- VTT Technical Research Centre of Finland Ltd., Espoo, Finland
By 2050, the world would need to produce 1,250 million tonnes of meat and dairy per year to meet global demand for animal-derived protein at current consumption levels. However, growing demand for protein will not be met sustainably by increasing meat and dairy production because of the low efficiency of converting feed to meat and dairy products. New solutions are needed. Single cell protein (SCP), i.e., protein produced in microbial and algal cells, is an option with potential. Much of the recent interest in SCP has focused on the valorisation of side streams by using microorganisms to improve their protein content, which can then be used in animal feed. There is also increased use of mixed populations, rather than pure strains in the production of SCP. In addition, the use of methane as a carbon source for SCP is reaching commercial scales and more protein-rich products are being derived from algae for both food and feed. The following review addresses the latest developments in SCP production from various organisms, giving an overview of commercial exploitation, a review of recent advances in the patent landscape (2001–2016) and a list of industrial players in the SCP field.
Introduction
Humans and animals consume protein as a source of nitrogen and essential amino acids, from which they build new structural and functional (e.g., enzymes and hormones) proteins that enable them to survive. In extreme conditions, proteins may also be used as a source of energy. The nutritional value of a protein is determined by the amino acid composition; 20 amino acids are commonly found in dietary protein, of which several (i.e., phenylalanine, valine, threonine, tryptophan, methionine, leucine, isoleucine, lysine, and histidine, with arginine, cysteine, glycine, glutamine, proline, and tyrosine also being beneficial) cannot be synthesised by humans or animals and are thus essential and have to be supplied through the diet (for a review see Wu, 2009).
Boland et al. (2013) have explored how an increasing demand for meat and dairy protein will require improvements in animal production, as well as openness to new sources of protein, both as animal feed and for direct human consumption. Animal and dairy production have been increasing steadily over recent decades and theoretically can continue to do so to meet the expected demand, even by 2050 when the demand for meat would surpass 400 M tonnes and that for dairy 800 M tonnes (Boland et al., 2013). However, because plant protein is converted rather inefficiently into meat protein (~6 kg of plant protein is needed to produce 1 kg of meat protein), increasing meat production to match the growing demand is ultimately not sustainable (WHO, 2015). The western world is also interested in developing healthier food, with optimal amino acid composition and low, but good quality fat, combined with ethically sustainable production. These are typically non-animal based, environmentally friendly processes, but may include novel processes such as the production of “cultured” meat, in which meat protein is more efficiently produced in vitro rather than by growing an entire animal (Kadim et al., 2015). Plant-based protein sources, such as beans, are nutritionally valuable sources of protein, but require arable land and water, both of which will become limiting as we strive to meet global protein demand. The protein content of meat is generally about 45%, while that of milk is about 25% and of soybean about 35% (Ghasemi et al., 2011).
Protein can also be provided through the cultivation of various microbes and algae, preferably those which contain more than 30% protein in their biomass and which can provide a healthy balance of essential amino acids. Microbial protein is generally referred to as single cell protein (SCP), although some of the producing microbes, such as filamentous fungi or filamentous algae, may be multicellular. In addition to direct use as SCP, microbes contribute to protein demand when they are used to upgrade the protein content or quality of fermented foods (Bourdichon et al., 2012). Although, microbial protein provides a relatively small proportion of current human nutrition, the growing global demand for protein is likely to make SCP increasingly important (Boland et al., 2013). High growth rates or ability to utilise unique substrates, such as CO2 or methane, result in processes which offer much higher efficiency and/or sustainability than is possible from traditional agriculture.
SCP is currently produced from a limited number of microbial species, particularly when considering human consumption. The range of sources for SCP used in animal feed is broader than that approved for human consumption and is expanding. As is reviewed below, products derived from algae, fungi (including yeast) and bacteria are all in use or under development. The production steps generally include (a) preparation of nutrient media, possibly from waste, (b) cultivation, including solid state fermentation, (c) separation and concentration of SCP, in some cases drying, and (d) final processing of SCP into ingredients and products.
SCP for human consumption is generally produced from food grade substrates, but there is hope that processes will be developed to produce SCP from inexpensive waste materials from the food and beverage processing industries, as well as directly from forestry and agricultural sources (Anbuselvi et al., 2014). Regulatory issues must always be taken into account. With the introduction of algae to microbial protein providers, production from CO2 has become possible, while the greenhouse gas methane is providing a novel source of carbon for SCP from bacteria.
The following review will give an introduction to SCP production and the organisms used as SCP, with a focus on commercially implemented developments in the field. More detailed reviews of research with specific organisms considered for SCP production are provided by Anupama and Ravindra (2000), Ugalde and Castrillo (2002), Rudravaram et al. (2009), Ghasemi et al. (2011), and Nasseri et al. (2011). Here we provide an update on recent advances in the patent landscape (2001–2016) and the current industrial players, based on company profiles found from the web, literature and patent databases.
SCP Production Systems with Different Substrates and Processes
Algae, fungi (filamentous fungi and yeast), and bacteria can all be used as SCP (Anupama and Ravindra, 2000). In the future, dietary protein may also be derived from proteins secreted by engineered microbial cells (e.g., milk or egg white proteins) and produced from animal and plant cell cultures, in which the cells are no longer microbes but are not animals or plants, either. Thus, the distinction of what is SCP and what is other protein becomes blurred.
SCP from Algae
Microalgae which are produced for human or animal consumption typically have high protein content (e.g., 60–70%; Table 1). They also provide fats (with ω-3 fatty acids and carotenoids being of particular interest), vitamins A, B, C, and E, mineral salts, and chlorophyll (Gouveia et al., 2008). They have relatively low nucleic acid content (3–8%; Nasseri et al., 2011).
Microalgae are currently used mainly in the form of supplements, available in tablet, capsule or liquid form, but they are increasingly also processed as ingredients which can be included in pastas, baked goods, snacks, and so on (Gouveia et al., 2008; Zimberoff, 2017). The most accessible commercial products are derived primarily from Arthrospira platensis and Arthrospira maxima (sold as spirulina, marketed by e.g., Hainan Simai Pharmacy Co., Earthrise Nutritionals, Cyanotech Corp., FEBICO, and Mayanmar Spriulina Factory), Chlorella (marketed by e.g., Taiwan Chlorella Manufacturing Co., FEBICO and Roquette Klötze GmbH & Co), Dunaliella salina (marketed by e.g., Qianqiu Biotechnology Co., Ltd., primarily for β-carotene) and Aphanizomenon flos-aquae (marketed by e.g., Blue Green Foods, Klamath Valley Botanicals LLC and E3Live; Gouveia et al., 2008). Euglena Co. Ltd. (Suzuki, 2017) and Algaeon (http://algaeon-inc.com/#products) are both selling products from Euglena, primarily for the β-glucan content, but including whole cell products. TerraVia does not specify the alga provided in their AlgaVia® food ingredient. Enzing et al. (2014) and Vigani et al. (2015) provide useful surveys of the companies and countries involved in production of microalgae as food or feed. Both reviews focus on the European Union, but take note of the involvement of numerous companies in Asia and North America in the industry.
Algae generally feed on CO2 and light, although some products such as AlgaVia® are produced by traditional fermentation rather than by photosynthesis. Outdoor production of algae in open ponds is common, but is subject to contamination (not only biological contamination, but also mineral contamination which affects the quality of the final product) and variation in the weather (Harun et al., 2010). Indoor photobioreactors are also being used to guarantee the supply of fresh algae as feed for aquaculture (molluscs, shrimp, fish; Henrikson, 2013; Mahmoud et al., 2016). Algae are primarily used in aquaculture as a source of omega fatty acids and carotenoid pigments, but their protein also contributes to animal nutrition (Muller-Feuga, 2000).
SCP from Fungi
A wide range of fungi have been considered for use as SCP, as reviewed by Anupama and Ravindra (2000), Rudravaram et al. (2009), and Nasseri et al. (2011). Table 2 lists some of the species that have been researched in recent years, with the protein content observed under the conditions in which they were grown. Products from Saccharomyces, Fusarium, and Torulopsis are commercially available.
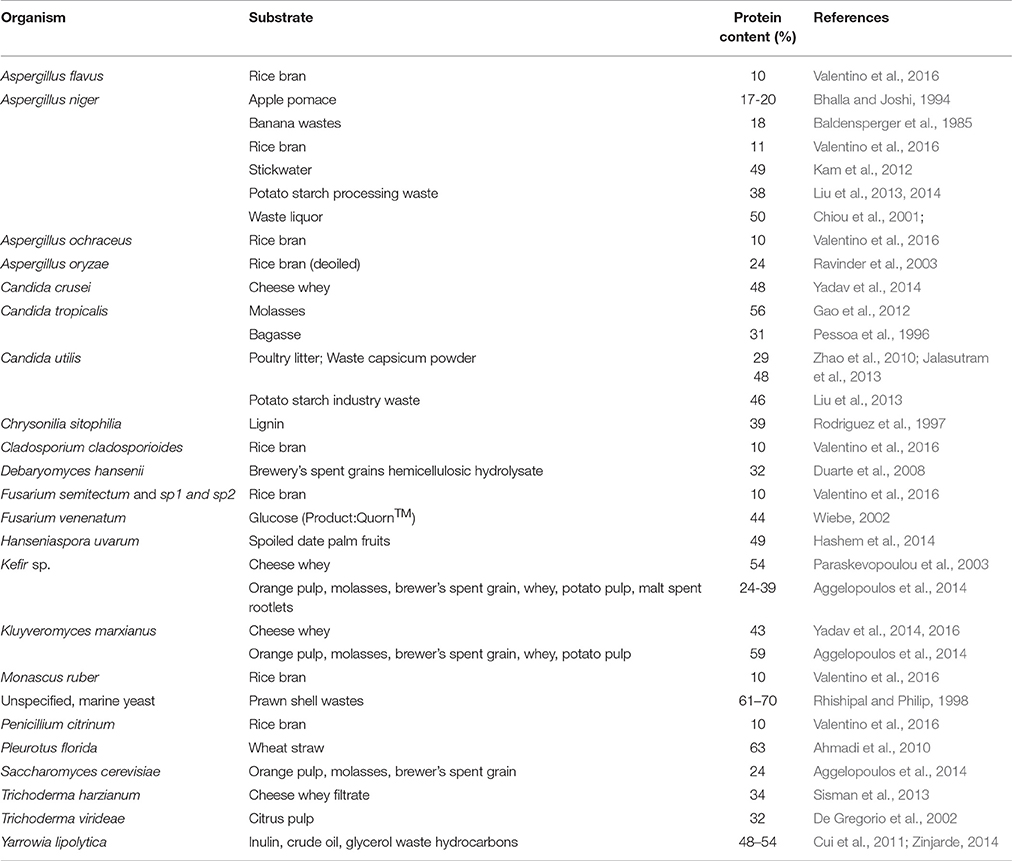
Table 2. Recent reports of fungal protein content produced from specific substrates for species investigated as potential sources of SCP.
