- 1Institute of Postharvest Technology of Agricultural Products, College of Food Science, Fujian Agriculture and Forestry University, Fuzhou, China
- 2Food Science Program, Division of Food Systems and Bioengineering, University of Missouri, Columbia, MO, United States
Phomopsis longanae Chi is a major pathogenic fungus that infects harvested longan fruit. This study aimed to investigate the effects of P. longanae on reactive oxygen species (ROS) metabolism and its relation to the pericarp browning and disease development of harvested longan fruit during storage at 28∘C and 90% relative humidity. Results showed that compared to the control longans, P. longanae-inoculated longans displayed higher indexes of pericarp browning and fruit disease, higher O2-. generation rate, higher accumulation of malondialdehyde (MDA), lower contents of glutathione (GSH) and ascorbic acid (AsA), lower 1,1-diphenyl-2-picrylhydrazyl (DPPH) radical scavenging ability and reducing power in pericarp. In addition, P. longanae-infected longans exhibited higher activities of superoxide dismutase (SOD), catalase (CAT), and ascorbate peroxidase (APX) in the first 2 days of storage, and lower activities of SOD, CAT, and APX during storage day 2–5 than those in the control longans. These findings indicated that pericarp browning and disease development of P. longanae-infected longan fruit might be the result of the reducing ROS scavenging ability and the increasing O2-. generation rate, which might lead to the peroxidation of membrane lipid, the loss of compartmentalization in longan pericarp cells, and subsequently cause polyphenol oxidase (PPO) and peroxidase (POD) to contact with phenolic substrates which result in enzymatic browning of longan pericarp, as well as cause the decrease of disease resistance to P. longanae and stimulate disease development of harvested longan fruit.
Introduction
Longan (Dimocarpus longan Lour.) is a popular tropical fruit famous for its appealing flavor and abundant nutritional ingredients (Lin et al., 2001; Chen et al., 2015). However, harvested longan fruits deteriorate rapidly due to water loss, injury, energy deficiency, pathogen infection, or damage caused by reactive oxygen, resulting in pericarp browning, decline of fruit quality and rot (Holcroft et al., 2005). Pericarp browning is a vital factor affecting edible quality and commercial value of postharvest longan fruits seriously (Jiang et al., 2002). There are abundant phenolic compounds in longan pericarp tissue (Prasad et al., 2009; Yang et al., 2011). Researches indicated that pericarp browning of postharvest longan fruit was mainly due to the formation of browning substances resulting from enzymatic browning (Wang et al., 2015; Lin et al., 2017b).
Reactive oxygen species (ROS), such as superoxide anion (O2-.)and hydrogen peroxide (H2O2), was reported to cause pericarp browning of fruits (Shah et al., 2017). Overaccumulation of ROS could lead to peroxidation of membrane lipid, loss of compartmentalization of cells and organelles, and formation of browning substances when peroxidase (POD) and polyphenol oxidase (PPO) contact with phenols (Lin Y.F. et al., 2016, Lin et al., 2017a; Sun et al., 2018). There are active oxygen-scavenging enzymes including catalase (CAT), superoxide dismutase (SOD), and ascorbate peroxidase (APX) (Jiang et al., 2015; Luo et al., 2015; Xu et al., 2017; Chen et al., 2019). Moreover, plant cells contain non-enzymatic endogenous antioxidant substances, including glutathione (GSH) and ascorbic acid (AsA) (Sun et al., 2018).
Fungal infection is a main problem in quality keeping for harvested longan fruits (Chen et al., 2014; Zhang et al., 2017, 2018). Phomopsis longanae Chi is a major fungus infecting postharvest longans (Chen et al., 2014, 2018b). P. longanae-inoculation could induce disease development and pericarp browning of postharvest longans (Chen et al., 2018a). However, there is no comprehensive understanding about the mechanisms of disease development and pericarp browning of postharvest longans induced by P. longanae.
This study aimed to analyze effects of P. longanae-inoculation on production rate of O2-., content of malondialdehyde (MDA) (resulting from oxidative damage of membrane lipids), activities of CAT, SOD, and APX, levels of GSH and AsA, reducing power and 1,1-diphenyl-2-picrylhydrazyl (DPPH) radical scavenging ability, and to explore mechanisms of pericarp browning and disease development of postharvest longans induced by P. longanae-infection.
Materials and Methods
Materials and Treatments
The spore suspension of P. longanae with the concentration of ×104 spores mL-1 was prepared according to Chen et al. (2014).
Longan (Dimocarpus longan Lour. cv. Fuyan) fruits at commercial maturity were harvested from an orchard in Quanzhou, Fujian, China, thereafter carried back by a refrigerated truck to our laboratory in less than 3 h.
Fruits were selected with absence of injury or diseases, and uniform size, color and maturity. and absence of injury, blemishes, insect pests or diseases. Fruits were surface-sterilized by dipped in 0.5% NaClO for 10 s, thereafter were air dried. Then 30,150 longans were used for experiment. Among them, 150 fruits were selected for used for analysis on the harvest day (day 0). The rest 3, 000 longans were divided into a control group (1,500 longans) and a P. longanae-inoculated group (1,500 longans). Longans in control group were dipped in sterile deionized water for 5 min, while longans in another group used for inoculation were treated by P. longanae spore suspension instead of sterile deionized water for 5 min. After air-dried, all the treated fruits were packed (50 fruits/bag) in polyethylene bags (0.015-mm-thick). Each treatment contains 30 bags. Then the fruits were stored under the same storage condition of 28°C and 90% relative humidity. 150 fruits (3 bags) from each treatment were randomly selected at each storage day to determine the physiological and biochemical indexes and their relations to longan pericarp browning and disease development caused by P. longanae (Chen et al., 2018a).
O2-. Generation Rate and MDA Content
One gram of pericarp tissue from 10 longans was used for analysis of O2-. the generation rate according to previously reported method (Lin et al., 2014). The O2-. generating rate was represented as nmol g-1 min-1.
One gram of pericarp tissue from 10 longans was used for measurement of MDA content according to previously reported method (Lin et al., 2014). The MDA content was represented as mmol g-1.
Activities of SOD, CAT, and APX
The activities of SOD, CAT, and APX, and the protein content were determined using one gram of pericarp from 10 longans, respectively, referring to previously reported method (Sun et al., 2018). Activities of these enzymes were represented as U mg-1 protein.
