- 1Department of Clinical and Molecular Medicine, Sant’Andrea University Hospital, Sapienza University of Rome, Rome, Italy
- 2Independent Researcher, Rome, Italy
In higher vertebrates, mucosal sites at the border between the internal and external environments, directly interact with bacteria, viruses, and fungi. Through co-evolution, hosts developed mechanisms of tolerance or ignorance toward some infectious agents, because hosts established “gain of function” interactions with symbiotic bacteria. Indeed, some bacteria assist hosts in different functions, among which are digestion of complex carbohydrates, and absorption and supply of vitamins. There is no doubt that microbiota modulate innate and acquired immune responses starting at birth. However, variations in quality and quantity of bacterial species interfere with the equilibrium between inflammation and tolerance. In fact, correlations between gut bacteria composition and the severity of inflammation were first described for inflammatory bowel diseases and later extended to other pathologies. The genetic background, environmental factors (e.g., stress or smoking), and diet can induce strong changes in the resident bacteria which can expose the intestinal epithelium to a variety of different metabolites, many of which have unknown functions and consequences. In addition, alterations in gut permeability may allow pathogens entry, thereby triggering infection and/or chronic inflammation. In this context, a local event occurring at a mucosal site may be the triggering cause of an autoimmune reaction that eventually involves distant sites or organs. Recently, several studies attributed a pathogenic role to altered oral microbiota in rheumatoid arthritis (RA) and to gut dysbiosis in spondyloarthritis (SpA). There is also growing evidence that different drugs, such as antibiotics and immunosuppressants, can influence and be influenced by the diversity and composition of microbiota in RA and SpA patients. Hence, in complex disorders such RA and SpA, not only the genetic background, gender, and immunologic context of the individual are relevant, but also the history of infections and the structure of the microbial community at mucosal sites should be considered. Here the role of the microbiota and infections in the initiation and progression of chronic arthritis is discussed, as well as how these factors can influence a patient’s response to synthetic and biologic immunosuppressive therapy.
Microbiota and the Immune System
Host–microbe interactions are the result of a co-evolution process, in which both partners have set borders and have developed mechanisms of tolerance or ignorance. In this context, hosts were able to establish a continuous connection with symbiotic bacteria, representing for the host a “gain of function.” Bacteria provide help for different functions, including the digestion of complex carbohydrates as well as the absorption and supply of vitamins (LeBlanc et al., 2013). Worth mentioning is that, in the complex network of interactions of the microbiome, the environment should be taken into account since the outbreak of an infection and/or an inflammatory disease relies on the complex relationships between the microorganisms, the host, and the environment (such as dietary components, pollutants and even drugs) (Kim et al., 2016).
Microbiota are composed of archaea, bacteria, fungi, and viruses forming a complex ecosystem of which bacteria are the most well-characterized member. Any microbial imbalance resulting in a shift (i.e., loss or overgrowth of a species) and/or reduction in microbial diversity is defined as dysbiosis.
The mucosa has a dual function in our body. It works as a physical barrier, promoting pathogen exclusion, and is an absorption site that allows entry of food-derived metabolites and gas exchange. The mucosal immune system is not fully developed when we are born because many of the lymphoid structures, especially in the gut, are formed only upon stimulation through exposure to microorganisms. In fact, mice bred and maintained in germ-free conditions have small Payer’s patches and lack isolated lymphoid follicles (ILF) in their bowel. Furthermore, serum levels of IgM natural antibodies and IgA secreting cells in the spleen and intestine are reduced in germ free mice (Rhee et al., 2004). This phenotype is rescued by gut colonization once the animals are transferred from isolators to conventional housing and food. At birth, colonization plays a crucial role in inducing and shaping the development of the secondary lymphoid organs as well as setting-up thresholds of reactivity for both innate and acquired immune responses. Thus, the neonate microbiota intervenes with the fine balance amongst infection, inflammation, and tolerance that may “imprint” the immune system for life.
Recently, Vatanen et al. (2016) followed-up the gut colonization of children from birth to 3 years of age. Children from Finland, Estonia and Russia were selected who shared a human leukocyte antigen (HLA) distribution typical of individuals at risk for autoimmunity (Vatanen et al., 2016). The study showed that, depending on the lipopolysaccharide (LPS) subtype produced by different Bacteroides species, Toll-like receptor-4 (TLR4) could be either activated or inhibited. This had an impact on tolerance to endotoxin, and, consequently, strongly conditioned the genetic predisposition of the infants to type I diabetes (T1D). Thus, the presence of different LPS subtypes in the gut could partially explain the difference in prevalence of early onset autoimmune diseases in Finland and Estonia compared to Russia. In line with the hygiene hypothesis, this work adds another clue to the correlation between exposure or lack of exposure to specific microorganisms in early life and the increase of autoimmune diseases in western countries.
The microbiota is comprised of both pathogenic and non-pathogenic bacteria, however, this distinction is not absolute. A microorganism may behave as commensal or as pathogenic with regards to the dietary components, nutritional milieu, broad-spectrum antibiotic treatment, co-infection or genetic background of its host. Moreover, even though commensals are by definition not supposed to induce immune responses, and lamina propria-derived antigen-presenting cells traffic preferentially to mucosal-associated lymphoid tissue (MALT) in order to restrict systemic immune responses against the microbiota, it is possible to find antibodies specific to commensals in the serum of healthy people, as well as circulating T cells reactive against non-pathogenic bacteria (Macpherson et al., 1996; Ergin et al., 2011). Also, in specific genetic conditions, such as in individuals with defects in interleukin-17 (IL17) signaling pathway, commensal microorganisms become pathogenic through invasion, causing fungal disease (Puel et al., 2011). Another condition in which commensals may be harmful is in mucosal leakage. Changes of permeability at mucosal sites or altered immune functions strongly condition the persistence of antigenic stimulation, not only at the place of microbial entry but also in faraway locations, such as articular sites (Asquith et al., 2014). Moreover, in frail patients, the administration of Saccharomyces cerevisiae as a probiotic has been anecdotally reported to induce sepsis (Montineri et al., 2008; Eren et al., 2014).
It is worth mentioning here that the microbiota itself plays a role in regulating the growth of opportunistic enteric infections, a mechanism termed colonization resistance (Stecher and Hardt, 2011). Often antibiotic administration, by altering the equilibrium of the gut microbiota, leads to overgrowth of Clostridium difficile. This infection represents, nowadays, a major clinical problem because C. difficile is one of the bacterial species with higher antibiotic resistance. Microbiota transplantation from healthy donors, by rescuing microbial equilibrium, has been one of the most successful strategies to treat serious C. difficile infection (Hand, 2016).
Bacterial products, such as the previously mentioned LPS, are sensed by enterocytes through TLRs. Microbial stimulation of enterocytes induce the production of antimicrobial peptides (AMPs) and the fucosylation of small intestinal epithelial cells by Fucosyltransferase 2 (Fut2), both of which can limit specific infections (Pickard et al., 2014). TLRs regulate innate immune responses but are also crucial for epithelial cell homeostasis at mucosal sites. Altered immune function of TLRs, for example due to different polymorphisms, may change binding to bacterial products, secretion of cytokines or chemokines, which consequently interferes with acquired immune cell generation/migration and inflammation. Thus, it can contribute to the persistence of inflammatory diseases such as rheumatoid arthritis (RA) (Rogier et al., 2015).
