- 1ANSES, Hygiene and Quality of Poultry and Pig Products Unit, Ploufragan-Plouzané Laboratory, Bretagne-Loire University, Ploufragan, France
- 2UFR of Life Sciences and Environment, University of Rennes 1, Rennes, France
Campylobacter is the leading cause of bacterial gastroenteritis in industrialized countries, with poultry reservoir as the main source of infection. Nevertheless, a recent study on source attribution showed that cattle could be a source of human contamination in France (Thépault et al., 2017). However, few data are available on thermophilic Campylobacter epidemiology in cattle in France. The aim of this study is to collect new data of thermophilic Campylobacter prevalence in these animals and to subtype C. jejuni isolates to assess the potential implication of cattle in campylobacteriosis. A 6-month survey was carried out in one of the largest European slaughterhouse of cattle. Based on a statistical representative sampling plan, 959 intestinal content samples (483 adult cattle and 476 calves) were collected. An adapted version of the ISO 10272 standard and Maldi-Tof were used for detection and speciation of thermophilic Campylobacter isolates. Within more than 2000 thermophilic Campylobacter isolates collected, a selection of 649 C. jejuni isolates was typed with Comparative Genomic Fingerprinting (CGF40) and a subset of 77 isolates was typed using Multilocus Sequence Typing (MLST). Simultaneously, clinical isolates occurred in France were genotyped. Prevalence of thermophilic Campylobacter in the global cattle population was 69.1% (CI95% = 66.1, 72.1) at slaughterhouse level. In adult cattle, the prevalence was 39.3%, while 99.4% of calves were contaminated, and C. jejuni was the most prevalent species with prevalence of 37.3 and 98.5%, respectively and a higher genetic diversity in adult cattle. The prevalence of C. coli was lower with 3% in adult cattle and 12.5% in calves. MLST and CGF40 genotyping did not showed a high number of clusters within cattle isolates but the predominance of few clusters accounted for a large part of the population (CC-21, CC-61, CC-48, and CC-257). By comparison with clinical genotypes, genetic diversity was significantly lower in cattle. Moreover, significant overlap was observed between genotypes from both origins, with 3 of the 4 main cattle clusters present in human isolates. This study provides new insights on the epidemiology of thermophilic Campylobacter and C. jejuni in cattle production in France and their potential implication in human infection.
Introduction
Thermophilic Campylobacter, especially C. jejuni and C. coli, are the primary cause of human bacterial gastroenteritis worldwide and are major zoonotic agents. In Europe, the number of reported confirmed cases of human campylobacteriosis was 229,213 with an European notification rate of 65.6 per 100,000 population in 2015 (EFSA and ECDC, 2016). However, these data are often an underestimate of the true incidence (Havelaar et al., 2013). Thus, in France a recent study estimated that more than 500,000 cases of campylobacteriosis occur each year (Van Cauteren et al., 2015), while EFSA and ECDC (2016) reported 6,000 cases in 2015. In France, C. jejuni is responsible for nearly 80% of human infections while C. coli accounted for 15% of campylobacteriosis between 2003 and 2010 (Bessède et al., 2014).
While poultry products are well-recognized sources of human infection, there is increasing evidence that ruminants also play a prominent role (Mughini Gras et al., 2012; Fernández et al., 2015). A recent study performed in France (Thépault et al., 2017), estimated that equal proportions of clinical isolates could be attributed to chicken and ruminant reservoirs, using 15 novel host-segregating markers for source attribution, defined on a gene-by-gene approach (Sheppard et al., 2012) based on a pan-genomic approach (Méric et al., 2014). In the same way, de Haan et al. (2010) showed that cases of human Campylobacter infections in Finland could be attributed equally to cattle and poultry using MLST data. Moreover, another study from the United-Kingdom using MLST genotype data, estimated that 42% of clinical cases from young children in rural areas arose via acquisition of C. jejuni from cattle sources and 12% from sheep sources (Wilson et al., 2008; Strachan et al., 2012). Nevertheless, this finding is not surprising since several studies reported that the cattle reservoir seems to be highly contaminated. Indeed, in the USA, a recent study described a prevalence of Campylobacter about 71% at three cattle farms (Cha et al., 2017). In Finland, the prevalence of Campylobacter spp. in cattle was evaluated at 31.1% in a 12-months sampling survey (Hakkinen et al., 2007), while it reached 54.6% in the United Kingdom (Milnes et al., 2008) and 22% in Scotland (Rotariu et al., 2009). Therefore, it is important to explore the epidemiology of cattle contamination by Campylobacter in order to explain the role of this animal reservoir in human infections. Actually, few data are available in France, since only one study has been conducted between 2002 and 2006, which showed a prevalence of thermophilic Campylobacter spp. of 4.6% in cull cows, 6% in young cattle, and 39.1% in calves (Châtre et al., 2010). However, relationships between thermophilic Campylobacter isolates from human and cattle were not investigated in this study. Therefore, the role of cattle in human contamination with thermophilic Campylobacter in France remains unknown.
In order to investigate the epidemiology of thermophilic Campylobacter spp., molecular subtyping methods with enhanced discriminatory power are required. In recent years the Comparative Genomic Fingerprinting (CGF) technique has been used to subtype C. jejuni and C. coli (Taboada et al., 2012; Deckert et al., 2014). This technique represents a high resolution subtyping approach which assesses genetic variability in the accessory genome. Moreover this technique is complementary to Multilocus Sequence Typing (MLST) which remains the gold standard for Campylobacter genotyping (Dingle et al., 2001).
