- 1Department of Respiratory Medicine, The First Hospital of Jilin University, Changchun, China
- 2Key Laboratory of Zoonosis, Ministry of Education, Institute of Zoonosis, College of Veterinary Medicine, Jilin University, Changchun, China
- 3Beijing Key Laboratory of Detection Technology for Animal-Derived Food Safety, College of Veterinary Medicine, China Agricultural University, Beijing, China
Carbapenem resistance among strains of the nosocomial pathogen Klebsiella pneumoniae is increasing worldwide, causing serious clinical infections and higher mortality rates. Polymyxins are some of the few “last resort” options for treatment of carbapenem-resistant Enterobacteriaceae, including K. pneumoniae, however, the emergence of plasmid-mediated colistin resistance gene mcr-1 has largely rendered polymyxin-class antibiotics ineffective in a clinical setting. We previously identified a natural compound, pterostilbene, which has a synergistic effect in combination with polymyxins. Here, we aimed to determine whether pterostilbene application can restore the bactericidal activity of polymyxins against mcr-1-positive K. pneumoniae. Checkerboard MIC studies confirmed that pterostilbene reduces the MIC of colistin against mcr-1-positive clinical K. pneumoniae isolates, with the bacteria going from resistant to sensitive, and also demonstrated a synergistic effect with colistin (FIC index = 0.11 ± 0.04 or 0.28 ± 0.00). Time-killing assays showed that individually, both pterostilbene and colistin failed to eradicate K. pneumoniae strains, while in combination, the two drugs effectively eliminated K. pneumoniae ZJ02 and K. pneumoniae ZJ05 by 1–3 h post-inoculation. The combined disk test also showed increases in the zones of inhibition only for mcr-1-positive Escherichia coli and K. pneumoniae isolates. A mouse infection model demonstrated that the survival rate of mice at 7 days post-intraperitoneal injection with a lethal dose of K. pneumoniae ZJ05 was significantly promoted from 0 to 67% following combination therapy. This is the first time a MCR-1 inhibitor has successfully been used in combination with colistin against human clinical MCR-1 producing K. pneumoniae ZJ05 isolate.
Introduction
The relentless increase in carbapenem-resistant Enterobacteriaceae (CRE) strains is now recognized as one of the most serious global threats to public health (Morrill et al., 2015). Carbapenem-resistant K. pneumoniae strains are especially worrying as they have higher morbidity and mortality rates, and treatment of these bacterial infections is frequently challenging because of the limited therapeutic options (Olaitan et al., 2014; Quan et al., 2017). K. pneumoniae is a common cause of pulmunary and bloodstream health care related infections and normally resides in the lower gastrointestinal tract, where it can acquire high-level antibiotic resistance (Hrabák et al., 2011). This eventuality forced a re-evaluation of the use of one of the earliest classes of antibiotics, polymyxins, for treatment of serious infections caused by carbapenem and multidrug resistant K. pneumoniae isolates often blaKPC or blaNDM- positive (Quan et al., 2017). In human clinical chemotherapy, polymyxin B and polymyxin E are usually used in combination, mainly because the dose escalation that is required to achieve sufficiently high concentrations under the currently recommended dosing protocols, risks the rapid onset of nephrotoxicity and neuromuscular blockade (Pogue et al., 2011).
Prior to the detection of the plasmid-mediated colistin resistance gene mcr-1, almost all studies of polymyxin resistance focused on the pmrAB and phoPQ two-component regulatory systems, inactivation of mgrB, or the lack of lipopolysaccharide (Halaby et al., 2013). mcr-1 encodes a phosphoethanolamine transferase that alters the charge on lipid A from electronegative to electropositive, thereby inhibiting the binding of polymyxins to target bacteria. mcr-1 determinant amongst CRE has almost eliminated their clinical susceptibility to polymyxin (Liu et al., 2016, 2017; Kieffer et al., 2017). Importantly, as mcr-1 is plasmid-mediated, resistance to polymyxins is no longer only associated with the chromosome, but can also be acquired by horizontal transmission (Giamarellou, 2016).
The loss of these last-line-of-defense antibiotics made necessary of the development of novel and effective strategies to deal with the serious challenges posed by MCR-1 expression, with the investment of large amounts of manpower and resources. It would also be useful to restore the efficacy of polymyxin to treat severe clinical bacterial infections caused by CRE (Bulman et al., 2017). Previously, we showed that a natural compound used in traditional Chinese medicine, pterostilbene, which has been extensively studied for its potent anti-cancer, anti-inflammatory, and anti-oxidant activities (Roupe et al., 2006), has a synergistic effect with polymyxin B against E. coli both in vitro and in vivo (Zhou et al., 2018). Because of its methoxyl substitution-induced hyperlipophilicity, pterostilbene may have higher bioactivity than resveratrol, making it potentially advantageous as a therapeutic agent (Cichocki et al., 2008; Kapetanovic et al., 2011). Here, we further characterized the efficacy of pterostilbene administrated together with polymyxins, and showed that it can help restore the bactericidal activity of polymyxins against mcr-1-positive K. pneumoniae.
Materials and Methods
Bacterial Strains and Chemicals
Human clinical MCR-1 producing isolates K. pneumoniae ZJ02, K. pneumoniae ZJ05 and E. coli ZJ40 were collected in our previous study (Wang et al., 2017). And the mcr-1 gene was chromosomally located in E. coli ZJ40. K. pneumoniae E8.31, K.pneumoniae 13b5 and K.pneumoniae L18 were collected from food animals. We also used E. coli strain DH5α (pUC19-mcr-1) (Zhou et al., 2018), which carries a mcr-1 gene originating from K. pneumoniae ZJ05. Polymyxin-resistant mcr-1-negative K. pneumoniae isolate 16ZJJ9-19BC was obtained from a chicken cloacae sample collected in Zhejiang, China. E. coli ATCC25922, K. pneumoniae ATCC700603 and K. pneumoniae K7 were used as quality control strains. Pterostilbene (≥97% HPLC-pure) was purchased from Sigma-Aldrich (St. Louis, MO, USA). Colistin sulfate, polymyxin B sulfate, penicillin, imipenem, gentamicin sulfate, and chloramphenicol were purchased from the National Institute for the Control of Pharmaceutical and Biological Products (Beijing, China). Cephalothin sodium, streptomycin sulfate, kanamycin sulfate, erythromycin, and acheomycin were purchased from Dalian Meilun Biotechnology Co. (Dalian, China). Stock solutions of pterostilbene were prepared in dimethyl sulfoxide (Sigma-Aldrich).