In addition to bacteria, bacterial-derived products can also control, directly or indirectly, the function of both epithelial and inflammatory cells. For example, two important metabolites are the short-chain fatty acids (SCFAs) and the lipid mediator prostaglandin E2 (PGE2). Recognition of the SCFAs by innate immune cells is important to modulate inflammation in response not only to intestinal but even to articular damage (Maslowski et al., 2009). Commensals are also able to regulate the inflammatory activity of monocytes, by promoting the release of PGE2 that, in turn, down-modulates the activation of tissue-damaging neutrophils (Grainger et al., 2013).
The gut is the main site for the generation of the two most important T cell populations, the inducible regulatory T cells (iTregs) and CD4IL17-producing cells (Th17), both of which play critical roles in the development and persistence of autoimmune disorders. Colonization of the distal small intestine, by segmented filamentous bacteria (SFB), is fundamental to development of resident lamina propria dendritic cells (DCs) able to release IL6 and IL22 that trigger the loop of Th17-Treg cell in the newborn gut (Ivanov et al., 2009; Maslowski et al., 2009). Although many studies have addressed gut microbiota composition in humans, we still do not know which would be the “best/healthiest” microbial composition for the maintenance of the balance between regulatory (iTregs) and inflammatory (Th17) cells (Hand, 2016). Recent studies revealed that the homeostasis of the colonic Treg compartment relies more on the synergistic effects of different bacterial strains than on a single species of that strain. This observation strongly suggests the importance of the entire bacterial community in shaping the appropriate microenvironment that is able to sustain the production and maintenance of the anti-inflammatory environment (Atarashi et al., 2013).
Oral/Gut Dysbiosis and Arthritis
Rheumatoid arthritis and Spondyloarthritis (SpA) are chronic inflammatory autoimmune diseases potentially leading to quality of life impairment and reduction in life expectancy. RA is characterized by the presence of autoantibodies [rheumatoid factor (RF) and anti-citrullinated protein antibodies (ACPAs)] and is characterized clinically by the emergence of erosive synovitis predominantly involving small joints, such as hands, wrists, and feet. The reported prevalence of the disease is 0.5–1% in the general population (Hayter and Cook, 2012).
Spondyloarthritis is a pleomorphic group of conditions that includes ankylosing spondylitis (AS), psoriatic arthritis (PsA), reactive arthritis (ReA), SpA associated to inflammatory bowel disease (IBD), acute anterior uveitis and axial non-radiographic SpA. The reported prevalence for AS is 2.4/1,000 (Dean et al., 2014). Arthritis is the most frequent extra-intestinal disease manifestation in patients with IBD (range 15–40%); conversely, up to 60% of patients with SpA present subclinical gut inflammation and 10% overt inflammation evolving to Crohn’s disease (Mielants et al., 1995; De Vos et al., 1996; Olivieri et al., 2014).
The evidence of a direct role for the gut microbiota in the development of autoimmune arthritis arises from experimental studies on germ-free mice in which a reduced severity and/or incidence of arthritis has been demonstrated (Carding et al., 2015).
However, in man, a direct correlation between gut dysfunction and systemic inflammation has been clearly established only in AS. Some studies by Ciccia et al. (2012) have identified several alterations of the ileum architecture in AS patients that may be the cause for chronic inflammation, both local and systemic. AS patients showed disruption of the basal membrane with compromised epithelial cell permeability, hyperplasia of goblet cells (with increased mucins production) (Ciccia et al., 2012) and activation of Paneth cells, producing high levels of adenosine monophosphate, such as α-defensin 5 (Ciccia et al., 2010) and proinflammatory cytokines, such as IL23 (Ciccia et al., 2009). These characteristics may be the cause or the consequence of dysbiosis, with changes in the diversity of the commensal bacteria (Costello et al., 2015). Increased intestinal permeability, probably determined by genetic factors such as HLA-B27 expression (Rosenbaum and Davey, 2011; Lin et al., 2014; Bowness, 2015), results in continuous antigenic stimulation with activation of effector T cells. These effector cells do not show a clearly defined Th17, Th1, and/or Th9 polarization, due to the over-expression of an IL23 response by Paneth cells (Ciccia et al., 2009). Worth noting is that IL23 can regulate the maturation of autoreactive Th17 cells but can also induce chronic inflammation by stimulating IL17, IL6, IL8, and tumour necrosis factor (TNF)-α production in neutrophils and macrophages. Furthermore, a link between IL23R polymorphisms and susceptibility to psoriasis, PsA and AS have been shown (Liu et al., 2008).
In patients with PsA, subclinical gut inflammation is characterized by the overexpression of IL9 and the generation of Th9, Th17, and Th22 responses. Th9 cells produced in the gut are found to be increased in the peripheral blood and they are able to migrate, enter synovial tissues and become established. In the meantime, gut-activated Paneth cells express both IL9 and IL9 receptors, creating a positive autocrine loop that perpetuates local inflammation (Ciccia et al., 2016).
Solid data suggest that alterations in the oral microbiota can influence both progression and disease outcome in RA patients (Diamanti et al., 2016; Table 1). It has been speculated that certain species of bacteria, mainly Porphyromonas gingivalis (PG) which may be present in the oral cavity, and are increased in patients affected by periodontitis, can induce loss of tolerance and lead to citrullination of several peptides and proteins (Wegner et al., 2010; Quirke et al., 2014; Ciccia et al., 2016).
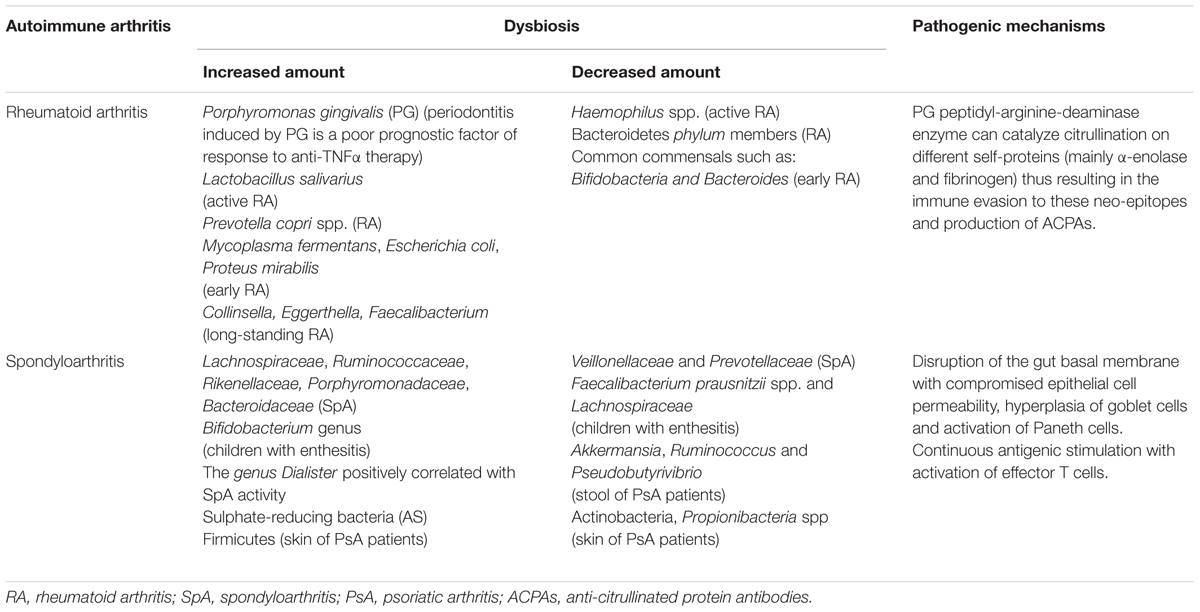
TABLE 1. Most frequently reported dysbiosis in RA and SpA patients and the proposed pathogenic mechanisms.