The purpose of this work was to conduct a representative investigation in the cattle production in France to estimate the prevalence of thermophilic Campylobacter spp. in this reservoir and characterize further C. jejuni isolates using CGF and MLST. Then, the genotypes from cattle isolates were compared to genotypes found in isolates from clinical cases occurred in 2009 and 2015 in France to assess the potential role of cattle in human campylobacteriosis.
Materials and Methods
Sampling Plan
A 6-month survey was carried out in one of the largest European slaughterhouse of cattle from June to December 2016. The number of intestinal contents to be sampled was calculated in relation to the number of slaughtered animals in the abattoir (60 000 calves and 140 000 adults bovines slaughtered each year), with an expected animal-prevalence of 16.5% (Châtre et al., 2010) and a precision of 20% with 95% confidence limits (Machin et al., 2009). Thus, the representative sampling plan consisted of sampling 959 intestinal contents taken from 483 adult cattle and 476 calves. The 959 samples were from 282 farms distributed among 32 French departments representative of the French production of cattle. Among the adult cattle (>8 months old), 270 animals were from beef breed and 213 from dairy breed, while among calves 143 were beef calves, 328 dairy calves, two animals belonged to mixed breed and breed information was missing for three calves (Table 1).The samples were collected weekly during 6 months at the evisceration level in the abattoir, transported to the laboratory in isotherm bag and stored at + 4°C until the analysis.
Isolation and Identification of Thermophilic Campylobacter Species
Isolation of thermophilic Campylobacter spp. was carried out 1 day after the sample collection according to an adapted version of the ISO standard 10272. Direct plating of one loop of intestinal content was performed on two media mCCDA (modified Charcoal Cefoperazone Deoxycholate Agar) and Butzler n°2 (Oxoid, Dardilly, France). The agar plates were incubated during 72 ± 2 h. In addition, ten grams of intestinal content were added to 90 ml of Preston broth for enrichment. After incubation during 24 ± 2 h, streaking of the broths was performed on mCCDA and Butzler n°2 plates whose were incubated during 48 h. All incubations of broths and plates were done in microaerophilic atmosphere (85% N2, 10% CO2, 5% O2) at 41.5°C. All the agar media were examined to detect the presence of thermophilic Campylobacter typical colonies. For each positive sample, one colony with thermophilic Campylobacter characteristics (morphology and mobility) per plate (two different media, two conditions for the detection: direct or after enrichment) were subcultured on blood agar. Thus, one to four isolates were collected from each positive sample leading to a collection of 2226 thermophilic Campylobacter isolates. The species of all the collected isolates were identified using MALDI-TOF MS (Bessède et al., 2011). The isolates were stored at −70°C in peptone broth containing 20% (v/v) glycerol and only C. jejuni isolates were characterized further in this study.
Clinical Isolates
Clinical isolates were obtained from the National Reference Center for Campylobacter and Helicobacter in France. These isolates were collected from campylobacteriosis cases which occurred in 2009 (n = 143) (Thépault et al., under review) and 2015 (n = 371) (Rose et al., 2017) in the 10 most populated departments in France.
CGF40 Typing of Campylobacter jejuni
A selection of 649 isolates of C. jejuni (one isolate per positive animal) was genotyped using the CGF typing method based on 40 assay genes (CGF40). This method was used to genotype a high number of isolates with a higher discriminatory power than MLST at lower cost and with highly concordant results (Taboada et al., 2012). Isolates were subcultured onto Campylobacter blood-free selective agar® (Karmali, Oxoid) in microaerobic conditions at 41.5°C for 48 h. The genomic DNA was extracted from 1-day single-colony cultures using the InstaGene® Matrix (BioRad Laboratories, Hercules, CA, USA) according to the manufacturer's instructions and quantified using a NanoDrop® ND-1000 (Thermo Scientific, Gometz le Châtel, France).
The experimental conditions to generate CGF fingerprints were adapted from the original protocol described by Taboada et al. (2012). The experimental details for the amplification of the 40 assay genes were previously published (Thépault et al., 2016). The CGF fingerprints were then visualized using a standard gel electrophoresis containing 2% of agarose colored with GelRed® (Interchim, Montluçon, France) in accordance with supplier recommendations. The reference strain NCTC11168, was used as positive control in all assays, since the strain is known to contain the 40 assay genes.
PCR results were converted into binary data corresponding to the absence (0) or the presence (1) of the genetic marker in bacterial genomes and were stored into BioNumerics® software (v 6.5, Applied Maths, Belgium). A dendrogram was built using the simple matching distance coefficient and unweighted-pair group method using average linkages (UPGMA) of clustering in BioNumerics®, as previously described (Taboada et al., 2012). Clusters were defined on a basis of 90% of fingerprinting similarity (CGF40–90% clusters) to analyze the population structure. CGF40–90% clusters including at least 4% of the isolates of cattle or human population were defined as predominant in the population.
Multilocus Sequence Typing (MLST)
Alleles of the seven housekeeping genes for MLST (aspA, glnA, gltA, glyA, pgm, tkt, and uncA) were determined within clinical isolates from 2009 in a previous study (Thépault et al., under review). In this study, alleles, sequence types (ST) and clonal complexes (CC) of isolates from cattle and clinical cases from 2015 were determined by Whole Genome Sequencing (WGS), using Ion Torrent technology available at the Anses sequencing platform, and by comparison of the sequences to the publically available PubMLST database (http://pubmlst.org/campylobacter) on BIGSdb (Jolley and Maiden, 2010). MLST characterization through WGS was performed on selected subsets of 77 and 79 isolates from cattle and clinical cases from 2015, respectively. Isolates selection was made to keep the same genetic structure in the subsets than in original populations, by selecting equal proportions of each CGF40 genotypes within cattle and clinical isolates from 2015, than proportions of these clusters observed in each original population. Clonal complexes including at least 4% of the isolates of cattle or human population were defined as predominant in the population.