MIC Determination and Growth Curves
The MIC assays were used to identify synergies between pterostilbene and colistin against polymyxin-resistant strains (positive for mcr-1), polymyxin-resistant strains (negative for mcr-1), and polymyxin-sensitive strains (negative for mcr-1), and were carried out using the broth microdilution method following the guidelines of the Clinical and Laboratory Standards Institute (Wiegand et al., 2008; Espinel-Ingroff et al., 2016). The remaining nine antibiotics were also tested in combination with pterostilbene. The efficacies of the combinations were evaluated by calculating the fractional inhibitory concentration (FIC) index values (Ma et al., 2016). A growth curve assay was also performed to evaluate the effect of pterostilbene on the growth of the tested strains (Li et al., 2011). Briefly, K. pneumoniae ZJ02 and K. pneumoniae ZJ05 were cultured in Luria-Bertani (LB) medium at 37°C with shaking at 200 rpm to obtain an OD600 value of 0.3. Aliquots (250 mL) of the culture were then transferred into six 50-mL Erlenmeyer flasks, and pterostilbene (or the dimethyl sulfoxide control) was added to the cultures at 0, 16, 32, 64, and 128 μg/mL, respectively. The bacteria were cultured at 37°C with shaking, and bacterial growth was estimated by measuring the OD600 every 30 min.
Time-Killing Assays
The potential bactericidal effect of pterostilbene in combination with colistin was evaluated by time-killing assays (Petersen et al., 2006). Mid-logarithmic-phase bacterial cells were diluted to 5 × 105 CFU/mL in LB broth supplemented with colistin (4 μg/mL), pterostilbene (16 μg/mL), colistin (4 μg/mL) in combination with pterostilbene (16 μg/mL), or DMSO (normal control). Cultures were incubated at 37°C with shaking and samples were removed at 0, 1, 3, 5, and 7 h post-inoculation for bacterial counts. Serial 10-fold dilutions of the samples were spread onto LB agar plates without antibiotics. Bacterial colonies were counted following incubation at 37°C for 24 h.
Combined Disk Test
The combined disk test (CDT) was carried out as described previously (Pournaras et al., 2013; Watts, 2013). Based on the results of the growth curve assay and checkerboard MIC studies, we selected pterostilbene concentrations of 0, 8, and 32 μg/mL, none of which resulted in an inhibitory effect against any of the screened strains. Colistin 10 μg disks (Oxoid Ltd., Basingstoke, United Kingdom) were first placed on Mueller-Hinton-Broth (MHB) agar plates inoculated with bacterial suspension at an OD600 = 0.1. Ten-microliter aliquots of the different concentrations of pterostilbene solution were then directly added to the disks. The diameters of the inhibition zones around the colistin disks (with and without pterostilbene) were measured and compared following incubation for 18–24 h at 37°C.
In Vivo Infection Model for K. pneumoniae ZJ05
A mouse model of endonasal pulmonary infection was used to determine the synergistic effect of pterostilbene in combination with colistin in vivo. Eight-week-old female C57BL/6J mice weighing 20 ± 2 g were obtained from the Experimental Animal Centre of Jilin University (Changchun, China). Animal experiments were approved by and conducted in accordance with the guidelines of the Animal Care and Use Committee of Jilin University. Five mice were housed per cage in a pathogen-free environment maintained at 24 ± 2°C and 50% ± 10% relative humidity and subjected to a 12 h light/12 h dark cycle. All mice were rested for 5 days prior to use to allow acclimatization.
Pneumonia was induced in the mice as described previously (Bowers et al., 2015; Zhou et al., 2017). K. pneumoniae ZJ05 was grown to mid-logarithmic phase (OD600 = 0.5) in LB medium at 37°C and then centrifuged at 5,000 × g for 5 min at 4°C. After washing three times with PBS, the bacteria were resuspended in PBS. The mice were divided randomly into five groups (solvent control for each treatment, pterostilbene alone, colistin alone, and pterostilbene in combination with colistin). Each experimental group contained 18 mice. For the survival experiments, the mice were lightly anesthetized by inhalation of isoflurane and then inoculated in the left nare with 20 μL of suspension containing 1 × 108 CFU of the prepared K. pneumoniae ZJ05 cells. The infected mice were subcutaneously administered colistin (8 mg/kg), pterostilbene (50 mg/kg), a combination of pterostilbene (50 mg/kg) and colistin (8 mg/kg), or solvent on the same schedule at 2 h post-infection and then at 8-h intervals. Mice were monitored until day 7 post-infection.
For histopathological analysis of lung infection and calculation of the wet/dry weight ratio, mice were inoculated with 5 × 107 CFU of prepared K. pneumoniae ZJ05 cells. The mice were killed with anesthesia followed by cervical dislocation at 48 h post-infection. Homogenates of lung tissue, which was collected from euthanized mice, were prepared in 1 ml of sterile PBS and used to calculate bacterial colony counts following serial dilution and smearing on LB agar plates. For histopathological analysis, the lungs were placed in 10% (v/v) formalin, followed by staining with hematoxylin and eosin and examination by light microscopy. The lungs were isolated to measure the wet weight, while the dry weight was measured after drying for 72 h at 70°C. The wet/dry weight ratio of the lung was then calculated.
