- 1State Key Laboratory of Food Science and Technology, Jiangnan University, Wuxi, China
- 2Key Laboratory of Industrial Biotechnology, Ministry of Education, School of Biotechnology, Jiangnan University, Wuxi, China
- 3International Joint Laboratory on Food Safety, Jiangnan University, Wuxi, China
Cronobacter species can cause necrotizing enterocolitis and meningitis in neonates and infants, their infection is closely relevant to their responses to extreme growth conditions. In this study, the response of Cronobacter species to amino acid deficiency has been investigated. Four Cronobacter species formed smooth colonies when grown on the solid LB medium, but formed mucoid colonies when grown on the amino acid deficient M9 medium. When the mucoid colonies were stained with tannin mordant, exopolysaccharide around the cells could be discerned. The exopolysaccharide was isolated, analyzed, and identified as colanic acid. When genes wcaD and wcaE relevant to colanic acid biosynthesis were deleted in Cronobacter sakazakii BAA-894, no exopolysaccharide could be produced, confirming the exopolysaccharide formed in C. sakazakii grown in M9 is colanic acid. On the other hand, when genes rcsA, rcsB, rcsC, rcsD, or rcsF relevant to Rcs phosphorelay system was deleted in C. sakazakii BAA-894, colanic acid could not be produced, suggesting that the production of colanic acid in C. sakazakii is regulated by Rcs phosphorelay system. Furthermore, C. sakazakii BAA-894 grown in M9 supplemented with amino acids could not produce exopolysaccharide. Transcriptomes of C. sakazakii BAA-894 grown in M9 or LB were analyzed. A total of 3956 genes were differentially expressed in M9, of which 2339 were up-regulated and 1617 were down-regulated. When C. sakazakii BAA-894 was grown in M9, the genes relevant to the biosynthesis of exopolysaccharide were significantly up-regulated; on the other hand, the genes relevant to the flagellum formation and chemotaxis were significantly down-regulated; in addition, most genes relevant to various amino acid biosynthesis were also significantly regulated. The results demonstrate that amino acid deficiency has a global impact on C. sakazakii cells.
Introduction
Cronobacter species are food-borne opportunistic pathogens that can cause necrotizing enterocolitis and meningitis in neonates and infants (Feeney et al., 2014; Forsythe, 2017). Cronobacter sakazakii, C. dublinensis, C. malonaticus, and C. turicensis are the common Cronobacter isolates, especially C. sakazakii which has been extensively studied (Zhang et al., 2014; Liang et al., 2017; Li et al., 2017). C. sakazakii is much more resistant than other Enterobacteriaceae to environmental stresses (Dancer et al., 2009), which might be associated with its pathogenesis (Alvarez-Ordóñez et al., 2014). Biofilm formation (Jung et al., 2013), exopolysaccharide production, and lipopolysaccharide structure modification (Wang and Quinn, 2010; Zhang et al., 2010; Wiederschain, 2011; Cai et al., 2013; Wang et al., 2015; Liu et al., 2016) are various strategies for C. sakazakii to cope with the environmental stress.
Bacteria tend to use abundant nutrients in the surroundings for its maximum growth but could also survive in the environment lacking enough nutrients. The shortage of amino acids, the most important nitrogen source for bacteria, can lead to a coordinated regulation of metabolism, which is very important for the bacteria adapting to the new environment (Kuroda et al., 1999). In Escherichia coli, amino acid starvation results in the phosphorylation of the response regulator NtrC, which activates RelA (Shyp et al., 2012). RelA is responsible for synthesizing the ppGpp, which causes the stringent response (Brown et al., 2014). Amino acid starvation can result in the coordinate inhibition of a variety of metabolic activities in E. coli, such as fatty acid and phospholipid biosynthesis (Podkovyrov and Larson, 1996). Amino acid starvation in C. sakazakii has not been investigated yet. Understanding the responses of C. sakazakii to amino acid starvation might provide important information for C. sakazakii infection.
In this study, the response of C. sakazakii BAA-894 cells to amino acid deficiency has been investigated. When amino acids were not available, the significantly regulated genes in C. sakazakii cells include not only the ones relevant to various amino acid biosynthesis, but also the ones relevant to the biosynthesis of exopolysaccharide, flagellum, and chemotaxis; the results suggest that amino acid deficiency has a global impact on C. sakazakii cells.
Materials and Methods
Bacterial Strains and Growth Conditions
Cronobacter species were grown at 30°C in Luria-Bertani (LB) and M9 media. Luria-Bertani medium contains 5 g/l yeast extract, 10 g/l tryptone, and 10 g/l NaCl. The M9 medium contains 17.1 g/l Na2HPO4⋅12H2O, 3 g/l KH2PO4, 4 g/l glucose, 1 g/l NH4Cl, 0.5 g/l NaCl, 0.24 g/l MgSO4, and 0.011 g/l CaCl2. Fifteen important amino acids, L-aspartic acid (Asp), L-glutamic acid (Glu), L-serine (Ser), L-histidine (His), L-glycine (Gly), L-threonine (Thr), L-arginine (Arg), L-alanine (Ala), L-cysteine (Cys), L-valine (Val), L-methionine (Met), L-phenylalanine (Phe), L-isoleucine (Ile), L-leucine (Leu), and L-lysine (Lys) with the final concentration of 0.5 or 5 mM were added in M9 medium when necessary.
Identification of Exopolysaccharide
Exopolysaccharide was identified by the tannin mordant staining method (Ren et al., 2016). Cronobacter species were grown on agar media of LB, M9, or M9 with amino acids at 30°C for 2 days. The bacterial colonies were fixed on slides by smearing and drying, and then treated with the fuchsin solution for 3 min. The fuchsin solution was composed of 0.3 g basic fuchsin, 10 ml 95% ethanol, and 90 ml 5% phenol. Thr freshly prepared mordant solution was then added, incubated for 3 min, and washed with distilled water. Mordant solution contains 2 volumes of 0.3 g/l FeCl3, 2 volumes of 1.5 g/l tannins, and 5 volumes of 2 g/l saturated potassium aluminum sulfate solution. Prior to examination of the samples under the oil immersion lens, 1% methylene blue was used to counterstain for 1 min. The cells were stained red and the exopolysaccharide blue.
Construction of Cronobacter sakazakii Mutants
The lambda Red recombinase system was used to delete genes in C. sakazakii BAA-894 (Datsenko and Wanner, 2000; Serra-Moreno et al., 2006). To delete the genes ESA_RS05320 and ESA_RS05325 (homologs of E. coli wcaD and wcaE, respectively), their upstream and downstream fragments were PCR amplified using the primer pairs wcaDE-U-F/wcaDE-U-R and wcaDE-D-F/wcaDE-D-R, respectively. The PCR product of the upstream fragment was digested with SacI and EcoRI, while that of the downstream fragment was digested with BamHI and NdeI. The DNA fragment loxP-kan-loxP containing the kanamycin resistance gene kan was amplified from pDTW202 using the primer pairs of kan-loxP-F/kan-loxP-R, and digested with EcoRI and BamHI. The three digested fragments were inserted into pBlueScript II SK (+) which was digested with SacI and NdeI, resulting the plasmid pBS-kan-wcaDE which carries the knockout DNA fragment wcaDEU-loxP-kan-loxP-wcaDED. The knockout DNA fragment was then PCR amplified using the primer pairs wcaDE-U-F/wcaDE-D-R, and transferred into BAA894 cells containing the plasmid pKD46 by electroporation, resulting in the replacement of ESA_RS05320 and ESA_RS05325 with loxP-kan-loxP in the chromosome. After the correct transformants were selected by growing cells on LB plates containing kanamycin, the temperature sensitive plasmid pKD46 was cured by growing the cells at 42°C. The plasmid pKD-Cre was then introduced into the cells, and the kan gene in the chromosome was removed by the loxP recombinase Cre. The temperature sensitive plasmid pKD-Cre was cured by growing at 42°C, resulting in the mutant strain ΔwcaDE. The successful insertion and deletion of kan in the chromosome were confirmed by PCR analysis. Similarly, the genes ESA_RS05720, ESA_RS04445, ESA_RS04440, ESA_RS04450, or ESA_RS14450 (homologs of E. coli rcsA, rcsB, rcsC, rcsD, or rcsF, respectively) in the chromosome of C. sakazakii BAA894 were removed, resulting in the mutants ΔrcsA, ΔrcsB, ΔrcsC, ΔrcsD, or ΔrcsF, respectively. All of the strains and plasmids used in this study are listed in Table 1. All primers used in this study are listed in Table 2. Since there are no selection markers left on the chromosomes of these mutant strains, they could grow in medium without the addition of any antibiotics.
Purification of Exopolysaccharide
Exopolysaccharide was purified from C. sakazakii BAA-894 according to the published procedure (Navasa et al., 2009) with minor modification. Cells were grown in M9 medium at 30°C and 200 rpm for 24 h and harvested by centrifugation. The supernatant was collected and mixed with 2 volumes of ice-cold anhydrous ethanol. After precipitating at 4°C for 3 h, the solid product was resuspended in water to a concentration of 100 mg/l. Nucleic acids were precipitated by adding 0.75% streptomycin sulfate (w/v), and removed by centrifugation at 13,800 × g for 30 min. Lipopolysaccharide was precipitated by treating with 1% glacial acetic acid (v/v) at 100°C for 2 h, and removed by centrifugation at 13,800 × g for 15 min at 4°C (Ren et al., 2016). The supernatant was extracted with 1 volume of chloroform–methanol (2:1, v:v), and the aqueous phase was collected, dialyzed against distilled water (MWCO, 3600) for 48 h, and then lyophilized.
Colanic Acid Analysis by High-Performance Liquid Chromatography
Two milligram colanic acid (CA) sample was mixed with 1 ml 4 M trifluoroacetic acid solution in a tube and heated at 110 °C for 1 h; 1 ml methanol was then added in the mixture and dried under N2. This process was repeated twice. The sample was then resolved in 1 ml deionized water and analyzed by high-performance liquid chromatography (HPLC) with an ion-exchange column (HPLC ICS-5000; Dionex Corporation, United States). A CarboPac PA20 column and a pulsed amperometry detector were used. Mobile phases used were 6.5 mM NaOH in the first 21 min, 6.5 mM NaOH and 50–200 mM NaAc for 21–30 min, and 200 mM NaOH for 30-50 min. The flow rate was 0.5 ml/min.
Quantitative Determination of Colanic Acid Production
Cronobacter sakazakii BAA-894 grown in liquid medium at 30°C for 48 h, and samples were collected at 12, 24, 36, and 48 h, then heated in a water bath at 90°C for 20 min to denature enzymes and release CA. After cooling, a 3 ml sample was centrifuged at 13,800 × g for 20 min, the supernatant was mixed with 2 volumes of ice-cold anhydrous ethanol, and incubated at 4°C for 3 h. The precipitate was collected by centrifugation at 10,000 × g for 15 min, dried and used for CA determination (Blumenkrantz and Asboe-Hansen, 1973). The sample was dissolved with 0.5 ml distilled water, treated with 2.5 ml sodium tetraborate/sulfuric acid solution (0.475 mg/l) and heated at 100°C for 3 min. After cooling, 100 μl hydroxy diphenyl (1.5 g/l) solution was added to the sample, and the absorbance at 526 nm was measured. The liquid medium was used as a control, and the standard curve was made using different concentrations of glucuronic acid. The number of cells used to determine the levels of CA production were normalized by serial dilution and the plating method (Ren et al., 2016).
