- 1Clinic for Vascular and Endovascular Surgery, University Hospital Münster, Münster, Germany
- 2Department of Vascular Surgery, St. Franziskus-Hospital Münster, Münster, Germany
- 3Institute of Medical Microbiology, University Hospital Münster, Münster, Germany
Objectives: Increasing resistance of microorganisms and particularly tolerance of bacterial biofilms against antibiotics require the need for alternative antimicrobial substances. S. aureus is the most frequent pathogen causing vascular graft infections. In order to evaluate the antimicrobial efficacy, quantification of the bacterial biofilms is necessary. Aim of the present study was the validation of an in vitro model for quantification of bacterial biofilm on vascular graft surfaces using three different assays.
Methods: Standardized discs of vascular graft material (Dacron or PTFE) or polystyrene (PS) as control surface with 0.25 cm2 surface area were inoculated with 10−3 diluted overnight culture of three biofilm-producing S. aureus isolates (BEB-029, BEB-295, SH1000) in 96-well PS culture plates. After incubation for 4 and 18 h, the biofilm was determined by three different methods: (a) mitochondrial ATP concentration as measure of bacterial viability (ATP), (b) crystal violet staining (Cry), and (c) vital cell count by calculation of colony-forming units (CFU). The experiments were performed three times. Quadruplicates were used for each isolate, time point, and method. In parallel, bacterial biofilms were documented via scanning electron microscopy.
Results: All three methods could quantify biofilms on the PS control. Time needed was 0:40, 13:10, and 14:30 h for ATP, Cry, and CFU, respectively. The Cry assay could not be used for vascular graft surfaces due to high unspecific background staining. However, ATP assay and CFU count showed comparable results on vascular graft material and control. The correlations between ATP and CFU assay differed according to the surface and incubation time and were significant only after 4 h on Dacron (BEB-029, p = 0.013) and on PS (BEB-029, p < 0.001). Between ATP and Cry assay on PS, a significant correlation could be detected after 4 h (BEB-295, p = 0.027) and after 18 h (all three strains, p < 0.026). The reproducibility of the ATP-assay presented as inter-assay-variance of 2.1 and as intra-assay variance of 8.1 on polystyrene.
Conclusion: The in-vitro model reproducibly quantifies biofilm on standardized vascular graft surfaces with ATP assay as detection system. The ATP assay allows accelerated microbial quantification, however the correlation with the CFU assay may be strain- and surface-dependent.
Introduction
Although bacterial biofilms are naturally occurring in dental plaque (Sanz et al., 2017), their presence is mainly associated to critical medical conditions such as infections of the upper respiratory or urogenital tract, peritonitis, and on implanted medical devices, which are increasingly used (Lynch and Robertson, 2008; Romling et al., 2014).
Vascular grafts are used in aortic reconstructions and for lack of autologous vein material also in peripheral bypass operations. Despite highest hygienic precautionary measures during surgery, vascular graft infections (VGIs) occur in 2–4% of cases and are associated with high morbidity and mortality rates (10–75%) (Hepp, 2009; Young et al., 2012). Gram-positive microorganisms such as Staphylococcus aureus and Staphylococcus epidermidis are typically the cause of aortic graft infections (Chaufour et al., 2017).
Bacterial colonization of the vascular graft surface is associated with bacterial biofilm production, the accumulation of extracellular polymer substance matrix (EPS), consisting of polysaccharides, proteins, glycolipids, and bacterial DNA (Becker et al., 2014). The EPS shields the bacteria by preventing the penetration of macromolecules like antibiotics and inflammatory cells of the immune defense into the biofilm matrix as well as by displaying a diffusion barrier for molecules with antimicrobial properties (Olsen, 2015). Within the biofilm, subpopulations can become metabolic inactive and form dormant persister cells. Since some antibiotics are only effective for metabolic active bacteria, the resting bacteria may be insensitive toward antibiotics (Lewis, 2007).
Next to standardized pre- and peri-surgical prevention measures against VGIs (pre-screening for multidrug resistant organisms, control of infection parameters, antimicrobial prophylaxis), also regional antibiotic release from vascular grafts (pre-soaking of the graft with antibiotics) could play an effective role (Keeling et al., 2009; Kuehn et al., 2010; Bisdas et al., 2012).
Growing resistance and tolerance of bacterial biofilms against antibiotics require the need for alternative antimicrobial substances. An alternative for therapy of S. aureus biofilms on vascular grafts could be for example bacteriophage endolysins, such as HY-133, with specific efficacy against S. aureus (Idelevich et al., 2011, 2016; Herten et al., 2017). In order to evaluate the antimicrobial efficacy of such alternative antimicrobial substances, the quantification of the bacterial biofilms is necessary.
Several methods are available for the detection of bacterial biofilms, either based on (a) the accumulation of dye/pigments binding to negative charges present at the EPS, e.g., crystal violet (O'toole et al., 2000; Stepanovic et al., 2000) or safranin (Patterson et al., 2010), or (b) DNA binding dyes (DAPI, Hoechst, SYTO9 or acridine orange, Palestrant et al., 2004; Peeters et al., 2008) or qPCR, or (c) cultural method [colony forming units (CFU) calculation (Alt et al., 2004)], time to regrowth to a specific turbidity], or (d) detecting the metabolic activity [ATP (Gracia et al., 1999; Kapoor and Yadav, 2010), tetrazolium salt MTT, resazurin (Alamar blue) (Hatzinger et al., 2003; Pettit et al., 2009)] or membrane integrity (e.g., live/dead staining SYTO9/propidium iodide, Tawakoli et al., 2013).
