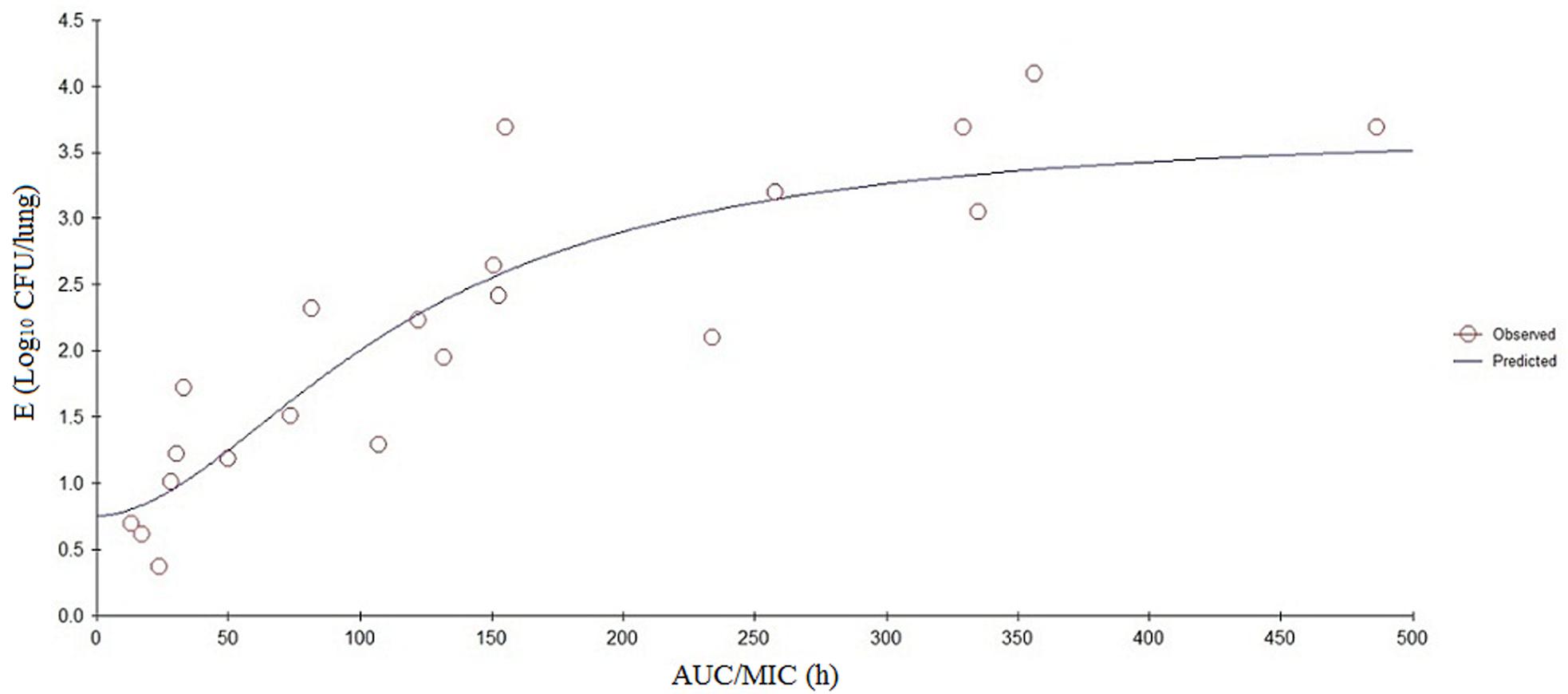
FIGURE 5. Sigmoid Emax relationships between antimycoplasmal effect (E, log10 CFU/lung) and in vivo AUC24/MIC ratio against M. gallisepticum in the lung tissues of chickens.
Dosage Calculation
Since there were not enough danofloxacin MIC data available to provide an estimate of the MIC90, the MIC of danofloxacin against M. gallisepticum strain S6 was substituted as a projected MIC90. The bioavailability had been taken into account, owing to the extravascular route of administration, and the free-drug fraction was not required for using PD data generated in vivo. A dosage of 5.5 mg/kg once daily for 3 days was calculated to be capable of a reduction of 3 log10 CFU/lung.
