- Microbial Pathogenicity Laboratory, Department of Microbiology, University of Delhi, New Delhi, India
The presence of antibiotic resistance genes (ARGs) including those expressing ESBLs and AmpC-β-lactamases in Escherichia coli inhabiting the aquatic environments is a serious health problem. The situation is further complicated by the fact that ARGs can be easily transferred among bacterial species with the help of mobile genetic elements – plasmids, integrons, insertion sequences (IS), and transposons. Therefore, the analysis of genetic environment and mobile genetic elements associated with ARGs is important as these provide useful information about the epidemiology of these genes. In our previous study, we had reported presence of various β-lactam resistance genes present in E. coli strains inhabiting the river Yamuna traversing the National Capital Territory of Delhi (India). In the present study, we have analyzed the genetic environment of three ARGs blaTEM-1, blaCTX-M-15, and blaCMY -42 of those E. coli strains. The structure of class 1 integrons and their gene cassettes was also analyzed. Insertion sequence IS26 was present upstream of blaTEM-1, ISEcp1 was present upstream of blaCTXM-15 gene and orf477 was present downstream of blaCTXM-15. ISEcp1 was also present upstream of blaCMY -42 and, blc and sugE genes were present in the downstream region of this gene. Thus, the overall genetic environment surrounding these genes was similar to that reported from E. coli strains isolated globally. Conjugation assays, isolation and analysis of plasmid DNA of the transconjugants indicated that blaTEM-1, blaCTX-M-15, blaCMY -42 and class 1 integron were plasmid-mediated and possibly transmit between genera through horizontal gene transfer (HGT). This might lead to dissemination of antimicrobial resistance genes in aquatic environment. The work embodied in this paper is the first describing the genetic environment of bla and integrons in aquatic E. coli isolated from India.
Introduction
Extensive use of third-generation cephalosporins for humans and veterinary purposes has led to an increased incidence and distribution of extended spectrum β-lactamases (ESBLs) and AmpC in bacteria (Bradford, 2001; Philippon et al., 2002; Bonnet, 2004; Jacoby, 2009). Antibiotic resistance in bacteria may emerge either due to genetic mutations in the antibiotic resistance genes (ARGs) present intrinsically or due to acquisition of foreign ARGs. The frequency of genetic mutations is normally low in nature (Zimmer et al., 1963; Gassmann et al., 2000), hence acquisition of ARGs through horizontal gene transfer (HGT) has been regarded as an important means for the wide spread antimicrobial resistance. It involves mobile genetic elements such as plasmids, transposons, and integrons.
The high prevalence of ESBLs and AmpC-β-lactamases in Escherichia coli is a world-wide public health concern, because E. coli is not only a common constituent of intestinal microbiota but also an important indicator of fecal contamination of aquatic environments. Most antibiotic-resistant E. coli strains enter the aquatic eco-system systems through various anthropogenic activities, discharge from livestock and poultry production, hospital and municipal wastewaters, etc. (Pruden et al., 2006; Pereira et al., 2013). Such waters when used for irrigation, drinking, or recreational activities disseminate antibiotic-resistant bacteria in the ecosystem (Pruden et al., 2006; Su et al., 2012; Pereira et al., 2013). The presence of antimicrobial resistance and their genes in E. coli in aquatic environments has been reported by many investigators (Koczura et al., 2013; Pereira et al., 2013). This is quite alarming, because such genes (ESBLs and AmpC) can be easily transferred among bacterial species with the help of mobile genetic elements, viz. plasmids, integrons, insertion sequences (ISs), and transposons (Liebana et al., 2013). Of these, integrons are of special concern because these are plasmid associated, hence can easily disseminate ARGs in bacterial species. These are very well-organized gene expression systems which can integrate one or several non-functional gene cassettes and convert these into functional genes (Recchia and Hall, 1995; Su et al., 2012). On the basis of the integrase gene (intI) integrons have been classified into three classes, class 1, 2, and 3 (Cambray et al., 2010; Su et al., 2012; Deng et al., 2015). IS elements have been closely associated with genes like blaESBLs and ampC and insertion sequence ISEcp1 helps in dissemination and expression of blaCTX-M in Enterobacteriaceae (Poirel et al., 2006). Various investigators have described the genetic environment of blaTEM, blaCTX-M-15, and blaCMY -42 in clinical E. coli isolates. The genes were reportedly plasmid mediated and IS26 was the most common IS element (Bailey et al., 2011; Dhanji et al., 2011; Hentschke et al., 2011; Mata et al., 2012). Also, ISEcp1 was reportedly associated with blaCTX-M-15 and blaCMY -42. orf477 and blc-sugE were present downstream of blaCTX-M-15 and blaCMY -42, respectively (Bailey et al., 2011; Dhanji et al., 2011; Hentschke et al., 2011; Mata et al., 2012).
In our previous study (Bajaj et al., 2015b) we had reported various β-lactam resistance genes present in E. coli strains inhabiting the river Yamuna which traverses the National Capital Territory of Delhi (India). Of the ARGs, blaTEM was the most widespread gene (100%), followed by blaCTX-M-15 (16%) and plasmid-mediated ampC (3%). Significant diversity was not observed in ESBLs as blaCTX-M-15 was the only ESBL detected. To the best of our knowledge, the genetic environment of bla has not been studied in E. coli isolated from the Indian aquatic environment. The analysis of genetic environment and mobile genetic elements associated with ARGs might provide useful information about the epidemiology of ARGs. Thus, the aim of the present study was to analyze the genetic environment associated with blaTEM-1, blaCTX-M-15, and blaCMY -42 in these E. coli strains. Detection of class 1 integrons and analyses of their gene cassettes was also carried out.
