- Department of Medical Microbiology, University Medical Center Groningen, University of Groningen, Groningen, Netherlands
Highly resistant microorganisms (HRMOs) may evade screening strategies used in routine diagnostics. Bacteria that have evolved to evade diagnostic tests may have a selective advantage in the nosocomial environment. Evasion of resistance detection can result from the following mechanisms: low-level expression of resistance genes not resulting in detectable resistance, slow growing variants, mimicry of wild-type-resistance, and resistance mechanisms that are only detected if induced by antibiotic pressure. We reviewed reports on hospital outbreaks in the Netherlands over the past 5 years. Remarkably, many outbreaks including major nation-wide outbreaks were caused by microorganisms able to evade resistance detection by diagnostic screening tests. We describe various examples of diagnostic evasion by several HRMOs and discuss this in a broad and international perspective. The epidemiology of hospital-associated bacteria may strongly be affected by diagnostic screening strategies. This may result in an increasing reservoir of resistance genes in hospital populations that is unnoticed. The resistance elements may horizontally transfer to hosts with systems for high-level expression, resulting in a clinically significant resistance problem. We advise to communicate the identification of HRMOs that evade diagnostics within national and regional networks. Such signaling networks may prevent inter-hospital outbreaks, and allow collaborative development of adapted diagnostic tests.
Introduction
Diagnostic screening provides hospitals a level of immunity to antibiotic resistance. When highly resistant microorganisms (HRMOs) are detected, transmission can be limited by treating the patient with isolation precautions. In addition, the carriage of HRMOs can be suppressed by antibiotic treatment or, in case of methicillin resistant Staphylococcus aureus (MRSA), even be eradicated. If the introduction of HRMOs in hospitals remains undetected, these bacteria can disseminate from patient-to-patient, and the mobile genetic elements carrying resistance genes can horizontally transfer from species-to-species. Thus, the epidemiology of nosocomial resistance may strongly be affected by our diagnostic screening strategies. Moreover, we postulate that evasion of diagnostic resistance screening could be considered as a critical factor for infection of hospitals with antibiotic resistance elements, similar to the concept that immune evasion is a critical factor of pathogens to infect the human host.
The Netherlands is a high-resource country. Surveillance on HRMOs is extensive in Dutch hospitals. For this, the Dutch situation is very proficient to observe effects of diagnostic screening on the characteristics of HRMOs that cause nosocomial outbreaks. The Dutch Society for Medical Microbiology (NVMM) provides guidelines for the detection of the HRMOs (Working group members NVMM, 2012). For the detection of HRMOs such as carbapenemase-producing Enterobacteriaceae (CPE), vancomycin-resistant enterococci (VRE), extended spectrum beta-lactamase (ESBL) – producing bacteria and MRSA, selective broth and/or selective media are used. Nosocomial outbreaks with HRMO are reported to “Hospital Acquired Infection and Antimicrobial Resistance Monitoring Group”, and the reports are communicated to clinical microbiologists. We searched these reports for outbreaks with micro-organisms harboring resistance mechanisms that were able to evade detection by routine diagnostics. In addition, we evaluated data from our hospital, and searched literature for outbreaks to assess the importance of diagnostic evasion. We here present the most explicit examples of CPE, VRE, ESBL-producing bacteria and MRSA outbreaks caused by isolates harboring diagnostic-evasive resistance mechanisms.
Diagnostic Evasion by CPE
In the Netherlands, the national laboratory guideline recommends the following screening strategy for the detection of HRMOs: a screening step, a genotypic confirmation step and an optional phenotypic confirmation step (Cohen Stuart et al., 2010; Working group members NVMM, 2012). According to this guideline, Enterobacteriaceae with an MIC for meropenem ≥ 0.50 mg/L, or imipenem ≥ 2.0 mg/L should be evaluated by molecular tests for carbapenemase gene detection. Optional phenotypic tests, which include the modified Hodge test, and tests based on inhibition of metallo-betalactamases by EDTA, and Class A carbapenemases by phenyl-boronic acid, may be used if genotypic confirmatory tests are not immediately available. Newer tests for non-genotypic detection of CPE include the carba-NP test, carbapenem-inactivation method (CIM-test), and immunochromotographic tests (Nordmann et al., 2012; van der Zwaluw et al., 2015; Dortet et al., 2016; Literacka et al., 2017). Genotypic confirmation comprises PCR and sequence based methods. Next-gen-sequencing facilities are increasingly accessible for routine diagnostic laboratories. This allows whole-genome sequence-based carbapenemase gene detection. In addition, specific primer/probe combinations for unique markers of an outbreak strains may be designed for high-throughput diagnostics to control outbreaks (Deurenberg et al., 2017).
