- 1Key Laboratory of Tea Biology and Resources Utilization, Ministry of Agriculture and Rural Affairs, National Center for Tea Improvement, Tea Research Institute of Chinese Academy of Agricultural Sciences, Hangzhou, China
- 2College of Horticulture and Forestry, Huazhong Agricultural University, Wuhan, China
Colletotrichum, the causative agent of anthracnose, is an important pathogen that invades the tea plant (Camellia sinensis). In this study, 38 isolates were obtained from the diseased leaves of tea plants collected in different areas of Zhejiang Province, China. A combination of multigene (ITS, ACT, GAPDH, TUB2, CAL, and GS) and morphology analyses showed that the 38 strains belonged to two different species, namely, C. camelliae (CC), and C. fructicola (CF). Pathogenicity tests revealed that CC was more invasive than CF. In vitro inoculation experiments demonstrated that CC formed acervuli at 72 hpi and developed appressoria on wound edges, but CF did not develop these structures. Under treatment with catechins and caffeine, the growth inhibition rates of CF were remarkably higher than those of CC, indicating that the nonpathogenic species CF was more vulnerable to catechins and caffeine. Growth condition testing indicated that CF grew at a wide temperature range of 15–35°C and that the optimum temperature for CC growth was 25°C. Growth of both CC and CF did not differ between acidic and weakly alkaline environments (pH 5–8), but the growth of CC was significantly reduced at pH values of 9 and 10. Furthermore, the PacC/RIM101 gene, which associated with pathogenicity, was identified from CC and CF genomes, and its expression was suppressed in the hyphae of both species under pH value of 5 and 10, and much lower expression level was detected in CC than that in CF at pH 6. These results indicated that temperature has more important effect than pH for the growth of two Colletotrichum species. In conclusion, the inhibition by secondary metabolite is an important reason why the pathogenicity by CC and CF are different to tea plant, although the environmental factors including pH and temperature effect the growth of two Colletotrichum species.
Introduction
Tea, which is produced by new shoots from tea plants [Camellia sinensis (L.) O. Kuntze], is one of the most popular nonalcoholic healthy beverages worldwide. The tea plant is an important economic woody crop and is widely planted in some Asian, African and South American countries, such as China, India, Japan, Sri Lanka, and Kenya. The buds and leaves of the tea plants suffer from many kinds of diseases, resulting in loss of revenue (Guo et al., 2014). Anthracnose is one of the main diseases of the tea plant. Survey results have shown that the morbidity associated with anthracnose, the development of which is related to temperature and humidity, is more serious in the southern region than in the northern region of China (Liu et al., 2016). Mutiple Colletotrichum species were the pathogen causing anthracnose of tea plant (Wang et al., 2016a). When Colletotrichum invades Ca. sinensis, bottle-green, watery lesions emerge on the surface of the leaves early in infection, and the scabs enlarge over time. In the final phase of infection, dense tiny black dots called acervuli form on the lesion; the acervuli, which are small asexual fruiting bodies that can produce conidia which facilitate disease transmission, are the origin of disease spread (Liu et al., 2016).
Colletotrichum is a large genus of Ascomycete fungi causing anthracnose disease in a wide range of host genera (Cannon et al., 2012; Takahara et al., 2016). In the tea plant, Colletotrichum exists as both a pathogen and an endophyte (Liu et al., 2015). Species of the genus Colletotrichum have been isolated from Ca. sinensis in China, and 17 known species and 1 indistinguishable strain have been identified based on morphological characteristics and multigene molecular phylogeny (Hyde et al., 2009; Cannon et al., 2012), with C. Camelliae (CC) and C. fructicola (CF) being the dominant pathogens identified based on the highly isolation rate from the main tea region in China and their pathogenicity (Liu et al., 2015; Wang et al., 2016a). The earliest known record of tea anthracnose in the tea plant was chronicled in 1899 by Massee, and this holotype was labeled CC (Willis, 1899). Glomerella cingulata f. sp. camelliae, which is the proposed causative agent of disease on Ca. saluenensis hybrids, is a homonym of CC (Cannon et al., 2012; Weir et al., 2012; Liu et al., 2015). CC and CF is both a vital pathogen and an endophyte of several plants, of which CC was only isolated from Camellia in previous study (Wang et al., 2016a). However, knowledge of the detailed traits of CC and CF in the tea plant is limited.