Materials and Methods
E. coli Isolates
A total of 61 well-characterized strains of E. coli belonging to four well-defined phylogroups (A, B1, B2, and D) isolated earlier from the river Yamuna and preserved at -80°C in 50% (v/v) glycerol were used in this study (Bajaj et al., 2015b). For subsequent experiments, the E. coli strains were revived in Luria-Bertani (LB) broth by overnight incubation at 37°C and 200 rpm. In the previous study from our laboratory, blaTEM was reportedly present in all the 61 strains, blaCTX-M-15 in 10 and ampC (blaCMY -42) in only 2 strains (Bajaj et al., 2015b). The azide-resistant E. coli strain J53 which was used as the recipient during conjugation experiments was a kind gift from Dr. George A. Jacoby and provided to us by Dr. Sulagna Basu (National Institute of Cholera and Enteric Diseases, Kolkata, India).
Characterization of Genetic Environment of blaTEM-1, blaCTX-M-15, blaCMY -42, Integrons, and Flanking Regions
DNA was extracted from E. coli strains by boiling lysis method (Rodríguez-Baño et al., 2004). The published primers (Lartigue et al., 2002) did not amplify the promoter region of blaTEM in all the 61 E. coli strains studied; hence new primers were designed using an E. coli plasmid nucleotide sequence available at the GenBank (NCBI) as reference sequence (NC_010378.1). As insertion sequence IS26 is reportedly present upstream of blaTEM gene (Bailey et al., 2011), its presence and orientation relative to blaTEM gene was investigated as described previously (Bailey et al., 2011; Ortega et al., 2012). The promoter and flanking regions of blaCTX-M-15 were successfully amplified using the published primers (Saladin et al., 2002; Dhanji et al., 2011) in all blaCTX-M-15 positive E. coli strains. The complete genetic environment of blaCMY -42 (ampC) was studied by overlapping PCR. The presence of genetic elements which frequently surround ampC was checked using the published primers (Pérez-Pérez and Hanson, 2002; Saladin et al., 2002). These were used to target the ISEcp1 insertion sequence present upstream of the blaCMY -42. A newly designed primer set was used for amplifying the downstream region of blaCMY -42. The integrase genes intI1, intI2, and intI3, and integron class 1 gene cassette were detected using published primers (Kraft et al., 1986; Goldstein et al., 2001; White et al., 2001). It has been reported that the 3′-conserved segment (CS) which flank gene cassettes contain qacEΔ1 and sul1 genes which confer resistance to sulfafurazole antibiotics. Their presence was checked in nine strains which harbored class 1 variable gene cassette using primers and methods described previously (Guo et al., 2011).
The 25 μl PCR reaction mixture prepared contained 2.5 μl of 1× buffer, 200 μM of each dNTPs (Thermo Fisher Scientific, Waltham, MA, United States), 20 pmol of each forward and reverse primers, 6 μl of template DNA and 1 U of Taq DNA polymerase. The details of the primers and the PCR conditions are listed in Table 1. PCR amplicons were electrophoresed on 1% agarose gels at 80 V, stained with ethidium bromide and visualized using a UV tansilluminator. The PCR amplicons were purified using Hi-YieldTM extraction kit (RBC Bioscience, New Taipei City, Taiwan) following manufacturer’s instructions and sequenced at a commercial facility using Sanger sequencing (Invitrogen BioServices India Pvt. Ltd., Bangalore, India). Homology search was performed for the nucleotide sequences using the BLAST algorithm available at NCBI1.
Conjugation and Analysis of Plasmid DNA
Conjugal transfer of plasmid-borne β-lactamase genes (blaTEM-1, blaCTX-M-15, blaCMY -42) and integrons was assessed by broth culture mating assay using E. coli J53 as recipient. After 24 h of incubation, mating mixtures of the donor and recipient were plated on agar containing sodium azide (100 μg/ml) and ampicillin (100 μg/ml) supplemented with either cefotaxime (8 μg/ml), or trimethoprim (10 μg/ml) or chloramphenicol (30 μg/ml). Plasmid DNA was extracted from the donors and transconjugants using a commercial kit (Plasmid Mini Kit, Qiagen GmbH, Hilden, Germany). The presence of the integrons and the resistance genes (blaTEM-1, blaCTX-M-15, blaCMY -42) associated with the plasmids was confirmed by PCR amplification of the plasmid DNA from transconjugant strains as template and analysis of the PCR amplicons after electrophoresis on 1% agarose gels.
Accession Numbers
As the sequence of blaTEM including its promoter region was identical in all the strains hence, the partial coding sequence (CDS) of only one representative strain (IP5N) was submitted to GenBank under the accession number: MF576132. Similarly, the partial CDS of IS26 linked blaTEM-1gene of strains E. coli KK45 and E. coli KP24 were submitted to GenBank under the accession number MF503681 and MF503682, respectively. The partial CDS of blaCTX-M-15 regions of 10 CTX-M-positive E. coli has been submitted under the accession numbers: MF462194 and MF477008–MF477016. The accession numbers of the blaCMY -42 regions of AmpC-positive strains E. coli ISE and E. coli IPE were: MF477017 and MF462195, respectively.
Results and Discussion
Genetic Environment of blaTEM-1
The genetic environment of blaTEM-1 is shown in Figure 1A. Analysis of the promoter regions of blaTEM-1 of all 61 strains revealed that promoters of blaTEM-1 of E. coli present in the river Yamuna were identical to the ‘P3-type promoter’ – the most commonly reported promoter associated with blaTEM-1 in Enterobacteriaceae. The typical regions at -10, TTCAAA and at -35, GACAAT were found to be 41 and 64 bp, respectively away from the starting codon. The blaTEM-1 gene of only three E. coli isolates, viz. KK45, IP24 and IST were linked to IS26 insertion sequence in the upstream region, but at different positions. The orientation of IS26 relative to blaTEM-1 in all the three Indian aquatic isolates was same as reported globally (Figure 1A; Bailey et al., 2011; Lin et al., 2014).
