- 1Department of Clinical Microbiology, Ruijin Hospital, Shanghai Jiao Tong University School of Medicine, Shanghai, China
- 2Department of Medical Microbiology and Parasitology, Institutes of Medical Sciences, Shanghai Jiao Tong University School of Medicine, Shanghai, China
- 3Department of Laboratory Medicine, Shandong Provincial Hospital, Shandong University, Jinan, China
ICESag37, a novel integrative and conjugative element carrying multidrug resistance and potential virulence factors, was characterized in a clinical isolate of Streptococcus agalactiae. Two clinical strains of S. agalactiae, Sag37 and Sag158, were isolated from blood samples of new-borns with bacteremia. Sag37 was highly resistant to erythromycin and tetracycline, and susceptible to levofloxacin and penicillin, while Sag158 was resistant to tetracycline and levofloxacin, and susceptible to erythromycin. Transfer experiments were performed and selection was carried out with suitable antibiotic concentrations. Through mating experiments, the erythromycin resistance gene was found to be transferable from Sag37 to Sag158. SmaI-PFGE revealed a new SmaI fragment, confirming the transfer of the fragment containing the erythromycin resistance gene. Whole genome sequencing and sequence analysis revealed a mobile element, ICESag37, which was characterized using several molecular methods and in silico analyses. ICESag37 was excised to generate a covalent circular intermediate, which was transferable to S. agalactiae. Inverse PCR was performed to detect the circular form. A serine family integrase mediated its chromosomal integration into rumA, which is a known hotspot for the integration of streptococcal ICEs. The integration site was confirmed using PCR. ICESag37 carried genes for resistance to multiple antibiotics, including erythromycin [erm(B)], tetracycline [tet(O)], and aminoglycosides [aadE, aphA, and ant(6)]. Potential virulence factors, including a two-component signal transduction system (nisK/nisR), were also observed in ICESag37. S1-PFGE analysis ruled out the existence of plasmids. ICESag37 is the first ICESa2603 family-like element identified in S. agalactiae carrying both resistance and potential virulence determinants. It might act as a vehicle for the dissemination of multidrug resistance and pathogenicity among S. agalactiae.
Introduction
Streptococcus agalactiae (group B streptococcus) is the leading cause of neonatal sepsis and meningitis in many countries. It is also an important pathogen among pregnant women, immunocompromised adults, and the elderly (Le Doare and Heath, 2013).
Erythromycin resistance in S. agalactiae has increased in the last decade in several countries, with slight geographical variations (Betriu et al., 2003). A recent study had reported that the prevalence of erythromycin resistance in S. agalactiae was 61.5% in Shanghai, China, and 69.8% of this was associated with the gene ermB (Yan et al., 2016). Macrolide resistance is attributed to two principal mechanisms, a methylase-mediated target site modification encoded by the erm genes, and an active efflux mediated by the mef genes (Leclercq, 2002; Varaldo et al., 2009). The erm genes modify the post-transcriptional methylation of an adenine residue in the 23S rRNA of bacterial ribosomes and render co-resistance to macrolides, lincosamides, and streptogramins B antibiotics (MLSB), both constitutive (cMLSB) and inducible (iMLSB) ones (Dzyubak and Yap, 2016; Mingoia et al., 2016). Insertions, duplications, deletions, and missense mutations within the leader regulatory regions upstream of the erm gene are commonly found in the constitutively expressed erm operons (Dzyubak and Yap, 2016). High rates of tetracycline resistance have also been observed in Streptococcus species (Luna and Roberts, 1998; Chopra and Roberts, 2001; Princivalli et al., 2009; Cattoir, 2016). Both tet(M) and tet(O) encode tetracycline resistance ribosomal protection proteins (RPRs). tet(M) is considered the most prevalent, due to its association with the broad host range Tn916/Tn1545 family of conjugative transposons (Roberts and Mullany, 2011; Warburton et al., 2016). tet(O) has been discovered chromosomally in several Gram-positive organisms such as Streptococcus and Staphylococcus species (Nguyen et al., 2014).
Integrative and conjugative elements (ICEs) comprise a diverse group of mobile genetic elements found in both Gram-positive and Gram-negative bacteria. They reside in the host chromosome, but retain the ability to excise and transfer through conjugation (Wozniak and Waldor, 2010). ICEs employ integrases to integrate into the host chromosome and a type IV secretion system is always encoded to mediate the conjugation (Johnson and Grossman, 2015). They bestow a wide range of phenotypes on their hosts through the cargo genes they carry, such as antibiotic resistance determinants, virulence factors, and metabolic genes (Wozniak and Waldor, 2010; Johnson and Grossman, 2015). The transfer of ICEs enhances the fitness of microbes in their hosts and exerts a major effect on bacterial evolution and adaption. So far, several ICEs have been reported in S. agalactiae, including ICESa2603 and ICESag2603VR-1 in S. agalactiae 2603V/R (Tettelin et al., 2002) and TnGBS1 and TnGBS2 in S. agalactiae NEM316 (Glaser et al., 2002).