Fungi grown as SCP will generally contain 30–50% protein (Anupama and Ravindra, 2000; Nasseri et al., 2011). The amino acid composition compares favourably with the FAO guidelines; threonine and lysine content is typically high, but methionine content relatively low, although still meeting the FAO/WHO recommendations (Anderson et al., 1975). The methionine content of some fungal products such as Marmite® is even lower. Sulphur containing amino acids have been enriched in SCP from K. fragilis by cultivation on whey (Willetts and Ugalde, 1987).
In addition to protein, SCP derived from fungi is expected to provide vitamins primarily from the B-complex group (thiamine, riboflavin, biotin, niacin, pantothenic acid, pyridoxine, choline, streptogenin, glutathione, folic acid, and p-amino benzoic acid). The cell walls of fungi are rich in glucans, which contribute fibre to the diet. Low-density lipoprotein cholesterol has been reduced in volunteers who consumed myco-protein from Fusarium venenatum (Turnbull et al., 1992) and blood glucose and insulin levels may also be favourably affected (Lang et al., 1999). Fungi are expected to have a moderate nucleic acid content (7–10%; Nasseri et al., 2011), which however is too high for human consumption and requires processing to reduce it (Edelman et al., 1983).
The Quorn™ brand (http://www.quorn.com/) was launched in 1985 by Marlow Foods (UK). Quorn™ products contain mycoprotein from the filamentous fungus F. venenatum. The fungal biomass provides a texture that resembles meat products. Quorn™ may be the only SCP product exclusively used for human nutrition and has been extensively branded, marketed and sold for that purpose. The company was recently (2015) acquired by the Philippine instant noodles maker Monde Nissin Corp for 550 million pounds (http://www.reuters.com/article/quorn-ma-idUSL5N1204C720151001).
Spent brewer's yeast (Saccharomyces cerevisiae) have been sold for more than a century in yeast extracts such as Marmite® (Unilever and Sanitarium Health Food), Vegemite® (Bega Cheese Ltd.), Cenovis® (Gustav Gerig AG), and Vitam-R® (VITAM Hefe-Produkt GmbH). Yeast extracts provide a good source of five important group-B vitamins, but also protein. Another commercially available yeast, Torula (Candida utilis, renamed as Pichia jadinii), a widely used flavoring agent, is also high in protein. Torula was used in Provesteen® T, produced by the Provesta Corporation in the 1980s, along with similar products using Pichia and Kluyveromyces yeast (Hitzman, 1986). Torula is rich in the amino acid glutamic acid and for this reason it has been used to replace the flavor enhancer monosodium glutamate (MSG).
A process called “Pekilo” was developed in Finland to produce SCP for animal feed from the sugars present in sulphite liquor of paper mill effluents (reviewed in Ugalde and Castrillo, 2002). The filamentous fungus Paecilomyces varioti was grown on sugars, including pentoses, in the sulphite waste liquor or wood hydrolysates. There were two factories operating in Finland in Mänttä and in Jämsänkoski during 1982–1991, but as the cellulose mills ceased operations, these factories were also closed. Although, the product was sold as animal feed, it was also investigated as a supplement in meat products such as sausages and meat balls (Koivurinta et al., 1979). The Pekilo process strain is available from the VTT Ltd. culture collection (www.culturecollection.vtt.fi/).
Quorn™ and yeast spreads like Marmite® are produced from starch-derived glucose, while the Pekilo process used lignocellulosic sugars. In addition to these carbon sources, alkanes and methanol have been used for SCP production by yeast and filamentous fungi. Methylotrophic yeasts, for example Komagataella pastoris (previously Pichia pastoris), produce biomass and protein from methanol (Rashad et al., 1990). Industrial scale production has been carried out, e.g., by Phillips Petroleum Company. Their yeast produced 130 g (DW)/l biomass, with a productivity of more than 10 g l−1 h−1 (Johnson, 2013).
British Petroleum pioneered production of Yarrowia lipolytica SCP for animal feed from waxy n-paraffins from an oil refinery in the 1970's, building a pilot plant with up to 100 kton annual production capacity (Groenewald et al., 2014). Although the product itself was considered safe, the plant failed to get the required production permits because of environmental concerns (Bamberg, 2000). Combined with the high price of substrate resulting from the 1973 oil crises, this led British Petroleum to abandon its interest in SCP (Groenewald et al., 2014). Yarrowia SCP is now available on a smaller scale as Yarrowia Technology products (Yarrowia Equinox and Yarrowia GoodStart products) from Skotan S.A. in Poland (http://www.yarrowiatechnology.com/?lang=3). Although, oils and carotenoids are the most common Yarrowia products for human use (Groenewald et al., 2014), the American-based Nucelis also offers a protein rich Yarrowia Flour (https://www.nucelis.com/products.php?product=oils#circles).
Research and development on SCP with various fungal species is active and ongoing and may lead to novel products or production processes. For example, Zhao et al. (2013) described a process in which antibacterial peptides would be produced and secreted by Y. lipolytica, generating a high-value product, while the spent yeast could be used as SCP, since its protein content was high. Much of the current research focuses on the use of waste substrates such as sugarcane bagasse (e.g., Penicillium janthinellum with 46% protein, Rao et al., 2010), brewery's spent grains, hemicellulosic hydrolysate (e.g., Debaryomyces hansenii, White et al., 2008; Kluyveromyces marxianus, Aggelopoulos et al., 2014), whey (mixed yeast cultures, Yadav et al., 2014, 2016; K. marxianus, Aggelopoulos et al., 2014), and mixtures of other common food industry wastes such as orange and potato residues, molasses, and malt spent rootlets (K. marxianus, Aggelopoulos et al., 2014). Aggelopoulos et al. (2014) used solid state fermentation (SSF) rather than submerged cultivation and also noted that higher value products could be extracted prior to use of the protein-enriched residues as animal feed.
SCP from Bacteria
Bacteria also have a long history of use as SCP, particularly in animal feed. Some of the more commonly studied species have been reviewed by Anupama and Ravindra (2000), Rudravaram et al. (2009), and Nasseri et al. (2011) and Table 3 provides a list of more recent research on bacterial SCP.
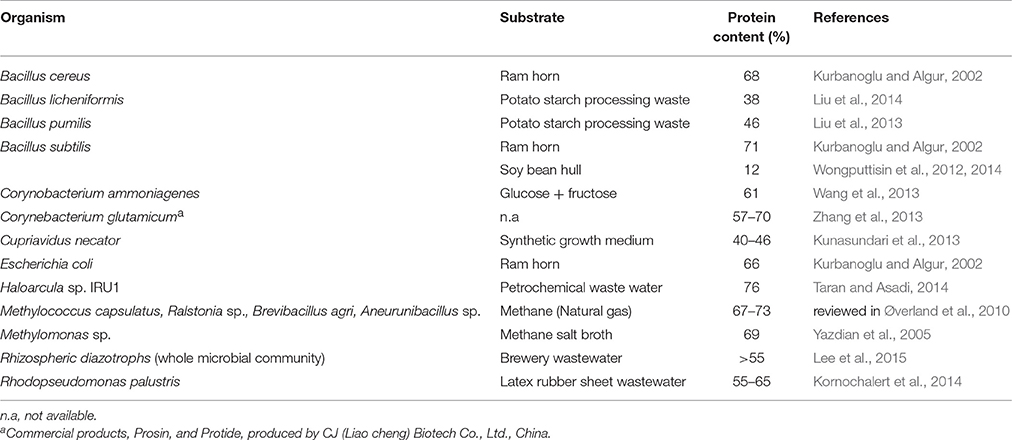
Table 3. Recent reports of bacterial protein content on specific substrates for species investigated as potential sources of SCP.
Bacterial SCP generally contains 50–80% protein on a dry weight basis (Anupama and Ravindra, 2000) and the essential amino acid content is expected to be comparable to or higher than the FAO recommendations (Erdman et al., 1977). Methionine content up to 3.0% has been reported (Schulz and Oslage, 1976), which is higher than that generally obtained in algal or fungal SCP. Similar amino acid composition is observed with methanol or methane grown bacteria (Øverland et al., 2010). As with fungi, bacterial SCP has high nucleic acid content (8–12%), especially RNA, and thus requires processing prior to usage as food/feed (Kihlberg, 1972; Nasseri et al., 2011; Strong et al., 2015). In addition to protein and nucleic acid, bacterial SCP provides some lipid and vitamins from the B group.
Imperial Chemical Industries developed a SCP (Pruteen) for animal feed from methanol, using the bacterium Methylophilus methylotrophus. Pruteen contained up to 70% protein and was used in pig feed (Johnson, 2013). Pruteen, however, could not compete with cheaper animal feeds that were available at the end of the 1970s and production was discontinued. Pruteen was produced from methanol, but methane is now gaining interest as a substrate for SCP. UniBio A/S (utilizing knowledge gained by Dansk BioProtein A/S) and Calysta Inc. have both developed fermentation technology to convert natural gas to animal feed protein by using methanotrophic bacteria. UniBio A/S uses a U-loop fermenter, to achieve a productivity of 4 kg m−3 h−1, producing UniProtein® with ~70% protein, which has been approved for use in animal feed (http://www.unibio.dk/company/subpage-1/). The U-loop fermenter is designed to enhance mass transfer rates of methane from the gas to the liquid phase, making more methane available for the bacteria (Petersen et al., 2017). Calysta Inc. opened a production facility for their product, FeedKind®, in the UK in 2016 and is partnering with Cargill to build a larger production facility in the U.S.A (http://calysta.com/commercialization/). FeedKind®, like UniProtein®, is used in animal feed. Methane is an interesting substrate, since it is a major by-product of cattle and pig farming (Philippe and Nicks, 2015), as well as being available from biogas production (landfills, waste). Excess methane is currently flared. VTT Ltd. is investigating the reactor design and options for coupling farm methane generation with the production of microbial oil and feed protein (http://www.vttresearch.com/media/news/protein-feed-and-bioplastic-from-farm-biogas) from the methanotrophic bacteria Methylococcus capsulatus (group I), Methylosinus trichosporium (group II), and Methylocystis parvus (group II).