Characterization of Point Mutations in DNA Gyrase and Topoisomerase IV Involved in Danofloxacin Resistance of Mycoplasma gallisepticum Mutants Recovered from In Vivo
The mutation in gyrA, gyrB, parC, and parE of the mutant strains obtained before and after the passages were the same. The mutant strains with increased MICs (0.6 and 4.8 mg/L) were selected from the two dosage of 1 and 2.5 mg/kg. Six colonies from each mutant strain were screened for each sample before and after the passage, and 20 samples (10 air sacs and 10 lung tissues) were collected from each dosage. Mutations in QRDR target genes are shown in Table 7. When the dose was 1 mg/kg, 16 samples had a (Ser-83→Arg) substitution at the position corresponding to amino acid 83 in gyrA of E. coli; and 4 samples had a (Glu-87→Gly) substitution at the position of 87 in gyrA of E. coli. When the drug was 2.5 mg/kg, 20 samples had a (Ser-83→Arg) substitution at the position corresponding to amino acid 83 in gyrA of E. coli; and 7 samples had an additional (Glu-84→Lys) substitution at the position corresponding to amino acid 84 in parC of E. coli. None of the mutants possessed any base changes in gyrB and parE (Table 7). No point mutations in QRDR targets were found in positive control group (M. gallisepticum strain S6).
Discussion
Mycoplasma gallisepticum not only causes economically significant respiratory disease in chickens and turkeys, but also is a primary pathogen for chukar, red-legged partridges, pheasants and members of the Fringillidae family (Benskin et al., 2009). Quinolones are broad-spectrum antimicrobials and effective in the treatment of M. gallisepticum infections (Gerchman et al., 2008; Zhang et al., 2017). The synthetic quinolone danofloxacin is widely used in China for the treatment of chicken respiratory infections caused by M. gallisepticum. In order to support it as an antimicrobial chemotherapy with an optimum dose response, in vivo model is essential to provide real conditions rather than in vitro model. However, quinolones resistance has emerged in M. gallisepticum due to improper use of those antimicrobial agents (Pakpinyo and Sasipreeyajan, 2007; Lysnyansky et al., 2008, 2012). In the current study, we strived to establish an in vivo infection model and investigate the efficacy of danofloxacin against M. gallisepticum using this model. In addition, resistant mutants were selected and preliminary mechanism of danofloxacin resistance was analyzed.
When the inoculum size of M. gallisepticum was 8.33 log10 CFU/mL, the morbidity, mortality, M. gallisepticum load in air sac and lung were consistent with an earlier report (Xiao et al., 2015b). Additionally, for the reason that the reduction of M. gallisepticum in air sac and lung was used to fit the PK–PD model by WinNonlin, the appreciative load of M. gallisepticum in infection site should be obtained from the in vivo infection model. The results showed that the maximum load of M. gallisepticum were observed (6.17 log10 CFU/air sac and 6.09 log10 CFU/lung) when the inoculum size was 9.31 log10 CFU/mL. Therefore, it is preferred to apply the inoculum size of 0.2-mL 109 CFU/mL twice daily for 3 days in in vivo M. gallisepticum infection model.
In the past decades, there were limited information about the in vivo infection model of antibacterial agents against mycoplasmas. This study is the first one to investigate the efficacy of danofloxacin against M. gallisepticum in chicken infection model. Comparing with the neutropenic chicken infection model (Xiao et al., 2015b), the main innovative points of this paper are as follows: (1) Not only the drug concentrations in plasma were detected, but also the concentrations in lung tissues were examined; (2) It was the first time to quantitate M. gallisepticum colonies in chicken by CFU method that were recorded from inverted microscope directly and the resistant mutants could be selected from the agar plate; (3) The point mutations in QRDR as well as the susceptibility to other quinolones of the selected mutant strains were determined.
In the present investigation, dose proportionality of danofloxacin PK in the range of 1 mg/kg to 20 mg/kg has been described using danofloxacin concentration in plasma and lung tissues in infected chickens. The PK profiles reveal that the average danofloxacin concentration in lung tissues was higher than the corresponding concentration in plasma (1.45–5.14 times higher). According to previously published articles, higher concentrations of danofloxacin in lung tissues than plasma have been reported in other animals as well. The value of peak lung tissues concentration/peak plasma concentration of danofloxacin were 4.5 in sheep (McKellar et al., 1998), 5.0 in pigs (Friis, 1993), and 4.1 in cattle (Giles et al., 1991). The PK of danofloxacin in healthy chickens has been reported by previous studies (Lynch et al., 1994; Knoll et al., 1999; Zeng et al., 2011). Comparing with those studies, there were no significant differences in PK of danofloxacin between the health and infected chickens. For example, Tmax of danofloxacin in health chickens in plasma ranged from 1.5 to 2.33 h; T1/2 ranged from 6.62 to 13.05 h. However, the Tmax and T1/2 of danofloxacin in M. gallisepticum infected chickens were 2.33 and 11.28 h, respectively. The exception was Cmax in lung of the infected chickens (1.14 μg/mL), which were lower than that of healthy chickens (4.33 μg/mL). Swell was observed in lung of the chickens infected with M. gallisepticum. We could speculate that the distribution of danofloxacin in lung may be reduced by these lesions.