In this study, we described the molecular characterization of a novel ∼75-kb erm(B)-carrying ICE, ICESag37, which has never before been reported in S. agalactiae.
Materials and Methods
Bacterial Strains and Antimicrobial Susceptibility Test
A clinical strain of S. agalactiae, Sag37, was isolated from the blood sample of a new-born with bacteremia in Shanghai in 2014. Another clinical strain of S. agalactiae, Sag158, was isolated from the blood sample of another new-born, and was used as the recipient. The minimum inhibitory concentrations (MICs) of erythromycin, tetracycline, levofloxacin, clindamycin, and penicillin were determined using the Etest (bioMe’rieux, France), and the results were interpreted based on the guidelines of the Clinical and Laboratory Standards Institutes [CLSI] (2014).
Multilocus Sequence Typing Analysis (MLST) and Serotyping
Multilocus sequence typing analysis (MLST) and serotyping was performed as previously described (Jones et al., 2003; Imperi et al., 2010). After MLST, an online database1 was used for assigning allele numbers, and the sequence types (STs) were obtained from the combination of the results.
Conjugal Transfer Experiments, Pulsed-Field Gel Electrophoresis (PFGE), and S1-Nuclease Pulsed-Field Gel Electrophoresis (S1-PFGE)
Conjugal transfer experiments were performed in broth culture using the strain Sag37 (erythromycin-resistant and levofloxacin-susceptible) as the donor and the strain Sag158 (erythromycin-susceptible and levofloxacin-resistant) as the recipient. Briefly, donor and recipient strains were grown to an OD600 of 0.4 and mixed in a ratio of 1:1 in 2 ml of culture. The suspension was pelleted, resuspended, and used for mating. Selection was performed with suitable concentrations of erythromycin (1 mg/L) and levofloxacin (8 mg/L). All transfer experiments were performed at least thrice. Sag158 and the transconjugant, Sag158-1, were both digested with SmaI endonuclease and S1 nuclease and subjected to PFGE and S1-PFGE, as described in previous studies (Barton et al., 1995; Elliott et al., 1998). Briefly, two or three colonies were selected from a fresh plate of overnight growth and incubated in Todd-Hewitt broth for 5 h at 35°C; this suspension was centrifuged and the pellet was re-suspended in Tris–NaCl buffer. The agarose-bacterium plugs were generated and incubated overnight at 35°C in lysis solution. The plugs were washed several times and digested with a suitable nuclease. The chromosomal digests were separated by PFGE. Pulsotypes were clustered based on a cut-off of 70% similarity.
DNA Sequencing and Sequence Analysis
The genomic DNA was extracted using the QIAGEN Midi Kit (Qiagen, Hilden, Germany). The DNA of Sag37 and Sag158 was sequenced using the pacbio RS II (Pacific Biosciences, Menlo Park, CA, United States). The reads were de novo assembled using the HGAP 3.0 SMRTTM Pipe. The transconjugant was sequenced using the illumina HiSeq sequencing approach. SOAP de novo2 was used to perform genome assembly. Sequence similarity searches were carried out using BLAST3. Open reading frames (ORFs) were predicted using the ORF Finder software4. Protein-coding genes were initially identified and annotated using RAST. The antimicrobial resistance genes were identified using ResFinder5.
Amplification Experiments
PCR assays were performed with three pairs of primers (Table 1), rumAF and SR; rumAR and EF; and rumAF and rumAR (Figure 1), to confirm its site-specific integration: rumA. Outward-directed primers (SR and EF) were used to detect the circular form of ICESag37. DNA preparation, amplification, and electrophoresis of the PCR products were carried out by previously established procedures (Hynes et al., 1992) in conditions indicated for the use of individual primer pairs. The PCR products were verified by sequencing.