Contents of GSH and AsA
One gram of pericarp tissue from 10 longans was used to measure contents of GSH and AsA, respectively, according to previously reported method (Sun et al., 2018). The contents of GSH and AsA were represented as mg kg-1.
DPPH Radical Scavenging Activity and Reducing Power
One gram of pericarp tissue from 10 longans was used to measure DPPH radical scavenging activity and reducing power, respectively, with previously reported method (Sun et al., 2018). The DPPH radical scavenging ability and reducing power were represented as % and g kg-1, respectively.
Statistical Analysis
All experiments were repeated for three times. Data were represented in form of means ± standard error. Statistical analyses were performed for analyzing the data by SPSS Statistics (version 17.0). Statistical differences were assessed with a significant level when P-value less than 0.05.
Results
Effects of P. longanae Infection on O2-. Generation Rate and MDA Content in Pericarp of Harvested Longan Fruit
The generation rate of O2-. in pericarp tissue of the control longan fruits rose rapidly within 0–2 days, then a slow increase from 2 to 3 days, thereafter a quick increase during 3–5 days of storage (Figure 1A). The O2-. generation rate in P. longanae-inoculated longan fruits showed a trend similar as the control longans. Moreover, the O2-. generation rate was higher (P < 0.05) in postharvest longans inoculated by P. longanae compared to the control longans during 1–5 days of storage.
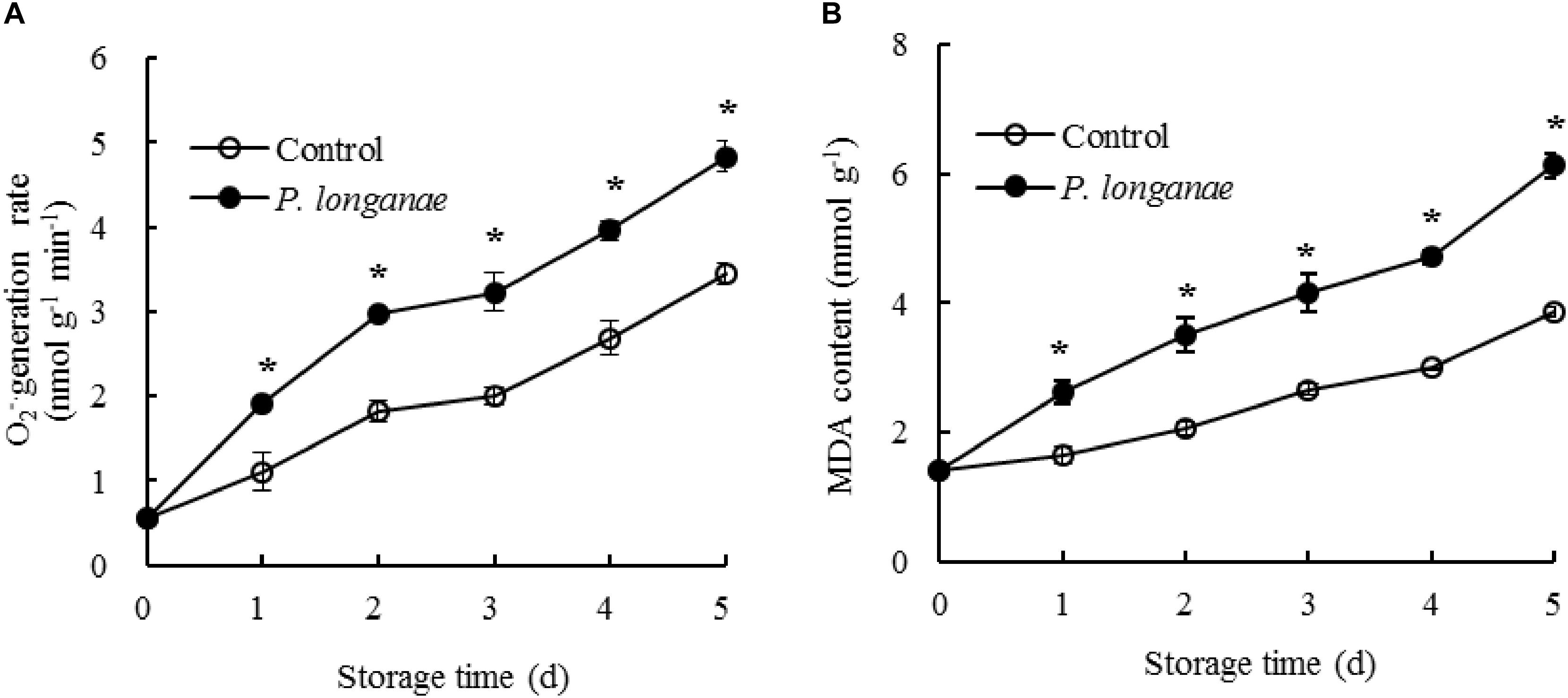
FIGURE 1. Effects on O2-. generation rate (A) and MDA content (B) in pericarp of postharvest longan fruits inoculated by P. longanae. The symbol “∗” indicates difference significant between control and P. longanae-inoculated fruit (P < 0.05).
The content of MDA in pericarp tissue of the control longan fruits presented a gradual rise in storage days 0–5 (Figure 1B). While the content of MDA in postharvest longans inoculated by P. longanae showed a rapid rise from 0 day of storage. For example, the MDA content of P. longanae-inoculated longan fruits increased about 4-fold from storage day 0 to day 5. Statistical comparison suggested that the MDA content in postharvest longans inoculated by P. longanae was higher (P < 0.05) compared to the control longan fruits during 1–5 days of storage.
Effects of P. longanae Infection on Activities of SOD, CAT, and APX in Pericarp of Harvested Longan Fruit
The SOD activity changed little during 0–1 day of storage, and thereafter declined rapidly in pericarp tissue of the control longan fruits (Figure 2A). While SOD activity increased within 0–2 days of storage, thereafter decreased quickly in longans inoculated by P. longanae. Compared to control longans, the P. longanae-inoculation treated longans showed a higher (P < 0.05) and a lower (P < 0.05) SOD activity during 1–3 and 4–5 days, respectively.