Statistical Analysis
The IBM Statistical Program for Social Sciences (SPSS) version 19.0 (IBM Corp. Armonk, NY, USA) was used to analyze experimental data, and data are presented as the mean ± standard deviation. An independent Student's t-test was used to determine significant differences, and differences were considered statistically significant when P-values were less than 0.05.
Results
Pterostilbene Showed a Synergistic Effect in Combination With Polymyxin Against mcr-1- Positive Bacteria
We previously showed that pterostilbene (trans-3,5-dimethoxy-4′-hydroxystilbene) had a synergistic effect with polymyxin B and colistin against polymyxin-resistant E. coli strains (positive for MCR-1) (Zhou et al., 2018). In view of the clinical significance of K. pneumoniae, and to determine the synergistic effect of pterostilbene in combination with polymyxin alone, mcr-1-positive K. pneumoniae isolates ZJ02, ZJ05, E8.31, 13B5, and L18 were examined in this study. Our results confirmed the synergistic effect of pterostilbene only in combination with colistin against both mcr-1-positive clinical K. pneumoniae isolates (FIC = 0.11 ± 0.04–0.28 ± 0.00, respectively, in the presence of 16 μg/mL of pterostilbene) using the broth microdilution checkerboard method. No synergy was observed with any of the other nine tested antibiotics against either the mcr-1-positive or polymyxin-sensitive isolates. However, the synergistic effect of pterostilbene and polymyxin against mcr-1-negative polymyxin-resistant K. pneumoniae strain 16ZJJ9-19BC differed from that observed using mcr-1-positive isolates (Table 1). The growth curve showed that none of the concentrations of pterostilbene (0–128 μg/mL) affected the growth of mcr-1-positive K. pneumoniae isolates ZJ02 and ZJ05 (Figures 1A,B).
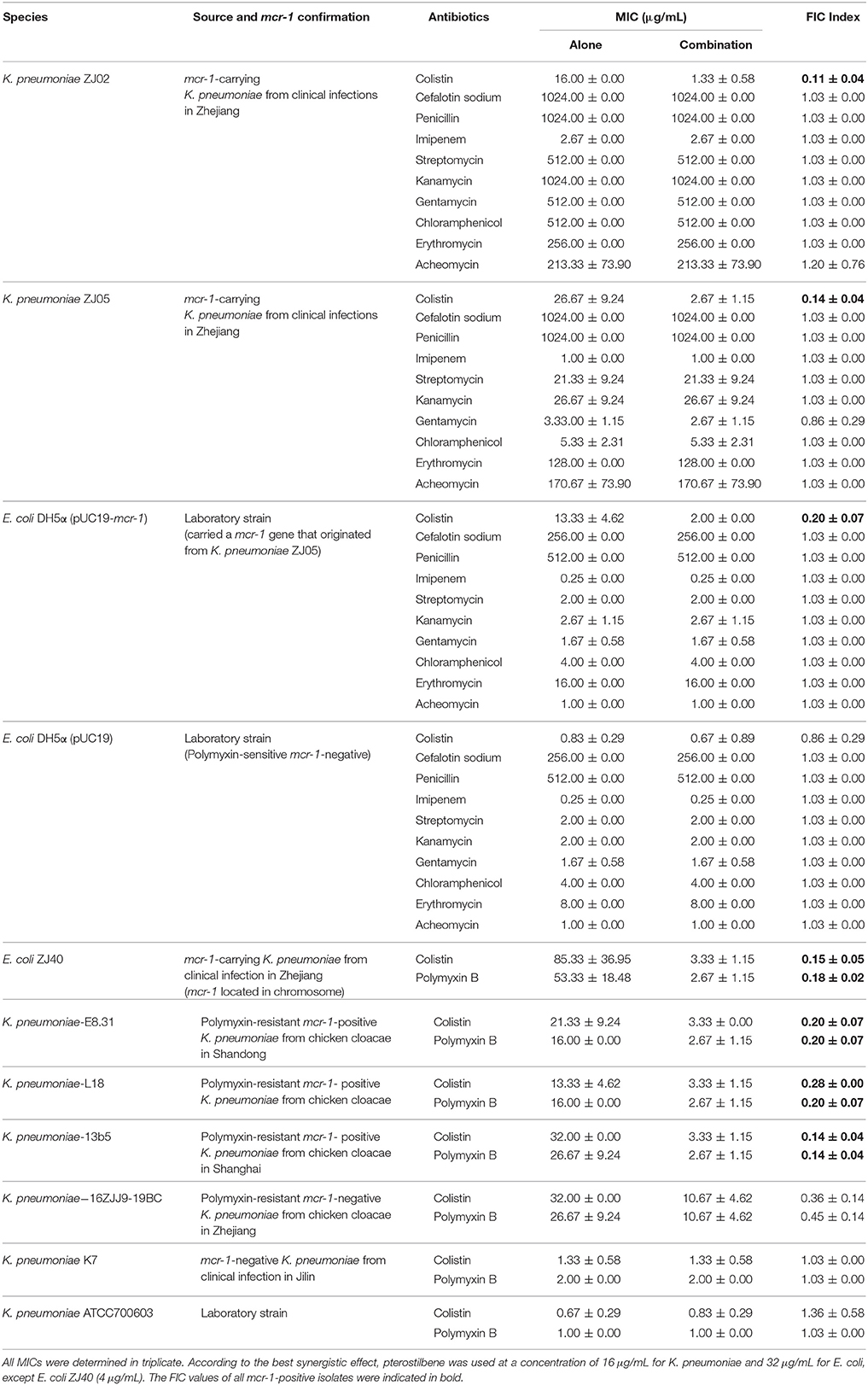
Table 1. MIC values for the different antibiotics used alone or in combination with pterostilbene against each of the tested bacterial isolates.