The majority of Colletotrichum have adopted a hemibiotrophic infection strategy to invade host plants; in this strategy, fungi initially develop biotrophic hyphae inside the living host, which later transition to necrotrophic secondary mycelia (O’Connell et al., 1985, 2004; Rao and Nandineni, 2017). In addition, the appressoria and conidia (acervuli) are responsible for initiating infection as well as disseminating the disease and are thus important factors impacting the pathogenicity of the fungus (Emmett and Parbery, 1975; Asakura et al., 2006). Moreover, the appressorium is an important specialized structure that penetrates the cuticle to infect the host plant, and it punctures host surfaces though various processes, such as cell wall melanization, glycerol accumulation, mechanical forcing and enzymatic degradation (Deising et al., 2000; O’Connell et al., 2012). The basal region of appressoria contains the penetration pore, from which effectors are secreted to suppress or evade basal resistance (Kleemann et al., 2012). C. higginsianum, C. graminicola, C. gloeosporioides, and other Colletotrichum species have been used as model organisms for the study of the infection process (O’Connell et al., 2012; Ko et al., 2016), but the infection strategies of CC and CF are unclear. Hence, we have limited information for interpreting the similarities and differences in the infection process of these two organisms.
Pathogenicity is the potential capacity to cause disease in host plants. Different fungal species display different levels of virulence toward their host plant. Wang et al. (2016a) considered CC, C. aenigma, and C. endophytica to be the fungal species most virulent to Ca. sinensis in China. Furthermore, the virulence of different isolates belonging to the same species varied. The growth of fungal hyphae, the differentiation of appressoria, and the development of acervuli are important for fungal virulence. Environmental conditions can affect the growth and biological characteristics of fungi. The germination time and growth rate of a fungus depend on temperature, humidity, and surface nutrients (Pasanen et al., 1991). Temperature is one of the vital factors affecting fungal growth. Growth rates decrease or cease at nonpermissive temperatures. The pH is another critical factor in fungal–host interactions. The environmental pH value can alter the activity of enzymes such as polygalacturonase (PG) and pectatelyase (PL) and consequently alter the virulence of pathogens (Mackenzie et al., 1993). Extracellular pH is controlled by a conserved fungal pH response pathway regulated by PacC/Rim101, which is a zinc finger transcription factor in filamentous fungi, contributing to fungal pathogenicity (Li et al., 2004; Antonin et al., 2010; Ment et al., 2014). Several species of Colletotrichum, such as C. higginsianum and C. gloeosporioides, alkalize the pH of their host (Miyara et al., 2010; O’Connell et al., 2012). In addition, PacC/Rim101 also plays an important role in filamentous fungi due to its regulation of pathogenicity genes under alkaline pH conditions (You et al., 2007; Miyara et al., 2010; Ment et al., 2014). Nonetheless, few reports have described the effect of ambient conditions and PacC/Rim101 regulation in CC and CF isolated from the tea plant.
Tea plants are rich in flavonoids and alkaloid, such as catechins and caffeine, which play roles in plant defense (Kuc, 1995). Several reports have indicated that alkaloids and phenolics can enhance plant resistance during pathogen–host interactions (Ahuja et al., 2012; Araujo et al., 2016). Catechins can inhibit the activity of PG and PL in C. gloeosporioides during interactions with avocado fruits and thereby slow the softening of avocado fruits after harvest (Prusky et al., 1988). Biotic stress can increase caffeine content, and plants may use endogenous caffeine to resist pathogens (Kim et al., 2010). However, there is little research on the relationship between secondary metabolites of tea plants and CC and CF.
In the present study, we aimed to identify Colletotrichum species isolated from Ca. sinensis by morphological characteristics and multigene molecular phylogeny, and then explored the difference in the biological characteristics of the strains, determined the pathogenicity of the different strains of Colletotrichum, and preliminarily clarified the reason that the discrepancy occurred.
Materials and Methods
Collection and Isolation
Leaves of tea plants were collected from the cities of Hangzhou, Lishui and Shaoxing in Zhejiang Province in China. Disease samples were collected from leaves showing visible anthracnose symptoms. The isolates were obtained by a single spore isolation technique described by Cai et al. (2009). The spores were dislodged from the anthracnose lesions with a sterilized small blade and were suspended in sterilized water. The suspension was distributed onto the surface of potato dextrose agar (PDA) culture medium, followed by incubation at 25°C. Single germinating conidia were picked to new PDA plates, and incubation at 25°C was continued to generate the pure isolates.