Citrullination is a post-translational modification that converts arginine residues into citrulline leading to relevant changes in the protein structure, charge and function. Porphyromonas gingivalis peptidyl-arginine-deiminases enzyme (PPAD) can catalyze citrullination on different self-proteins (mainly α-enolase and fibrinogen) thus resulting in immune evasion by these neo-epitopes and the production of ACPAs (McInnes and Schett, 2011).
Indeed, it has been reported a direct correlation exists between the serum level of antibodies to PG and ACPAs in RA patients (Mercado et al., 2001; Dissick et al., 2010; Scher et al., 2012; Lange et al., 2016). This observation has been confirmed by the increased prevalence of PG-induced periodontitis in RA patients as compared to healthy individuals. Likewise, other studies have demonstrated that the presence of periodontitis is a poor prognostic factor in RA patients treated with anti-TNFα agents. The clearing of a periodontal infection can reduce the severity of active RA and lead to a better disease outcome during synthetic and biologic immunosuppressant treatment (Ortiz et al., 2009; Savioli et al., 2012; Kaur et al., 2014).
In line with the above described observations, a case of complete and long-lasting recovery in a patient with early RA after PD treatment has been recently reported, suggesting that, at the onset of disease, periodontitis treatment may avoid the development of a chronic and progressive arthritis (Salemi et al., 2014).
The influence of the intestinal microbiota on RA is still a matter of debate. A lower representation of common commensals such as Bifidobacteria and Bacteroides species (Vaahtovuo et al., 2008) and an increase in Mycoplasma fermentans (Johnson et al., 2000), Escherichia coli (Newkirk et al., 2010) and Proteus mirabilis (Senior et al., 1999) has been reported in early RA patients, whereas another study showed that stool samples from early RA patients had significantly more Lactobacillus communities than healthy subjects (Liu et al., 2013). In patients with long-standing RA a decreased gut microbial diversity seems to correlate with disease duration and autoantibody levels as well as expansion of rare genera, Collinsella, Eggerthella, and Faecalibacterium (Chen et al., 2016). Other authors have reported, in the fecal microbiota of RA patients, an increase of Prevotella copri spp. with concomitant reduction of Bacteroidetes phylum members (Scher et al., 2013). Recently Zhang et al. (2015) described a dysbiosis of both fecal and oral samples from RA patients using metagenomic shotgun sequencing and a metagenome-wide association study; these concordant abnormalities in mouth and gut microflora were restored after immunosuppressive treatment. In particular, Haemophilus spp. was strongly reduced, whereas Lactobacillus salivarius was increased especially in oral microbiota of patients with active RA disease (Zhang et al., 2015; Table 1).
The role of the gastrointestinal tract in the pathogenesis of SpA was proposed by Costello et al. (2015) who have evaluated biopsy specimens from 9 early SpA patients finding a higher abundance of five families of bacteria (Lachnospiraceae, Ruminococcaceae, Rikenellaceae, Porphyromonadaceae, and Bacteroidaceae), accompanied by a reduction of Veillonellaceae and Prevotellaceae with respect to healthy controls. Tito et al analyzed the gut microbiota (colon biopsy specimens) of 27 patients with SpA and 15 healthy controls and showed that the intestinal inflammation was associated with the mucosal microbiota composition. Of particular interest, they found that the genus Dialister positively correlated with the SpA activity (Tito et al., 2016). Stebbings et al. (2002) were the first group that reported differences in the fecal samples of AS patients compared to healthy controls by using molecular analysis. They did not find significant differences in the microbiota composition but did find a higher proportion of sulfate-reducing bacteria in AS patients (Stebbings et al., 2002). Fecal samples and blood specimens obtained from children with enthesitis showed less Faecalibacterium prausnitzii spp. and Lachnospiraceae family members and a significant increase in the Bifidobacterium genus (Stoll et al., 2014). Finally, other authors have reported a significant increase in Firmicutes associated with a reduction in members of Actinobacteria phylum and Propionibacteria spp in the skin samples of patients with psoriasis, compared to healthy controls (Fahlén et al., 2012). The gut microbiota observed in patients with PsA also showed limited diversity compared to healthy controls. In particular, it has been demonstrated that there is a decrease in the bacterial genus Akkermansia, Ruminococcus, and Pseudobutyrivibrio in fecal samples from PsA patients (Scher et al., 2015; Table 1).
There is a growing awareness that the microbiota diversity and composition can influence a patient’s response to synthetic and biologic immunosuppressive therapy. Immunosuppressive drugs, such as cyclophosphamide and methotrexate (MTX), can induce a diffuse depletion of the gut microbiota associated with a decrease in the commensal anaerobic species in favor of potential pathogens that can damage the gut barrier, altering epithelial cell permeability with consequent bacterial translocation (Viaud et al., 2013; Karin et al., 2014). Different authors have underlined the importance of an intact intestinal microbiota in the response to sulphasalazine (SSA), a synthetic drug frequently used in autoimmune arthritis. They found a decreased efficacy of SSA therapy in patients with ulcerative colitis previously treated with severe antibiotic regimens (Das et al., 1973; Peppercorn and Goldman, 1972). Conversely, the use of a multi-strain probiotic regimen does not seem to interfere with the metabolism of SSA in RA patients (Lee et al., 2010).
By now, data on the impact of microbiota in patients receiving immunosuppressive biologics are limited and only refer to IBD patients. One research group has found a reduction in the bacterial species Firmicutes phylum in patients affected by relapsing Crohn’s disease as compared to clinically stable patients with administration of anti-TNFα agents. This modification in gut microbiota was associated with a reduction in F. prausnitzii spp. predicting clinical relapse of the Crohn’s disease (Rajca et al., 2014). More recently, Busquets et al. (2015) have demonstrated that adalimumab (fully human anti-TNFα monoclonal antibody) could partially restore a healthy microbiota in a small group of patients affected by Crohn’s disease. Treatment resulted in the recovery of Firmicutes, Bacteroides and Actinobacteria diversity species whereas E. coli spp. was decreased (Busquets et al., 2015).
Infections and Arthritis
In autoimmune arthritis, such as RA and SpA, the hypothesis of an infectious event as the cause for disease induction and relapse has been under consideration for a long time. Arleevskaya et al. (2014) have shown during a 10-year follow-up study that minor infections are more frequent and prolonged in RA patients and their relatives than in families without a history of autoimmunity. This study suggests a role for some predisposing defects of anti-bacterial defense mechanisms, more than for a specific microorganism. However, although by now no causative pathogen has been clearly identified nor a direct correlation with a specific infection has been established, it does not imply its absence. In fact, many pathogens have been indicated as possible triggers for RA disease but heterogeneity of the studies impairs forming any conclusions (Arleevskaya et al., 2016).
The most intriguing and supported idea for a role of infection in triggering RA, is that the microorganism can enter to and be located in the intestine, lungs or mouth; where it can interact with environmental factors such as smoke and drugs and, with a genetic predisposition, lead to mucosal inflammation. This, in turn, results in increased immune activation and spreading of inflammatory mediators, which locally perpetuate the disease. Exacerbated immune activation may also lead to aberrant migration of intestinal macrophages and lymphocytes from inflamed mucosa to the joints (Salmi et al., 1995).