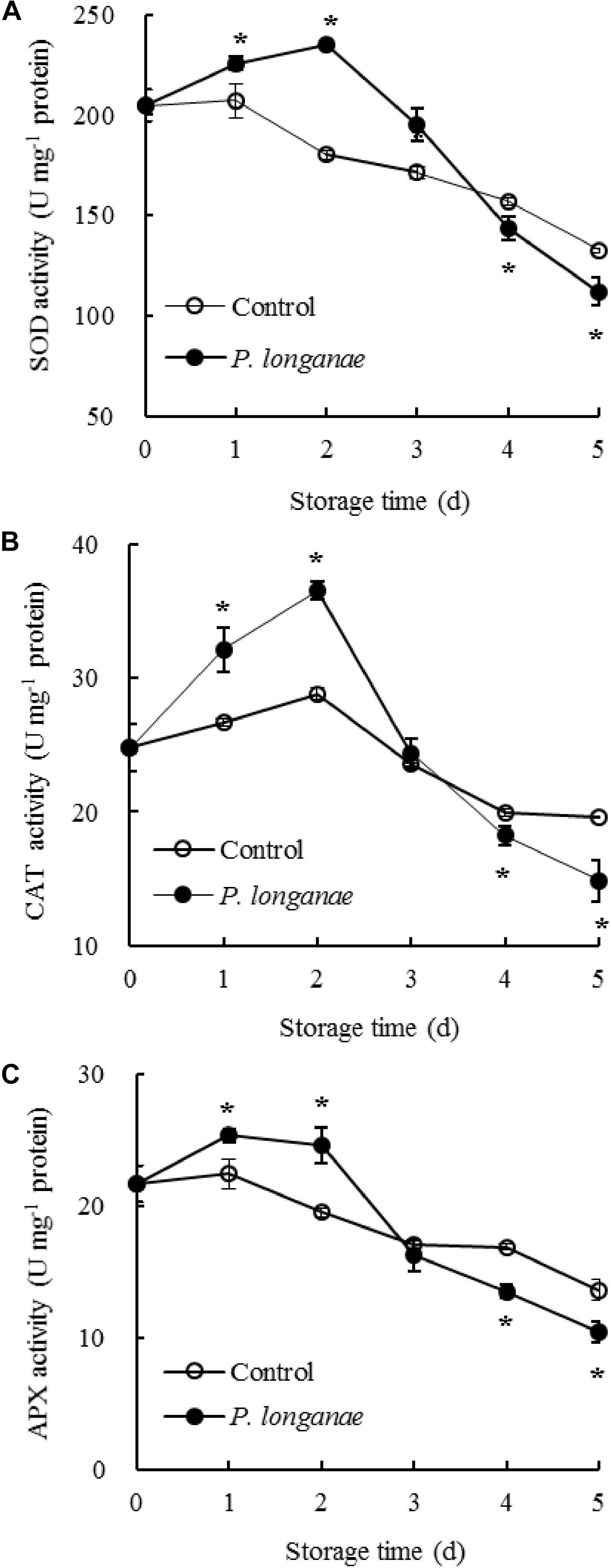
FIGURE 2. Effects on activities of SOD (A), CAT (B), and APX (C) in pericarp of postharvest longans inoculated by P. longanae. The symbol “∗” indicates difference significant between control and P. longanae-inoculated fruit (P < 0.05).
The CAT activity in the control longan pericarp tissue rose slightly during the first 0–2 days, then declined rapidly after 2 days of storage (Figure 2B). Whereas, the CAT activity showed a dramatical rise in the first 0–2 days of storage and declined sharply thereafter in P. longanae-inoculated longans. Further comparison showed CAT activity was higher (P < 0.05) in pericarp tissue of postharvest longans inoculated by P. longanae within 0–2 days of storage, and lower (P < 0.05) on the storage day 5 compared to the control longans.
Figure 2C showed that in control longan pericarp tissue the APX activity changed little within 0–1 day, followed by a reduction from 1 to 5 days. However, the APX activity in P. longanae-inoculated longan fruits showed a noticeably rise during 0–1 day, and changed little during 1–2 days, then declined rapidly during 2–5 days. Furthermore, in pericarp tissue of longans inoculated by P. longanae, APX activity was higher (P < 0.05) within the first 2 days, and lower (P < 0.05) during 4–5 days of storage compared to control longans.
Effects of P. longanae Infection on Contents of GSH and AsA in Pericarp of Harvested Longan Fruit
Contents of GSH and AsA reduced rapidly as storage time prolonging in pericarp tissue of the control longan fruit (Figure 3). Compared to control longan fruit, P. longanae-inoculated longans displayed faster decline of contents of GSH and AsA from 0 to 5 days. Moreover, the contents of GSH and ASA were lower (P < 0.05) in longans inoculated by P. longanae compared to the control longans during 2–5 days of storage.