Despite this huge arsenal of CPE-detection methods, CPEs are still able to evade our diagnostic screening strategies. In the Netherlands, an inter-hospital outbreak with OXA-48-producing Enterobacteriaceae from 2009 to 2011 has been reported (Dautzenberg et al., 2014). The outbreak had been uncontrolled for 2 years. The plasmids carrying blaOXA-48 had disseminated to 15 (sub)- species. Predominantly OXA-48-producing Escherichia coli and Klebsiella pneumoniae isolates were detected. Heterogeneity in resistance to carbapenems within, and across the OXA-48-producing species was observed. All OXA-48-producing E. coli isolates had meropenem MICs of <1 mg/L, a concentration that is commonly used in screening plates, whereas the meropenem susceptibility breakpoint for meropenem is 2 mg/L according to EUCAST (EUCAST, 2017). In addition, if the OXA-48 was not co-expressed with an ESBL gene, no hydrolysis of 3th generation cephalosporins was detected in the majority of isolates. These diagnostic stealth-features have undoubtedly contributed to the magnitude of this outbreak.
The emergence and spread of OXA-48 producing CPEs have been reported in several countries in Europe (Grundmann et al., 2017). The outbreaks concerned predominantly K. pneumoniae clones. A successful K. pneumoniae clone carrying OXA-48 is ST11, reported in many countries (Lee et al., 2016), amongst others in Greece (Voulgari et al., 2013), Spain (Oteo et al., 2015), and Belgium (De Laveleye et al., 2017). Other clones associated with OXA-48 are ST14, ST15, ST101, SST147, and ST405 (Liapis et al., 2014; Oteo et al., 2015; Lee et al., 2016). In a Belgian multi-center study, less than 50% of CPEs were carbapenem non-susceptible (De Laveleye et al., 2017).
Given the fact that OXA-48 is difficulty to detect, there is a need to adapt surveillance strategies to detect CPEs. The EUCAST-guideline advises to screen for CPE if isolates have a MIC to meropenem > 0.12 mg/L (EUCAST, 2017). Unfortunately, widely used automated susceptibility testing (AST) systems do not detect MICs below 0.5 mg/L. The meropenem MIC distribution of OXA-48-producing Enterobacteriaceae, however, shows a peak at MIC = 0.25 mg/L (Fattouh et al., 2015). These isolates will remain undetected if screened by AST only.
When using screening cut-off MICs for CPE detection, which are lower than the susceptibility cut-offs, the sensitivity is still just 80% (Huang et al., 2014). Mainly OXA-48-, and some VIM-producers would remain undetected using meropenem screening cut-offs. Since carbapenem-resistant isolates are usually send to reference centers for CPE detection, this may result in an underestimation of true prevalence numbers (Kaase et al., 2016). In our hospital, we use both culture on screening agars and carbapenemase gene detection directly on rectum samples in patients with a high risk on CPE-carriage to increase the sensitivity of surveillance cultures (Bathoorn et al., 2013). Direct screening of rectal swabs for carbapenemases by real-time PCR performed on enrichment broth showed a higher sensitivity than culturing on selective agar plates (Singh et al., 2012). However, relying on genotypic tests alone may also be a pitfall. For instance, molecular panels for detection of CPE may have a limited number of carbapenemase gene targets. CPEs that are not detected by the panel may have an evolutionary advantage caused by the limitations of this diagnostic method.
Diagnostic Evasion by VRE
A second example of successful diagnostic evasion by HRMOs is the nationwide emergence of nosocomial outbreaks with vancomycin-resistant Enterococci (VRE) in the Netherlands. In the period 2012–2014, 26 outbreaks with VRE have been reported, including reports of local and inter-hospital transmissions (van der Bij et al., 2015). Outbreaks predominantly occurred with VanA- and VanB-type Enterococcus faecium, that confer resistance to glycopeptides. VanB VRE can easily remain undetected by culturing in routine diagnostics. In addition to the fact that fecal VRE carriage often is detected in very low amounts, vancomycin resistance in vanB VRE is not always expressed. These diagnostic challenges have been an important factor in the ongoing transmission of VRE in hospitals in the Netherlands. Several phenotypic screening methods, such as the use of chromogenic agars, have been suggested to identify vanB VRE with varying vancomycin MICs (Klare et al., 2012). However, VRE suspected colonies growing on Chrome-agars may test vancomycin susceptible in routine AST systems despite positive genotypic confirmation of vanB. This could lead to an unnoticed and uncontrolled spread of vanB VRE.
In our hospital, patients are screened on a PCR-based method for VRE on admission at the intensive care unit and if patients are transferred from or recently have been admitted in another hospital in the Netherlands or a foreign hospital. If an unexpected VRE case is found, screening is performed in those patients who are at risk of VRE transmission.
We have reviewed VRE data from 2013 to 2016 in our own hospitals. We searched for all VRE positive patients and selected their first VRE sample. A total of 106 patients were found, all isolates were vanB E. faecium. Of these vanB VREs, 26 isolates (24.5%) were tested vancomycin-susceptible by Vitek2 (bioMérieux) according to the EUCAST susceptibility breakpoint of ≤4 mg/L (European Committee on Antimicrobial Susceptibility Testing, 2014). Vancomycin 5 μg paperdisks (Becton Dickenson) were used to phenotypically detect the resistance mechanism, which showed an hazy edge also in the vanB positive vancomycin-susceptible isolates. Of these 26 isolates, 24 were outbreak related (92.3%). The two non-outbreak related isolates in the vancomycin-susceptible group were found in a patient transferred from another Dutch hospital and in a patient transferred from a foreign hospital. The other 80 isolates (75.5%) were tested resistant to vancomycin. Of these, 65 isolates (81.3%) were outbreak related (Figure 1). The 15 non-outbreak related isolates in this group were from the surveillance cultures of patients transferred from hospitals abroad (n = 1), patients transferred from other Dutch hospitals (n = 2), in patients admitted to the ICU (n = 8), and in clinical samples (n = 4). Noticeably, among these 80 patients with vancomycin-resistant vanB, we also detected vanB positive vancomycin-susceptible E. faecium isolates in follow-up samples from 13 patients. These results are in line with reports in literature. A VRE outbreak in a neonatal ICU in Germany has been reported, in which even 55% of the vanB positive VRE isolates were tested vancomycin susceptible (Werner et al., 2012). These data show the possible pitfalls in detecting vanB VRE in a significant population when only using phenotypic screening tests.