Data Analysis
A chi-square test was used to assess the statistical difference between data collected from thermophilic Campylobacter prevalence analysis. The genetic diversity of C. jejuni within the different populations of isolates was assessed using Simpson's diversity index (ID) (Hunter and Gaston, 1988), calculated using the online tool “Comparing Partitions” from the website http://www.comparingpartitions.info (Carriço et al., 2006). The calculation of the confidence intervals for clustering agreement measures at 95% was performed using the resampling technique jackknife (Severiano et al., 2011).
Results
Prevalence of Thermophilic Campylobacter spp.
In this study, the overall prevalence of thermophilic Campylobacter spp. in cattle was 69.1% (CI95% = 66.1, 72.1), and a significant difference was observed between the contamination of adult cattle (39.3%; CI95% = 34.9, 43.9) and calves (99.4%; CI95% = 98.2, 99.9) with only three thermophilic Campylobacter negative calves (p < 0.001; Table 1). Within the adult cattle, the prevalence of thermophilic Campylobacter in dairy animals (46.9%; CI95% = 40.1, 53.9) was significantly higher than among beef animals (33.3%; CI95% = 27.8, 39.3) (p < 0.001; Table 1), while no significant difference was observed between dairy and beef calves.
The most common thermophilic Campylobacter species found in cattle of all ages was C. jejuni with a prevalence of 67.7% (CI95% = 64.6, 70.6), followed by C. coli with a prevalence of 7.6% (CI95% = 6.0, 9.5). Regarding C. jejuni prevalence according to cattle ages and breed, we observed in adult animals a prevalence of 37.3% with a significant difference (p < 0.001) between beef (31.5%) and dairy cattle (44.6%; Table 1). On another hand, within calves, the prevalence of C. jejuni was very high (98.5%; CI95% = 96.9, 99.4) regardless of the breed (Table 1). Additional Campylobacter species were isolated from cattle to a lesser extent. Indeed, one dairy cattle was contaminated by C. lari and one beef cattle by C. fetus, while one beef calf carried C. hyointestinalis (Table 1). Interestingly, in almost 10% of animals, co-infections with several Campylobacter species were observed. Indeed, six adult cows and 54 calves were co-infected by C. jejuni and C. coli, while three different Campylobacter species were isolated from one calf (C. jejuni, C. coli, and C. hyointestinalis).
CGF40
CGF40–90% analysis revealed 45 distinct profiles among the 649 C. jejuni isolates collected in cattle and six clusters accounted for 76.58% of the isolates: the clusters 110, 35, 62, 103, 18, and 49 (Figure 1). Within calves isolates, which showed 29 different CGF40–90% clusters (Table 2), 6 clusters were predominant accounting for 81.66% of the isolates: the clusters 110, 35, 62, 103, 49, and 112 (Figure 2). These six clusters also predominated among dairy calves isolates, while only four (35, 110, 62, and 49) of these clusters were predominant within beef calves. Within beef calves, four supplementary main clusters were shown: clusters 18, 63, 37, and 84.
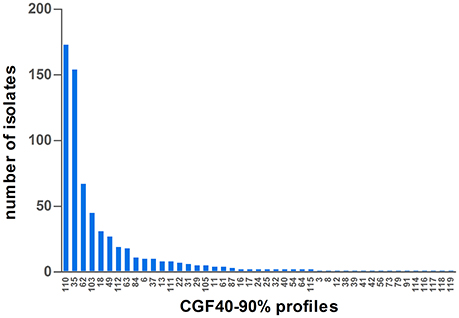
Figure 1. Distribution of CGF40–90% profiles within the population of 649 isolates of C. jejuni isolated from cattle in France.
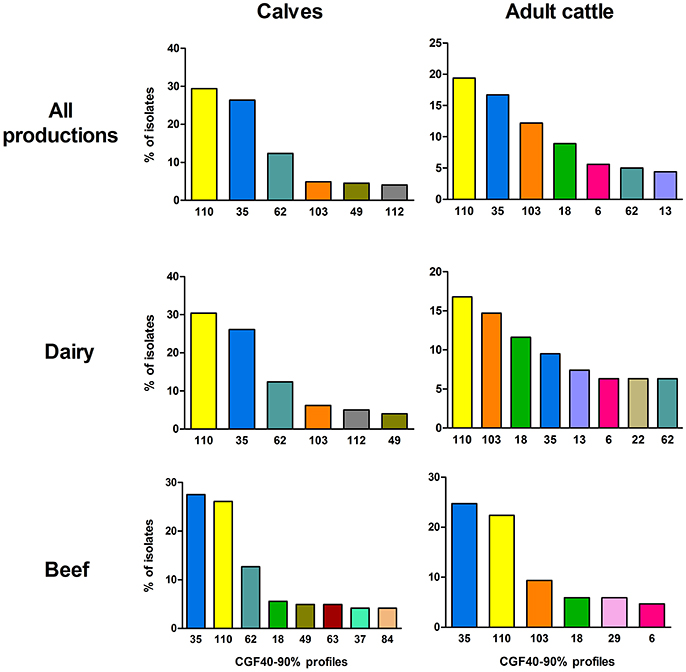
Figure 2. Prevalence of the most frequent CGF40–90% profiles (including ≥4% of isolates) within the different populations of C. jejuni isolates from calves and adults cattle.