Mutations in QRDR of DNA gyrase and topoisomerase IV were the primary quinolone resistance mechanisms for mycoplasma (Bailey et al., 1996; Reinhardt et al., 2002a,b; Gruson et al., 2005; Xie and Zhang, 2006). The substitutions of Ser-83→Arg or Glu-87→Gly in gyrA and Glu-84→Lys in parC have been described previously (Reinhardt et al., 2002a,b). In a previous report investigated the mechanism of quinolones resistance (Reinhardt et al., 2002a), four different substitutions of gyrB in the mutant strains were found. The resistant strains were selected from in vitro by incubating in broth medium containing gradually increasing drug concentrations and serial transfers. However, no gyrB mutation was observed in the resistant mutant strains selected from in vivo infection model. In vitro models cannot provide all in vivo conditions, because not all conditions for antibacterial agents and bacteria including drug formulation, dosing regimen, sick animals and infecting pathogen have been taken into account in in vitro models. Therefore, it is understandable that difference in mutations may be caused by the different infection models.
For quinolones used in both humans and animals, they all exhibit the antibacterial activity by inhibition of bacterial topoisomerase and gyrase, which interfere the replication of DNA (Hopkins et al., 2005). If mutant strains were resistant to one quinolone, resistance to other quinolones were always observed due to the same mechanism of resistance (Hopkins et al., 2005). Our results demonstrate that the danofloxacin-resistant M. gallisepticum strains isolated from the infected chickens also conferred the resistance to enrofloxacin, ofloxacin, levofloxacin, gatifloxacin, and norfloxacin. The point mutations in positions 83 and 87 of gyrA are the most frequent positions that are the active site of DNA gyrase, which are associated with the replication of DNA during the topoisomerase reaction. The binding of quinolones to the site was altered by the mutations at this active site, resulting in the increased MICs and resistance to quinolones (Willmott and Maxwell, 1993). Our results indicated that MICs of quinolones (danofloxacin, enrofloxacin, ofloxacin, levofloxacin, gatifloxacin, and norfloxacin) against the resistant mutant strains that carried the single mutation in position 83 were higher than that with a single mutation in position 87 of gyrA. Similar results were also observed in Escherichia coli and Salmonella (Ozeki et al., 1997; Bagel et al., 1999; Giraud et al., 1999; Liebana et al., 2002).
In the previous studies (Lysnyansky et al., 2008, 2012), molecular characterization of the QRDRs in enrofloxacin-resistant M. gallisepticum strains isolated from various countries was analyzed, revealing that the mutations correlated with the resistant phenotype were the substitutions of Ser-83→Ile or Glu-87→Lys in gyrA; Ser-80→Leu in parC. However, our results showed that the substitution of Ser83→Arg or Glu-87→Gly in gyrA; Glu-84→Lys in parC were related to the danofloxacin-resistant M. gallisepticum strain S6 isolated from the infected chickens. The difference may be caused by the following reasons: (1) Although the drug and the strains of M. gallisepticum were similar, they were not virtually identical; (2) We could speculate that the mutations in clinical isolates were driven by sustained exposure to antimicrobials for many years. These mutant strains survived through a process of natural selection and the mutations could be inherited steadily. However, the mutations in our study were selected under the pressure of a short-term effect of drug, the viability and inheritance of the resistant strains in nature were unknown. Therefore, it is reasonable that the prevalence of QRDR mutations in clinical isolates were different with the strains isolated from laboratory conditions.