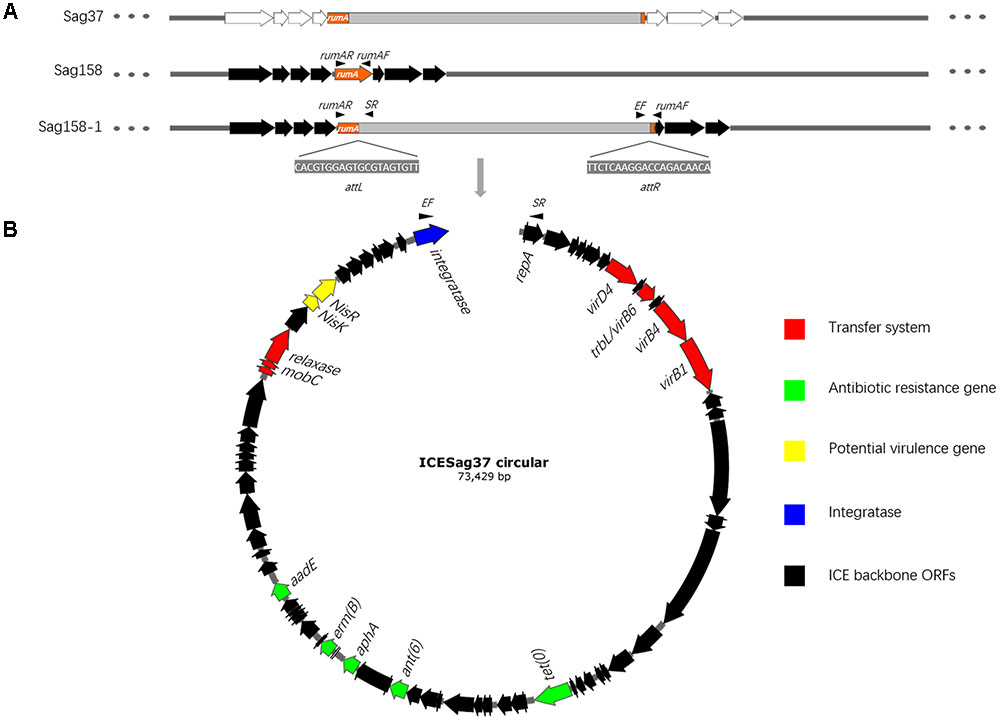
FIGURE 1. (A) Gray and orange fragments represent ICESag37 and the rumA gene, respectively. The surrounding genes are colored white and black. The site-specific integration of ICESag37 into the rumA gene is clearly visible. The 20-bp attL and attR sequences of ICESag37 and primers, rumAF and rumAR, are also shown. (B) Schematic diagram of the circular form of ICESag37. Black arrows indicate the positions and directions of outward-directed primers, SR and EF. The core genes are shown in the circular form of ICESag37. Color codes are defined in the inset.
Nucleotide Sequence Accession Numbers
The nucleotide sequences of Sag37 and Sag158 have been deposited in the Genbank sequence database under the accession numbers CP019978 and CP019979, respectively. The sequence read archive (SRA) of Sag158-1 has been deposited in Genbank under the accession number SRR5585701.
Results
Early Characterization of Sag37 and Sag158
Sag37 was highly resistant to erythromycin, clindamycin, and tetracycline and susceptible to levofloxacin and penicillin, while Sag158 was resistant to tetracycline and levofloxacin, and susceptible to erythromycin, clindamycin, and penicillin. Sag37 and Sag158 displayed different STs (12 and 19, respectively) and serotypes (Ib and III, respectively). All these results, the isolation data, and the resistance genes of the two strains are summarized in Table 2.
Transfer of Erythromycin Resistance
Through mating experiments, erythromycin resistance was transferred from the donor, Sag37 (erythromycin-resistant and levofloxacin-susceptible), to the recipient, Sag158 (erythromycin-susceptible and levofloxacin-resistant), at a frequency of ∼3 × 10-7. Randomly selected erythromycin-resistant transconjugants were used for further experiments. The principle features and MICs of the transconjugant, Sag158-1, are summarized in Table 1. Sag158-1 showed the same erythromycin MIC as the donor and a higher tetracycline MIC than the donor strain. SmaI-PFGE analysis indicated a new SmaI fragment (Figure 2). S1-PFGE revealed that Sag37 harbored no plasmids. The erythromycin resistance was also transferred from Sag37 to two other clinical S. agalactiae recipients, although the data is not shown in this paper.