However, quantifications of bacterial biofilms were mainly performed on polystyrene surface, only few methods are available for other surfaces (Pitts et al., 2003). For the quantification of biofilms on indwelling devices, the CFU count seems to be the standard method until now and replaced the past radioactive labeling of the bacteria (Schmitt et al., 1986).
Aim of the present study was the validation of an in vitro model for rapid quantification of bacterial biofilm adherence on vascular graft material with an ATP assay compared to CFU count and crystal violet (Cry) assay.
Methods
Overnight Culture of Bacteria
Two clinical biofilm-producing S. aureus (BEB-029 and BEB-295) isolates as well as laboratory strain SH1000 were used for experiments. Species affiliation was confirmed by detection of the S. aureus-specific nuc gene and methicillin resistance was determined by mecA gene detection as described elsewhere (Becker et al., 2006). The isolates were incubated overnight in tryptic soy broth (TSB) at 36C°.
Experiment Set Up
Vascular graft material (Dacron (polyester), knitted, (Uni-Graft® K DV, B. Braun, Melsungen, Germany and PTFE MAXIFLO™ Ultrathin, Vascutek, Terumo, Frankfurt, Germany) were dissected with biopsy punches (ø 5 mm, pmf medical AG, Cologne, Germany) in order to yield a standardized surface area of 0.25 cm2 (Figure 1), The discs were positioned into 96-well polystyrene (PS) culture plates and submerged using peg lids (MBEC Assay, St. Edmonton, Canada). Polystyrene without graft material discs served as control surface. All 96 wells (with and without discs) were inoculated with a 10−3 diluted overnight culture in TSB with 0.25% glucose. Sterility controls were additionally performed. After 4 and 18 h incubation at 36°C, the planktonic bacteria were rinsed off twice with phosphate-buffered saline (PBS). The biofilm concentration was measured on the graft disc and on the control wells. The experiments were performed three times.
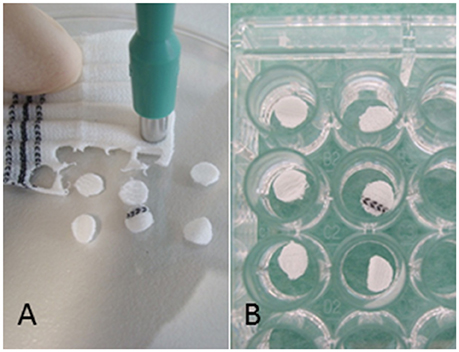
Figure 1. Experiment set-up: (A) Vascular graft material was dissected with biopsy punches resulting in a standardized surface area of 0.25 cm2 and (B) placed into 96-well polystyrene (PS) cell culture plates.
SEM Documentation of Bacterial Biofilms
Overnight cultures were diluted 10−3 and vascular grafts (Dacron, PTFE) inoculated as described above. After 18 h cultivation, the discs were rinsed twice with PBS and the bacterial biofilm was fixed in 2.5% glutardialdehyde in PBS for 24 h at 8°C. Post-fixation was performed with 1% osmium tetroxide in PBS for 20 min at room temperature. After dehydration through an ascending series of ethanol ending in 100% ethanol, the samples were dried via critical point drying (Emitech, Ashford, UK/CPD 030, Bal-Tec AG, Balzers, Liechtenstein) according to the manufacturer instructions. Afterward the discs were gold-sputtered (E5100, Polaron Instruments, UK/SC0005 Bal-Tec AG, Balzers, Liechtenstein) and analyzed with a sequential electron microscope (S800, Hitachi, Düsseldorf, Germany).
Biofilm Quantification
All tests were performed in quadruplicates for each isolate (3 strains), culture time (4 and 18 h), cultivation surface (Dacron, PTFE and PS control), and assay type.
ATP Assay
The mitochondrial adenosine triphosphate (ATP) concentration was measured using the BacTiter-Glo™ Microbial Cell Viability assay (Promega, Mannheim, Germany) as described before (Ickert et al., 2015; Herten et al., 2017). The number of viable bacteria is quantified by their amount of ATP and is directly proportional to the ATP concentration. The assay is based on the reaction of luciferase, which catalyzes the reaction of luciferin and ATP to oxyluciferin by the emission of a luminescent signal, which is measured using a multilabel plate reader (FLX800, Biotek, Bad Friedrichshall, Germany). Preceding experiments were performed with varying amounts of lysis solution in order to determine the concentration necessary to lyse the S. aureus cells. The optimal relation of PBS and lysis solution was 1:3. In brief, after washing, 50 μl PBS and 150 μl BacTiter-Glo™ solution was added to each well. After an incubation period of 5 min at room temperature, the luminescent signal was recorded in counts per second. Additionally, standard measurements with defined numbers of S. aureus was performed, and ATP standard curves were made with defined ATP-concentrations (0.1–50 μM) for each measurement. Hands-on time for the ATP assay was 0:30 min, results were available after 0:40 min.