As with SCP from fungi, other developments in the production of bacterial SCP focus on upgrading various waste substrates or valorisation of waste water treatment. Examples include the treatment of potato starch processing waste in a two-step process using Aspergillus niger to degrade fibres in the potato residue and Bacillus licheniformis to produce protein (Liu et al., 2014). Economic analyses indicated that the process could address not only the pollution problem of the starch industry, but also the shortage of protein for animal feed in China (Liu et al., 2014). Another example of simultaneous waste water management and SCP production was reported by Kornochalert et al. (2014) for rubber sheet factory waste. They demonstrated that the chemical oxygen demand, suspended solids and total sulfides in the waste water was reduced by the purple nonsulfur bacterium, Rhodopseudomonas palustris, to levels that met the guidelines for use as irrigation water in Thailand and that the biomass produced was suitable for SCP (Kornochalert et al., 2014).
Soy-bean hull has been fermented with B. subtilis to improve its nutritional value as a feed for monogastric animals (Wongputtisin et al., 2014).
Kunasundari et al. (2013) describe a novel secondary product, co-produced with bacterial SCP. They cultivated Cupriavidus necator in a large scale to produce biomass high in both protein and polyhydroxyalkanoate (PHA). This biomass was fed to rats. The feed was not only well-tolerated and safe for rats, but the rats also produced faecal pellets containing PHA granules, which enabled the purification of substantial amounts of PHA without use of strong solvents (Kunasundari et al., 2013).
Processing of SCP
Depending on the substrate material and intended food/feed application, various processing steps are required prior to formulation of the final SCP product. In the following section we review the most relevant processing needs for SCP.
Cell Wall Degradation in Single Cell Protein Products
Some SCP are used as whole cell preparations, while in others the cell wall may be broken down to make the protein more accessible. SCP, such as Quorn™, may be consumed without degradation of the cell wall, in which case chitin and glucan from fungal cell walls contribute fibre to the diet (Wiebe, 2004). SCP derived from Euglena does not require dirsuption since the cells have proteinaceous pellicles, rather than cell walls, making it more readily digestible.
Various methods have been used to disrupt the cell wall, including mechanical forces (crushing, crumbling, grinding, pressure homogenization, or ultra-sonication), hydrolytic enzymes (endogenous or exogenous), chemical disruption with detergents, or combinations of these methods (reviewed in Nasseri et al., 2011). Cell disruption may affect the quality and quantity of protein and other components in the SCP. Products such as Marmite® and Vegemite® are cell extracts, generated by heating the cells to 45–50°C long enough for intracellular enzymes to partially hydrolyse the cell wall; the proteins are also reduced to smaller peptides (Trevelyan, 1976; Ugalde and Castrillo, 2002).
Nucleic Acid Removal in Single Cell Protein Products
Although algae generally have low nucleic acid content, the rapidly proliferating bacterial and fungal species have high nucleic acid (RNA) content. RNA content and degradation are affected by growth conditions, growth rate, and the carbon-nitrogen ratio (Trevelyan, 1976). When SCP is produced for human consumption, high nucleic acid content is a problem because ingestion of purine compounds derived from RNA breakdown increases uric acid concentrations in plasma, which can cause gout and kidney stones (Edelman et al., 1983). SCP with high nucleic acid content which is intended as animal feed is recommended only for feeding animals with short life spans (Strong et al., 2015). Gao and Xu (2015) and Xu (2015) have recently described methods for measuring the nucleotide content of complex SCP products.
Various methods to decrease the RNA content in SCP have been developed (Sinskey and Tannenbaum, 1975) and continue to be in use. Endogenous RNA degrading enzymes (ribonucleases) can be exploited in degradation of RNA, after activation with heat treatment (60–70°C) as used in the production of Quorn™ (Anderson and Solomons, 1984). Ribonucleases can also be added to the process or used as immobilized enzymes (Martinez et al., 1990; Hameş and Demir, 2015). Degraded RNA components diffuse out of the cells, but biomass loss (35–38%) also occurs. The process was improved by using higher temperatures (72–74°C) for 30–45 min, with less loss of biomass (30–33% loss; Ward, 1998). The temperature increase requires steam input, which is a cost factor, but heat is also needed for final treatment of the biomass at 90°C after the RNAse activation (Knight et al., 2001).
Alkaline hydrolysis and chemical extraction methods have also been studied. Viikari and Linko (1977) used an alkali treatment to reduce RNA in P. varioti biomass, used in for Pekilo-process, to below 2%. Treatment at 65°C, pH 7.5–8.5, to activate endogenous ribonuclease, also reduced the RNA content to <2%, while the protein content stayed at 50%.
Safety of SCPs
As for any food or feed product, SCP needs to be safe to produce and use. Regulations exist in most regions to ensure that food or feed are safe for consumption (Bagchi, 2006). Typically these distinguish not only between food (for humans) and feed (for animals), but also between food (providing nutrition and potentially taste and aroma) and food additives (preservatives, colourants, texture modifiers, etc.), or feed and feed additives. Exact definitions may differ between regions, but international standards, regulated through the Joint FAO/WHO Expert Committee on Food Additives, apply to internationally traded products (WHO, 2017). Regulations differ depending on the intended purpose of the product, and although SCP is expected to be either food or feed (providing nutrition), some products may enter the market as additives (e.g., providing colour), rather than as SCP, even though protein is present in the product, limiting the extent to which they are added and their value as SCP. Coppens et al. (2006) summarised the European regulations related to food and food supplements, concluding that “the process of having ‘functional foods’ ready for the market is certainly a costly and time-consuming task,” but also that the process can be successful.
Smedley (2013) provides useful references to the specific regulations related to feed and feed additives in Brazil, Canada, China, the European Union, Japan, South Africa, and the United States, and the differences between the regulations in these regions. It should be noted that not all animals are regarded the same in all regions, thus pet food is regulated as feed in some areas, but not in others. Authorisation is required before sale of new feed or additives (Smedley, 2013).
Key concerns are the RNA content, toxins produced by microbes (production hosts or contaminants), potential allergy symptoms, and harmful substances derived from the feedstock such as heavy metals. Methods have been developed and are in industrial use to decrease the RNA content to acceptable levels, as discussed above.
The challenge of toxins is overcome by carefully selecting the production organism, the process conditions, and the product formulation. Some fungi produce mycotoxins and this makes them undesirable sources of SCP (Anupama and Ravindra, 2000). The effects of fungal toxins range from allergic reactions to carcinogenesis and death. Both humans and animals are affected, so mycotoxins cannot be tolerated in SCP for either human or animal consumption. Quorn™ mycoprotein underwent extensive testing for the presence of mycotoxins or other toxic compounds before being approved for human consumption (Wiebe, 2004). The particular strain of F. venenatum does not produce mycotoxins under production conditions, but the process is still monitored to ensure none are present. The initial safety testing for Quorn™ mycoprotein involved 16 years, with many more years required to gain approval for sale outside the UK (Solomons, 1986). Y. lipolytica is another fungus whose safety has been extensively assessed, demonstrating that it would be safe to use in a variety of food applications, including as SCP (Groenewald et al., 2014).
Bacteria may also produce toxins which limit their use as SCP. Toxins may be extracellular (exotoxins) or cell bound (endotoxins). For example, both Pseudomonas spp. and Methylomonas methanica produce high levels of protein and have been assessed for use as SCP. Both also produce endotoxins that cause febrile reactions (Rudravaram et al., 2009). These can be destroyed by heating. Further, a study on immunogenicity of SCP from M. capsulatus showed that the cell-free preparation (i.e., the cell wall is removed) did not cause immune responses in mice, although whole cell preparations did (Steinmann et al., 1990).
The use of varying waste types of raw materials for SCP production is appealing from the cost and sustainability point of view, but may be challenging from the safety perspective and the origin of the feedstock must be carefully considered. For example, Quorn™ is produced in a chemically defined medium from glucose (hydrolysed starch) in a well-defined process which meets GLP standards (Wiebe, 2002, 2004). Any product for human consumption which would be produced from biomass hydrolysates or waste streams would need to provide an equivalent safety record before finding approval in Europe or North America. In addition to the safety requirements associated with the use of waste-derived substrates for SCP, public perception and acceptance of waste-derived foods would be a key element to consider when implementing SCPs in human diets.
Genetically Modified Organisms in SCP Production—Future Possibilities
Use of genetically modified organisms (GMO) in food and feed has not yet found public acceptance in Europe, although there is more acceptance elsewhere in the world. As data regarding GMO consumption accumulates, they may gain further acceptance as protein sources become scarcer, particularly if a market develops for healthy or personalized nutrition. GMO yeast from bioethanol factories can already be used as cattle feed in some countries. Use of genetic elements from the host itself (self-cloning) often means that no foreign DNA is introduced.
Although, Goldberg (1988) discussed the prospect of using genetically engineered microbes as SCP in the 1980s as a means of improving process economics by producing co-products (e.g., an enzyme, organic acid, or antibiotic), the concept was not pursued and has only gained more interest and acceptance in recent years. A wide range of advantages in SCP products from genetic modification has been considered. For example, DuPont has genetically engineered a yeast to produce long-chain omega-3 fatty acids, which are essential to human health (Xie et al., 2015). Genome sequencing and genetic engineering also allow disruption of genes involved in toxin production and thus improved safety of some SCP products. Disruption of genes can be achieved by traditional mutagenesis and screening, but the process may introduce undesired mutations into the product, whereas genetic modification is quicker and more specific. This will be aided by new technologies, such as Clustered Regularly Interspaced Short Palindromic Repeats (CRISPR) that allows specific editing of the genome without introduction of new DNA. Strains which have been modified using CRISPR are not necessarily considered GMOs. CRISPR methodology can also eliminate the introduction of antibiotic resistance genes to the organism, avoiding concern about the spread of antibiotic resistance genes through the use of GMOs.
Carbon source metabolism is another target for improving SCP production processes, since the carbon source can be a major cost in SCP manufacture (Ugalde and Castrillo, 2002). Genetic engineering could broaden the range of substrates used by the production organism or increase the efficiency of their use, enabling the use of multiple feed stocks and ensuring that all potential carbon in the raw material is used. For example, Ren et al. (2016) introduced xylose fermentation capability from Candida intermedia to S. cerevisiae by genome suffling to enable ethanol production from glucose and SCP production from xylose, while Cui et al. (2011) introduced inulase to Y. lipolytica. Similarly, expression of one or more hydrolytic enzymes has improved use of polymeric substrates (Song et al., 2017). Cellulose, starch, or whey could be used in consolidated bioprocesses by an organism modified to produce the tailor-made enzyme cocktail suitable for the particular raw material. Organisms could also be engineered to have improved tolerance to acid, alkali or other compounds associated with specific substrates.