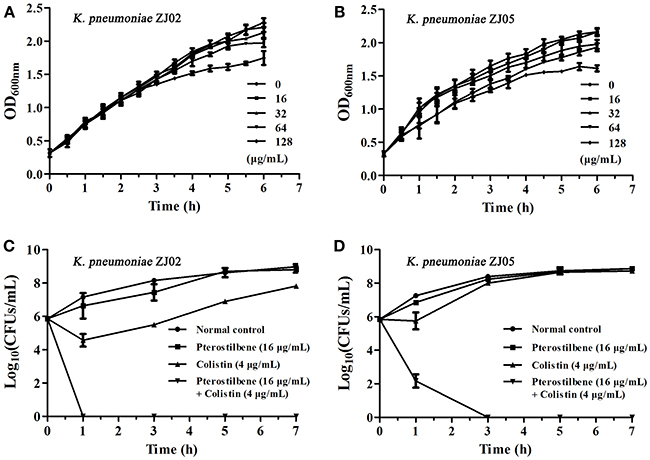
Figure 1. Pterostilbene in combination with colistin restores the in vitro sensitivity of K. pneumoniae to polymyxins. (A,B) Growth curves for K. pneumoniae ZJ02 (A) and K. pneumoniae ZJ05 (B) cultured in the presence of various concentrations (0–128 μg/mL) of pterostilbene. Values represent the averages of three independent experiments. (C,D) Time-killing curves for colistin, pterostilbene, colistin + pterostilbene, and control treatment (medium only) against K. pneumoniae ZJ02 (C) and K. pneumoniae ZJ05 (D). Values represent the averages of three independent experiments.
The combination of pterostilbene and colistin resulted in the lowest FIC index value, and thus was examined further via time-killing assays. The time-killing assays were performed using 16 μg/mL of pterostilbene and 4 μg/mL of colistin against K. pneumoniae grown in LB broth. When used alone, pterostilbene and colistin had little effect on bacterial growth. In contrast, the combination of pterostilbene and colistin resulted in the elimination of K. pneumoniae ZJ02 and K. pneumoniae ZJ05 at 1 h and 3 h post-administration, respectively (Figures 1C,D). Based on the results of the growth curve, pterostilbene concentrations of 0, 8, and 32 μg/mL were chosen for CDT assays. The results showed increases in the zones of inhibition only for mcr-1-positive E. coli and K. pneumoniae isolates (2.67 ± 0.58 mm, 4.33 ± 0.29 mm, and 4.67 ± 0.29 mm) using disks containing 10 μg of colistin plus 32 μg/mL of pterostilbene in comparison with the inhibition zones of disks containing 10 μg of colistin alone (Table 2 and Figure 2), and mcr-1-negative E. coli ATCC 25922 had little increases in the zones of inhibition with different concentrations of pterostilbene. We also confirmed that pterostilbene in combination with colistin increased the size of the inhibition zones in a dose-dependent manner.
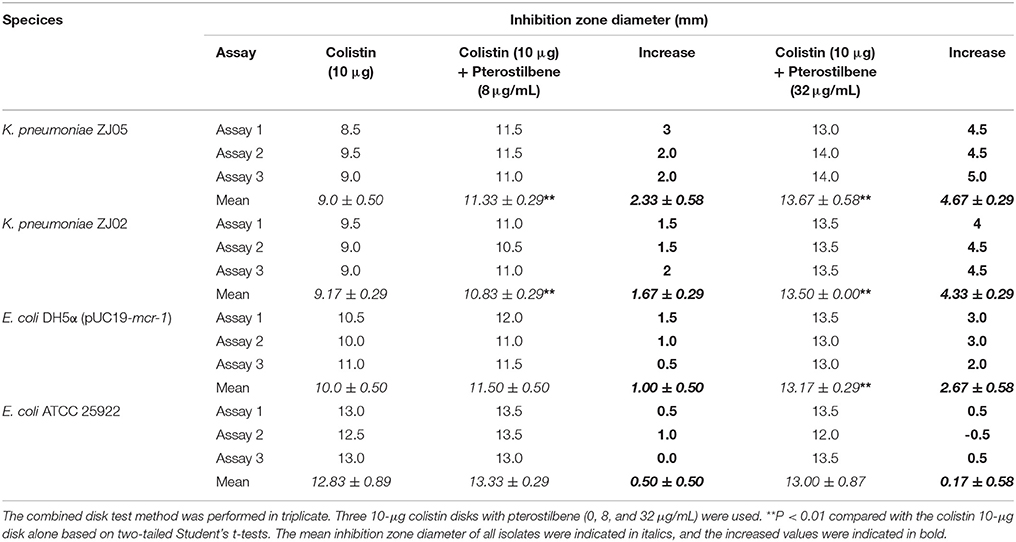
Table 2. Combined disk test for colistin in combination with pterostilbene for each of the tested bacterial isolates.
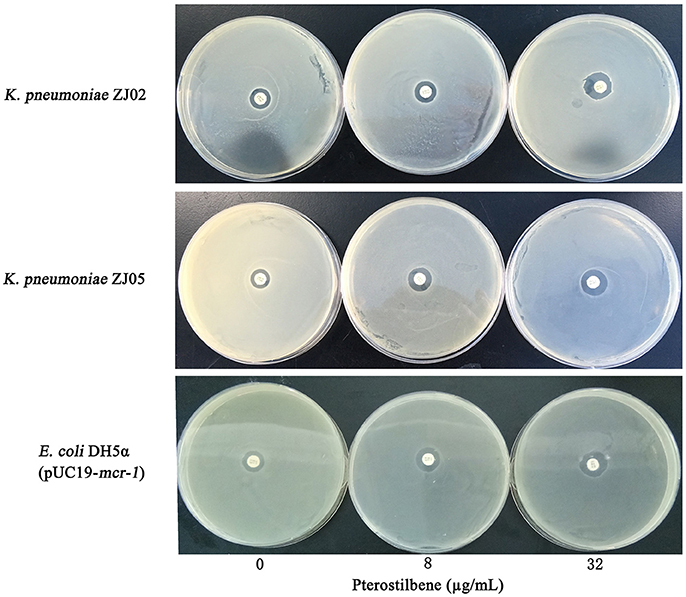
Figure 2. Zones of inhibition surrounding colistin disks supplemented with 0, 8, or 32 μg/mL of pterostilbene on lawns of K. pneumoniae ZJ02, K. pneumoniae ZJ05, and E. coli DH5α (pUC19-mcr-1) on MHB agar plates.
