- 1Nematology Institute of Northern China, Shenyang Agricultural University, Shenyang, China
- 2Institute of Plant Protection, Liaoning Academy of Agricultural Sciences, Shenyang, China
- 3College of Biological Science and Technology, Shenyang Agricultural University, Shenyang, China
- 4College of Plant Protection, Shenyang Agricultural University, Shenyang, China
- 5College of Sciences, Shenyang Agricultural University, Shenyang, China
Soybean is an important economic crop that is often adversely affected by infection in the field with the soybean cyst nematode Heterodera glycines. Biological control is an eco-friendly method used to protect the crop against disease. The bacterium Klebsiella pneumoniae has been reported to protect rice from sheath blight and seedling blight, but its role in the control of nematode is unclear. In this study, the effect of K. pneumoniae SnebYK on the control of H. glycines was assessed. Potting experiment results showed that coating soybean seeds with K. pneumoniae SnebYK not only reduced the infection rate of H. glycines but also decreased the proportion of adult female nematodes. Field experiment results showed that K. pneumoniae SnebYK reduced both the number of H. glycines in soybean roots and the number of adult females. However, K. pneumoniae SnebYK caused low juvenile mortality in an in vitro assay. To further analyze the role of K. pneumoniae SnebYK in the inhibition of H. glycines infection, split root experiments were conducted. The results indicated that K. pneumoniae SnebYK controls H. glycines via induced systemic resistance, which reduces H. glycines penetration. Klebsiella pneumoniae SnebYK treatment also significantly increased the proportion of second-stage juveniles and decreased the proportions of third- and fourth-stage juveniles in the H. glycines population. Moreover, 48 h after inoculation with H. glycines, the expression levels of PR1, PR2, PR5, and PDF1.2 were significantly higher in soybeans pretreated with K. pneumoniae SnebYK than in control soybeans. Interestingly, besides providing protection against nematodes, K. pneumoniae SnebYK fixed nitrogen, produced ammonia, solubilized phosphate, and produced siderophores, leading to well-developed root system and an increase in soybean seedling fresh weight. These results demonstrate for the first time that K. pneumoniae SnebYK not only promotes soybean growth but also inhibits the invasion and development of H. glycines by inducing systemic resistance.
Introduction
Soybean (Glycine max) is an important crop worldwide due to its nutritive and commercial value. The soybean cyst nematode (Heterodera glycines Ichinohe) is a soil-borne plant-parasitic nematode that disturbs the root growth of soybean and causes the early yellowing of plants (Subbotin et al., 2010). As a result of the widespread distribution of H. glycines and its ability to reduce seed yield, H. glycines is considered the most harmful pest that endangers soybean yield globally (Koenning and Wrather, 2010; Rincker et al., 2017). Depending on the severity of the infection, H. glycines generally decreases soybean production by 5–100% in northeast China, a major soybean production region (Duan, 2011). Meanwhile, in the United States, the loss caused by H. glycines has been estimated to exceed one billion USD annually (Koenning and Wrather, 2010). Thus, finding a simple, eco-friendly, and effective approach to control H. glycines is imperative for modern agriculture.
The use of plant growth-promoting bacteria (PGPB) is an ideal approach for the prevention and control of nematodes. PGPB promote plant growth and also induce systemic resistance (Wei et al., 1996; Kloepper et al., 2004). Induced systemic resistance (ISR) is a phenomenon whereby resistance to an infectious disease is systemically induced by localized infection, by treatment with microbial components or products or with a diverse group of structurally unrelated organic and inorganic compounds (Kuć, 2001). Jasmonic acid (JA)/ethylene (ET), and/or salicylic acid (SA) signaling pathways are important in the regulation of beneficial microbes-mediated ISR (Pieterse et al., 2014; Vryzas, 2016). In recent years, ISR mediated by PGPB (e.g., Bacillus sp., Bacterium sp., Methylomonas methanica, Rhizobium etli) has been intensively studied against a wide range of important plant-parasitic nematodes, such as Meloidogyne incognita (Anter et al., 2014; Alfianny et al., 2017), H. glycines (Xiang et al., 2013), and Globodera pallida (Reitz et al., 2000). Tian et al. (2014) reported that Sinorhizobium fredii decreased the infection rate of H. glycines by triggering ISR and also prolonged the developmental stage of H. glycines in the root to 30 days, compared with 27 days in the control. Xiang et al. (2013) also reported that treating soybean seeds with B. simplex reduced the H. glycines population by triggering ISR. Therefore, seed coating with PGPB is a simple and cost-effective strategy that elicits ISR in host plants against pathogens (Van Loon et al., 1998; Ramamoorthy et al., 2001; Pathak et al., 2016). After seed coating, these plants acquire broad-spectrum resistance without any changes to their genome sequences, and respond more rapidly and strongly to various stresses (Pathak et al., 2016).
Klebsiella pneumoniae is a strain of the PGPB that promotes plant growth by fixing nitrogen (Iniguez et al., 2004); producing 1-aminocyclopropane-1-carboxylate deaminase (Singh et al., 2015), indole-3-acetic acid (Sachdev et al., 2009), gibberellic acid (Singh et al., 2015), and siderophores; and solubilizing phosphate (Ji et al., 2014). Klebsiella sp. KW7-S06 elicited ISR to the pathogenic fungi Fusarium oxysporum and Rhizoctonia solani in rice, alleviating disease symptoms (Ji et al., 2014). However, reports of K. pneumoniae-elicited ISR to nematodes are few. Furthermore, finding a candidate biocontrol agent against H. glycines would have great significance for the soybean industry. In the present study, we aimed to (1) assess the plant growth-promoting properties of the K. pneumoniae strain SnebYK and its effect on soybean growth, (2) evaluate the effect of the K. pneumoniae strain SnebYK on the control of H. glycines via seed coating, and (3) investigate the ability of the K. pneumoniae strain SnebYK to induce systemic resistance to H. glycines in soybean.
Materials and Methods
Strain, Fermentation Broth, and Nematode
Klebsiella pneumoniae strain SnebYK, subsequently referred to as SnebYK, is a Gram-negative facultatively anaerobic bacterium that was isolated from mud at the outlet of a pesticide manufacturing factory in Liaoning Province, China. SnebYK was identified by Luo (2010) and stored at -80°C at the Nematology Institute of Northern China at Shenyang Agricultural University. Before use, the strain was streaked onto a nutrient agar medium and incubated at 28°C to confirm purity. A single colony was selected, transferred to nutrient agar medium, and incubated for 48 h at 28°C. The SnebYK fermentation broth (1 × 109 CFU ml-1) was prepared according to the method previously described by Chen et al. (2014).
The population of H. glycines (race 3, HG type 0) was maintained on soybean [Glycine max (L.) Merr. ‘Liaodou15’] grown in the experimental field of the Nematology Institute of Northern China. Heterodera glycines specimens were morphologically identified following the method previously described by Subbotin et al. (2010) prior to use in the experiment. Cysts were extracted from soybean rhizosphere soil in the experimental field using a floating sieve, and then surface-sterilized with 0.5% sodium hypochlorite. Second-stage juveniles (J2) were incubated as described by Tian et al. (2014). The hatched J2 were assessed for survival and collected in a beaker for inoculation.
Biochemical Assays of Putative Plant Growth-Promoting Properties
The siderophore production of SnebYK was determined as described by Schwyn and Neilands (1987). SnebYK was inoculated in the center of a chrome azurol S agar plate and incubated at 28°C for 7 days; plates were observed to determine whether a yellow–orange halo appeared around the colony. Assays of inorganic and organic phosphate solubilization by SnebYK were performed using the National Botanical Research Institute’s phosphate growth medium and Mongina organic culture medium with lecithin, respectively (Hao et al., 2012; Ji et al., 2014). SnebYK was spot-inoculated onto the plate and incubated at 28°C for at least 7 days. A clear zone around the colony indicated that the strain tested had phosphate solubilization activity. The phosphate-solubilizing ability of SnebYK was evaluated using the phosphomolybdate blue colorimetric method (Murphy and Riley, 1962). Ammonia production was detected with Nessler’s reagent (Singh et al., 2015).
The nitrogen-fixing ability of SnebYK was tested using ACCC55 agar plates (Gao et al., 2015). The nitrogenase activity of SnebYK was evaluated through an acetylene reduction assay, following the protocol of Boddey and Knowles (1987). To analyze the nifH gene of SnebYK, genomic DNA was extracted from the SnebYK strain using a bacterial genomic DNA extraction kit (Tiangen Biotech, China). The primers nifH1 (5′-ADNGCCATCATYTCNCC-3′) and nifH2 (5′-TGYGAYCCNAARGCNGA-3′) were used for polymerase chain reaction (PCR) amplification of the nifH gene (Gaby and Buckley, 2012). The PCR amplification conditions were 5 min at 94°C for initial denaturation; 35 cycles of 94°C for 1 min, 57°C for 1 min, and 72°C for 1 min, followed by a 72°C extension for 10 min (Liu et al., 2011). PCR products were analyzed by electrophoresis on 1.2% agarose gels and then sequenced by Sangon Biotech Co. Ltd., Shanghai, China. The obtained sequences were compared with those in the GenBank database (RRID: SCR_002760) using the NCBI BLAST algorithm (Pinto-Tomás et al., 2009; Liu et al., 2011; Lin et al., 2012). The phylogenetic tree was established using the neighbor-joining method in Mega 7.0.26. To detect the expression of nifH, reverse transcription (RT)-PCR analysis was conducted. SnebYK colonies grown on ACCC55 agar plates were collected, and colonies grown on nutrient agar plates were also collected as controls. Total RNA was extracted from the samples using a bacterial total RNA extraction kit (Tiangen Biotech, China), and the PrimeScript RT Reagent kit (TaKaRa, China) was used for cDNA synthesis following the manufacturer’s instructions. The primers used to amplify nifH were PolF (5′-TGCGAYCCSAARGCBGACTC-3′) and PolR (5′-ATSGCCATCATYTCRCCGGA-3′) (Poly et al., 2001). The 16S rRNA gene was used as an internal control. For the PCR, 100 ng cDNA was used, with the following program: 94°C for 4 min; 30 cycles of 94°C for 30 s, 60°C for 30 s, and 72°C for 30 s, followed by an extension at 72°C for 5 min. PCR products were visualized using the GoldView DNA staining reagent (Aidlab Biotech, China) following agarose gel electrophoresis and analysis.