Genetic modifications could also increase the nutraceutical value of the biomass, either by optimising the amino acid composition or by increasing the content of specific vitamins (e.g., D-vitamin, B-vitamin, biotin), fatty acids, glutathione, etc. along with the protein. There is considerable scope for creating SCPs with tailor made, personalized, nutritional composition.
Genetic engineering may also provide new ways of harvesting the proteins for inclusion in food or feed. For example, modification to improve flocculation could reduce costs in collection of cells or the cells could be modified to have a set of cell wall degrading enzymes that would be activated by specific extracellular stimuli to provide proteins without cell walls. Similarly, ribonucleases could be designed to be activated at a specific time in conditions in which proteases would not be activated. Morphological characteristics could also potentially be engineered to provide specific organoleptic properties.
Economic Aspects
Development of SCP processes has always been driven by a need for protein, and this continues to be an important driver in the development of both old and new processes. The valorisation of readily available substrate and waste streams has also been a strong driver and continues to be so. SCP is frequently seen as a potential co-product that could strengthen the economic potential of an otherwise unprofitable biorefinery process, as well as a means of reducing the downstream processing costs required to dispose of process waste. Selling residual biomass as feed is preferable to selling as fertiliser. This is seen in the numerous publications and patents (not addressed in this review) in which specific waste products are converted to SCP and are assessed as food for specific animals. However, environmental concerns also now play a strong role in driving the development of novel SCP products. This is seen particularly in the processes which utilise greenhouse gases: algal SCP from CO2 and bacterial SCP from methane. Such processes are unlikely to be economically viable in the short term, since there are still many problems to overcome in large scale cultivation, but may survive where they are able to benefit from a green premium. In addition, environmental concerns, as well as economic concerns, are helping to drive the development of products from waste streams.
Apart from the environmental benefits, the key elements in estimating the economic viability of a SCP production process are total product cost, capital investment and profitability. Ugalde and Castrillo (2002) estimated that in fungal SCP production 62% of the total product cost would come from the raw material and 19% from the production process. According to Aggelopoulos et al. (2014), raw material costs vary from 35 to 55% of the manufacturing costs, whereas the operation costs, including labour, energy, and consumables take 45–55%. Utilising side-streams and waste biomass is sometimes viewed as a means to reduce the substrate costs, in cases when the substrate does not compromise the usability of the final product.
Scale is also important to the economic viability of SCP production. An empirical relationship exists between cost and scale of production. Continuous operations have been proven to be the most profitable ones and the majority of the SCP processes which have been implemented at industrial scale have been adjusted to continuous design (Ugalde and Castrillo, 2002). On the other hand, small scale, household production of some products may become feasible, in much the way that home yoghurt production or mushroom production has, and as has been suggested for plant cell nutrition without plants (Poutanen et al., 2017).
Update on Industrial Production of SCP—Players and Capacities
Table 4, lists companies reported to produce or to have an interest in SCP, with website and patent information provided when available. A short description of some active companies is given below.
Algaeon Inc. produces β-glucan and whole cell products from the photosynthetic protist Euglena gracillis. Algaeon was started in 2011 and is based in the U.S.A.
BlueBioTech Int. GmbH, a microalgal biotechnology company, which has operated for more than 10 years, producing large quantities of Spirulina and Chlorella.
Calysta Inc. was founded as a private company in 2011. It produces FeedKind® from methane at a pilot facility in the UK, and began distributing commercial samples in 2017. It plans to open a larger facility (producing up to 20,000 tonnes per year) in the U.S.A. in 2019.
Cangzhou Tianyu Feed Additive Co., Ltd is a manufacturer and trading company located in Hebei, China since 2004. Their main products are Yeast Powder, Choline Chloride, Betaine, and Allicin having markets in Southeast Asia, Eastern Asia, Oceania, South Asia, and South America. The company employs 50 people and their total revenue is 5–10 million US$.
CBH Qingdao Co., Ltd has been an established company for decades supplying a range of ingredients and additives for feed and food industries. They can supply products which meet FAMI-QS, ISO, GMP, KOSHER, and HALAL standards.
Cyanotech Corporation is one of the world leading producers of Spirulina with sales in the US and 30 other countries. Their turnover in 2016 was almost 32 million US$. FDA has given GRAS status for Cyanotech's Spirulina as a food ingredient.
The progenitor of Earthrise, Proteus Corporation was founded in 1976. They produce Spirulina with GRAS status. They are GMP certified and have Food Safety System Certification (FSSC) 22000:2011.
E.I.D Parry Ltd., Parry Nutraceuticals Division is part of the 4.4 billion US$ Murugappa Group. They use micro-algal technology to produce nutraceuticals like Spirulina and Chlorella. Their products are sold in more than 40 countries and their main markets are in North America, Europe, South East Asia, and the Far East.
Euglena Co. Ltd. was founded in Japan in 2005. Amongst other products derived from Euglena gracillis, Euglena Co. Ltd. is developing de-fatted Euglena as a source of protein-rich animal feed.
KnipBio was founded in 2013 in the U.S.A. with a focus of providing affordable feed for aquaculture. They produce KnipBio Meal from methanol using a methylotrophic bacterium and plan to start commercial production in 2018.
Lallemand Inc. is a Canadian company specializing in the development, production, and marketing of yeast and bacteria. There are two major groups in the company: the Yeast Group (based in Montreal, Canada) and the Specialties Group (based in Toulouse, France). They produce SCP for human consumption (LBI, Lake States®, Engevita™) from the yeast S. cerevisiae and Torula.
LeSaffre produces yeast (S. cerevisiae) and yeast derived products including SCPs such as Lynside® Nutri, Lynside® ProteYn and related products (Lesaffre Human Care products), as well as yeast-based flavour ingredients (Biospringer products). The company has 7,700 employees and more than 80 subsidiaries in over 40 countries. Their products and services are sold in more than 180 countries and their turnover was ~1.6 billion € in 2013.
Marlow Foods Ltd produces the mycoprotein Quorn™. The Quorn development project started already in the 1960s, when they started to look for a microbial protein source that humans would find enjoyable. Quorn is classified as a safe, well-tolerated food by regulatory bodies across the world, including FDA, and the UK's Food Standards Agency (FSA). The company was acquired by Monde Nissin Corporation in the Philippines for 831 million US$ in 2015.
Nucelis Inc. was founded in 2010 in the U.S.A., but became a subsidiary of Cibus Global in 2014. Along with squalene, vitamin D and nutritional oils, Nucelis Inc. is developing high protein flour from the yeast Yarrowia.
Nutrinsic is based in the USA, with subsidiaries in China. Nutrinsic focuses on the use of waste waters from the food, beverage and biofuel industries to generate feed and fertiliser products. They market a SCP for animal feed called ProFloc™, which is described as having a protein content around 60%. They opened their first USA production facility in 2015, using waste water from a local brewery.
Tangshan Top Bio-Technology Co., Ltd is a manufacturer and trading company located in Hebei, China (Mainland). Their main products are: brewer's yeast, autolyzed yeast, yeast cell wall and yeast extract, including a 100% natural, non-GMO, pure yeast powder as animal feed additive for 1,100–1,250 US$ per ton and a production capacity of 15,000 tons per year per production line. The company was established in 2009 and has ~200 employees. Their main markets are in China, Eastern Asia, Western Europe, Southeast Asia and Mid East, with 40–50% of their products exported.
TerraVia Holdings, Inc. is a publicly held American company which focuses on providing ingredients for food and care products from eukaryotic algae. TerraVia appeared in 2016, but is derived from Solazyme Inc. which was founded in 2003. TerraVia uses traditional stirred tank reactors to cultivate its algae.
UniBio A/S, Denmark is an SME that owns rights to a unique fermentation technology—the U-Loop technology, which enables natural gas to be converted into a high protein product—UniProtein®. UniProtein® has a protein content of ~71% and can be used in feed for animals. UniBio A/S was established in 2001.
Unilever produces yeast extract Marmite® from brewer's spent grain. The number of employees is around 169,000 and the turnover of the company was $52.7 billion in 2016.
Vega Pharma Ltd is located in Zhejiang, China—the Vega Group is developing, manufacturing, and marketing pharmaceuticals, nutritional ingredients, animal health products, and probiotics. They offer a SCP, with up to 65% protein and containing relatively high threonine levels, for animal feed as a by-product of monosodium glutamate production.
Recent Patents (2001–2016)
Recent patents (2001–2016) related to SCP production with algae, fungi, bacteria and mixed microbial populations are listed in Tables 5–8. Some of the patents owned by industrial operators are also shown in Table 4. The number of patents related to the use of algae, bacteria, yeast, or mixed populations is relatively evenly divided. Many patents have also been filed in which microbial biomass forms a component of a feed mixture which is intended to provide protein and other nutrients to fish or farmed animals. These have not been included in Tables 5–8, since it is not clear how much protein is provided by the microbe and how much by other components such as soy, bean, or fish meal.