Morphological Characterization
The colony and conidial characteristics were determined using methods described by Cai et al. (2009). Mycelial discs (5 mm diameter) were taken from the margin of 5 days old cultures, transferred to PDA plates, and incubated in the dark at 25°C. Each isolate was measured in triplicate. The colony diameter was measured daily beginning 3 days after inoculation in order to calculate the growth rate (mm/d). The colony characteristics were also recorded. Appressoria were generated using a slide culture technique and were observed on the bottom surface of synthetic nutrient-poor agar (SNA) cultures (Cai et al., 2009; Damm et al., 2009). The shape and size of 30 conidia, conidiophores, and appressoria were measured.
Molecular Characterization
The isolates were incubated on PDA culture medium at 25°C for 7–10 days. Total genomic DNA was extracted with an Ezup Column Fungi Genomic DNA Purification Kit [Sangon Biotech (Shanghai) Company Limited, Shanghai, China] and was stored at −20°C. The DNA samples were used as the templates for PCR amplification. The ribosomal internal transcribed spacer (ITS), actin (ACT), glyceraldehyde-3-phosphate dehydrogenase (GAPDH), beta-tubulin (TUB2), calmodulin (CAL), and glutamine synthetase (GS) genes were amplified (Table 1). The PCR conditions for ITS were as follows: 4 min at 94°C; followed by 35 cycles of 94°C for 30 s, 55°C for 30 s, and 72°C for 30 s; and 10 min at 72°C. The annealing temperatures differed among the genes; the optimum annealing temperature for each gene follows: ACT: 58°C, CAL: 59°C, GAPDH: 60°C, GS: 52°C and TUB2: 55°C. Each PCR mixture (50 μL) included Premix TaqTM (25 μL) (TaKaRa Biomedical Technology Company Limited, Beijing, China), ddH2O (22 μL), each primer (1 μL), and genomic DNA (1 μL). The PCR products were examined with electrophoresis on a 1.0% agarose gel. Sequencing of the PCR products was performed by Shanghai Huagene Biotech Company Limited, Shanghai, China (Wang et al., 2016a).
Phylogenetic Analysis
The accession numbers of the sequences were obtained from NCBI GenBank and are listed in Table 2. SeqMan and DNAStar were used to generate the consensus sequences from the sequences of the forward and reverse primers. A phylogenetic tree was constructed using multilocus sequence analysis. Bayesian inference (BI) was used to construct the phylogenies using MrBayes v. 3.2. MrModel test v. 2.3 was used to select the best-fit models of nucleotide substitution. The dataset was assembled using MAFFT v.7 and modulated by MEGA v. 6.0. Ambiguously aligned regions were excluded, and all gaps were regarded as missing data. Markov chain Monte Carlo (MCMC) sampling was used to reconstruct the phylogenies in MrBayes v. 3.2. Analyses of 6 MCMC chains based on the full dataset were run for 2 × 107 generations and sampled every 100 generations (Yan et al., 2015; Wang et al., 2016a).