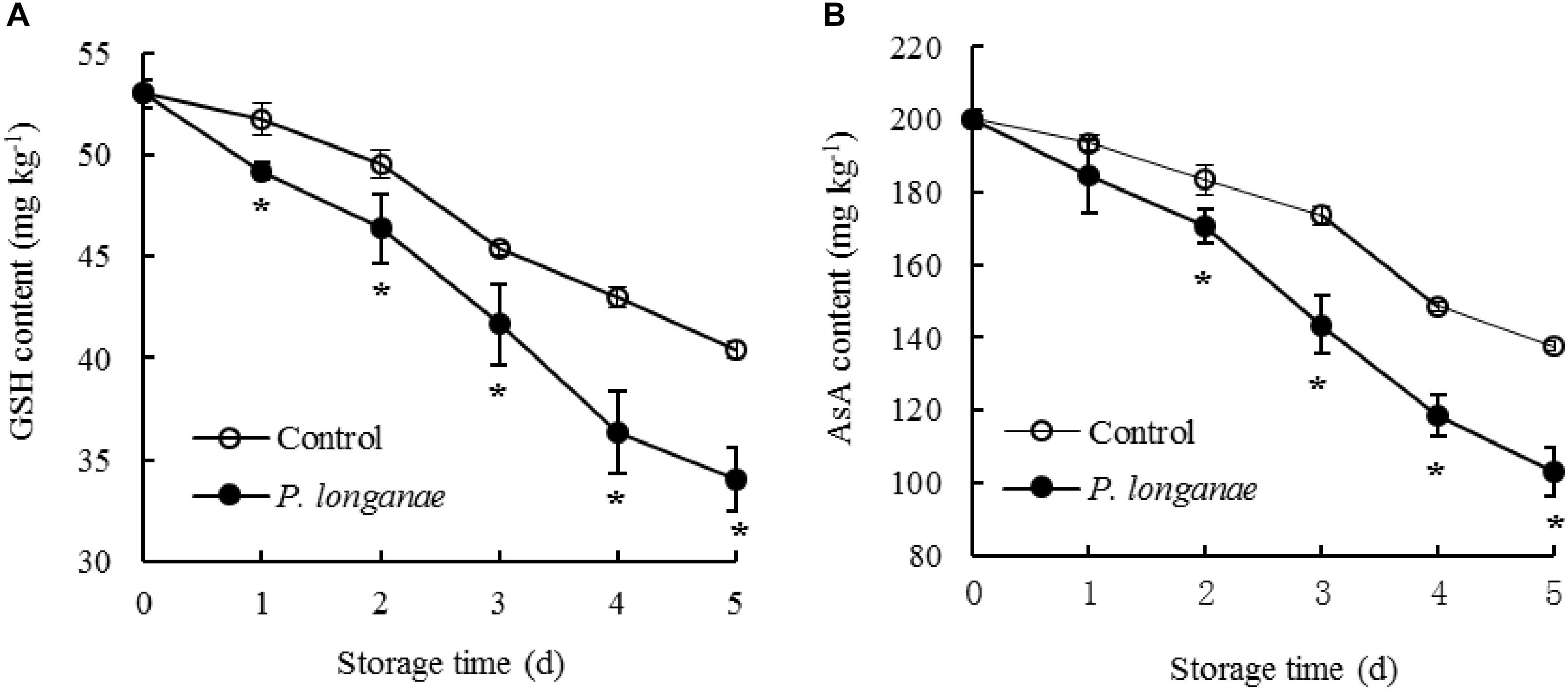
FIGURE 3. Effects on contents of GSH (A) and AsA (B) in pericarp of postharvest longans inoculated by P. longanae. The symbol “∗” indicates difference significant between control and P. longanae-inoculated fruit (P < 0.05).
Effects of P. longanae Infection on DPPH Radical Scavenging Ability and Reducing Power in Pericarp of Harvested Longan Fruit
The DPPH radical scavenging ability in control longan pericarp tissue exhibited a slightly decrease from 0 to 1 day, followed by a faster decreased from 1 to 5 days of storage (Figure 4A). Whereas, the DPPH radical scavenging ability in longans inoculated by P. longanae exhibited a rapid decline from 0 d, thereafter a decrease during 1–3 days, a sharp reduction during 3–4 days, then a fast decline during 4–5 days. Furthermore, there was a lower (P < 0.05) DPPH radical scavenging ability in longans inoculated by P. longanae compared to the control longans within 1–5 days except storage day 3.
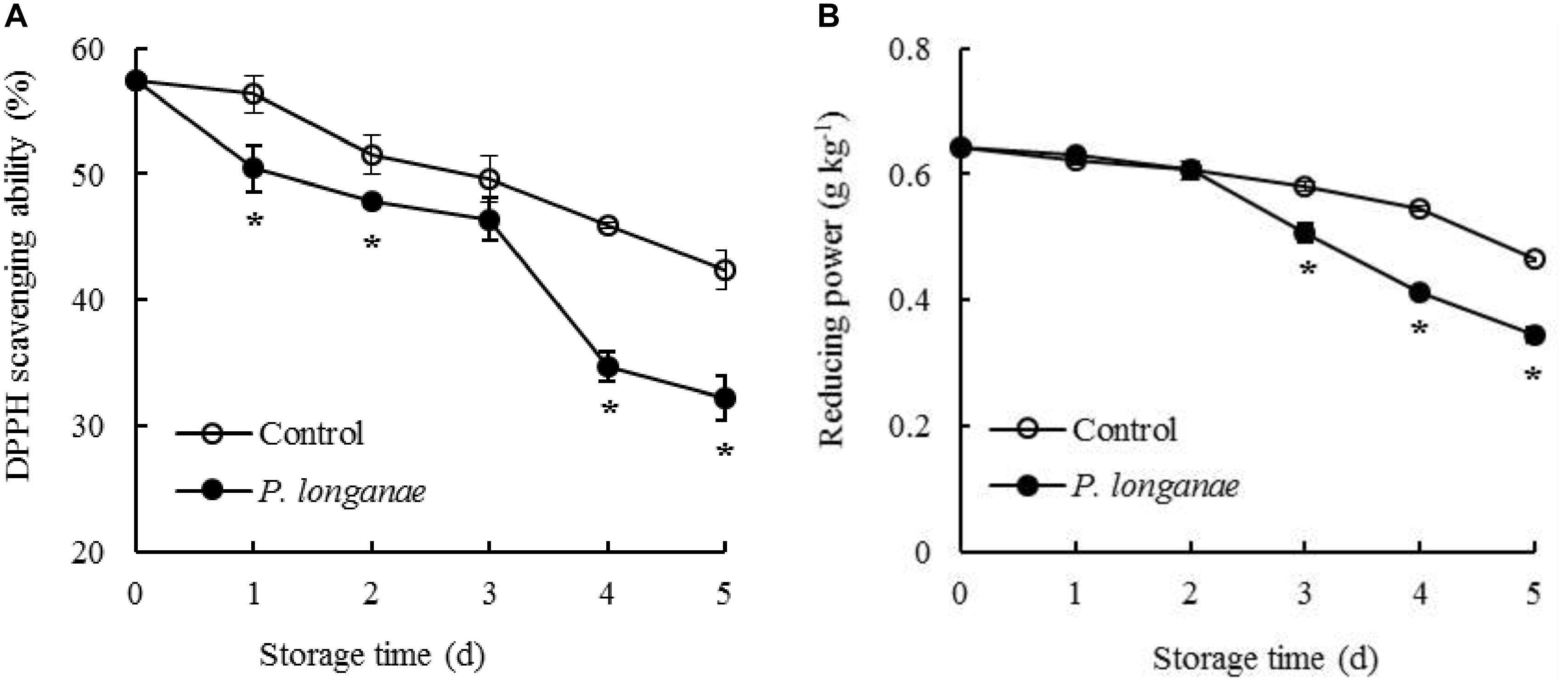
FIGURE 4. Effects on DPPH radical scavenging ability (A) and reducing power (B) of postharvest longans inoculated by P. longanae. The symbol “∗” indicates difference significant between control and P. longanae-inoculated fruit (P < 0.05).