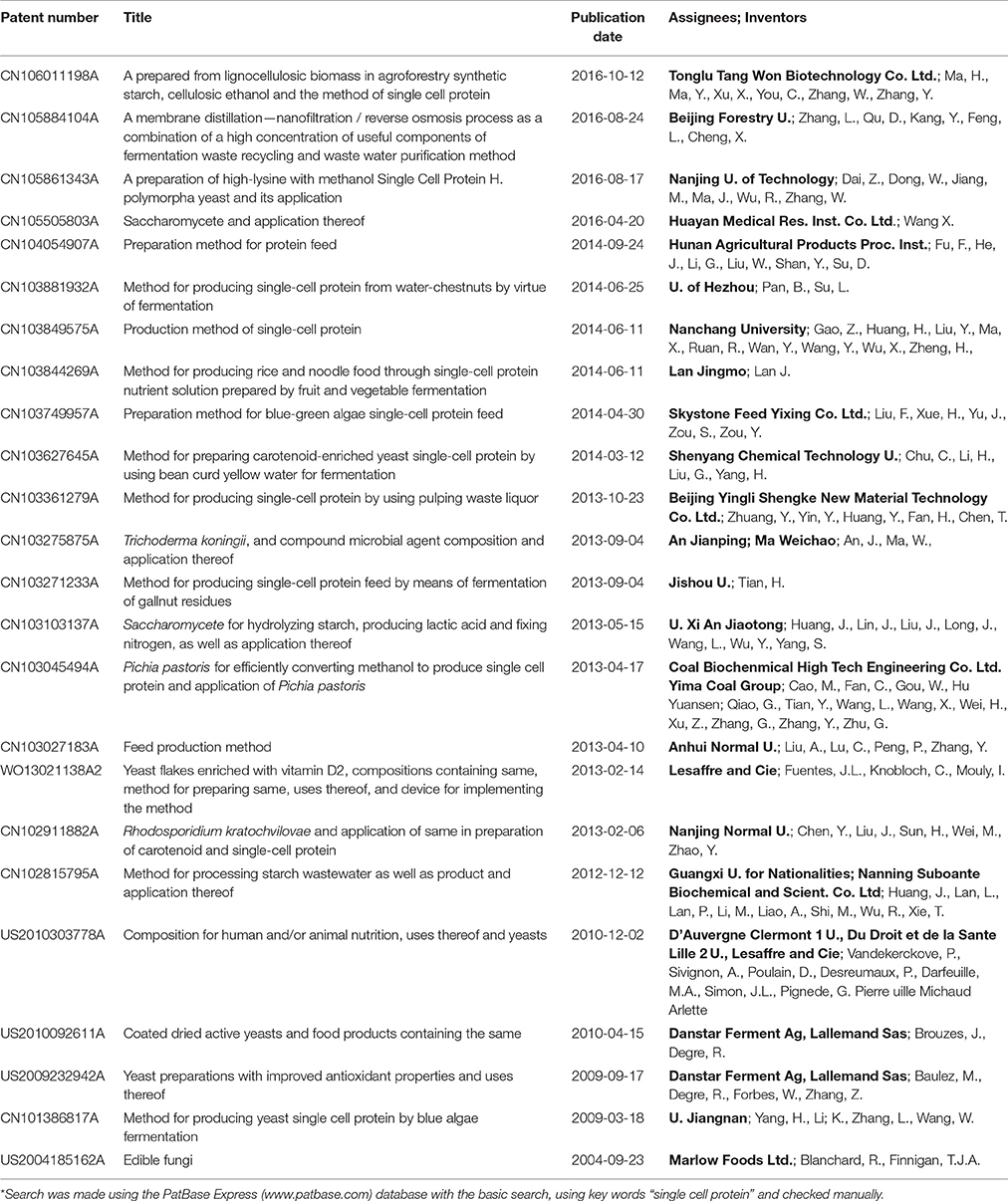
Table 6. Patents related to the production of SCP from yeast or filamentous fungi during 2001–2016*.
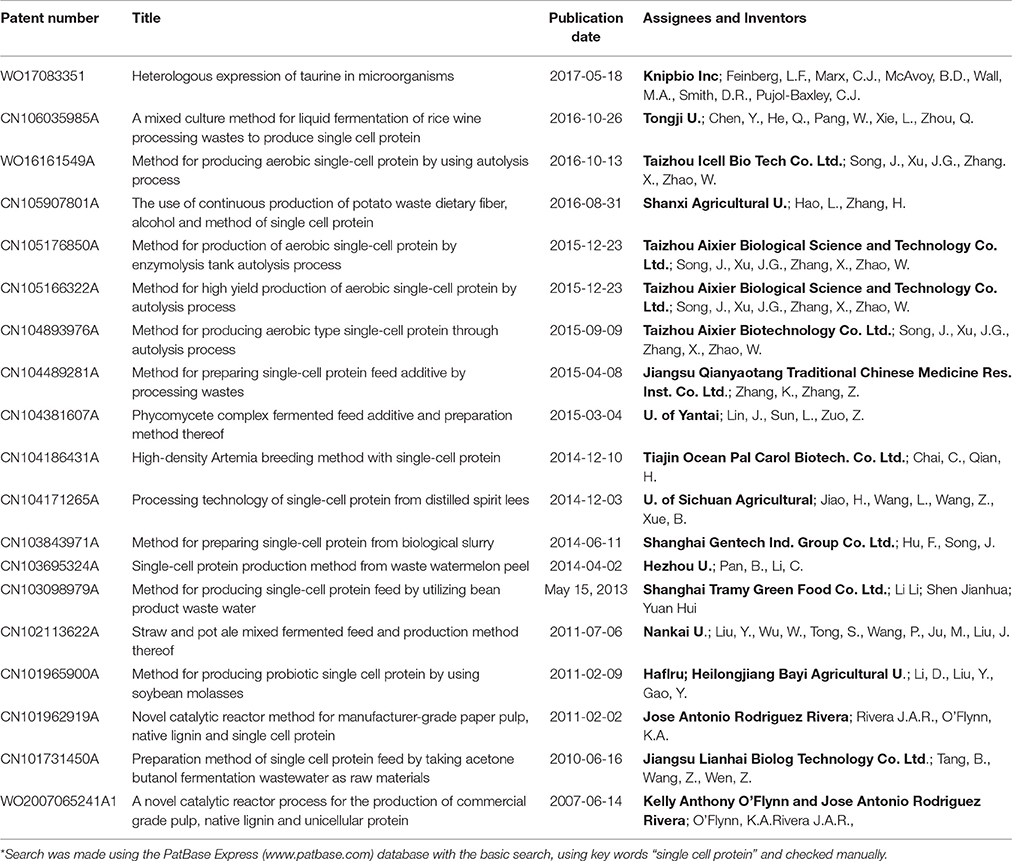
Table 8. Patents related to the production of SCP from mixed microbial populations (bacteria and/or yeast and/or algae) or in which the microorganism was not specified during 2001–2016*.
Industries and universities in China have been particularly active in filing patents related to SCP in recent years, with about 70% of patents awarded since 2001 having been filed in China. In China, there has been a strong emphasis on the production of SCP by fermenting agricultural or food residues with bacteria, yeast and mixed populations. SCP production is thus often combined with bioremediation and waste processing.
Several important patents related to the use of C1 compounds such as methanol and methane were filed before 2001 and have not been included in this review. However, there were two new patents on the production of SCP from methanol and six on producing SCP from methane (Table 7). SCP from algae also continues to generate patents, with formulation of products attracting attention as well as continued developments in the cultivation methods (Table 5).
Concluding Remarks
As seen in Table 4, there are a wide range of industries involved in SCP production, some producing SCP as a by-product of other processes, and others which focus primarily on SCP. SCP from filamentous fungi and yeast continues to dominate the established markets, particularly when considering SCP for human consumption. Yeast SCPs have a long history of use, which facilitates their continued acceptance in the market. SCP for humans from filamentous fungi, however, is likely to remain restricted to F. venenatum (Quorn™) and solid-state fermentations with other food fungi, because of the risk of mycotoxins and the long path to regulatory acceptance. Yeast also have a long history of use as supplements to the feed industry. Much of the fungal SCP provided for animal feed is a byproduct of the food and beverage industries and of biorefineries, in which the fungus first acts as the biocatalyst to create the main product and then provides protein-enriched residues which are sold as feed. Fungal SCPs offer the advantages of familiarity, with well-established processing approaches, and availability. The main barrier is in the introduction of SCP from new species, which generate academic and patent interest, but which are difficult to bring into the market.
Algae also have well-established markets for both food and feed applications, although these are not traditionally focused on algae as SCP, but rather as food supplements providing omega-3 fatty acids, carotenoids and vitamins, with protein as a corollary benefit. Since products have been treated as supplements or colorants, the regulatory requirements are different than those for direct food or feed use, facilitating the introduction of new species for potential products. Algal products typically have flavours which may limit the amount a person would want to consume, reducing the need for extensive processing to reduce RNA, but also limiting the amount of protein provided to the diet. However, several of the young SMEs which have entered the market are developing processes to produce low-flavour products which could expand the contribution of algal protein in human diets. Algal SCP offers the advantages of providing healthy lipids along with the protein, while potentially consuming CO2. It has the benefit of being seen as environmentally friendly and very “green.” The main barriers are cost of production and the need for novel formulation to make it acceptable for humans. Algal SCP is likely to be strong in the feed industry, if the production costs can be reduced.
Bacterial SCP is primarily restricted to the feed industry, if not including cyanobacterial products with non-photosynthetic bacteria. Some bacterial SCP is currently a by-product of other industries such as monosodium glutamate production, and this type of feed product is expected to increase with the expansion of biorefineries, as with yeast. However, the most interesting current bacterial SCP developments relate to the use of methane as a carbon source. Although, the use of methane to produce bacterial biomass is not new, the drivers pushing developments have shifted from methane as a cheap carbon source to bacteria as a means of reducing green-house-gas emissions and the potential integration of feed production with animal farming. The low solubility of methane, coupled with low growth rates of the bacteria, poses a strong barrier to success in this area. However, young SMEs like UniBio and Calysta Inc. believe that the barriers can be overcome. Bacterial SCP, other than from methane, offers advantages in high production rates, but is disadvantaged by low familiarity and high nucleic acid content which adds to the processing costs.
SCP initially gained importance in human nutrition during times of war, when traditional sources of protein became scarce. It again became of interest during the latter half of the twentieth century because of concern about meeting the protein demands of the world's ever increasing population. These concerns were global, but when we consider current interest in SCP, we observe that the countries now driving research and development of new SCP are generally those with large populations (e.g., China and India) and problems with malnutrition. Most recent patents related to SCP have been filed from China, indicating the importance of SCP for future food and feed there. Fast growth of SCP products can be expected in China and perhaps in the whole of Asia. Development of algal SCP forms an exception to this observation—since many companies have been established around the world in recent years to develop products which can exploit the current excess availability of CO2. The drivers for development of algal SCP are thus somewhat different from those for the development of bacterial and fungal SCP. Production of SCP from methane shares this environmental concern and opportunity with the algal developments.
An increase in biorefinery processes, as part of the expansion of the bio-economy and circular economy concepts, also acts as a driver for the development of SCPs for use as animal feed, since conversion of waste material to animal feed offers better returns on investment than burning residual microbial biomass or utilising it as fertiliser. Regulatory clearance is still needed for use of novel products in animal feed, but this differs from that needed for human food use, and a wider range of substrates are considered acceptable when the product is intended for animal use. Thus, greater expansion in available SCPs for animal feed than for human food can be expected. None-the-less, there is a growing appreciation of the inefficiency of converting plant biomass into SCP which is fed to animals, rather than directly to humans, which will push development of safe SCP as food also.