Crystal Violet Assay
One hundred microliters of 1% crystal violet solution were added to each well of a microtiter plate containing graft discs and incubated at room temperature for 5 min. Subsequently, the graft discs were transferred into the wells of another microtiter plate to wash out the excessive amounts of crystal violet twice with 200μl PBS. After the aspiration of PBS and drying overnight, 50 μl of absolute ethanol were added, followed by shaking the plate and incubation for 10 min. After the graft discs were removed, absorbance was measured at 570 nm via a spectrophotometer. Hands-on time for the Cry assay was 1:10 h, incubation time 12 h, results were available after 13:10 h.
CFU Count
The number of CFUs of vital bacteria was measured by plating of serial dilutions onto the solid medium. For this, the vascular grafts were rinsed with 100 μl PBS and sonicated for 10 min in order to detach and disrupt the biofilm from the graft material into a recovery medium. After removal of graft discs, the serial dilutions of well content were plated on tryptic soy agar (TSA) in triplicate. After incubation for 18 ± 2 h at 36°C the numbers of colonies were counted. Hands-on time for the CFU assay was 2:30 h, incubation time 12 h, results were available after ~14:30 h.
Statistical Analysis
The statistical analysis was performed with software IBM SPSS Statistics for Windows (release 24, Chicago, IL; USA). Categorical variables are expressed as frequency and percentage whereas continuous variables were presented as mean ± SD. Before statistically testing, the Kolmogorov-Smirnov test was used to analyze each continuous variable for its normal distribution. Because the samples were not normally distributed, the non-parametric tests were used. The Mann-Whitney U-test was used for comparison of non-parametric variables of two independent study groups. The Pearson correlation coefficient (r) was used to evaluate the relationship between two continuous variables ATP vs. CFU on polystyrene surface, on Dacron and on PTFE and while CFU vs. Cry could be only determined on polystyrene surface because of the high staining background of Cry on the vascular material. Linear regression model was used to estimate the relationship between the dependent variable ATP and the independent variable CFU or Cry. The coefficient of determination (R2) was calculated as a statistical measure of how well the regression line approximates the real data points. Differences were considered significant at p < 0.05. Measurement accuracy of the ATP assay was calculated as coefficient of variability (CV) by estimating inter- and intra-assay variance.
Results
SEM Documentation of Bacterial Biofilms
SEM documentation of bacterial biofilms revealed a distinctly recognizable biofilm on the single threads of the knitted Dacron surface (Figures 2A–C). In contrast, the much smoother PTFE surface revealed accumulated bacterial groups, which were more scattered (Figures 2D–F).
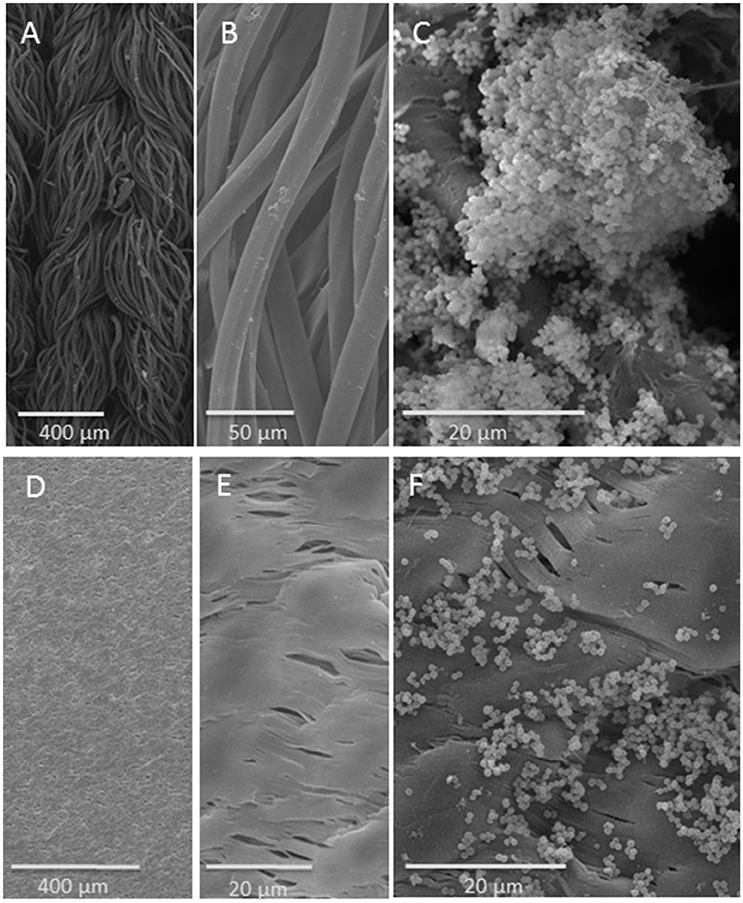
Figure 2. SEM analysis of vascular graft material: upper row: on Dacron fiber, lower row: on PTFE surface: (A) knitted structure; (B) fibers; (C) S. aureus strain SH1000 biofilm on Dacron fiber. (D,E) PTFE surface in different magnifications; (F) S. aureus strain SH1000 on PTFE.
Biofilm Quantification
All three assay methods could quantify biofilms on the PS control surface (Figure 3). The reproducibility of the ATP-assay presented an inter-assay-variance of 2.1 and an intra-assay variance of 8.1 on polystyrene. The growth pattern of the biofilm revealed an increase in bacterial biomass from 4 to 18 h incubation time in all three assay methods.