Quantification of Amino Acids
Cronobacter sakazakii BAA-894 cells were grown in medium of LB, M9, and M9 containing 0.5 or 5 mM amino acids at 30°C for 48 h, and centrifuged at 13800 × g for 20 min. The supernatant was mixed with the same volume of 10% Trichloroacetic acid to sediment at least 5 h. All samples were centrifuged at 13800 × g for 20 min again and filtered; the levels of 15 amino acids in the sample were then determined by HPLC (Agilent Technologies 1200 series, United States). The aqueous phase included 3.02 g/l sodium acetate, 200 μl/l trimethylamine and 5 ml/l tetrahydrofuran; the organic phase was composed of 3.02 g/l sodium acetate, 400 ml/l methanol and 400 ml/l acetonitrile. The pH of the two phases was adjusted to 7.2 with acetic acid.
Whole Genome Transcriptional Analysis of Cronobacter sakazakii
For transcriptome analysis, C. sakazakii BAA-894 cells were cultivated in LB or M9 media at 30°C to the later exponential phase. BAA-894 cells were grown in LB for 6 h or in M9 for 9 h until they reached the mid-log phase. The samples were collected at 13,800 × g for 10 min and washed by using phosphate buffer solution. The whole libraries of RNA were prepared and sequenced using an Illumina HiSeq 2000 (BGI Shenzhen, China). The whole genome transcriptional analysis was established according to a published method (Marisch et al., 2013). The differentially expressed genes were determined based on their expression levels in the different strains (Mortazavi et al., 2008), and the KEGG (Kyoto Encyclopedia of Genes and Genomes) pathway and GO (Gene ontology) analysis were performed. The significance of the differences in gene expression was judged by the thresholds of false discovery rate (FDR ≤ 0.001) and the ratio of gene expression in the M9 medium versus the same gene in the LB medium using a logarithmic scale to base 2 (log2R ≥ 1) (Kim and van de Wiel, 2008). The KEGG was used to perform the pathway enrichment analysis of the differentially expressed genes and to identify the signal transduction pathways or significantly enriched metabolic pathways based on the differentially expressed genes using the major public pathway-related database1 (Kanehisa et al., 2008). After correction with a significant level of 0.05, data analysis and the overrepresented biological process categories were generated. The GO analysis provides all GO terms that were significantly enriched in the list of differentially expressed genes. All differentially expressed genes were mapped to the GO terms in the database2, and the gene numbers for each term were calculated using GO-TermFinder3 (Ball et al., 2005); then, the hypergeometric test was used to find the significantly enriched GO terms in the input list of the differentially expressed genes. Transcriptome data have been deposited in the NCBI Sequence Read Archive database and linked with accession numbers SAMN08723122 (BAA-894 in M9 medium) and SAMN08723122 (BAA-894 in LB medium), respectively.
RNA Extraction and Transcriptional Analysis Through RT-PCR
Total RNA was prepared using the Qiagen RNeasy Total RNA kit. For RT-PCR assays, 1 μg RNA sample was treated with RNase-free DNase I Set (Qiagen) and transcribed to cDNA with SuperScript II reverse transcriptase (Thermo Fisher) using random hexamers (Thermo Fisher) as primers. RT-PCR reactions were performed using the Applied Biosystems step one real-time PCR system (Life Technologies, California, United States). Relative transcript abundance was calculated using the ΔΔCT method (Livak and Schmittgen, 2001). Transcriptional data were normalized, using 16s RNA as a control. The transcription of a given gene was calculated as the difference in qPCR threshold cycles (ΔCT). As one PCR cycle represents a twofold difference in template abundance, fold change values are calculated as 2-ΔΔCT. Three independent experiments were performed.
Results
Cronobacter Species Produce a Large Amount of Exopolysaccharide When Grown in M9
When four Cronobacter species (C. turicensis DSM18703, C. dublinensis DSM18705, C. muytjensii ATCC51329, and C. sakazakii BAA-894) were grown on solid M9 medium, they all formed mucoid colonies; however, when they were grown on solid LB medium, no mucoid colonies were observed. Mucoid colonies are usually associated with excessive production of exopolysaccharide, which can be confirmed by tannin mordant staining. Therefore, colonies of Cronobacter species DSM18703, DSM18705, ATCC51329, and BAA-894 grown on solid LB or M9 media were stained with tannin mordant and observed under microscopy (Figure 1). Large amounts of bluish substance were observed around the cells of Cronobacter species DSM18703, DSM18705, ATCC51329, or BAA-894 grown on M9, but no bluish substance was observed around the cells grown on LB (Figure 1). The LB medium contains tryptone, a pancreatic digest of casein which contains various amino acids, while M9 medium contains no amino acids. The results demonstrate that Cronobacter species could produce a large amount of exopolysaccharide when grown in the amino acid deficient medium M9 but not LB, suggesting the production of exopolysaccharide might be related to the availability of amino acids in the medium.
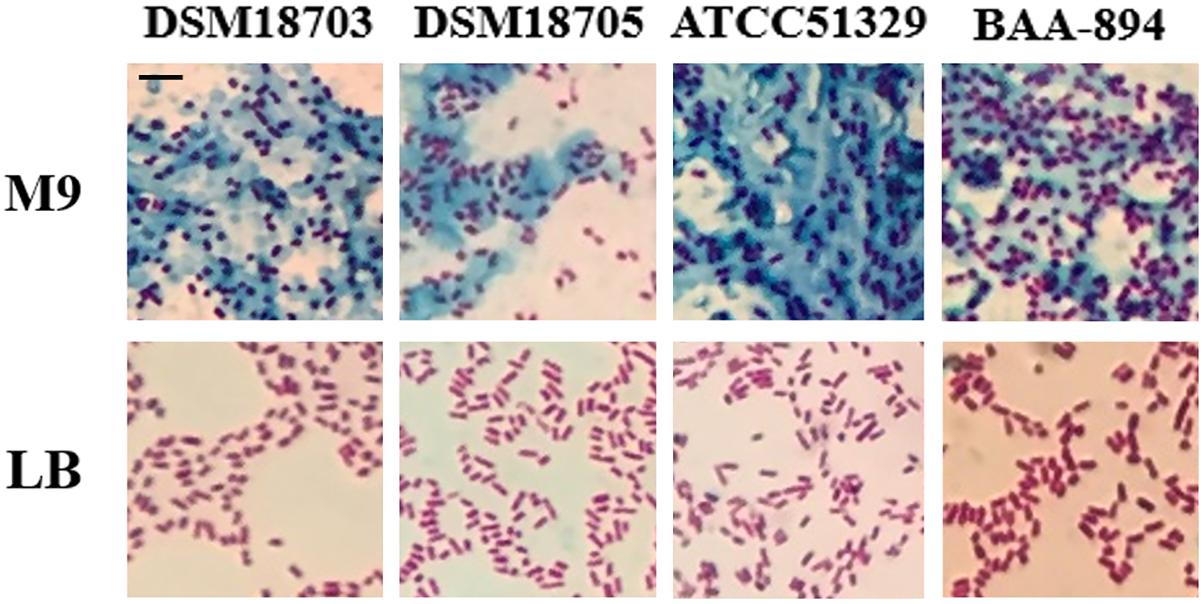
FIGURE 1. Light microscopic observation of four Cronobacter species grown on solid LB or M9 media and stained with mordant. Scale bar, 10 μm.
Colanic Acid Is Produced in C. sakazakii BAA-894 Grown in M9 and the Production Is Regulated by the Rcs Phosphorelay System
Several types of exopolysaccharides can be produced in C. sakazakii (Ogrodzki and Forsythe, 2017). To determine the type of the exopolysaccharide produced by C. sakazakii grown in M9, C. sakazakii BAA-894 cells grown in liquid M9 medium, exopolysaccharide was collected from the cell culture, purified, hydrolyzed, and analyzed by HPLC (Figure 2). In the chromatogram, five peaks with different retention times (3.3, 9.0, 10.6, 26.3, and 29.2 min) were observed. Under the same analysis condition, fucose, glucose, galactose, and glucuronic acid yielded peaks at 3.3, 9.1, 10.6, and 29.2 min, respectively. Fucose, glucose, galactose, and glucuronic acid are the major components of E. coli CA (Sutherland, 1969). This analysis demonstrates that the major exopolysaccharide secreted by BAA-894 grown in M9 is CA, and C. sakazakii and E. coli share the similar CA structure. The strong peak around 26.2 min observed in all spectra was caused by the gradient change of the mobile phase during analysis (Figure 2).
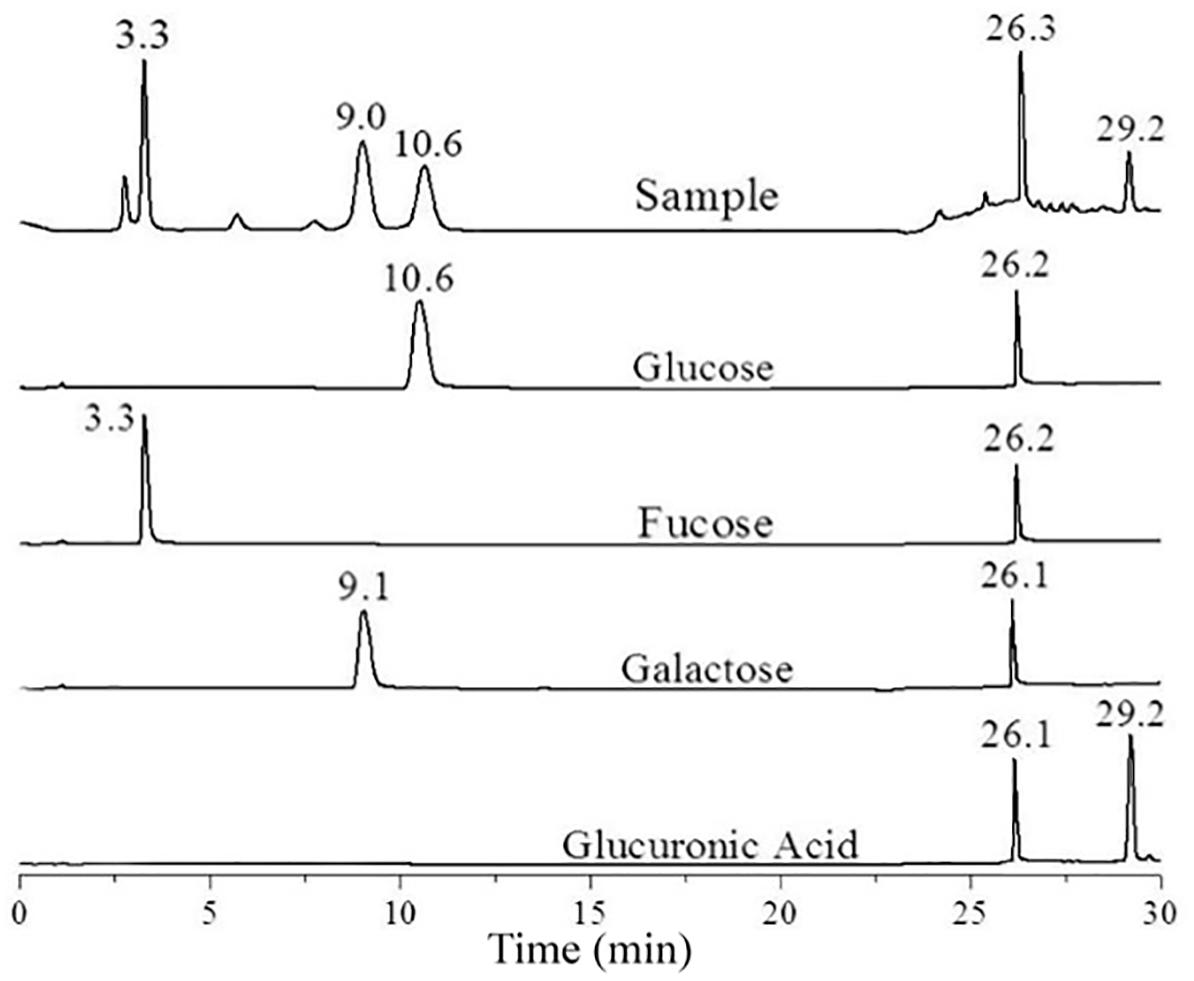
FIGURE 2. High-performance liquid chromatography analysis of the hydrolyzed exopolysaccharide isolated from Cronobacter sakazakii BAA-894 cells grown in liquid M9 medium.