Conclusion
Inoculating with a 0.2-mL aliquot solution containing 109 CFU/mL of the M. gallisepticum (twice a day) via intratracheal injection for 3 days was used to establish the ideal in vivo M. gallisepticum infection model. In M. gallisepticum infected chickens, danofloxacin concentration in lung tissues was higher than plasma. Danofloxacin showed greatly effectiveness against M. gallisepticum infection. Mycoplasmacidal activity was found when infected chickens were exposed to danofloxacin at the dose group above 2.5 mg/kg and resistant mutant strains were selected at the dose group of 1 and 2.5 mg/kg. AUC24/MIC correlated well with antimycoplasmal efficacy (R2 = 0.8901). The ratios of AUC24/MIC for 2 log10 (CFU/mL) reduction and 3 log10 (CFU/mL) reduction were 31.97 and 97.98 L⋅h/kg, respectively. Substitutions of Ser-83→Arg or Glu-87→Gly in gyrA, Glu-84→Lys in parC were detected in the danofloxacin-resistant mutant strains. In addition, the susceptibility of danofloxacin, enrofloxacin, ofloxacin, levofloxacin, gatifloxacin, and norfloxacin against those resistant mutants with a single mutation in position 83 were lower than that with a single mutation in position 87. The results showed that danofloxacin at a dose of 5.5 mg/kg resulted in a reduction of 3 log10 CFU/lung.
Author Contributions
Methodology, software, validation, formal analysis, data curation, writing (original draft preparation), writing (review and editing), visualization, and project administration: NZ. Investigation: NZ, YW, ZH, LY, and LZ; resources: NZ, QC, XS, HJ, and HD. Supervision: HD. Funding acquisition: HD.
Conflict of Interest Statement
The authors declare that the research was conducted in the absence of any commercial or financial relationships that could be construed as a potential conflict of interest.
Acknowledgments
This study is supported financially by National Key Research and Development Program of China (2016YFD0501300/2016YFD0501310) and Special Program for Science and Technology Development of Guangdong Province (2015AD20209130).
References
Adler, H. E., Fabricant, J., Yamamoto, R., and Berg, J. (1958). Symposium on chronic respiratory diseases of poultry. I. Isolation and identification of pleuropneumonia-like organisms of avian origin. Am. J. Vet. Res. 19, 440–447.
Bagel, S., Hullen, V., Wiedemann, B., and Heisig, P. (1999). Impact of gyrA and parC mutations on quinolone resistance, doubling time, and supercoiling degree of Escherichia coli. Antimicrob. Agents Chemother. 43, 868–875.
Bailey, C. C., Younkins, R., Huang, W. M., and Bott, K. F. (1996). Characterization of genes encoding topoisomerase IV of Mycoplasma genitalium. Gene 168, 77–80. doi: 10.1016/0378-1119(95)00718-0
Benskin, C. M., Wilson, K., Jones, K., and Hartley, I. R. (2009). Bacterial pathogens in wild birds: a review of the frequency and effects of infection. Biol. Rev. Camb. Philos. Soc. 84, 349–373. doi: 10.1111/j.1469-185X.2008.00076.x
Friis, C. (1993). Penetration of danofloxacin into the respiratory tract tissues and secretions in calves. Am. J. Vet. Res. 54, 1122–1127.
Gerchman, I., Lysnyansky, I., Perk, S., and Levisohn, S. (2008). In vitro susceptibilities to quinolones in current and archived Mycoplasma gallisepticum and Mycoplasma synoviae isolates from meat-type turkeys. Vet. Microbiol. 131, 266–276. doi: 10.1016/j.vetmic.2008.04.006
Giles, C. J., Magonigle, R. A., Grimshaw, W. T., Tanner, A. C., Risk, J. E., Lynch, M. J., et al. (1991). Clinical pharmacokinetics of parenterally administered danofloxacin in cattle. J. Vet. Pharmacol. Ther. 14, 400–410. doi: 10.1111/j.1365-2885.1991.tb00854.x
Giraud, E., Brisabois, A., Martel, J. L., and Chaslus-Dancla, E. (1999). Comparative studies of mutations in animal isolates and experimental in vitro- and in vivo-selected mutants of Salmonella spp. suggest a counterselection of highly quinolone-resistant strains in the field. Antimicrob. Agents Chemother. 43, 2131–2137.
Greenberg-Ofrath, N., Terespolosky, Y., Kahane, I., and Bar, R. (1993). Cyclodextrins as carriers of cholesterol and fatty acids in cultivation of mycoplasmas. Appl. Environ. Microbiol. 59, 547–551.