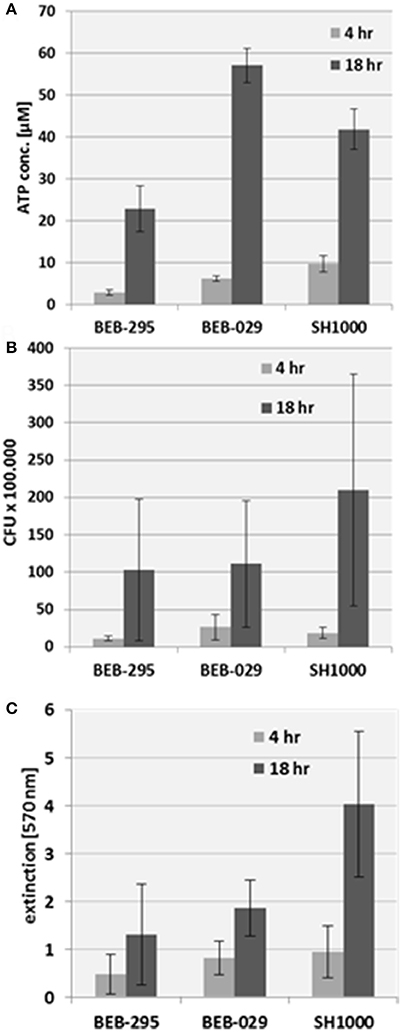
Figure 3. Biofilm quantification on the control surface polystyrene. (A) Mitochondrial ATP concentration, (B) CFU count, (C) Crystal violet staining.
The measurement of Cry via spectrophotometry was not suitable for vascular graft surfaces because of the high unspecific background staining.
Regarding the bacterial growth pattern on the different graft materials, S. aureus strain BEB-029 and BEB-295 displayed their highest ATP concentration and CFU count, respectively, on the PS control, followed by Dacron and the least on PTFE (Figure 4). This is in accordance with the growth intensity displayed on the SEM pictures. For the S. aureus strain SH1000, the highest ATP concentration was demonstrated on Dacron material followed by PS and PTFE, while in the CFU assay strain SH1000 showed the highest values on PS (Figure 4 lower row).
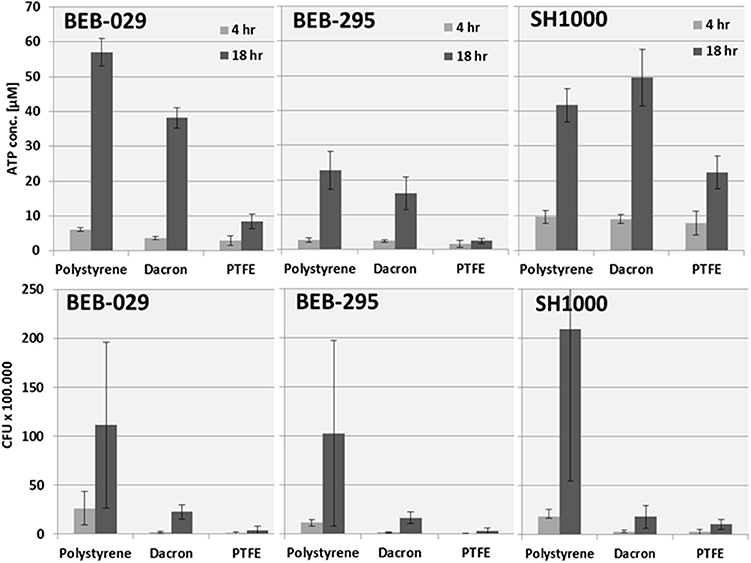
Figure 4. Quantification of the S. aureus strains on different surfaces: polystyrene, Dacron and PTFE. Upper: ATP concentration. Lower: CFU counts. Data for the polystyrene surface were additionally depicted in Figure 3.
The Pearson's correlation coefficient depicted a strong correlation coefficient between ATP and CFU on Dacron after 4 h for BEB-029 (0.606, p = 0.013) and a moderately strong for BEB-295 (0.442, p = 0.086) (Table 1, Table S1). On PS a very strong correlation was detectable for BEB-029 (0.858, p < 0.001) after 4 h and a moderate strong one for SH1000 (0.548, p = 0.065) after 18 h. Likewise, a strong correlation on PS surface could be detected between ATP and Cry for BEB-295 after 4 h (0.633, p = 0.027), a moderately strong one for BEB-029 (0.408, p = 0.188) and strong to very strong correlations after 18 h for all three strains (r ≥ 0.741, p < 0.026).
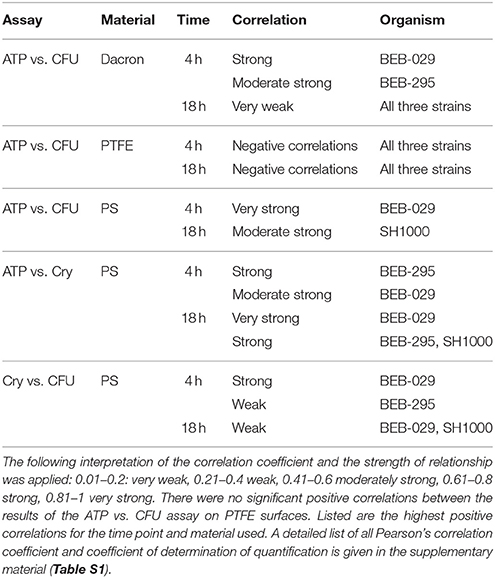
Table 1. Pearson's correlation coefficient of the S. aureus strains on the different materials (Dacron or polystyrene) for ATP vs. CFU, ATP vs. Cry, and CFU vs. Cry.