Growth Promotion Assay in Soybeans Treated With SnebYK
Soybean seeds were surface-sterilized with 0.5% sodium hypochlorite, washed with sterilized water at least three times, and then dried at room temperature. Treated soybean seeds were coated with the SnebYK fermentation broth at a 70:1 mass ratio. Control soybean seeds were coated with sterilized water. All seeds were sown in 10 cm × 10 cm plastic pots containing a sterilized mixture of soil and sand (1:1, v/v) and grown in a greenhouse at 26/21°C with a 16/8 h photoperiod. The soybean seedlings were thinned to one plant per pot once two euphylla were observed. Each treatment included 10 replicates and was arranged in a completely randomized design. Thirty days after sowing, soybean seedlings were harvested from all pots, the soil adhered to the roots was carefully removed with tap water, and the shoot length, taproot length, and fresh weight of the plants in each treatment were measured. Root morphology images were obtained using a scanner (Expression 10000 XL, Epson, Japan) and then analyzed using the WinRHIZO image analysis system (V4.1c, Regent Instruments, Canada).
Effect of SnebYK on H. glycines Control
Juvenile Mortality in Vitro Assay
To obtain the culture filtrate, SnebYK fermentation broth (1 × 109 CFU ml-1) was centrifuged for 30 min at 12,000 rpm. SnebYK filtrate (1 ml) was added to each well of a 12-well tissue culture plate containing 1 ml suspension of freshly hatched H. glycines J2 (35–50 juveniles ml-1, surface-sterilized). Filtrate of B. megaterium fermentation broth (1 × 109 CFU ml-1), which showed high contact toxicity to H. glycines J2 in our previous work (Zhou et al., 2017), was used as a positive control; sterile water was used as a negative control. The plate was incubated in the dark at 25 ± 2°C. Immotile J2 were considered dead when they were needled without reaction (Cayrol et al., 1989). The number of dead J2 was counted under a microscope (SMZ800, Nikon, Japan) after 12 and 24 h of incubation. Each treatment included three replicates, and the experiment was repeated three times.
Potting and Field Experiments
Soybean seeds were surface-sterilized and coated with SnebYK as described above, and the control seeds were coated with sterilized water. When soybean seedlings grew to the two-euphylla stage, their roots were inoculated with 2,000 J2 of H. glycines. Thirty days after nematode inoculation, the soybean roots were harvested, and the adult female nematodes on the entire root surface were counted. The roots were then washed with tap water, stained with NaClO-acid fuchsin, and the number of H. glycines in each root was counted (Bybd et al., 1983). Adult females and nematodes in the soil were screened by sieving and counted under a stereomicroscope (Liu, 1995). To ascertain the effect of SnebYK on soybean seedlings growth under H. glycines stress, the shoot length, taproot length, and fresh weight of seedlings were measured. The root morphology was analyzed using the WinRHIZO image analysis system as previously described. In each case, there were seven replicates, and the entire experiment was repeated twice.
Field trials were carried out in a field infested with H. glycines at the experimental test site of the Modern Agricultural Industry Technology System of China in Kangping, located at 42°43′N, 123°24′E, during the growing seasons of 2016 and 2017. Soybean seeds were coated with SnebYK or sterile water in a 70:1 mass ratio. Seeds for each treatment were then sown in six rows of 70 seeds with 10 cm intervals between seeds, in a 3.5 m × 7 m plot. A randomized complete block design was adopted in this experiment, and each treatment included five plots. Thirty days after sowing, 12 seedlings were randomly selected from the inner four rows of each plot and nematode infections were evaluated. Adult females of H. glycines on the roots and in the rhizosphere soil (100 ml), and the nematodes in the roots, were counted as described above. The shoot length, taproot length, and fresh weight of the soybean seedlings were also measured. The field experiment was conducted in a fenced test field kept separate from animals and other crops.
Split Root Experiment
Soybean seeds were surface-sterilized as described and sown in pots containing the sterilized soil mixture, with one seed per pot. When soybean seedlings had two euphylla, the roots were removed from the mixture and washed thoroughly with running tap water. Roots of approximately the same size were selected and divided into two equal parts with a scalpel: one part was designated the inducer root system, and the other part was designated the responder root system (Adam et al., 2014). The inducer and responder roots were transplanted into two plastic pots, with 200 ml of sterilized mixture in each. When the two parts of the root systems had been successfully fixed, the inducer root system was inoculated either with SnebYK fermentation broth (10 ml, 1 × 109 CFU ml-1) or with sterile water as a control. After 5 days of treatment, all responder roots were inoculated with 2,000 J2 of H. glycines as shown in Figure 1A. Fifteen days after nematode inoculation, the responder roots were stained with NaClO-acid fuchsin (Bybd et al., 1983). The number of H. glycines in the roots was counted, and the developmental stages of H. glycines were recorded simultaneously. Each treatment had four repetitions, and the entire experiment was repeated twice.
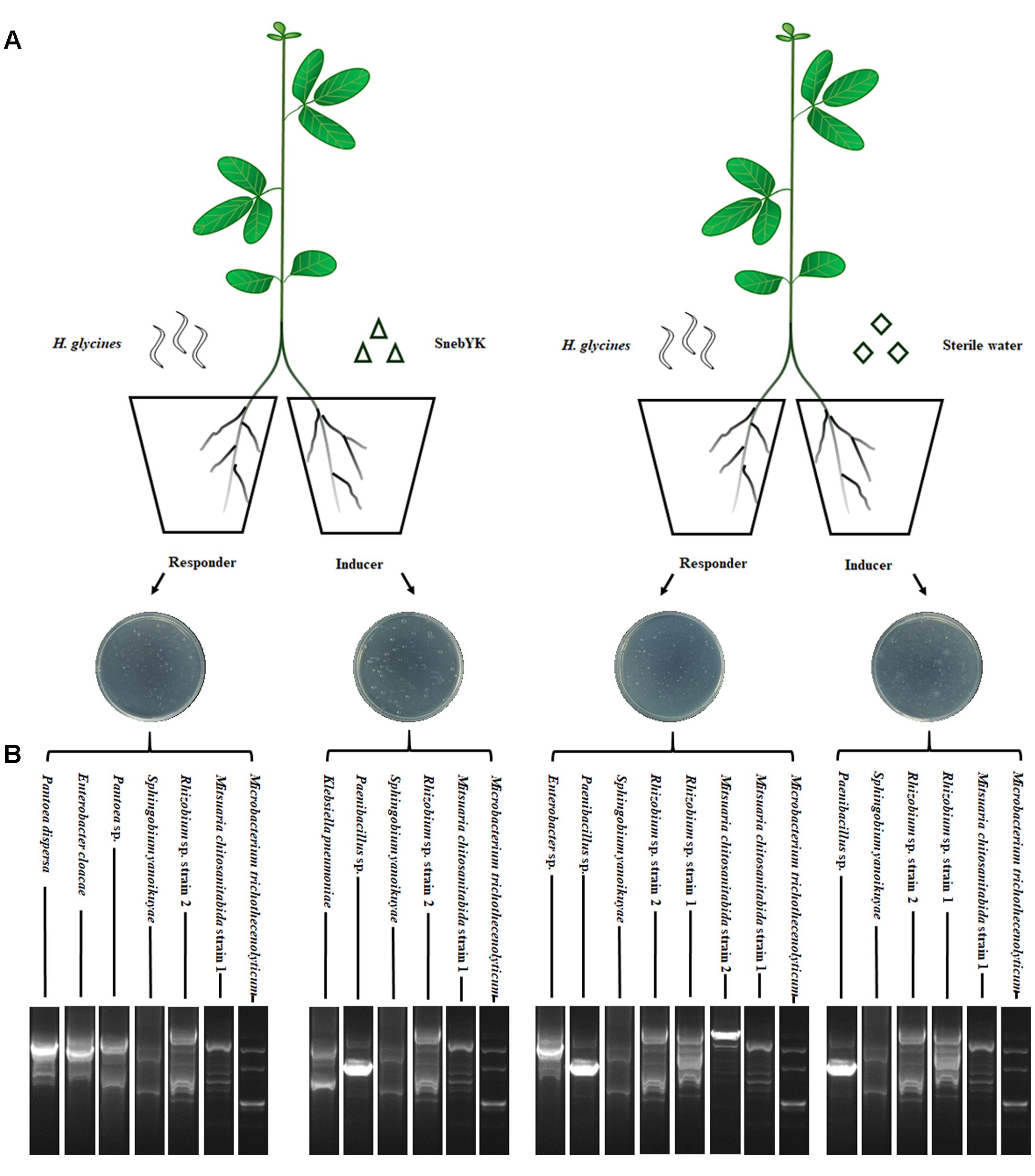
FIGURE 1. Split root system used to study induced systemic resistance against Heterodera glycines in soybean. (A) SnebYK and H. glycines were inoculated into opposite pots containing the inducer and responder root systems, respectively. Controls were inoculated with sterile water. (B) BOX-PCR fingerprints and 16S rRNA identification of rhizosphere/endophytic bacteria in the split root system.
To verify the validity of the split root system (SRS), BOX-PCR analysis and 16S rRNA gene sequencing were performed to test the colonization of the root system by SnebYK. Twenty days after the inducer roots were inoculated with SnebYK or sterile water, the responder and inducer root systems were removed. Immediately, 0.5 g of each root was collected and ground with a tissue grinder to release the rhizosphere and endophytic bacteria. A bacterial suspension was obtained after adding 1 ml of sterile water to the tissue grinder. After further dilution with sterile water (1,000×), 100 μl of the suspension was spread onto an ACCC55 agar plate and incubated at 28°C. Each treatment included three replications, and all steps were conducted under sterile conditions. After 72 h, morphologically distinct colonies of bacteria were selected and cultured separately. The genomic DNA of each strain was extracted with a bacterial genomic DNA extraction kit (Tiangen Biotech, China). The electrophoretograms of the BOX-PCR products were used to screen out different bacterial strains and could also be used to investigate the colonization of SnebYK by comparing them with the SnebYK electrophoretogram. The primer used for the BOX-PCR was BOXA1R (5′-CTACGGCAAGGCGACGCTGACG-3′) (Versalovic et al., 1994). The amplification was conducted following the method reported by Ateba and Mbewe (2013) in a 25 μl reaction mixture with the DNA samples obtained from the bacterial strains found in the SRS. Strains with different fingerprinting profiles were identified by 16S rRNA gene sequencing as described by Sarma and Saikia (2014).