The complex and interwoven link between microbes and joint inflammation is a frequent challenge for clinicians.
A severe increase in the serologic inflammatory parameters (i.e., erythrocyte sedimentation rate and C reactive protein), induced by a concomitant infection in a patient with autoimmune arthritis under immunosuppressive therapy is commonly encountered in the rheumatologic clinical practice. Indeed, the stimulation of systemic inflammation induced by the infectious pathogen can resemble a reactivation of the autoimmune arthritis, leading clinicians to increase the immunosuppressive therapy with a consequent worsening and dissemination of the infection.
The link between microbes and arthritis also has a broad spectrum of clinical expression. In fact, it can range from septic arthritis, in which the pathogen is directly responsible for joint inflammation and damage, to ReA. In the latter, infections by microorganisms, mainly Gram negative, such as Chlamydia trachomatis, Yersinia, Salmonella, Shigella, and Campylobacter (Espinoza and García-Valladares, 2013), find a genetically predisposed background (i.e., HLAB27) eventually resulting in chronic inflammation. However, the mechanism underlying the immune stimulation and consequent migration to distant synovial tissue is poorly understood (Keat et al., 1978; Granfors et al., 1990; Merilahti-Palo et al., 1991). It is worth mentioning that T cell clones specific for enteric bacteria have been found in synovial tissue of patients with ReA (Hermann et al., 1990; Probst et al., 1993).
It can be argued that the early recognition and eradication of the infective focus can lead to a resolution of the inflammatory stimulus and consequent interruption of the chronic immune loop. This can be the case of periodontal treatment in early RA, antibiotic therapy for beta-hemolytic Streptococcus in rheumatic fever and anti-hepatitis C virus (HCV) treatment for mixed cryoglobulinemia.
Recently, Campisi et al. (2016) using a complex and elegant set of experiments in mice, demonstrated that microbial infection at mucosal sites can elicit a break in tolerance by promoting the presentation of self-antigens derived from epithelial cell apoptotic bodies. Apoptosis of infected colonic cells leads to the generation of both anti-pathogen and autoreactive T cells, mainly Th17 cells, with production of auto-antibodies and inflammation (Campisi et al., 2016). These results have important implications for understanding how infections can stimulate the development of autoimmunity in an immunological context different from bystander activation of autoreactive T cells or epitope mimicry. Further studies are needed to establish similar correlations in humans.
The risk of infections in autoimmune patients is higher than in healthy individuals, due to endogenous (dysfunctional immune system) and external factors (i.e., comorbidities and immunosuppressive therapy). The risk has been reported to be approximately double for infections of bone and joints, skin, soft tissues and respiratory tract (Myllykangas-Luosujarvi et al., 1995). Moreover, it is known that infections can induce disease relapses, present a more severe clinical outcome and can be a frequent cause of death in immune-suppressed subjects (Gonzalez et al., 2007).
Immunosuppressive treatment is the main exogenous factor contributing to the increased risk of infections in autoimmune patients. TNFα blocking agents are the first biological immunosuppressive therapy approved for both RA and SpA. Currently five different drugs targeting TNFα are available: infliximab (the first antibody to be approved) is a chimeric monoclonal antibody (mAb) with approximately one third murine and two thirds human sequences; adalimumab, a fully human mAb produced by chinese hamster ovary (CHO) cells; golimumab a fully human mAb; certolizumab pegol, a poly-etyhlene-glycol (PEG)ylated humanized Fab’ fragment; and etanercept a fusion protein that consists of the p75 part of TNFR2 and a human IgG1 Fc domain (Salemi et al., 2015; Dieterich et al., 2016). Although these agents have shown a good safety and efficacy profile, up to 40% of the patients may present a primary or secondary drug failure or treatment-related adverse events (Day, 2002).
In the last decade, several research groups have studied in depth the molecular pathways involved in the development of autoimmune arthritis, allowing identification of new therapeutic targets. Among those, the anti-CD20 chimeric mAb (rituximab) (de la Torre et al., 2012); the humanized anti-human IL6 receptor mAb (tocilizumab) (Nishimoto et al., 2007), and the CTLA-4-Ig (abatacept), a fusion protein that works as an inhibitor of T cell activation (Moreland et al., 2006), are currently available for RA patients. More recently, mAb against the p40 subunit of interleukin 12/23 ustekinumab (Gottlieb et al., 2009) and the mAb against the IL17A, secukinumab have been introduced for the treatment of patients with SpA (Patel et al., 2013).
Different authors have evaluated the infection risk induced by corticosteroids (CS) as well as synthetic and biologic immunosuppressant drugs in SpA and RA patients. Studies generally showed that synthetic immunosuppressive therapy (mainly MTX), is relatively safe, whereas data on the possible increase in the number and/or severity of infections in patients taking TNFα blocking agents are contrasting. Doran et al. (2002) showed that the use of CS, older age, extra-articular manifestations of RA, leukopenia, and comorbidities, are strong predictors of infections, whereas the use of synthetic immunosuppressive agents was not associated with increased risk. These results were partially confirmed by Lacaille et al. (2008), who reported an increased risk of infections in RA patients taking CS, but not synthetic immunosuppressive therapy. Data on the infection risk for biologic drugs derives mainly from national registries among which are the British Biologics Register and the RABBIT German Registry. Data from these registries estimated a two-fold increased risk of serious infections which was directly related to the dose of CS, mainly when CS were associated with TNFα inhibitors (Dixon et al., 2006; Zink et al., 2014). Results obtained from meta-analysis studies have also indicated a significant increase in the occurrence of serious infections (40%), in patients receiving anti-TNFα agents, while the data for opportunistic infections were not conclusive (Bongartz et al., 2006; Minozzi et al., 2016).
We have recently described, in a retrospective observational cohort study, that the combination of anti-TNFα with CS appears to be the most “pro-infective” treatment, whereas synthetic immunosuppressant drugs, either alone or associated with CS, were relatively safe in both patients with RA or with SpA (Germano et al., 2014). In contrast with these real-life data, the placebo-controlled trials evaluating TNFα blockers in RA patients, did not find a higher rate of infections in treated patients with respect to the placebo group (Lipsky et al., 2000; Klareskog et al., 2004; Weinblatt et al., 2005).
Conclusion
Patients with autoimmune arthritis are at high risk of infections, due to endogenous (dysfunctional immune system) and external factors (i.e., comorbidities, drugs).
Infections can induce disease relapses and be characterized by a severe clinical outcome in these patients, representing a frequent cause of death. Furthermore, the stimulation of systemic inflammation induced by the infectious pathogen, mimicking a reactivation of the autoimmune arthritis, frequently represents a confounding and dangerous factor for clinicians.
In the last few years the efforts of several researchers have focused on the gut and mouth microflora leading to the identification of specific abnormalities in both its composition and diversity. In susceptible individuals, the presence of PG could be a trigger factor for RA development by inducing the generation of citrullinated proteins via a PPAD enzyme on different self-proteins that results in the production of ACPAs. In SpA patients the increased intestinal permeability, probably induced by genetic factors (e.g., HLA-B27) could lead to a disruption of the basal membrane, hyperplasia of goblet cells and activation of Paneth cells producing high levels of AMPs and IL23. It would result in a continuous antigenic stimulation with activation of effector T cells.