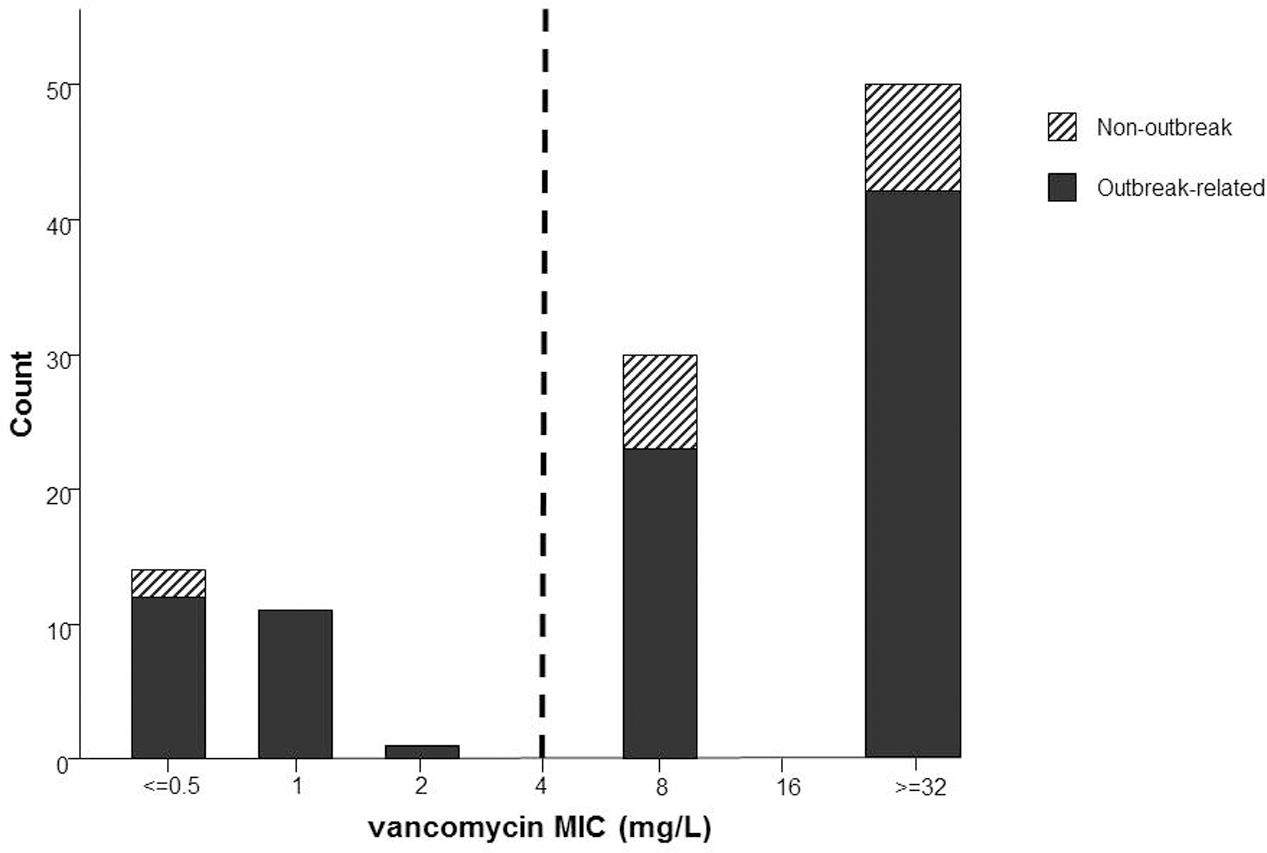
FIGURE 1. Number of first VRE (all vanB Enterococcus faecium) isolates from patients during 2013–2016 and their corresponding MIC values. The dashed line represents the vancomycin susceptibly breakpoint of 4 mg/L.
Pitfalls in detecting vanA VRE have been described due to an altered phenotype of vanA VRE. The expression of teicoplanin resistance can be heterogenous conferring into a VanB phenotype (Park et al., 2008). Moreover, isolates can even test vancomycin susceptible due to a silenced vanA gene which can easily lead to uncontrolled outbreaks (Gagnon et al., 2011; Sivertsen et al., 2016).
