- 1Department of Microbiology, The Chinese University of Hong Kong, Shatin, Hong Kong
- 2Department of Microbiology, Faculty of Medicine, University of Peradeniya, Kandy, Sri Lanka
- 3Department of Anesthesiology and Critical Care, Faculty of Medicine, University of Peradeniya, Kandy, Sri Lanka
Limited data is available on the epidemiology and characteristics of carbapenem-resistant Enterobacteriaceae (CRE) and their associated plasmids or virulence determinants from Sri Lanka. Through whole genome sequencing of CREs from the intensive care units of a Sri Lankan teaching hospital, we identified a carbapenemase gene, blaOXA–181 in 10 carbapenemase-producing Klebsiella pneumoniae isolates (two strains of ST437 and eight strains of ST147) from 379 respiratory specimens. blaOXA–181 was carried in three variants of ColE-type plasmids. K. pneumoniae strains with ompK36 variants showed high minimum inhibitory concentrations to carbapenem. Furthermore, genes encoding for extended spectrum β-lactamases (ESBL), plasmid-mediated quinolone resistance (PMQR) determinants (qnr, aac(6′)-Ib-cr, and oqxAB) were present in all 10 strains. Amino acid substitution in chromosomal quinolone resistance-determining regions (QRDRs) gyrA (Ser83Ile) and parC (Ser80Ile) were also observed. All strains had yersiniabactin genes on mobile element ICEkp. Strict infection control practices and judicious use of antibiotics are warranted to prevent further spread of multidrug-resistant K. pneumoniae.
Introduction
Carbapenem-resistant Enterobacteriaceae (CRE) is a global threat and infections caused by CRE are associated with high morbidity and mortality (Centers for Disease Control and Prevention, 2013). Among all the resistance mechanisms, plasmid-mediated horizontal transfer of carbapenemase genes is the main route for acquiring resistance in CRE (Nordmann et al., 2012). These mobile elements are capable of transferring resistance between different lineages and thus, pose a potential for dissemination. Three types of carbapenemases (class A: blaKPC; class B: blaNDM, blaIMP, and blaV IM; class D: blaOXA-48-like) can hydrolyze carbapenems at varying levels along with other mechanisms, such as porin mutation and overexpression of efflux pump proteins (Poirel et al., 2012; Zhang et al., 2014; Lunha et al., 2016). Klebsiella pneumoniae clonal complex 258 (CC258) have been known to associate with epidemic plasmids carrying numerous antimicrobial resistance genes and virulence factors like yersiniabactin. These interactions were hypothesized to provide a survival advantage for these clones (Holt et al., 2015). Sri Lanka sits in the southern tip of the Indian subcontinent. Although the epidemiology and diversity of carbapenemases, have been reported in India, the epidemiology and characteristics of the CRE and resistance determinants in Sri Lanka is lacking. Furthermore, the single study currently available on CRE from Sri Lanka does not contain information on associated plasmids or virulence determinants (Hall et al., 2014). Hence, we sequenced the CRE strains isolated from patient specimens in the intensive care units of one Sri Lankan hospital using whole genome sequencing (WGS) to describe their antimicrobial resistance and genetic profiles so as to provide a more in-depth view of the CRE in Sri Lanka.
Materials and Methods
Bacterial Isolates
Single patient isolates were obtained from the respiratory specimens received from inpatients admitted to the intensive care units of the Teaching Hospital, Peradeniya, Sri Lanka between February to September 2015. During this period, a total of 379 respiratory specimens were processed and 64 coliforms were obtained. Among these coliforms, 2.6% (10 isolates) were found to be resistant to carbapenems using Stokes sensitivity testing method (Sri Lanka College of Microbiologists, 2011) and were saved for further study. The study was approved by the Institutional Ethical Committee of the Faculty of Medicine, University of Peradeniya.
Bacterial Identification and Antimicrobial Resistance
Bacterial strains were plated on blood agar and incubated at 37°C overnight and the identification of all strains were confirmed by matrix-assisted laser desorption ionization-time of flight (MALDI-TOF) mass spectrometry at the Department of Microbiology, the Chinese University of Hong Kong. Minimum inhibitory concentrations (MICs) of antimicrobials were determined by the microbroth dilution method according to Clinical and Laboratory Standards Institute (CLSI) guideline (CLSI, 2018). The following antibiotics were tested: amikacin (AK); ceftazidime (CAZ); ciprofloxacin (CIP); gentamicin (GN); colistin (CT); cefotaxime (CTX); ertapenem (ETP); fosfomycin (FOS); imipenem (IPM); meropenem (MEM); and tigecycline (TG).