RNA Isolation and Real-Time PCR Analysis of Gene Expression
Soybeans were treated with SnebYK and grown as previously described for the pot experiment. Sterilized water was used as control. When soybean seedlings reached the two-euphylla stage, their roots were inoculated with 2,000 J2 of H. glycines. To measure the transcript levels of defense-related genes in real time, soybean roots were collected at 0, 24, and 48 h after nematode inoculation, froze immediately in liquid nitrogen and stored at -80°C until use. Total RNA was extracted from the soybean roots using the MiniBEST Universal RNA Extraction kit (TaKaRa, China). Total RNA from each sample (1 μg) was reverse-transcribed using the PrimeScript RT Reagent kit (TaKaRa, China). Quantitative real-time PCR (qRT-PCR) was performed in a CFX Connect Real-Time PCR Detection System (Bio-Rad, United States) using a SYBR green I quantitative PCR master mix (TaKaRa, China). The reaction conditions were 95°C for 30 s followed by 40 cycles at 95°C for 5 s, and 60°C for 30 s (fluorescence measurement step). After 40 cycles, a melting-curve analysis was performed (15 s at 95°C, 30 s at 60°C, and 15 s at 95°C, fluorescence measurement step). Each sample included three replicates. Expression of the soybean gene Actin11 was used as an internal standard for normalization, and the data were quantified using the 2-ΔΔCt method (Livak and Schmittgen, 2001).
Statistical Analysis
All data were analyzed using SPSS Statistics 19.0 (RRID: SCR_002865) and expressed as the mean ± standard deviation. Significant differences among treatments in the in vitro juvenile mortality assay were determined according to Duncan’s multiple range test (P < 0.05). Significant differences between treatments in other experiments were evaluated using t-tests (P < 0.05).
Results
Plant Growth-Promoting Properties of SnebYK
Klebsiella pneumoniae has been reported to possess many plant growth-promoting properties. Therefore, several such properties, including nitrogen fixation, ammonia production, phosphate solubilization, and siderophore production, were analyzed in SnebYK (Table 1). An orange halo zone was observed on the chrome azurol S agar plate, indicating that SnebYK produced siderophores. The ability of SnebYK to solubilize inorganic phosphate was confirmed by the clear zone around SnebYK on the National Botanical Research Institute’s phosphate agar plate. However, nothing was observed on the Mongina agar plate, which indicates that SnebYK could not solubilize organic phosphate. SnebYK grown on the nitrogen-free medium showed high nitrogenase activity. The yellow color observed following the addition of Nessler’s reagent to culture filtrate indicated that SnebYK could produce ammonia. A 363 bp amplicon specific to nifH (GenBank accession number: MF580385), a functioning gene encoding nitrogenase iron protein, was obtained from the genomic DNA of SnebYK (Figure 2A). The RT-PCR results showed that the nifH gene was expressed (1.24-fold the level of the internal control) when SnebYK was cultured under nitrogen-free conditions but was not expressed when SnebYK was cultured in a medium with sufficient nitrogen (Figure 2B). Phylogenetic analysis revealed that the nifH sequence of SnebYK was closely related to that of the K. pneumoniae strain NG14 and other strains in the genus Klebsiella (Figure 2C).
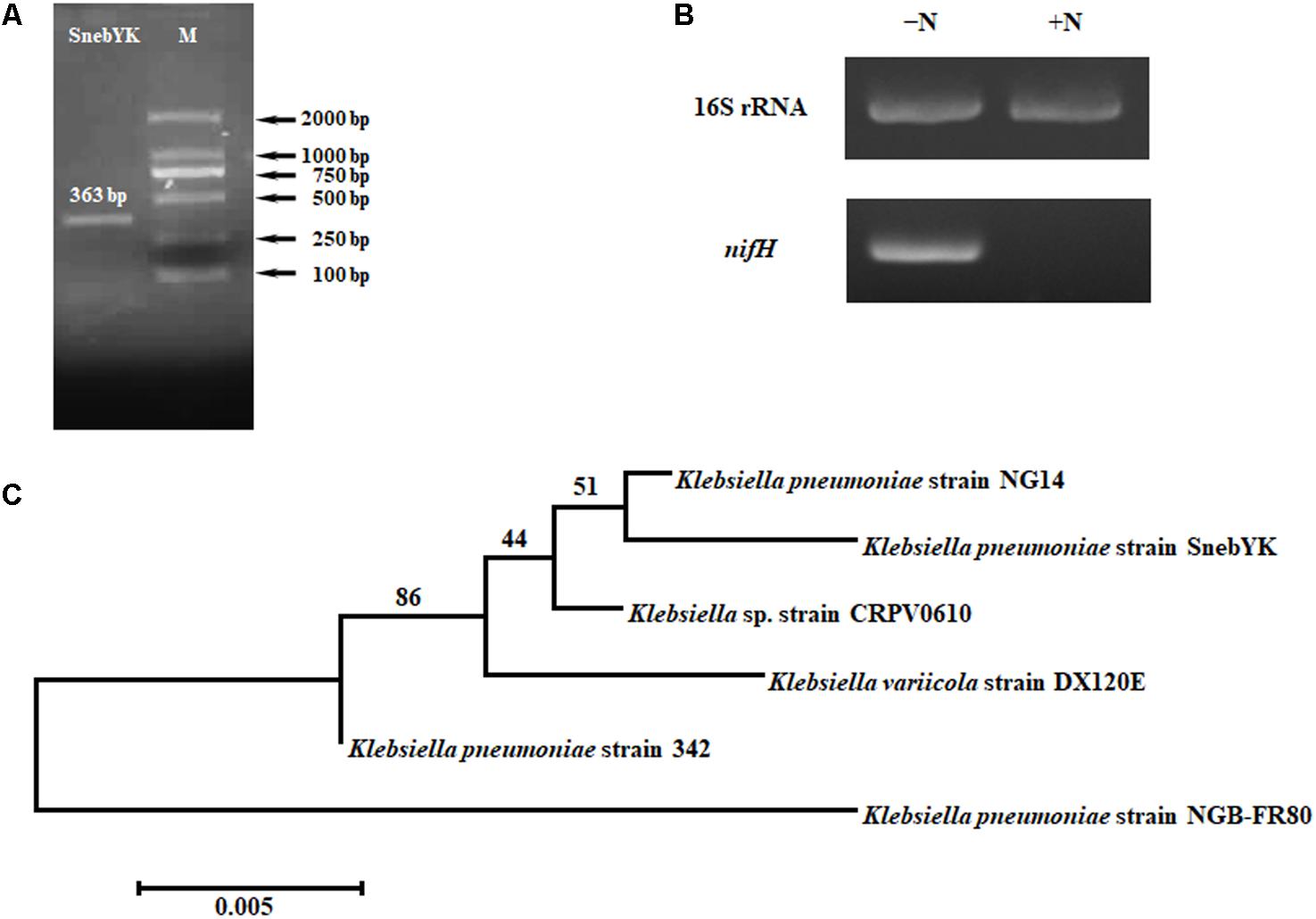
FIGURE 2. Amplification and analysis of the nifH gene of SnebYK. (A) Amplicon of SnebYK nifH (363 bp) in the left lane and DNA ladder in the right lane. (B) Transcriptional analysis of the SnebYK nifH gene in nitrogen-free medium determined with RT-PCR. The lane labeled “-N” shows the expression of the 16S rRNA and nifH gene of SnebYK grown in ACCC55 nitrogen-free medium; the lane labeled “+N” shows the expression of the 16S rRNA and nifH gene of SnebYK grown in nutrient agar medium. The transcript level of the 16S rRNA was used as a loading control; the transcript level of nifH of SnebYK grown in nutrient agar medium was used as a negative control. (C) Dendrogram based on the nifH sequences of SnebYK and other strains in the genus Klebsiella with similar nifH sequences. This analysis was performed using the neighbor-joining method in Mega 7.0.26 with a bootstrap value of n = 1000.
Effect of SnebYK on the Growth of Soybean Seedlings
Since SnebYK exhibits a variety of growth-promoting properties, the effect of SnebYK on soybean growth was evaluated. SnebYK-treated soybean plants had well-developed second compound leaves and roots (Figure 3). The second compound leaves of untreated soybean were not fully expanded, and their roots were sparse. In the presence of SnebYK, soybean seedlings exhibited increased biomass (Table 2). SnebYK caused significant increases in the shoot length and fresh weight, which were 9.18% and 39.51% higher, respectively, than those in the control. However, SnebYK did not affect taproot length or the root–shoot ratio. SnebYK also had a considerable impact on root growth. SnebYK increased the total root length, total root surface area, and total root volume by 52.10%, 47.73%, and 42.72%, respectively, compared to those of the untreated control.
Effect of SnebYK on the Control of H. glycines in the Pot and the Field
SnebYK had low contact toxicity to H. glycines, as the mortality rate after 24 h was only 18.23% (Figure 4A). To evaluate whether coating seeds with SnebYK provided protection against H. glycines, seeds were sown in pots after coating and then the soybeans were inoculated with H. glycines. The number of females significantly influences the nematode population. Thus, we evaluated the effect of SnebYK on the control of both the adult female and the total population of H. glycines. Thirty days after H. glycines inoculation, juveniles and adults, especially adult females, in the soil and the soybean roots were counted. The nematode population of SnebYK-treated soybean was significantly smaller than that of the control, with inhibition rates reaching 47.32% (Figure 4B). Analysis of the population revealed that SnebYK dramatically decreased the numbers of both juveniles and adult males, as well as adult females. Interestingly, the proportion of adult females in the H. glycines population was 40.88% (±4.77%) after treatment with SnebYK, while that in the control was 52.42% (±2.19%). This indicates that SnebYK significantly decreased the proportion of adult females, demonstrating that SnebYK effectively restrained the population of H. glycines and prevented their development into females. In the presence of H. glycines, SnebYK-treated soybean maintained a higher fresh root weight than the untreated control, concomitantly with a significantly increased root–shoot ratio (Table 3). After analysis of the root system, we found that the total root volume of SnebYK-treated soybean was significantly higher, by 28.74%, than that in the control (Table 3).