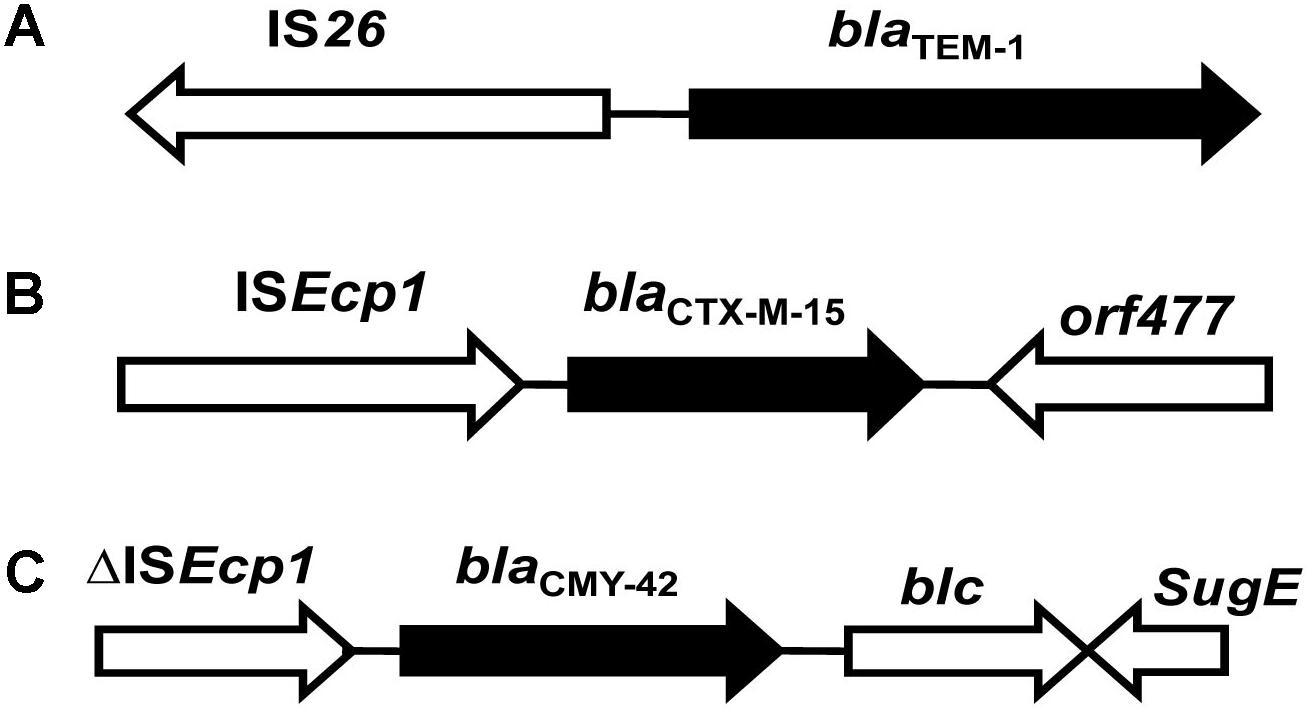
FIGURE 1. Schematic representation of genetic environment of blaTEM-1, blaCTX-M-15, and blacmy-42 genes. The arrows indicate bla genes and upstream and downstream sequences detected by PCR. The direction of the arrows indicates the orientation of gene transcription. (A–C) Represents genetic environment of blaTEM-1, blaCTX-M-15, and blacmy-42, respectively.
Expression of blaTEM is associated with four different types of promoters, viz. P3, Pa/Pb, P4, and P5 in the family Enterobacteriaceae, and Haemophilus influenzae (Lartigue et al., 2002; Tristram and Nichols, 2006; García-Cobos et al., 2008). Overexpression of blaTEM-1 has been associated mostly with Pa/Pb and P4 type promoters. The Indian aquatic strains harbored the most commonly reported promoter upstream of blaTEM-1, i.e., P3 type. Previous studies have reported more than 50% prevalence of P3 promoters in TEM-1-positive, amoxicillin-clavulanate (AMC)-resistant E. coli clinical strains (Ortega et al., 2012). However, in our study no association was observed between AMC resistance and P3/TEM-1 in E. coli aquatic isolates as all the strains harbored P3 type promoters irrespective of AMC resistance or sensitivity (Bajaj et al., 2015b). This suggested that the implications of the origin of E. coli, whether clinical or aquatic, on variations in promoter sequences and AMC resistance need to be investigated further.
Although blaTEM-1 was detected in all the 61 E. coli strains, insertion sequence IS26 was linked with blaTEM-1 in only three strains, but at different positions. Many studies have reported the presence of insertion sequence IS26 at different positions upstream of blaTEM (Bailey et al., 2011; Ortega et al., 2012; Lin et al., 2014). It was observed that the blaTEM genes preceded by IS26 were also regulated by a P3-type promoter in these strains. Previous reports have shown that IS26 can acquire two possible orientations relative to blaTEM (Bailey et al., 2011). In the present study, it was observed that IS26 acquired the most commonly reported orientation relative to blaTEM (Bailey et al., 2011) as depicted in Figure 1A. It has been reported that the presence of a similar IS26-blaTEM-1 configuration in different species might indicate the geographical spread of a species, because IS26 is often associated with transposons (Wain et al., 2003; Bailey et al., 2011). Researchers have so far not been able to understand the effect of IS26 on the expression of blaTEM (Ortega et al., 2012). IS26-blaTEM-1 configuration in Indian strains was similar to that reported for strains isolated from other parts of the world (Wain et al., 2003; Cain et al., 2010; Bailey et al., 2011).