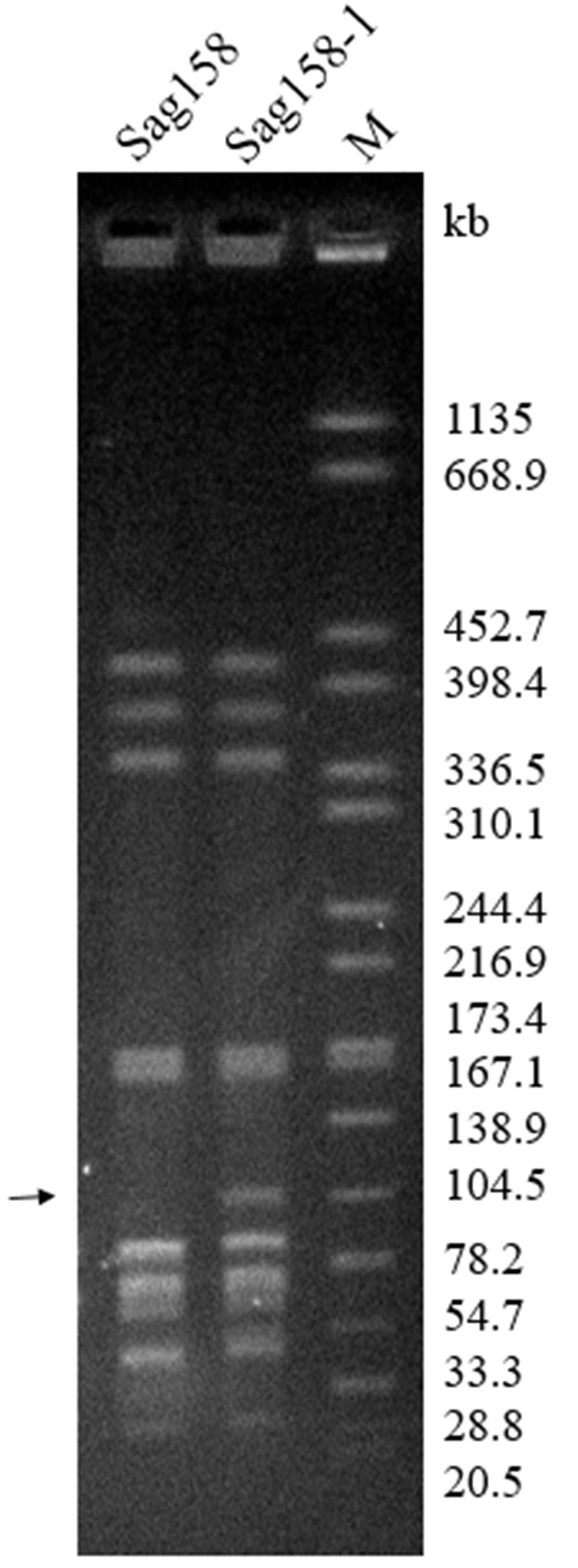
FIGURE 2. Pulsed-field gel electrophoresis (PFGE) profiles of S. agalactiae Sag158 and its transconjugant, Sag158-1. PFGE analysis showed the appearance of a new SmaI fragment (black arrow). M, Salmonella enterica serotype Braenderup H9812 was digested with XbaI and used as a molecular size marker.
General Genomic Features and Detection of the Mobile Element
Whole genome sequencing was carried out for the two strains investigated in this study, Sag37 and Sag158. The genomes of Sag37 and Sag158 comprised a single circular chromosome and were 2,198,785 and 2,096,882 bp in length, respectively, with average G + C contents of 35.77 and 35.66%, respectively. A total of 2180 protein coding sequences (CDS) were predicted in Sag37, while 2093 CDS were predicted in Sag158. Different substitutions were found in the quinolone resistance-determining regions of Sag158 (Ser81Leu in gyrA and Ser79Tyr and Ser140Pro in parC), which were not found in Sag37.
In order to track possible genomic clues regarding the mobile elements, comparative genome analyses were performed for Sag37, Sag158, and Sag158-1. We identified a contig in Sag158-1, which contained all the resistance genes that were present in Sag37, but were absent in Sag158. We aligned this contig with Sag37 and Sag 158, and confirmed that it did exist in Sag37, but was absent in Sag158. Further sequence analysis confirmed the presence of a ∼75-kb mobile element carrying erm(B) and tet(O).
Circularization and Integration Site of ICESag37
PCR assays using outward-directed primers (SR and EF) revealed that the mobile element could excise and form a covalently circular intermediate in both Sag37 and Sag158-1 (Figure 1). Sequence analysis of the amplicons and the left and right junctions of the mobile element enabled us to identify the putative core sites (attI, attL, and attR) and obtain the complete sequence.
Sequences adjacent to the ends of the mobile element in Sag37 and Sag158-1 suggested a possible integration site. PCR assays with three pairs of primers, rumAF and SR; rumAR and EF; and rumAF and rumAR (Figure 1), and sequence analysis of the amplicons confirmed the site-specific integration. The mobile element encoded a serine family integrase, which mediated chromosomal integration at the 3′ end of the conserved gene, rumA (23S rRNA [uracil-5-]-methyltransferase). The integrase was also confirmed through a PCR assay.
Characterization of ICESag37
The results of the mating experiments, the PFGE, and the PCR assays provided a glimpse of the mobile element. The results of the whole genome sequencing and the extensive sequence analysis revealed its full picture. It was designated ICESag37 and is schematically represented in Figure 3. ICESag37 was found to be 73,429 kb in size (629058–702486 bp in Sag37) and predicted to contain 79 ORFs. In addition to the serine family integrase, ICESag37 also contained several genes belong to a type IV secretion system (T4SS), as well as genes encoding putative mobilization proteins of the MobC family and relaxases (Figure 1). The transferability of ICESag37, as shown in the conjugation experiments, strongly suggested that it had an intact and feasible transfer system.
