- 1Laboratoire d'Ecologie Microbienne, Faculté des Sciences de la Nature et de la Vie, Université de Bejaia, Bejaia, Algeria
- 2Laboratory for Food Safety, Agence Nationale de Sécurité Sanitaire de l'Alimentation, de l'Environnement et du Travail (ANSES), Université Paris-Est, Maisons-Alfort, France
- 3Nancy Laboratory for Hydrology, Agence Nationale de Sécurité Sanitaire de l'Alimentation, de l'Environnement et du Travail (ANSES), Nancy, France
- 4Angers Laboratory for Plant Health, Agence Nationale de Sécurité Sanitaire de l'Alimentation, de l'Environnement et du Travail (ANSES), Angers, France
- 5Ecole Nationale Vétérinaire d'Alfort, Université Paris-Est, Maisons-Alfort, France
The role of food in human exposure to antimicrobial-resistant bacteria is a growing food safety issue. The contribution of fruits and vegetables eaten raw to this exposure is still unclear. The evaluation of contamination levels of fruits, vegetables and the agricultural environment by third-generation cephalosporin (3GC)-resistant Gram-negative bacteria was performed by analyzing 491 samples of fruits and vegetables collected from 5 markets and 7 farms in Bejaia area, north-eastern Mediterranean coast of Algeria. Ninety soil samples and 45 irrigation water samples were also sampled in farms in order to assess them as potential inoculum sources. All samples were investigated at the same time on ceftazidime-containing selective media for 3GC-resistant Gram-negative bacteria detection and on Hektoen media, for Salmonella spp. presence. The bacteria isolated (n = 30) from fruits and vegetables, soil and irrigation water collected in the farms were almost all non-fermenting bacterial species (Stenotrophomonas, Acinetobacter, Pseudomonas, Ochrobactrum) except one strain of Enterobacter cloacae and two strains of Citrobacter murliniae, isolated on one cucumber and two tomato samples in the same farm. Greater diversity in bacterial species and antimicrobial resistance profiles was observed at markets: Enterobacteriaceae (n = 41) were as strongly represented as non-fermenting bacteria (n = 37). Among Enterobacteriaceae, E. cloacae (n = 21), and Klebsiella pneumoniae (n = 13) were the most common isolates. Most of the K. pneumoniae isolates were extended-spectrum beta-lactamase (ESBL) producers (n = 11). No Salmonella spp. was recovered in any sample. This study showed that fruits and vegetables including those which may be eaten up raw constitute a reservoir of 3GC-resistant Gram-negative bacteria and multi-drug resistant-bacteria in general that can be transferred to humans through food. The general public should be informed of this hazard for health in order to encourage good domestic hygiene practices. In addition, further investigation is needed throughout the production chain to enrol professionals in actions to reduce this contamination.
Introduction
Fresh fruits and vegetables are essential components of a healthy human diet. They provide essential nutrients, such as vitamins, fibers, minerals, and have many health benefits. Therefore, a large number of public health institutions encourage the consumption of fruits and vegetables, and recommend eating at least five fruits and vegetables daily to protect against a range of cardiovascular diseases and cancers (Abadias et al., 2008; Callejón et al., 2015).
Currently, there is a growing demand for these fresh fruits and vegetables for health benefits and at the same time, as lifestyles are changing, current trends show a decrease in the time spent preparing meals (Abadias et al., 2008).
In fact, fresh fruits and vegetables have recently become increasingly recognized as potential vehicles of foodborne diseases (Lynch et al., 2009; Olaimat and Holley, 2012). Many food-borne illness outbreaks in numerous countries have been associated with consumption of contaminated fresh fruits and vegetables, such as fenugreek seed sprouts contaminated with Escherichia coli O104:H4 in Europe in 2011, and tomatoes and spinach contaminated with Salmonella and E. coli O157 in the United States of America in 2013 (AIT, 2013).
The use of antibiotics to treat humans and animals or in agriculture can lead to the selection of antibiotic-resistant bacteria that escape in the environment (Durso and Cook, 2014). Environmental isolates of Enterobacteriaceae that have acquired resistance to third-generation cephalosporins (3GCs) constitute a crucial threat for public health as a source of resistance traits for pathogenic bacterial strains that could lead to a failure in antibiotherapy (Blaak et al., 2014).
The role of food in human exposure to antimicrobial-resistant bacteria, including zoonotic pathogens, as well as commensal and environmental bacteria serving as a reservoir for resistance genes, is becoming a growing food safety issue (Campos et al., 2013; Zurfluh et al., 2015). This contamination may thus occur by different means, such as exposure of products to manure, soil, irrigation water, or animal feces harboring these microorganisms. This contamination can also occur during harvesting, post-harvest handling, or distribution due to lack of compliance with elementary food safety and hygiene measures (Abadias et al., 2008; Seo and Matthews, 2014).
Enterobacteriaceae are part of the environmental microflora and include common animals' commensals. Enterobacteriaceae strains ingested through food may contain Extended Spectrum Beta Lactamases (ESBL) and plasmidic AmpC (pAmpC) genes found on mobile genetic elements. These isolates can then colonize humans or their genes can be transferred to other bacteria during transit in the intestinal tract (Thanner et al., 2016).
There is limited information concerning the nature and ecology of antibiotic-resistant bacteria associated with fresh fruits and vegetables (Ruimy et al., 2010; Blaak et al., 2014; Reuland et al., 2014; Veldman et al., 2014). In addition, no study on that topic has been performed in Algeria to date. The aim of our study was therefore firstly to evaluate the level of contamination of fruits, vegetables and the agricultural environment by 3GC-resistant Gram-negative bacteria; secondly, to search for the source of this contamination; and thirdly, to determine the resistance phenotype of these isolates. To establish whether ESBL-producing Enterobacteriaceae on fresh fruits and vegetables reflect those present in the farm environment, fruits and vegetables were collected in farms and at the market as well.