In a multicenter study, the EUCAST disk diffusion method performed significantly better than the Vitek2 system for the detection of clinical enterococci isolates with low and medium level vancomycin resistance (Hegstad et al., 2014). For rapid detection of VRE carriage, diagnostic strategies using selective enrichment broths and molecular detection can be used to increase the sensitivity of diagnostic procedures (Zhou et al., 2014). Based on above findings, genotypic testing of invasive vancomycin-susceptible enterococci by PCR can be advised. All three diagnostic strategies are being used in our routine diagnostic laboratory.
Diagnostic Evasion by MRSA
To detect MRSA carriage, the Dutch laboratory guideline recommends to take samples of the throat, nose, and perineum (Working group members NVMM, 2012). Additional body sites should be sampled depending on clinical signs such as wounds, productive cough, skin lesions, or indwelling catheters. To optimize the sensitivity of the cultures, incubation in relatively non-selective enrichment salt-only broths is recommended, followed by culturing for 48 h on selective MRSA screening agars. Additional rapid molecular test are recommended in case of urgency.
In the Netherlands, patients with risk factors for MRSA–carriage such as recent hospitalization abroad, or farm workers at pig farms, cattle farms, or poultry farms are treated upon admission in strict isolation until rapid PCR-based diagnostics are negative. In case of MRSA carriage, patients are treated in isolation and MRSA eradication therapy can be started. This is known as the search and destroy policy (Wertheim et al., 2004). However, PCR-based diagnostics for screening alone would not detect all cases of MRSA-carriage. In a meta-analysis, a sensitivity of 92.5% for the overall pooled PCR estimate has been reported, with a high level of heterogeneity among the studies (Luteijn et al., 2011). PCR-based false negative MRSA results are in our experience usually in patients with a low-level carriage of MRSA. In these cases, culture on chromogenic agar after incubation in broth is more sensitive. In our hospital we use the GeneXpert, an automated PCR-based method to detect MRSA. The lower detection limit for the Xpert MRSA SA nasal assay is about 70 colony forming units (CFUs)/sample according to the manufacturer.
A second reason for failure to detect MRSA is that sporadic Staphylococcal Cassette Chromosome mec (SCCmec)-cassette subtypes, which are a common target in commercial tests, may not be detected by PCR. There is a high diversity in SCCmec-cassettes: already 11 SCCmec-types and numerous subtypes have been designated (Liu et al., 2016). The detection of SCCmec by PCR-based tests is still improving, and the coverage has expanded over the recent years. However, since there may be shifts in common lineages, we should be aware of sporadic nosocomial MRSA that may emerge as successful clones, and are undetectable by commercial tests (Kinnevey et al., 2014). Variety in the mecA/mecC target may also result in failure of MRSA detection by PCR. For instance, MRSAs with the divergent homolog mecA (mecALGA251) would not be detected by the Xpert MRSA assays (Garcia-Alvarez et al., 2011).
False-negative PCR results may have a considerable impact in hospitals. Since patients are discharged from strict isolation after negative PCR results, the isolate has an opportunity to spread until the MRSA is identified by culture and the patient is in strict isolation again. To prevent further spread, contact investigations among patients in the same room and health care workers are performed in these cases in the Netherlands. Since PCR-based detection is not reliable in screening for such isolates, the investigation of contacts has to be performed by culture, which delays the time to detection of secondary transmissions.
Not only PCR-based diagnostics, but also culture-based detection may be evaded by MRSA. In 2014, clinical microbiologists were alerted by a report of the monitoring group on an outbreak with a MRSA strain that could easily be missed by routine diagnostics. Although the numbers of transmissions were largely reduced, total control of the outbreak was difficult due to detection problems using conventional culturing. The mecA-positive isolate was difficult to culture as the oxacillin MIC was low, ranging from 0.5 to 6.0 μg/mL. Growth on ChromIDTM MRSA agar (bioMérieux) plates was strongly inhibited. We tested the outbreak isolate in our own laboratory and found a more then 10-fold decrease in colony numbers if cultured on ChromIDTM MRSA plates compared to blood agar, resulting in a detection limit on ChromIDTM MRSA below 0.5 × 103 colonies/100 μL. Molecular testing and prolonged subculturing in broths was advised to detect this isolate.
Diagnostic Evasion by ESBL
Extended spectrum beta-lactamase-detection can be complicated in natural AmpC-producers such as Citrobacter freundii, Enterobacter spp., Hafnia alvei, Morganella morganii, Serratia spp. and Providencia spp, since it mimicks their natural resistance pattern. Antibiotics can select for mutants with derepressed AmpC expression, resulting in resistance to cephalosporins during therapy. Thus, antibiotic treatment with cephalosporins is not recommended (Livermore et al., 2004). Presence of natural AmpC alone is no condition for HRMO and infection prevention measures.
However, in 2015, several outbreaks in various hospitals in the Netherlands were reported with natural AmpC-producing Enterobacteriaceae that acquired additional ESBL genes. This has no consequences for antibiotic therapy choices, however, infection prevention measures need to be taken.
This combined “AmpC-plus-ESBL” phenotype is difficult to distinguish from derepressed-AmpC wild-type resistance. The Dutch laboratory guideline recommend to use cefotaxim and/or ceftazidim to screen for ESBLs with cut-off MIC values for both cephalosporins of >1 mg/L. This screening strategy is also for Enterobacteriaceae with natural AmpCs. This leads to many false positive results due to derepressed AmpCs. Phenotypic confirmation based on inhibition ESBL activity by clavulanic acid or cefepime hydrolysis by disk diffusion, Etest or broth microdilution methods is recommended.