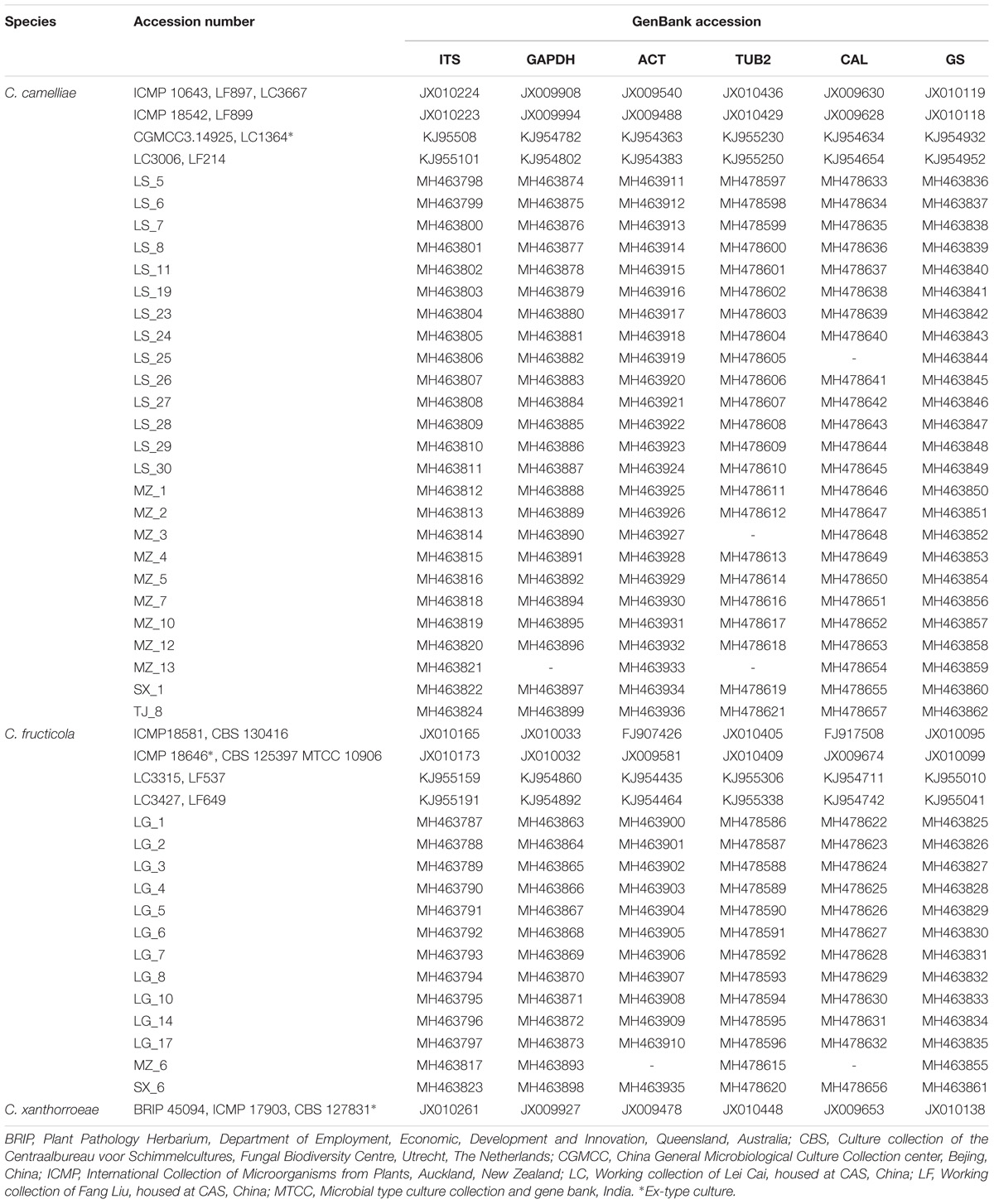
Table 2. Isolates of the Colletotrichum spp. studied and GenBank accession numbers of the generated sequences.
Pathogenicity Tests
In this study, the pathogenicity experiments were based on the method described by Wang et al. (2016a). First, healthy, coincident, nonwounded mature tea leaves were washed with tap water and then sterilized in 1% sodium hypochlorite for 3 min. Then, the tea leaves were punctured by sterile needles. Leaves inoculated with a conidial suspension (106 spores/mL) were used as the treated samples, and leaves inoculated with sterile water were used as the controls. The inoculated samples were added to 12 cm plastic Petri dishes and cultured in a growth cabinet at 25°C with a 12/12 h (day/night) photoperiod for 7 days. Finally, Koch’s postulates were employed to investigate the conidia of each strain.
Plant Material and Light Microscopy
Tea plants (Ca. sinensis cv. Longjing 43) (LJ43) were obtained from the Tea Research Institute of the Chinese Academy of Agricultural Sciences, Hangzhou, Zhejiang Province, China. Plants infected with the selected strains were grown at 25°C and 90% relative humidity with a 12/12 h photoperiod. Samples were collected at 12, 24, 48, 72, and 96 h postinoculation (hpi).
To visualize fungal colonization on host tissues, samples were destained in ethanol-chloroform (3:1, vol/vol) and stained with 0.025% (wt/vol) aniline blue in lactophenol (O’Connell et al., 2004). For microscopic observation, the samples were mounted on glass slides in 50% glycerol and examined under a Nikon 80i microscope (Japan) equipped with a differential interference contrast (DIC) objective.