Then, with regards to the adult cattle isolates population, which showed 29 CGF40–90% clusters (Table 2), seven main clusters gathered 72.22% of the isolates: the clusters 110, 35, 103, 18, 6, 62, and 13 (Figure 2). Only four main clusters (110, 35, 62, and 103) were common to adult cattle and calves (Figure 2). In the same way than within the calves, the distribution of the main clusters between dairy and beef breed was different. Among dairy cattle, eight main clusters (110, 103, 18, 35, 13, 6, 22, and 62) gathered 78.94% of the isolates while within beef cattle, only six predominant clusters (35, 110, 103, 18, 29, and 6) grouped 72.94% of the isolates.
According to the Simpson's diversity indexes and their confidence intervals (CI95%), C. jejuni isolates collected from adult cattle showed a higher significant genetic diversity than isolates from calves as no overlap was observed between their CI95% (Table 2). On the contrary, no significant difference of genetic diversity was observed between isolates collected from different cattle breeds (dairy vs. beef).
Nevertheless, genetic diversity of cattle C. jejuni isolates was significantly lower than that observed among clinical isolates (Table 2). Indeed, 98 different CGF40–90% profiles were shown among the 514 clinical isolates from 2009 and 2015 typed. However, the genetic diversity in clinical isolates seems to be stable overtime since the main clinical clusters (clusters 62, 103, 110, and 54) observed in 2009 (55.2%) and 2015 (32.6%) are identical. These four main profiles gathered 38.9% of all clinical isolates, (Figure 3) and 49 of the 98 clusters appeared only once in the population (unique clusters). Interestingly, among the four main prevalent clusters within the clinical isolates, 3 were also predominant within the cattle isolates: the clusters 62, 103, and 110. Moreover, the 3 other main clusters within the cattle isolates, clusters 49, 18, and 35, were also shown by 11, 9, and 5 clinical isolates respectively (Figure 3).
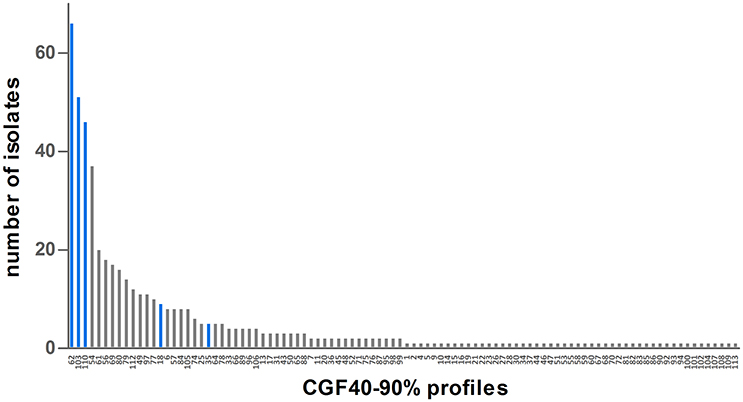
Figure 3. Distribution of CGF40–90% profiles within the population of 514 clinical isolates from France. Blue bars represent CGF40–90% clusters which are the most frequently found in cattle isolates.
MLST
From the selection of 77 cattle isolates, 26 STs were identified, of which 14 appeared only once in the data set. Except ST-2217, ST-5707, ST-8968, ST-8967, and ST-8969, each represented by a single isolate, all STs identified were grouped into 9 clonal complexes, with more than 80% of isolates belonging to the four most prevalent lineages (CC-21, CC-61, CC-48, and CC-257, Table 3). From the 222 clinical isolates typed (including isolates from 2009 and isolates from 2015), 94 STs were identified, of which 58 appeared only once in the data set and more than 93% of isolates were grouped into 24 clonal complexes (Table 3). The 6 main clonal complexes (CC-21, CC-206, CC-48, CC-353, CC-354, and CC-257) gathered almost 65% of the isolates (Table 3).
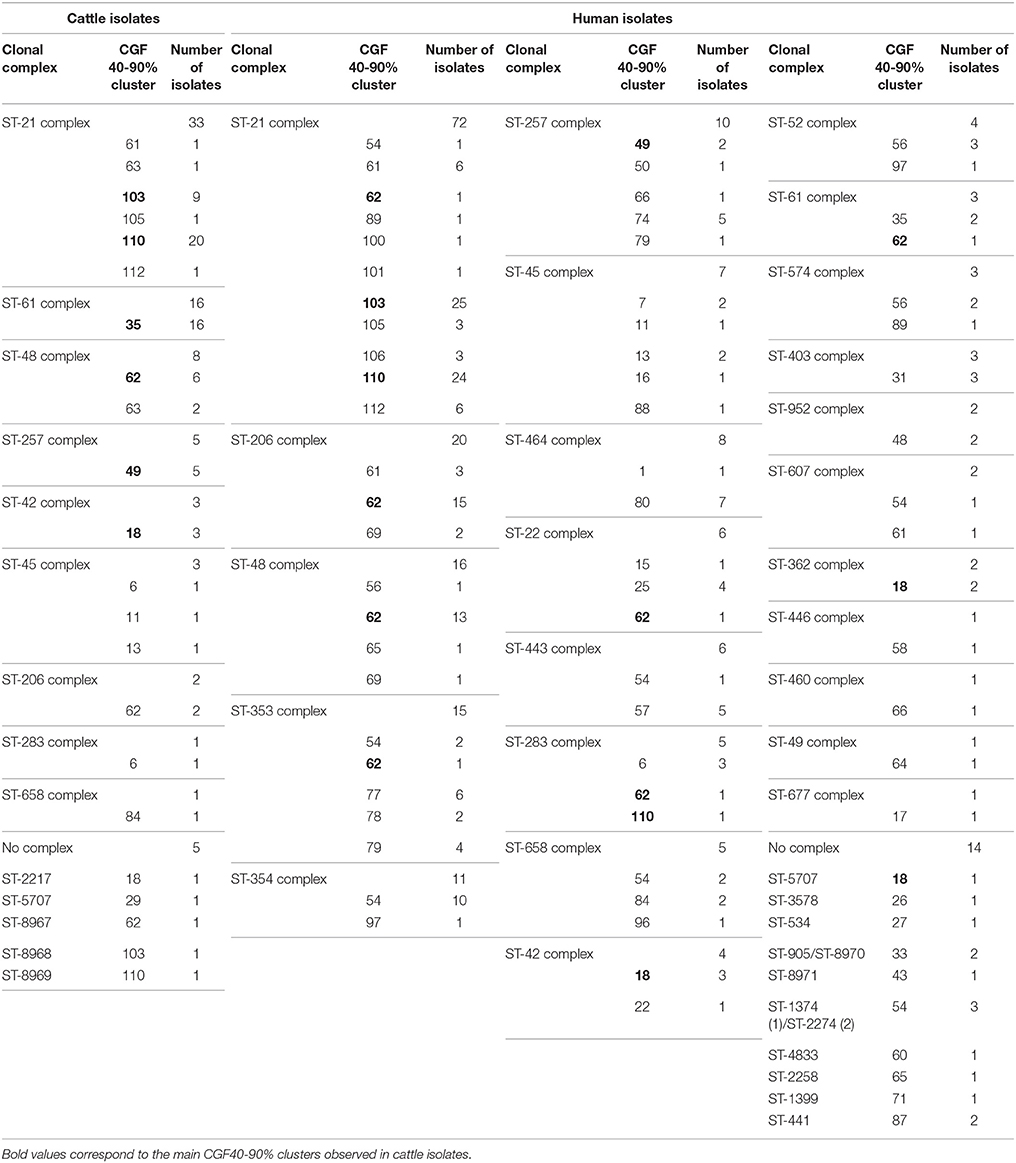
Table 3. Clonal complexes found among the cattle and clinical isolates and link with the CGF40–90% clusters.
Discussion