In contrast, there were only negative correlations between the ATP and CFU assays for the PTFE surface (range r = −0.224 until −0.811). The assay time until result was 0:40 h for ATP, 13:10 h for Cry, and 14:30 h for CFU assays.
Comparing CFU count with Cry assay, a strong coefficient was present only after 4 h for BEB-029 (0.628, p = 0.029).
Discussion
In Vitro Model for Rapid Quantification
This study evaluated an in vitro model for rapid quantification of bacterial biofilm adherence on vascular graft material with an ATP assay. In particular, the ATP assay was compared to the CFU count, as the gold standard method, and to the crystal violet staining. Using BEB-029, the ATP assay correlated significantly with the CFU method at 4 h where it was useful as a rapid assay for biofilm quantification. However, the correlation with CFU assay was generally strain- and surface-dependent (Table 1, Table S1). In addition, correlation between CFU and ATP assays was generally better for immature biofilm (4 h) than mature biofilm (18 h).
A comparison of ATP and Cry assay on the PS control surface revealed correlations for both biofilm types: at 4 h strong correlation for BEB-295, moderately strong ones for BEB-029 and at 18 h very strong correlation for BEB-029 and strong one for the two other strains.
Concerning the only moderately strong correlation between ATP assay and CFU count on mature biofilms on PS, it can be pointed out, that both assay systems ATP and Cry showed comparable correlations even for mature biofilms and both did not correlate with the CFU method in mature biofilm. Both assays do not require any detaching and separation of bacterial biofilm, which might be a reason for the missing correlations with the CFU in mature biofilm. Biofilm quantification revealed that the colonization of the materials used (PTFE vs. Dacron) was different over time for the three S. aureus biofilm-producing strains. On PTFE, the bacterial colonization was lower as determined by ATP assay and CFU counting. These quantitative results could be confirmed by qualitative visualization by SEM.
In this study, we used a static biofilm model, which delivers helpful insights in the usefulness of different assays for biofilm quantification. Dynamic biofilm models may provide more differentiated information and should be included in further investigations of vascular grafts exposed to a continuous blood flow.
ATP Assay on Polystyrene (PS) Surface
The ATP assay has been used previously for detection of bacterial biofilms on polystyrene (PS) surface. Gracia et al. could show that for bacterial ATP extraction from in vitro developed S. aureus biofilms on coated PS surface with high bacterial density, DMSO was found preferable in relation to commercially available bacteria lysis reagent (upper detection limits 2.3 × 109 and 2 × 108 CFU/ml respectively) (Gracia et al., 1999).
Kapoor et al. used the ATP bioluminescence assay for biocidal susceptibility testing of rapidly growing mycobacteria and compared it to the conventional agar plating method. They demonstrated that the ATP assay was rapid (1.5 h) and showed high sensitivity and specificity. Using this method, the ATP assay revealed a linear relationship between intracellular ATP and the cell number (CFU/ml) in the CFU range of 103-107 CFU/ml. (Kapoor and Yadav, 2010). In the present study, the detection limit for the ATP assay for S. aureus was in the range from 1.5 × 102 up to more than 108 cells as described in the instructions for use, thus being comparable to the detection limits described by Gracia and Kapoor (Gracia et al., 1999; Kapoor and Yadav, 2010).
Villain-Guillot et al. detected S. epidermidis biofilms in 96-well polystyrene microtiter plates. They presented very good correlation curves (r = 1.0) between the ATP-counting luminescence values and the amount of biofilm-embedded S. epidermidis determined by serial dilution and cultivation (CFU assay) (Villain-Guillot et al., 2007). But in contrast to our method, each biofilm was sonicated, the bacterial suspension was serially diluted and part of it was used for ATP assay and for classical plate counting. Therefore, both assays had the same diluted bacterial samples as starting material omitting any possible bias resulting from the detaching. In the present study, the ATP assay was used as rapid assay leaving out the detaching and dilutions steps. On PS surface, the correlations between ATP assay and CFU count were very strong at 4 h and moderately strong at 18 h.
ATP Assay on Indwelling Medical Devices/Biomaterials
Next to polystyrene, the ATP assay has also been applied for the detection of bacterial biofilms on indwelling medical devices/biomaterials. Gracia et al. investigated the adherence potential of different biofilm producing and non-biofilm-producing strains of S. aureus strains on biomaterials used in orthopedic surgery (polymethylmethacrylate, fresh bone, steel, and titanium alloys and on glass) (Gracia et al., 1997). The device material was sonicated to disintegrate bacterial clumps. They used a calibration curve of bacterial ATP vs. CFU to convert ATP moles to CFU counts. A linear relationship was found between the detected bacterial ATP and the number of bacteria (CFU/ml) in the interval between 3.5 × 105 CFU/ml and 3.5 × 109 CFU/ml with a high correlation (r = 0.99). In the present study, the ATP assay and the CFU counting method were compared displaying strong or moderate correlations on Dacron at 4 h and weak correlations at 18 h and without positive correlations on PTFE. Our data cannot be directly compared since Gracia et al. used the detached bacteria solution for the standard curve.