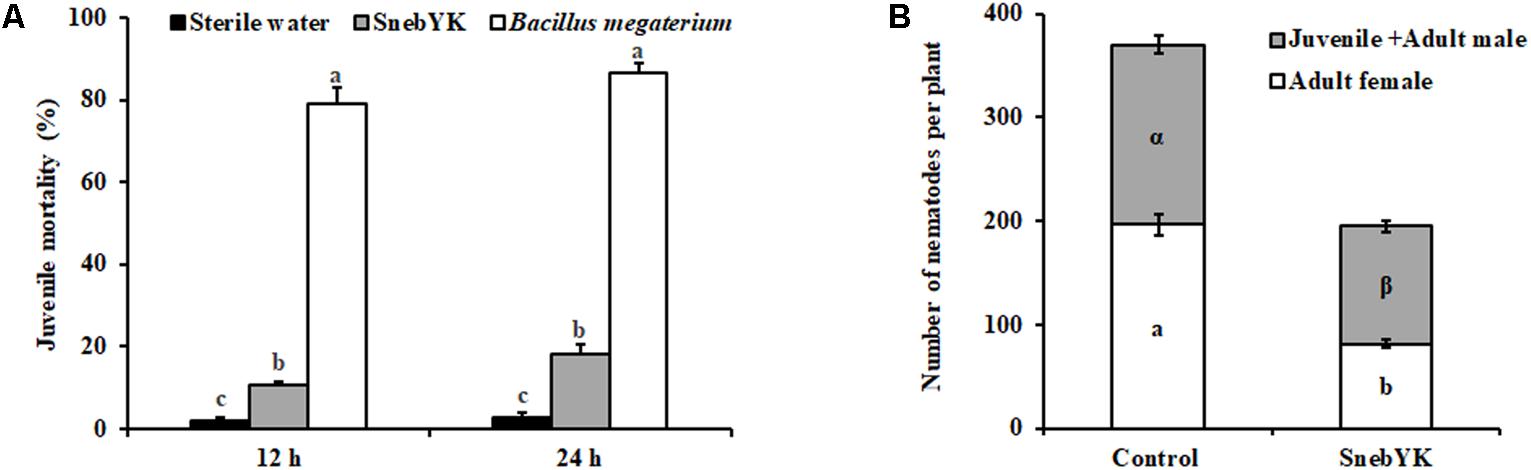
FIGURE 4. Effects of SnebYK on the control of Heterodera glycines in vitro and in the potting experiment. (A) In vitro juvenile mortality of H. glycines at 12 and 24 h after treatment with culture filtrates of SnebYK. Sterile water was used as a negative control; Bacillus megaterium was used as a positive control. The error bars represent standard deviations, and different letters (a, b) above the bars indicate significant differences at P < 0.05 according to Duncan’s multiple range test (n = 3). (B) Effect of SnebYK on the control of H. glycines in pots following seed coating. The numbers of H. glycines in the roots and soil were counted 30 days after inoculation. Different letters (a, b or α, β) indicate statistically significant differences (t-test with P < 0.05); a or b indicates a significant difference in the number of adult females, and α or β indicates a significant difference in the number of juveniles + adult males between treatments.
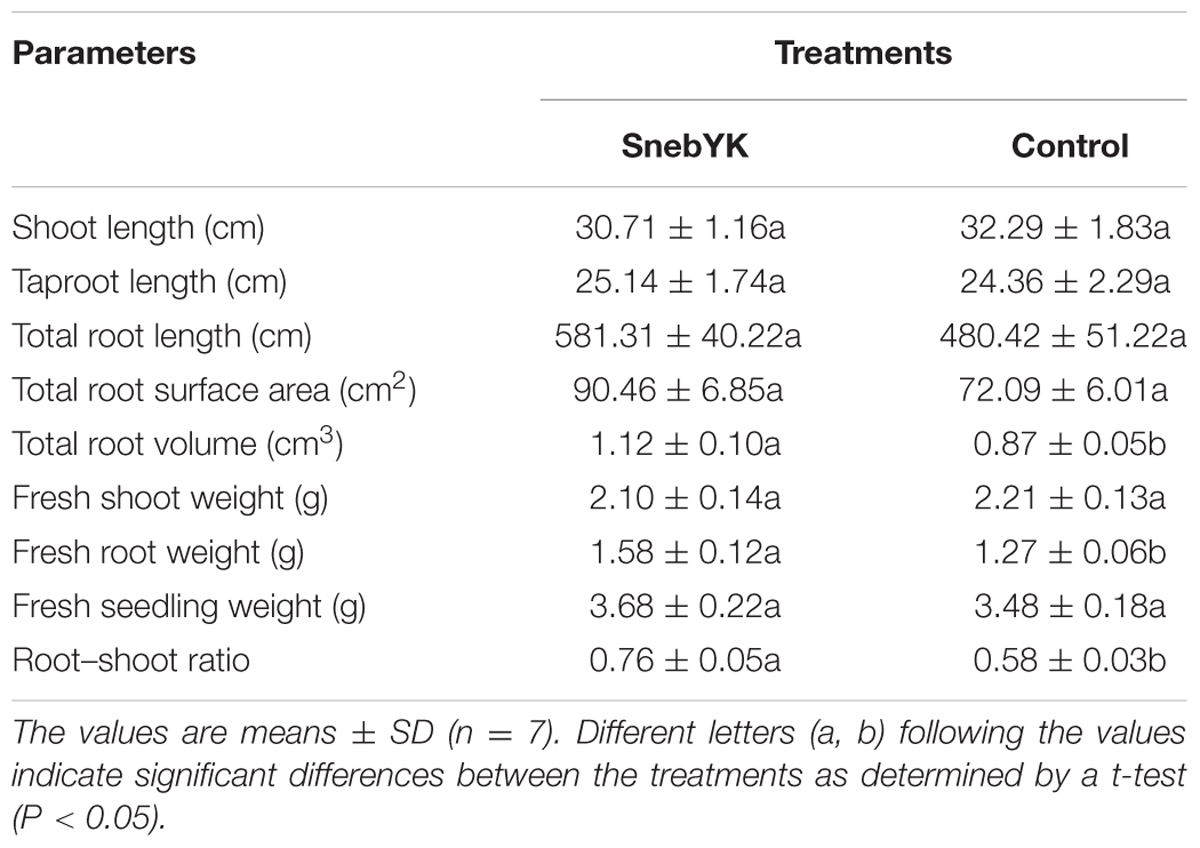
TABLE 3. Effects of SnebYK on the growth of soybean seedlings in the presence of Heterodera glycines.
To determine the effect of SnebYK on the control of H. glycines in the field, experiments were conducted at the experimental test site in Kangping. We observed that SnebYK effectively reduced the number of H. glycines in per root by 53.23% and 33.75% in 2016 and 2017, respectively (Figure 5A). The 2016 data showed that SnebYK did not reduce the number of adult females on the soybean roots but did reduce their abundance in the soil by 40.11%. The 2017 data showed that SnebYK greatly reduced the number of adult females both on the roots and in the soil (Figures 5B,C). Figures 5D–F summarize the effect of SnebYK on soybean growth under H. glycines infection in the field trials. The results indicated that, although SnebYK-treated soybeans had a higher fresh weight than that of the untreated soybeans, the difference was not significant.
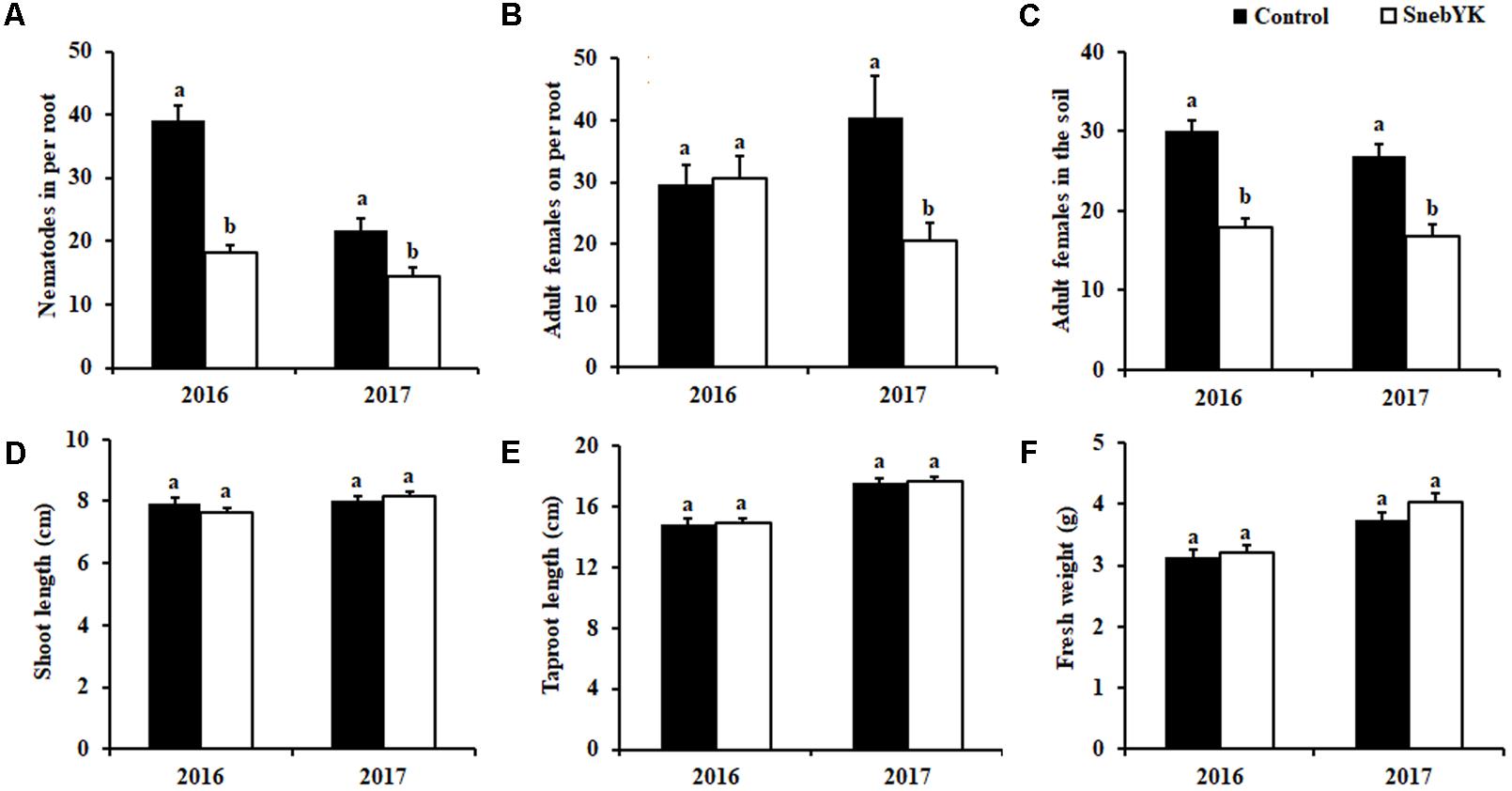
FIGURE 5. Effects of SnebYK on the control of Heterodera glycines and on soybean growth in the field following soybean seed coating. (A) Effect of SnebYK on the control of H. glycines in soybean roots. (B) Effect of SnebYK on the control of adult females of H. glycines on soybean roots. (C) Effect of SnebYK on the control of adult females of H. glycines in 100 ml rhizosphere soil. (D) Effect of SnebYK on the shoot length of soybean infested with H. glycines in the field. (E) Effect of SnebYK on the taproot length of soybean infested with H. glycines in the field. (F) Effect of SnebYK on the fresh weight of soybean infested with H. glycines in the field. Error bars represent standard deviations, and different letters (a, b) above the bars indicate significant differences at P < 0.05 according to a t-test (n = 60).