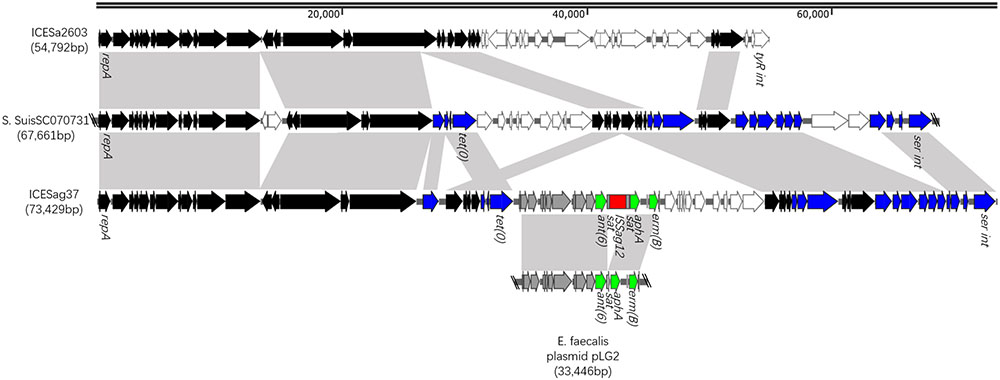
FIGURE 3. Schematic, but to scale, representation of ICESag37 from S. agalactiae Sag37 and linear DNA comparison against ICESa2603 and fragments of S. suis SC070731 anE. faecalis plasmid. The 30 conserved core genes of the ICESa2603 family backbone are indicated by black arrows. Genes homologous to S. suis SC070731 and E. faecalis plasmid are indicated by blue and gray arrows, respectively. Homologous regions are shaded in gray.
ICESag37 carried resistance genes to multiple antibiotics, including erythromycin [erm(B)], tetracycline [tet(O)], and aminoglycosides [aadE, aphA, and ant(6)]. The mosaic genes from the Enterococcus faecalis plasmid, pLG2, were also observed in ICESag37 (Figure 2), and it was this plasmid that introduced the erythromycin resistance determinant, erm(B), as well as the aminoglycoside resistance gene cluster containing ant(6) and aphA, into ICESag37. ISSag12 was integrated into the mosaic genes, disrupting the sat gene. tet(O) and aadE were also embedded in ICESag37, although the source was not known. In addition to the antibiotic resistance genes, ICESag37 also contained genes predicted to be involved in virulence, a two-component signal transduction system (nisK/nisR), from S. suis.
Discussion
A recent study had shown that erm(B) accounts for the vast majority of erythromycin resistance in S. agalactiae in China, with the most predominant phenotype being cMLSB (77.5%) (Yan et al., 2016). The strain used in this study, S. agalactiae Sag37, also constitutively expressed MLSB resistance. The leader peptide in ICESag37 contained a duplicated TAAA motif, generating an earlier stop codon and resulting in a leader peptide that was 9 amino acids shorter (27 amino acids). Various point mutations were also observed in the leading peptide, including substitutions of ATT for GTT and GTA for GCA at leader codons 15 and 25, respectively. However, these changes were observed in both inducible and constitutive strains (Oh et al., 1998; Rosato et al., 1999; Min et al., 2008; Feld et al., 2009); therefore, a firm conclusion cannot be stated and further investigation will be required to explain the mechanism.
ICESag37 was discovered in the strain S. agalactiae Sag37 in this study. Interestingly, homology searches of the ICESag37 sequence showed that it had the highest similarity to S. suis SC070731 (Genbank No. CP003922), but also shared its backbone with ICESa2603. ICESa2603, a ∼54-kb ICE first discovered in S. agalactiae 2603V/R (Tettelin et al., 2002), is considered the prototype of the ICE family, based on the integrases (Bi et al., 2012). ICESag37 contained all 30 conserved core genes present in the ICESa2603 family backbone (Huang et al., 2016), but encoded a serine family integrase instead of intICESa2603, a tyrosine family integrase. It is to be noted that a known hypervariable region in ICESa2603 (white arrows in Figure 2) (Huang et al., 2016) corresponded to the region where we found the mosaic genes in ICESag37. Thus, we referred to ICESag37 as ICESa2603 family-like ICE. The serine family integrase it encoded mediated its chromosomal integration into the 3′ end of the conserved gene, rumA (23S rRNA [uracil-5-]-methyltransferase), which is a known hotspot for the integration of streptococcal ICEs (Ambroset et al., 2016).