Bacterial Adherence to Vascular Grafts
Regarding bacterial adherence to vascular grafts some in vitro studies have been published. All of them determined bacterial adherence as the number of CFUs dislodged from graft material by sonication.
Schmitt et al. used 1 cm2 squares of graft material (n = 5/6) immersed in the bacterial suspension of S. aureus, Escherichia coli, and S. epidermidis. Bacterial adherence depended on Dacron, and was least on PTFE graft material (Schmitt et al., 1986). This is in line with our findings of the SEM documentation (more scattered accumulated bacterial groups on PTFE) and was confirmed by the ATP assay and the CFU count. Kuehn et al. used the same model for evaluation antibiotic pretreatment of vascular prostheses using seven grafts per group. They could show that the risk of vascular graft infection was reduced by pretreating the prostheses with antibiotics while an antibiotic/fibrin compound exhibited an effect of delayed antibiotic release (Kuehn et al., 2010). In the present study, we used standardized vascular graft surfaces cut with a biopsy punch with a defined area which might be easier than individually cut small matching squares.
Sasaki investigated the bacterial penetration of Pseudomonas aeruginosa from the outer surface of vascular grafts material comparing elastomer-sealed and gelatin-coated surface treatment of the outside of the graft. In the experimental setup, 12 or 18 grafts were cut into 6-cm segments, placed in a U-shaped configuration on culture plates and inoculated at the outer graft surface. Data revealed a bacterial invasion through the prosthetic graft material starting 6 h post-bacterial immersion with no significant differences between the two different treatments of the outer polyester surface (Sasaki, 2014).
It is noticeable that the group size was 5, 6, or 7 and the experiments were performed only once. This could be due to the fact that the determination of bacterial adherence via CFUs is time- and labor-consuming.
The CFU assay requires the incubation of diluted bacteria sample. Mandatory prerequisite for the dilution is the dissolving of the bacterial biofilm which may be a distinct bias of the different methods available.
Several investigators have reported inconsistent results regarding the development of infection- resistant vascular grafts. In addition, numerous antibiotics have been investigated, sometimes with different carriers to encourage a prolonged antimicrobial effect (Kuehn et al., 2010).
Conclusion
The in-vitro model could be used for reproducible quantification of biofilm adhesion on standardized vascular graft surfaces with ATP assay as detection system. Compared to the CFU assay, the ATP assay allows accelerated quantification of bacteria, however the correlation with CFU assay may be strain- and surface-dependent.
Author Contributions
MH and EAI: designed this project; MH, TB, DK, KB, NO, GT, and EAI: contributed to the experiments, analysis and interpretation of the work; MH: drafted the manuscript; MH, TB, DK, KB, NO, GT, and EAI: reviewed the manuscript for intellectual content.
Conflict of Interest Statement
The authors declare that the research was conducted in the absence of any commercial or financial relationships that could be construed as a potential conflict of interest.
Acknowledgments
This work was supported by the fund: “Innovative Medical Research” from Medical School of the University of Münster (ID 111317). We thank Damayanti Kaiser from the Institute of Medical Microbiology for excellent technical support and Ulrike Keller and Harald Nüsse from the Institute of Medical Physics and Biophysics, University of Münster, for the SEM analysis. Vascular graft material was provided by B. Braun, Melsungen, Germany (Uni-Graft® K DV), and Vascutek, Terumo, Frankfurt, Germany (PTFE MAXIFLO™ Ultrathin).
Supplementary Material
The Supplementary Material for this article can be found online at: https://www.frontiersin.org/articles//10.3389/fmicb.2017.02333/full#supplementary-material
Table S1. Pearson's correlation coefficient and coefficient of determination of quantification for all assays, surfaces, and time points.
Abbreviations
ATP, mitochondrial ATP concentration as measure of bacterial viability; BEB-029, BEB-295, SH1000, biofilm-producing S. aureus isolates; CFU, colony-forming units; CV, coefficient of variability; Cry, crystal violet staining; EPS, extracellular polymer substance matrix; MTT, 3-(4,5-dimethylthiazol-2-yl)-2,5-diphenyltetrazolium bromide for assessing cell metabolic activity; PTFE, polytetrafluorethylene; R2, coefficient of determination; r, Pearson correlation coefficient; SEM, scanning electron microscopy; SYTO9, cell-permeant nucleic acid stains; VGI, vascular graft infections.