In this large scale study designed to be representative for the cattle production in France, we assessed the contamination of cattle by thermophilic Campylobacter spp. and described the genetic structure of C. jejuni isolates circulating in these animals using CGF40 and MLST. Here, we reported a high contamination of cattle by thermophilic Campylobacter spp. (69.1%), with the predominance of C. jejuni which showed a prevalence of 67.7%. C. jejuni is the main species reported in cattle (Kwan et al., 2008; Fernandez and Hitschfeld, 2009), but wide range of prevalence estimates were described. They can vary for Campylobacter spp. from 16.5 to 89.4% according to the study (Stanley et al., 1998; Hakkinen et al., 2007; Kwan et al., 2008; Fernandez and Hitschfeld, 2009; Rotariu et al., 2009; Châtre et al., 2010). Surprisingly, the comparison of prevalence estimates obtained in the present study with results from a previous work performed on cattle in France (Châtre et al., 2010), revealed significant discrepancies between prevalence estimates (69.1 vs. 16.5% for thermophilic Campylobacter spp.). One of the most likely hypotheses to explain the discrepancies is the differences in protocols used for the detection of thermophilic Campylobacter, and especially the absence of an enrichment step for the isolation of thermophilic Campylobacter in Châtre et al. (2010) work. Thus, if we consider only direct platting results in our study, the thermophilic Campylobacter prevalence would have been 86.3% in adult cattle and 13.7% in calves, instead of 99.4 and 39.3%, respectively. Indeed, since the contamination level of cattle by Campylobacter is thought to be generally low (102 to 104 CFU/g) compared with chicken (Nielsen, 2002; Hakkinen et al., 2007; Rotariu et al., 2009), and since a significant microbial flora can be observed on agar plate with cattle samples (Workman et al., 2005), less isolation of Campylobacter can occur from the most weakly contaminated animals without enrichment step. On another hand, it has been reported that the search of Campylobacter in intestinal samples generally gave higher estimates than study with feces (Stanley and Jones, 2003).
In addition to technical differences in protocols experiment, several other factors may influence prevalence estimates, such as the size and type (breed) of herd, the season, animal ages, geography, or husbandry practices (Stanley and Jones, 2003). The impact of animal age on Campylobacter prevalence has been widely described (Nielsen, 2002; Johnsen et al., 2006; Châtre et al., 2010) and our results emphasize this finding. In the present work, thermophilic Campylobacter prevalence in calves was shown to be significantly higher than adult cattle with 99 and 39% of positive animals respectively. Several hypotheses may explain this difference, such as more frequent exposure to Campylobacter in calves, or the acquired immunity in adult animals which may prevent infection (Johnsen et al., 2006). In this study, we also highlighted differences in prevalence estimates according to the type of herd. Indeed, a significantly higher contamination of dairy adult cattle compared with beef adult cattle was observed, while this difference was not observed between calves. However, a previous study highlighting differences according to the herd type, showed the opposite result with a higher prevalence rate in beef cattle compared with dairy animals (Fernandez and Hitschfeld, 2009). Fernandez and Hitschfeld (2009) suggested that this higher prevalence in beef cattle was due to husbandry conditions of animals with a higher exposition to the environment in beef animals. Then, seasonal variations are also described in some studies (Stanley et al., 1998; Hakkinen and Hanninen, 2009).