Effect of SnebYK on Inducing Systemic Resistance to H. glycines in Soybean
While SnebYK resulted in low H. glycines mortality in the in vitro assay, it exhibited the capacity to control H. glycines in both the potting and field experiments when used to coat soybean seeds. Therefore, we conducted experiments to verify whether SnebYK could elicit systemic resistance in soybean against H. glycines. The SRS is considered an effective tool for demonstrating induced resistance, and in this study, we confirmed the validity of the SRS before using it. Rhizosphere and endophytic bacteria from the SRS were cultured on ACCC55 agar plates. After 1 day, several bacterial colonies (with morphologies similar to that of SnebYK) were observed on only the agar plate that was inoculated with the bacteria from the inducer root treated with SnebYK. More bacterial colonies appeared after 2 days. When comparing the BOX-PCR fingerprints and 16S rRNA identifications of rhizosphere and endophytic bacteria from the SRS, we found K. pneumoniae in only the inducer roots treated with SnebYK (Figure 1B). Since the responder roots were not colonized by SnebYK, the SRS used in this experiment was valid. Next, we conducted a split root experiment to determine whether SnebYK elicited ISR to H. glycines in soybean. Penetration of the responder roots of the SRS, assessed 15 days after H. glycines inoculation, was significantly reduced when the inducer roots of the soybean were treated with SnebYK. This treatment caused a 61.78% reduction in the total nematode penetration when compared with that in the untreated control. Specifically, the numbers of J2, third-stage juveniles (J3), and fourth-stage juveniles (J4) were significantly lower in the responder roots than in the control group (Figure 6A). The proportion of H. glycines juveniles of each stage in the responder roots is summarized in Figure 6B. The proportions of J2, J3, and J4 in the control group were 60.59%, 34.62%, and 4.8%, respectively. Meanwhile, the proportions in the SnebYK-treated group were 73.27%, 25.1%, and 1.63%, respectively. Therefore, inoculation with SnebYK significantly increased the proportion of J2 by 12.68% and decreased the proportions of J3 and J4 by 9.52% and 3.17%, respectively.
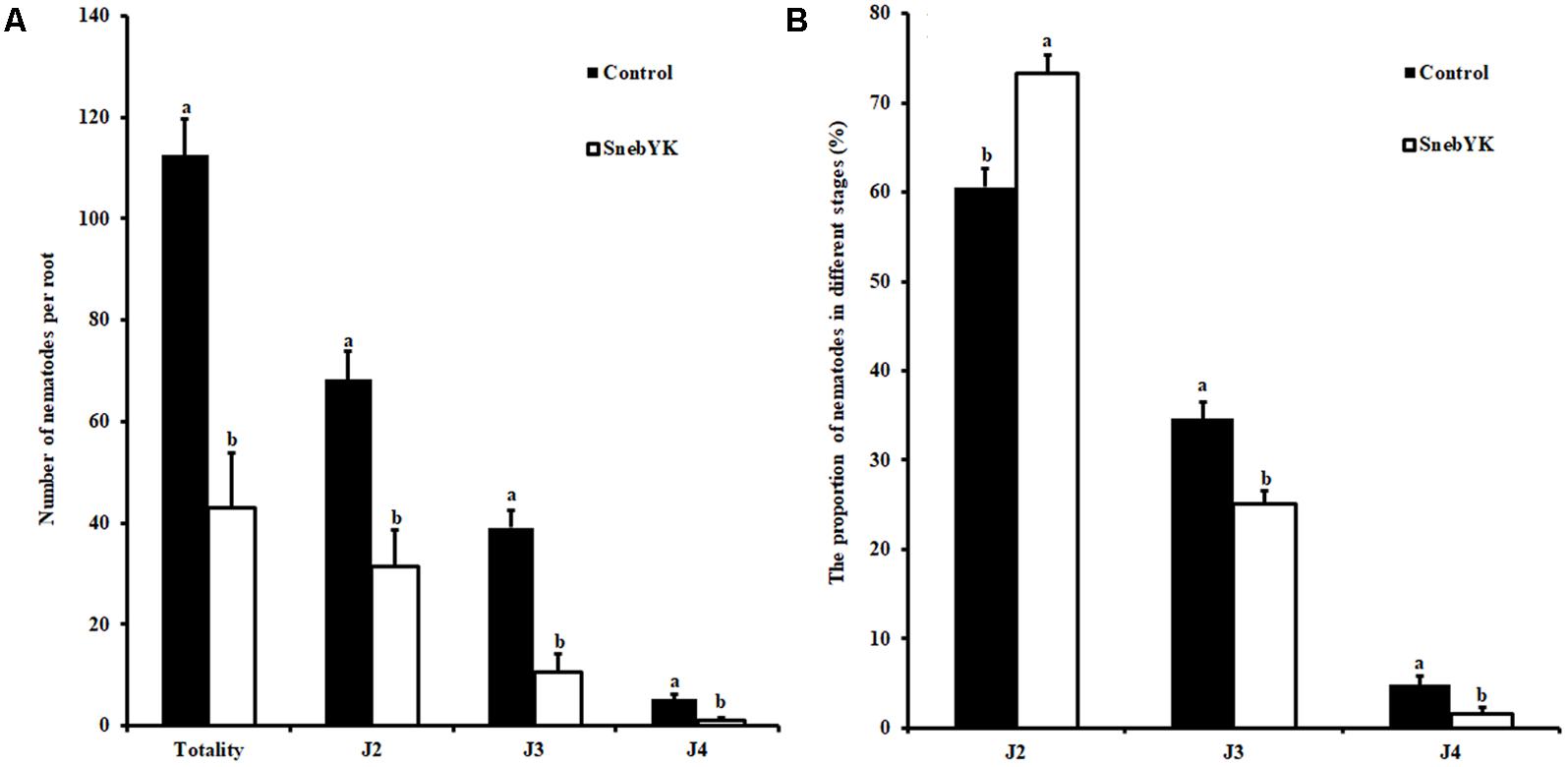
FIGURE 6. Effect of SnebYK on inducing systemic resistance to Heterodera glycines using a split root system in soybean. (A) Effect of SnebYK on the penetration and development of H. glycines in the responder root system. (B) Effect of SnebYK on the proportion of each developmental stage of H. glycines in the responder root system. Numbers of H. glycines in responder roots were counted 15 days after inoculation. J2, J3, and J4 indicate second-, third-, and fourth- stage juveniles of H. glycines, respectively. Error bars represent standard deviations, and different letters (a, b) above the bars indicate significant differences at P < 0.05 according to a t-test (n = 4).
To investigate whether SnebYK-mediated ISR was accompanied by primed defense responses to H. glycines in the roots, the expression levels of PR1, PR2, PR3, PR5, PR9, PR10, PDF1.2, NPR1-1, and NPR1-2 in soybeans treated or not treated with SnebYK were assessed using qRT-PCR. Transcript levels of the defense genes analyzed did not differ in the non-challenged soybeans regardless of the presence of SnebYK. After H. glycines inoculation, elevated PR5 expression was detected in control roots, indicating that soybean responds rapidly to H. glycines infection. Moreover, transcription of PR5 in soybeans pretreated with SnebYK was 5.04-fold and 3.23-fold higher at 24 and 48 h after inoculation, respectively, than those in control soybeans. Differences were also found in the expression levels of PR1, PR2, PR3, PR9, PR10, PDF1.2, and NPR1-2 between untreated soybeans and soybeans pretreated with SnebYK upon challenge with H. glycines. Twenty-four hours after H. glycines inoculation, these genes were induced in control soybeans, whereas the increase upon H. glycines challenge was lower in soybeans previously treated with SnebYK. However, 48 h after inoculation of H. glycines, the expression levels of PR1, PR2 and PDF1.2 were significantly higher in soybeans pretreated with SnebYK than in control soybeans; the transcript levels of PR9, PR10 and NPR1-2 did not differ significantly between the treatments; and the expression of PR3 was much lower in SnebYK-treated soybean roots than in untreated soybean roots (Figure 7). The transcript levels of NPR1-1 did not differ between soybeans pretreated with SnebYK and soybeans not treated with SnebYK following the inoculation of H. glycines.
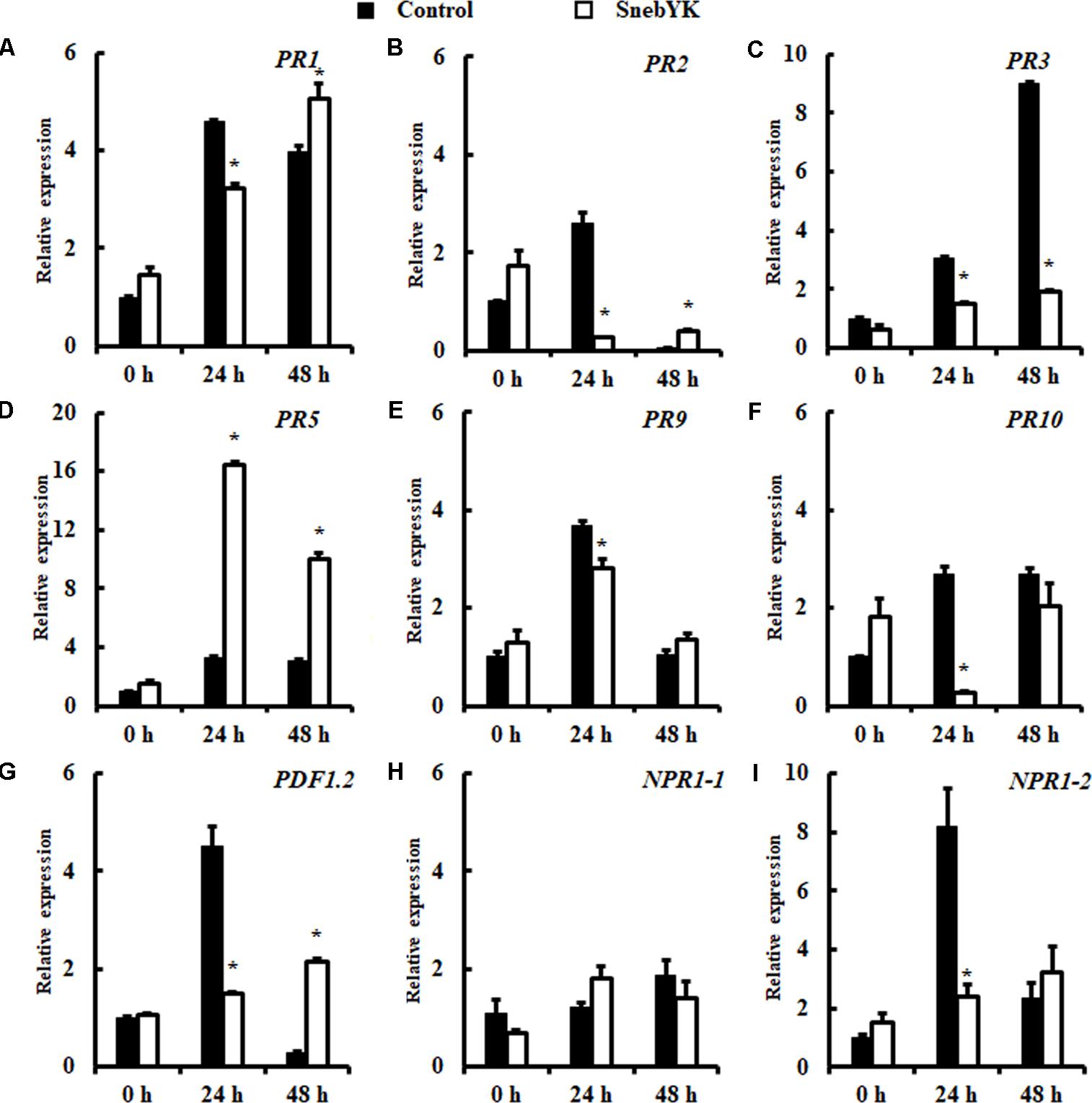
FIGURE 7. Induction of defense genes in soybean roots after Heterodera glycines inoculation. Soybean root samples were taken at 0, 24, and 48 h after H. glycines inoculation, and the expression levels of nine defense genes were analyzed by qRT-PCR: (A) PR1, (B) PR2, (C) PR3, (D) PR5, (E) PR9, (F) PR10, (G) PDF1.2, (H) NPR1-1, and (I) NPR1-2. Error bars represent standard deviations; a t-test was used to determine significant differences between the SnebYK-treated sample and the control (∗P < 0.05).