The reducing power decreased slowly within 0–4 days in pericarp tissue of the control longan fruits, thereafter declined rapidly from 4 to 5 days of storage (Figure 4B). While the reducing power in longans inoculated by P. longanae decreased slightly during 0–2 days, then declined remarkably from 4.06 g kg-1 on storage day 3 to 2.76 g kg-1 on day 5. Compared to the control longans, the P. longanae-inoculation treated longan fruits showed a lower (P < 0.05) reducing power in pericarp tissue during 3–5 days of storage.
Discussion
Longan fruit is prone to turn to pericarp browning and diseased after harvest, which results in a decline in fruit quality and commercial value, and is the main limitation of its long-time storage and long-distant transport (Sun et al., 2018). Pathogen infection is a major reason accounting for disease and pericarp browning of postharvest longans (Chen et al., 2014). Disease development and pericarp browning of postharvest longans was thought to be related to reactive oxygen metabolism disorder and accumulation of ROS (Chomkitichai et al., 2014; Lin Y.F. et al., 2016). It has been reported that H2O2 and O2-. accumulated during the process of disease and pericarp browning development in harvested longans (Lin et al., 2017a). Chen et al. (2018a) reported that compared to the control longans, P. longanae-inoculated longans displayed higher indexes of pericarp browning and fruit disease. In present work, results showed that P. longanae-infection induced the rise of O2-. generation rate (Figure 1A). During 0–2 days of storage, the rise of O2-. generation rate might be related with the defensive reaction against the infection of P. longanae (Sun et al., 2018). MDA is a product resulting from oxidative damage of membrane lipids (Wang et al., 2013, 2014, 2015). In this study, the content of MDA accumulated as storage time prolonging (Figure 1B). However, P. longanae-infection enhanced the accumulation of MDA, indicating that the biomembrane system was seriously damaged in P. longanae-inoculate longan fruit.
In plant cells, there are ROS scavenging enzymes playing crucial roles in protecting cells from oxidative stress (Bloknina et al., 2003; Luo et al., 2015; Xu et al., 2017; Chen et al., 2019). SOD can catalyze two O2-. to H2O2 and O2 (Duan et al., 2007). Both CAT and APX could catalyze H2O2 to H2O and O2 (Yi et al., 2010). Under normal circumstances, the ROS is in a dynamic equilibrium between generation and scavenging. However, when the equilibrium is broken under stress conditions, ROS would be accumulated (Yi et al., 2008; Sun et al., 2018). The increase of ROS account for membrane lipid peroxidation and cellular compartmentalization lost, leading to the disease increase and enzymatic browning resulting from oxidation of phenolic substrates by PPO and POD (Sun et al., 2010; Lin et al., 2013, 2017c, 2018; Jiang et al., 2018). It has been reported that the enhanced activities of CAT, SOD, and APX might be caused by pathogen infection in early infection stage to scavenge excessed ROS in harvested longans (Lin et al., 2015). Whereas, the activities of CAT, SOD, and APX decreased with prolonged storage time, leading to the increase of O2-. content, peroxidation of membrane lipid and increased browning of harvested longans (Sun et al., 2018).
In present study, activities of SOD, CAT, and APX showed rise within 0–2 days in P. longanae-inoculated longans (Figure 2). Meanwhile, the generation rate of O2-. increase quickly (Figure 1A). Thus, the enhanced activities of SOD, CAT, and APX when O2-. generation rate rise in early stage (0–2 days of storage) of P. longanae-infection might be a defense to reduce contents of ROS. Besides, in longans infected by P. longanae activities of CAT, SOD, and APX decreased rapidly during 2–5 days of storage while the O2-. generation rate still exhibited a trend of increase. This indicated that the equilibrium between ROS scavenging enzymes and ROS was broken during 2–5 days of storage, resulting in the rise of O2-. generation rate, membrane lipid peroxidation, and accumulation of MDA.
In addition, non-enzymatic antioxidant substances, including GSH and AsA, exist in fruit tissues, playing important roles in ROS elimination (Jiang et al., 2015, 2018). AsA and GSH participate in the reaction of catalyzing H2O2 to H2O by APX (Lin et al., 2017a). Our results presented that contents of GSH and AsA decreased quickly in longans inoculated by P. longanae and showed a lower (P < 0.05) compared with the control longan fruits during 2–5 days of storage (Figure 3). It can be inferred that P. longanae-infection induced decrease of GSH and AsA contents in pericarp tissue of postharvest longan fruits, which might account for the increase of O2-. generation rate during storage.
Besides, there is another free radical-scavenging system for inhibiting lipid peroxidation (Sun et al., 2018). The reducing power and DPPH radical scavenging activity were generally tested to analysis the antioxidant activity (Sun et al., 2007; Lin et al., 2017a). The browning in pathogen infected fruit pericarp tissue was thought to be correlated with the reduced reducing power and the decline of DPPH radical scavenging activity (Sun et al., 2018). In this research, the P. longanae-inoculated longans showed lower reducing power and DPPH radical scavenging ability compared to control longans in late storage period (4–5 days). Meanwhile, the O2-. generation rate (Figure 1A), pericarp browning developed faster in P. longanae-inoculated longan compared with control longans (Chen et al., 2018a). This suggested that the rising O2-. generation rate and browning index of P. longanae-inoculated longans might due to the reduced antioxidant activity.
Conclusion
In conclusion, the disruption of reactive oxygen scavenging system by P. longanae inoculation might be a vital reason causing pericarp browning accelerated and disease increased of postharvest longan fruits. The increasing O2-. generation rate and content of MDA in longans infected by P. longanae might be the result of the decreased ROS scavenging enzyme activities, the reduced the contents of non-enzymatic antioxidant substances, the declined reducing power, and the lowered DPPH radical scavenging ability, which leads to the peroxidation of membrane lipid, the loss of compartmentalization in longan pericarp cells, and subsequently cause PPO and POD to contact with phenolic substrates, in turn, result in enzymatic browning of longan pericarp, as well as cause the decrease of disease resistance to P. longanae and stimulate disease development of harvested longan fruit. The probable mechanism of P. longanae-induced pericarp browning and disease development of postharvest longan fruit via acting on ROS metabolism was demonstrated in Figure 5.