Here, we thus identified cattle as an important reservoir for thermophilic Campylobacter and for C. jejuni especially. Genotyping using CGF40–90% of C. jejuni isolates highlighted the existence of 45 clusters within 649 isolates, in which six profiles were most prevalent accounting for 76.58% of the overall cattle isolates population. Some of these six most prevalent profiles were frequently found in a large proportion in the different populations of cattle isolates (dairy or beef calves and dairy or beef adult cattle). Nevertheless, each population of isolates showed in addition their own most prevalent profiles (e.g., clusters 63, 37, or 84 in beef calves). Genotyping of C. jejuni isolates from cattle showed the CC-21, CC-61, CC-48, and CC-257 as the most common CCs found in these animals, and are in accordance with a previous study which also described CC-61 or CC-21 as predominant in cattle (Kwan et al., 2008; Grove-White et al., 2011). Based on CGF40–90% clusters, Simpson's diversity index calculated for each C. jejuni population of isolates from cattle did not show any differences in genetic diversity with the exception of adult cattle and calves. Indeed, C. jejuni from calves showed significantly lower genetic diversity than C. jejuni from adults. When compared to clinical isolates, cattle isolates showed a significantly lower genetic diversity.
We genotyped in this study isolates from clinical cases to assess the potential link between cattle and clinical isolates. A significant genotypes overlap was found between clinical and cattle isolates, since 3 of the 4 main clinical CGF40–90% clusters were predominant in cattle isolates, suggesting a significant implication of cattle in clinical cases. However, this observation needs to be nuanced, since with regards to MLST genotypes, 3 of the 4 main CCs found in common between cattle and clinical cases (CC-21, CC-48, CC-257) are frequently described in other hosts (Sheppard et al., 2014; Guyard-Nicodème et al., 2015; Thépault et al., under review). Indeed, CC-21 and CC-48 are defined as host generalist clonal complexes, as they are frequently isolated from various reservoirs (including poultry and pets), while the CC-257 is described as chicken specialist (Sheppard et al., 2014). Therefore, no direct link can be made between these cattle and clinical isolates.
In conclusion, this large scale survey allowed the identification of cattle as a significant reservoir for thermophilic Campylobacter, and especially for C. jejuni. Calves appeared to be significantly more contaminated than adults, as well as dairy cattle compared with beef animals. Genotyping of isolates highlighted the lower genetic diversity of cattle isolates compared with clinical C. jejuni and enabled the first insights in the description of the genetic structure of C. jejuni in cattle. Clonal complexes found in cattle in France are consistent with previously described, but included for the most part of them host generalist clonal complexes. The significant overlap between genotypes found in cattle and clinical isolates from 2009 and 2015 suggest a potential important implication of this animal reservoir in human campylobacteriosis.
Author Contributions
AT, KR, and MC contributed to the conception and design of the work, carried out sampling, and bacteriological analysis, analyzed data, and wrote the paper. TP and SQ carried out sampling and bacteriological analysis. SQ, VR, and AT performed typing experiments.
Funding
This work was supported by France AgriMer (Ministère de l'Agriculture et de l'Alimentation), the General Council of Côtes d'Armor (Conseil Départemental des Côtes d'Armor) and the General Directorate for Army (Direction Générale de l'Armement).
Conflict of Interest Statement
The authors declare that the research was conducted in the absence of any commercial or financial relationships that could be construed as a potential conflict of interest.
Acknowledgments
We would like to thank the slaughterhouse in which the survey was conducted for having accepted to host this study. We would like to thank the National Reference Center for Campylobacter and Helicobacter in France for kindly providing the clinical strains. We also thank Stéphanie Bougeard and Nicolas Rose for their help in statistics.
References
Bessède, E., Lehours, P., Labadi, L., Bakiri, S., and Mégraud, F. (2014). Comparison of characteristics of patients infected by Campylobacter jejuni, Campylobacter coli, and Campylobacter fetus. J. Clin. Microbiol. 52, 328–330. doi: 10.1128/JCM.03029-13
Bessède, E., Solecki, O., Sifré, E., Labadi, L., and Mégraud, F. (2011). Identification of Campylobacter species and related organisms by matrix assisted laser desorption ionization-time of flight (MALDI-TOF) mass spectrometry. Clin. Microbiol. Infect. 17, 1735–1739. doi: 10.1111/j.1469-0691.2011.03468.x
Carriço, J. A., Silva-Costa, C., Melo-Cristino, J., Pinto, F. R., de Lencastre, H., Almeida, J. S., et al. (2006). Illustration of a common framework for relating multiple typing methods by application to macrolide-resistant Streptococcus pyogenes. J. Clin. Microbiol. 44, 2524–2532. doi: 10.1128/JCM.02536-05
Cha, W., Mosci, R. E., Wengert, S. L., Venegas Vargas, C., Rust, S. R., Bartlett, P. C., et al. (2017). Comparing the genetic diversity and antimicrobial resistance profiles of Campylobacter jejuni recovered from cattle and humans. Front. Microbiol. 8:818. doi: 10.3389/fmicb.2017.00818
Châtre, P., Haenni, M., Meunier, D., Botrel, M. A., Calavas, D., and Madec, J. Y. (2010). Prevalence and antimicrobial resistance of Campylobacter jejuni and Campylobacter coli isolated from cattle between 2002 and 2006 in France. J. Food Prot. 73, 825–831. doi: 10.4315/0362-028X-73.5.825
Deckert, A. E., Taboada, E., Mutschall, S., Poljak, Z., Reid-Smith, R. J., Tamblyn, S., et al. (2014). Molecular epidemiology of Campylobacter jejuni human and chicken isolates from two health units. Foodborne Pathog. Dis. 11, 150–155. doi: 10.1089/fpd.2013.1610
de Haan, C. P., Kivistö, R. I., Hakkinen, M., Corander, J., and Hänninen, M. L. (2010). Multilocus sequence types of Finnish bovine Campylobacter jejuni isolates and their attribution to human infections. BMC Microbiol. 10:200. doi: 10.1186/1471-2180-10-200
Dingle, K. E., Colles, F. M., Wareing, D. R., Ure, R., Fox, A. J., Bolton, F. E., et al. (2001). Multilocus sequence typing system for Campylobacter jejuni. J. Clin. Microbiol. 39, 14–23.