Combination Therapy Had a Synergistic Effect in Vivo in Comparison With Monotherapy or the Control
Based on the above results, we attempted to determine whether the synergistic effects could be replicated in vivo in a mouse model of pneumonia induced by K. pneumoniae. Mice were intranasally inoculated with K. pneumoniae ZJ05 and then treated with colistin (8 mg/kg), pterostilbene (50 mg/kg), pterostilbene (50 mg/kg) in combination with colistin (8 mg/kg), or PBS as a control at 2 h post-infection, and bacterial burden was assessed at 24 h post-infection. The combination of colistin and pterostilbene resulted in a significant reduction of the bacterial load in the lung compared with the monotherapy treatments (P < 0.01; Figure 3A), although the colistin-treated group also showed a significant decrease in CFU compared with the control group (P < 0.01).
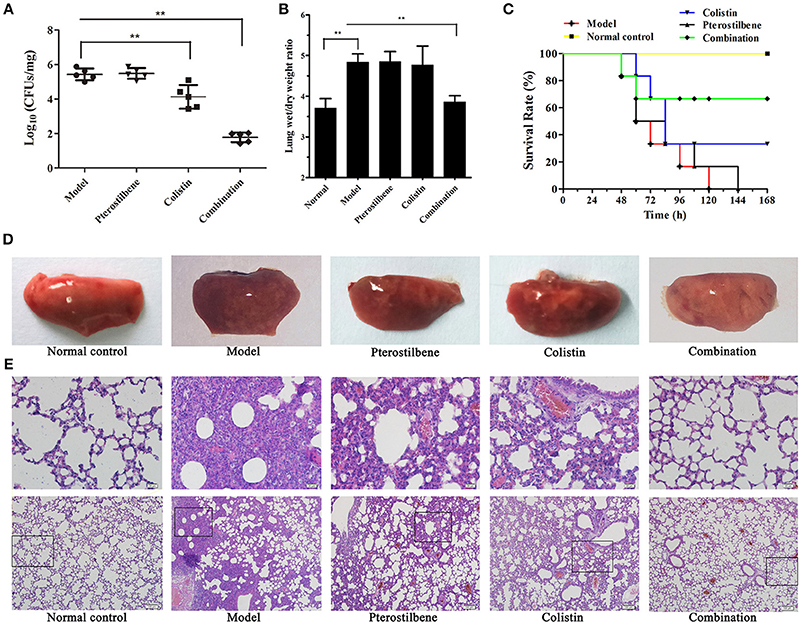
Figure 3. Effects of pterostilbene and colistin combination therapy in vivo. Mice were infected with K. pneumoniae ZJ05 and then treated with pterostilbene, colistin, pterostilbene combined with colistin (combination), or control solvent treatment (model). Uninfected mice were used as a healthy control (normal control). At 48 h post-inoculation, mice were euthanized and the bacterial burden (A) and wet/dry weight ratio (B) of lungs were calculated. **P < 0.01. (C) Survival curve of mice infected with K. pneumoniae ZJ05 and monitored for 7 days post-infection. The data represent the means and standard deviations from three separate experiments (18 mice per group). Gross pathological changes (D) and histopathology (E) of the lung tissue of mice from the first experiment were also assessed.
We assessed the degree of pulmonary edema via the wet/dry weight ratio of the left lung. The results showed that mice treated with the combination therapy had a significant decrease in wet/dry weight ratio compared with the other groups (Figure 3B). Histopathological analysis of lung tissue was also performed to evaluate the treatment efficacy of pterostilbene in combination with colistin against pulmonary injury. Gross macroscopic inspection revealed that the lungs of infected mice that receiving either of the monotherapies or the control treatment were crimson and exhibited severe congestion and pulmonary edema. In contrast, the lung tissue of mice treated with combination therapy remained pink and fungous (Figure 3D). Examination of the pathologic manifestations (Figure 3E) revealed that the infected mice in either the untreated or monotherapy-treated groups exhibited severe tissue injury and inflammatory cell aggregation. In contrast, the tissue sections of the mice in the combination therapy group were similar to those of the normal mice.
The combination therapy was further tested using a mouse survival model. Following infection with 1 × 108 CFU of K. pneumoniae ZJ05, the majority of mice treated with a single agent or the control succumbed to infection within 168 h. However, as shown in Figure 3C, 67% (12/18) of the mice treated with a combination of pterostilbene and colistin survived until the end of the experiment.