In E. coli, the wca cluster encodes the enzymes for CA biosynthesis (Stevenson et al., 1996), and its expression is regulated by the Rcs phosphorelay system (Majdalani and Gottesman, 2005). All the homolog genes relevant to CA biosynthesis and the Rcs phosphorelay system also exist in C. sakazakii BAA-894 (Figure 3). Two variants of the CA synthesis gene cluster (CA1 and CA2) are found in C. sakazakii isolates, and the ones containing CA2 are associated with neonatal meningitis and necrotizing enterocolitis (Ogrodzki and Forsythe, 2015). The CA1 and CA2 differ with the absence of galE in CA2. C. sakazakii BAA-894 does contain galE in its CA cluster (Figure 3A), but its identity with E. coli galE is only 30%, while the other genes in the cluster show at least 69% identity with their E. coli counterparts. To confirm if the exopolysaccharide secreted by C. sakazakii BAA-894 cells is CA, two contiguous genes ESA_RS05320 (homolog of E. coli wcaD) and ESA_RS05325 (homolog of E. coli wcaE) in the chromosome of BAA-894 were deleted, resulting in the mutant strain ΔwcaDE. WcaD is the CA polymerase and plays an important role in the CA biosynthesis. When ΔwcaDE grown on solid M9 medium and stained with tannin mordant, blue mucoid exopolysaccharide was not observed around the cells (Figure 4), suggesting that the exopolysaccharide secreted by C. sakazakii BAA-894 cells grown on M9 is CA. E. coli Rcs phosphorelay system contains proteins encoded by the genes rcsA, rcsB, rcsC, rcsD, and rcsF. ESA_RS05720 (homolog of rcsA), ESA_RS04445 (homolog of rcsB), ESA_RS04440 (homolog of rcsC), ESA_RS04450 (homolog of rcsD), or ESA_RS14450 (homolog of rcsF) were individually deleted in C. sakazakii BAA-894, resulting in the mutant strains ΔrcsA, ΔrcsB, ΔrcsC, ΔrcsD, and ΔrcsF, respectively. When grown on solid M9 medium and stained with tannin mordant, no blueish mucoid CA was observed around cells of ΔrcsA, ΔrcsB, ΔrcsC, or ΔrcsD (Figure 4), suggesting that CA production in C. sakazakii cells is regulated by Rcs phosphorelay system. Interestingly, a small amount of bluish mucoid CA was observed around cells of ΔrcsF (Figure 4). Considering RcsF is the sensor of Rcs system, the results suggest that the production of CA in C. sakazakii cells is not 100% controlled by the Rcs system or there might be another protein which can function as RcsF.
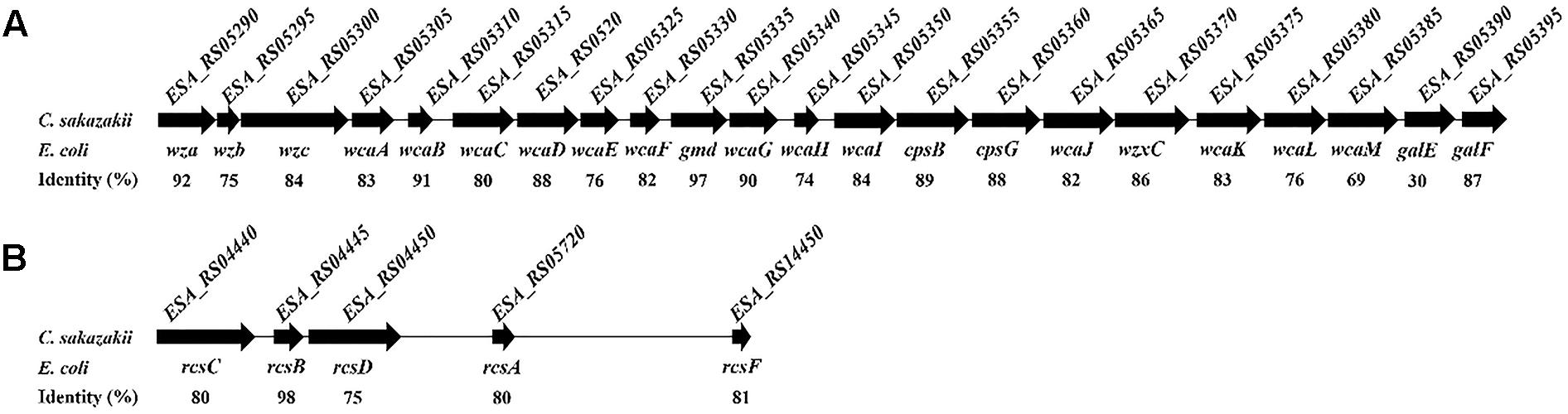
FIGURE 3. Homolog genes of Escherichia coli wca cluster (A) and Rcs phosphorelay system (B) exist in C. sakazakii BAA-894.
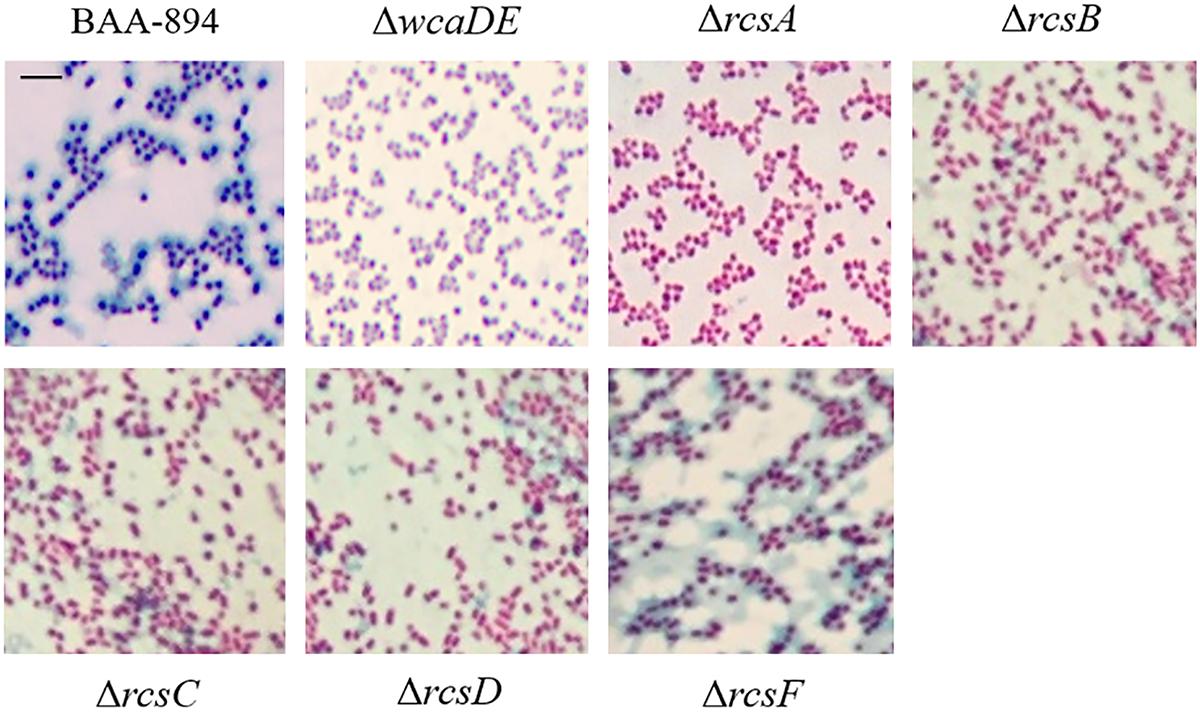
FIGURE 4. Light microscopic observation of wild type BAA-894 and various C. sakazakii mutants grown on M9 solid media and stained with mordant. Scale bar, 10 μm.
Colanic Acid Production in C. sakazakii BAA-894 Is Dependent on the Availability of Amino Acids
Since amino acids are not contained in M9, C. sakazakii BAA-894 cells were also grown on solid M9 medium supplemented with 0.5 mM or 5 mM of 15 important amino acids (Asp, Glu, Ser, His, Gly, Thr, Arg, Ala, Cys, Val, Met, Phe, Ile, Leu, and Lys), and the colonies were stained with tannin mordant and observed under microscopy (Figure 5A). Compared to BAA-894 cells grown on M9, much less bluish substance was observed around BAA-894 cells grown on M9 supplemented with 0.5 mM amino acids, but no bluish substance was observed around BAA-894 cells grown on M9 supplemented with 5 mM amino acids (Figure 5A). This indicates that CA production in C. sakazakii BAA-894 is dependent on the availability of amino acids.
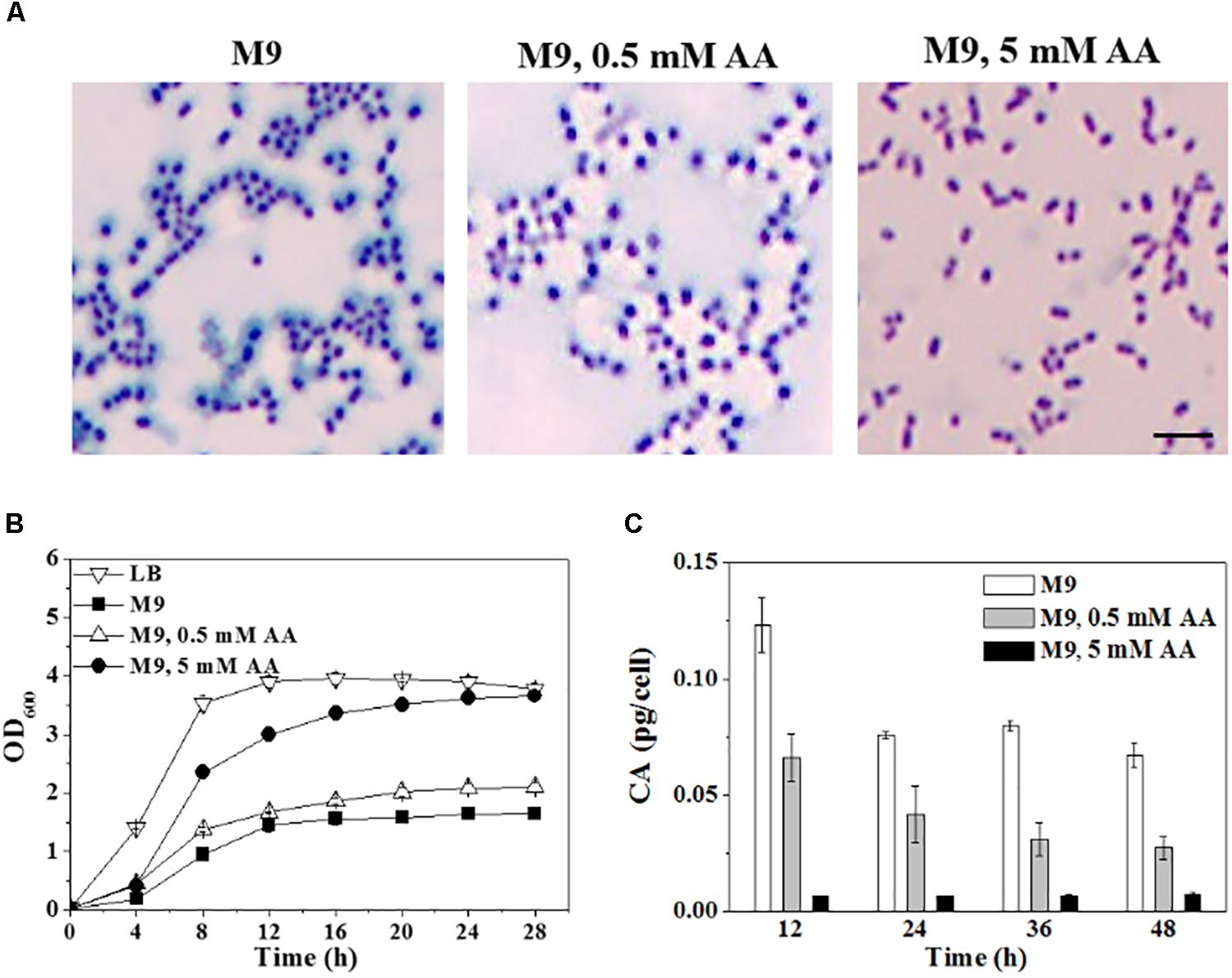
FIGURE 5. (A) Light microscopic observation of BAA-894 grown on media of M9, M9 containing 0.5 mM or 5 mM amino acids (AA) and stained with mordant. Scale bar, 10 μm. (B) Growth comparison of C. sakazakii BAA-894 cells grown in media of M9, M9 containing 0.5 mM or 5 mM AA. (C) Quantification of colanic acid produced in C. sakazakii BAA-894 grown in different liquid media.