Discussion
Soybean is an important legume, and its production is greatly threatened by H. glycines. PGPB provide one of the most efficient control strategies in modern agriculture. In this study, the effect of SnebYK on the control of nematodes was evaluated. Data collected in potting experiment demonstrated that the population of H. glycines in SnebYK-treated soybean roots decreased by 47.32%; concomitantly, the number of adult females was reduced by 58.56% compared to that in the control. The number of female nematodes has an important effect on population size (Triantaphyllou, 1973) and was therefore a focus of this study. The results of the field trials also confirmed the ability of SnebYK to control H. glycines. By coating soybean seeds, SnebYK not only reduced the number of H. glycines in the soybean roots but also suppressed the number of adult females. Our data showed that SnebYK could effectively delay the development of juvenile nematodes into adult females, hindering mating and reproduction and thereby decreasing the abundance of H. glycines. These results are consistent with previous reports of PGPB and other beneficial microbes (Martinuz et al., 2013; Séry et al., 2016; De Medeiros et al., 2017; Kumari, 2017; Zhou et al., 2017). However, we found that SnebYK exhibited only a weak ability to kill H. glycines in vitro. Therefore, we speculated that SnebYK prevented H. glycines by inducing resistance.
Although some studies have demonstrated that K. pneumoniae can elicit ISR to pathogenic fungi and phytotoxicity in plants (Luo, 2010; Ji et al., 2014), these studies employed seed treatment. By establishing a split root experiment, we gained better insight into K. pneumoniae-elicited induced resistance. In the present study, we designed experiments to confirm that no colonization of SnebYK was present in the responder root system, to guarantee that bioprotection occurred when SnebYK and H. glycines were spatially separated. Indeed, SnebYK in the inducer roots reduced the H. glycines population in the responder roots, which clearly demonstrates that SnebYK-mediated bioprotection was at least partially systemically induced. The results of the split root experiment showed that SnebYK effectively triggered ISR in soybean against H. glycines and resulted in significantly reduced penetration. Similar results have been observed in R. etli (Martinuz et al., 2012), B. subtilis (Adam et al., 2014), and S. fredii (Tian et al., 2014) in defense against nematodes. In the split root experiment, the proportion of J2 H. glycines in SnebYK-treated soybean was significantly higher than that in the untreated control; correspondingly, the proportions of J3 and J4 decreased substantially. This result suggests that SnebYK plays a prominent role in delaying the development of H. glycines via ISR. Martinuz et al. (2013) reported similar results following R. etli G12 treatment, which reduced M. incognita penetration, delayed J2 development to J3, and reduced the numbers of females and eggs. Plants accumulate pathogenesis-related (PR) proteins in response to various pathogens, such as fungi, bacteria, viruses, insects, and nematodes (Kitajima and Sato, 1999; Van Loon et al., 2006). During H. schachtii infection of Arabidopsis, the expression of PR1, PR2, and PR5 was induced, and that of PR3 and PR4 was not altered in roots (Hamamouch et al., 2011). Non-expressor of pathogenesis related genes-1 (NPR1) is a transcriptional co-activator of PR gene expression (Dong, 2004), and transgenic tobacco plants constitutively expressing AtNPR1 exhibited resistance to M. incognita (Priya et al., 2011). In our study, SnebYK-mediated resistance to H. glycines was also accompanied by boosts in the expression of defense genes. Forty-eight hours after inoculation with H. glycines, the transcript levels of PR1, PR2, PR5, and PDF1.2 increased in SnebYK-pretreated soybeans compared with those in non-pretreated soybeans; however, the expression of PR3 decreased. Plant resistance to pathogens involves the plant hormones SA, JA, and ET. PR1, PR2, and PR5 are considered to be markers of SA-dependent signaling pathways (Delaney et al., 1994; Penninckx et al., 1996; Thomma et al., 1998), while PR3, PR4, and PDF1.2 are marker genes for JA/ET-dependent signaling pathways (Penninckx et al., 1998; Thomma et al., 1998). The PR genes with significant expression differences in the present study are all marker genes in the SA or JA/ET signaling pathways, including PR1, PR2, PR3, PR5, and PDF1.2. ISR often relies on pathways regulated by JA/ET (Van Oosten et al., 2008; Pieterse et al., 2009). However, dependence on both SA and JA/ET signaling pathways has also been reported (Niu et al., 2011; Salas-Marina et al., 2011). Our results implied that SnebYK might also activate SA and JA/ET signaling pathways to induce plant defense against H. glycines.
Treatment of soybean seeds with SnebYK significantly improved soybean seedling growth, especially that of the root. Several mechanisms may contribute to the increase in biomass. SnebYK carries the nitrogen-fixing gene nifH and expresses it under nitrogen-deficient conditions. SnebYK fixes atmospheric nitrogen, as shown by its ability to reduce acetylene. Non-symbiotic N2-fixing bacteria interact with plants to provide available nitrogen, increasing their vegetative growth and yield (Elkoca et al., 2010; Hayat et al., 2010). Klebsiella pneumoniae 342 is well known for its nitrogen-fixing capabilities. When K. pneumoniae 342 was applied to wheat, the dry weight of the plant increased; when the nifH gene of this strain was knocked out, the growth-promoting effect disappeared (Iniguez et al., 2004). Several experiments have examined the effects of K. pneumoniae, as a non-symbiotic N2-fixing bacterium, on the growth of monocotyledonous plants, such as rice (Ji et al., 2014; Banik et al., 2016), maize (Kuan et al., 2016), and wheat (Remus et al., 2000). However, few data are available on the effects of K. pneumoniae on the dicotyledonous plants growth. Our results demonstrated that SnebYK exhibited growth-promoting effects on the dicotyledonous soybean. Soybean roots treated with SnebYK were larger than those of the controls. PGPB affect root morphology, particularly by increasing root surface area (Larraburu et al., 2007; Wang et al., 2015) and weight (Islam et al., 2016; Singh et al., 2017), thereby improving nutrient uptake. This mechanism is even more important than nitrogen fixation in some PGPB (Vessey, 2003). SnebYK produces large amounts of ammonia, which may play an important role in the suppression of nematode populations (Rodriguez-Kabana, 1986; Khan et al., 2012). Phosphorus is also a key nutrient element for plants. SnebYK can convert tricalcium phosphate to a form that is accessible to the host. SnebYK also produces siderophores, which block the proliferation of pathogenic microorganisms by chelating Fe3+ and act as one of the major determinants of ISR (Hiifte et al., 1994; Ramamoorthy et al., 2001). The main signaling steps during the development of ISR require gene expression and consume resources (Buell, 1999; Heil, 2001), and SnebYK treatment activated the expression of PR genes under H. glycines infection. Allocation of limited plant resources to defense, means they cannot be used for plant growth (Herms and Mattson, 1992; Heil, 2001). Our data indicated that SnebYK fixes nitrogen and solubilizes inorganic phosphate, implying that SnebYK might provide nutrition to plants. This additional nutrition might be a reason that SnebYK-treated soybean exhibited a higher biomass and resistance to H. glycines at the same time. Further experiments are required to explore the regulatory mechanism in detail.
Conclusion
Our results support a role for the PGPB strain K. pneumoniae SnebYK in the induction of ISR against H. glycines in soybean. This study is the first report of K. pneumoniae eliciting ISR against H. glycines. SnebYK showed an ability to inhibit both the invasion and the development of nematodes. It induced the expression of defense genes in soybean following H. glycines infection and suppressed H. glycines in the field after coating soybean seeds, suggesting this may be an efficient and economical method to control H. glycines. SnebYK also presented remarkable plant growth-promoting properties, which promoted the growth of soybean. The overall results of the present study support K. pneumoniae SnebYK as a potential biocontrol agent for H. glycines.
Author Contributions
DL, LC, and YD conceived and designed the research. DL, LC, XZ, YW, and YX conducted all experiments and data analyses. The manuscript was written by DL and YD. XL and LJC provided the instruments and critically revised the manuscript. YD provided the final approval of the version to be published. All authors read and approved the final manuscript.
Funding
This research was supported by the National Natural Science Foundation of China (31330063), the Special Fund for Agro-Scientific Research in the Public Interest of China (201503114-12), and the Special Fund for the Modern Agricultural Industry Technology System of China (CARS-04-PS13).
Conflict of Interest Statement
The authors declare that the research was conducted in the absence of any commercial or financial relationships that could be construed as a potential conflict of interest.
Acknowledgments
We thank Dr. Liang Wenju for critical reading and suggestions to improve this manuscript.
References
Adam, M., Heuer, H., and Hallmann, J. (2014). Bacterial antagonists of fungal pathogens also control root-knot nematodes by induced systemic resistance of tomato plants. PLoS One 9:e90402. doi: 10.1371/journal.pone.009040
Alfianny, R., Aryantha, I. N. P., and Syamsudin, T. S. (2017). Role of indigenous rhizosphere bacteria in suppressing root-knot nematode and improve plant growth tomato. Plant Pathol. J. 16, 25–32. doi: 10.3923/ppj.2017.25.32
Anter, A. A., Amin, A. W., Ashoub, A. H., and El-Nuby, A. S. (2014). Evaluation of some rhizobacteria as induce systemic resistance or bio-control agents in controlling root-knot nematode, Meloidogyne incognita on tomato. J. Agronematol. 13, 107–123.