Discussion
Because of the significant burden of mcr-1-positive K. pneumoniae in a clinical setting, we investigated whether pterostilbene in combination with colistin could be used as a treatment for infections caused by colistin-resistant K. pneumoniae. Pterostilbene (trans-3,5-dimethoxy-4′-hydroxystilbene) is a naturally occurring phytoalexin found in several plant species. It has more favorable pharmacological properties than fellow phytoalexin resveratrol, including greater oral absorption efficiency, potential for greater cellular uptake, and a longer half-life. Moreover, it exhibits antibacterial activity against drug-resistant Staphylococcus aureus strains without inducing unacceptably high levels of cytotoxicity (0.125 mM) in mammalian cells. For example, administration of pterostilbene (3,000 mg/kg, daily, p.o.) for approximately 30 days did not result in remarkable local or systemic toxicity in mice. Another study showed that pretreatment of A/J mice with pterostilbene at doses of 50 and 250 mg/kg (i.p.) five times per week for 21 continuous weeks produced no signs of toxicity, such as changes in fur color, motor or behavioral abnormalities, or palpable masses (Chen et al., 2012). Pterostilbene is also generally safe for human consumption at doses of up to 250 mg per day, and is used as a dietary supplement to decrease the risk of coronary heart disease (Riche et al., 2013). Therefore, all studies confirm that pterostilbene has no measurable toxicity in animals or humans, regardless of the route of administration, and suggest that this natural compound is likely to be safe if applied in human clinical practice.
Although there is a significant synergistic effect of pterostilbene in combination with polymyxin, it is not enough to warrant the development of a therapeutic agent for clinical use. Therefore, it is necessary to study the molecular structure of pterostilbene, including modifications of the main chemical functional groups, which may be useful for reducing any potential side effects for clinical use. There are several limitations to the use of pterostilbene, including its low bioavailability and poor water solubility (Chen et al., 2012). However, compared with resveratrol, pterostilbene has a higher bioavailability and is processed more slowly (glucuronidated or sulfated) in vivo, which may increase the functionality of pterostilbene when applied in systemic infections (Chiou et al., 2011).
The mechanisms of resistance to polymyxins, including mutations in the PmrAB/PhoPQ two-component regulatory systems, loss of lipopolysaccharide, MgrB inactivation, and plasmid-mediated colistin resistance, all involve the modification of lipid A, resulting in a reduction of polymyxin affinity(Ah et al., 2014; Antonelli et al., 2017). A variety of polymyxin resistance mechanisms are present in Enterobacteriaceae species, with some strains containing two or more pathways (Baron et al., 2016; Poirel et al., 2017). Therefore, we need to further explore the mechanism of resistance in mcr-1-negative colistin-resistant K. pneumoniae isolates. In the current study, we used several standard methods to determine the synergy of pterostilbene, including disk diffusion assays carried out as described by the Clinical and Laboratory Standards Institute. This technique is still used for in vivo susceptibility testing in many countries despite the fact that polymyxins do not readily diffuse in agar, resulting in reduced reliability of the method for measuring MIC (Boyen et al., 2010; Albur et al., 2014; Esposito et al., 2017). Despite the limitations of this assay method, we observed significant differences in MCR-1-producing isolates K. pneumoniae ZJ02, K. pneumoniae ZJ05, and E. coli DH5α (pUC19-mcr-1) compared with E. coli ATCC25922.
In summary, this study shows that a combination of polymyxins and pterostilbene could be a viable alternative treatment option for combating K. pneumoniae strains harboring mobile polymyxin resistance gene mcr-1. In addition, this alternative strategy provides potential opportunities to abate pathogenicity and its consequences without placing selective pressure on the target bacterium (Song et al., 2017). Furthermore, by reducing the amount of polymyxins used in clinical therapy, this strategy may also decrease the possibility of mutations arising in LPS modification pathways in K. pneumoniae, as can occur following long-term use of polymyxins. Further studies, including elucidation of the mechanism of inhibition of MCR-1 by pterostilbene, are needed to optimize the effects of combination therapy.
Conclusion
In this study, we identified a natural compound of a Traditional Chinese Medicine, pterostilbene, when used in combination with colistin, regain its bactericidal activity against the mcr-1-positive K. pneumoniae. The microdilution checkerboard method confirmed that the pterostilbene reduces the MIC of colistin in mcr-1-positive K. pneumoniae strains from resistance to sensitive. The time-killing assays showed that either pterostilbene or colistin failed to eradicate ZJ02 and ZJ05, but the combination eliminated ZJ02 and ZJ05 by 1–3 h post-inoculation. The mouse infection model demonstrated that the survival rate of mice following the infection with ZJ05 was significantly promoted from 0% in the group of the administrated as monotherapy to 67% in the group of combination therapy applied.
Author Contributions
XD, YW, and YZ: Study design. YZ, TW, and YG: Experimental studies. SL, YS, and ST: Data analysis, interpretation. YZ and JW: Statistical analysis. XD, YW, and YZ: Manuscript preparation.
Conflict of Interest Statement
The authors declare that the research was conducted in the absence of any commercial or financial relationships that could be construed as a potential conflict of interest.
Acknowledgments
This work was supported by the National Key R&D Program of China (2017YFD0501500), the National Natural Science Foundation of China (31422055 and 81661138002), and the National Key Technology R&D Program (no. 2016YFD 05013).