To confirm whether amino acid availability is the major factor for the slow growth of bacteria in M9, C. sakazakii BAA-894 cells were grown in liquid M9 supplemented with the 15 amino acids. When 0.5 mM amino acids were added in M9, the cell growth slightly improved (Figure 5B). When 5 mM amino acids were added in M9, the cell growth significantly improved, and its maximum OD600 reached 3.6, similar to the cells grown in LB (Figure 5B). The results suggest that C. sakazakii can quickly adapt to the environment without amino acids, and efficiently consume the amino acids when available.
Colanic acid production were also analyzed when C. sakazakii BAA-894 grown in liquid media of M9 and M9 supplemented with 0.5 mM or 5 mM amino acids (Figure 5C). Samples were collected at 12, 24, 36, and 48 h, and the CA levels were determined. A significant amount of CA was detected in cells grown in M9, and a small amount of CA was detected in cells grown in M9 supplemented with 0.5 mM amino acids; however, only a negligible amount of CA was detected in cells grown in M9 supplemented with 5 mM amino acids. Colanic acid production is closely related to the cell growth of bacteria. The worst the cells grow; the more CA be produced.
Since amino acid availability is important for the cell growth and CA production in C. sakazakii, the levels of the 15 important amino acids produced by E. coli MG1655 grown in M9, C. sakazakii BAA-894 grown in M9 and LB were analyzed. M9 medium contains no amino acids, C. sakazakii BAA-894 grown in M9 medium accumulated a large amount of Asp and Val (Figure 6A), while E. coli MG1655 grown in M9 accumulated a large amount of Glu (Figure 6B). This indicates that the response mechanism of C. sakazakii and E. coli to amino acid deficiency might be quite different. The LB medium contains all the 15 amino acids, except for Cys; the top four high content amino acids (about 1–2 mM) are Leu, Lys, Glu, and Ala. When grown in LB, C. sakazakii BAA-894 consumed all Glu, Ser, Gly, Thr, Arg, and Ala in 12 h, but accumulated Asp, His, Val, Met, Phe, Ile, Leu, and Lys for 48 h (Figure 6C). This indicates that priority for amino acid synthesis and accumulation in C. sakazakii depend on the growth condition.
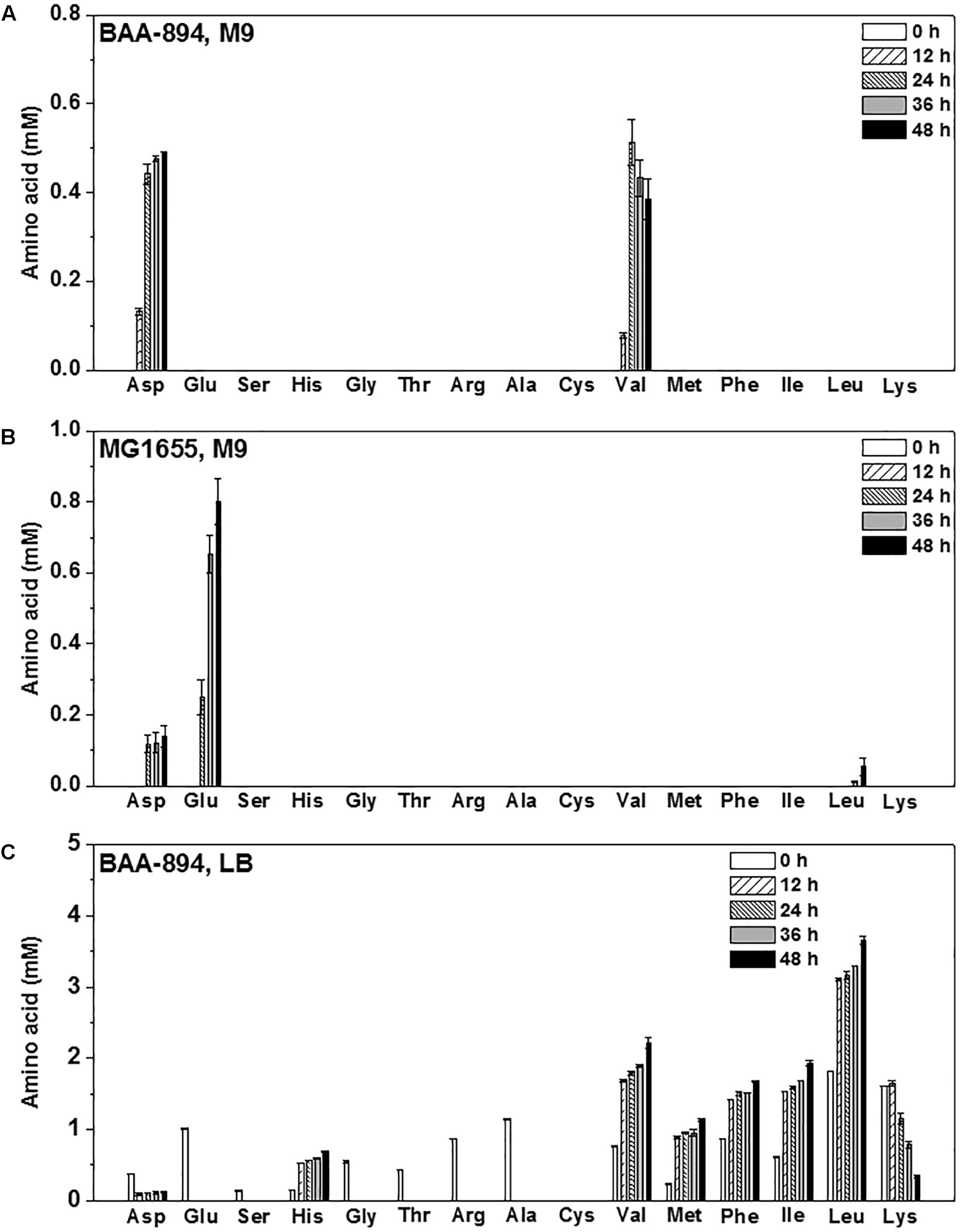
FIGURE 6. Levels of 15 amino acids in the culture of C. sakazakii BAA-894 grown in M9 (A), E. coli MG1655 grown in M9 (B), and BAA-894 grown in LB (C) medium at different time points.
Antibiotic Resistance of Cronobacter sakazakii BAA-894 Depends on the Growth Medium
Antibiotic therapy is the common way to prevent the Cronobacter infection in humans. Therefore, the resistance of C. sakazakii BAA-894 grown in different media to 16 antibiotics was evaluated with an antibiotic disk diffusion assay (Figure 7). C. sakazakii BAA-894 grown in M9 displayed sensitivity to at least 12 antibiotics. Interestingly, the resistance patterns for the 16 antibiotics for C. sakazakii BAA-894 grown in M9 (Figure 7A) are the same for C. sakazakii grown in M9 supplemented with 5 mM AA (Figure 7B), suggesting that amino acid availability does not affect the antibiotic resistance of C. sakazakii grown in M9. C. sakazakii BAA-894 grown in LB displayed resistance to at least 10 antibiotics (Figure 7C). C. sakazakii BAA-894 cells were very sensitive to norfloxacin no matter they were grown on LB or M9. C. sakazakii BAA-894 cells grown on M9 were also very sensitive to chloromycetin, tetracycline, ampicillin and amoxicillin. Norfloxacin can interfere with DNA synthesis by inhibiting bacterial DNA gyrase. Ampicillin and amoxicillin can inhibit the synthesis of the bacterial cell wall. Tetracycline can bind to the 30S subunit of ribosome, thereby inhibiting protein synthesis in bacteria. Generally, C. sakazakii BAA-894 was less sensitive to antibiotics when grown in LB than in M9 or M9 supplemented with 5 mM AA, possibly because its growth rate was better in LB than in M9 (Figure 5B). The bacterial cell fitness might play an important role for antibiotic resistance.
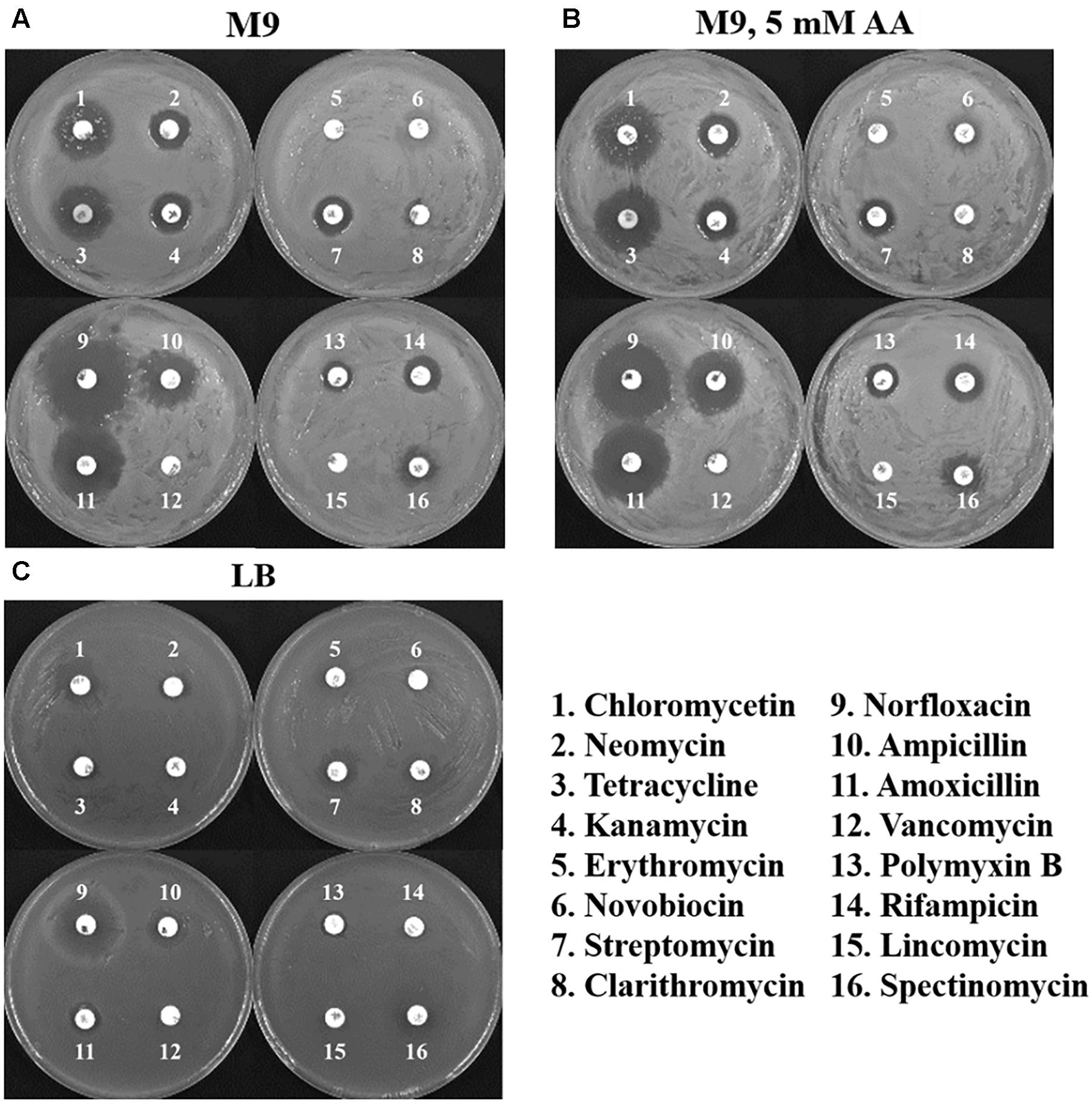
FIGURE 7. Disk diffusion assays of C. sakazakii BAA-894 cells grown in the medium of M9 (A), M9 containing 5 mM AA (B), and LB (C).