Ateba, C. N., and Mbewe, M. (2013). Determination of the genetic similarities of fingerprints from Escherichia coli O157: H7 isolated from different sources in the North West Province, South Africa using ISR, BOXAIR and REP-PCR analysis. Microbiol. Res. 168, 438–446. doi: 10.1016/j.micres.2013.02.003
Banik, A., Mukhopadhaya, S. K., and Dangar, T. K. (2016). Characterization of N2-fixing plant growth promoting endophytic and epiphytic bacterial community of Indian cultivated and wild rice (Oryza spp.) genotypes. Planta 243, 799–812. doi: 10.1007/s00425-015-2444-8
Boddey, R. M., and Knowles, R. (1987). Methods for quantification of nitrogen fixation associated with gramineae. Crit. Rev. Plant Sci. 6, 209–266. doi: 10.1080/07352688709382251
Buell, C. R. (1999). “Genes involved in plant-pathogen interactions,” in Induced Plant Defenses against Pathogens and Herbivores: Biochemistry, Ecology, and Agriculture, eds A. A. Agrawal, S. Tuzun, and E. Bent (St. Paul, MN: APS Press), 73–93.
Bybd, D. W., Kirkpatrick, T., and Barker, K. R. (1983). An improved technique for clearing and staining plant tissues for detection of nematodes. J. Nematol. 15, 142–143.
Cayrol, J. C., Djian, C., and Pijarowiski, L. (1989). Study on the nematicidal properties of the culture filtrate of the nematophagus fungus Paecilomyces lilacinus. Rev. Nematol. 12, 331–336.
Chen, L., Zhu, X. F., Wang, Y. Y., Chen, L. J., and Duan, Y. X. (2014). Proteomic analysis of the bacterial induction of resistance to atrazine in soybean leaves. Acta Physiol. Plant. 36, 2229–2241. doi: 10.1007/s11738-014-1601-1
De Medeiros, H. A., de Araújo Filho, J. V., De Freitas, L. G., Castillo, P., Rubio, M. B., Hermosa, R., et al. (2017). Tomato progeny inherit resistance to the nematode Meloidogyne javanica linked to plant growth induced by the biocontrol fungus Trichoderma atroviride. Sci. Rep. 7:40216. doi: 10.1038/srep40216
Delaney, T. P., Uknes, S., Vernooij, B., Friedrich, L., Weymann, K., Negrotto, D., et al. (1994). A central role of salicylic acid in plant disease resistance. Science 266, 1247–1250. doi: 10.1126/science.266.5188.1247
Dong, X. (2004). NPR1, all things considered. Curr. Opin. Plant Biol. 7, 547–552. doi: 10.1016/j.pbi.2004.07.005
Elkoca, E., Turan, M., and Donmez, M. F. (2010). Effects of single, dual and triple inoculations with Bacillus subtilis, Bacillus megaterium and Rhizobium leguminosarum bv. phaseoli on nodulation, nutrient uptake, yield and yield parameters of common bean (Phaseolus vulgaris L. cv. ‘ELKOCA-05’). J. Plant Nutr. 33, 2104–2119. doi: 10.1080/01904167.2010.519084
Gaby, J. C., and Buckley, D. H. (2012). A comprehensive evaluation of PCR primers to amplify the nifH gene of nitrogenase. PLoS One 7:e42149. doi: 10.1371/journal.pone.0042149
Gao, M., Zhou, J. J., Wang, E. T., Chen, Q., Xu, J., and Sun, J. G. (2015). Multiphasic characterization of a plant growth promoting bacterial strain, Burkholderia sp. 7016 and its effect on tomato growth in the field. J. Integr. Agric. 14, 1855–1863. doi: 10.1016/S2095-3119(14)60932-1
Hamamouch, N., Li, C., Seo, P. J., Park, C. M., and Davis, E. L. (2011). Expression of Arabidopsis pathogenesis-related genes during nematode infection. Mol. Plant Pathol. 12, 355–364. doi: 10.1111/j.1364-3703.2010.00675.x
Hao, X. L., Xie, P., Johnstone, L., Miller, S. J., Rensing, C., and Wei, G. H. (2012). Genome sequence and mutational analysis of plant-growth-promoting bacterium Agrobacterium tumefaciens CCNWGS0286 isolated from a zinc-lead mine tailing. Appl. Environ. Microbiol. 78, 5384–5394. doi: 10.1128/AEM.01200-12
Hayat, R., Ali, S., Amara, U., Khalid, R., and Ahmed, I. (2010). Soil beneficial bacteria and their role in plant growth promotion: a review. Ann. Microbiol. 60, 579–598. doi: 10.1007/s13213-010-0117-1
Heil, M. (2001). The ecological concept of costs of induced systemic resistance (ISR). Eur. J. Plant Pathol. 107, 137–146. doi: 10.1023/A:1008793009517
Herms, D. A., and Mattson, W. J. (1992). The dilemma of plants: to grow or defend. Q. Rev. Biol. 67, 283–335. doi: 10.1086/417659
Hiifte, M., Vande Woestyne, M., and Verstraete, W. (1994). “Role of siderophores in plant growth promotion and plant protection by fluorescent Pseudomonads,” in Biochemistry of Metal Micronutrients in the Rhizosphere, eds J. A. Manthey, D. E. Crowley, and D. G. Luster (Boca Raton, FL: CRC Press), 81–92.
Iniguez, A. L., Dong, Y. M., and Triplett, E. W. (2004). Nitrogen fixation in wheat provided by Klebsiella pneumoniae 342. Mol. Plant Microbe Interact. 17, 1078–1085. doi: 10.1094/MPMI.2004.17.10.1078
Islam, S., Akanda, A. M., Prova, A., Islam, M. T., and Hossain, M. M. (2016). Isolation and identification of plant growth promoting rhizobacteria from cucumber rhizosphere and their effect on plant growth promotion and disease suppression. Front. Microbiol. 6:1360. doi: 10.3389/fmicb.2015.01360
Ji, S. H., Gururani, M. A., and Chun, S. (2014). Isolation and characterization of plant growth promoting endophytic diazotrophic bacteria from Korean rice cultivars. Microbiol. Res. 169, 83–98. doi: 10.1016/j.micres.2013.06.003
Khan, Z., Tiyagi, S. A., Mahmood, I., and Rizvi, R. (2012). Effects of N fertilisation, organic matter, and biofertilisers on the growth and yield of chilli in relation to management of plant-parasitic nematodes. Turk J. Bot. 36, 73–81. doi: 10.3906/bot-1009-60
Kitajima, S., and Sato, F. (1999). Plant pathogenesis-related proteins: molecular mechanisms of gene expression and protein function. J. Biochem. 125, 1–8. doi: 10.1093/oxfordjournals.jbchem.a022244
Kloepper, J. W., Ryu, C. M., and Zhang, S. (2004). Induced systemic resistance and promotion of plant growth by Bacillus spp. Phytopathology 94, 1259–1266. doi: 10.1094/PHYTO.2004.94.11.1259
Koenning, S. R., and Wrather, J. A. (2010). Suppression of soybean yield potential in the continental United States by plant diseases from 2006 to 2009. Plant Health Prog. doi: 10.1094/PHP-2010-1122-01-RS
Kuan, K. B., Othman, R., Rahim, K. A., and Shamsuddin, Z. H. (2016). Plant growth-promoting rhizobacteria inoculation to enhance vegetative growth, nitrogen fixation and nitrogen remobilisation of maize under greenhouse conditions. PLoS One 11:e0152478. doi: 10.1371/journal.pone.0152478
Kuć, J. (2001). Concepts and direction of induced systemic resistance in plants and its application. Eur. J. Plant Pathol. 107, 7–12. doi: 10.1023/A:1008718824105
Kumari, N. S. (2017). Role of bacterial bioagent, Pasteuria penetrans in the management of root knot nematode, Meloidogyne incognita by altering the lifecycle. Pest Manage. Hortic. Ecosyst. 23, 76–80.
Larraburu, E. E., Carletti, S. M., Cáceres, E. A. R., and Llorente, B. E. (2007). Micropropagation of photinia employing rhizobacteria to promote root development. Plant Cell Rep. 26, 711–717. doi: 10.1007/s00299-006-0279-2
Lin, L., Li, Z. Y., Hu, C. J., Zhang, X. C., Chang, S. P., Yang, L. T., et al. (2012). Plant growth-promoting nitrogen-fixing enterobacteria are in association with sugarcane plants growing in Guangxi. China. Microbes Environ. 27, 391–398. doi: 10.1264/jsme2.ME11275
Liu, W. Z. (1995). The Research Technique of Plant Pathogenic Nematodes. Shenyang: Liaoning Science and Technology Press.
Liu, Y., Wang, H., Sun, X. L., Yang, H. L., Wang, Y. S., and Song, W. (2011). Study on mechanisms of colonization of nitrogen-fixing PGPB, Klebsiella pneumoniae NG14 on the root surface of rice and the formation of biofilm. Curr. Microbiol. 62, 1113–1122. doi: 10.1007/s00284-010-9835-7
Livak, K. J., and Schmittgen, T. D. (2001). Analysis of relative gene expression data using real-time quantitative PCR and the 2 -ΔΔCT method. Methods 25, 402–408. doi: 10.1006/meth.2001.1262
Luo, Z. Q. (2010). Induced Resistance to Atrazine by Bacterial Fermentation Fluid for Soybean. Master’s thesis, Shenyang Agricultural University, Shenyang.