Whole Genome Sequencing and Data Analysis
Bacterial DNA was extracted with Wizard genomic DNA purification kit (Promega, Madison, WI, United States). WGS was performed using the Illumina HiSeq 2500 platform, and unique index-tagged libraries were created for each sample to generate 90 bp paired-end reads (Global Biologics, LLC). The libraries gave 100× average coverage for each strain. Quality control of the raw reads was performed by FastQC (Andrews, 2010). Genomes were assembled using SPAdes assembler (version 3.5.0) (Bankevich et al., 2012). Contigs of ≥500 bp from each genome were included in the analyses. Prokka (version 1.9) software was used for genome annotation, including ORF finding and gene function annotation (Seemann, 2014). Raw reads and assembled contigs were used for multilocus sequence typing (MLST) analysis. SRST2 (Version 0.1.5) was used to map raw reads to the following databases: pubMLST database for sequence types; ARG-ANNOT V3 database for resistance genes; PlasmidFinder database (Updated 20170220) for plasmid replicons; and K. pneumoniae BIGSdb virulence gene database for virulence genes at http://bigsdb.web.pasteur.fr (Accession date: 20180313) (Carattoli et al., 2014; Gupta et al., 2014; Inouye et al., 2014). The contig containing carbapenemase for each genome was matched against the public database with NCBI BLAST to find the top hit plasmids of at least 95% identity and 95% coverage and were matched back to the plasmids in our genome dataset to extract possible plasmid contigs (Altschul et al., 1990). Extracted contigs were further aligned to the reference plasmid using Mauve Alignment software to check for similarity and coverage (Darling et al., 2004). Gaps were filled by PCR with primers designed from our genome data. Pan-genome dendrogram was created by Roary (Page et al., 2015). Contig files of all 10 strains in this study were deposited to GenBank BioProject under the accession number PRJNA439172.
Results
Antimicrobial Susceptibilities and Molecular Characteristics of the CRE Isolates
Of the 10 CRE isolates included in the study, three were from the subsidiary ICU and seven were from the main ICU. All strains were confirmed K. pneumoniae and the susceptibility testing revealed all strains to be resistant to CIP, CTX, and sensitive to FOS and AK, while MIC for CT was ≤0.5 mg/L and MIC for TG was ≤2 mg/L (Table 1). All except one strain (SL50) were resistant to CAZ. All of the strains except SL33, SL49, and SL54 were resistant to ETP or MEM with MICs ≥16 and ≥8 mg/L, respectively, while resistance to IPM varied among isolates. In previous studies, mutations of K. pneumoniae outer membrane proteins ompK35 and ompK36 were found to confer increased MIC to carbapenems (Garcia-Fernandez et al., 2010; Zhang et al., 2014; Lunha et al., 2016). There were neither mutations nor insertions at ompK35 and its promoter in all of our strains when we aligned them to the wild-type gene in public database (GenBank Accession No. AJ011501). OmpK36 was identical in six highly resistant strains (ompK36-sl1). SL33 and SL49 shared the same gene (ompK36-sl2), but SL54 contained a unique variation (ompK36-sl3). The difference of ompK36-sl1 and ompK36-sl2 was the insertion of amino acids glycine and aspartate after PEFXG domain within the L3 loop and one mutation in loop L4 and alpha-helix, respectively. The PEFXG domain (porin size determinant) insertion was also observed in several studies that addressed strains with high resistance to carbapenems (Garcia-Fernandez et al., 2010; Hall et al., 2014; Zhang et al., 2014). OmpK36-sl3 was different from the others in the region between loop L3 and loop L6, but there was no insertion interruption in the PEFXG domain.
The K. pneumoniae isolates belonged to ST437 (2 strains) and ST147 (8 strains) (Table 1). Capsular genes showed two wzi alleles 64 and 109 in strains ST147 and ST437, respectively (Figure 1). Only one carbapenemase gene, blaOXA–181 was found in all isolates. All isolates harbored β-lactamase genes, including blaTEM-1, and blaOXA-1, blaSHV -11, and to extended-β-lactamase, blaCTX-M-15. Plasmid-mediated quinolone resistance (PMQR) determinants (qnr, aac(6′)-Ib-cr, and oqxAB) were also detected. We also found two amino acid substitutions at gyrA (S83I) and parC (S80I) in all strains, which have been frequently reported in quinolone-resistant K. pneumoniae worldwide (Aldred et al., 2014).