Materials and Methods
Sampling
A total number of 491 fruit and vegetable samples were collected from seven selected farms and five markets in Bejaia area, Algeria, during a time-period from April 2013 to March 2014.
Farms in the vicinity of Bejaia were contacted in order to ask them access for research purposes. Four large farms and three small family farms allowed full access to their agricultural exploitation. During each visit, samples were taken on any production ready for harvesting. Large farms were each sampled four times; the family farms were sampled only once. A total of 181 samples collected from the farms were analyzed, including 126 tomatoes, 6 zucchini, 12 cucumbers, 21 chilies, and peppers, 1 lettuce, 1 celery, 2 parsleys, 2 mints, 2 garlics, 3 chards, 3 onions, and 2 walnuts. A set of 165 out of these samples originated from large commercial farms while the 16 remaining samples were collected from small family farms (Supplementary Table 1). In addition, 135 samples of soil and irrigation water were sampled in all places (Supplementary Table 1). All farms use poultry droppings, manure, and fertilizer for soil fertilization (Supplementary Table 1).
In parallel, 310 samples were collected in five selected markets located inside the city of Bejaia. During each of the visits to the markets, the sampler behaved like an average Algerian consumer and shopped the most common fruits and vegetables from the regular Algerian regimen. The plant samples included 41 lettuces, 51 tomatoes, 4 cucumbers, 6 celeries, 10 mints, 9 beets, 14 apples, 26 chilies, 28 peppers, 30 grapes, 2 dates, 3 prickly-pears, 11 parsleys, 15 peaches, 24 watermelons, 7 pears, 16 carrots, 7 fennels, 3 turnips, and 3 nectarines (Supplementary Table 2).
For fruit and vegetable samples, we have recorded sample name, date (season), country of origin, number of samples, irrigation water type and the type of fertilization, depending on the availability (Supplementary Tables 1, 2). Information on irrigation water and soil at different farms were also recorded (Supplementary Table 1).
All samples were collected aseptically and then packaged in sterile polyethylene zip bags and transported to the laboratory in aseptic conditions in a cold box within 2 h. All samples were analyzed within 2 h after their arrival at the laboratory. A sharp sterile knife was used to cut samples in sterile trays.
Microbiological Analysis
Detection of Salmonella
Water and soil samples
Isolation of Salmonella from irrigation water was carried out by membrane filtration. About 3 liters of irrigation water were filtered through a 0.45 μm filtration membrane. The filter membranes were then placed in 50 mL of buffered peptone water (BPW) (Fluka), and incubated at 37°C for 18 ± 2 h (NF EN; ISO 19250, 2010) for enrichment.
Soil samples (25 g each) were suspended in 225 mL of BPW (Fluka), vigorously shaken and the suspensions were then incubated at 37°C for 18 ± 2 h.
Fruit and vegetable samples
Salmonella detection was performed following the ISO standard NF EN ISO 6579, 2002. In brief, 25 g of samples of fruit or vegetable were placed aseptically in a sterile plastic bag containing 225 mL of BPW, vigorously shaken and the suspensions were then incubated at 37°C for 18 ± 2 h.
After pre-enrichment, 0.1 mL of each of these incubated samples was then inoculated in 10 mL of Rappaport-Vassiliadis (RV) broth (Fluka), while in parallel 1 mL of the pre-enriched sample was inoculated in 10 mL Muller-Kauffmann (MK) broth (Fluka). Afterwards, these two different broths were incubated at 41.5 ± 1°C and 37°C, respectively for 24 ± 3 h. After incubation, 10 μL of culture from each enrichment broth were streaked on Hektoen enteric agar (HE) plates (Fluka).
Presumptive Salmonella isolates were screened biochemically on triple sugar iron (TSI) and identified with an API 20E system (Bio-Merieux, Marcy L'Etoile, France).
Isolation and Identification of 3GC-Resistant Gram-Negative Bacteria
In parallel to Salmonella detection, 10 μL of the overnight culture in BPW (see above) was streaked onto MacConkey's agar (MAC) plates (Fluka) supplemented with 8 mg/L of ceftazidime and incubated for 18–24 h at 37°C for isolation of 3GC-resistant Gram-negative bacteria. Vancomycin was added to the medium (8 mg/L) to ensure inhibition of the growth of Gram-positive bacteria. One colony per each morphology and color observed on the agar plate after incubation was preserved. Each preserved isolate, Enterobacteriaceae or not, was identified by MALDI-TOF-MS (matrix-assisted laser desorption/ionization time-of-flight mass spectrometry) using a Microflex LT® and Biotype 3.0 software (Bruker Daltonik, GmbH, Germany). Analyses were performed on bacterial cells grown for 21 ± 3 h on plate count agar (PCA) at 36 ± 2°C. Direct spotting of bacteria cells and full protein extraction using absolute ethanol, formic acid and acetonitrile were performed following the manufacturer's recommendations with the aim of obtaining a correct identification score. After drying each spot at room temperature, 1 μL matrix1 HCCA (α-cyano-4-hydroxycinnamic acid in 50% acetonitrile/2.5% trifluoroacetic acid) was added before analysis. A bacterial test standard (BTS, Bruker Daltonik, Germany) was also included bacterial sample lots to assess the efficiency of the process. The identification criteria used were those recommended by the manufacturer. Log scores ≥ 2 were considered reliable for species identifications, log scores ≥ 1.7 and <2.0 were defined as reliable for genus identification, and log scores <1.7 as non-reliable identification.