Genetic Environment of blaCTX-M-15
Investigation of the promoter regions of blaCTX-M-15 in all CTX-M-15-positive E. coli strains revealed the presence of insertion sequence ISEcp1 containing typical -10 TACAAT and -35 TTGAA promoters region within its 3′ terminus, 48 bp away from the start codon. Analysis of the downstream region of the blaCTX-M-15 gene revealed presence of orf477 which encodes for a hypothetical protein (Figure 1B).
Analysis of sequences flanking upstream and downstream of blaCTX-M-15 revealed the presence of ISEcp1 upstream of all blaCTX-M-15-positive E. coli strains. The intact ISEcp1 was located 48 bp upstream of blaCTX-M-15 acquiring its preferential insertion sites which are usually located 42–266 bp upstream of different blaCTX-M genes like CTX-M-1, CTX-M-2, and CTX-M-9 clusters. All the blaCTX-M-15-positive E. coli strains harbored the international blaCTX-M-15-type genetic environment. This organization has been reported previously in several E. coli strains isolated from France, Canada, Italy, United Kingdom, Spain, and China (Saladin et al., 2002; Boyd et al., 2004; Canton and Coque, 2006; Lavollay et al., 2006; Dhanji et al., 2011; Lin et al., 2014). The close association of blaCTX-M and ISEcp1 is well known, and has been extensively reported from E. coli strains isolated from various geographical regions of the world highlighting the evolutionary association between ISEcp1 with blaCTX-M (Karim et al., 2001; Saladin et al., 2002; Dhanji et al., 2011; Lin et al., 2014; Wang et al., 2014). ISEcp1 is a member of the family IS1380 (IS Database home page2) and is weakly related to other IS elements (Lin et al., 2014). Zong et al. (2010) have reported the ability of ISEcp1 to mobilize an adjacent gene as a part of transposition units of different sizes. A single copy of ISEcp1 located upstream of blaCTX-M successfully mobilized a chromosomal gene of a Kluyvera strain (Lartigue et al., 2006). It has also been reported that ISEcp1 improves the expression of blaCTX-M, which is low in its natural source species but becomes high once acquired by a member of the family Enterobacteriaceae (Poirel et al., 2003). The intact ISEcp1 element contained the -10 TACAAT and -35 TTGAA promoter sequences within the 3′ non-coding region which were involved in transcription of blaCTX-M-15. IS26 was reportedly associated with different variants of blaCTX-M including blaCTX-M-15 in E. coli isolated from India and different parts of the world (Eckert et al., 2006; Ensor et al., 2006; Dhanji et al., 2011; Shahid et al., 2012; Lin et al., 2014; Wang et al., 2014). However, in our study, aquatic CTX-M-15-positive E. coli strains, unlike the clinical strains from India and abroad, did not show the presence of insertion sequence IS26. However, orf477 was found to be present in the downstream region of blaCTX-M-15, as reported earlier (Eckert et al., 2006; Dhanji et al., 2011; Wang et al., 2014).
Genetic Environment of blaCMY -42 (ampC)
It was observed that blaCMY -42 genes harbored ISEcp1 insertion sequence in the upstream and blc and sugE genes in the downstream region (Figure 1C).
Analysis of flanking regions of blaCMY -42 by overlapping PCR and sequencing revealed the presence of ISEcp1, upstream of the gene (Hentschke et al., 2011; Mata et al., 2012; Tamang et al., 2012). However, the ISEcp1 detected was found to be truncated at the 5′ end, as reported by several other researchers (Hentschke et al., 2011; Mata et al., 2012; Tamang et al., 2012). The genetic surroundings of blaCMY -42, in which the insertion sequence ISEcp1 has been reported to be disrupted by IS1 (Hentschke et al., 2011) was not observed in any of the two CMY-42 positive aquatic E. coli strains. The genes encoding blc (outer-membrane protein) and sugE (drug-efflux channel) flanked the downstream region of blaCMY -42.
Characterization of Class 1 Integrons and Flanking Sequences
Of the 61 E. coli strains, intI1 was present in 30 strains. Nine of these strains harbored five different types of class 1 variable region gene cassette arrays which were present downstream of intI1 and ranged between 0.9 and 2.8 kb in size (Table 2). The 5-gene cassette arrays contained a total of 18 gene cassettes, as follows: the dihydrofolate reductase (dfr) resistance gene family (dfrA17, dfrA1, and dfrA7), the aminoglycoside (aad) resistance gene family (aadA5, aadA1, aadA2, aacA4) and chloramphenicol (CHL) resistance gene catB3. In seven of these strains, variable gene cassette arrays were found associated with sul1and qacEΔ1 genes flanking the 3′-CS. The 3′-CS is known to contain qacEΔ1 and sul1 genes which confer resistance to sulfafurazole antibiotics.
It has been reported that integrons carrying antimicrobial resistance gene cassettes were highly prevalent in aquatic environment (Guo et al., 2011; Canal et al., 2016). Moreover, Class 1 integrons have been reported widely in Gram-negative bacteria which were responsible for both the spread and increase of antimicrobial resistance throughout the world (Koczura et al., 2013; Canal et al., 2016). Our analysis also revealed that Class 1 integrons were common in E. coli isolates of river Yamuna (50%), more than those reported from strains isolated from Malaysia (21%) (Ghaderpour et al., 2015), France and Portugal (11%) (Laroche et al., 2009; Pereira et al., 2013), and Czechia (15%) (Dolejská et al., 2009). Class 2 and 3 integrons were absent in these strains. Class 3 integrons have also been reportedly absent in E. coli isolated from global aquatic habitats (Laroche et al., 2009; Su et al., 2012; Pereira et al., 2013). It would be pertinent to mention here that integrons of class 3 are rarely reported, even among E. coli isolated from humans and/or animals. In the current study, of the 30 integrase-positive isolates, variable regions were detected only in nine strains. This might be due to the presence of a cassette array that was too large to be amplified by the primers used as reported by other researchers also (Yu et al., 2003; Partridge et al., 2009; Ndi and Barton, 2011). Another reason might be the presence of a non-classic structure in the integron with the tni region or various ISs (Partridge et al., 2009).