References
Ah, Y. M., Kim, A. J., and Lee, J. Y. (2014). Colistin resistance in Klebsiella pneumoniae. Int. J. Antimicrob. Agents 44, 8–15. doi: 10.1016/j.ijantimicag.2014.02.016
Albur, M., Noel, A., Bowker, K., and Macgowan, A. (2014). Colistin susceptibility testing: time for a review. J. Antimicrob. Chemother. 69, 1432–1434. doi: 10.1093/jac/dkt503
Antonelli, A., D'Andrea, M. M., Giani, T., and Rossolini, G. M. (2017). Plasmid-mediated or chromosomally mediated colistin resistance in Klebsiella pneumoniae? Lancet Infect. Dis. 17, 26–27. doi: 10.1016/S1473-3099(16)30552-7
Baron, S., Hadjadj, L., Rolain, J. M., and Olaitan, A. O. (2016). Molecular mechanisms of polymyxin resistance: knowns and unknowns. Int. J. Antimicrob. Agents 48, 583–591. doi: 10.1016/j.ijantimicag.2016.06.023
Bowers, D. R., Cao, H., Zhou, J., Ledesma, K. R., Sun, D., Lomovskaya, O., et al. (2015). Assessment of minocycline and polymyxin B combination against Acinetobacter baumannii. Antimicrob. Agents Chemother. 59, 2720–2725. doi: 10.1128/AAC.04110-14
Boyen, F., Vangroenweghe, F., Butaye, P., De Graef, E., Castryck, F., Heylen, P., et al. (2010). Disk prediffusion is a reliable method for testing colistin susceptibility in porcine E. coli strains. Vet. Microbiol. 144, 359–362. doi: 10.1016/j.vetmic.2010.01.010
Bulman, Z. P., Chen, L., Walsh, T. J., Satlin, M. J., Qian, Y., Bulitta, J. B., et al. (2017). Polymyxin combinations combat Escherichia coli harboring mcr-1 and blaNDM-5: preparation for a postantibiotic era. MBio 8:e00540-17. doi: 10.1128/mBio.00540-17
Chen, R. J., Tsai, S. J., Ho, C. T., Pan, M. H., Ho, Y. S., Wu, C. H., et al. (2012). Chemopreventive effects of pterostilbene on urethane-induced lung carcinogenesis in mice via the inhibition of EGFR-mediated pathways and the induction of apoptosis and autophagy. J Agric. Food Chem. 60, 11533–11541. doi: 10.1021/jf302778a
Chiou, Y. S., Tsai, M. L., Nagabhushanam, K., Wang, Y. J., Wu, C. H., Ho, C. T., et al. (2011). Pterostilbene is more potent than resveratrol in preventing azoxymethane (AOM)-induced colon tumorigenesis via activation of the NF-E2-Related Factor 2 (Nrf2)-mediated antioxidant signaling pathway. J Agric. Food Chem. 59, 2725–2733. doi: 10.1021/jf2000103
Cichocki, M., Paluszczak, J., Szaefer, H., Piechowiak, A., Rimando, A. M., and Baerdubowska, W. (2008). Pterostilbene is equally potent as resveratrol in inhibiting 12-O-tetradecanoylphorbol-13-acetate activated NFkappaB, AP-1, COX-2, and iNOS in mouse epidermis. Mol. Nutr. Food Res. 52(Suppl. 1):S62–S70 doi: 10.1002/mnfr.200700466
Espinel-Ingroff, A., Colombo, A. L., Cordoba, S., Dufresne, P. J., Fuller, J., Ghannoum, M., et al. (2016). International evaluation of MIC distributions and epidemiological cutoff value (ECV) definitions for fusarium species identified by molecular methods for the CLSI broth microdilution method. Antimicrob. Agents Chemother. 60, 1079–1084. doi: 10.1128/AAC.02456-15
Esposito, F., Fernandes, M. R., Lopes, R., Muñoz, M., Sabino, C. P., Cunha, M. P., et al. (2017). Detection of colistin-resistant MCR-1-positive Escherichia coli using inhibition by EDTA and zeta potential assays. J. Clin. Microbiol. 55, 3454–3465. doi: 10.1128/JCM.00835-17
Giamarellou, H. (2016). Epidemiology of infections caused by polymyxin-resistant pathogens. Int. J. Antimicrob. Agents 48, 614–621. doi: 10.1016/j.ijantimicag.2016.09.025
Halaby, T., Al Naiemi, N., Kluytmans, J., van der Palen, J., and Vandenbroucke-Grauls, C. M. (2013). Emergence of colistin resistance in Enterobacteriaceae after the introduction of selective digestive tract decontamination in an intensive care unit. Antimicrob. Agents Chemother. 57, 3224–3229. doi: 10.1128/AAC.02634-12
Hrabák, J., Niemczyková, J., Chudácková, E., Fridrichová, M., Studentová, V., Cervená, D., et al. (2011). KPC-2-producing Klebsiella pneumoniae isolated from a Czech patient previously hospitalized in Greece and in vivo selection of colistin resistance. Folia. Microbiol. (Praha) 56, 361–365. doi: 10.1007/s12223-011-0057-6
Kapetanovic, I. M., Muzzio, M., Huang, Z., Thompson, T. N., and McCormick, D. L. (2011). Pharmacokinetics, oral bioavailability, and metabolic profile of resveratrol and its dimethylether analog, pterostilbene, in rats. Cancer Chemother. Pharmacol. 68, 593–601. doi: 10.1007/s00280-010-1525-4
Kieffer, N., Aires-de-Sousa, M., Nordmann, P., and Poirel, L. (2017). High Rate of MCR-1-Producing Escherichia coli and Klebsiella pneumoniae among Pigs, Portugal. Emerg. Infect. Dis. 23, 2023–2029. doi: 10.3201/eid2312.170883
Li, J., Dong, J., Qiu, J. Z., Wang, J. F., Luo, M. J., Li, H. E., et al. (2011). Peppermint oil decreases the production of virulence-associated exoproteins by Staphylococcus aureus. Molecules 16, 1642–1654. doi: 10.3390/molecules16021642
Liu, Y. Y., Chandler, C. E., Leung, L. M., McElheny, C. L., Mettus, R. T., Shanks, R. M. Q., et al. (2017). Structural modification of lipopolysaccharide conferred by mcr-1 in gram-negative ESKAPE pathogens. Antimicrob. Agents Chemother. 61:e00580-17. doi: 10.1128/AAC.00580-17