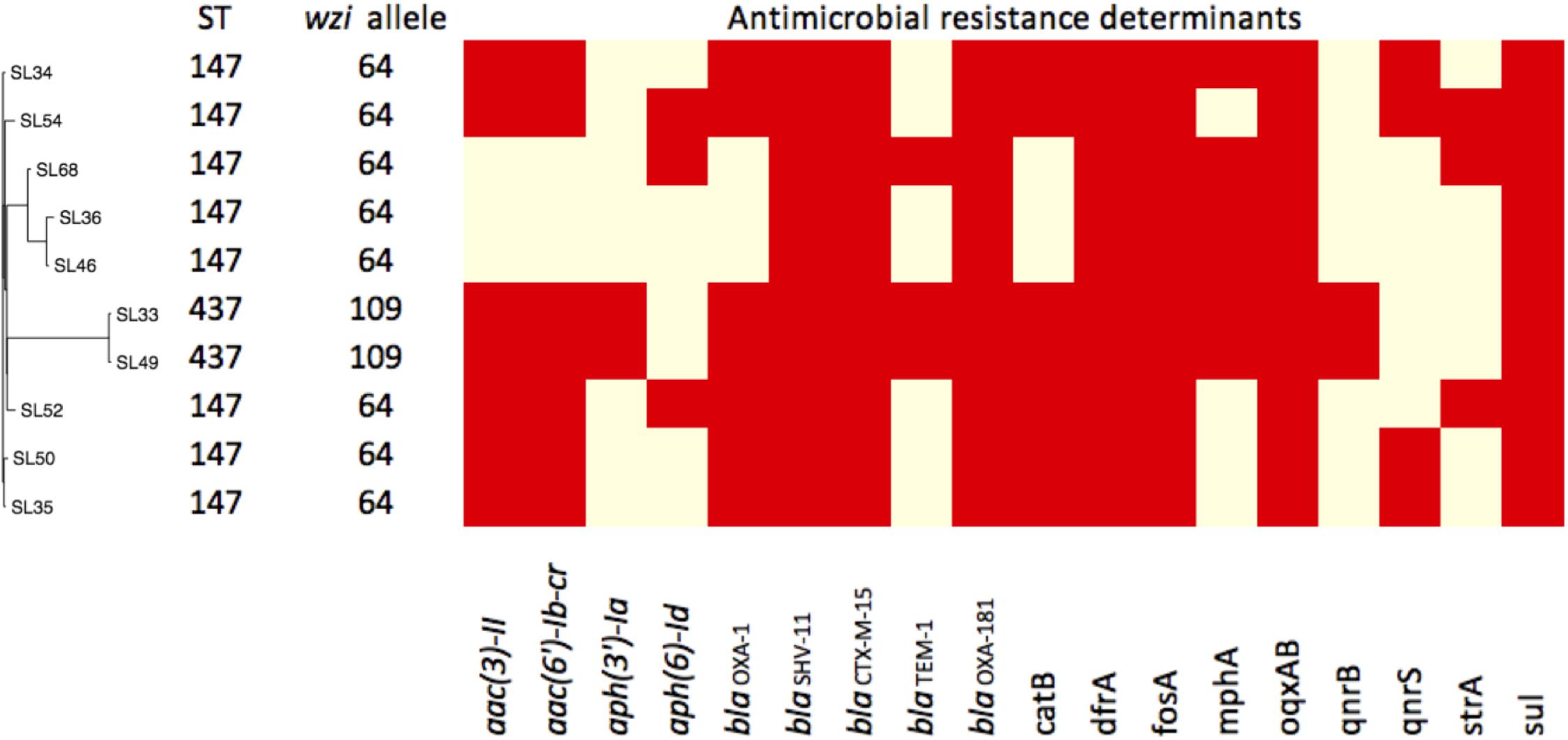
FIGURE 1. Pan-genome dendrogram of 10 Sri Lanka strains with annotation. ∗Red boxes indicate presence and yellow for absence.
The virulence profiles among the 10 strains were the same and all carried yersiniabactin genes (ybt, irp1, irp2, and fuyA) and kfu, mrk, but were absent of rmpA or rmpA2 genes. Yersiniabactin genes were found on mobile element ICEkp. 4437 genes and 2182 accessory genes were used to establish the dendrogram of their pan-genomes (Figure 1). The distinct accessory gene profiles of ST437 and ST147 indicated that ST437 was a distant cluster. The pan-genome dendrogram also revealed a cluster of ST147 (SL36, SL46, and SL68) that contained fewer resistance genes (Figure 1) and were sensitive to GN and with lower MIC (8 mg/L) to CIP compared with other ST147 strains (Table 1).