EFSA and ECDC (2016). The European Union summary report on trends and sources of zoonoses, zoonotic agents and food-borne outbreaks in 2015. EFSA J. 14:4634. doi: 10.2903/j.efsa.2016.4634
Fernández, A. M., Balasegaram, S., Willis, C., Wimalarathna, H. M., Maiden, M. C., and McCarthy, N. D. (2015). Partial failure of milk pasteurization as a risk for the transmission of Campylobacter from cattle to humans. Clin. Infect. Dis. 61, 903–909. doi: 10.1093/cid/civ431
Fernandez, H., and Hitschfeld, M. (2009). Occurrence of Campylobacter jejuni and Campylobacter coli and their biotypes in beef and dairy cattle from the south of Chile. Braz. J. Microbiol. 40, 450–454. doi: 10.1590/S1517-83822009000300005
Grove-White, D. H., Leatherbarrow, A. J., Cripps, P. J., Diggle, P. J., and French, N. P. (2011). Molecular epidemiology and genetic diversity of Campylobacter jejuni in ruminants. Epidemiol. Infect. 139, 1661–1671. doi: 10.1017/S0950268810002736
Guyard-Nicodème, M., Rivoal, K., Houard, E., Rose, V., Quesne, S., Mourand, G., et al. (2015). Prevalence and characterization of Campylobacter jejuni from chicken meat sold in French retail outlets. Int. J. Food Microbiol. 203, 8–14. doi: 10.1016/j.ijfoodmicro.2015.02.013
Hakkinen, M., and Hanninen, M. L. (2009). Shedding of Campylobacter spp. in Finnish cattle on dairy farms. J. Appl. Microbiol. 107, 898–905. doi: 10.1111/j.1365-2672.2009.04269.x
Hakkinen, M., Heiska, H., and Hanninen, M. L. (2007). Prevalence of Campylobacter spp. in cattle in Finland and antimicrobial susceptibilities of bovine Campylobacter jejuni strains. Appl. Environ. Microbiol. 73, 3232–3238. doi: 10.1128/AEM.02579-06
Havelaar, A. H., Ivarsson, S., Löfdahl, M., and Nauta, M. J. (2013). Estimating the true incidence of campylobacteriosis and salmonellosis in the European Union (2009). Epidemiol. Infect. 141, 293–302. doi: 10.1017/S0950268812000568
Hunter, P. R., and Gaston, M. A. (1988). Numerical index of the discriminatory ability of typing systems: an application of Simpson's index of diversity. J. Clin. Microbiol. 26, 2465–2466.
Johnsen, G., Zimmerman, K., Lindstedt, B. A., Vardund, T., Herikstad, H., and Kapperud, G. (2006). Intestinal carriage of Campylobacter jejuni and Campylobacter coli among cattle from south-western Norway and comparative genotyping of bovine and human isolates by amplified-fragment length polymorphism. Acta Vet. Scand. 48:4. doi: 10.1186/1751-0147-48-4
Jolley, K. A., and Maiden, M. C. (2010). BIGSdb: scalable analysis of bacterial genome variation at the population level. BMC Bioinformatics 11:595. doi: 10.1186/1471-2105-11-595
Kwan, P. S., Birtles, A., Bolton, F. J., French, N. P., Robinson, S. E., Newbold, L. S., et al. (2008). Longitudinal study of the molecular epidemiology of Campylobacter jejuni in cattle on dairy farms. Appl. Environ. Microbiol. 74:3626–3633. doi: 10.1128/AEM.01669-07
Machin, D., Campbell, M. J., Tan, S.-B., and Tan, S.-H. (2009). Sample Size Tables for Clinical Studies, 3rd Edn. Oxford: John Wiley and Sons.
Méric, G., Yahara, K., Mageiros, L., Pascoe, B., Maiden, M. C., Jolley, K. A., et al. (2014). A reference pan-genome approach to comparative bacterial genomics: identification of novel epidemiological markers in pathogenic Campylobacter. PLoS ONE 9:e92798. doi: 10.1371/journal.pone.0092798
Milnes, A. S., Stewart, I., Clifton-Hadley, F. A., Davies, R. H., Newell, D. G., Sayers, A. R., et al. (2008). Intestinal carriage of verocytotoxigenic Escherichia coli O157, Salmonella, thermophilic Campylobacter and Yersinia enterocolitica, in cattle, sheep and pigs at slaughter in Great Britain during 2003. Epidemiol. Infect. 136, 739–751. doi: 10.1017/S0950268807009223
Mughini Gras, L., Smid, J. H., Wagenaar, J. A., de Boer, A. G., Havelaar, A. H., Friesema, I. H., et al. (2012). Risk factors for campylobacteriosis of chicken, ruminant, and environmental origin: a combined case-control and source attribution analysis. PLoS ONE 7:e42599. doi: 10.1371/journal.pone.0042599
Nielsen, E. M. (2002). Occurrence and strain diversity of thermophilic campylobacters in cattle of different age groups in dairy herds. Lett. Appl. Microbiol. 35, 85–89. doi: 10.1046/j.1472-765X.2002.01143.x
Rose, V., Thépault, A., Lemercier, A., Mégraud, F., Chemaly, M., and Rivoal, K. (2017). “A stable and highly diverse genetic structure in clinical Campylobacter jejuni isolates in 2009 and 2015,” in Abstracts of the 19th International Workshop on Campylobacter, Helicobacter and related organisms (CHRO), 10-14 September 2017 (Nantes), 148.