The variable gene cassette regions of E. coli strains inhabiting the river Yamuna showed the presence of genes encoding for dihydrofolate reductase, dfr family (dfrA17, dfrA1, dfrA7), aminoglycoside adenyltransferase enzymes, the aad family (aadA5, aadA1, aadA2, aacA4) and chloramphenicol (CHL) resistance gene catB3. Since sulfonamide resistance gene (sul1) and quaternary ammonium compounds resistance genes (qacEΔ1) are often associated with the class 1 variable gene cassette region (Paulsen et al., 1993), their presence was also checked in the 3′-CS region of nine strains that harbored the variable gene cassette region. Of these, sul1 and qacEΔ1 genes were detected in seven isolates. Earlier reports in the literature indicated that sul1 and qacEΔ1 were not always present in the 3′-CS region of variable gene cassettes (Nass et al., 1998; Sáenz et al., 2004).
Conjugation and Analysis of Transconjugants
Transferability of β-lactamase genes (blaTEM-1, blaCTX-M-15, blaCMY -42) and of class1 integron was checked by conjugation assay using all 61 E. coli strains as donor strains. Analysis of the plasmid DNA isolated from the recipients (transconjugants) revealed that blaTEM-1 present in all 61 strains, blaCTXM-15 in 10 strains, blaCMY -42 in 2 strains and the class 1 integron in 9 strains were plasmid mediated, and transferrable.
Conjugation assays indicated the transferability of resistance determinants (blaTEM-1 blaCTX-M-15, blaCMY -42, and class1 integrons) to a recipient strain of E. coli J53. Earlier studies have proved the transferability of resistant determinants like blaCTX-M-15, blaTEM, and plasmid-mediated quinolone resistance genes and integrons. These observations also indicated the possible transmission of these genes between genera through HGT (Guo et al., 2011; Haque et al., 2012; Bajaj et al., 2015a). It would be instructive to assess the enormity of such resistance gene transfer in the environment.
Conclusion
The genetic environment of blaTEM-1, blaCTX-M-15, and blaCMY -42 in E. coli strains present in the urban aquatic environment of India has been reported for the first time. The overall genetic environment of β-lactamases was found to be similar to that reported from E. coli strains isolated globally. The Indian aquatic isolates harbored the most commonly reported P3-type promoter upstream of blaTEM-1. While P3/TEM-1 has reportedly been seen in AMC-resistant E. coli clinical strains worldwide, Indian aquatic isolates did not exhibit this organization. The blaTEM-1 was linked with IS26 in its most commonly observed configuration as reported globally (Figure 1A). The Indian isolates harbored the international blaCTX-M-15-type genetic environment and ISEcp1 was present upstream of blaCTX-M-15. Contrary to what has been reported for clinical strains isolated from India and elsewhere, aquatic E. coli strains lacked IS26 element, but orf477 was present downstream of blaCTX-M-15. Class 2 and 3 integrons were absent, but class I integrons were found to be more common in E. coli isolates of the river Yamuna than that reported globally. The variable gene cassette regions revealed the presence of genes encoding for dfr family (dfrA17, dfrA1, dfrA7), the aad family (aadA5, aadA1, aadA2, aacA4), and catB3. The 3′-CS region showed the presence of sul1 and qacEΔ1 genes. The conjugation assays for class 1 integron and blaTEM-1, blaCTXM-15, and blaCMY -42 indicated simultaneous transfer of class 1 variable resistance gene cassettes and β-lactamases genes (blaTEM-1, blaCTX-M-15, blaCMY -42) implying that these were plasmid-mediated and possibly transmit between genera through HGT.
Author Contributions
NSS and JV conceived and designed the experiments. NSS and NS did the data analysis. All the authors wrote the manuscript.
Funding
NSS sincerely thanks Indian Council of Medical Research (ICMR), New Delhi for the financial support (No. 80/919/2014-ECD-I). NS was supported by SERB, DST (SB/YS/LS-156/2014).
Conflict of Interest Statement
The authors declare that the research was conducted in the absence of any commercial or financial relationships that could be construed as a potential conflict of interest.
Acknowledgments
We thank Mr. Rajesh Kumar Mahto for the technical help.
Footnotes
References
Bailey, J. K., Pinyon, J. L., Anantham, S., and Hall, R. M. (2011). Distribution of the blaTEM gene and blaTEM-containing transposons in commensal Escherichia coli. J. Antimicrob. Chemother. 66, 745–751. doi: 10.1093/jac/dkq529
Bajaj, P., Kanaujia, P. K., Singh, N. S., Sharma, S., Kumar, S., and Virdi, J. S. (2015a). Quinolone co-resistance in ESBL- or AmpC-producing Escherichia coli from an Indian urban aquatic environment and their public health implications. Environ. Sci. Pollut. Res. 23, 1954–1959.
Bajaj, P., Singh, N. S., Kanaujia, P. K., and Virdi, J. S. (2015b). Distribution and molecular characterization of genes encoding CTX-M and AmpC β-lactamases in Escherichia coli isolated from an Indian urban aquatic environment. Sci. Total Environ. 505, 350–356. doi: 10.1016/j.scitotenv.2014.09.084
Bass, L., Liebert, C. A., Lee, M. D., Summers, A. O., White, D. G., Thayer, S. G., et al. (1999). Incidence and characterization of integrons, genetic elements mediating multiple-drug resistance, in avian Escherichia coli. Antimicrob. Agents Chemother. 43, 2925–29299.
Bonnet, R. (2004). Growing group of extended-spectrum beta-lactamases: the CTX-M enzymes. Antimicrob. Agents Chemother. 48, 1–14.