Antimicrobial Susceptibility Testing
Antibiotic susceptibility was tested by the disk diffusion method according to the CLSI protocol (Clinical and Laboratory standards Institute, 2012) on Mueller-Hinton agar (Bio-Rad, Marnes-la-Coquette France). E. coli ATCC 25922 was used as a control strain and antimicrobials tested on all isolates were (abbreviations and amounts in parentheses): amoxicillin/clavulanic acid (AMC; 30 μg), ampicillin (AMP; 10 μg), cephalothin (CEF; 30 μg), cefuroxime (CXM; 30 μg), cefamandole (FAM; 30 μg), ceftriaxone (CRO; 30 μg), cefotaxime (CTX; 30 μg), ceftazidime (CAZ; 30 μg), ticarcillin (TIC; 75 μg), cefoxitin (FOX; 30 μg), aztreonam (ATM; 30 μg), cefepime (FEP; 30 μg), temocillin (TMC; 30 μg), ertapenem (ETP; 10 μg), imipenem (IMP; 10 μg), chloramphenicol (CHL, 30 μg), trimethoprim (TMP; 5 μg), sulfonamides (SSS; 300 μg), trimethoprim-sulfamethoxazole (SXT; 1.25+23.75 μg), streptomycin (STR, 10 U), gentamicin (GEN; 10 μg), kanamycin (KAN; 30 UI), tetracycline (TET; 30 UI), tigecycline (TGC; 15 μg), nalidixic acid (NAL; 30 μg), ciprofloxacin (CIP; 5 μg), pefloxacin (PEF, 5 μg), cefotaxime+clavulanic acid (CTC; 30+10 μg), ceftazidime+clavulanic acid (CZC; 30+10 μg; Bio-Rad). Colistin disk (CST; 10 μg) was used on each plate on quality management purposes to ensure the absence of contamination and assess the bacterial identification (Bio-Rad).
Isolates were classified as susceptible, intermediate or resistant according to the clinical interpretative criteria recommended by the CLSI (CLSI, 2013). Multi-drug resistance (MDR) was considered when the isolates were resistant to three or more antibiotic classes (Magiorakos et al., 2012). The detection of ESBL phenotype was performed by either the double disk synergy test (EUCAST, 2013) or combinaison disk test with cefotaxime and ceftazidime (CLSI, 2013; EUCAST, 2013). AmpC phenotype, due to production of an acquired cephalosporinase, was considered present in isolates resistant to cefoxitine and cefotaxime or ceftazidime (EUCAST, 2013). Finally, to be able to detect an ESBL phenotype in presence of a cephalosporinase, combinaison disk test with cefepime were performed and inhibition diameters were compared. For combinaison disk tests, a 5 mm difference at least was considered as positive for ESBL presence (EUCAST, 2013).
Detection of Carbapenemases
To rapidly identify carbapenemase producers in Enterobacteriaceae, the Carba NP test and a CIM test (carbapenem inactivation method) were performed according to the methods described by Nordmann et al. (2012) and Van der Zwaluw et al. (2015), respectively.
Statistical Methods
Factors associated with contamination of fruits and vegetables by 3GC-resistant Gram negative bacteria were analyzed using the Chi2 test, according to the place of isolation (farms vs. markets), consumption modes (raw vs. cooked vs. raw/cooked), distance to the soil (above vs. on vs. in the soil) and the season (autumn vs. winter vs. spring vs. summer). Statistical analyses were performed with MS Excel. The Bonferroni correction has also been applied on the set of data: this correction is based on rejecting the null hypothesis if the likelihood of the observed data under the null hypotheses is low. If multiple comparisons are done or multiple hypotheses are tested, the chance of a rare event increases, and therefore, the likelihood of incorrectly rejecting a null hypothesis increases. The Bonferroni correction compensates for that increase by testing each individual hypothesis at a significance level of α/m. where α is the desired overall alpha level and m is the number of hypotheses.
Results
Microbiological Evaluation of Fruits, Vegetables, Soil, and Water Samples
In total, 108 different 3GC-resistant Gram-negative bacteria were isolated from all samples (97 isolates from fruits and vegetables and 11 from soil and water); while no Salmonella was recovered from any of the samples. A large number of fruits and vegetables, 84 out of 491 (17%; Table 1), were found positive for the occurrence of Gram-negative bacteria resistant to 3GC and other antibiotic classes. In total, 97 different 3GC-resistant Gram-negative bacteria were isolated from 84 positive samples. The contamination frequency of each category of fruits and vegetables varied greatly among selected farms and markets.
In the farms, nearly 10% (18/181) of sampled fruits and vegetables were contaminated with Gram-negative bacteria resistant to 3GC; among them, only 4% (7/165) of the samples collected on the four large commercial farms were found to be positive, while 69% (11/16) of the samples collected from the three small familial farms were contaminated. At the markets, an overall higher and significantly different contamination frequency was found, with 21% (66/310) of the samples found to be positive (Table 1).
The soil and irrigation water contamination frequency (agricultural environment) on farms was also 5% (5/90) and 9% (4/45) respectively.
Fruits and vegetables were found contaminated by a variety of bacteria (Tables 2, 3).
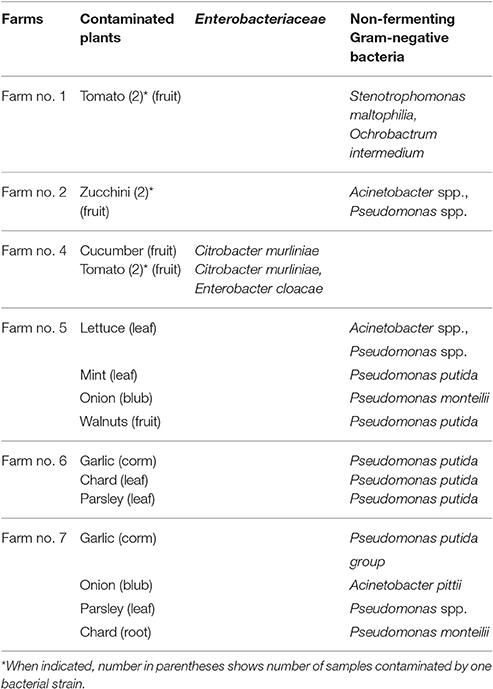
Table 2. Description of samples positive for 3GC-resistant Gram-negative bacteria in the Bejaia farms.
The 108 different bacterial isolates from this study were identified by MALDI-TOF-MS. MALDI-TOF allowed the identification of species for 95 strains, and identification of the genus for 13 other strains with an orientation toward the most likely species.