Plasmids Harboring blaOXA–181
Three different ColE-type plasmids were identified (Figure 2). One plasmid (CUHK_SL-A) was identical to KP3 (GenBank accession no. JN205800) and was found in the two ST437 strains. CUHK_SL-A was a short plasmid (7,606 bp) harboring only one resistance gene and was previously well described (Potron et al., 2011a). Another plasmid (CUHK_SL-B) was found in 7 ST147 strains of this study with a deletion of an insertion sequence (ISEcp1) when compared to CUHK_SL-A. Several studies from the United States, Germany, and France have reported this plasmid in both K. pneumoniae and Escherichia coli (GenBank Accession No. CP006802, CP016038, and JX423831). The third plasmid (CUHK_SL-C) was found in strain SL54 with a ∗∗mobile gene deletion (mobC) when compared to CUHK_SL-B. This plasmid was not reported before.
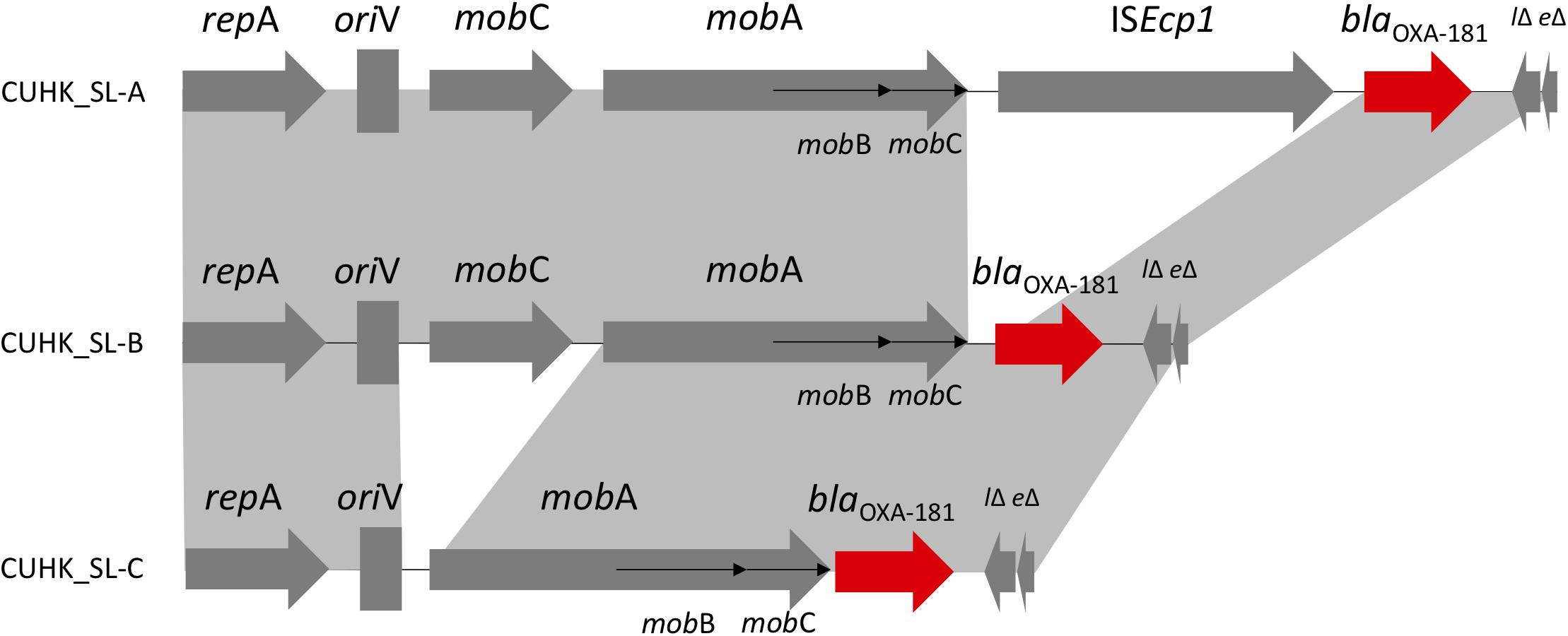
FIGURE 2. ColE-type plasmids in Sri Lanka. ∗CUHK_SL-B had ISEcp1 deletion; CUHK_SL-C had ISEcp1 and mobC deletion.