Martinuz, A., Schouten, A., and Sikora, R. A. (2012). Systemically induced resistance and microbial competitive exclusion: implications on biological control. Phytopathology 102, 260–266. doi: 10.1094/PHYTO-04-11-0120
Martinuz, A., Schouten, A., and Sikora, R. A. (2013). Post-infection development of Meloidogyne incognita on tomato treated with the endophytes Fusarium oxysporum strain Fo162 and Rhizobium etli strain G12. Biocontrol 58, 95–104. doi: 10.1007/s10526-012-9471-1
Murphy, J., and Riley, J. P. (1962). A modified single solution method for the determination of phosphate in natural waters. Anal. Chim. Acta 27, 31–36. doi: 10.1016/S0003-2670(00)88444-5
Niu, D. D., Liu, H. X., Jiang, C. H., Wang, Y. P., Wang, Q. Y., Jin, H. L., et al. (2011). The plant growth–promoting rhizobacterium Bacillus cereus AR156 induces systemic resistance in Arabidopsis thaliana by simultaneously activating salicylate-and jasmonate/ethylene-dependent signaling pathways. Mol. Plant Microbe Interact. 24, 533–542. doi: 10.1094/MPMI-09-10-0213
Pathak, R., Gehlot, P., and Singh, S. K. (2016). “Seed priming-mediated induced disease resistance in arid zone plant,” in Microbial-Mediated Induced Systemic Resistance in Plants, eds D. K. Choudhary and A. Varma (New York, NY: Springer), 57–67. doi: 10.1007/978-981-10-0388-2_5
Penninckx, I. A., Eggermont, K., Terras, F. R., Thomma, B. P., De Samblanx, G. W., Buchala, A., et al. (1996). Pathogen-induced systemic activation of a plant defensin gene in Arabidopsis follows a salicylic acid-independent pathway. Plant Cell 8, 2309–2323. doi: 10.1105/tpc.8.12.2309
Penninckx, I. A., Thomma, B. P., Buchala, A., Métraux, J. P., and Broekaert, W. F. (1998). Concomitant activation of jasmonate and ethylene response pathways is required for induction of a plant defensin gene in Arabidopsis. Plant Cell 10, 2103–2113. doi: 10.1105/tpc.10.12.2103
Pieterse, C. M. J., Leon-Reyes, A., Van der Ent, S., and Van Wees, S. C. (2009). Networking by small-molecule hormones in plant immunity. Nat. Chem. Biol. 5, 308–316. doi: 10.1038/nchembio.164
Pieterse, C. M. J., Zamioudis, C., Berendsen, R. L., Weller, D. M., Van Wees, S. C., and Bakker, P. A. (2014). Induced systemic resistance by beneficial microbes. Annu. Rev. Phytopathol. 52, 347–375. doi: 10.1146/annurev-phyto-082712-102340
Pinto-Tomás, A. A., Anderson, M. A., Suen, G., Stevenson, D. M., and Chu, F. S. (2009). Symbiotic nitrogen fixation in the fungus gardens of leaf-cutter ants. Science 326, 1120–1123. doi: 10.1126/science.1173036
Poly, F., Monrozier, L. J., and Bally, R. (2001). Improvement in the RFLP procedure for studying the diversity of nifH genes in communities of nitrogen fixers in soil. Res. Microbiol. 152, 95–103. doi: 10.1016/S0923-2508(00)01172-4
Priya, D. B., Somasekhar, N., Prasad, J. S., and Kirti, P. B. (2011). Transgenic tobacco plants constitutively expressing Arabidopsis NPR1 show enhanced resistance to root-knot nematode, Meloidogyne incognita. BMC Res. Notes 4:231. doi: 10.1186/1756-0500-4-231
Ramamoorthy, V., Viswanathan, R., Raguchander, T., Prakasam, V., and Samiyappan, R. (2001). Induction of systemic resistance by plant growth promoting rhizobacteria in crop plants against pests and diseases. Crop Prot. 20, 1–11. doi: 10.1016/S0261-2194(00)00056-9
Reitz, M., Rudolph, K., Schroder, I., Hoffmann-Hergartem, S., Hallmann, J., and Sikora, R. A. (2000). Lipopolysaccharides of Rhizobium etli strain G12 act in potato roots as an inducing agent of systemic resistance to infection by the cyst nematode Globodera pallida. Appl. Environ. Microb. 66, 3515–3518. doi: 10.1128/AEM.66.8.3515-3518.2000
Remus, R., Ruppel, S., Jacob, H. J., Hecht-Buchholz, C., and Merbach, W. (2000). Colonization behavior of two enterobacterial strains on cereals. Biol. Fert. Soils 30, 550–557. doi: 10.1007/s003740050035
Rincker, K., Cary, T., and Diers, B. W. (2017). Impact of soybean cyst nematode resistance on soybean yield. Crop Sci. 57, 1–10. doi: 10.2135/cropsci2016.07.0628
Rodriguez-Kabana, R. (1986). Organic and inorganic nitrogen amendments to soil as nematode suppressants. J. Nematol. 18, 129–135.
Sachdev, D. P., Chaudhari, H. G., Kasture, V. M., Dhavale, D. D., and Chopade, B. A. (2009). Isolation and characterization of indole acetic acid (IAA) producing Klebsiella pneumoniae strains from rhizosphere of wheat (Triticum aestivum) and their effect on plant growth. Indian J. Exp. Biol. 47, 993–1000.
Salas-Marina, M. A., Silva-Flores, M. A., Uresti-Rivera, E. E., Castro-Longoria, E., Herrera-Estrella, A., and Casas-Flores, S. (2011). Colonization of Arabidopsis roots by Trichoderma atroviride promotes growth and enhances systemic disease resistance through jasmonic acid/ethylene and salicylic acid pathways. Eur. J. Plant Pathol. 131, 15–26. doi: 10.1007/s10658-011-9782-6
Sarma, R. K., and Saikia, R. (2014). Alleviation of drought stress in mung bean by strain Pseudomonas aeruginosa GGRJ21. Plant Soil 377, 111–126. doi: 10.1007/s11104-013-1981-9
Schwyn, B., and Neilands, J. B. (1987). Universal chemical assay for the detection and determination of siderophores. Anal. Biochem. 160, 47–56. doi: 10.1016/0003-2697(87)90612-9
Séry, D. J. M., Kouadjo, Z. C., Voko, B. R., and Zézé, A. (2016). Selecting native arbuscular mycorrhizal fungi to promote Cassava growth and increase yield under field conditions. Front. Microbiol. 7:2063. doi: 10.3389/fmicb.2016.02063
Singh, R. P., Jha, P., and Jha, P. N. (2015). The plant-growth-promoting bacterium Klebsiella sp. SBP-8 confers induced systemic tolerance in wheat (Triticum aestivum) under salt stress. J. Plant Physiol. 184, 57–67. doi: 10.1016/j.jplph.2015.07.002
Singh, R. P., Jha, P., and Jha, P. N. (2017). Bio-inoculation of plant growth-promoting rhizobacterium Enterobacter cloacae ZNP-3 increased resistance against salt and temperature stresses in wheat plant (Triticum aestivum L.). J. Plant Growth Regul. 36, 783–798. doi: 10.1007/s00344-017-9683-9
Subbotin, S. A., Mundo-Ocampo, M., and Baldwin, J. G. (2010). Systematics of Cyst Nematodes (Nematoda:Heteroderinae), Part B. Leiden: Brill.
Thomma, B. P., Eggermont, K., Penninckx, I. A., Mauch-Mani, B., Vogelsang, R., Cammue, B. P., et al. (1998). Separate jasmonate-dependent and salicylate-dependent defense-response pathways in Arabidopsis are essential for resistance to distinct microbial pathogens. Proc. Natl. Acad. Sci. U.S.A. 95, 15107–15111. doi: 10.1073/pnas.95.25.15107
Tian, F., Wang, Y. Y., Zhu, X. F., Chen, L. J., and Duan, Y. X. (2014). Effect of Sinorhizobium fredii strain Sneb183 on the biological control of soybean cyst nematode in soybean. J. Basic Microb. 54, 1258–1263. doi: 10.1002/jobm.201301014
Triantaphyllou, A. C. (1973). Environmental sex differentiation of nematodes in relation to pest management. Annu. Rev. Phytopathol. 11, 441–462. doi: 10.1146/annurev.py.11.090173.002301
Van Loon, L. C., Bakker, P. A. H. M., and Pieterse, C. M. J. (1998). Systemic resistance induced by rhizosphere bacteria. Annu. Rev. Phytopathol. 36, 453–483. doi: 10.1146/annurev.phyto.36.1.453
Van Loon, L. C., Rep, M., and Pieterse, C. M. J. (2006). Significance of inducible defense-related proteins in infected plants. Annu. Rev. Phytopathol. 44, 135–162. doi: 10.1146/annurev.phyto.44.070505.143425
Van Oosten, V. R., Bodenhausen, N., Reymond, P., Van Pelt, J. A., Van Loon, L. C., Dicke, M., et al. (2008). Differential effectiveness of microbially induced resistance against herbivorous insects in Arabidopsis. Mol. Plant Microbe Interact. 21, 919–930. doi: 10.1094/MPMI-21-7-0919
Versalovic, J., Schneider, M., De Bruijn, F. J., and Lupski, J. R. (1994). Genomic fingerprinting of bacteria using repetitive sequence-based polymerase chain reaction. Methods Mol. Cell. Biol. 5, 25–40.
Vessey, J. K. (2003). Plant growth promoting rhizobacteria as biofertilizers. Plant Soil 255, 571–586. doi: 10.1023/a:1026037216893
Vryzas, Z. (2016). The plant as metaorganism and research on next-generation systemic pesticides – prospects and challenges. Front. Microbiol. 7:1968. doi: 10.3389/fmicb.2016.01968
Wang, B., Mei, C., and Seiler, J. R. (2015). Early growth promotion and leaf level physiology changes in Burkholderia phytofirmans strain PsJN inoculated switchgrass. Plant Physiol. Biochem. 86, 16–23. doi: 10.1016/j.plaphy.2014.11.008
Wei, G., Kloepper, J. W., and Tuzun, S. (1996). Induced systemic resistance to cucumber diseases and increased plant growth by plant growth-promoting rhizobacteria under field conditions. Phytopathology 86, 221–224. doi: 10.1094/Phyto-86-221
Xiang, P., Chen, L. J., Zhu, X. F., Wang, Y. Y., and Duan, Y. X. (2013). Screening and identification of bacterium to induce resistance of soybean against Heterodera glycines. Chin. J. Biol. Control 29, 661–666.
Keywords: induced systemic resistance, plant growth-promoting bacteria, Klebsiella pneumoniae, Heterodera glycines, soybean
Citation: Liu D, Chen L, Zhu X, Wang Y, Xuan Y, Liu X, Chen L and Duan Y (2018) Klebsiella pneumoniae SnebYK Mediates Resistance Against Heterodera glycines and Promotes Soybean Growth. Front. Microbiol. 9:1134. doi: 10.3389/fmicb.2018.01134
Received: 13 August 2017; Accepted: 14 May 2018;
Published: 01 June 2018.
Edited by:
Corné M. J. Pieterse, Utrecht University, NetherlandsReviewed by:
Govindan Rajamohan, Institute of Microbial Technology (CSIR), IndiaAinhoa Martinez Medina, German Center for Integrative Biodiversity Research, Germany
Copyright © 2018 Liu, Chen, Zhu, Wang, Xuan, Liu, Chen and Duan. This is an open-access article distributed under the terms of the Creative Commons Attribution License (CC BY). The use, distribution or reproduction in other forums is permitted, provided the original author(s) and the copyright owner are credited and that the original publication in this journal is cited, in accordance with accepted academic practice. No use, distribution or reproduction is permitted which does not comply with these terms.
*Correspondence: Yuxi Duan, ZHVhbnl4NjQwN0AxNjMuY29t