In the farms, a total number of 19 different bacterial strains were isolated from the 18 positive samples of fruits and vegetables: 16 of them were identified as non-fermenting bacteria, whereas only 3 (3/19) were identified as Enterobacteriaceae (Table 2). By contrast, a total number of 78 different bacterial strains were isolated from the 66 positive samples of fruits and vegetables collected at the markets: we found as many samples contaminated with non-fermenting bacteria (37/78) as with Enterobacteriaceae (41/78; Table 3).
Only eleven 3GC-resistant bacteria were isolated from samples of soil and water (Table 4). All of them are non-fermenting bacteria.
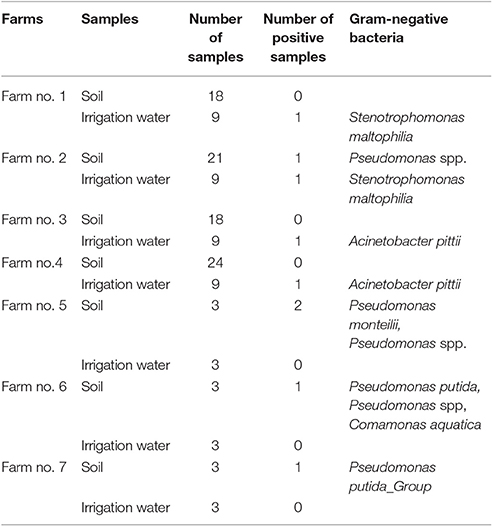
Table 4. Soil and irrigation water samples contaminated with 3GC- resistant Gram-negative bacteria in Bejaia farms.
In summary, a total of 44 Enterobacteriaceae isolates resistant to expanded-spectrum cephalosporins (3GC) were isolated from 491 fruit and vegetable samples (Tables 2, 3), including 21 E. cloacae, 13 K. pneumoniae, 3 Citrobacter freundii, 3 C. murliniae, 1 E. asburiae, 1 E. aerogenes, and 2 Kluyvera ascorbata. Among these isolates, only three were collected in the farms: 2 C. murliniae isolates (tomato and cucumber) and 1 E. cloacae isolate (tomato). All of them were recovered on the same farm (farm no. 4; Table 2). At markets, places of origin of fruits and vegetables contaminated by 3GC-resistant Enterobacteriaceae were multiple (Table 3).
Frequency of contamination and species distribution did not differ according to the type of fruits or vegetables. This contamination frequency was different regarding the way the vegetable is to be eaten, i.e., raw or cooked (Table 5). In this case, the contamination of fruits and vegetables usually eaten raw or cooked was significantly different: contamination of vegetables usually eaten cooked was 39%, nevertheless, the contamination of fruits and vegetables usually eaten raw is not negligible, 17%. The fact that fruits or vegetables are in close contact with the soil or not (Table 6) appears to be a determining factor, with fruits and vegetables grown on or in the soil being more contaminated. When considering the season (Table 7), fruits, and vegetables grown in autumn and winter were more contaminated.
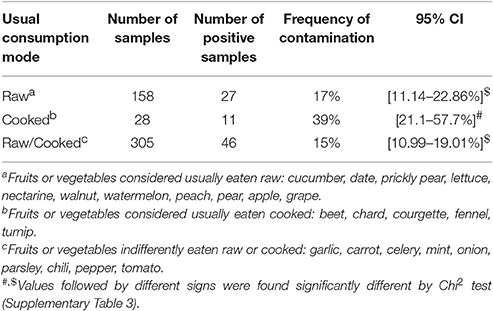
Table 5. Frequency of fruits and vegetables contaminated with 3GC-resistant Gram-negative bacteria according to usual consumption mode.
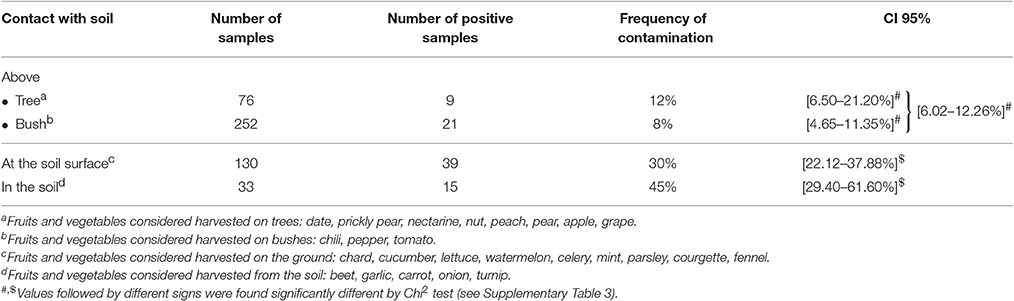
Table 6. Contamination frequency of fruits and vegetables by 3GC-resistant Gram-negative bacteria according to their distance from the soil.
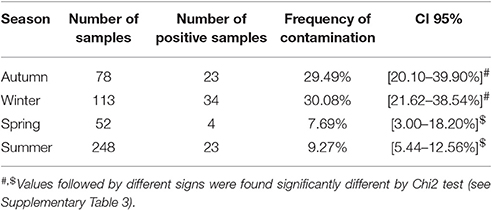
Table 7. Contamination frequency of fruits and vegetables by 3GC-resistant Gram-negative bacteria depending on the season.
Antimicrobial Susceptibility
A total of 97 suspected 3GC-resistant Gram-negative bacteria isolate from 84 positive fruit and vegetable samples and the 11 non-fermenting bacteria isolates from soil and irrigation water were analyzed using disc diffusion. All isolates were confirmed to be resistant to cefotaxime and ceftazidime.
Resistance profiles were diverse: non-fermenting bacterial strains were resistant to 3rd generation cephalosporins, but with roughly a wild-type profile, while Enterobacteriaceae exhibited profiles pointing to acquired resistance to 3rd generation cephalosporins and other antimicrobial classes.