Discussion
Carbapenemase blaOXA–181 was first described in India as a Class D blaOXA-48-like enzyme from clinical samples in year 2006 and 2007 (Castanheira et al., 2011). It was thought to originate from an environmental strain as a chromosomal gene (Potron et al., 2011b). Although it has been detected worldwide, most of the patients have a travel history to the Indian subcontinent, especially India (Poirel et al., 2012). In 2014, blaOXA–181 and blaNDM were reported in K. pneumoniae in Sri Lanka, and included mainly ST14 and ST147 (Hall et al., 2014). ST437 belongs to CC258 and is a single locus variant of the globally prevalent ST258, carrying blaKPC in Brazil and blaNDM, blaOXA-245 (plasmid: IncL/M) in Spain (Seki et al., 2011; Oteo et al., 2013). blaOXA–181 was previously described in plasmid ColE, IncT and in the chromosome, which were all isolated from patients transferred from India, as well as described in plasmid IncX3 from China (Potron et al., 2011a; Dimou et al., 2012; Villa et al., 2013; Kayama et al., 2015; Liu et al., 2015; Partridge et al., 2015). ColE plasmid encoding various β-lactamase genes and blaOXA–181 is related to transposon Tn2013. Insertion sequence ISEcp1 was considered to be associated with blaOXA–181 acquisition, and its deletion may stabilize the resistance gene in the plasmid (Potron et al., 2013). mobC gene deletion in the strains may affect the frequency of conjugal plasmid mobilization (Zhang and Meyer, 1997).
In this study, all strains harbored quinolone-resistant determinants with quinolone resistance-determining region (QRDR) mutation on their chromosomes. A recent epidemiology study has shown the correlation of quinolone consumption and CRE in United States military health system, and another case-control study of CRE outbreaks in the Netherlands have determined quinolone use as the only risk factor for the acquisition of blaOXA-48-like producing Enterobacteriaceae compared to other antibiotics use (Lesho et al., 2015; Dautzenberg et al., 2016). Possible mechanisms may be due to co-transfer of two plasmids bearing dual resistance mechanisms or recombination into one plasmid as described before (Rodríguez Martínez et al., 2014; Liu et al., 2015). Furthermore, the impact of different plasmids and fluoroquinolone resistant determinants on fitness cost has been found to vary with strains of different STs. This may have contributed to the spread of this particular clone (Tóth et al., 2014; Johnson et al., 2015). It was suggested that energetically favorable mutations with the double serine mutations in the QRDR region in the current study have been described to favor the fitness and dissemination of such clones (Fuzi et al., 2017). Although all strains were non-hypervirulent K. pneumoniae (negative for rmpA/rmpA2 genes, non K1/K2 capsule serotypes), all strains encoded several yersiniabactin genes. These genes being on the integrative conjugative elements (ICEKp) can contribute to the spread of K. pneumoniae in the population and may serve as a predictor of invasive infection (Lam et al., 2018).
In conclusion, we explored the genetic profile of multidrug resistant CRE in Sri Lanka. blaOXA–181 (through ColE-type plasmid) and yersiniabactin genes have disseminated to different STs of K. pneumoniae. We recommend active surveillance of high risk inpatients and long term studies to determine possible intra-unit transfer and facilitate infection control, especially as the length of stay in all instances where data was available is >72 h. Judicious antibiotics use especially that of quinolones is also recommended.
Author Contributions
VP and ND conceptualized, collected, preliminarily analyzed, and approved the manuscript. MI, MH, and VL conceptualized, conducted, analyzed, drafted, and approved the manuscript. CZ, NL, KW, and CL contributed to sample storage and preparation, molecular analysis, and approval of the manuscript. CZ prepared the first draft of the manuscript.
Funding
This research was supported by Health and Medical Research Fund, Food and Health Bureau of the Hong Kong Special Administrative Region to PI: MI, Grant no: CU-15-B4 and CU-16-C8.
Conflict of Interest Statement
The authors declare that the research was conducted in the absence of any commercial or financial relationships that could be construed as a potential conflict of interest.
Acknowledgments
We thank the Food and Health Bureau of Hong Kong Special Administrative Region for the support from Health and Medical Research Commissioned Fund.