Careful monitoring for infectious foci, especially in the mouth and intestine, should be recommended in order to allow their early recognition and eradication, thus resulting in removal of the inflammatory stimulus, inhibition of the chronic immune loop and improvement of the arthritis.
Author Contributions
AP-D and MR drafted the manuscript and performed the revision of the literature. RD critically revised the manuscript giving important intellectual contribution.
Conflict of Interest Statement
The authors declare that the research was conducted in the absence of any commercial or financial relationships that could be construed as a potential conflict of interest.
References
Arleevskaya, M. I., Gabdoulkhakova, A. G., Filina, Y. V., Miftakhova, R. R., Bredberg, A., and Tsybulkin, A. P. (2014). A transient peak of infections during onset of rheumatoid arthritis: a 10-year prospective cohort study. BMJ Open 4:e005254. doi: 10.1136/bmjopen-2014-005254
Arleevskaya, M. I., Kravtsova, O. A., Lemerle, J., Renaudineau, Y., and Tsibulkin, A. P. (2016). How rheumatoid arthritis can result from provocation of the immune system by microorganisms and viruses. Front. Microbiol. 7:1296 doi: 10.3389/fmicb.2016.01296
Asquith, M., Elewaut, D., Lin, P., and Rosenbaum, J. T. (2014). The role of the gut and microbes in the pathogenesis of spondyloarthritis. Best Pract. Res. Clin. Rheumatol. 28, 687–702. doi: 10.1016/j.berh.2014.10.018
Atarashi, K., Tanoue, T., Oshima, K., Suda, W., Nagano, Y., Nishikawa, H., et al. (2013). Treg induction by a rationally selected mixture of Clostridia strains from the human microbiota. Nature 500, 232–236. doi: 10.1038/nature12331
Bongartz, T., Sutton, A. J., Sweeting, M. J., Buchan, I., Matteson, E. L., and Montori, V. (2006). Anti-TNF antibody therapy in rheumatoid arthritis and the risk of serious infections and malignancies: systematic review and meta-analysis of rare harmful effects in randomized controlled trials. JAMA 295, 2275–2285. doi: 10.1001/jama.295.19.2275
Bowness, P. (2015). HLA-B27. Ann. Rev. Immunol. 33, 29–48. doi: 10.1146/annurev-immunol-032414-112110
Busquets, D., Mas-de-Xaxars, T., López-Siles, M., Martínez-Medina, M., Bahí, A., et al. (2015). Anti-tumour necrosis factor. treatment with adalimumab induces changes in the microbiota of Crohn’s disease. J. Crohns Colitis 9, 899–906 doi: 10.1093/ecco-jcc/jjv119
Campisi, L., Barbet, G., Ding, Y., Esplugues, E., Flavell, R. A., and Blander, J. M. (2016). Apoptosis in response to microbial infection induces autoreactive TH17 cells. Nat. Immunol. 17, 1084–1092. doi: 10.1038/ni.3512
Carding, A., Verbeke, K., Vipond, D. T., Corfe, B. M., and Owen, L. J. (2015). Dysbiosis of the gut microbiota in disease. Microb. Ecol. Health Dis. 26:26191 doi: 10.3402/mehd.v26.26191
Chen, J., Wright, K., Davis, J. M., Jeraldo, P., Marietta, E. V., Murray, J., et al. (2016). An expansion of rare lineage intestinal microbes characterizes rheumatoid arthritis. Genome Med. 8:43. doi: 10.1186/s13073-016-0299-7
Ciccia, F., Accardo-Palumbo, A., Alessandro, R., Rizzo, A., Principe, S., Peralta, S., et al. (2012). Interleukin-22 and interleukin-22-producing NKp44Ϸ NK cells in the subclinical gut inflammation of patients with ankylosing spondylitis. Arthritis Rheum. 64, 1869–1878. doi: 10.1002/art.34355
Ciccia, F., Bombardieri, M., Principato, A., Giardina, A., Tripodo, C., Porcasi, R., et al. (2009). Overexpression of interleukin-23, but not interleukin-17, as an immunologic signature of subclinical intestinal inflammation in ankylosing spondylitis. Arthritis Rheum. 60, 955–965. doi: 10.1002/art.24389
Ciccia, F., Bombardieri, M., Rizzo, A., Principato, A., Giardina, A. R., Raiata, F., et al. (2010). Over expression of paneth cell derived antimicrobial peptides in the gut of patients with ankylosing spondylitis and subclinical intestinal inflammation. Rheumatology 49, 2076–2083. doi: 10.1093/rheumatology/keq239
Ciccia, F., Guggino, G., Ferrante, A., Raimondo, S., Bignone, R., Rodolico, V., et al. (2016). Interleukin-9 overexpression and Th9 polarization characterize the inflamed gut,the synovial tissue, and the peripheral blood of patients with psoriatic arthritis. Arthritis Rheumatol. 68, 1922–1931. doi: 10.1002/art.39649
Costello, M. E., Ciccia, F., Willner, D., Warrington, N., Robinson, P. C., Gardiner, B., et al. (2015). Intestinal dysbiosis in ankylosing & spondylitis. Arthritis Rheumatol. 67, 686–691. doi: 10.1002/art.38967
Das, K. M., Eastwood, M. A., McManus, J. P., and Sircus, W. (1973). The metabolism of salicylazosulphapyridine in ulcerative colitis. I. The relationship between metabolites and the response to treatment in inpatients. Gut 14, 631–641
Day, R. (2002). Adverse reactions to TNF-alpha inhibitors in rheumatoid arthritis. Lancet 359, 540–541. doi: 10.1016/S0140-6736(02)07718-8
de la Torre, I., Leandro, M. J., Edwards, J. C., and Cambridge, G. (2012). Baseline serum immunoglobulin levels in patients with rheumatoid arthritis: relationships with clinical parameters and with B-cell dynamics following rituximab. Clin. Exp. Rheumatol. 30, 554–560.