Regarding other beta-lactams, all Enterobacteriaceae isolates were resistant to different generations of cephalosporins, including 1st generation cephalosporins (CEF), 2nd Generation cephalosporins [CXM, FAM, except FOX (73%)], and 3rd generation cephalosporins (CTX, CAZ, CRO); 27% of them were resistant to 4th generation cephalosporins (FEP), all of them were resistant to ampicillin and ticarcillin (AMP, TIC), 95% were resistant to AMC, and 78% to monobactam (ATM; Table 8).
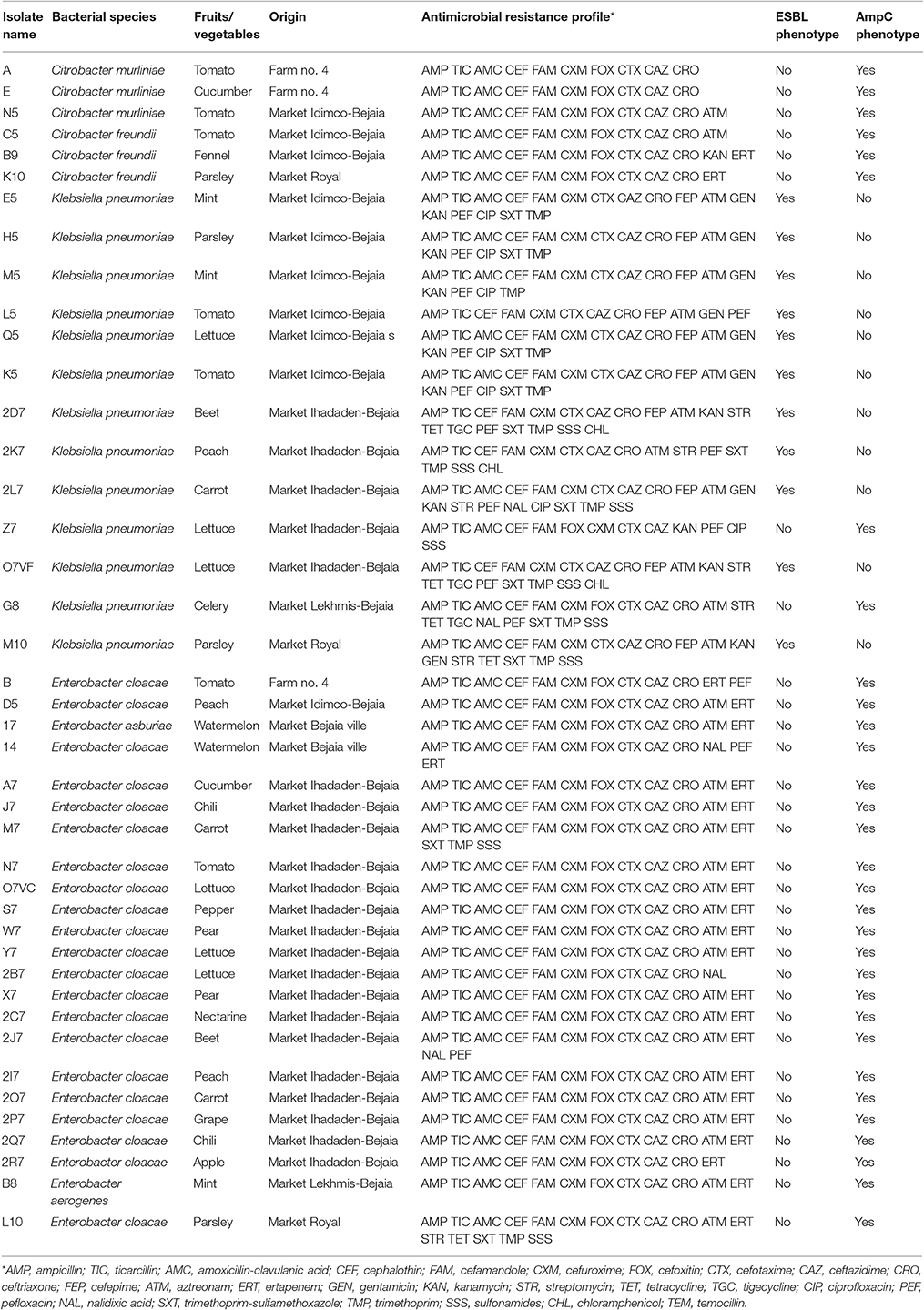
Table 8. General characteristics of 3GC-resistant Enterobacteriaceae obtained from fruits and vegetables in Bejaia, north-eastern Algeria.
All tested Enterobacteriaceae isolates were susceptible to imipenem by disc diffusion. However, E. cloacae, E. asburiae, and E. aerogenes showed decreased susceptibility to ertapenem (≤23 mm). Nonetheless, these isolates tested negative for carbapenemase production by both Carba NP test and CIM test.
Resistance to non-beta-lactams antibiotics was also encountered: resistance was observed to sulphonamides (29% SXT, 31% TMP, and 20% SSS), aminoglycosides (20% GEN, 27% KAN, and 16% STR), tetracyclines (11% TET, 7% TGC), fluoroquinolones (18% CIP, 36% PEF, and 11% NAL) and phenicols (7% CHL; Table 8).
The proportion of isolates belonging to the different species identified in this study and that are resistant to studied antimicrobial agents are shown in Table 8. ESBL producing bacteria were K. pneumoniae strains (n = 11), while AmpC producers belonged to various bacterial species (2 K. pneumoniae, 3 C. murliniae and 3 C. freundii).
Discussion
Our results document the presence of ESBL-producing and AmpC harboring Enterobacteriaceae in retail raw fruits and vegetables that were isolated in Algeria, which implies that vegetables may be a source of resistance genes for human microflora. These results are in accordance with other studies identifying vegetables as a possible route for the dissemination of resistance genes in the community (Mesa et al., 2006; Reuland et al., 2014; Ben Said et al., 2015; Zurfluh et al., 2015). Bacteria that were found in this study are not specifically related to fruits and vegetables; they are also frequently isolated from animals or humans. Indeed, Enterobacteriaceae such as E. cloacae, K. pneumonia, and Citrobacter are ubiquitous bacteria that are frequently recovered in the intestines of animals and humans. It is likely that the plants were contaminated indirectly by fecal bacteria from animals during the fertilization process or through direct contact with humans during harvesting, handling and packaging of products due to insufficient hygiene measures (Lynch et al., 2009).