Liu, Y. Y., Wang, Y., Walsh, T. R., Yi, L. X., Zhang, R., Spencer, J., et al. (2016). Emergence of plasmid-mediated colistin resistance mechanism MCR-1 in animals and human beings in China: a microbiological and molecular biological study. Lancet Infect. Dis. 16, 161–168. doi: 10.1016/S1473-3099(15)00424-7
Ma, L., Wang, Y., Wang, M., Tian, Y., Kang, W., Liu, H., et al. (2016). Effective antimicrobial activity of Cbf-14, derived from a cathelin-like domain, against penicillin-resistant bacteria. Biomaterials 87, 32–45. doi: 10.1016/j.biomaterials.2016.02.011
Morrill, H. J., Pogue, J. M., Kaye, K. S., and LaPlante, K. L. (2015). Treatment options for carbapenem-resistant enterobacteriaceae infections. Open Forum Infect. Dis. 2:ofv050. doi: 10.1093/ofid/ofv050
Olaitan, A. O., Morand, S., and Rolain, J. M. (2014). Mechanisms of polymyxin resistance: acquired and intrinsic resistance in bacteria. Front. Microbiol. 5:643. doi: 10.3389/fmicb.2014.00643
Petersen, P. J., Labthavikul, P., Jones, C. H., and Bradford, P. A. (2006). In vitro antibacterial activities of tigecycline in combination with other antimicrobial agents determined by chequerboard and time-kill kinetic analysis. J. Antimicrob. Chemother. 57, 573–576. doi: 10.1093/jac/dki477
Pogue, J. M., Lee, J., Marchaim, D., Yee, V., Zhao, J. J., Chopra, T., et al. (2011). Incidence of and risk factors for colistin-associated nephrotoxicity in a large academic health system. Clin. Infect. Dis. 53, 879–884. doi: 10.1093/cid/cir611
Poirel, L., Jayol, A., and Nordmann, P. (2017). Polymyxins: antibacterial activity, susceptibility testing, and resistance mechanisms encoded by plasmids or chromosomes. Clin. Microbiol. Rev. 30, 557–596. doi: 10.1128/CMR.00064-16
Pournaras, S., Zarkotou, O., Poulou, A., Kristo, I., Vrioni, G., Themeli-Digalaki, K., et al. (2013). A combined disk test for direct differentiation of carbapenemase-producing enterobacteriaceae in surveillance rectal swabs. J. Clin. Microbiol. 51, 2986–2990. doi: 10.1128/JCM.00901-13
Quan, J., Li, X., Chen, Y., Jiang, Y., Zhou, Z., Zhang, H., et al. (2017). Prevalence of mcr-1 in Escherichia coli and Klebsiella pneumoniae recovered from bloodstream infections in China: a multicentre longitudinal study. Lancet Infect. Dis. 17, 400–410. doi: 10.1016/S1473-3099(16)30528-X
Riche, D. M., McEwen, C. L., Riche, K. D., Sherman, J. J., Wofford, M. R., Deschamp, D., et al. (2013). Analysis of safety from a human clinical trial with pterostilbene. J. Toxicol. 2013:463595. doi: 10.1155/2013/463595
Roupe, K. A., Remsberg, C. M., Yáñez, J. A., and Davies, N. M. (2006). Pharmacometrics of stilbenes: seguing towards the clinic. Curr. Clin. Pharmacol. 1, 81–101. doi: 10.2174/157488406775268246
Song, M., Teng, Z., Li, M., Niu, X., Wang, J., and Deng, X. (2017). Epigallocatechin gallate inhibits Streptococcus pneumoniae virulence by simultaneously targeting pneumolysin and sortase A. J. Cell Mol. Med. 21, 2586–2598. doi: 10.1111/jcmm.13179
Wang, Y., Tian, G. B., Zhang, R., Shen, Y., Tyrrell, J. M., Huang, X., et al. (2017). Prevalence, risk factors, outcomes, and molecular epidemiology of mcr-1-positive Enterobacteriaceae in patients and healthy adults from China: an epidemiological and clinical study. Lancet Infect. Dis. 17, 390–399. doi: 10.1016/S1473-3099(16)30527-8
Watts, J. L. (2013). Performance Standards for Antimicrobial Disk and Dilution Susceptibilty Tests for Bacteria Isolated From Animals; Approved Standard. Pennsylvania, PA: Clinical and Laboratory Standards Institute.
Wiegand, I., Hilpert, K., and Hancock, R. E. (2008). Agar and broth dilution methods to determine the minimal inhibitory concentration (MIC) of antimicrobial substances. Nat. Protoc. 3, 163–175. doi: 10.1038/nprot.2007.521
Zhou, Y. F., Tao, M. T., Huo, W., Liao, X. P., Sun, J., and Liu, Y. H. (2017). In vivo pharmacokinetic and pharmacodynamic profiles of antofloxacin against Klebsiella pneumoniae in a neutropenic murine lung infection model. Antimicrob. Agents Chemother. 61:e02691-16. doi: 10.1128/AAC.02691-16
Keywords: K. pneumonia, MCR-1 inhibitor, pterostilbene, colistin, combination therapy
Citation: Zhou Y, Wang T, Guo Y, Liu S, Wang J, Shen Y, Tang S, Wang Y and Deng X (2018) In Vitro/Vivo Activity of Potential MCR-1 Inhibitor in Combination With Colistin Againsts mcr-1-Positive Klebsiella pneumonia. Front. Microbiol. 9:1615. doi: 10.3389/fmicb.2018.01615
Received: 09 May 2018; Accepted: 28 June 2018;
Published: 17 July 2018.
Edited by:
Raffaele Zarrilli, University of Naples Federico II, ItalyReviewed by:
Antonio Cannatelli, University of Siena, ItalyRoberta Migliavacca, University of Pavia, Italy
Copyright © 2018 Zhou, Wang, Guo, Liu, Wang, Shen, Tang, Wang and Deng. This is an open-access article distributed under the terms of the Creative Commons Attribution License (CC BY). The use, distribution or reproduction in other forums is permitted, provided the original author(s) and the copyright owner(s) are credited and that the original publication in this journal is cited, in accordance with accepted academic practice. No use, distribution or reproduction is permitted which does not comply with these terms.
*Correspondence: Yang Wang, wangyang@cau.edu.cn
Xuming Deng, dengxm@jlu.edu.cn
†These authors have contributed equally to this work.