In the west, interest in healthy diets and novelty food is helping to drive a new interest in SCP, while also blurring the edges of what products might be included in SCP. Cell cultures of both plant and animal cells may contribute to food supply in the future (Poutanen et al., 2017), but do not conform to the definition of SCP as being derived from microbial cells. In addition, the forms in which SCP may be consumed are continuing to evolve. Yeast SCP has been consumed for decades as a cell extract in the form of pastes which can be spread on bread, whereas the fungal SCP which is used in Quorn™ was deliberately developed as a product which could be formulated into chunks and slices which would more closely resemble meat. More recently developed products are often formulated as dry powders or flours, which are intended to be mixed with other ingredients to create products in which the individual components are not perceived. Such products are suitable for incorporation into protein bars and beverages such as smoothies, which are currently popular. Additionally, solid state fermentations continue to be developed which use microbes to upgrade the protein quality and palatability of low nutrient plant products or ingredients. These are not strictly speaking SCP, since both the microbe and the original substrate contribute to the final product, but they will also contribute to the protein supply of the future. Having a broad range of food products which incorporate SCP should encourage further expansion of the market.
Author Contributions
AR, SH, and MT contributed equally to the researching and writing of this article. MW provided information on algal and fungal SCP, contributed to writing the article and reviewed and edited the manuscript.
Funding
The authors thank VTT Ltd. for financial support.
Conflict of Interest Statement
The authors are employees of VTT Technical Research Centre of Finland Ltd. and declare that the research was conducted in the absence of any commercial or financial relationships that could be construed as a potential conflict of interest. MW received financial support from Marlow Foods in 1986-1989 and worked in projects supported by Marlow Foods from 1989 to 1994, but has had no ongoing collaboration with them.
The other authors declare that the research was conducted in the absence of any commercial or financial relationships that could be construed as a potential conflict of interest.
Acknowledgments
The authors thank Drs. Johanna Buchert, Laura Ruohonen, and Tiina Nakari-Setälä for requesting the review and encouraging its publication.
Abbreviations
DSP, Downstream processing; GMO, Genetically modified organism; GRAS, Generally recognised as safe; SCP, Single cell protein; QPS, Qualified presumption of safety of micro-organisms in food and feed applications.
References
Aggelopoulos, T., Katsieris, K., Bekatorou, A., Pandey, A., Banat, I. M., and Koutinas, A. A. (2014). Solid state fermentation of food waste mixtures for single cell protein, aroma volatiles and fat production. Food Chem. 145, 710–716. doi: 10.1016/j.foodchem.2013.07.105
Ahmadi, A. R., Ghoorchian, H., Hajihosaini, R., and Khanifar, J. (2010). Detremination of the amount of protein and amino acids extracted from the microbial protein (SCP) of lignocellulosic wastes. Pakistan J. Biol. Sci. 13, 355–361. doi: 10.3923/pjbs.2010.355.361
Anbuselvi, A., Mahalanobis, S., and Jha, M. (2014). Optimization of single-cell protein using green gram husk and Bengal gram husk using yeast. Int. J. Pharm. Sci. Rev. Res. 28, 188–190.
Anderson, C., and Solomons, G. L. (1984). “Primary metabolism and biomass production from Fusarium,” in The Applied Mycology of Fusarium, eds M. O. Moss and J. E. Smith (Cambridge: Cambridge University Press), 231–250.
Anderson, C., Longton, J., Maddix, C., Scammell, G. W., and Solomons, G. L. (1975). “The growth of microfungi on carbohydrates,” in Single-Cell Protein II, eds S. R. Tannenbaum and D. I. C. Wang (Cambridge, MA: MIT Press), 314–329.
Anupama, and Ravindra, P. (2000). Value-added food: single cell protein. Biotechnol. Adv. 18, 459–479. doi: 10.1016/S0734-9750(00)00045-8
Apandi, N. M., Mohamed, R. M. S. R., Latiffi, N. A. A., Rozlan, N. F. M., and Al-Gheethi, A. A. S. (2017). Protein and lipid content of microalgae Scenedesmus sp. biomass grown in wet market wastewater. MATEC Web Conf. 103:06011. doi: 10.1051/matecconf/201710306011
Bagchi, D. (2006). Nutraceuticals and functional foods regulations in the United States and around the world. Toxicology 221, 1–3. doi: 10.1016/j.tox.2006.01.001
Baldensperger, J., Le Mer, J., Hannibal, L., and Quinto, P. J. (1985). Solid state fermentation of banana wastes. Biotechnol. Lett. 7, 743–748. doi: 10.1007/BF01032289
Bamberg, J. (2000). British Petroleum and Global Oil 1950-1975: The Challenge of Nationalism. Cambridge: Cambridge University Press.
Bhalla, T. C., and Joshi, M. (1994). Protein enrichment of apple pomace by co-culture of cellulolytic moulds and yeasts. World J. Microbiol. Biotechnol. 10, 116–117. doi: 10.1007/BF00357577
Boland, M. J., Rae, A. N., Vereijken, J. M., Meuwissen, M. P. M., Fischer, A. R. H., van Boekel, M. A. J. S., et al. (2013). The future supply of animal-derived protein for human consumption. Trends Food Sci. Technol. 29, 62–73. doi: 10.1016/j.tifs.2012.07.002
Bourdichon, F., Casaregola, S., Farrokh, C., Frisvad, J. C., Gerds, M. L., Hammes, W. P., et al. (2012). Food fermentations: microorganisms with technological beneficial use. Int. J. Food Microbiol. 154, 87–97. doi: 10.1016/j.ijfoodmicro.2011.12.030
Chiou, P. W. S., Chiu, S. W., and Chen, C. R. (2001). Value of Aspergillus niger fermentation product as a dietary ingredient for broiler chickens. Animal Feed Sci. Technol. 91, 171–182. doi: 10.1016/S0377-8401(01)00224-3
Coppens, P., da Silva, M. F., and Pettman, S. (2006). European regulations on nutraceuticals, dietary supplements and functional foods: a framework based on safety. Toxicology 221, 59–74. doi: 10.1016/j.tox.2005.12.022
Cui, W., Wang, Q., Zhang, F., Zhang, S.-C., Chi, Z.-M., and Madzak, C. (2011). Direct conversion of inulin into single cell protein by the engineered Yarrowia lipolytica carrying inulinase gene. Process Biochem. 46, 1442–1448. doi: 10.1016/j.procbio.2011.03.017
De Gregorio, A., Mandalari, G., Arena, N., Nucita, F., Tripodo, M. M., and Lo Curto, R. B. (2002). SCP and crude pectinase production by slurry-state fermentation of lemon pulps. Bioresour. Technol. 83, 89–94. doi: 10.1016/S0960-8524(01)00209-7
De Oliveira, M. A. C. L., Monteiro, M. P. C., Robbs, P. G., and Leite, S. G. F. (1999). Growth and chemical composition of Spirulina maxima and Spirulina platensis biomass at different temperatures. Aquacult. Int. 7, 261–275. doi: 10.1023/A:1009233230706
Duarte, L. C., Carvalheiro, F., Lopes, S., Neves, I., and Gírio, F. M. (2008). Yeast biomass production in brewery's spent grains hemicellulosic hydrolysate. Appl. Biochem. Biotechnol. 148, 119–129. doi: 10.1007/s12010-007-8046-6
Duong, V. T., Ahmed, F., Thomas-Hall, S. R., Quigley, S., Nowak, E., and Schenk, P. M. (2015). High protein- and high lipid-producing microalgae from northern Australia as potential feedstock for animal feed and biodiesel. Front. Bioeng. Biotechnol. 3:53. doi: 10.3389/fbioe.2015.00053
Edelman, J., Fewell, A., and Solomons, G. L. (1983). Myco-protein – a new food. Nutr. Abstr. Rev. Clin. Nutr. 53, 471–480.
Enzing, C., Ploeg, M., Barbosa, M., and Sijtsma, L. (2014). Microalgae-Based Products for the Food and Feed Sector: An Outlook for Europe. JRC scientific and policy reports (JRC85709), eds M. Vigani, C. Parisi, and E. Rodríguez-Cerezo (Publications Office of the European Union).
Erdman, M. D., Bergen, W. G., and Reddy, C. A. (1977). Amino acid profiles and presumptive nutritional assessment of single-cell protein from certain Lactobacilli. Appl. Environ. Microbiol. 33, 901–905.
Gao, H., and Xu, J. G. (2015). Method for Measuring Total Nucleotide in Protein Products. Patent No. CN104316621A. State Intellectual Property Office of the People's Republic of China.
Gao, Y., Ki, D., and Liu, Y. (2012). Production of single cell protein from soy molasses using Candida tropicalis. Ann. Microbiol. 62, 1165–1172. doi: 10.1007/s13213-011-0356-9
Ghasemi, Y., Rasoul-Amini, S., and Morowvat, M. H. (2011). “Algae for the production of SCP,” in Biochemistry Research trends. Bioprocess Sciences and Technology, ed. M.-T. Liong (New York: Hauppage) 163–184.
Goldberg, I. (1988). Future prospects of genetically engineered single cell protein. Trends Biotechnol. 6, 32–34. doi: 10.1016/0167-7799(88)90030-3
Gouveia, L., Batista, A. B., Sousa, I., Raymundo, A., and Bandarra, N. M. (2008). “Microalgae in novel food products,” in Food Chemistry Research Developments, ed. K.N. Papadopoulos (New York, NY: Nova Science Publishers), 75–111.
Groenewald, M., Boekhout, T., Neuvéglise, C., Gaillardin, C., van Dijck, P. W. M., and Wyss, M. (2014). Yarrowia lipolytica: safety assessment of an oleaginous yeast with a great industrial potential. Crit. rev. Microbiol. 40, 187–206. doi: 10.3109/1040841X.2013.770386
Hameş, E. E., and Demir, T. (2015). Microbial ribonucleases (RNases): production and application potential. World J. Microbiol. Biotechnol. 31, 1853–1862. doi: 10.1007/s11274-015-1945-8
Harun, R., Singh, M., Forde, G. M., and Danquah, M. K. (2010). Bioprocess engineering of microalgae to produce a variety of consumer products. Renew. Sust. Energy Rev. 14, 1037–1047. doi: 10.1016/j.rser.2009.11.004
Hashem, M., Hesham, A. E.-L., Alamri, S. A., and Alrumman, S. A. (2014). Production of single-cell protein from wasted date fruits by Hanseniaspora uvarum KKUY-0084 and Zygosaccharomyces rouxii KKUY-0157. Ann. Microbiol. 64, 1505–1511. doi: 10.1007/s13213-013-0793-8
Henrikson, R. (June 6, 2013). Scalable algae microfarms, Part 5. Algae Industry Magazine.com. Available online at: http://www.algaeindustrymagazine.com/scalable-algae-microfarms-part-5/
Hitzman, D. O. (1986). “The Provesteen process – an ultra-high density fermentation,” in Microbial Biomass Proteins, eds. M. Moo-Young and K. F. Gregory (London: Elsevier Applied Science), 27–32.