Although we do not have sufficient data to pinpoint the origins and assembly of ICESag37, it was apparent that mobilization and recombination events played key roles in its assembly. ICESag37 comprised genes from both phylogenetically close and distant species of Streptococcus. Notably, the antibiotic resistance gene cluster in ICESag37 was homologous to a plasmid in E. faecalis, indicating that inter-species genetic exchange also occurred, thus broadening the host range and dissemination of combined cargo genes. Although the sources of tet(O) and aadE were not clear, genes involved in mobility, including the TnpV and recombinase genes, were found surrounding them. Thus, we proposed that the two resistance genes also originated from mobile elements. However, further research is needed to characterize the mechanisms behind the transfer and recombination of ICEs. Whole-genome comparisons revealed the diversity of the flexible gene pool of S. agalactiae, suggesting the open nature of its pan-genome (Tettelin et al., 2002; Brochet et al., 2008). This result indicated that the genome microevolution of pathogens should be viewed from a different perspective, as the ICE pool may be communal.
Although many ICEs have been characterized in Streptococcus species, to the best of our knowledge, ICESag37 is the first ICESa2603 family-like element carrying both resistance and potential virulence determinants identified in S. agalactiae. Many ICEs containing both resistance and virulence determinants have been reported in S. suis isolated from diseased pigs or humans, such as ICESsu32457, ICESluvan, and ICESsu (BM407)2 (Holden et al., 2009; Palmieri et al., 2012; Bjorkeng et al., 2013). S. suis infection is notorious for causing serious zoonotic diseases and for causing large-scale outbreaks of STSS in China (Chen et al., 2007). Remarkably, ICESag37 showed a striking similarity to S. suis SC070731 and contained its two-component signal transduction system, nisK/nisR, which has been proven to contribute to the virulence of S. suis in vitro and in vivo (Xu et al., 2014). Thus, we proposed that the novel ICE might be a vehicle for the dissemination of not only multidrug resistance, but also potential pathogenicity, among S. agalactiae and that extensive screening for ICESag37 is required.
Author Contributions
All authors listed have made a substantial, direct and intellectual contribution to the work, and approved it for publication.
Conflict of Interest Statement
The authors declare that the research was conducted in the absence of any commercial or financial relationships that could be construed as a potential conflict of interest.
Acknowledgments
This work, including the efforts of Yuxing Ni, was funded by the National Natural Science Foundation of China (NSFC) (81472010), and JS was funded by the Medical-engineering cross project of Shanghai Jiao Tong University (No. YG2015MS59). We acknowledge Hongyu Ou from Shanghai Jiaotong University for technical assistance.
Footnotes
- ^http://pubmlst.org/sagalatiae
- ^http://soap.genomics.org.cn/
- ^http://www.ncbi.nlm.nih.gov
- ^http://ncbi.nlm.nih.gov/gorf/gorf.html
- ^https://cge.cbs.dtu.dk/services/ResFinder/
References
Ambroset, C., Coluzzi, C., Guedon, G., Devignes, M. D., Loux, V., Lacroix, T., et al. (2016). New insights into the classification and integration specificity of Streptococcus integrative conjugative elements through extensive genome exploration. Front. Microbiol. 6:1483. doi: 10.3389/fmicb.2015.01483
Barton, B. M., Harding, G. P., and Zuccarelli, A. J. (1995). A general method for detecting and sizing large plasmids. Anal. Biochem. 226, 235–240. doi: 10.1006/abio.1995.1220
Betriu, C., Culebras, E., Gomez, M., Rodriguez-Avial, I., Sanchez, B. A., Agreda, M. C., et al. (2003). Erythromycin and clindamycin resistance and telithromycin susceptibility in Streptococcus agalactiae. Antimicrob. Agents Chemother. 47, 1112–1114. doi: 10.1128/Aac.47.3.1112-1114.2003
Bi, D., Xu, Z., Harrison, E. M., Tai, C., Wei, Y., He, X., et al. (2012). ICEberg: a web-based resource for integrative and conjugative elements found in Bacteria. Nucleic Acids Res. 40, D621–D626. doi: 10.1093/nar/gkr846
Bjorkeng, E. K., Hjerde, E., Pedersen, T., Sundsfjord, A., and Hegstad, K. (2013). ICESluvan, a 94-kilobase mosaic integrative conjugative element conferring interspecies transfer of VanB-type glycopeptide resistance, a novel bacitracin resistance locus, and a toxin-antitoxin stabilization system. J. Bacteriol. 195, 5381–5390. doi: 10.1128/JB.02165-12
Brochet, M., Couve, E., Glaser, P., Guedon, G., and Payot, S. (2008). Integrative conjugative elements and related elements are major contributors to the genome diversity of Streptococcus agalactiae. J. Bacteriol. 190, 6913–6917. doi: 10.1128/JB.00824-08
Cattoir, V. (2016). “Mechanisms of antibiotic resistance,” in Streptococcus pyogenes: Basic Biology to Clinical Manifestations, eds J. J. Ferretti, D. L. Stevens, and V. A. Fischetti (Oklahoma City, OK: University of Oklahoma Health Sciences Center).