References
Aldred, K. J., Kerns, R. J., and Osheroff, N. (2014). Mechanism of quinolone action and resistance. Biochemistry 53, 1565–1574. doi: 10.1021/bi5000564
Altschul, S. F., Gish, W., Miller, W., Myers, E. W., and Lipman, D. J. (1990). Basic local alignment search tool. J. Mol. Biol. 215, 403–410. doi: 10.1016/S0022-2836(05)80360-2
Andrews, S. (2010). FastQC: A Quality Control Tool for High Throughput Sequence Data. Available at: http://www.bioinformatics.babraham.ac.uk/projects/fastqc/
Bankevich, A., Nurk, S., Antipov, D., Gurevich, A. A., Dvorkin, M., Kulikov, A. S., et al. (2012). SPAdes: a new genome assembly algorithm and its applications to single-cell sequencing. J. Comput. Biol. 19, 455–477. doi: 10.1089/cmb.2012.0021
Carattoli, A., Zankari, E., García-Fernández, A., Voldby Larsen, M., Lund, O., Villa, L., et al. (2014). In silico detection and typing of plasmids using plasmidfinder and plasmid multilocus sequence typing. antimicrob. Agents Chemother. 58, 3895–3903. doi: 10.1128/AAC.02412-14
Castanheira, M., Deshpande, L. M., Mathai, D., Bell, J. M., Jones, R. N., and Mendes, R. E. (2011). Early dissemination of NDM-1- and OXA-181-producing enterobacteriaceae in indian hospitals: report from the SENTRY antimicrobial surveillance program, 2006-2007. Antimicrob. Agents Chemother. 55, 1274–1278. doi: 10.1128/AAC.01497-10
Centers for Disease Control and Prevention (2013). Antibiotic Resistance Threats in the United States, 2013. Atlanta, GA: Centers for Disease Control and Prevention. Available at: www.cdc.gov/drugresistance/threat-report-2013
CLSI (2018). Performance Standards for Antimicrobial Susceptibility Testing. CLSI supplement M100, 28th Edn. Wayne, PA: Clinical and Laboratory Standards Institute.
Darling, A. C. E., Mau, B., Blattner, F. R., and Perna, N. T. (2004). Mauve: multiple alignment of conserved genomic sequence with rearrangements. Genome Res. 14, 1394–403. doi: 10.1101/gr.2289704
Dautzenberg, M. J. D., Ossewaarde, J. M., de Greeff, S. C., Troelstra, A., and Bonten, M. J. M. (2016). Risk factors for the acquisition of OXA-48-producing Enterobacteriaceae in a hospital outbreak setting: a matched case-control study. J. Antimicrob. Chemother. 71, 2273–2279. doi: 10.1093/jac/dkw119
Dimou, V., Dhanji, H., Pike, R., Livermore, D. M., and Woodford, N. (2012). Characterization of enterobacteriaceae producing OXA-48-like carbapenemases in the UK. J. Antimicrob. Chemother. 67, 1660–1665. doi: 10.1093/jac/dks124
Fuzi, M., Szabo, D., and Csercsik, R. (2017). Double-serine fluoroquinolone resistance mutations advance major international clones and lineages of various multi-drug resistant bacteria. Front. Microbiol. 8:2261. doi: 10.3389/fmicb.2017.02261
Garcia-Fernandez, A., Miriagou, V., Papagiannitsis, C. C., Giordano, A., Venditti, M., Mancini, C., et al. (2010). An ertapenem-resistant extended-spectrum- -lactamase-producing Klebsiella pneumoniae clone carries a novel OmpK36 porin variant. Antimicrob. Agents Chemother. 54, 4178–4184. doi: 10.1128/AAC.01301-09
Gupta, S. K., Padmanabhan, B. R., Diene, S. M., Lopez-Rojas, R., Kempf, M., Landraud, L., et al. (2014). ARG-ANNOT, a new bioinformatic tool to discover antibiotic resistance genes in bacterial genomes. Antimicrob. Agents Chemother. 58, 212–20. doi: 10.1128/AAC.01310-13
Hall, J. M., Corea, E., Anusha Sanjeewani, H. D., and Inglis, T. J. J. (2014). Molecular mechanisms of β-lactam resistance in carbapenemase-producing Klebsiella pneumoniae from Sri Lanka. J. Med. Microbiol. 63, 1087–1092. doi: 10.1099/jmm.0.076760-0