Natural AmpC-producing Enterobacteriaceae that acquired additional ESBL genes are common in Dutch nosocomial isolates. Citrobacter freundii and Enterobacter cloacae showed the highest percentages of confirmed ESBL co-producers: 3% of Citrobacter freundii (total n = 9.432), and 2% of Enterobacter cloacae (n = 28.027) were recorded by the Dutch national antibiotic resistance surveillance system (ISIS-AR). Microbiologist were explicitly warned for outbreaks with these difficult to detect HRMOs in a report by the monitoring group.
The substantial presence of ESBLs in Enterobacteriaceae with natural AmpCs has been underlined in an Asian study (Choi et al., 2007). The ESBLs confer additional resistance to fourth generation cephalosporins, compared to the natural broad-spectrum AmpCs. These isolate may represent a hidden reservoir of ESBL-carrying plasmids, which can transfer across species. Numerous outbreaks with ESBL natural AmpC producers have been reported in international literature (Mezzatesta et al., 2012). Since resistance to 3th generation cephlosporins is very common in natural AmpC producers that do not carry ESBLs (Jacobson et al., 1995), the dissemination of ESBL-carrying isolates in hospitals may remain unnoticed.
Implications and Future Directions
We observed that highly-resistant microorganisms adapt to evade screening strategies. One can consider this process as a prey that evolves to escape from predators. Microbiologists, in their evolutionary role as predators hunting for HRMOs, also have to keep on innovating to update the detection strategies for these micro-organisms that are trying to evade. This may result in an arms race. In evolutionary biology, such an arms race is known as the Red Queens hypothesis (Castrodeza, 1979). The name of the theory is based on a quote from Lewis Carroll’s Through the Looking-Glass: “Now, here, you see, it takes all the running you can do, to keep in the same place. If you want to get somewhere else, you must run at least twice as fast as that!”
To run twice at fast, communication within networks of health care professionals is crucial. In our perspective, we described examples of how Dutch clinical microbiologist were alarmed by a national monitoring group on successful HRMOs that evade routine screening tests. Specific recommendations to adjust diagnostic strategies to detect these pathogens were provided. Additionally, rapid communication within regional networks is of utmost importance. Inter-hospital patient traffic is highest between hospitals in the same regions. As a consequence, hospitals within the same region are at immediate risk of introduction of HRMOs that evade diagnostics and cause outbreaks. We recommend to identify your region of hospitals that are most connected by patient traffic, and set-up communication networks to alarm for difficult to detect HRMO’s. Experiences and adjusted diagnostic screening tests should be shared within these networks. Such a regional approach has successfully been applied in the control of MRSA in the Dutch-German cross-border region (Jurke et al., 2013).
We should be aware of the impact of our diagnostics on the introduction and dissemination of resistance elements in our hospitals. The Government of the Netherlands has a national and international mission to combat antimicrobial resistance (AMR). Therefore the NVMM has composed a vision document to maintain the low prevalence of CPE in the Netherlands (Kluytmans et al., 2015). By taking CPE as a biological indicator, it is implicitly assumed that other HRMOs will be included in the combat of AMR. To realize the goals, it is of utmost importance that diagnostic methods are continuously innovated and used.
We are aware that optimizing diagnostic screening will increase costs. On the other hand, our examples have shown that failure of detection by routine diagnostics may lead to uncontrolled outbreaks. These outbreaks can lead to enormous financial expenses; costs may rise up to €1,369 per patient per day (Dik et al., 2016). Moreover, detection of HRMO carriage allows for directed antibiotic treatment of patients developing infections by these HRMOs.
Cost reductions in innovation of diagnostics for screening purposes are foretold to result in nosocomial outbreaks with HRMOs evading our screenings methods. We would be outsmarted by prokaryotes.
Author Contributions
XZ and EB wrote this perspective article. AF carefully reviewed it.
Conflict of Interest Statement
The authors declare that the research was conducted in the absence of any commercial or financial relationships that could be construed as a potential conflict of interest.