De Vos, M., Mielants, H., Cuvelier, C., Elewaut, A., and Veys, E. (1996). Long term evolution of gut inflammation in patients with spondyloarthropathy. Gastroenterology 110, 1696–1703. doi: 10.1053/gast.1996.v110.pm8964393
Dean, L. E., Jones, G. T., MacDonald, A. G., Downham, C., Sturrock, R. D., and Macfarlane, G. J. (2014). Global prevalence of ankylosing spondylitis. Rheumatology 53, 650–657. doi: 10.1093/rheumatology/ket387
Diamanti, A. P., Manuela Rosado, M., Laganà, B., and D’Amelio, R. (2016). Microbiota and chronic inflammatory arthritis: an interwoven link. J. Transl. Med. 14:233 doi: 10.1186/s12967-016-0989-3
Dieterich, W., Neurath, M. F., and Atreya, R. (2016). Molecular mechanism of action of anti-tumor necrosis factor antibodies in inflammatory bowel diseases. World J. Gastroenterol. 22, 9300–9313
Dissick, A., Redman, R. S., Jones, M., Rangan, B. V., Reimold, A., Griffiths, G. R., et al. (2010). Association of periodontitis with rheumatoid arthritis: a pilot study. J. Periodontol. 81, 223–230. doi: 10.1902/jop.2009.090309
Dixon, W. G., Watson, K., and Lunt, M. (2006). Rates of serious infection, including site-specific and bacterial intracellular infection, in rheumatoid arthritis patients receiving anti-tumor necrosis factor therapy. Results from the British society for rheumatology biologics register. Arthritis Rheum. 54, 2368–2376
Doran, M. F., Crowson, C. S., Pond, G. R., O’Fallon, W. M., and Gabriel, S. E. (2002). Predictors of infection in Rheumatoid arthritis. Arthritis Rheum. 49, 2294–2300. doi: 10.1002/art.10529
Eren, Z., Gurol, Y., Sonmezoglu, M., Eren, H. S., Celik, G., and Kantarci, G., (2014). Saccharomyces cerevisiae fungemia in an elderly patient following probiotic treatment. Mikrobiyol. Bul. 48, 351–355 doi: 10.5578/mb.6970
Ergin, A., Syrbe, U., and Scheer, R. et al. (2011). Impaired peripheral Th1 CD4+ T cell response to Escherichia coli proteins in patients with Crohn’s disease and ankylosing spondylitis. J. Clin. Immunol. 31, 998–1009. doi: 10.1007/s10875-011-9575-x
Espinoza, L. R., and García-Valladares, I. (2013). Of bugs and joints: the relationship between infection and joints. Reumatol Clin. 9, 229–238 doi: 10.1016/j.reuma.2012.06.008
Fahlén, A., Engstrand, L., Baker, B. S., Powles, A., and Fry, L. (2012). Comparison of bacterial microbiota in skin biopsies from normal and psoriatic skin. Arch. Dermatol. Res. 304, 15–22. doi: 10.1007/s00403-011-1189-x
Germano, V., Cattaruzza, M. S., Osborn, J., Tarantino, A., Di Rosa, R., Salemi, S., et al. (2014). Infection risk in rheumatoid arthritis and spondyloarthropathy patients under treatment with DMARDs, corticosteroids and TNF-α antagonists. J. Transl. Med. 12:77 doi: 10.1186/1479-5876-12-77
Gonzalez, A., Kremers, M. H., Crowson, C. S., Nicola, P. J., Davis, J. M., III., Therneau, T. M. et al. (2007). The widening mortality gap, between rheumatoid arthritis patients and the general population. Arthritis Rheum. 56, 3583–3587. doi: 10.1002/art.22979
Gottlieb, A., Menter, A., Mendelsohn, A., Shen, Y. K., Li, S., Guzzo, C., et al. (2009). Ustekinumab, a human interleukin 12/23 monoclonal antibody, for psoriatic arthritis: randomised, double-blind, placebo-controlled, crossover trial. Lancet 373, 633–640. doi: 10.1016/S0140-6736(09)60140-9
Grainger, J. R., Wohlfert, E. A., Fuss, I. J., Bouladoux, N., Askenase, M. H., Legrand, F., et al. (2013). Inflammatory monocytes regulate pathologic responses to commensals during acute gastrointestinal infection. Nat. Med. 19, 713–721. doi: 10.1038/nm.3189
Granfors, K., Jalkanen, S., Lindberg, A. A., Mäki-Ikola, O., von Essen, R., Lahesmaa-Rantala, R., et al. (1990). Salmonella lipopolysaccharide in synovial cells from patients with reactive arthritis. Lancet 335, 685–688; doi: 10.1016/0140-6736(90)90804-E
Hand, T. W. (2016). The role of the microbiota in shaping infectious immunity. Trends Immunol. 37, 647–658. doi: 10.1016/j.it.2016.08.007
Hayter, S. M., and Cook, M. C. (2012). Updated assessment of the prevalence, spectrum and case definition of autoimmune disease. Autoimmun. Rev. 11, 754–765. doi: 10.1016/j.autrev.2012.02.001
Hermann, E., Mayet, W. J., Poralla, T., Meyer zum Büschenfelde, K. H., and Fleischer, B. (1990). Salmonella-reactive synovial fluid T-cell clones in a patient with post-infectious Salmonella arthritis. Scand. J. Rheumatol. 19, 350–355 doi: 10.3109/03009749009096790
Ivanov, I. I., Atarashi, K., and Manel, N., Brodie, E. L., Shima, T., Karaoz, U., et al. (2009). Induction of intestinal Th17 cells by segmented filamentous bacteria. Cell 139, 485–498. doi: 10.1016/j.cell.2009.09.033
Johnson, S., Sidebottom, D., Bruckner, F., and Collins, D. (2000). Identification of Mycoplasma fermentans in synovial fluid samples from arthritis patients with inflammatory disease. J. Clin. Microbiol. 38, 90–93.
Karin, M., Jobin, C., and Balkwill, F. (2014). Chemotherapy, immunity and microbiota–a new triumvirate? Nat. Med. 20, 126–127. doi: 10.1038/nm.3473
Kaur, S., Bright, R., Proudman, S. M., and Bartold, P. M. (2014). Does periodontal treatment influence clinical and biochemical measures for rheumatoid arthritis? A systematic review and meta-analysis. Semin. Arthritis Rheum. 44, 113–122. doi: 10.1016/j.semarthrit.2014.04.009
Keat, A. C., Maini, R. N., Nkwazi, G. C., Pegrum, G. D., Ridgway, G. L., and Scott, J. T. (1978). Role of Chlamydia trachomatis and HLA-B27 in sexually acquired reactive arthritis. Br. Med. J. 1, 605–607 doi: 10.1136/bmj.1.6113.605
Kim, K. S., Hong, S. W., Han, D., Yi, J., Jung, J., Yang, B. G., Lee, J. Y., et al. (2016). Dietary antigens limit mucosal immunity by inducing regulatory T cells in the small intestine. Science 351, 858–863. doi: 10.1126/science.aac5560
Klareskog, L., van der Heijde, D., De Jager, J. P., Gough, A., Kalden, J., Malaise, M., et al. (2004). Therapeutic effect of the combination of etanercept and methotrexate compared with each treatment alone in patients with rheumatoid arthritis: double-blind randomised controlled trial. Lancet 363, 675–681. doi: 10.1016/S0140-6736(04)15640-7
Lacaille, D., Guh, D. P., Abrahamowicz, M., Anis, A. H., and Esdaile, J. M. (2008). Use of non biologic disease-modifying antirheumatic drugs and risk of infection in patients with rheumatoid arthritis. Arthritis Rheum. 59, 1074–1081. doi: 10.1002/art.23913
Lange, L., Thiele, G. M., McCracken, C., Wang, G., Ponder, L. A., Angeles-Han, S. T., et al. (2016). Symptoms of periodontitis and antibody responses to Porphyromonas gingivalis in juvenile idiopathic arthritis. Pediatr. Rheumatol. Online J. 14:8. doi: 10.1186/s12969-016-0068-6
LeBlanc, J. G., Milani, C., de Giori, G. S., Sesma, F., van Sinderen, D., and Ventura, M. (2013). Bacteria as vitamin suppliers to their host: a gut microbiota perspective. Curr. Opin. Biotechnol. 24, 160–168. doi: 10.1016/j.copbio.2012.08.005
Lee, H. J., Waller, R. D., Stebbings, S., Highton, J., Orlovich, D. A., Schmierer, D., et al. (2010). The effects of an orally administered probiotic on sulfasalazine metabolism in individuals with rheumatoid arthritis: a preliminary study. Int. J. Rheum. Dis. 13, 48–54 doi: 10.1111/j.1756-185X.2009.01449.x
Lin, P., Bach, M., Asquith, M., Lee, A. Y., Akileswaran, L., Stauffer, P., et al. (2014). HLA-B27 and human beta2-microglobulin affect the gut microbiota of transgenic rats. PLOS ONE 9:e105684. doi: 10.1371/journal.pone.0105684
Lipsky, P. E., van der Heijde, D. M., St Clair, E. W., Furst, D. E., Breedveld, F. C., Kalden, J. R., et al. (2000). Anti-tumor necrosis factor trial in rheumatoid arthritis with concomitant therapy study group infliximab and methotrexate in the treatment of rheumatoid arthritis. N. Engl. J. Med. 343:1594–1602.