Holt, K. E., Wertheim, H., Zadoks, R. N., Baker, S., Whitehouse, C. A., Dance, D., et al. (2015). Genomic analysis of diversity, population structure, virulence, and antimicrobial resistance in Klebsiella pneumoniae, an urgent threat to public health. Proc. Natl. Acad. Sci. U.S.A. 112, E3574–E3581. doi: 10.1073/pnas.1501049112
Inouye, M., Dashnow, H., Raven, L.-A., Schultz, M. B., Pope, B. J., Tomita, T., et al. (2014). SRST2: rapid genomic surveillance for public health and hospital microbiology labs. Genome Med. 6:90. doi: 10.1186/s13073-014-0090-6
Johnson, J. R., Johnston, B., Kuskowski, M. A., Sokurenko, E. V., and Tchesnokova, V. (2015). Intensity and mechanisms of fluoroquinolone resistance within the H30 and H30Rx subclones of Escherichia coli sequence type 131 compared with other fluoroquinolone-resistant E. coli. Antimicrob. Agents Chemother. 59, 4471–4480. doi: 10.1128/AAC.00673-15
Kayama, S., Koba, Y., Shigemoto, N., Kuwahara, R., Kakuhama, T., Kimura, K., et al. (2015). Imipenem-susceptible, meropenem-resistant Klebsiella pneumoniae producing OXA-181 in Japan. Antimicrob. Agents Chemother. 59, 1379–1380. doi: 10.1128/AAC.04330-14
Lam, M. M. C., Wick, R. R., Wyres, K. L., Gorrie, C. L., Judd, L. M., Jenney, A. W. J., et al. (2018). Genetic diversity, mobilisation and spread of the yersiniabactin-encoding mobile element ICEKp in Klebsiella pneumoniae populations. Microb. Genom. doi: 10.1099/mgen.0.000196 [Epub ahead of print].
Lesho, E. P., Clifford, R. J., Chukwuma, U., Kwak, Y. I., Maneval, M., Neumann, C., et al. (2015). Carbapenem-resistant enterobacteriaceae and the correlation between carbapenem and fluoroquinolone usage and resistance in the US military health system. Diagn. Microbiol. Infect. Dis. 81, 119–125. doi: 10.1016/j.diagmicrobio.2014.09.017
Liu, Y., Feng, Y., Wu, W., Xie, Y., Wang, X., Zhang, X., et al. (2015). First report of OXA-181-producing Escherichia coli in China and characterization of the isolate using whole-genome sequencing. Antimicrob. Agents Chemother. 59, 5022–50255. doi: 10.1128/AAC.00442-15
Lunha, K., Chanawong, A., Lulitanond, A., Wilailuckana, C., Charoensri, N., Wonglakorn, L., et al. (2016). High-level carbapenem-resistant OXA-48-producing Klebsiella pneumoniae with a novel OmpK36 variant and low-level, carbapenem-resistant, non-porin-deficient, OXA-181-producing Escherichia coli from Thailand. Diagn. Microbiol. Infect. Dis. 85, 221–226. doi: 10.1016/j.diagmicrobio.2016.03.009
Nordmann, P., Dortet, L., and Poirel, L. (2012). Carbapenem resistance in Enterobacteriaceae: here is the storm! Trends Mol. Med. 18, 263–272. doi: 10.1016/j.molmed.2012.03.003
Oteo, J., Hernandez, J. M., Espasa, M., Fleites, A., Saez, D., Bautista, V., et al. (2013). Emergence of OXA-48-producing Klebsiella pneumoniae and the novel carbapenemases OXA-244 and OXA-245 in Spain. J. Antimicrob. Chemother. 68, 317–321. doi: 10.1093/jac/dks383
Page, A. J., Cummins, C. A., Hunt, M., Wong, V. K., Reuter, S., Holden, M. T. G., et al. (2015). Roary: rapid large-scale prokaryote pan genome analysis. Bioinformatics 31, 3691–3693. doi: 10.1093/bioinformatics/btv421
Partridge, S. R., Ginn, A. N., Wiklendt, A. M., Ellem, J., Wong, J. S. J., Ingram, P., et al. (2015). Emergence of blaKPC carbapenemase genes in Australia. Int. J. Antimicrob. Agents 45, 130–136. doi: 10.1016/j.ijantimicag.2014.10.006
Poirel, L., Potron, A., and Nordmann, P. (2012). OXA-48-like carbapenemases: the phantom menace. J. Antimicrob. Chemother. 67, 1597–1606. doi: 10.1093/jac/dks121
Potron, A., Nordmann, P., Lafeuille, E., Al Maskari, Z., Al Rashdi, F., and Poirel, L. (2011a). Characterization of OXA-181, a carbapenem-hydrolyzing class D -lactamase from Klebsiella pneumoniae. Antimicrob. Agents Chemother. 55, 4896–4899. doi: 10.1128/AAC.00481-11