Gruson, D., Pereyre, S., Renaudin, H., Charron, A., Bebear, C., and Bebear, C. M. (2005). In vitro development of resistance to six and four quinolones in Mycoplasma pneumoniae and Mycoplasma hominis, respectively. Antimicrob. Agents Chemother. 49, 1190–1193. doi: 10.1128/AAC.49.3.1190-1193.2005
Hannan, P. C. (2000). Guidelines and recommendations for antimicrobial minimum inhibitory concentration (MIC) testing against veterinary mycoplasma species. International Research Programme on Comparative Mycoplasmology. Vet. Res. 31, 373–395. doi: 10.1051/vetres:2000100
Hopkins, K. L., Davies, R. H., and Threlfall, E. J. (2005). Mechanisms of quinolone resistance in Escherichia coli and Salmonella: recent developments. Int. J. Antimicrob. Agents 25, 358–373. doi: 10.1016/j.ijantimicag.2005.02.006
Jordan, F. T., Horrocks, B. K., Jones, S. K., Cooper, A. C., and Giles, C. J. (1993). A comparison of the efficacy of danofloxacin and tylosin in the control of Mycoplasma gallisepticum infection in broiler chicks. J. Vet. Pharmacol. Ther. 16, 79–86. doi: 10.1111/j.1365-2885.1993.tb00292.x
Kleven, S. (1990). Summary of discussions of avain mycoplasma team international researh program on comparative mycoplasmology babolna, Hungary. Avian Pathol. 19, 795–800. doi: 10.1080/03079459008418732
Knoll, U., Glunder, G., and Kietzmann, M. (1999). Comparative study of the plasma pharmacokinetics and tissue concentrations of danofloxacin and enrofloxacin in broiler chikens. J. Vet. Pharmacol. Ther. 22, 239–246. doi: 10.1046/j.1365-2885.1999.00217.x
Levisohn, S., and Kleven, S. (2000). Avian mycoplasmosis (Mycoplasma gallisepticum). Rev. Sci. Tech. 19, 425–442. doi: 10.20506/rst.19.2.1232
Ley, D. H., Berkhoff, J. E., and Levisohn, S. (1997). Molecular epidemiologic investigations of Mycoplasma gallisepticum conjunctivitis in songbirds by random amplified polymorphic DNA analyses. Emerg. Infect. Dis 3, 375. doi: 10.3201/eid0303.970318
Liebana, E., Clouting, C., Cassar, C. A., Randall, L. P., Walker, R. A., Threlfall, E. J., et al. (2002). Comparison of gyrA mutations, cyclohexane resistance, and the presence of class I integrons in Salmonella enterica from farm animals in England and Wales. J. Clin. Microbiol. 40, 1481–1486. doi: 10.1128/JCM.40.4.1481-1486.2002
Lynch, M. J., Rice, J. R., Ericson, J. F., Mosher, F. R., Millas, W. J., Harran, L. P., et al. (1994). Residue depletion studies on danofloxacin in the chicken. J. Agric. Food Chem. 42, 289–294. doi: 10.1021/jf00038a012
Lysnyansky, I., Gerchman, I., Levisohn, S., Mikula, I., Feberwee, A., Ferguson, N. M., et al. (2012). Discrepancy between minimal inhibitory concentration to enrofloxacin and mutations present in the quinolone-resistance determining regions of Mycoplasma gallisepticum field strains. Vet. Microbiol. 160, 222–226. doi: 10.1016/j.vetmic.2012.05.002
Lysnyansky, I., Gerchman, I., Perk, S., and Levisohn, S. (2008). Molecular characterization and typing of enrofloxacin-resistant clinical isolates of Mycoplasma gallisepticum. Avian Dis. 52, 685–689. doi: 10.1637/8386-063008-RESNOTE.1
McKellar, Q. A., Gibson, I. F., and McCormack, R. Z. (1998). Pharmacokinetics and tissue disposition of danofloxacin in sheep. Biopharm. Drug Dispos. 19, 123–129. doi: 10.1002/(SICI)1099-081X(199803)19:2<123::AID-BDD89>3.0.CO;2-G
Ozeki, S., Deguchi, T., Yasuda, M., Nakano, M., Kawamura, T., Nishino, Y., et al. (1997). Development of a rapid assay for detecting gyrA mutations in Escherichia coli and determination of incidence of gyrA mutations in clinical strains isolated from patients with complicated urinary tract infections. J. Clin. Microbiol. 35, 2315–2319.
Pakpinyo, S., and Sasipreeyajan, J. (2007). Molecular characterization and determination of antimicrobial resistance of Mycoplasma gallisepticum isolated from chickens. Vet. Microbiol. 125, 59–65. doi: 10.1016/j.vetmic.2007.05.011
Razin, S., and Tully, J. G. (1970). Cholesterol requirement of mycoplasmas. J. Bacteriol. 102, 306–310.