It is interesting to note that no contamination by 3rd generation cephalosporin-resistant Enterobacteriaceae was detected in the agricultural environment (water and soil), unlike Ben Said's study (Ben Said et al., 2015), they detected ESBL-producing Enterobacteriaceae in the soil, water, as well as on fruits and vegetables. This may be due to the fact that the farms where these bacteria were isolated from use treated wastewater, unlike the farms in our study that use drinking water from wells.
Pignato and al. have reported the potential transfer of antibiotic-resistant bacteria through treated-wastewater (TWW) use in agriculture (Pignato et al., 2009). By contrast, Negreanu et al. did not found so clear relationship and concluded that the impact of TWW-associated bacteria on the soil microbiome is on the whole negligible (Negreanu et al., 2012). In addition, Bartz et al. found that concentration of coliforms in fruits and vegetables matched the contamination of workers' hands rather than water contamination, suggesting that irrigation water is the less relevant sample for detecting the source of contamination of fruits and vegetables (Bartz et al., 2017).
The comparison between the results obtained on fruit and vegetable samples from farms with those from markets might confirm the hypothesis of contamination of fruits and vegetables by humans. In fact, contamination of different fruits and vegetables on different successive vendors' stalls by the same bacterial species was documented. For instance, numerous samples at Ihadaden market were harboring E. cloacae (Supplementary Table 4) with the same resistance profile (Table 8).
The comparison between strains isolated from irrigation water and soil with those found on fruits and vegetables leads to conclude that in our study contamination of fruits and vegetables by Enterobacteriaceae does not seem to be mainly linked to the agricultural environment. Schwaiger et al. (2011) did not reach the same results and conclusions; they found that contamination of fruits and vegetables is higher at the farm level. They explain this result by the fact that resistance is at the expense of bacterial viability, since vegetables purchased directly at the farm are probably fresher than at the supermarket, and they have not been exposed to stress factors (Schwaiger et al., 2011). This might also be due to different hygiene procedures applied between harvest and sale that are not documented. In addition, we can probably also explain these divergent results by different procedures concerning hygiene either during cultivation or from harvest to the consumer's plate between Algeria and Germany.
The contamination frequency of fruits and vegetables depends on several parameters: type of fruit and/or vegetable, contact with the soil, and season. Here, fruits and vegetables are contaminated with different bacterial species. Fruits and vegetables cultivated and harvested on the surface or in the soil are more commonly contaminated (30 and 45%, respectively), probably due to contact with soil, manure, irrigation water, waste, and animal excrement. This is consistent with findings reported by Ruimy et al. (2010). The variation in fruit and vegetable contamination depending on the season was clearly established. Surprisingly, despite climatic favorable factors in summer and spring contamination was found much higher in winter and autumn (30 and 29%, respectively), than in summer and spring (9 and 8%): the low frequency of contamination of fruits and vegetables in spring could be explained by the fact that fruits and vegetables were harvested in Bejaia and were therefore probably handled to a lesser extent by fewer operators.
This study focused on the detection of 3GC-resistant Gram-negative bacteria from fruits and vegetables, and in addition to 3GC resistance, the isolates showed resistance to between 4 and 10 antimicrobial families used in humans. The detection of human and animal fecal bacterial species with resistance profiles similar to those encountered among hospital isolates is of concern. The abundance of K. pneumoniae, E. cloacae, and Citrobacter resistant to all β-lactams and other families suggests that fruits and vegetables may constitute a real threat to public health because of the transmissible character of this resistance (Thanner et al., 2016). Even though Ruimy et al. (2010) did not reach the same conclusion, others (Blaak et al., 2014; Reuland et al., 2014; Veldman et al., 2014; Van Hoek et al., 2015) tend to find, like here, various multidrug resistant bacteria in fruits and vegetables. Stenotrophomonas, Acinetobacter, Pseudomonas, Ochrobactrum, and Kluyvera were frequently encountered. Nevertheless, they are considered to be mostly opportunistic pathogens, associated with patients in poor health conditions, who are frequently immunocompromised.
Many bacteria survive the ingestion process and may contribute to the spread of antimicrobial resistance genes through intestinal tract flora (Schwaiger et al., 2011). The ingestion of bacteria resistant to 3rd generation cephalosporins and their hosting in the intestines can cause the spread of ESBLs and AmpC genes to commensal intestinal flora. Exchanges of these genes between commensal and pathogenic bacteria in the intestinal tract can cause infections that are difficult to treat.
Several studies have shown that consumption of fruits and vegetables can constitute a serious risk for health (Viswanathan and Kaur, 2001; Campos et al., 2013; Warning and Datta, 2013; Blaak et al., 2014; Veldman et al., 2014; Ben Said et al., 2015; Zurfluh et al., 2015). Washing vegetables before eating them raw might reduce not only the risk of infection by pathogenic bacteria, but also the risk of ingesting, hosting and spreading antibiotic-resistant bacteria (Schwaiger et al., 2011).
Conclusion
In conclusion, poorly washed or insufficiently cooked fruits and vegetables may constitute a public health hazard as they are associated with Gram-negative bacteria resistant to various antibiotics used to treat critical human infections. Fruits and vegetables grown on and in the soil are the most highly contaminated. Contamination frequency of samples from farms is significantly lower than that of samples from markets. This enhanced contamination at the market might reflect poor practices during harvesting and handling of fruits and vegetables.
These results should be communicated to the general public and to fruit and vegetable professionals. First, in order to appreciate the need for good domestic hygiene practices, the consumer has to be aware of the health hazards related to consuming fruits and vegetables that are not properly washed. Second, in order to enroll professionals in actions, close observation and evidence of at-risk practices from harvest to retail are needed.