References
Bathoorn, E., Friedrich, A. W., Zhou, K., Arends, J. P., Borst, D. M., Grundmann, H., et al. (2013). Latent introduction to the Netherlands of multiple antibiotic resistance including NDM-1 after hospitalisation in Egypt, august 2013. Euro Surveil. 18:20610. doi: 10.2807/1560-7917.ES2013.18.42.20610
Castrodeza, C. (1979). Non-progressive evolution, the red queen hypothesis, and the balance of nature. Acta Biotheor. 28, 11–18. doi: 10.1007/BF00054677
Choi, S. H., Lee, J. E., Park, S. J., Kim, M. N., Choo, E. J., Kwak, Y. G., et al. (2007). Prevalence, microbiology, and clinical characteristics of extended-spectrum beta-lactamase-producing Enterobacter spp., Serratia marcescens, Citrobacter freundii, and Morganella morganii in Korea. Eur. J. Clin. Microbiol. Infect. Dis. 26, 557–561. doi: 10.1007/s10096-007-0308-2
Cohen Stuart, J., Leverstein-Van Hall, M. A., and Dutch Working Party on the Detection of Highly Resistant Microorganisms (2010). Guideline for phenotypic screening and confirmation of carbapenemases in Enterobacteriaceae. Int. J. Antimicrob. Agents 36, 205–210. doi: 10.1016/j.ijantimicag.2010.05.014
Dautzenberg, M. J., Ossewaarde, J. M., de Kraker, M. E., van der Zee, A., van Burgh, S., de Greeff, S. C., et al. (2014). Successful control of a hospital-wide outbreak of OXA-48 producing Enterobacteriaceae in the Netherlands, 2009 to 2011. Euro Surveil. 19:20723. doi: 10.2807/1560-7917.ES2014.19.9.20723
De Laveleye, M., Huang, T. D., Bogaerts, P., Berhin, C., Bauraing, C., Sacre, P., et al. (2017). Increasing incidence of carbapenemase-producing Escherichia coli and Klebsiella pneumoniae in Belgian hospitals. Eur. J. Clin. Microbiol. Infect. Dis. 36, 139–146. doi: 10.1007/s10096-016-2782-x
Deurenberg, R. H., Bathoorn, E., Chlebowicz, M. A., Couto, N., Ferdous, M., Garcia-Cobos, S., et al. (2017). Application of next generation sequencing in clinical microbiology and infection prevention. J. Biotechnol. 243, 16–24. doi: 10.1016/j.jbiotec.2016.12.022
Dik, J. W., Dinkelacker, A. G., Vemer, P., Lo-Ten-Foe, J. R., Lokate, M., Sinha, B., et al. (2016). Cost-analysis of seven nosocomial outbreaks in an academic hospital. PLOS ONE 11:e0149226. doi: 10.1371/journal.pone.0149226
Dortet, L., Jousset, A., Sainte-Rose, V., Cuzon, G., and Naas, T. (2016). Prospective evaluation of the OXA-48 K-SeT assay, an immunochromatographic test for the rapid detection of OXA-48-type carbapenemases. J. Antimicrob. Chemother. 71, 1834–1840. doi: 10.1093/jac/dkw058
EUCAST (2017). EUCAST Guidelines for Detection of Resistance Mechanisms and Specific Resistances of Clinical and/or Epidemiological Importance. Available at: http://www.eucast.org/resistance_mechanisms/
European Committee on Antimicrobial Susceptibility Testing (2014). The European Committee on Antimicrobial Susceptibility Testing. Breakpoint Tables for Interpretation of MICs and Zone Diameters. Version 7.1, 2017. Available at: http://www.eucast.org
Fattouh, R., Tijet, N., McGeer, A., Poutanen, S. M., Melano, R. G., and Patel, S. N. (2015). What is the appropriate meropenem MIC for screening of carbapenemase-producing Enterobacteriaceae in low-prevalence settings? Antimicrob. Agents Chemother. 60, 1556–1559. doi: 10.1128/AAC.02304-15
Gagnon, S., Levesque, S., Lefebvre, B., Bourgault, A. M., Labbe, A. C., and Roger, M. (2011). vanA-containing Enterococcus faecium susceptible to vancomycin and teicoplanin because of major nucleotide deletions in Tn1546. J. Antimicrob. Chemother. 66, 2758–2762. doi: 10.1093/jac/dkr379
Garcia-Alvarez, L., Holden, M. T., Lindsay, H., Webb, C. R., Brown, D. F., Curran, M. D., et al. (2011). Meticillin-resistant Staphylococcus aureus with a novel mecA homologue in human and bovine populations in the UK and Denmark: a descriptive study. Lancet Infect. Dis. 11, 595–603. doi: 10.1016/S1473-3099(11)70126-8
Grundmann, H., Glasner, C., Albiger, B., Aanensen, D. M., Tomlinson, C. T., Andrasevic, A. T., et al. (2017). Occurrence of carbapenemase-producing Klebsiella pneumoniae and Escherichia coli in the European survey of carbapenemase-producing Enterobacteriaceae (EuSCAPE): a prospective, multinational study. Lancet Infect. Dis. 17, 153–163. doi: 10.1016/S1473-3099(16)30257-2
Hegstad, K., Giske, C. G., Haldorsen, B., Matuschek, E., Schonning, K., Leegaard, T. M., et al. (2014). Performance of the EUCAST disk diffusion method, the CLSI agar screen method, and the Vitek 2 automated antimicrobial susceptibility testing system for detection of clinical isolates of enterococci with low- and medium-level VanB-type vancomycin resistance: a multicenter study. J. Clin. Microbiol. 52, 1582–1589. doi: 10.1128/JCM.03544-13