Boyd, D. A., Tyler, S., Christianson, S., McGeer, A., Muller, M. P., Willey, B. M., et al. (2004). Complete nucleotide sequence of a 92-kilobase plasmid harbouring the CTX-M-15 extended-spectrum β-lactamase involved in an outbreak in long-term-care facilities in Toronto, Canada. Antimicrob. Agents Chemother. 48, 3758–3764.
Bradford, P. A. (2001). Extended-spectrum beta-lactamases in the 21st century: characterization, epidemiology, and detection of this important resistance threat. Clin. Microbiol. Rev. 14, 933–951.
Cain, A. K., Liu, X., Djordjevic, S. P., and Hall, R. M. (2010). Transposons related to Tn1696 in IncHI2 plasmids in multiply antibiotic resistant Salmonella enterica serovar Typhimurium from Australian animals. Microb. Drug Resist. 16, 197–202.
Cambray, G., Guerout, A., and Mazel, D. (2010). Integrons. Annu. Rev. Genet. 44, 141–166. doi: 10.1146/annurev-genet-102209-163504
Canal, N., Meneghetti, K. L., Almeida, C. P., Bastos, M. D. R., Otton, L. M., and Corcao, G. (2016). Characterization of the variable region in the class 1 integron of antimicrobial-resistant Escherichia coli isolated from surface water. Braz. J. Microbiol. 4, 337–344. doi: 10.1016/j.bjm.2016.01.015
Canton, R., and Coque, T. M. (2006). The CTX-M beta-lactamase pandemic. Curr. Opin. Microbiol. 9, 466–475.
Deng, Y., Bao, X., Ji, L., Chen, L., Liu, J., Miao, J., et al. (2015). Resistance integrons: class 1, 2 and 3 integrons. Ann. Clin. Microbiol. Antimicrob. 14, 45–55. doi: 10.1186/s12941-015-0100-6
Dhanji, H., Patel, R., Wall, R., Doumith, M., Patel, B., Hope, R., et al. (2011). Variation in the genetic environments of blaCTX-M-15 in Escherichia coli from the faeces of travellers returning to the United Kingdom. J. Antimicrob. Chemother. 66, 1005–1012. doi: 10.1093/jac/dkr041
Dolejská, M., Bierosová, B., Kohoutová, L., Literak, I., and Cízek, A. (2009). Antibiotic resistant Salmonella and Escherichia coli isolates with integrons and extended spectrum beta-lactamases in surface water and sympatric black-headed gulls. J. Appl. Microbiol. 106, 1941–1950. doi: 10.1111/j.1365-2672.2009.04155.x
Eckert, C., Gautier, V., and Arlet, G. (2006). DNA sequence analysis of the genetic environment of various blaCTX-M genes. J. Antimicrob. Chemother. 57, 14–23.
Ensor, V. M., Shahid, M., Evans, J. T., and Hawkey, P. M. (2006). Occurrence, prevalence and genetic environment of CTX-M β-lactamases in Enterobacteriaceae from Indian hospitals. J. Antimicrob. Chemother. 58, 1260–1263.
García-Cobos, S., Campos, J., Cercenado, E., Román, F., Lázaro, E., Pérez-Vázquez, M., et al. (2008). Antibiotic resistance in Haemophilus influenzae decreased, except for beta-lactamase-negative amoxicillin-resistant isolates, in parallel with community antibiotic consumption in Spain from 1997 to 2007. Antimicrob. Agents Chemother. 52, 2760–2766. doi: 10.1128/AAC.01674-07
Gassmann, W., Dahlbeck, D., Chesnokova, O., Minsavage, G. V., Jones, J. B., and Staskawicz, B. J. (2000). Molecular evolution of virulence in natural field strains of Xanthomonas campestris pv. vesicatoria. J. Bacteriol. 182, 7053–7059.
Ghaderpour, A., Ho, W. S., Chew, L. L., Bong, C. W., Chong, V. C., Thong, K. L., et al. (2015). Diverse and abundant multi-drug resistant E. coli in Matang mangrove estuaries, Malaysia. Front. Microbiol. 6:977. doi: 10.3389/fmicb.2015.00977
Goldstein, C., Lee, M. D., Sanchez, S., Hudson, C., Phillips, B., Register, B., et al. (2001). Incidence of class 1 and 2 integrases in clinical and commensal bacteria from livestock, companion animals, and exotics. Antimicrob. Agents Chemother. 45, 723–726.
Guo, X., Xia, R., Han, N., and Xu, H. (2011). Genetic diversity analyses of class 1 integrons and their associated antimicrobial resistance genes in Enterobacteriaceae strains recovered from aquatic habitats in China. Lett. Appl. Microbiol. 52, 667–675. doi: 10.1111/j.1472-765X.2011.03059.x
Haque, S. F., Ali, S. Z., Tp, M., and Khan, A. U. (2012). Prevalence of plasmid mediated blaTEM-1 and blaCTX-M-15 type extended spectrum beta-lactamases in patients with sepsis. Asian Pac. J. Trop. Med. 5, 98–102. doi: 10.1016/S1995-7645(12)60003-0
Hentschke, M., Kotsakis, S. D., Wolters, M., Heisig, P., Miriagou, V., and Aepfelbacher, M. (2011). CMY-42, a novel plasmid-mediated CMY-2 variant AmpC beta-lactamase. Microb. Drug Resist. 17, 165–169. doi: 10.1089/mdr.2010.0137
Jacoby, G. A. (2009). AmpC beta-lactamases. Clin. Microbiol. Rev. 22, 161–182. doi: 10.1128/CMR.00036-08
Karim, A., Poirel, L., Nagarajan, S., and Nordmann, P. (2001). Plasmid-mediated extended-spectrum beta-lactamase (CTX-M-3 like) from India and gene association with insertion sequence ISEcp1. FEMS Microbiol. Lett. 201, 237–241.