When Cronobacter sakazakii BAA-894 Was Grown in M9, Genes Relevant to Exopolysaccharide Biosynthesis Were Significantly Up-Regulated, but Genes Relevant to Flagellum Biosynthesis and Chemotaxis Were Significantly Down-Regulated
To investigate the response of C. sakazakii BAA-894 to amino acid deficiency, total RNAs isolated from BAA-894 grown in LB or M9 media were analyzed. The total number of detected genes from BAA-894 grown in M9 and LB media were 3998 and 3981, respectively. Comparing the BAA-894 cells grown in LB, 2339 genes were up-regulated and 1617 genes were down-regulated in BAA-894 cells grown in M9, and the number of significantly modulated genes was 1263 (785 up-regulated and 478 down-regulated) (Table 3 and Supplementary Table S1).
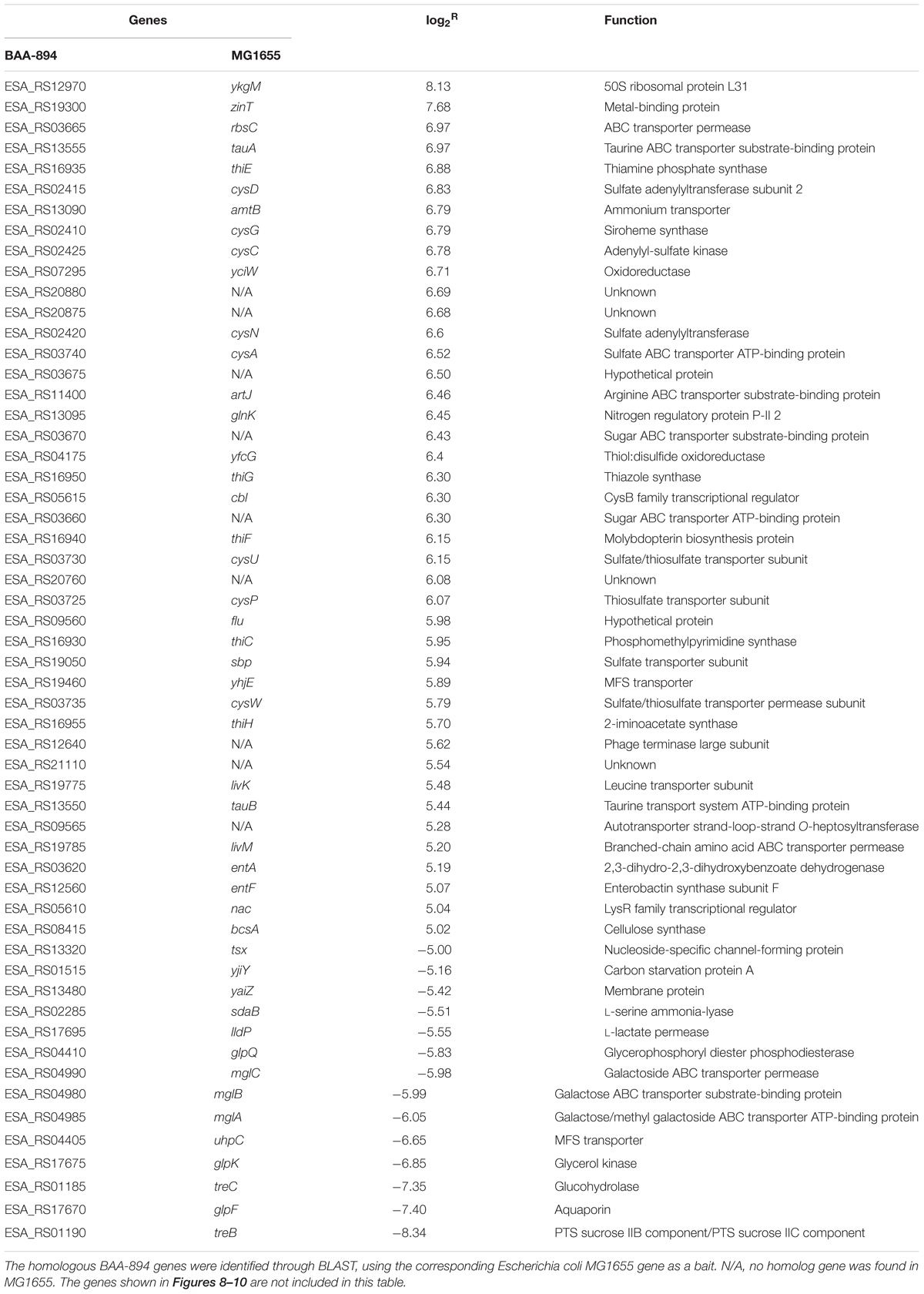
TABLE 3. List of the significantly regulated genes (| log2R|≥ 5) in Cronobacter sakazakii BAA-894 cells grown in M9 medium, using the same strain grown in LB medium as the control.
When grown in M9 medium, the transcriptional levels of 19 homolog genes involved in the biosynthesis of CA in E. coli were significantly up-regulated in C. sakazakii BAA-894 (Figure 8A). These transcriptomic analysis results were further confirmed by using RT-PCR analysis (Figure 8B). This suggests that the transcriptomic analysis used in this study is reliable. Interestingly, RT-PCR analysis showed that the transcriptional levels of some key genes involved in exopolysaccharide biosynthesis in C. sakazakii BAA-894 grown in M9 medium were similar to those grown in M9 with 5 mM amino acids (Figure 8C). The yjbEFGH operon is involved in the production of another exopolysaccharide in E. coli (Ferrieres et al., 2007). When grown in M9 medium, the transcriptional levels of the four homolog genes in the yjbEFGH operon were also significantly up-regulated in C. sakazakii BAA-894 (Figure 8A). The results are consistent with the observation of exopolysaccharide around cells of C. sakazakii BAA-894 grown in M9 as shown in Figure 1.
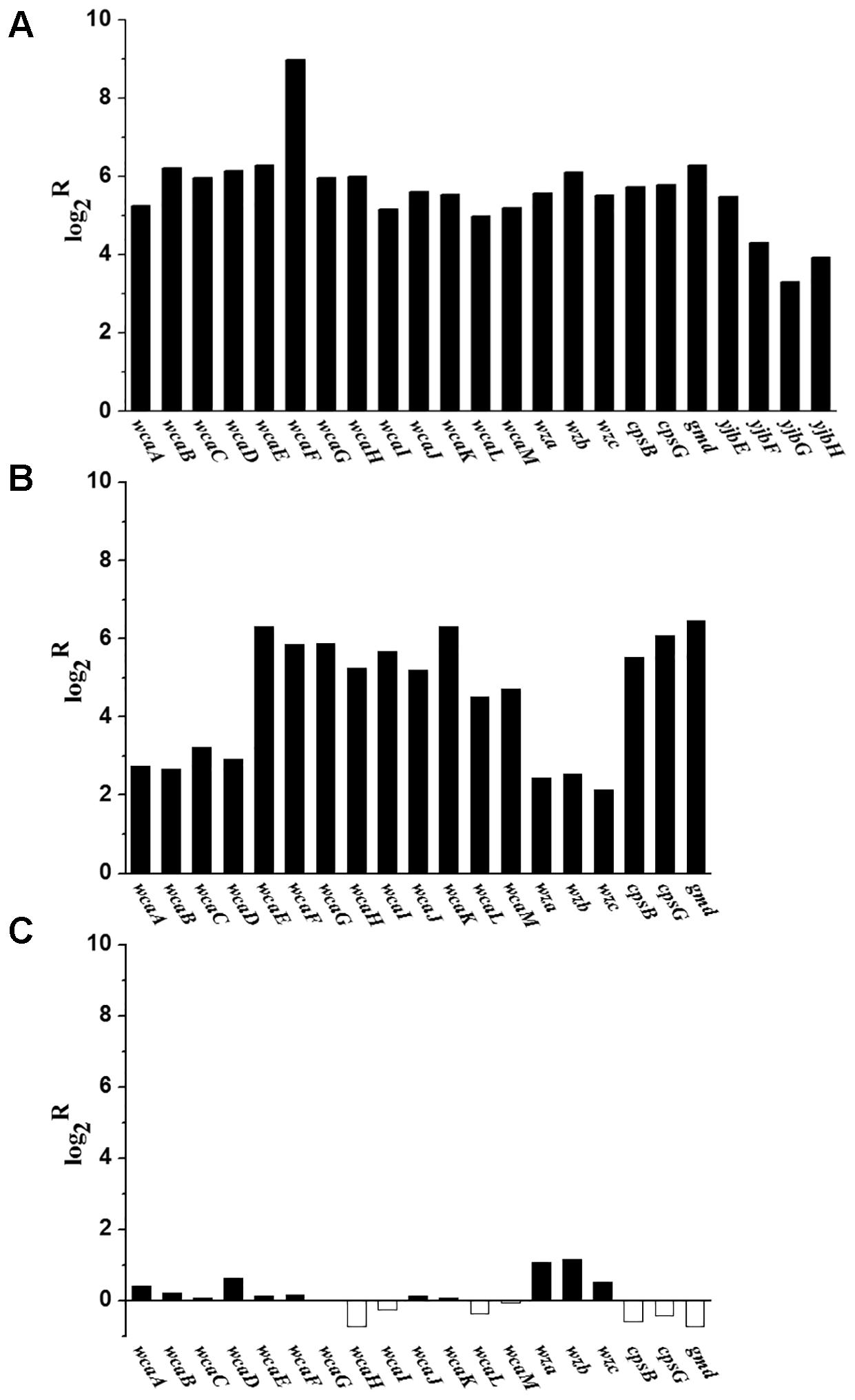
FIGURE 8. (A) Transcriptomic analysis showed that most genes involved in exopolysaccharide biosynthesis were up-regulated in C. sakazakii BAA-894 grown in M9 medium, using the ones grown in LB as the control. (B) RT-PCR analysis for transcriptional levels of some key genes involved in exopolysaccharide biosynthesis in C. sakazakii BAA-894 grown in M9 medium, using the ones grown in LB as the control. (C) RT-PCR analysis for transcriptional levels of some key genes involved in exopolysaccharide biosynthesis in C. sakazakii BAA-894 grown in M9 medium, using the ones grown in M9 with 5 mM amino acids as the control.