References
Alt, V., Bechert, T., Steinrucke, P., Wagener, M., Seidel, P., Dingeldein, E., et al. (2004). In vitro testing of antimicrobial activity of bone cement. Antimicrob. Agents Chemother. 48, 4084–4088. doi: 10.1128/AAC.48.11.4084-4088.2004
Becker, K., Heilmann, C., and Peters, G. (2014). Coagulase-negative staphylococci. Clin. Microbiol. Rev. 27, 870–926. doi: 10.1128/CMR.00109-13
Becker, K., Pagnier, I., Schuhen, B., Wenzelburger, F., Friedrich, A. W., Kipp, F., et al. (2006). Does nasal cocolonization by methicillin-resistant coagulase-negative staphylococci and methicillin-susceptible Staphylococcus aureus strains occur frequently enough to represent a risk of false-positive methicillin-resistant S. aureus determinations by molecular methods? J. Clin. Microbiol. 44, 229–231. doi: 10.1128/JCM.44.1.229-231.2006
Bisdas, T., Beckmann, E., Marsch, G., Burgwitz, K., Wilhelmi, M., Kuehn, C., et al. (2012). Prevention of vascular graft infections with antibiotic graft impregnation prior to implantation: in vitro comparison between daptomycin, rifampin and nebacetin. Eur. J. Vasc. Endovasc. Surg. 43, 448–456. doi: 10.1016/j.ejvs.2011.12.029
Chaufour, X., Gaudric, J., Goueffic, Y., Khodja, R. H., Feugier, P., Malikov, S., et al. (2017). A multicenter experience with infected abdominal aortic endograft explantation. J. Vasc. Surg. 65, 372–380. doi: 10.1016/j.jvs.2016.07.126
Gracia, E., Fernandez, A., Conchello, P., Alabart, J. L., Perez, M., and Amorena, B. (1999). In vitro development of Staphylococcus aureus biofilms using slime-producing variants and ATP-bioluminescence for automated bacterial quantification. Luminescence 14, 23–31. doi: 10.1002/(SICI)1522-7243(199901/02)14:1<23::AID-BIO513>3.0.CO;2-M
Gracia, E., Fernandez, A., Conchello, P., Lacleriga, A., Paniagua, L., Seral, F., et al. (1997). Adherence of Staphylococcus aureus slime-producing strain variants to biomaterials used in orthopaedic surgery. Int. Orthop. 21, 46–51. doi: 10.1007/s002640050116
Hatzinger, P. B., Palmer, P., Smith, R. L., Penarrieta, C. T., and Yoshinari, T. (2003). Applicability of tetrazolium salts for the measurement of respiratory activity and viability of groundwater bacteria. J. Microbiol. Methods 52, 47–58. doi: 10.1016/S0167-7012(02)00132-X
Hepp, W. (2009). “Postoperative Infekte,” in Gefäßchirurgie, eds W. Hepp and H. Kogel (München; Jena: Urban & Fischer Verlag/Elsevier GmbH), 669–683.
Herten, M., Idelevich, E. A., Sielker, S., Becker, K., Scherzinger, A. S., Osada, N., et al. (2017). Vascular graft impregnation with antibiotics: the influence of high concentrations of rifampin, vancomycin, daptomycin, and bacteriophage endolysin HY-133 on viability of vascular cells. Med. Sci. Monit. Basic Res. 23, 250–257. doi: 10.12659/MSMBR.902879
Ickert, I., Herten, M., Vogl, M., Ziskoven, C., Zilkens, C., Krauspe, R., et al. (2015). Opioids as an alternative to amide-type local anaesthetics for intra-articular application. Knee Surg. Sports Traumatol. Arthrosc. 23, 2674–2681. doi: 10.1007/s00167-014-2989-2
Idelevich, E. A., Schaumburg, F., Knaack, D., Scherzinger, A. S., Mutter, W., Peters, G., et al. (2016). The recombinant bacteriophage endolysin HY-133 exhibits in vitro activity against different african clonal lineages of the Staphylococcus aureus complex, including Staphylococcus schweitzeri. Antimicrob. Agents Chemother. 60, 2551–2553. doi: 10.1128/AAC.02859-15
Idelevich, E. A., Von Eiff, C., Friedrich, A. W., Iannelli, D., Xia, G., Peters, G., et al. (2011). In vitro activity against Staphylococcus aureus of a novel antimicrobial agent, PRF-119, a recombinant chimeric bacteriophage endolysin. Antimicrob. Agents Chemother. 55, 4416–4419. doi: 10.1128/AAC.00217-11
Kapoor, R., and Yadav, J. S. (2010). Development of a rapid ATP bioluminescence assay for biocidal susceptibility testing of rapidly growing mycobacteria. J. Clin. Microbiol. 48, 3725–3728. doi: 10.1128/JCM.01482-10
Keeling, W. B., Myers, A. R., Stone, P. A., Heller, L., Widen, R., Back, M. R., et al. (2009). Regional antibiotic delivery for the treatment of experimental prosthetic graft infections. J. Surg. Res. 157, 223–226. doi: 10.1016/j.jss.2008.06.050
Kuehn, C., Graf, K., Mashaqi, B., Pichlmaier, M., Heuer, W., Hilfiker, A., et al. (2010). Prevention of early vascular graft infection using regional antibiotic release. J. Surg. Res. 164, e185–e191. doi: 10.1016/j.jss.2010.05.029
Lewis, K. (2007). Persister cells, dormancy and infectious disease. Nat. Rev. Microbiol. 5, 48–56. doi: 10.1038/nrmicro1557
Lynch, A. S., and Robertson, G. T. (2008). Bacterial and fungal biofilm infections. Annu. Rev. Med. 59, 415–428. doi: 10.1146/annurev.med.59.110106.132000