Jalasutram, V., Kataram, S., Gandu, B., and Anupoju, G. R. (2013). Single cell protein production from digested and undigested poultry litter by Candida utilis: optimization of process parameters using response surface methodology. Clean Technol. Environ. Policy 15, 265–273. doi: 10.1007/s10098-012-0504-3
Johnson, E. A. (2013). Biotechnology of non-Saccharomyces yeasts – the ascomycetes. Appl. Microbiol. Biotechnol. 97, 503–517. doi: 10.1007/s00253-012-4497-y
Kadim, I. T., Mahgoub, O., Baqir, S., Faye, B., and Purchas, R. (2015). Cultured meat from muscle stem cells: a review of challenges and prospects. J. Integr. Agric. 14, 222–233. doi: 10.1016/S2095-3119(14)60881-9
Kam, S., Abedian Kenari, A., and Younesi, H. (2012). Production of single cell protein in stickwater by Lactobacillus acidophilus and Aspergillus niger. J. Aquat. Food Prod. Technol. 21, 403–417. doi: 10.1080/10498850.2011.605539
Kihlberg, R. (1972). The Microbe as a source of food. Ann. Rev. Microbiol. 26, 427–466. doi: 10.1146/annurev.mi.26.100172.002235
Knight, N., Roberts, G., and Shelton, D. (2001). The thermal stability of QuornTM pieces. Int. J. Food Sci. Technol. 36, 47–52. doi: 10.1046/j.1365-2621.2001.00424.x
Koivurinta, J., Kurkela, R., and Koivistoinen, P. (1979). Uses of Pekilo, a microfungus biomass from Paecilomyces varioti in sausage and meat balls. Int. J Food Sci. Technol. 14, 561–570.
Kornochalert, N., Kantachote, D., Chaiprapat, S., and Techkarnjanaruk, S. (2014). Use of Rhodopseudomonas palustris P1 stimulated growth by fermented pineapple extract to treat latex rubber sheet wastewater to obtain single cell protein. Ann. Microbiol. 64, 1021–1032. doi: 10.1007/s13213-013-0739-1
Kunasundari, B., Murugaiyah, V., Kaur, G., Maurer, F. H. J., and Kumar, S. (2013). Revisiting the single cell protein application of Cupriavidus necator H16 and recovering bioplastic granules simultaneously. PLoS ONE 8:e78528. doi: 10.1371/journal.pone.0078528
Kurbanoglu, E. B., and Algur, O. F. (2002). Single-cell protein production from ram horn hydrolysate by bacteria. Bioresour. Technol. 85, 125–129. doi: 10.1016/S0960-8524(02)00094-9
Lang, V., Bellisle, F., Alamowitch, C., Craplet, C., Bornet, F. R. J., Slama, G., et al. (1999). Varying the protein source in mixed meal modifies glucose, insulin and glucagon kinetics in healthy men, has weak effects on subjective satiety and fails to affect food intake. Eur. J. Clin. Nutr. 53, 959–965. doi: 10.1038/sj.ejcn.1600881
Lee, J. Z., Logan, A., Terry, S., and Spear, J. R. (2015). Microbial response to single-cell protein production and brewery wastewater treatment. Microbial Biotechnol. 8, 65–76. doi: 10.1111/1751-7915.12128
Li, C., Yang, H., Li, Y., Cheng, L., Zhang, M., Zhang, L., et al. (2013). Novel bioconversions of municipal effluent and CO2 into protein riched Chlorella vulgaris biomass. Bioresour. Technol. 132, 171–177. doi: 10.1016/j.biortech.2012.12.017
Liu, B., Li, Y., Song, J., Zhang, L., Dong, J., and Yang, Q. (2014). Production of single-cell protein with two-step fermentation for treatment of potato starch processing waste. Cellulose 21, 3637–3645. doi: 10.1007/s10570-014-0400-6
Liu, B., Song, J., Li, Y., Niu, J., Wang, Z., and Yang, Q. (2013). Towards industrially feasible treatment of potato starch processing waste by mixed cultures. Appl. Biochem. Biotechnol. 171, 1001–1010. doi: 10.1007/s12010-013-0401-1
Mahmoud, R., Ibrahim, M., and Ali, G. (2016). Closed photobioreactor for microalgae biomass production under indoor growth conditions. J. Algal Biomass Utln. 7, 86–92.
Martinez, M. C., Sanchez-Montero, J. M., Sinisterra, J. V., and Ballesteros, A. (1990). New insolubilized derivatives of ribonuclease and endonuclease for elimination of nucleic acids in single cell protein concentrates. Biotechnol. Appl. Biochem. 12, 643–652.
Muller-Feuga, A. (2000). The role of microalgae in aquaculture: situation and trends. J. Appl. Phycol. 12, 527–534. doi: 10.1023/A:1008106304417
Nasseri, A. T., Rasoul-Amini, S., Morowvat, M. H., and Ghasemi, Y. (2011). Single cell protein: production and process. Am. J. Food Technol. 6, 103–116. doi: 10.3923/ajft.2011.103.116
Øverland, M., Tauson, A. H., Shearer, K., and Skrede, A. (2010). Evaluation of methane-utilising bacteria products as feed ingredients for monogastric animals. Arch. Anim. Nutr. 64, 171–189. doi: 10.1080/17450391003691534
Paraskevopoulou, A., Athanasiadis, I., Kanellaki, M., Bekatourou, A., Blekas, G., and Kiosseoglou, V. (2003). Functional properties of sigle cell protein produced by kefir microflora. Food Res. Int. 36, 431–438. doi: 10.1016/S0963-9969(02)00176-X
Pessoa, A. Jr., Mancilha, I. M., and Sato, S. (1996). Cultivation of Candida tropicalis in sugar cane hemicellulosic hydrolyzate for microbial protein production. J. Biotechnol. 51, 83–88. doi: 10.1016/0168-1656(96)01572-6
Petersen, L. A. H., Villadsen, J., Jørgensen, S. B., and Gernaey, K. V. (2017). Mixing and mass transfer in a pilot scale U-loop bioreactor. Biotechnol. Bioeng. 114, 344–354. doi: 10.1002/bit.26084
Philippe, F.-X., and Nicks, B. (2015). Review on greenhouse gas emissions from pig houses: production of carbon dioxide, methane and nitrous oxide by animals and manure. Agric. Ecosyst. Environ. 199, 10–25. doi: 10.1016/j.agee.2014.08.015
Poutanen, K., Nordlund, E., Paasi, J., Vehmas, K., and Åkerman, M. (2017). Food Economy 4.0: VTT's Vision of An Era of Smart Consumer-Centric Food Production. Helsinki: VTT Technical Research Centre of Finland Ltd. Available online at: http://www.vtt.fi/inf/pdf/visions/2017/V10.pdf) VTT VISIONS 10
Rafiqul, I. M., Jalal, K. C. A., and Alam, M. Z. (2005). Environmental factors for optimisation of spirulina biomass in laboratory culture. Biotechnology 4, 19–22. doi: 10.3923/biotech.2005.19.22
Rao, M., Varma, A. J., and Deshmukh, S. S. (2010). Production of single cell protein, essential amino acids, and xylanase by Penicillium janthinellum. BioResource 5, 2470–2477.
Rashad, M. M., Moharib, S. A., and Jwanny, E. W. (1990). Yeast conversion of mango waste or methanol to single cell protein and other metabolites. Biol. Wastes 32, 277–284. doi: 10.1016/0269-7483(90)90059-2
Ravinder, R., Venkateshwar Rao, L., and Ravindra, P. (2003). Studies on Aspergillus oryzae mutants for the production of single cell proteins from deoiled rice bran. Food Technol. Biotechnol. 41, 243–246.
Ren, X., Wang, J., Yu, H., Peng, C., Hu, J., Ruan, Z., et al. (2016). Anaerobic and sequential aerobic production of high-titer ethanol and single cell protein from NaOH-pretreated corn stover by a genome shuffling-modified Saccharomyces cerevisiae strain. Bioresour. Technol. 218, 623–630. doi: 10.1016/j.biortech.2016.06.118
Rhishipal, R., and Philip, R. (1998). Selection of marine yeasts for the generation of single cell protein from prawn-shell waste. Bioresource Technol. 65, 255–256. doi: 10.1016/S0960-8524(97)00179-X
Rodriguez, J., Ferraz, A., Nogueira, R. F. P., Ferrer, I., Esposito, E., and Duran, N. (1997). Lignin biodegradation by the ascomycete Chrysondia sitophila. Appl. Biochem. Biotechnol. 62, 233–242. doi: 10.1007/BF02787999
Rodríguez-Zavala, J. S., Ortiz-Cruz, M. A., Mendoza-Hernández, G., and Moreno-Sánchez, R. (2010). Increased synthesis of a-tocopherol, paramylon and tyrosine by Euglena gracilis under conditions of high biomass production. J. Appl. Microbiol. 109, 2160–2172. doi: 10.1111/j.1365-2672.2010.04848.x
Rudravaram, R., Chandel, A. K., Rao, L. V., Hui, Y. Z., and Ravindra, P. (2009). “Bio (Single Cell) protein: issues of production, toxins and commercialisation status,” in Agricultural wastes, eds G. S. Ashworth and P. Azevedo (New York, NY: Hauppage), 129–153.
Safafar, H., Nørregaard, P. U., Ljubic, A., Møller, P., Holdt, S. L., and Jacobsen, C. (2016). Enhancement of protein and pigment content in two Chlorella species cultivated on industrial process water. J. Mar. Sci. Eng. 4:84. doi: 10.3390/jmse4040084
Schulz, E., and Oslage, H. J. (1976). Composition and nutritive value of single-cell protein (SCP). Anim. Feed Sci. Technol. 1, 9–24. doi: 10.1016/0377-8401(76)90003-1
Sinskey, A. J., and Tannenbaum, S. R. (1975). “Removal of nucleic acids in SCP” in Single-Cell Protein II, eds S. R. Tannenbaum and D. I. C. Wang (Cambridge, MA: MIT Press), 158–178.