Koczura, R., Mokracka, J., Barczak, A., Krysiak, N., and Kaznowski, A. (2013). Association between the presence of class 1 integrons, virulence genes, and phylogenetic groups of Escherichia coli isolates from river water. Microb. Ecol. 65, 84–90. doi: 10.1007/s00248-012-0101-3
Kraft, C. A., Timbury, M. C., and Platt, D. J. (1986). Distribution and genetic location of Tn7 in trimethoprim-resistant Escherichia coli. J. Med. Microbiol. 22, 125–131.
Laroche, E., Pawlak, B., Berthe, T., Skurnik, D., and Petit, F. (2009). Occurrence of antibiotic resistance and class 1, 2 and 3 integrons in Escherichia coli isolated from a densely populated estuary (Seine, France). FEMS Microbiol. Ecol. 68, 118–130. doi: 10.1111/j.1574-6941.2009.00655.x
Lartigue, M. F., Leflon-Guibout, V., Poirel, L., Nordmann, P., and Nicolas- Chanoine, M. H. (2002). Promoters P3, Pa/Pb, P4, and P5 upstream from blaTEM genes and their relationship to beta-lactam resistance. Antimicrob. Agents Chemother. 46, 4035–4037.
Lartigue, M. F., Poirel, L., Aubert, D., and Nordmann, P. (2006). In vitro analysis of ISEcp1B-mediated mobilization of naturally occurring beta-lactamase gene blaCTX-M of Kluyvera ascorbata. Antimicrob. Agents Chemother. 50, 1282–1286.
Lavollay, M., Mamlouk, K., Frank, T., Akpabie, A., Burghoffer, B., Ben Redjeb, S., et al. (2006). Clonal dissemination of a CTX-M-15 β-lactamase-producing Escherichia coli strain in the Paris area, Tunis, and Bangui. Antimicrob. Agents Chemother. 50, 2433–2438.
Liebana, E., Carattoli, A., Coque, T. M., Hasman, H., Magiorakos, A. P., Mevius, D., et al. (2013). Public health risks of enterobacterial isolates producing extended-spectrum beta-lactamases or AmpC beta-lactamases in food and food-producing animals: an EU perspective of epidemiology, analytical methods, risk factors, and control options. Clin. Infect. Dis. 56, 1030–1037. doi: 10.1093/cid/cis1043
Lin, L., Xiaorong, W., Shuchang, A., Xiangyan, Z., Lin, C., Yuqian, L., et al. (2014). Genetic environment of β-lactamase genes of extended-spectrum β-lactamase producing Klebsiella pneumoniae isolates from patients with lower respiratory tract infection in China. Chin. Med. J. 127, 2445–2450.
Mata, C., Miró, E., Alvarado, A., Garcillán-Barcia, M. P., Toleman, M., Walsh, T. R., et al. (2012). Plasmid typing and genetic context of AmpC β-lactamases in Enterobacteriaceae lacking inducible chromosomal ampC genes: findings from a Spanish hospital 1999–2007. J. Antimicrob. Chemother. 67, 115–122.
Nandi, S., Maurer, J. J., Hofacre, C., and Summers, A. O. (2004). Gram-positive bacteria are a major reservoir of class 1 antibiotic resistance integrons in poultry litter. Proc. Natl. Acad. Sci. U.S.A. 4, 7118–7122.
Nass, T., Sougakof, W., Casetta, A., and Nordmann, P. (1998). Molecular characterization of OXA-20, a novel class D β-lactamase, and its integron from Pseudomonas aeruginosa. Antimicrob. Agents Chemother. 42, 2074–2083.
Ndi, O. L., and Barton, M. D. (2011). Incidence of class 1 integron and other antibiotic resistance determinants in Aeromonas spp. from rainbow trout farms in Australia. J. Fish Dis. 34, 589–599. doi: 10.1111/j.1365-2761.2011.01272.x
Ortega, A., Oteo, J., Aranzamendi-Zaldumbide, M., Bartolomé, R. M., Bou, G., Cercenado, E., et al. (2012). Spanish multicenter study of the epidemiology and mechanisms of amoxicillin clavulanate resistance in Escherichia coli. Antimicrob. Agents Chemother. 56, 3576–3581. doi: 10.1128/AAC.06393-11
Partridge, S. R., Tsafnat, G., Coiera, E., and Iredell, J. R. (2009). Gene cassette and cassette arrays in mobile resistance integrons. FEMS Microbiol. Rev. 33, 757–784. doi: 10.1111/j.1574-6976.2009.00175.x
Paulsen, I. T., Littlejohn, T. G., Rådström, P., Sundström, L., Sköld, O., Swedberg, G., et al. (1993). The 3′ conserved segment of integrons contains a gene associated with multidrug resistance to antiseptics and disinfectants. Antimicrob. Agents Chemother. 37, 761–768.
Pereira, A., Santos, A., Tacão, M., Alves, A., Henriques, I., and Correia, A. (2013). Genetic diversity and antimicrobial resistance of Escherichia coli from Tagus estuary (Portugal). Sci. Total Environ. 461, 65–71. doi: 10.1016/j.scitotenv.2013.04.067
Pérez-Pérez, F. J., and Hanson, N. D. (2002). Detection of plasmid-mediated AmpC beta-lactamase genes in clinical isolates by using multiplex PCR. J. Clin. Microbiol. 40, 2153–2162.
Philippon, A., Arlet, G., and Jacoby, G. A. (2002). Plasmid-determined AmpC-type beta-lactamases. Antimicrob. Agents Chemother. 46, 1–11.
Poirel, L., Decousser, J. W., and Nordmann, P. (2003). Insertion sequence ISEcp1B is involved in expression and mobilization of a blaCTX-M beta-lactamase gene. Antimicrob. Agents Chemother. 47, 2938–2945.