In vitro Effects on Mycelial Growth in CC and CF
The effects of the tea polyphenols (TP), catechins and caffeine (Aladdin, China) on mycelia growth in CC and CF were assessed using the mycelial growth rate method in solid media (Zhang et al., 2014). The compounds were dissolved in distilled water and then mixed with sterile melting PDA medium to obtain final concentrations ranging from 0.03125 to 16 mg⋅mL−1. The PDA medium was poured into 90 mm diameter Petri plates that were then inoculated with 7 mm discs of CC and CF. Each treatment was performed in triplicate, and PDA plates containing distilled water were used as the control. Mycelial growth diameters were measured. The relative inhibition ratio was calculated using the following formula: I (%) = [(C−d)–(T−d)]/(C−d) × 100, where I (%) is the inhibition, d is the diameter of the fungal disc (7 mm), C and T are the colony diameters of the control and treatment, respectively.
Environmental Impact on Fungal Growth
The effect of temperature on fungal growth was explored. PDA was poured into 90 mm diameter sterile plates. Hyphae was picked from the slant culture with a sterilized wire loop and inoculated on a PDA plate. The isolates were incubated on PDA for 5 days at 15, 20, 25, 30, 35, and 40°C in the dark. Each treatment was performed in triplicate and was incubated separately. After incubation for 5 days, the colony diameters were recorded (Chavan and Dhutraj, 2017).
The cultural characteristics of the isolates were studied under different pH conditions. Various amounts of hydrochloric acid and sodium hydroxide were used to regulate the pH of the PDA medium. The final pH values of the media were 5, 6, 7, 8, 9, and 10. After the agar solidified, 5 mm discs of Colletotrichum strains from the actively growing cultures were removed using a sterile puncher. Each treatment was performed in triplicate, and the plates were incubated at 20°C in the dark for 5 days, after which the colony diameters were recorded (Liu, 2013).
RNA Extraction, PacC/Rim101 Identification, and qRT-PCR Analysis
Hyphae used in the qRT-PCR experiment were grown in potato dextrose broth (PDB) medium under different pH conditions at 25°C. Total RNA was extracted from hyphae cultivated in PDB medium using the Fungal Total RNA Isolation Kit (Sangon Biotech) according to the manufacturer’s instructions. Total RNA (1 μg) was used in the cDNA reactions with the PrimeScriptTM II 1st Strand cDNA Synthesis Kit (TaKaRa). The cDNA products were diluted 10-fold with RNAase-free water before being used as a template for real-time PCR. The reaction mixture contained 5 μL of SYBR Green, 0.8 μL of the forward and reverse primers, 3.2 μL of double-distilled water and 1 μL of template cDNA. The PCR amplification was performed with a LightCycler® 480 system (Roche) according to the manufacturer’s instructions. The PacC/Rim101 gene was identified by OrthoVenn using comparative genome analysis (E-value: 1e-10) (Wang et al., 2015). The C. gloeosporioides (Cg-14) genome was downloaded from GenBank (accession number SUB133583). CC and CF have been sequenced (unpublished data). The homologous gene of PacC/Rim101 in other Colletotrichum species were identified by conducting BLASTP search of PacC/Rim101 gene sequences against the annotated Colletotrichum protein sequences. The following primers for PacC/RIM101 were used in the quantitative PCR analysis: 5′-CGATCACCTCAGGACCAGGGGA-3′ and 5′-CGGGGAGGGTTCAAGGGTTGTT-3′. Each experiment was performed in triplicate. For each treatment, one representative set of results is presented as the mean2−ΔΔCTvalue ± SEM.
Statistical Analysis
The experimental data were subjected to analysis of variance (ANOVA) using the SPSS 18 software (IBM). The mean values were compared by the least significant difference (LSD) method, and differences were considered significant at P < 0.05. All of the data are represented as the mean ± SEM of at least three independent experiments.
Results
Identification and Characterization of the Isolates
In our study, we collected 38 isolates of Colletotrichum from tea plants in various regions of Zhejiang Province. Phylogenetic trees (Figure 1A) were constructed using the combined ITS, ACT, GAPDH, TUB2, CAL, and GS gene data that encompassed 47 Colletotrichum strains, including C. xanthorrhoeae (CBS 127831) as the outgroup. The combined gene alignment, including gaps, comprised 3135 characters. The isolates were found to belong to two subclades, namely, CC and CF. Of our 38 isolates, 25 clustered with the CC ex-type culture (LC1364), and 13 clustered with the type strain of CF (ICMP 18646). The phylogenetic tree had Bayesian posterior probability values ≥ 0.95.