Sisman, T., Özlem, G., Dogan, N., Özdal, M., Algur, Ö. F., and Ergon, T. (2013). Single-cell protein as an alternative food for zebrafish, Danio rerio: a toxicological assessment. Toxicol. Ind. Health 29, 792–799. doi: 10.1177/0748233712442711
Smedley, K. O. (2013). Comparison of Regulatory Management of Authorized Ingredients, Approval Processes, and Risk-Assessment Procedures for Feed Ingredients. Jurisdictions Covered: Brazil, Canada, China, European Union, Japan, South Africa, and United States. International Feed Industry Federation, July 12, 2013.
Solomons, G. L. (1986). “Microbial proteins and regulatory clearance for RHM myco-protein,” in Microbial Biomass Proteins, eds M. Moo-Young and K. F. Gregory (London: Elsevier Applied Science), 19–26.
Song, H. T., Yang, Y. M., Liu, D. K., Xu, X. Q., Xiao, W. J., Liu, Z. L., et al. (2017). Construction of recombinant Yarrowia lipolytica and its application in bio-transformation of lignocellulose. Bioengineered 17, 1–6. doi: 10.1080/21655979.2017.1293219
Steinmann, J., Wottge, H. U., and Müller-Ruchholtz, W. (1990). Immunogenicity testing of food proteins: in vitro and in vivo trials in rats. Int. Arch. Allergy Immunol. 91, 62–65. doi: 10.1159/000235091
Strong, P. J., Xie, S., and Clarke, W. P. (2015). Methane as a resource: can the methanotrophs add value? Environ. Sci. Technol. 49, 4001–4018. doi: 10.1021/es504242n
Suzuki, K. (2017). Large-scale cultivation of euglena. Adv. Exp. Med. Biol. 979, 285–293. doi: 10.1007/978-3-319-54910-1_14
Taran, M., and Asadi, N. (2014). A novel approach for environmentally friendly production of single cell protein from petrochemical wastewater using a halophilic microorganism in different conditions. Petroleum Sci. Technol. 32, 625–630. doi: 10.1080/10916466.2011.596888
Trevelyan, W. E. (1976). Chemical methods for the reduction of the purine content of the baker's yeast, a form of single cell protein. J. Sci. Food Agric. 27, 225–230. doi: 10.1002/jsfa.2740270305
Turnbull, W. H., Leeds, A. R., and Edwards, G. D. (1992). Mycoprotein reduces blood lipids in free-living subjects. Am. J. Clin. Nutr. 55, 415–419.
Ugalde, U., and Castrillo, J. I. (2002). “Single cell proteins from yeast and fungi,” in Applied Mycology and Biotechnology, Vol II Agriculture and Food Production 2002, eds G. G. Khachatourians and D. K. Arora (Amsterdam: Elsevier Science B.V.) 123–149.
Valentino, M. J. G., Ganado, L. S., and Undan, J. R. (2016). Single cell protein potential of endophytic fungi associated with bamboo using rice bran as substrate. Adv. Appl. Sci. Res. 7, 68–72.
Vigani, M., Parisi, C., Rodríguez-Cerezo, E., Barbosa, M. J., Sijtsma, L., Ploeg, M., and Enzing, C. (2015). Food and feed products from micro-algae: market opportunities and challenges for the EU. Trends Food Sci. Technol. 42, 81–92. doi: 10.1016/j.tifs.2014.12.004
Viikari, L., and Linko, M. (1977). Reduction of nucleic acid content of SCP. Process Biochem. 12, 35.
Waghmare, A. G., Salve, M. K., LeBlanc, J. G., and Arya, S. S. (2016). Concentration and characterization of microalgae proteins from Chlorella pyrenoidosa. Bioresour. Bioprocess. 3, 16. doi: 10.1186/s40643-016-0094-8
Wang, J. P., Kim, J. D., Kim, J. E., and Kim, I. H. (2013). Amino acid digestibility of single cell protein from Corynebacterium ammoniagenes in growing pigs. Anim. Feed Sci. Technol. 180, 111–114. doi: 10.1016/j.anifeedsci.2012.12.006
Ward, P. N. (1998). Production of Food. U.S. Patent No. 5739030. Washington, DC: U.S. Patent and Trademark Office.
White, J. S., Yohannan, B. K., and Walker, G. M. (2008). Bioconversion of brewer's spent grains to bioethanol. FEMS Yeast Res. 8, 1175–1184. doi: 10.1111/j.1567-1364.2008.00390.x
WHO (2015). Avaiable online at: http://www.who.int/nutrition/topics/3_foodconsumption/en/index4.html
WHO (2017). Avaiable online at: http://www.who.int/mediacentre/factsheets/food-additives/en/
Wiebe, M. G. (2002). Myco-protein from Fusarium venenatum: a well-established product for human consumption. Appl. Microbiol. Biotechnol. 58, 421–427. doi: 10.1007/s00253-002-0931-x
Wiebe, M. G. (2004). QuornTM Myco-protein – Overview of a successful fungal product. Mycologist 18, 17–20. doi: 10.1017/S0269915X04001089
Willetts, A., and Ugalde, U. (1987). The production of single-cell protein from whey. Biotechnol. Lett. 9, 795–800. doi: 10.1007/BF01028286
Wongputtisin, P., Khanongnuch, C., Khongbantad, W., Niamsup, P., and Lumyong, S. (2012). Screening and selection of Bacillus spp. for fermented corticated soybean meal production. J. Appl. Micobiol. 113, 798–806. doi: 10.1111/j.1365-2672.2012.05395.x
Wongputtisin, P., Khanongnuch, C., Kongbuntad, W., Niamsup, P., Lumyong, S., and Sarkar, P. K. (2014). Use of Bacillus subtilis isolates from Tua-nao towards nutritional improvement of soya bean hull for monogastric feed application. Lett. Appl. Microbiol. 59, 328–333. doi: 10.1111/lam.12279
Wu, G. (2009). Amino acids: metabolism, functions, and nutrition. Amino Acids 37, 1–17. doi: 10.1007/s00726-009-0269-0
Xie, D., Jackson, E. N., and Zhu, Q. (2015). Sustainable source of omega-3 eicosapentaenoic acid from metabolically engineered Yarrowia lipolytica: from fundamental research to commercial production. Appl. Microbiol. Biotechnol. 99, 1599–1610. doi: 10.1007/s00253-014-6318-y
Xu, J. G. (2015). Method for Evaluating Destroy Degree on Nucleotide of Determination Method and Production Technology of Free Nucleic Acid Hydrolysate in Protein Product. Patent No. CN104515817A. State Intellectual Property Office of the People's Republic of China.
Yadav, J. S. S., Bezawada, J., Ajila, C. M., Yan, S., Tyagi, R. D., and Surampalli, R. Y. (2014). Mixed culture of Kluyveromyces marxianus and Candida krusei for single-cell protein production and organic load removal from whey. Bioresour. Technol. 164, 119–127. doi: 10.1016/j.biortech.2014.04.069
Yadav, J. S. S., Yan, S., Ajila, C. M., Bezawada, J., Tyagi, R. D., and Surampalli, R. Y. (2016). Food-grade single-cell protein production, characterization and ultrafiltration recovery of residual fermented whey proteins from whey. Food Bioprod. Process 99, 156–165. doi: 10.1016/j.fbp.2016.04.012
Yazdian, F., Hajizadeh, S., Shojaosadati, S. A., Khalilzadeh, R., Jahanshahi, M., and Nosrati, M. (2005). Production of single cell protein from natural gas: Parameter optimization and RNA evaluation. Iranian J. Biotech. 3, 235–242.
Zepka, L. Q., Jacob-Lopez, E., Goldbeck, R., Souza-Soares, L. A., and Queiroz, M. I. (2010). Nutritional evaluation of single-cell protein produced by Aphanothece microscopica Nägeli. Bioresour. Technol. 101, 7107–7111. doi: 10.1016/j.biortech.2010.04.001
Zhang, H. Y., Piao, X. S., Li, P., Yi, J. Q., Zhang, Q., Li, Q. Y., et al. (2013). Effects of single cell protein replacing fish meal in diet on growth performance, nutrient digestibility and intestinal morphology in weaned pigs. Asian Australas J. Anim. Sci. 26, 1320–1328. doi: 10.5713/ajas.2013.13200
Zhao, G., Zhang, W., and Zhang, G. (2010). Production of single cell protein using waste capsicum powder produced during capsanthin extraction. Lett. Appl. Microbiol. 50, 187–191. doi: 10.1111/j.1472-765X.2009.02773.x
Zhao, M.-X., Chi, Z., Chi, Z.-M., and Madzak, C. (2013). The simultaneous production of single-cell protein and a recombinant antibacterial peptide by expression of an antibacterial peptide gene in Yarrowia lipolytica. Process Biochem. 48, 212–217. doi: 10.1016/j.procbio.2013.01.003
Zimberoff, L. (2017). Hope You Like Algae, Because It's Going To Be In Everything You Eat. Fast Company 02.14.17. Available online at: https://www.fastcompany.com/3067961/hope-you-like-algae-because-its-going-to-be-in-everything-you-eat (Accessed April 04, 2017).
Keywords: single cell protein, SCP, algae, bacteria, fungi, microbial protein, Quorn™
Citation: Ritala A, Häkkinen ST, Toivari M and Wiebe MG (2017) Single Cell Protein—State-of-the-Art, Industrial Landscape and Patents 2001–2016. Front. Microbiol. 8:2009. doi: 10.3389/fmicb.2017.02009
Received: 03 August 2017; Accepted: 29 September 2017;
Published: 13 October 2017.
Edited by:
Andrea Gomez-Zavaglia, Center for Research and Development in Food Cryotechnology (CIDCA, CONICET), ArgentinaReviewed by:
Hong-Bo Xin, Nanchang University, ChinaKlaas J. Jan Hellingwerf, University of Amsterdam, Netherlands
Copyright © 2017 Ritala, Häkkinen, Toivari and Wiebe. This is an open-access article distributed under the terms of the Creative Commons Attribution License (CC BY). The use, distribution or reproduction in other forums is permitted, provided the original author(s) or licensor are credited and that the original publication in this journal is cited, in accordance with accepted academic practice. No use, distribution or reproduction is permitted which does not comply with these terms.
*Correspondence: Marilyn G. Wiebe, bWFyaWx5bi53aWViZUB2dHQuZmk=