Flagellum is an accessory structure that protrudes from the bacterial cells, its primary role is locomotion, but it also functions as a sensory organelle to sense chemicals outside the cell (Silflow and Lefebvre, 2001; Bardy et al., 2003; Wang et al., 2005). When grown in M9 medium, the transcriptional levels of 35 genes relevant to flagellar biosynthesis and 11 genes relevant to chemotaxes were significantly down-regulated in C. sakazakii BAA-894 (Figure 9A). Similar transcriptional levels of some key genes relevant to flagellar biosynthesis were also observed by using RT-PCR analysis (Figure 9B). This suggests that the transcriptomic analysis used in this study is reliable. RT-PCR analysis also showed that the transcriptional levels of some key genes relevant to flagellar biosynthesis in C. sakazakii BAA-894 grown in M9 medium were down-regulated when compared to those grown in M9 with 5 mM amino acids (Figure 9C). This suggests that flagella might not be synthesized in C. sakazakii grown in M9 medium.
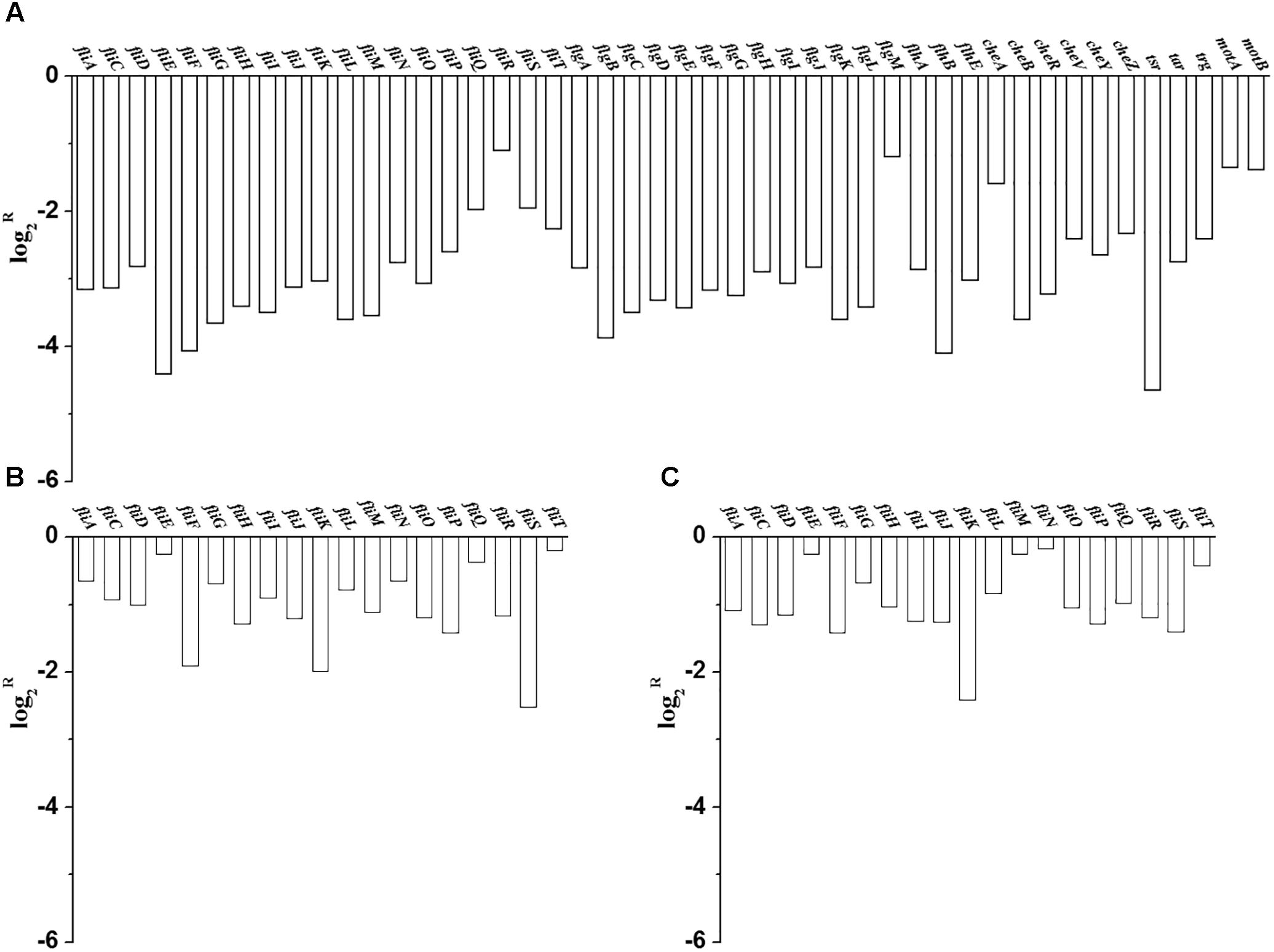
FIGURE 9. (A) Transcriptomic analysis showed that most genes involved in flagellum formation and chemotaxis were down-regulated in C. sakazakii BAA-894 grown in M9 medium. (B). RT-PCR analysis for transcriptional levels of some key genes involved in flagellum formation in C. sakazakii BAA-894 grown in M9 medium, using the ones grown in LB as the control. (C) RT-PCR analysis for transcriptional levels of some key genes involved in flagellum formation in C. sakazakii BAA-894 grown in M9 medium, using the ones grown in M9 with 5 mM amino acids as the control.
Many Genes Relevant to the Biosynthesis of Various Amino Acids Were Significantly Regulated in Cronobacter sakazakii BAA-894 Grown in M9 Medium
When C. sakazakii BAA-894 cells were grown in M9 medium, genes sucC, sdhA, sdhB, sdhC, sdhD, and fumA were down-regulated, suggesting that the TCA cycle was weakened (Figure 10). The carbon flowed out from oxaloacetate, α-ketoglutarate and pyruvate to increase the production of various amino acids (Figure 10).
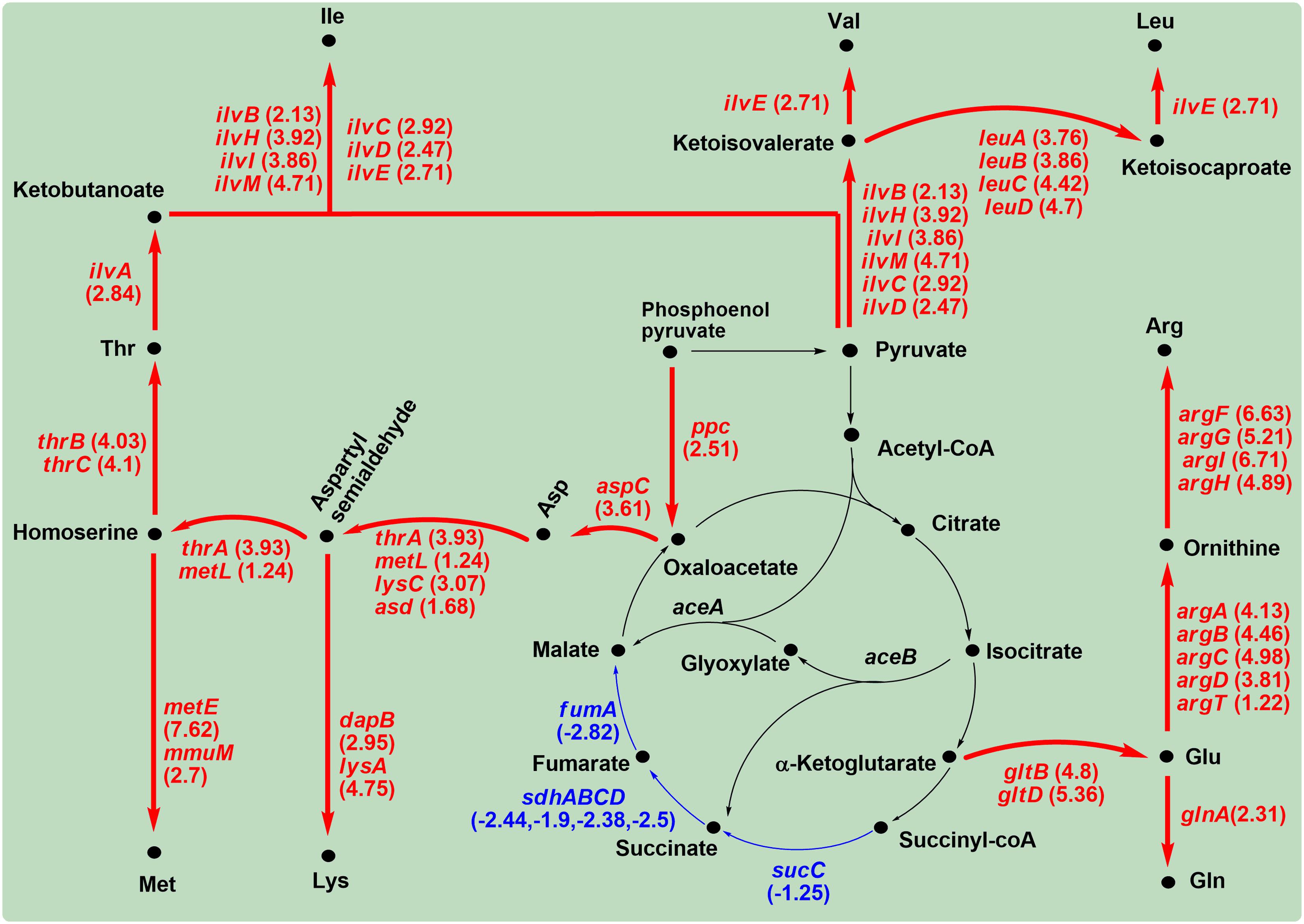
FIGURE 10. Significantly modulated genes involved in amino acid regulation in C. sakazakii BAA-894 grown in M9 medium. The up-regulated genes are shown in red, and the down-regulated genes shown in blue.
The up-regulated ppc and aspC directed the carbon flow from phosphoenol pyruvate to Asp. This is consistent with the high accumulation of Asp in C. sakazakii grown in M9 medium (Figure 6A). The up-regulated thrA, metL, lysC, asd, thrB, and thrC further convert Asp to Thr (Dong et al., 2011). Ile biosynthesis from Thr is a five-step pathway that shares its last four steps with the Val biosynthesis. The first step is catalyzed by IlvA; the second step can be catalyzed by each of the three enzyme pairs, IlvG-1/IlvM, IlvI/IlvH, and IlvB/IlvN; the last three steps are catalyzed by IlvC, IlvD, and IlvE, respectively. The key genes (ilvA, ilvB, ilvC, ilvD, ilvE, ilvH, ilvI, ilvM) encoding the enzymes for the five-step reactions were significantly up-regulated in C. sakazakii BAA-894 grown in M9 (Figure 10). Leu biosynthesis involves a five-step process starting from ketoisovalerate, and is catalyzed by LeuA, LeuB, LeuC, LeuD, and IlvE, respectively. The genes (leuA, leuB, leuC, leuD, ilvE) encoding these enzymes were also significantly up-regulated in C. sakazakii BAA-894 grown in M9 (Figure 10). LeuO is a Leu transcriptional activator. The ABC transporter complex LivFGHMJ can transport Leu, Ile, or Val, while the complex LivFGHMK is specific for transporting Leu. The genes (livF, livG, livH, livJ, livK, livM, and leuO) encoding these enzymes were significantly up-regulated in C. sakazakii BAA-894 grown in M9 medium (Table 3). The data suggest that the biosynthesis of Leu, Ile, and Val were enhanced in C. sakazakii in response to an extremely low concentration of amino acids in M9 medium. The biosynthesis of Lys and Met might also be enhanced, considering they also use Asp as the precursor. LysA catalyzes the last reaction for the biosynthesis of Lys; the transcriptional level of lysA was significantly up-regulated (Log2R = 4.75) in C. sakazakii BAA-894 grown in M9 medium, and another key gene dapB in the Lys biosynthetic pathway was also up-regulated. MetE and MmuM catalyze the last reaction for the biosynthesis of Met; the transcriptional level of metE (Log2R = 7.62) and mmuM (Log2R = 2.7) was significantly up-regulated in C. sakazakii BAA-894 grown in M9 medium (Figure 10).