Reinhardt, A. K., Bébéar, C., Kobisch, M., Kempf, I., and Gautier-Bouchardon, A. (2002a). Characterization of mutations in DNA gyrase and topoisomerase IV involved in quinolone resistance of Mycoplasma gallisepticum mutants obtained in vitro. Antimicrob. Agents Chemother. 46, 590–593.
Reinhardt, A. K., Kempf, I., Kobisch, M., and Gautier-Bouchardon, A. V. (2002b). Quinolone resistance in Mycoplasma gallisepticum: DNA gyrase as primary target of enrofloxacin and impact of mutations in topoisomerases on resistance level. J. Antimicrob. Chemother. 50, 589–592.
Toutain, P. L., Bousquet-Melou, A., and Martinez, M. (2007). AUC/MIC: a PK/PD index for antibiotics with a time dimension or simply a dimensionless scoring factor? J. Antimicrob. Chemother. 60, 1185–1188. doi: 10.1093/jac/dkm360
Vinks, A., Derendorf, H., and Mouton, J. (2014). Fundamentals of Antimicrobial Pharmacokinetics and Pharmacodynamics. Berlin: Springer.
Willmott, C. J., and Maxwell, A. (1993). A single point mutation in the DNA gyrase A protein greatly reduces binding of quinolones to the gyrase-DNA complex. Antimicrob. Agents Chemother. 37, 126–127. doi: 10.1128/AAC.37.1.126
Xiao, X., Sun, J., Chen, Y., Zou, M., Zhao, D.-H., and Liu, Y.-H. (2015a). Ex vivo pharmacokinetic and pharmacodynamic analysis of valnemulin against Mycoplasma gallisepticum S6 in Mycoplasma gallisepticum and Escherichia coli co-infected chickens. Vet. J. 204, 54–59. doi: 10.1016/j.tvjl.2015.01.020
Xiao, X., Sun, J., Yang, T., Fang, X., Wu, D., Xiong, Y. Q., et al. (2015b). In Vivo pharmacokinetic/pharmacodynamic profiles of valnemulin in an experimental intratracheal Mycoplasma gallisepticum infection model. Antimicrob. Agents Chemother. 59, 3754–3760. doi: 10.1128/AAC.00200-15
Xie, X., and Zhang, J. (2006). Trends in the rates of resistance of Ureaplasma urealyticum to antibiotics and identification of the mutation site in the quinolone resistance-determining region in Chinese patients. FEMS Microbiol. Lett. 259, 181–186. doi: 10.1111/j.1574-6968.2006.00239.x
Zeng, Z., Deng, G., Shen, X., Zeng, D., and Ding, H. (2011). Plasma and tissue pharmacokinetics of danofloxacin in healthy and in experimentally infected chickens with Pasteurella multocida. J. Vet. Pharmacol. Ther. 34, 101–104. doi: 10.1111/j.1365-2885.2010.01223.x
Zhang, N., Gu, X., Ye, X., Wu, X., Zhang, B., Zhang, L., et al. (2016). The PK/PD interactions of doxycycline against Mycoplasma gallisepticum. Front. Microbiol. 7:653. doi: 10.3389/fmicb.2016.00653
Keywords: Mycoplasma gallisepticum, danofloxacin, in vivo infection model, mutation, killing
Citation: Zhang N, Wu Y, Huang Z, Yao L, Zhang L, Cai Q, Shen X, Jiang H and Ding H (2017) The PK–PD Relationship and Resistance Development of Danofloxacin against Mycoplasma gallisepticum in An In Vivo Infection Model. Front. Microbiol. 8:926. doi: 10.3389/fmicb.2017.00926
Received: 19 January 2017; Accepted: 08 May 2017;
Published: 30 May 2017.
Edited by:
Dongsheng Zhou, Beijing Institute of Microbiology and Epidemiology, ChinaReviewed by:
Liang Li, LA BioMed at Harbor-UCLA Medical Center, United StatesOsmar Nascimento Silva, Universidade Católica Dom Bosco, Brazil
Sarah Victoria Harding, Defence Science and Technology Laboratory, United Kingdom
Copyright © 2017 Zhang, Wu, Huang, Yao, Zhang, Cai, Shen, Jiang and Ding. This is an open-access article distributed under the terms of the Creative Commons Attribution License (CC BY). The use, distribution or reproduction in other forums is permitted, provided the original author(s) or licensor are credited and that the original publication in this journal is cited, in accordance with accepted academic practice. No use, distribution or reproduction is permitted which does not comply with these terms.
*Correspondence: Huanzhong Ding, aHpkaW5nQHNhY3UuZWR1LmNu