Potron, A., Poirel, L., and Nordmann, P. (2011b). Origin of OXA-181, an emerging carbapenem-hydrolyzing oxacillinase, as a chromosomal gene in Shewanella xiamenensis. Antimicrob. Agents Chemother. 55, 4405–4407. doi: 10.1128/AAC.00681-11
Potron, A., Rondinaud, E., Poirel, L., Belmonte, O., Boyer, S., Camiade, S., et al. (2013). Genetic and biochemical characterisation of OXA-232, a carbapenem- hydrolysing class D β-lactamase from Enterobacteriaceae. Int. J. Antimicrob. Agents 41, 325–329. doi: 10.1016/j.ijantimicag.2012.11.007
Rodríguez Martínez, J. M., Díaz-De Alba, P., Cerero, L., Ruiz-Carrascoso, G., Gomez-Gil, R., and Pascual, A. (2014). Presence of quinolone resistance to qnrB1 genes and blaOXA-48 carbapenemase in clinical isolates of Klebsiella pneumoniae in Spain. Enferm. Infecc. Microbiol. Clin. 32, 441–442. doi: 10.1016/j.eimc.2014.02.013
Seemann, T. (2014). Prokka: rapid prokaryotic genome annotation. Bioinformatics 30, 2068–2069. doi: 10.1093/bioinformatics/btu153
Seki, L. M., Pereira, P. S., de Souza Mda, P., Conceição, M. de S., Marques, E. A., Porto, C. O., et al. (2011). Molecular epidemiology of KPC-2- producing Klebsiella pneumoniae isolates in Brazil: the predominance of sequence type 437. Diagn. Microbiol. Infect. Dis. 70, 274–277. doi: 10.1016/J.DIAGMICROBIO.2011.01.006
Sri Lanka College of Microbiologists (2011). Laboratory Manual in Microbiology, 2nd Edn. Colombo: Ananda Press.
Tóth,Á., Kocsis, B., Damjanova, I., Kristóf, K., Jánvári, L., Pászti, J., et al. (2014). Fitness cost associated with resistance to fluoroquinolones is diverse across clones of Klebsiella pneumoniae and may select for CTX-M-15 type extended-spectrum β-lactamase. Eur. J. Clin. Microbiol. Infect. Dis. 33, 837–843. doi: 10.1007/s10096-013-2022-6
Villa, L., Carattoli, A., Nordmann, P., Carta, C., and Poirel, L. (2013). Complete sequence of the IncT-type plasmid pT-OXA-181 carrying the bla OXA-181 carbapenemase gene from Citrobacter freundii. Antimicrob. Agents Chemother. 57, 1965–1967. doi: 10.1128/AAC.01297-12
Zhang, S., and Meyer, R. (1997). The relaxosome protein MobC promotes conjugal plasmid mobilization by extending DNA strand separation to the nick site at the origin of transfer. Mol. Microbiol. 25, 509–516. doi: 10.1046/j.1365-2958.1997.4861849.x
Keywords: OXA-181, quinolone, yersiniabactin, Klebsiella pneumoniae, Sri Lanka
Citation: Zhu C, Liyanapathirana V, Li C, Pinto V, Hui M, Lo N, Wong KT, Dissanayake N and Ip M (2018) Characterizing Mobilized Virulence Factors and Multidrug Resistance Genes in Carbapenemase-Producing Klebsiella pneumoniae in a Sri Lankan Hospital. Front. Microbiol. 9:2044. doi: 10.3389/fmicb.2018.02044
Received: 14 June 2018; Accepted: 13 August 2018;
Published: 31 August 2018.
Edited by:
Yi-Wei Tang, Memorial Sloan Kettering Cancer Center, United StatesReviewed by:
Miklos Fuzi, Semmelweis University, HungaryLiang Li, Los Angeles Biomedical Research Institute, United States
Copyright © 2018 Zhu, Liyanapathirana, Li, Pinto, Hui, Lo, Wong, Dissanayake and Ip. This is an open-access article distributed under the terms of the Creative Commons Attribution License (CC BY). The use, distribution or reproduction in other forums is permitted, provided the original author(s) and the copyright owner(s) are credited and that the original publication in this journal is cited, in accordance with accepted academic practice. No use, distribution or reproduction is permitted which does not comply with these terms.
*Correspondence: Margaret Ip, bWFyZ2FyZXRpcEBjdWhrLmVkdS5oaw==
†These authors have contributed equally to this work