Liu, X., Zou, Q., Zeng, B., Fang, Y., and Wei, H. (2013). Analysis of fecal lactobacillus community structure in patients with early rheumatoid arthritis. Curr. Microbiol. 67, 170–176. doi: 10.1007/s00284-013-0338-1
Liu, Y., Helms, C., Liao, W., Zaba, L. C., Duan, S., Gardner, J., et al. (2008). A genome-wide association study of psoriasis and psoriatic arthritis identifies new disease loci. PLOS Genet. 4:e1000041 doi: 10.1371/journal.pgen.1000041
Macpherson, A., Khoo, U. Y., Forgacs, I., Philpott-Howard, J., and Bjarnason, I. (1996). Mucosal antibodies in inflammatory bowel disease are directed against intestinal bacteria. Gut 38, 365–375. doi: 10.1136/gut.38.3.365
Maslowski, K. M., Vieira, A. T., Ng, A., Kranich, J., Sierro, F., Yu, D., et al. (2009). Regulation of inflammatory responses by gut microbiota and chemoattractant receptor GPR43. Nature 461, 1282–1286 doi: 10.1038/nature08530
McInnes, I. B., and Schett, G. (2011). The pathogenesis of rheumatoid arthritis. N. Engl. J. Med. 365, 2205–2219. doi: 10.1056/NEJMra1004965
Mercado, F. B., Marshall, R. I., Klestov, A. C., and Bartold, P. M. (2001). Relationship between rheumatoid arthritis and periodontitis. J. Periodontol. 72, 779–787. doi: 10.1902/jop.2001.72.6.779
Merilahti-Palo, R., Söderström, K. O., Lahesmaa-Rantala, R., Granfors, K., and Toivanen, A. (1991). Bacterial antigens in synovial biopsy specimens in yersinia triggered reactive arthritis. Ann. Rheum. Dis. 50, 87–90 doi: 10.1136/ard.50.2.87
Mielants, H., Veys, E. M., Cuvelier, C., De Vos, M., Goemaere, S., De Clercq, L., et al. (1995). The evolution of spondyloarthropathies in relation to gut histology. II. Histological aspects. J. Rheumatol. 22, 2273–2278.
Minozzi, S., Bonovas, S., Lytras, T., Pecoraro, V., González-Lorenzo, M., Bastiampillai A. J., et al. (2016). Risk of infections using anti-TNF agents in rheumatoid arthritis, psoriatic arthritis, and ankylosing spondylitis: a systematic review and meta-analysis. Expert Opin. Drug Saf. 15 (Suppl.1), 11–34. doi: 10.1080/14740338.2016.1240783
Montineri, A., Iacobello, C., Larocca, L., La Rosa, R., Nigro, L., and Fatuzzo, F. (2008). Saccharomyces cerevisiae fungemia associated with multifocal pneumonia in a patient with alcohol-related hepatic cirrhosis. Infez. Med. 16, 227–229.
Moreland, L., Bate, G., and Kirkpatrick, P. (2006). Abatacept. Nat. Rev. Drug Discov. 5, 185–186. doi: 10.1038/nrd1989
Myllykangas-Luosujarvi, R., Aho, K., Kautiainen, H., and Isomaki, H. (1995). Shortening of life span and causes of excess mortality in a population-based series of subjects with rheumatoid arthritis. Clin. Exp. Rheumatol. 13, 149–153.
Newkirk, M. M., Zbar, A., Baron, M., Manges, A. R. (2010). Distinct bacterial colonization patterns of Escherichia coli subtypes associate with rheumatoid factor status in early inflammatory arthritis. Rheumatology (Oxford) 49, 1311–1316. doi: 10.1093/rheumatology/keq088
Nishimoto, N., Hashimoto, J., Miyasaka, N., Yamamoto, K., Kawai, S., Takeuchi, T., et al. (2007). Study of active controlled monotherapy used for rheumatoid arthritis, an IL-6 inhibitor (SAMURAI): evidence of clinical and radiographic benefit from an x-ray reader-blinded radnomised controlled trial of tocilizumab. Ann. Rheum. Dis. 66, 1162–1167. doi: 10.1136/ard.2006.068064
Olivieri, I., Cantini, F., Castiglione, F., Felice, C., Gionchetti, P., Orlando, A., et al. (2014). Italian Expert Panel on the management of patients with coexisting spondyloarthritis and inflammatory bowel disease. Autoimmun. Rev. 13, 822–830. doi: 10.1016/j.autrev.2014.04.003
Ortiz, P., Bissada, N. F., Palomo, L., Han, Y. W., Al-Zahrani, M. S., Panneerselvam, A., et al. (2009). Periodontal therapy reduces the severity of active rheumatoid arthritis in patients treated with or without tumor necrosis factor inhibitors. J. Periodontol. 80, 535–540. doi: 10.1902/jop.2009.080447
Patel, D. D., Lee, D. M., Kolbinger, F., and Antoni, C. (2013). Effect of IL-17A blockade with secukinumab in autoimmune diseases. Ann. Rheum. Dis. 72(Suppl. 2), 116–123. doi: 10.1136/annrheumdis-2012-202371
Peppercorn, M. A., and Goldman, P. (1972). The role of intestinal bacteria in the metabolism of salicylazosulfapyridine. J. Pharmacol. Exp. Ther. 181, 555–562.