In E. coli, Glu provides nitrogen for other amino acids such as Asp, His, and Arg (Reitzer, 2003). Glu can also be synthesized from α-ketoglutarate by Glu synthase composed of GltB and GltD (Castaño et al., 1992). GlnA catalyzes the reaction of Glu and ammonia to generate glutamine Gln. When C. sakazakii BAA-894 was grown in M9 medium, the transcriptional levels of glnA, gltB, and gltD were significantly up-regulated (Figure 10). Glu can also be converted into L-ornithine by enzymes ArgA, ArgB, ArgC, ArgD, and ArgT, and L-ornithine can be further converted to Arg by enzymes ArgF, ArgI, ArgG, and ArgH. When C. sakazakii BAA-894 was grown in M9 medium, the transcriptional levels of argA, argB, argC, argD, argF, argI, argG, argH, and argT were significantly up-regulated (Figure 10).
TrpA, TrpD, and TrpE are key enzymes in the biosynthetic pathway of L-tryptophan. When grown in M9 medium, the transcriptional levels of trpA, trpD, and trpE were significantly up-regulated in C. sakazakii BAA-894 (Table 3). His biosynthesis is carried out by enzymes HisA, HisB, HisC, HisD, HisF, HisG, HisH, and HisI. HisFH catalyzes the fifth step of His biosynthesis. When grown in M9 medium, the transcriptional levels of hisA, hisB, hisC, hisD, hisG, hisH, and hisI were significantly up-regulated in C. sakazakii BAA-894 (Table 3). These data suggest that the L-tryptophan and His biosynthesis were enhanced in C. sakazakii grown in M9.
Discussion
Bacteria can survive in various environments by modulating their intracellular metabolism and coordinating uptake of the primary substrates for biomass production (Doucette et al., 2011). The genes relevant to flagellum formation and chemotaxis were significantly down-regulated in C. sakazakii BAA-894 grown in the amino acid deficient medium M9 (Figure 9). The amino acid content is not the only variable in M9 and LB; another variable is glucose which is contained in M9 medium but not in LB. Glucose represses flagellar synthesis via cyclic-AMP, and the repression of flagellar genes in M9 can be attributed to glucose, and not differences in amino acid content. The lower expression of genes emrA (log2R = -1.43), emrD (log2R = -1.84), emrE (log2R = -1.57), and emrR (log2R = -1.34) encoding the multidrug resistance pumps C. sakazakii BAA-894 grown in M9 might also be attributable to glucose, considering the increased antibiotic sensitivity of C. sakazakii BAA-894 grown in M9 does not change after supplemented with 5 mM amino acids (Figure 7).
Because of the amino acid deficiency and the high glucose concentration in M9, the ratio of intracellular carbon to nitrogen might be much higher in M9 than in LB; the redundant carbon source in C. sakazakii might be used for synthesizing exopolysaccharide (Figure 1) to protect cells against environmental stress. Based on the transcriptomic analysis, the genes relevant to CA biosynthesis were significantly up-regulated (Figure 8). At least four types of exopolysaccharides (CA, an exopolysaccharide produced by the yjbEFGH operon, K-antigen, and cellulose) can be produced in C. sakazakii (Ferrieres et al., 2007; Ogrodzki and Forsythe, 2017). When C. sakazakii BAA-894 cells were grown in M9, all 19 genes relevant to CA production and all 4 genes in the yjbEFGH operon were significantly up-regulated, whereas only one gene kpsS (Log2R = 1.75) relevant to K-antigen production was slightly up-regulated and one gene bcsA (Log2R = 5.02) relevant to cellulose production were significantly up-regulated. Our experiments demonstrate that CA is the major exopolysaccharide produced by C. sakazakii grown in M9, its production is dependent on the availability of amino acids and is regulated by the Rcs phosphorelay system; the detailed mechanism remains to be determined.
Amino acids are the most important nitrogen source for bacteria, their availability can lead to a coordinated regulation of metabolism. In E. coli, nitrogen regulation two-component system NtrB and NtrC are encoded by glnL and glnG, respectively (Zimmer et al., 2000), and nitrogen starvation results in the phosphorylation of the response regulator NtrC and the activation of RelA (Kuroda et al., 1999). RelA is responsible for synthesizing the alarmone ppGpp which causes a massive re-programming of the transcriptional profile known as the stringent response (Rombel et al., 1998; Wendrich et al., 2002; Shyp et al., 2012; Schumacher et al., 2013; Brown et al., 2014). In E. coli, the nitrogen stress response allows cells to rapidly sense nitrogen limitation, scavenge for alternative nitrogen sources through the transcriptional activation of transport systems and catabolic and biosynthetic operons, and adapt to nitrogen limitation. However, in C. sakazakii BAA-894 when grown in M9, though glnG and glnL were significantly up-regulated, the transcriptional level of relA did not change, suggesting that the nitrogen stress response did not occur in C. sakazakii cells. In fact, M9 medium contains 0.1% NH4Cl (18 mM), although it is amino acid deficient, it should be nitrogen rich. In E. coli, the phosphatase and kinase activities of NtrB are regulated by GlnB and GlnK; GlnK is tightly regulated under nitrogen-rich conditions, but expressed during ammonium starvation (Gosztolai et al., 2017). In C. sakazakii grown in M9, glnK (Log2R = 6.45) and amtB (Log2R = 6.79) encoding an ammonium transporter AmtB were significantly up-regulated. This suggests that the response to amino acid deficiency in C. sakazakii is different from that in E. coli. GlnK provides a functional link between nitrogen and carbon metabolisms (van Heeswijk et al., 2013; Gosztolai et al., 2017), therefore, the overexpression of AmtB and GlnK might balance the ratio of carbon to nitrogen for the benefit of C. sakazakii under amino acid deficiency.
Since M9 medium contains no amino acids, C. sakazakii grown in M9 must synthesize the necessary amino acids for cell growth. The transcriptional levels of most genes related to biosynthesis of various amino acids were significantly regulated in C. sakazakii BAA-894 grown in M9 (Figure 10). This indicates that balancing the amount of various amino acids is the major task for C. sakazakii to grow under the condition of amino acid deficiency. With respect to the amino acid accumulation in the medium, Glu and Asp accumulation in E. coli makes sense; because the concentration of Glu is high, and reversible transamination elevates Asp. The surprising result is that Asp and Val are high in C. sakazakii; Asp is high possibly because of high phosphoenol pyruvate, and Val is high possibly because of high pyruvate (Figure 10). These differences suggest that the response mechanism to amino acid deficiency in C. sakazakii is quite different from that in E. coli.
Conclusion
Cronobacter species can cause necrotizing enterocolitis and meningitis. Their responses to extreme growth conditions could provide important information on their infection mechanism. This study investigated the response of C. sakazakii to amino acid deficiency. C. sakazakii produced CA when grown in the amino acid deficient M9 but not in the amino acid rich LB media; CA production is regulated by the Rcs phosphorelay system and depends on the availability of amino acids. Transcriptomes C. sakazakii BAA-894 grown in M9 or LB showed that 3956 genes were differentially expressed. When C. sakazakii BAA-894 was grown in M9, the genes relevant to the biosynthesis of CA were significantly up-regulated, but the genes relevant to the flagellum formation and chemotaxis were significantly down-regulated; most genes relevant to various amino acid biosynthesis were also significantly regulated. The results demonstrate that amino acid deficiency has a global impact on C. sakazakii cells. Since CA, flagella and chemotaxis are associated with pathogenesis of C. sakazakii, the data on C. sakazakii responses to amino acid deficiency might provide information for better control the infection.
Author Contributions
SC, YL, and XW conceived and designed the experiments. SC, QZ, XT, and GR performed the experiments. SC and XW analyzed the data. XW and YL contributed reagents, materials, and analysis tools. SC and XW wrote the paper.
Funding
This study was supported by the National Key R&D Program of China (2017YFC1600102), the National First-Class Discipline Program of Light Industry Technology and Engineering (LITE2018-10), and the Collaborative Innovation Center of Jiangsu Modern Industrial Fermentation.
Conflict of Interest Statement
The authors declare that the research was conducted in the absence of any commercial or financial relationships that could be construed as a potential conflict of interest.