Huang, T. D., Poirel, L., Bogaerts, P., Berhin, C., Nordmann, P., and Glupczynski, Y. (2014). Temocillin and piperacillin/tazobactam resistance by disc diffusion as antimicrobial surrogate markers for the detection of carbapenemase-producing Enterobacteriaceae in geographical areas with a high prevalence of OXA-48 producers. J. Antimicrob. Chemother. 69, 445–450. doi: 10.1093/jac/dkt367
Jacobson, K. L., Cohen, S. H., Inciardi, J. F., King, J. H., Lippert, W. E., Iglesias, T., et al. (1995). The relationship between antecedent antibiotic use and resistance to extended-spectrum cephalosporins in group I beta-lactamase-producing organisms. Clin. Infect. Dis. 21, 1107–1113. doi: 10.1093/clinids/21.5.1107
Jurke, A., Kock, R., Becker, K., Thole, S., Hendrix, R., Rossen, J., et al. (2013). Reduction of the nosocomial meticillin-resistant Staphylococcus aureus incidence density by a region-wide search and follow-strategy in forty German hospitals of the EUREGIO, 2009 to 2011. Euro Surveil. 18:20579. doi: 10.2807/1560-7917.ES2013.18.36.20579
Kaase, M., Schimanski, S., Schiller, R., Beyreiss, B., Thurmer, A., Steinmann, J., et al. (2016). Multicentre investigation of carbapenemase-producing Escherichia coli and Klebsiella pneumoniae in German hospitals. Int. J. Med. Microbiol. 306, 415–420. doi: 10.1016/j.ijmm.2016.05.009
Kinnevey, P. M., Shore, A. C., Brennan, G. I., Sullivan, D. J., Ehricht, R., Monecke, S., et al. (2014). Extensive genetic diversity identified among sporadic methicillin-resistant Staphylococcus aureus isolates recovered in Irish hospitals between 2000 and 2012. Antimicrob. Agents Chemother. 58, 1907–1917. doi: 10.1128/AAC.02653-13
Klare, I., Fleige, C., Geringer, U., Witte, W., and Werner, G. (2012). Performance of three chromogenic VRE screening agars, two etest((R)) vancomycin protocols, and different microdilution methods in detecting vanB genotype Enterococcus faecium with varying vancomycin MICs. Diagn. Microbiol. Infect. Dis. 74, 171–176. doi: 10.1016/j.diagmicrobio.2012.06.020
Kluytmans, J., Vos, G., Vandenbroucke-Grauls, C., Friedrich, A. W., Voss, A., and Bonten, M. (2015). Nederland CRE-groen: “optimalisatie van de beheersing van antimicrobiële resistentie door regionale netwerkvorming”. Available at: http://www.nvmm.nl/media/1189/20151006_visiedocument_nl-cre-groen.pdf
Lee, C. R., Lee, J. H., Park, K. S., Kim, Y. B., Jeong, B. C., and Lee, S. H. (2016). Global dissemination of carbapenemase-producing Klebsiella pneumoniae: epidemiology, genetic context, treatment options, and detection methods. Front. Microbiol. 7:895. doi: 10.3389/fmicb.2016.00895
Liapis, E., Pantel, A., Robert, J., Nicolas-Chanoine, M. H., Cavalie, L., van der Mee-Marquet, N., et al. (2014). Molecular epidemiology of OXA-48-producing Klebsiella pneumoniae in France. Clin. Microbiol. Infect. 20, O1121–O1123. doi: 10.1111/1469-0691.12727
Literacka, E., Herda, M., Baraniak, A., Zabicka, D., Hryniewicz, W., Skoczynska, A., et al. (2017). Evaluation of the Carba NP test for carbapenemase detection in Enterobacteriaceae, Pseudomonas spp. and Acinetobacter spp., and its practical use in the routine work of a national reference laboratory for susceptibility testing. Eur. J. Clin. Microbiol. Infect. Dis. 36, 2281–2287. doi: 10.1007/s10096-017-3062-0
Liu, J., Chen, D., Peters, B. M., Li, L., Li, B., Xu, Z., et al. (2016). Staphylococcal chromosomal cassettes mec (SCCmec): a mobile genetic element in methicillin-resistant Staphylococcus aureus. Microb. Pathog. 101, 56–67. doi: 10.1016/j.micpath.2016.10.028
Livermore, D. M., Brown, D. F., Quinn, J. P., Carmeli, Y., Paterson, D. L., and Yu, V. L. (2004). Should third-generation cephalosporins be avoided against AmpC-inducible Enterobacteriaceae? Clin. Microbiol. Infect. 10, 84–85. doi: 10.1111/j.1469-0691.2004.00831.x
Luteijn, J. M., Hubben, G. A., Pechlivanoglou, P., Bonten, M. J., and Postma, M. J. (2011). Diagnostic accuracy of culture-based and PCR-based detection tests for methicillin-resistant Staphylococcus aureus: a meta-analysis. Clin. Microbiol. Infect. 17, 146–154. doi: 10.1111/j.1469-0691.2010.03202.x
Mezzatesta, M. L., Gona, F., and Stefani, S. (2012). Enterobacter cloacae complex: clinical impact and emerging antibiotic resistance. Future Microbiol. 7, 887–902. doi: 10.2217/fmb.12.61