Author Contributions
FM, SG, AT, and YM: designed the study. FM: Proceeded to sample collection, preparation and bacterial isolation. FM, SG, MM, LY, and BG: Performed laboratory experiments. FM, SG, CM, AT, and YM: Participated to the analyses and discussion of the results.
Funding
This work was financially supported by “Soutien de Programme—Direction Générale de l'Enseignement et de la Recherche” from the French Ministry for Agriculture and by “Programme National Exceptionnel (P.N.E) scholarship” from the Algerian Ministry of Higher Education and Scientific Research.
Conflict of Interest Statement
The authors declare that the research was conducted in the absence of any commercial or financial relationships that could be construed as a potential conflict of interest.
Acknowledgments
Authors are especially grateful to Loic Desquilbet for his assistance in the statistical analysis of the datasets. Authors also would like to acknowledge the master trainees: Kandi Malika, Hammiche Bania, Bendaoud Fazia, Metrouh Amal, and Boukhedami Safia for their kind help in sampling collection.
Supplementary Material
The Supplementary Material for this article can be found online at: http://journal.frontiersin.org/article/10.3389/fmicb.2017.01569/full#supplementary-material
References
Abadias, M., Usall, J., Anguera, M., Solsana, C., and Vinas, I. (2008). Microbiological quality of fresh, minimally-processed fruit and vegetables, and sprouts from retail establishments. Int. J. Food Microbiol. 123, 121–129. doi: 10.1016/j.ijfoodmicro.2007.12.013
Austrian Institute of Technology GmbH (AIT) (2013). Food of Plant Origin: Production Methods and Microbiological Hazards Linked to Food-Borne Disease. Reference: CFT/EFSA/BIOHAZ/2012/01 Lot 1 (Food of Plant Origin with High Water Content Such as Fruits, Vegetables, Juices and Herbs). Austrian Institute of Technology GmbH (AIT).
Bartz, F. E., Lickness, J. S., Heredia, N., Fabiszewski de Aceituno, A., Newman, K. L., Hodgea, D. W., et al. (2017). Contamination of Fresh produce by microbial indicators on farms and in packing facilities: elucidation of environmental routes. Appl. Environ. Microbiol. 83, e02984–e02916. doi: 10.1128/AEM.02984-16
Ben Said, L., Jouini, A., Klibi, N., Dziri, R., Alonso, C. A., Boudabous, A., et al. (2015). Detection of extended-spectrum beta-lactamase (ESBL)-producing Enterobacteriaceae in vegetables, soil and water of the farm environment in Tunisia. Int. J. Food Microbiol. 203, 86–92. doi: 10.1016/j.ijfoodmicro.2015.02.023
Blaak, H., van Hoek, A. H., Veenman, C., van Leeuwen, A. E., Lynch, G., van Overbeek, W. M., et al. (2014). Extended spectrum ß-lactamase- and constitutively AmpC-producing Enterobacteriaceae on fresh produce and in the agricultural environment. Int. J. Food Microbiol. 168–169, 8–16. doi: 10.1016/j.ijfoodmicro.2013.10.006
Callejón, R. M., Rodríguez-Naranjo, M., Ubeda, C., Hornedo-Ortega, R., Garcia-Parrilla, M. C., and Troncoso, A. M. (2015). Reported foodborne outbreaks due to produce in the United States and European Union: trends and causes. Foodborne Pathog. Dis. 12, 32–38. doi: 10.1089/fpd.2014.1821
Campos, J., Mourao, J., Pestana, N., Peixe, L., Novais, C., and Antunes, P. (2013). Microbiological quality of ready-to-eat salads: an underestimated vehicle of bacteria and clinically relevant antibiotic resistance genes. Int. J. Food Microbiol. 166, 464–470. doi: 10.1016/j.ijfoodmicro.2013.08.005
CLSI (2012). Performance Standards for Antimicrobial Susceptibility Testing; 11th Edn., CLSI Supplement M02. Wayne, PA: Clinical and Laboratory Standards Institute.
CLSI (2013). Performance Standards for Antimicrobial Susceptibility Testing; 23th Edn., CLSI Supplement M100S. Wayne, PA: Clinical and Laboratory Standards Institute
Durso, L. M., and Cook, K. L. (2014). Impacts of antibiotic use in agriculture: what are the benefits and risks? Curr. Opin. Microbiol. 19, 37–44. doi: 10.1016/j.mib.2014.05.019
EUCAST (2013). EUCAST Guidelines for Detection of Resistance Mechanisms and Specific Resistances of Clinical and/or Epidemiological Importance. Version 1.0. Available online at: http://www.eucast.org/fileadmin/src/media/PDFs/EUCAST_files/Resistance_mechanisms/EUCAST_detection_of_resistance_mechanisms_v1.0_20131211.pdf
ISO 6579 (2002). Microbiology of Food and Animal Feeding Stuffs – Horizontal Method for the Detection of Salmonella spp. ISO.