Pickard, J. M., Maurice, C. F., Kinnebrew, M. A., Abt, M. C., Schenten, D., Golovkina, T. V., et al. (2014). Rapid fucosylation of intestinal epithelium sustains host-commensal symbiosis in sickness. Nature 514:638–641 doi: 10.1038/nature13823
Probst, P., Hermann, E., Meyer zum Büschenfelde, K. H., and Fleischer, B. (1993). Multiclonal synovial T cell response to Yersinia enterocolitica in reactive arthritis: the Yersinia 61-kDa heat-shock protein is not the major target antigen. J. Infect. Dis. 167, 385–391
Puel, A., Cypowyj, S., Bustamante, J., Wright, J. F., Liu, L., Lim, H. K., et al. (2011). Chronic mucocutaneous candidiasis in humans with inborn errors of interleukin-17 immunity. Science 332, 65–68. doi: 10.1126/science.1200439
Quirke, A. M., Lugli, E. B., Wegner, N., Hamilton, B. C., Charles, P., Chowdhury, M., et al. (2014). Heightened immune response to autocitrullinated Porphyromonas gingivalis peptidylarginine deiminase: a potential mechanism for breaching immunologic tolerance in rheumatoid arthritis. Ann. Rheum. Dis. 73, 263–269. doi: 10.1136/annrheumdis-2012-202726
Rajca, S., Grondin, V., Louis, E., Vernier-Massouille, G., Grimaud, J. C., Bouhnik, Y., et al. (2014). Alterations in the intestinal microbiome (dysbiosis) as a predictor of relapse after infliximab withdrawal in Crohn’s disease. Inflamm. Bowel Dis. 20, 978–986. doi: 10.1097/MIB.0000000000000036
Rhee, K. J., Sethupathi, P., Driks, A., Lanning, D. K., and Knight, K. L. (2004). Role of commensal bacteria in development of gut-associated lymphoid tissues and preimmune antibody repertoire. J. Immunol. 172, 1118–1124. doi: 10.4049/jimmunol.172.2.1118
Rogier, R., Koenders, M. I., and Abdollahi-Roodsaz, S. (2015). Toll-like receptor mediated modulation of T cell response by commensal intestinal microbiota as a trigger for autoimmune arthritis. J. Immunol. Res. 2015:527696. doi: 10.1155/2015/527696
Rosenbaum, J. T., and Davey, M. P. (2011). Time for a gut check: evidence for the hypothesis that HLA-B27 predisposes to ankylosing spondylitis by altering the microbiome. Arthritis Rheum. 63, 3195–3198. doi: 10.1002/art.30558
Salemi, S., Biondo, M. I., Fiorentino, C., Argento, G., Paolantonio, M., Di Murro, C., et al. (2014). Could early rheumatoid arthritis resolve after periodontitis treatment only? Case report and review of the literature. Medicine 93:e195. doi: 10.1097/MD.0000000000000195
Salemi, S., Markovic, M., Martini, G., and D’Amelio, R. (2015). The expanding role of therapeutic antibodies. Int. Rev. Immunol. 34, 202–264 doi: 10.3109/08830185.2013.863304
Salmi, M., Andrew, D. P., Butcher, E. C., and Jalkanen, S. (1995). Dual binding capacity of mucosal immunoblasts to mucosal and synovial endothelium in humans: dissection of the molecular mechanisms. J. Exp. Med. 181, 137–149. doi: 10.1084/jem.181.1.137
Savioli, C., Ribeiro, A. C., Fabri, G. M., Calich, A. L., Carvalho, J., Silva, C. A., et al. (2012). Persistent periodontal disease hampers anti-tumor necrosis factor treatment response in rheumatoid arthritis. J. Clin. Rheumatol. 18, 180–184. doi: 10.1097/RHU.0b013e31825828be
Scher, J. U., Sczesnak, A., Longman, R. S., Segata, N., Ubeda, C., Bielski, C., et al. (2013). Expansion of intestinal prevotella copri correlates with enhanced susceptibility to arthritis. Elife 2:e01202. doi: 10.7554/eLife.01202
Scher, J. U., Ubeda, C., Artacho, A., Attur, M., Isaac, S., Reddy, S. M., et al. (2015). Decreased bacterial diversity characterizes the altered gut microbiota in patients with psoriatic arthritis, resembling dysbiosis in inflammatory bowel disease. Arthritis Rheumatol. 67, 128–139. doi: 10.1002/art.38892
Scher, J. U., Ubeda, C., Equinda, M., Khanin, R., Buischi, Y., Viale, A., et al. (2012). Periodontal disease and the oral microbiota in new-onset rheumatoid arthritis. Arthritis Rheum. 64, 3083–3094. doi: 10.1002/art.34539
Senior, B. W., Anderson, G. A., Morley, K. D., and Kerr, M. A. (1999). Evidence that patients with rheumatoid arthritis have asymptomatic ’non-significant’ Proteus mirabilis bacteriuria more frequently than healthy controls. J. Infect. 38, 99–106. doi: 10.1016/S0163-4453(99)90076-2
Stebbings, S., Munro, K., Simon, M. A., Tannock, G., Highton, J., Harmsen, H., et al. (2002). Comparison of the fecal microflora of patients with ankylosing spondylitis and controls using molecular methods of analysis. Rheumatology 41, 1395–1401. doi: 10.1093/rheumatology/41.12.1395
Stecher, B., and Hardt, W. D. (2011). Mechanisms controlling pathogen colonization of the gut. Curr. Opin. Microbiol. 14, 82–91. doi: 10.1016/j.mib.2010.10.003
Stoll, M. L., Kumar, R., Morrow, C. D., Lefkowitz, E. J., Cui, X., Genin, A., et al. (2014). Altered microbiota associated with abnormal humoral immune responses to commensal organisms in enthesitis-related arthritis. Arthritis Res. Ther. 16:486. doi: 10.1186/s13075-014-0486-0
Tito, R. Y., Cypers, H., Joossens, M., Varkas, G., Van Praet, L., Glorieus, E., et al. (2016). Dialister as a microbial marker of disease activity in spondyloarthritis. Arthritis Rheumatol. 69, 114–121. doi: 10.1002/art.39802
Vaahtovuo, J., Munukka, E., Korkeamäki, M., Luukkainen, R., and Toivanen, P. (2008). Fecal microbiota in early rheumatoid arthritis. J. Rheumatol. 35, 1500–1505.
Vatanen, T., Kostic, A. D., and d’Hennezel, E., Siljander, H., Franzosa, E. A., Yassour, M., et al. (2016). Variation in microbiome LPS immunogenicity contributes to autoimmunity in humans. Cell 165, 842–853 doi: 10.1016/j.cell.2016.04.007
Viaud, S., Saccheri, F., Mignot, G., Yamazaki, T., Daillère, R., Hannani, D., et al. (2013). The intestinal microbiota modulates the anticancer immune effects of cyclophosphamide. Science 342, 971–976. doi: 10.1126/science.1240537
Wegner, N., Wait, R., Sroka, A., Eick, S., Nguyen, K. A., Lundberg, K., et al. (2010). Peptidylarginine deiminase from Porphyromonas gingivalis citrullinates human fibrinogen and α-enolase: implications for autoimmunity in rheumatoid arthritis. Arthritis Rheum. 62, 2662–2672. doi: 10.1002/art.27552
Weinblatt, M. E., Keystone, E. C., and Furst, D. E. (2005). Long-term efficacy and safety of adalimumab plus methotrexate in patients with rheumatoid arthritis: ARMADA 4-year extended study. Ann. Rheum. Dis. 65, 753–759. doi: 10.1136/ard.2005.044404
Zhang, X., Zhang, D., Jia, H., Feng, Q., Wang, D., Liang, D., et al. (2015). The oral and gut microbiomes are perturbed in rheumatoid arthritis and partly normalized after treatment. Nat. Med. 21, 895–905. doi: 10.1038/nm.3914
Keywords: microbiota, autoimmune arthritis, infections, immunosuppressive therapy, biologics
Citation: Picchianti-Diamanti A, Rosado MM and D’Amelio R (2018) Infectious Agents and Inflammation: The Role of Microbiota in Autoimmune Arthritis. Front. Microbiol. 8:2696. doi: 10.3389/fmicb.2017.02696
Received: 29 July 2017; Accepted: 26 December 2017;
Published: 16 January 2018.
Edited by:
Marina I. Arleevskaya, Kazan State Medical Academy, RussiaReviewed by:
Adriana Gruppi, Decision Research, United StatesGeorg Singer, Medical University of Graz, Austria
Yuji Naito, Kyoto Prefectural University of Medicine, Japan
Copyright © 2018 Picchianti-Diamanti, Rosado and D’Amelio. This is an open-access article distributed under the terms of the Creative Commons Attribution License (CC BY). The use, distribution or reproduction in other forums is permitted, provided the original author(s) or licensor are credited and that the original publication in this journal is cited, in accordance with accepted academic practice. No use, distribution or reproduction is permitted which does not comply with these terms.
*Correspondence: Raffaele D’Amelio, cmFmZmFlbGUuZGFtZWxpb0B1bmlyb21hMS5pdA==