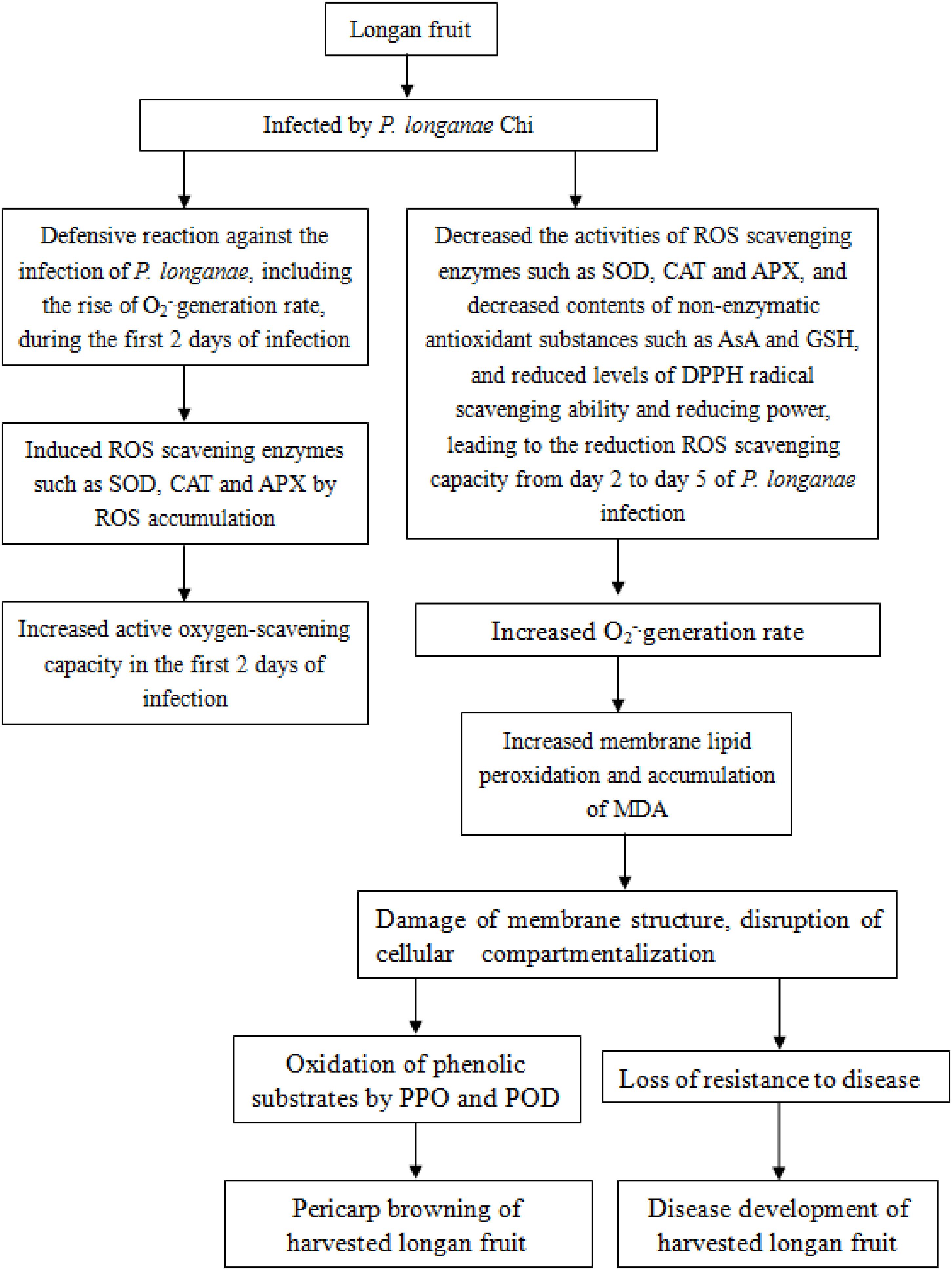
FIGURE 5. The probable mechanism of P. longanae-induced pericarp browning and disease development of postharvest longan fruit via acting on ROS metabolism.
Author Contributions
HW and YC performed the experiments. HL designed the research. JS and YL conducted the experiments and analyzed the data. HW and YC wrote the manuscript. HL revised the manuscript. ML edited English language of the manuscript. All authors have approved the submission and publication of the manuscript.
Funding
This work was supported by the National Natural Science Foundation of China (Grant Nos. 31671914, 31171776, and 31772035), the Natural Science Foundation of Fujian Province of China (Grant No. 2017J01429), the National Science Fund for Distinguished Young Scholars at Fujian Province University of China (Grant No. KLa16036A), and the Science Fund for Distinguished Young Scholars at Fujian Agriculture and Forestry University of China (Grant No. XJQ20 1512).
Conflict of Interest Statement
The authors declare that the research was conducted in the absence of any commercial or financial relationships that could be construed as a potential conflict of interest.