Chen, C., Tang, J., Dong, W., Wang, C., Feng, Y., Wang, J., et al. (2007). A glimpse of streptococcal toxic shock syndrome from comparative genomics of S. suis 2 Chinese isolates. PLOS ONE 2:e315. doi: 10.1371/journal.pone.0000315
Chopra, I., and Roberts, M. (2001). Tetracycline antibiotics: mode of action, applications, molecular biology, and epidemiology of bacterial resistance. Microbiol. Mol. Biol. Rev. 65, 232–260. doi: 10.1128/MMBR.65.2.232-260.2001
Clinical and Laboratory Standards Institutes [CLSI] (2014). Performance Standards for Antimicrobial Susceptibility Testing; 24th Informational Supplement, M100-S24. Wayne, PA: CLSI.
Dzyubak, E., and Yap, M. N. (2016). The expression of antibiotic resistance methyltransferase correlates with mRNA stability independently of ribosome stalling. Antimicrob. Agents Chemother. 60, 7178–7188. doi: 10.1128/AAC.01806-16
Elliott, J. A., Farmer, K. D., and Facklam, R. R. (1998). Sudden increase in isolation of group B streptococci, serotype V, is not due to emergence of a new pulsed-field gel electrophoresis type. J. Clin. Microbiol. 36, 2115–2116.
Feld, L., Bielak, E., Hammer, K., and Wilcks, A. (2009). Characterization of a small erythromycin resistance plasmid pLFE1 from the food-isolate Lactobacillus plantarum M345. Plasmid 61, 159–170. doi: 10.1016/j.plasmid.2009.01.002
Glaser, P., Rusniok, C., Buchrieser, C., Chevalier, F., Frangeul, L., Msadek, T., et al. (2002). Genome sequence of Streptococcus agalactiae, a pathogen causing invasive neonatal disease. Mol. Microbiol. 45, 1499–1513. doi: 10.1046/j.1365-2958.2002.03126.x
Holden, M. T., Hauser, H., Sanders, M., Ngo, T. H., Cherevach, I., Cronin, A., et al. (2009). Rapid evolution of virulence and drug resistance in the emerging zoonotic pathogen Streptococcus suis. PLOS ONE 4:e6072. doi: 10.1371/journal.pone.0006072
Huang, J., Liang, Y., Guo, D., Shang, K., Ge, L., Kashif, J., et al. (2016). Comparative genomic analysis of the ICESa2603 family ICEs and spread of erm(B)- and tet(O)-carrying transferable 89K-subtype ICEs in swine and bovine isolates in China. Front. Microbiol. 7:55. doi: 10.3389/fmicb.2016.00055
Hynes, W. L., Ferretti, J. J., Gilmore, M. S., and Segarra, R. A. (1992). PCR amplification of streptococcal DNA using crude cell lysates. FEMS Microbiol. Lett. 73, 139–142. doi: 10.1111/j.1574-6968.1992.tb05303.x
Imperi, M., Pataracchia, M., Alfarone, G., Baldassarri, L., Orefici, G., and Creti, R. (2010). A multiplex PCR assay for the direct identification of the capsular type (Ia to IX) of Streptococcus agalactiae. J. Microbiol. Methods 80, 212–214. doi: 10.1016/j.mimet.2009.11.010
Johnson, C. M., and Grossman, A. D. (2015). Integrative and conjugative elements (ICEs): what they do and how they work. Annu. Rev. Genet. 49, 577–601. doi: 10.1146/annurev-genet-112414-055018
Jones, N., Bohnsack, J. F., Takahashi, S., Oliver, K. A., Chan, M. S., Kunst, F., et al. (2003). Multilocus sequence typing system for group B streptococcus. J. Clin. Microbiol. 41, 2530–2536. doi: 10.1128/JCM.41.6.2530-2536.2003
Le Doare, K., and Heath, P. T. (2013). An overview of global GBS epidemiology. Vaccine 31(Suppl. 4), D7–D12. doi: 10.1016/j.vaccine.2013.01.009
Leclercq, R. (2002). Mechanisms of resistance to macrolides and lincosamides: nature of the resistance elements and their clinical implications. Clin. Infect. Dis. 34, 482–492. doi: 10.1086/324626
Luna, V. A., and Roberts, M. C. (1998). The presence of the tetO gene in a variety of tetracycline-resistant Streptococcus pneumoniae serotypes from Washington State. J. Antimicrob. Chemother. 42, 613–619. doi: 10.1093/jac/42.5.613
Min, Y. H., Kwon, A. R., Yoon, J. M., Yoon, E. J., Shim, M. J., and Choi, E. C. (2008). Molecular analysis of constitutive mutations in ermB and ermA selected in vitro from inducibly MLSB-resistant enterococci. Arch. Pharm. Res. 31, 377–380. doi: 10.1007/s12272-001-1167-8
Mingoia, M., Morici, E., Marini, E., Brenciani, A., Giovanetti, E., and Varaldo, P. E. (2016). Macrolide resistance gene erm(TR) and erm(TR)-carrying genetic elements in Streptococcus agalactiae: characterization of ICESagTR7, a new composite element containing IMESp2907. J. Antimicrob. Chemother. 71, 593–600. doi: 10.1093/jac/dkv408
Nguyen, F., Starosta, A. L., Arenz, S., Sohmen, D., Donhofer, A., and Wilson, D. N. (2014). Tetracycline antibiotics and resistance mechanisms. Biol. Chem. 395, 559–575. doi: 10.1515/hsz-2013-0292
Oh, T. G., Kwon, A. R., and Choi, E. C. (1998). Induction of ermAMR from a clinical strain of Enterococcus faecalis by 16-membered-ring macrolide antibiotics. J. Bacteriol. 180, 5788–5791.