Rotariu, O., Dallas, J. F., Ogden, I. D., MacRae, M., Sheppard, S. K., Maiden, M. C., et al. (2009). Spatiotemporal homogeneity of Campylobacter subtypes from cattle and sheep across northeastern and southwestern Scotland. Appl. Environ. Microbiol. 75, 6275–6281. doi: 10.1128/AEM.00499-09
Severiano, A., Carriço, J. A., Robinson, D. A., Ramirez, M., and Pinto, F. R. (2011). Evaluation of jackknife and bootstrap for defining confidence intervals for pairwise agreement measures. PLoS ONE 6:e19539. doi: 10.1371/journal.pone.0019539
Sheppard, S. K., Cheng, L., Méric, G., de Haan, C. P., Llarena, A. K., Marttinen, P., et al. (2014). Cryptic ecology among host generalist Campylobacter jejuni in domestic animals. Mol. Ecol. 23:2442–2451. doi: 10.1111/mec.12742
Sheppard, S. K., Jolley, K. A., and Maiden, M. C. (2012). A Gene-by-Gene approach to bacterial population genomics: whole genome MLST of Campylobacter. Genes 3, 261–277. doi: 10.3390/genes3020261
Stanley, K., and Jones, K. (2003). Cattle and sheep farms as reservoirs of Campylobacter. J Appl Microbiol 94(Suppl.), 104S−113S. doi: 10.1046/j.1365-2672.94.s1.12.x
Stanley, K. N., Wallace, J. S., Currie, J. E., Diggle, P. J., and Jones, K. (1998). The seasonal variation of thermophilic campylobacters in beef cattle, dairy cattle and calves. J. Appl. Microbiol. 85, 472–480. doi: 10.1046/j.1365-2672.1998.853511.x
Strachan, N. J., MacRae, M., Thomson, A., Rotariu, O., Ogden, I. D., and Forbes, K. J. (2012). Source attribution, prevalence and enumeration of Campylobacter spp. from retail liver. Int. J. Food Microbiol. 153, 234–236. doi: 10.1016/j.ijfoodmicro.2011.10.033
Taboada, E. N., Ross, S. L., Mutschall, S. K., Mackinnon, J. M., Roberts, M. J., Buchanan, C. J., et al. (2012). Development and validation of a comparative genomic fingerprinting method for high-resolution genotyping of Campylobacter jejuni. J. Clin. Microbiol. 50, 788–797. doi: 10.1128/JCM.00669-11
Thépault, A., Rose, V., Gourmelon, M., Mégraud, F., Chemaly, M., and Rivoal, K. (2016). “A large scale survey describing the relationship between different animal reservoirs and human campylobacteriosis,” in International Association for Food Protection Annual Meeting (Saint Louis, MI).
Thépault, A., Méric, G., Rivoal, K., Pascoe, B., Mageiros, L., Touzain, F., et al. (2017). Genome-wide identification of host-segregating epidemiological markers for source attribution in Campylobacter jejuni. Appl. Environ. Microbiol. 83:e03085-16. doi: 10.1128/AEM.03085-16
Van Cauteren, D., De Valk, H., Sommen, C., King, L. A., Jourdan-Da Silva, N., Weill, F. X., et al. (2015). Community incidence of Campylobacteriosis and Nontyphoidal Salmonellosis, France, 2008-2013. Foodborne Pathog. Dis. 12, 664–669. doi: 10.1089/fpd.2015.1964
Wilson, D. J., Gabriel, E., Leatherbarrow, A. J., Cheesbrough, J., Gee, S., Bolton, E., et al. (2008). Tracing the source of campylobacteriosis. PLoS Genet. 4:e1000203. doi: 10.1371/journal.pgen.1000203
Keywords: Campylobacter jejuni, MLST, CGF40, bovine, foodborne disease
Citation: Thépault A, Poezevara T, Quesne S, Rose V, Chemaly M and Rivoal K (2018) Prevalence of Thermophilic Campylobacter in Cattle Production at Slaughterhouse Level in France and Link Between C. jejuni Bovine Strains and Campylobacteriosis. Front. Microbiol. 9:471. doi: 10.3389/fmicb.2018.00471
Received: 26 December 2017; Accepted: 28 February 2018;
Published: 19 March 2018.
Edited by:
Adolfo J. Martinez-Rodriguez, Instituto de Investigación en Ciencias de la Alimentación (CIAL), SpainReviewed by:
Beatrix Stessl, Veterinärmedizinische Universität Wien, AustriaBeatriz Melero, University of Burgos, Spain
Copyright © 2018 Thépault, Poezevara, Quesne, Rose, Chemaly and Rivoal. This is an open-access article distributed under the terms of the Creative Commons Attribution License (CC BY). The use, distribution or reproduction in other forums is permitted, provided the original author(s) and the copyright owner are credited and that the original publication in this journal is cited, in accordance with accepted academic practice. No use, distribution or reproduction is permitted which does not comply with these terms.
*Correspondence: Katell Rivoal, katell.rivoal@anses.fr