References
Bloknina, O., Virolainen, E., and Fagerstedt, K. V. (2003). Antioxidants, oxidative damage and oxygen deprivation stress: a review. Ann. Bot. 91, 179–194. doi: 10.1093/aob/mcf118
Chen, M. Y., Lin, H. T., Zhang, S., Lin, Y. F., Chen, Y. H., and Lin, Y. X. (2015). Effects of adenosine triphosphate (ATP) treatment on postharvest physiology, quality and storage behavior of longan fruit. Food Bioproc. Technol. 8, 971–982. doi: 10.1007/s11947-014-1462-z
Chen, Y., Lin, H., Jiang, Y., Zhang, S., Lin, Y., and Wang, Z. (2014). Phomopsis longanae Chi-induced pericarp browning and disease development of harvested longan fruit in association with energy status. Postharvest Biol. Technol. 93, 24–28. doi: 10.1016/j.postharvbio.2014.02.003
Chen, Y. H., Hung, Y. C., Chen, M. Y., Lin, M. S., and Lin, H. T. (2019). Enhanced storability of blueberries by acidic electrolyzed oxidizing water application may be mediated by regulating ROS metabolism. Food Chem. 270, 229–235. doi: 10.1016/j.foodchem.2018.07.095
Chen, Y. H., Lin, H. T., Zhang, S., Sun, J. Z., Lin, Y. F., Wang, H., et al. (2018a). Phomopsis longanae Chi-induced disease development and pericarp browning of harvested longan fruit in association with energy metabolism. Front. Microbiol. 9:1454. doi: 10.3389/fmicb.2018.01454
Chen, Y. H., Zhang, S., Lin, H. T., Sun, J. Z., Lin, Y. F., Wang, H., et al. (2018b). Phomopsis longanae Chi-induced changes in activities of cell wall-degrading enzymes and contents of cell wall components in pericarp of harvested longan fruit and its relation to disease development. Front. Microbiol. 9:1051. doi: 10.3389/fmicb.2018.01051
Chomkitichai, W., Faiyue, B., Rachtanapun, P., Uthaibutra, J., and Saengnil, K. (2014). Enhancement of the antioxidant defense system of post-harvested ‘Daw’ longan fruit by chlorine dioxide fumigation. Sci. Hortic. 178, 138–144. doi: 10.1016/j.scienta.2014.08.016
Duan, X. W., Su, X. G., You, Y. L., Qu, H. X., Li, Y. B., and Jiang, Y. M. (2007). Effect of nitric oxide on pericarp browning of harvested longan fruit in relation to phenolic metabolism. Food Chem. 104, 571–576. doi: 10.1016/j.foodchem.2006.12.007
Holcroft, D. M., Lin, H. T., and Ketsa, S. (2005). “Harvesting and storage,” in Litchi and longan: Botany, Production and Uses, eds C. M. Menzel and G. K. Waite (Wallingford: CAB International), 273–295. doi: 10.1079/9780851996967.0273
Jiang, T. J., Luo, Z. S., and Ying, T. J. (2015). Fumigation with essential oils improves sensory quality and enhanced antioxidant ability of shiitake mushroom (Lentinus edodes). Food Chem. 172, 692–698. doi: 10.1016/j.foodchem.2014.09.130
Jiang, X. J., Lin, H. T., Lin, M. S., Chen, Y. H., Wang, H., Lin, Y. X., et al. (2018). A novel chitosan formulation treatment induces disease resistance of harvested litchi fruit to Peronophythora litchii in association with ROS metabolism. Food Chem. 266, 299–308. doi: 10.1016/j.foodchem.2018.01.095
Jiang, Y. M., Zhang, Z. Q., Joyce, D. C., and Ketsa, S. (2002). Postharvest biology and handling of longan fruit (Dimocarpus longan Lour.). Postharvest Biol. Technol. 26, 241–252. doi: 10.1016/S0925-5214(02)00047-9
Lin, H. T., Chen, S. J., Chen, J. Q., and Hong, Q. Z. (2001). Current situation and advances in post-harvest storage and transportation technologies of longan fruit. Acta Hortic. 558, 343–351. doi: 10.17660/ActaHortic.2001.558.56
Lin, Y. F., Chen, M. Y., Lin, H. T., Hung, Y. C., Lin, Y. X., Chen, Y. H., et al. (2017a). DNP and ATP induced alteration in disease development of Phomopsis longanae Chi inoculated longan fruit by acting on energy status and reactive oxygen species production-scavenging system. Food Chem. 228, 497–505. doi: 10.1016/j.foodchem.2017.02.045
Lin, Y. F., Lin, Y. X., Lin, H. T., Ritenour, M. A., Shi, J., Zhang, S., et al. (2017b). Hydrogen peroxide-induced pericarp browning of harvested longan fruit in association with energy metabolism. Food Chem. 225, 31–36. doi: 10.1016/j.foodchem.2016.12.088
Lin, Y. F., Lin, Y. X., Lin, H. T., Shi, J., Chen, Y. H., and Wang, H. (2017c). Inhibitory effects of propyl gallate on membrane lipids metabolism and its relation to increasing storability of harvested longan fruit. Food Chem. 217, 133–138. doi: 10.1016/j.foodchem.2016.08.065
Lin, Y. F., Hu, Y. H., Lin, H. T., Liu, X., Chen, Y. H., Zhang, S., et al. (2013). Inhibitory effects of propyl gallate on tyrosinase and its application in controlling pericarp browning of harvested longan longans. J. Agric. Food Chem. 61, 2889–2895. doi: 10.1021/jf305481h
Lin, Y. F., Lin, H. T., Lin, Y. X., Zhang, S., Chen, Y. H., and Jiang, X. J. (2016). The roles of metabolism of membrane lipids and phenolics in hydrogen peroxide-induced pericarp browning of harvested longan fruit. Postharvest Biol. Technol. 111, 53–61. doi: 10.1016/j.postharvbio.2015.07.030
Lin, Y. F., Lin, H. T., Zhang, S., Chen, Y. H., Chen, M. Y., and Lin, Y. X. (2014). The role of active oxygen metabolism in hydrogen peroxide-induced pericarp browning of harvested longan fruit. Postharvest Biol. Technol. 96, 42–48. doi: 10.1016/j.postharvbio.2014.05.001
Lin, Y. F., Lin, Y. X., Lin, H. T., Chen, Y. H., Wang, H., and Shi, J. (2018). Application of propyl gallate alleviates pericarp browning in harvested longan fruit by modulating metabolisms of respiration and energy. Food Chem. 240, 863–869. doi: 10.1016/j.foodchem.2017.07.118
Lin, Y. F., Lin, Y. X., Lin, H. T., Zhang, S., Chen, Y. H., and Shi, J. (2015). Inhibitory effects of propyl gallate on browning and its relationship to active oxygen metabolism in pericarp of harvested longan fruit. LWT Food Sci. Technol. 60, 1122–1128. doi: 10.1016/j.lwt.2014.10.008