Nordmann, P., Gniadkowski, M., Giske, C. G., Poirel, L., Woodford, N., Miriagou, V., et al. (2012). Identification and screening of carbapenemase-producing Enterobacteriaceae. Clin. Microbiol. Infect. 18, 432–438. doi: 10.1111/j.1469-0691.2012.03815.x
Oteo, J., Ortega, A., Bartolome, R., Bou, G., Conejo, C., Fernandez-Martinez, M., et al. (2015). Prospective multicenter study of carbapenemase-producing Enterobacteriaceae from 83 hospitals in Spain reveals high in vitro susceptibility to colistin and meropenem. Antimicrob. Agents Chemother. 59, 3406–3412. doi: 10.1128/AAC.00086-15
Park, I. J., Lee, W. G., Shin, J. H., Lee, K. W., and Woo, G. J. (2008). VanB phenotype-vanA genotype Enterococcus faecium with heterogeneous expression of teicoplanin resistance. J. Clin. Microbiol. 46, 3091–3093. doi: 10.1128/JCM.00712-08
Singh, K., Mangold, K. A., Wyant, K., Schora, D. M., Voss, B., Kaul, K. L., et al. (2012). Rectal screening for Klebsiella pneumoniae carbapenemases: comparison of real-time PCR and culture using two selective screening agar plates. J. Clin. Microbiol. 50, 2596–2600. doi: 10.1128/JCM.00654-12
Sivertsen, A., Pedersen, T., Larssen, K. W., Bergh, K., Ronning, T. G., Radtke, A., et al. (2016). A silenced vanA gene cluster on a transferable plasmid caused an outbreak of vancomycin-variable enterococci. Antimicrob. Agents Chemother. 60, 4119–4127. doi: 10.1128/AAC.00286-16
van der Bij, A. K., Kardamanidis, K., Frakking, F. N., Bonten, M. J., and Signaleringsoverleg Ziekenhuisinfecties en Antimicrobiele Resistentie. (2015). Nosocomial outbreaks and resistant microorganisms. Ned. Tijdschr. Geneeskd. 159, A8585.
van der Zwaluw, K., de Haan, A., Pluister, G. N., Bootsma, H. J., de Neeling, A. J., and Schouls, L. M. (2015). The carbapenem inactivation method (CIM), a simple and low-cost alternative for the Carba NP test to assess phenotypic carbapenemase activity in gram-negative rods. PLOS ONE 10:e0123690. doi: 10.1371/journal.pone.0123690
Voulgari, E., Zarkotou, O., Ranellou, K., Karageorgopoulos, D. E., Vrioni, G., Mamali, V., et al. (2013). Outbreak of OXA-48 carbapenemase-producing Klebsiella pneumoniae in Greece involving an ST11 clone. J. Antimicrob. Chemother. 68, 84–88. doi: 10.1093/jac/dks356
Werner, G., Klare, I., Fleige, C., Geringer, U., Witte, W., Just, H. M., et al. (2012). Vancomycin-resistant vanB-type Enterococcus faecium isolates expressing varying levels of vancomycin resistance and being highly prevalent among neonatal patients in a single ICU. Antimicrob. Resist. Infect. Control 1:21. doi: 10.1186/2047-2994-1-21
Wertheim, H. F., Vos, M. C., Boelens, H. A., Voss, A., Vandenbroucke-Grauls, C. M., Meester, M. H., et al. (2004). Low prevalence of methicillin-resistant Staphylococcus aureus (MRSA) at hospital admission in the Netherlands: the value of search and destroy and restrictive antibiotic use. J. Hosp. Infect. 56, 321–325. doi: 10.1016/j.jhin.2004.01.026
Working group members NVMM (2012). NVMM Guideline Laboratory Detection of Highly Resistant Microorganisms (HRMO). Version 2. Available at: http://oudewebsite.nvmm.nl/system/files/2012.11.15%20richtlijn%20BRMO%20%28version%202.0%29%20-%20RICHTLIJN.pdf
Zhou, X., Arends, J. P., Kampinga, G. A., Ahmad, H. M., Dijkhuizen, B., van Barneveld, P., et al. (2014). Evaluation of the xpert vanA/vanB assay using enriched inoculated broths for direct detection of vanB vancomycin-resistant enterococci. J. Clin. Microbiol. 52, 4293–4297. doi: 10.1128/JCM.01125-14
Keywords: diagnostic evasion, outbreaks, HRMO, signaling networks, antibiotic resistance
Citation: Zhou X, Friedrich AW and Bathoorn E (2017) Diagnostic Evasion of Highly-Resistant Microorganisms: A Critical Factor in Nosocomial Outbreaks. Front. Microbiol. 8:2128. doi: 10.3389/fmicb.2017.02128
Received: 28 February 2017; Accepted: 18 October 2017;
Published: 03 November 2017.
Edited by:
Manuela Caniça, Instituto Nacional de Saúde, PortugalReviewed by:
Govindan Rajamohan, Institute of Microbial Technology (CSIR), IndiaDiamantis Plachouras, European Centre for Disease Prevention and Control, Sweden
Copyright © 2017 Zhou, Friedrich and Bathoorn. This is an open-access article distributed under the terms of the Creative Commons Attribution License (CC BY). The use, distribution or reproduction in other forums is permitted, provided the original author(s) or licensor are credited and that the original publication in this journal is cited, in accordance with accepted academic practice. No use, distribution or reproduction is permitted which does not comply with these terms.
*Correspondence: Alexander W. Friedrich, YWxleC5mcmllZHJpY2hAdW1jZy5ubA==