Supplementary Material
The Supplementary Material for this article can be found online at: https://www.frontiersin.org/articles/10.3389/fmicb.2018.01875/full#supplementary-material
Footnotes
- ^http://www.kegg.jp/kegg/kegg2.html
- ^http://www.geneontology.org/
- ^http://search.cpan.org/dist/GO-TermFinder/
References
Alvarez-Ordóñez, A., Cummins, C., Deasy, T., Clifford, T., Begley, M., and Hill, C. (2014). Acid stress management by Cronobacter sakazakii. Int. J. Food Microbiol. 178, 21–28. doi: 10.1016/j.ijfoodmicro.2014.03.001
Ball, C. A., Awad, I. A., Demeter, J., Gollub, J., Hebert, J. M., Hernandez-Boussard, T., et al. (2005). The stanford microarray database accommodates additional microarray platforms and data formats. Nucleic Acids Res. 33, D580–D582. doi: 10.1093/nar/gki006
Bardy, S. L., Ng, S. Y., and Jarrell, K. F. (2003). Prokaryotic motility structures. Microbiology 149(Pt 2), 295–304. doi: 10.1099/mic.0.25948-0
Blumenkrantz, N., and Asboe-Hansen, G. (1973). New method for quantitative determination of uronic acids. Anal. Biochem. 54, 484–489. doi: 10.1016/0003-2697(73)90377-1
Brown, D. R., Barton, G., Pan, Z., Buck, M., and Wigneshweraraj, S. (2014). Nitrogen stress response and stringent response are coupled in Escherichia coli. Nat. Commun. 5:4115. doi: 10.1038/ncomms5115
Cai, L., Li, Y., Tao, G., Guo, W., Zhang, C., and Wang, X. (2013). Identification of three genes encoding for the late acyltransferases of lipid A in Cronobacter sakazakii. Mar Drugs 11, 377–386. doi: 10.3390/md11020377
Castaño, I., Flores, N., Valle, F., Covarrubias, A. A., and Bolivar, F. (1992). gltF, a member of the gltBDF operon of Escherichia coli, is involved in nitrogen-regulated gene expression. Mol. Microbiol. 6, 2733–2741. doi: 10.1111/j.1365-2958.1992.tb01450.x
Dancer, G. I., Mah, J. H., Rhee, M. S., Hwang, I. G., and Kang, D. H. (2009). Resistance of Enterobacter sakazakii (Cronobacter spp.) to environmental stresses. J. Appl. Microbiol. 107, 1606–1614. doi: 10.1111/j.1365-2672.2009.04347.x
Datsenko, K. A., and Wanner, B. L. (2000). One-step inactivation of chromosomal genes in Escherichia coli K-12 using PCR products. Proc. Natl. Acad. Sci. U.S.A. 97, 6640–6645. doi: 10.1073/pnas.120163297
Dong, X., Quinn, P. J., and Wang, X. (2011). Metabolic engineering of Escherichia coli and Corynebacterium glutamicum for the production of L-threonine. Biotechnol. Adv. 29, 11–23. doi: 10.1016/j.biotechadv.2010.07.009
Doucette, C. D., Schwab, D. J., Wingreen, N. S., and Rabinowitz, J. D. (2011). alpha-Ketoglutarate coordinates carbon and nitrogen utilization via enzyme I inhibition. Nat. Chem. Biol. 7, 894–901. doi: 10.1038/nchembio.685
Feeney, A., Kropp, K. A., O’Connor, R., and Sleator, R. D. (2014). Cronobacter sakazakii: stress survival and virulence potential in an opportunistic foodborne pathogen. Gut Microbes 5, 711–718. doi: 10.4161/19490976.2014.983774
Ferrieres, L., Aslam, S. N., Cooper, R. M., and Clarke, D. J. (2007). The yjbEFGH locus in Escherichia coli K-12 is an operon encoding proteins involved in exopolysaccharide production. Microbiology 153(Pt 4), 1070–1080. doi: 10.1099/mic.0.2006/002907-0
Forsythe, S. J. (2017). Updates on the Cronobacter genus. Annu. Rev. Food Sci. Technol. 9, 2.1–2.22. doi: 10.1146/annurev-food-030117012246
Gosztolai, A., Schumacher, J., Behrends, V., Bundy, J. G., Heydenreich, F., Bennett, M. H., et al. (2017). GlnK facilitates the dynamic regulation of bacterial nitrogen assimilation. Biophys. J. 112, 2219–2230. doi: 10.1101/127662
Han, Y., Li, Y., Chen, J., Tan, Y., Guan, F., and Wang, X. (2013). Construction of monophosphoryl lipid A producing Escherichia coli mutants and comparison of immuno-stimulatory activities of their lipopolysaccharides. Mar Drugs 11, 363–376. doi: 10.3390/md11020363
Jung, J. H., Choi, N. Y., and Lee, S. Y. (2013). Biofilm formation and exopolysaccharide (EPS) production by Cronobacter sakazakii depending on environmental conditions. Food Microbiol. 34, 70–80. doi: 10.1016/j.fm.2012.11.008
Kanehisa, M., Araki, M., Goto, S., Hattori, M., Hirakawa, M., Itoh, M., et al. (2008). KEGG for linking genomes to life and the environment. Nucleic Acids Res. 36, D480–D484. doi: 10.1093/nar/gkm882
Kim, K. I., and van de Wiel, M. A. (2008). Effects of dependence in high-dimensional multiple testing problems. BMC Bioinformatics 9:114. doi: 10.1186/1471-2105-9-114
Kuroda, A., Tanaka, S., Ikeda, T., Kato, J., Takiguchi, N., and Ohtake, H. (1999). Inorganic polyphosphate kinase is required to stimulate protein degradation and for adaptation to amino acid starvation in Escherichia coli. Proc. Natl. Acad. Sci. U.S.A. 96, 14264–14269. doi: 10.1073/pnas.96.25.14264
Li, Y., Yu, H., Jiang, H., Jiao, Y., Zhang, Y., and Shao, J. (2017). Genetic diversity, antimicrobial susceptibility, and biofilm formation of Cronobacter spp. Recovered from Spices and Cereals. Front. Microbiol. 8:2567. doi: 10.3389/fmicb.2017.02567
Liang, X., Hu, X., Wang, X., Wang, J., Fang, Y., and Li, Y. (2017). Characterization of the phosphate-specific transport system in Cronobacter sakazakii BAA-894. J. Appl. Microbiol. 123, 710–723. doi: 10.1111/jam.13524
Liu, L., Li, Y., Wang, X., and Guo, W. (2016). A phosphoethanolamine transferase specific for the 4’-phosphate residue of Cronobacter sakazakii lipid A. J. Appl. Microbiol. 121, 1444–1456. doi: 10.1111/jam.13280
Livak, K. J., and Schmittgen, T. D. (2001). Analysis of relative gene expression data using real-time quantitative PCR and the 2(-Delta Delta C(T)) Method. Methods 25, 402–408. doi: 10.1006/meth.2001.1262
Majdalani, N., and Gottesman, S. (2005). The RCS phosphorelay: a complex signal transduction system. Annu. Rev. Microbiol. 59, 379–405.
Marisch, K., Bayer, K., Scharl, T., Mairhofer, J., Krempl, P. M., Hummel, K., et al. (2013). A comparative analysis of industrial Escherichia coli K-12 and B strains in high-glucose batch cultivations on process-, transcriptome- and proteome level. PLoS One 8:e70516. doi: 10.1371/journal.pone.0070516
Mortazavi, A., Williams, B. A., McCue, K., Schaeffer, L., and Wold, B. (2008). Mapping and quantifying mammalian transcriptomes by RNA-Seq. Nat. Methods 5, 621–628. doi: 10.1038/nmeth.1226
Navasa, N., Rodriguez-Aparicio, L., Martinez-Blanco, H., Arcos, M., and Ferrero, M. A. (2009). Temperature has reciprocal effects on colanic acid and polysialic acid biosynthesis in E. coli K92. Appl. Microbiol. Biot. 82, 721–729. doi: 10.1007/s00253-008-1840-4
Ogrodzki, P., and Forsythe, S. (2015). Capsular profiling of the Cronobacter genus and the association of specific Cronobacter sakazakii and C. malonaticus capsule types with neonatal meningitis and necrotizing enterocolitis. BMC Genomics 16:758. doi: 10.1186/s12864-015-1960-z
Ogrodzki, P., and Forsythe, S. J. (2017). DNA-Sequence Based Typing of the Cronobacter Genus Using MLST. CRISPR-cas array and capsular profiling. Front. Microbiol. 8:1875. doi: 10.3389/fmicb.2017.01875
Podkovyrov, S. M., and Larson, T. J. (1996). Identification of promoter and stringent regulation of transcription of the fabH, fabD and fabG genes encoding fatty acid biosynthetic enzymes of Escherichia coli. Nucleic Acids Res. 24, 1747–1752. doi: 10.1093/nar/24.9.1747
Reitzer, L. (2003). Nitrogen assimilation and global regulation in Escherichia coli. Annu. Rev. Microbiol. 57, 155–176. doi: 10.1146/annurev.micro.57.030502.090820
Ren, G., Wang, Z., Li, Y., Hu, X., and Wang, X. (2016). Effects of Lipopolysaccharide Core sugar deficiency on colanic acid biosynthesis in Escherichia coli. J. Bacteriol. 198, 1576–1584.
Rombel, I., North, A., Hwang, I., Wyman, C., and Kustu, S. (1998). The bacterial enhancer-binding protein NtrC as a molecular machine. Cold. Spring Harb. Symp. Quant. Biol. 63, 157–166. doi: 10.1101/sqb.1998.63.157
Schumacher, J., Behrends, V., Pan, Z., Brown, D. R., Heydenreich, F., Lewis, M. R., et al. (2013). Nitrogen and carbon status are integrated at the transcriptional level by the nitrogen regulator NtrC in vivo. mBio 4:e881–e813. doi: 10.1128/mBio.00881-13
Serra-Moreno, R., Acosta, S., Hernalsteens, J. P., Jofre, J., and Muniesa, M. (2006). Use of the lambda Red recombinase system to produce recombinant prophages carrying antibiotic resistance genes. BMC Mol. Biol. 7:31. doi: 10.1186/1471-2199-7-31
Shyp, V., Tankov, S., Ermakov, A., Kudrin, P., English, B. P., Ehrenberg, M., et al. (2012). Positive allosteric feedback regulation of the stringent response enzyme RelA by its product. EMBO Rep. 13, 835–839. doi: 10.1038/embor.2012.106
Silflow, C. D., and Lefebvre, P. A. (2001). Assembly and motility of eukaryotic cilia and flagella. Lessons from Chlamydomonas reinhardtii. Plant Physiol. 127, 1500–1507. doi: 10.1104/pp.010807
Stevenson, G., Andrianopoulos, K., Hobbs, M., and Reeves, P. (1996). Organization of the Escherichia coli K-12 gene cluster responsible for production of the extracellular polysaccharide colanic acid. J. Bacteriol. 178, 4885–4893. doi: 10.1128/jb.178.16.4885-4893.1996
Sutherland, I. W. (1969). Structural studies on colanic acid, the common exopolysaccharide found in the Enterobacteriaceae, by partial acid hydrolysis, Oligosaccharides from colanic acid. Biochem. J. 115, 935–945. doi: 10.1042/bj1150935
van Heeswijk, W. C., Westerhoff, H. V., and Boogerd, F. C. (2013). Nitrogen assimilation in Escherichia coli: putting molecular data into a systems perspective. Microbiol. Mol. Biol. Rev. 77, 628–695. doi: 10.1128/MMBR.00025-13
Wang, Q., Suzuki, A., Mariconda, S., Porwollik, S., and Harshey, R. M. (2005). Sensing wetness: a new role for the bacterial flagellum. EMBO J. 24, 2034–2042. doi: 10.1038/sj.emboj.7600668
Wang, X., and Quinn, P. J. (2010). Endotoxins: lipopolysaccharides of gram-negative bacteria. Subcell Biochem. 53, 3–25. doi: 10.1007/978-90-481-9078-2_1
Wang, X., Quinn, P. J., and Yan, A. (2015). Kdo2 -lipid a: structural diversity and impact on immunopharmacology. Biol. Rev. Camb. Philos. Soc. 90, 408–427. doi: 10.1111/brv.12114
Wendrich, T., Blaha, G., Wilson, D., and Marahiel, M. (2002). Dissection of the mechanism for the stringent factor RelA. Mol. Cell. 10, 779–788. doi: 10.1016/S1097-2765(02)00656-1
Wiederschain, G. Y. (2011). Endotoxins: structure, function and recognition. Biochemistry (Moscow) 76, 606–606. doi: 10.1134/s0006297911050129
Zhang, C., Li, Y., Tao, G., Li, Y., and Wang, X. (2010). Characterization of lipid A Cronobacter sakazakii. Eur. J. Mass Spectrom. (Chichester) 16, 531–538. doi: 10.1255/ejms.1074
Zhang, W., Hu, X., Wang, L., and Wang, X. (2014). Reconstruction of the carotenoid biosynthetic pathway of Cronobacter sakazakii BAA894 in Escherichia coli. PLoS One 9:e86739. doi: 10.1371/journal.pone.0086739
Zimmer, D. P., Soupene, E., Lee, H. L., Wendisch, V. F., Khodursky, A. B., Peter, B. J., et al. (2000). Nitrogen regulatory protein C-controlled genes of Escherichia coli: scavenging as a defense against nitrogen limitation. Proc. Natl. Acad. Sci. U.S.A. 97, 14674–14679. doi: 10.1073/pnas.97.26.14674
Keywords: Cronobacter sakazakii, colanic acids, exopolysaccharide, amino acid deficiency, transcriptomic analysis
Citation: Chen S, Zhou Q, Tan X, Li Y, Ren G and Wang X (2018) The Global Response of Cronobacter sakazakii Cells to Amino Acid Deficiency. Front. Microbiol. 9:1875. doi: 10.3389/fmicb.2018.01875
Received: 12 May 2018; Accepted: 25 July 2018;
Published: 14 August 2018.
Edited by:
Julio Parra-Flores, University of the Bío Bío, ChileReviewed by:
Paola Sperandeo, Università degli Studi di Milano, ItalyShu-Sin Chng, National University of Singapore, Singapore
Ariadnna Cruz-Córdova, Hospital Infantil de México Federico Gómez, Mexico
Copyright © 2018 Chen, Zhou, Tan, Li, Ren and Wang. This is an open-access article distributed under the terms of the Creative Commons Attribution License (CC BY). The use, distribution or reproduction in other forums is permitted, provided the original author(s) and the copyright owner(s) are credited and that the original publication in this journal is cited, in accordance with accepted academic practice. No use, distribution or reproduction is permitted which does not comply with these terms.
*Correspondence: Xiaoyuan Wang, eHdhbmdAamlhbmduYW4uZWR1LmNu