Olsen, I. (2015). Biofilm-specific antibiotic tolerance and resistance. Eur. J. Clin. Microbiol. Infect. Dis. 34, 877–886. doi: 10.1007/s10096-015-2323-z
O'toole, G., Kaplan, H. B., and Kolter, R. (2000). Biofilm formation as microbial development. Annu. Rev. Microbiol. 54, 49–79. doi: 10.1146/annurev.micro.54.1.49
Palestrant, D., Holzknecht, Z. E., Collins, B. H., Parker, W., Miller, S. E., and Bollinger, R. R. (2004). Microbial biofilms in the gut: visualization by electron microscopy and by acridine orange staining. Ultrastruct. Pathol. 28, 23–27. doi: 10.1080/01913120490275196
Patterson, J. L., Stull-Lane, A., Girerd, P. H., and Jefferson, K. K. (2010). Analysis of adherence, biofilm formation and cytotoxicity suggests a greater virulence potential of Gardnerella vaginalis relative to other bacterial-vaginosis-associated anaerobes. Microbiology 156, 392–399. doi: 10.1099/mic.0.034280-0
Peeters, E., Nelis, H. J., and Coenye, T. (2008). Comparison of multiple methods for quantification of microbial biofilms grown in microtiter plates. J. Microbiol. Methods 72, 157–165. doi: 10.1016/j.mimet.2007.11.010
Pettit, R. K., Weber, C. A., and Pettit, G. R. (2009). Application of a high throughput Alamar blue biofilm susceptibility assay to Staphylococcus aureus biofilms. Ann. Clin. Microbiol. Antimicrob. 8:28. doi: 10.1186/1476-0711-8-28
Pitts, B., Hamilton, M. A., Zelver, N., and Stewart, P. S. (2003). A microtiter-plate screening method for biofilm disinfection and removal. J. Microbiol. Methods 54, 269–276. doi: 10.1016/S0167-7012(03)00034-4
Romling, U., Kjelleberg, S., Normark, S., Nyman, L., Uhlin, B. E., and Akerlund, B. (2014). Microbial biofilm formation: a need to act. J. Intern. Med. 276, 98–110. doi: 10.1111/joim.12242
Sanz, M., Beighton, D., Curtis, M. A., Cury, J. A., Dige, I., Dommisch, H., et al. (2017). Role of microbial biofilms in the maintenance of oral health and in the development of dental caries and periodontal diseases. Consensus report of group 1 of the joint EFP/ORCA workshop on the boundaries between caries and periodontal disease. J. Clin. Periodontol. 44(Suppl. 18), S5–S11. doi: 10.1111/jcpe.12682
Sasaki, Y. (2014). The in vitro research of bacterial invasion of prosthetic vascular grafts: comparison of elastomer-sealed and gelatin-coated Dacron vascular grafts. Surg. Today 44, 1542–1547. doi: 10.1007/s00595-013-0761-8
Schmitt, D. D., Bandyk, D. F., Pequet, A. J., and Towne, J. B. (1986). Bacterial adherence to vascular prostheses. A determinant of graft infectivity. J. Vasc. Surg. 3, 732–740. doi: 10.1016/0741-5214(86)90037-6
Stepanovic, S., Vukovic, D., Dakic, I., Savic, B., and Svabic-Vlahovic, M. (2000). A modified microtiter-plate test for quantification of staphylococcal biofilm formation. J. Microbiol. Methods 40, 175–179. doi: 10.1016/S0167-7012(00)00122-6
Tawakoli, P. N., Al-Ahmad, A., Hoth-Hannig, W., Hannig, M., and Hannig, C. (2013). Comparison of different live/dead stainings for detection and quantification of adherent microorganisms in the initial oral biofilm. Clin. Oral Investig. 17, 841–850. doi: 10.1007/s00784-012-0792-3
Villain-Guillot, P., Gualtieri, M., Bastide, L., and Leonetti, J. P. (2007). In vitro activities of different inhibitors of bacterial transcription against Staphylococcus epidermidis biofilm. Antimicrob. Agents Chemother. 51, 3117–3121. doi: 10.1128/AAC.00343-07
Keywords: antimicrobial activity, ATP assay, biofilm quantification, colony-forming units (CFU), crystal violet staining (Cry), vascular graft
Citation: Herten M, Bisdas T, Knaack D, Becker K, Osada N, Torsello GB and Idelevich EA (2017) Rapid in Vitro Quantification of S. aureus Biofilms on Vascular Graft Surfaces. Front. Microbiol. 8:2333. doi: 10.3389/fmicb.2017.02333
Received: 18 August 2017; Accepted: 13 November 2017;
Published: 05 December 2017.
Edited by:
Felipe Lombó, Universidad de Oviedo Mieres, SpainReviewed by:
Mathias Schmelcher, ETH Zurich, SwitzerlandArun K. Bhunia, Purdue University, United States
Copyright © 2017 Herten, Bisdas, Knaack, Becker, Osada, Torsello and Idelevich. This is an open-access article distributed under the terms of the Creative Commons Attribution License (CC BY). The use, distribution or reproduction in other forums is permitted, provided the original author(s) or licensor are credited and that the original publication in this journal is cited, in accordance with accepted academic practice. No use, distribution or reproduction is permitted which does not comply with these terms.
*Correspondence: Monika Herten, bW9oZXJ0ZW5Ad2ViLmRl