Poirel, L., Lartigue, M. F., Decousser, J. W., and Nordmann, P. (2006). ISEcp1B-mediated transposition of blaCTX-M in Escherichia coli. Antimicrob. Agents Chemother. 49, 447–450.
Pruden, A., Pei, R., Storteboom, H., and Carlson, K. H. (2006). Antibiotic resistance genes as emerging contaminants: studies in northern Colorado. Environ. Sci. Technol. 40, 7445–7450.
Recchia, G. D., and Hall, R. M. (1995). Gene cassettes: a new class of mobile element. Microbiology 141, 3015–3027.
Rodríguez-Baño, J., Navarro, M. D., Romero, L., Martínez-Martínez, L., Muniain, M. A., Perea, E. J., et al. (2004). Epidemiology and clinical features of infections caused by extended-spectrum beta-lactamase-producing Escherichia coli in non-hospitalized patients. J. Clin. Microbiol. 42, 1089–1094.
Sáenz, Y., Briñas, L., Domínguez, E., Ruiz, J., Zarazaga, M., Vila, J., et al. (2004). Mechanisms of resistance in multiple-antibiotic resistant Escherichia coli strains of human, animal, and food origins. Antimicrob. Agents Chemother. 48, 3996–4001.
Saladin, M., Cao, V. T. B., Lambert, T., Donay, J. L., Herrmann, J. L., Ould-Hocine, Z., et al. (2002). Diversity of CTX-M beta-lactamases and their promoter regions from Enterobacteriaceae isolated in three Parisian hospitals. FEMS Microbiol. Lett. 209, 161–168.
Shahid, M., Sobia, F., Singh, A., and Khan, H. M. (2012). Concurrent occurrence of blaampC families and blaCTX-M genogroups and association with mobile genetic elements ISEcp1, IS26, ISCR1, and sul1-type class 1 integrons in Escherichia coli and Klebsiella pneumoniae isolates originating from India. J. Clin. Microbiol. 50, 1779–1782. doi: 10.1128/JCM.06661-11
Su, H. C., Ying, G. G., Tao, R., Zhang, Q. R., Zhao, L. J., and Liu, S. Y. (2012). Class 1 and 2 integrons, sul resistance genes and antibiotic resistance in Escherichia coli isolated from Dongjiang River, South China. Environ. Pollut. 169, 42–49. doi: 10.1016/j.envpol.2012.05.007
Tamang, M. D., Nam, H.-M., Jang, G.-C., Kim, S. R., Chae, M. H., Jung, S. C., et al. (2012). Molecular characterization of extended-spectrum-β-lactamase-producing and plasmid-mediated AmpC β-lactamase-producing Escherichia coli isolated from stray dogs in South Korea. Antimicrob. Agents Chemother. 56, 2705–2712. doi: 10.1128/AAC.05598-11
Tristram, S. G., and Nichols, S. (2006). A multiplex PCR for beta-lactamase genes of Haemophilus influenzae and description of a new blaTEM promoter variant. J. Antimicrob. Chemother. 58, 183–185.
Wain, J., Diem Nga, L. T., Kidgell, C., James, K., Fortune, S., Song Diep, T., et al. (2003). Molecular analysis of IncHI1 antimicrobial resistance plasmids from Salmonella serovar Typhi strains associated with typhoid fever. Antimicrob. Agents Chemother. 47, 2732–2739.
Wang, J., Stephan, R., Zurfluh, K., Hachler, H., and Fanning, S. (2014). Characterization of the genetic environment of blaESBL genes, integrons and toxin-antitoxin systems identified on large transferrable plasmids in multi-drug resistant Escherichia coli. Front. Microbiol. 5:716. doi: 10.3389/fmicb.2014.00716
White, P. A., MacIver, C. J., and Rawlinson, W. D. (2001). Integrons and gene cassettes in the Enterobacteriaceae. Antimicrob. Agents Chemother. 45, 2658–2661.
Yu, H. S., Lee, J. C., Kang, H. Y., Ro, D. W., Chung, J. Y., Jeong, Y. S., et al. (2003). Changes in gene cassettes of class 1 integrons among Escherichia coli isolates from urine specimens collected in Korea during the last two decades. J. Clin. Microbiol. 41, 5429–5433.
Zimmer, D. E., Schafer, J. F., and Patterson, F. L. (1963). Mutations for virulence in Puccinia coronata. Phytopathology 53, 171–176.
Keywords: Escherichia coli, blaCTX-M-15, blaCMY-42, IS26, ISEcp1, integrons, genetic environment, horizontal gene transfer
Citation: Singh NS, Singhal N and Virdi JS (2018) Genetic Environment of blaTEM-1, blaCTX-M-15, blaCMY-42 and Characterization of Integrons of Escherichia coli Isolated From an Indian Urban Aquatic Environment. Front. Microbiol. 9:382. doi: 10.3389/fmicb.2018.00382
Received: 21 November 2017; Accepted: 20 February 2018;
Published: 07 March 2018.
Edited by:
Santi M. Mandal, Indian Institute of Technology Kharagpur, IndiaReviewed by:
Silvia García Cobos, University Medical Center Groningen, NetherlandsVartul Sangal, Northumbria University, United Kingdom
Copyright © 2018 Singh, Singhal and Virdi. This is an open-access article distributed under the terms of the Creative Commons Attribution License (CC BY). The use, distribution or reproduction in other forums is permitted, provided the original author(s) and the copyright owner are credited and that the original publication in this journal is cited, in accordance with accepted academic practice. No use, distribution or reproduction is permitted which does not comply with these terms.
*Correspondence: Jugsharan S. Virdi, dmlyZGlfZHVzY0ByZWRpZmZtYWlsLmNvbQ==