Lin, Y. X., Lin, Y. F., Chen, Y. H., Wang, H., Shi, J., and Lin, H. T. (2016). Hydrogen peroxide induced changes in energy status and respiration metabolism of harvested longan fruit in relation to pericarp browning. J. Agric. Food Chem. 64, 4627–4632. doi: 10.1016/j.foodchem.2016.12.088
Luo, Z. S., Li, D. D., Du, R. X., Mou, W. S., and Mao, L. C. (2015). Hydrogen sulfide alleviates chilling injury of banana fruit by enhanced antioxidant capacity and proline content. Sci. Hortic. 183, 144–151. doi: 10.1016/j.scienta.2014.12.021
Prasad, K. N., Hao, J., Shi, J., Liu, T., Li, J., Wei, X. Y., et al. (2009). Antioxidant and anticancer activities of high pressure-assisted extract of longan (Dimocarpus longan Lour) fruit pericarp. Innov. Food Sci. Emerg. 10, 413–419. doi: 10.1016/j.ifset.2009.04.003
Shah, H. M. S., Khan, A. S., and Ali, S. (2017). Pre-storage kojic acid application delays pericarp browning and maintains antioxidant activities of litchi fruit. Postharvest Biol. Technol. 132, 154–161. doi: 10.1016/j.postharvbio.2017.06.004
Sun, J., Lin, H., Zhang, S., Lin, Y., Wang, H., Lin, M., et al. (2018). The roles of ROS production-scavenging system in Lasiodiplodia theobromae (Pat.) Griff. & Maubl.-induced pericarp browning and disease development of harvested longan fruit. Food Chem. 247, 16–22. doi: 10.1016/j.foodchem.2017.12.017
Sun, J., Shi, J., Jiang, Y. M., Xue, S. J., and Wei, X. Y. (2007). Identification of two polyphenolic compounds with antioxidant activities in longan pericarp tissues. J. Agric. Food Chem. 55, 5864–5868. doi: 10.1021/jf070839z
Sun, J., Zhang, Z., Xu, L. X., Li, Z. C., Wang, Z. X., and Li, C. B. (2010). Comparison on characterization of longan (Dimocarpus longan Lour) polyphenoloxidase using endogenous and exogenous substrates. J. Agric. Food Chem. 58, 10195–10201. doi: 10.1021/jf101639d
Wang, H., Zhi, W., Qu, H. X., Lin, H. T., and Jiang, Y. M. (2015). Application of α-aminoisobutyric acid and β-aminoisobutyric acid inhibits pericarp browning of harvested longan fruit. Chem. Cent. J. 9:54. doi: 10.1186/s13065-015-0124-1
Wang, Y. S., Luo, Z. S., Du, R. X., Liu, Y., Ying, T. J., and Mao, L. C. (2013). Effect of nitric oxide on antioxidative response and proline metabolism in banana during cold storage. J. Agric. Food Chem. 61, 8880–8887. doi: 10.1021/jf401447y
Wang, Y. S., Luo, Z. S., Huang, X. D., Yang, K. L., and Gao, S. J. (2014). Effect of exogenous γ-aminobutyric acid (GABA) treatment on chilling injury and antioxidant capacity in banana. Sci. Hortic. 168, 132–137. doi: 10.1016/j.scienta.2014.01.022
Xu, Y. Q., Luo, Z. S., Charles, M. T., Rolland, D., and Roussel, D. (2017). Pre-harvest UV-C irradiation triggers VOCs accumulation with alteration of antioxidant enzymes and phytohormones in strawberry leaves. J. Plant Physiol. 218, 265–274. doi: 10.1016/j.jplph.2017.09.002
Yang, B., Jiang, Y., Shi, J., Chen, F., and Ashraf, M. (2011). Extraction and pharmacological properties of bioactive compounds from longan (Dimocarpus longan Lour.) fruit–a review. Food Res. Int. 44, 1837–1842. doi: 10.1016/j.foodres.2010.10.019
Yi, C., Jiang, Y. M., Shi, J., Qu, H. X., Xue, S., Duan, X. W., et al. (2010). ATP regulation of antioxidant properties and phenolics in litchi fruit during browning and pathogen infection process. Food Chem. 118, 42–47. doi: 10.1016/j.foodchem.2009.04.074
Yi, C., Qu, H. X., Jiang, Y. M., Shi, J., Duan, X. W., Joyce, D. C., et al. (2008). ATP induced changes in energy status and membrane integrity of harvested litchi fruit and its relation to pathogen resistance. J. Phytopathol. 156, 365–371. doi: 10.1111/j.1439-0434.2007.01371.x
Zhang, S., Lin, H. T., Lin, Y. F., Lin, Y. X., Hung, Y. C., Chen, Y. H., et al. (2017). Energy status regulates disease development and respiratory metabolism of Lasiodiplodia theobromae (Pat.) Griff. & Maubl.-infected longan fruit. Food Chem. 231, 238–246. doi: 10.1016/j.foodchem.2017.03.132
Zhang, S., Lin, Y. Z., Lin, H. T., Lin, Y. X., Chen, Y. H., Wang, H., et al. (2018). Lasiodiplodia theobromae (Pat.) Griff. & Maubl.-induced disease development and pericarp browning of harvested longan fruit in association with membrane lipids metabolism. Food Chem. 244, 93–101. doi: 10.1016/j.foodchem.2017.10.020
Keywords: longan fruit, disease development, pericarp browning, reactive oxygen species (ROS), ROS metabolism, ROS scavenging ability, Phomopsis longanae Chi
Citation: Wang H, Chen Y, Lin H, Sun J, Lin Y and Lin M (2018) Phomopsis longanae Chi-Induced Change in ROS Metabolism and Its Relation to Pericarp Browning and Disease Development of Harvested Longan Fruit. Front. Microbiol. 9:2466. doi: 10.3389/fmicb.2018.02466
Received: 30 June 2018; Accepted: 26 September 2018;
Published: 16 October 2018.
Edited by:
Hongyin Zhang, Jiangsu University, ChinaReviewed by:
Jinhe Bai, U.S. Horticultural Research Laboratory, United StatesZisheng Luo, Zhejiang University, China
Copyright © 2018 Wang, Chen, Lin, Sun, Lin and Lin. This is an open-access article distributed under the terms of the Creative Commons Attribution License (CC BY). The use, distribution or reproduction in other forums is permitted, provided the original author(s) and the copyright owner(s) are credited and that the original publication in this journal is cited, in accordance with accepted academic practice. No use, distribution or reproduction is permitted which does not comply with these terms.
*Correspondence: Hetong Lin, aGV0b25nbGluQDEyNi5jb20=; aGV0b25nbGluQDE2My5jb20=