Palmieri, C., Magi, G., Mingoia, M., Bagnarelli, P., Ripa, S., Varaldo, P. E., et al. (2012). Characterization of a Streptococcus suis tet(O/W/32/O)-carrying element transferable to major streptococcal pathogens. Antimicrob. Agents Chemother. 56, 4697–4702. doi: 10.1128/AAC.00629-12
Princivalli, M. S., Palmieri, C., Magi, G., Vignaroli, C., Manzin, A., Camporese, A., et al. (2009). Genetic diversity of Streptococcus suis clinical isolates from pigs and humans in Italy (2003-2007). Euro Surveill. 14:19310.
Roberts, A. P., and Mullany, P. (2011). Tn916-like genetic elements: a diverse group of modular mobile elements conferring antibiotic resistance. FEMS Microbiol. Rev. 35, 856–871. doi: 10.1111/j.1574-6976.2011.00283.x
Rosato, A., Vicarini, H., and Leclercq, R. (1999). Inducible or constitutive expression of resistance in clinical isolates of streptococci and enterococci cross-resistant to erythromycin and lincomycin. J. Antimicrob. Chemother. 43, 559–562. doi: 10.1093/jac/43.4.559
Tettelin, H., Masignani, V., Cieslewicz, M. J., Eisen, J. A., Peterson, S., Wessels, M. R., et al. (2002). Complete genome sequence and comparative genomic analysis of an emerging human pathogen, serotype V Streptococcus agalactiae. Proc. Natl. Acad. Sci. U.S.A. 99, 12391–12396. doi: 10.1073/pnas.18280799
Varaldo, P. E., Montanari, M. P., and Giovanetti, E. (2009). Genetic elements responsible for erythromycin resistance in streptococci. Antimicrob. Agents Chemother. 53, 343–353. doi: 10.1128/Aac.00781-08
Warburton, P. J., Amodeo, N., and Roberts, A. P. (2016). Mosaic tetracycline resistance genes encoding ribosomal protection proteins. J. Antimicrob. Chemother. 71, 3333–3339. doi: 10.1093/jac/dkw304
Wozniak, R. A., and Waldor, M. K. (2010). Integrative and conjugative elements: mosaic mobile genetic elements enabling dynamic lateral gene flow. Nat. Rev. Microbiol. 8, 552–563. doi: 10.1038/nrmicro2382
Xu, J., Fu, S., Liu, M., Xu, Q., Bei, W., Chen, H., et al. (2014). The two-component system NisK/NisR contributes to the virulence of Streptococcus suis serotype 2. Microbiol. Res. 169, 541–546. doi: 10.1016/j.micres.2013.11.002
Keywords: Streptococcus agalactiae, MDR, integrative and conjugative element (ICE), virulence, ICESa2603 family
Citation: Zhou K, Xie L, Han L, Guo X, Wang Y and Sun J (2017) ICESag37, a Novel Integrative and Conjugative Element Carrying Antimicrobial Resistance Genes and Potential Virulence Factors in Streptococcus agalactiae. Front. Microbiol. 8:1921. doi: 10.3389/fmicb.2017.01921
Received: 19 July 2017; Accepted: 21 September 2017;
Published: 05 October 2017.
Edited by:
Teresa M. Coque, Instituto Ramón y Cajal de Investigación Sanitaria, SpainReviewed by:
Marco Rinaldo Oggioni, University of Leicester, United KingdomAtte Von Wright, University of Eastern Finland, Finland
Copyright © 2017 Zhou, Xie, Han, Guo, Wang and Sun. This is an open-access article distributed under the terms of the Creative Commons Attribution License (CC BY). The use, distribution or reproduction in other forums is permitted, provided the original author(s) or licensor are credited and that the original publication in this journal is cited, in accordance with accepted academic practice. No use, distribution or reproduction is permitted which does not comply with these terms.
*Correspondence: Yong Wang, c2R3YW5neW9uZ0AxMjYuY29t Jingyong Sun, MTM2NzE1Nzg4OTlAMTI2LmNvbQ==