Lynch, M. F., Tauxe, R. V., and Hedberg, C. W. (2009). The growing burden of food-borne outbreaks due to contaminated fresh produce: risks and opportunities. Epidemiol. Infect. 137, 307–315. doi: 10.1017/S0950268808001969
Magiorakos, A. P., Srinivasan, A., Carey, R. B., Carmeli, Y., Falagas, M. E., Giske, C. G., et al. (2012). Multidrug-resistant, extensively drug-resistant and pandrug-resistant bacteria: an international expert proposal for interim standard definitions for acquired resistance. Clin. Microbiol. Infect. 18, 268–281. doi: 10.1111/j.1469-0691.2011.03570.x
Mesa, R. J., Blanc, V., Blanch, A. R., Cortés, P., González, J. J., Lavilla, S., et al. (2006). Extended-spectrum beta-lactamase-producing Enterobacteriaceae in different environments (humans, food, animal farms and sewage). J. Antimicrob. Chemother 58, 211–215. doi: 10.1093/jac/dkl211
Negreanu, Y., Pasternak, Z., Jurkevitch, E., and Cytryn, E. (2012). Impact of treated wastewater irrigation on antibiotic resistance in agricultural soils. Environ. Sci. Technol. 46, 4800–4808. doi: 10.1021/es204665b
Nordmann, P., Poirel, L., and Dortet, L. (2012). Rapid detection of carbapenemase-producing Enterobacteriaceae. Emerg. Infect. Dis. 18, 1503–1507. doi: 10.3201/eid1809.120355
Olaimat, A. O., and Holley, R. A. (2012). Factors influencing the microbial safety of fresh produce: a review. Food Microbiol. 32, 1–19. doi: 10.1016/j.fm.2012.04.016
Pignato, S., Coniglio, M. A., Faro, G., Weill, F. X., and Giammanco, G. (2009). Plasmid-mediated multiple antibiotic resistance of Escherichia coli in crude and treated wastewater used in agriculture. J. Water Health 7, 251–258. doi: 10.2166/wh.2009.019
Reuland, E. A., Al Naiemi, N., Raadsen, S. A., Savelkoul, P. H. M., Kluytmans, J. A., and Vandenbroucke-Grauls, C. M. (2014). Prevalence of ESBL-producing Enterobacteriaceae in raw vegetables. Eur. J. Clin. Microbiol. Infect. Dis. 33, 1843–1846. doi: 10.1007/s10096-014-2142-7
Ruimy, R., Brisabois, A., Bernede, C., Skurnik, D., Barnat, S., Arlet, G., et al. (2010). Organic and conventional fruits and vegetables contain equivalent counts of Gram-negative bacteria expressing resistance to antibacterial agents. Environ. Microbiol. 12, 608–615. doi: 10.1111/j.1462-2920.2009.02100.x
Schwaiger, K., Helmke, K., Hölzel, C. S., and Bauer, J. (2011). Antibiotic resistance in bacteria isolated from vegetables with regards to the marketing stage (farm vs. supermarket). Int. J. Food Microbiol. 148, 191–196. doi: 10.1016/j.ijfoodmicro.2011.06.001
Seo, S., and Matthews, K. R. (2014). Exposure of Escherichia coli O157:H7 to soil, manure, or water influences its survival on plants and initiation of plant defense response. Food Microbiol. 38, 87–92. doi: 10.1016/j.fm.2013.08.015
Thanner, S., Drissner, D., and Walsh, F. (2016). Antimicrobial resistance in agriculture. mBio 7, e02227–e02215. doi: 10.1128/mBio.02227-15
Van der Zwaluw, K., de Haan, A., Pluister, G. N., Bootsma, H. J., de Neeling, A. J., and Schouls, L. M. (2015). The carbapenem inactivation method (CIM), a simple and low-cost alternative for the Carba NP test to assess phenotypic carbapenemase activity in gram-negative rods. PLoS ONE 10:e0123690. doi: 10.1371/journal.pone.0123690
Van Hoek, A. H., Veenman, C., van Overbeek, W. M., Lynch, G., de Roda Husman, A. M., and Blaak, H. (2015). Prevalence and characterization of ESBL- and AmpC-producing Enterobacteriaceae on retail vegetables. Int. J. Food Microbiol. 204, 1–8. doi: 10.1016/j.ijfoodmicro.2015.03.014
Veldman, K., Kant, A., Dierikx, C., van Essen-Zandbergen, A., Wit, B., and Mevius, D. (2014). Enterobacteriaceae resistant to third-generation cephalosporins and quinolones in fresh culinary herbs imported from Southeast Asia. Int. J. Food Microbiol. 177, 72–77. doi: 10.1016/j.ijfoodmicro.2014.02.014
Viswanathan, P., and Kaur, R. (2001). Prevalence and growth of pathogens on salad, vegetables, fruits and sprouts. Int. J. Hyg. Environ. Health. 203, 205–213. doi: 10.1078/S1438-4639(04)70030-9
Warning, A., and Datta, A. K. (2013). Interdisciplinary engineering approaches to study how pathogenic bacteria interact with fresh produce. J. Food Eng. 114, 426–448. doi: 10.1016/j.jfoodeng.2012.09.004
Zurfluh, K., Nüesch-Inderbinen, M., Morach, M., Zihler Berner, A., Hächler, H., and Stephan, R. (2015). Extended-spectrum ß-lactamase-producing-Enterobacteriaceae in vegetables imported from the Dominican Republic, India, Thailand and Vietnam. Appl. Environ. Microbiol. 81, 3115–3120. doi: 10.1128/AEM.00258-15
Keywords: antimicrobial resistance, Gram negative bacteria, third-generation cephalosporin, fruits, vegetables, farm, market, North Africa
Citation: Mesbah Zekar F, Granier SA, Marault M, Yaici L, Gassilloud B, Manceau C, Touati A and Millemann Y (2017) From Farms to Markets: Gram-Negative Bacteria Resistant to Third-Generation Cephalosporins in Fruits and Vegetables in a Region of North Africa. Front. Microbiol. 8:1569. doi: 10.3389/fmicb.2017.01569
Received: 13 June 2017; Accepted: 02 August 2017;
Published: 24 August 2017.
Edited by:
David Rodriguez-Lazaro, University of Burgos, SpainReviewed by:
Alberto Quesada, University of Extremadura, SpainRoger Stephan, University of Zurich, Switzerland
Marta Hernandez, University of Valladolid, Spain
Copyright © 2017 Mesbah Zekar, Granier, Marault, Yaici, Gassilloud, Manceau, Touati and Millemann. This is an open-access article distributed under the terms of the Creative Commons Attribution License (CC BY). The use, distribution or reproduction in other forums is permitted, provided the original author(s) or licensor are credited and that the original publication in this journal is cited, in accordance with accepted academic practice. No use, distribution or reproduction is permitted which does not comply with these terms.
*Correspondence: Yves Millemann, eXZlcy5taWxsZW1hbm5AdmV0LWFsZm9ydC5mcg==