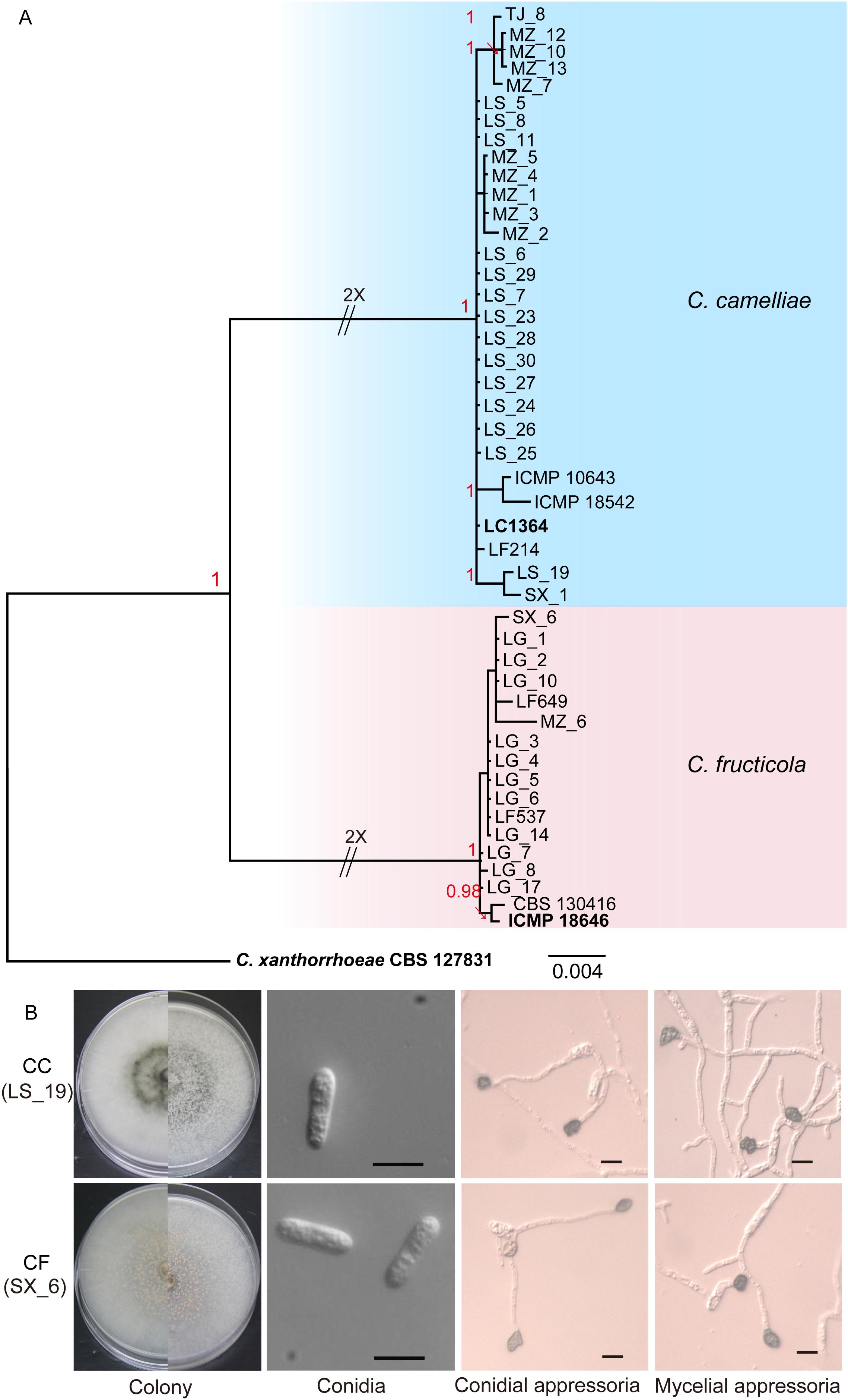
Figure 1. Isolation and identification of Colletotrichum. (A) Phylogenetic tree generated by Bayesian analysis based on a 6-gene combined dataset (ITS, ACT, GAPDH, TUB2, CAL, and GS) showing the phylogenetic relationships of Colletotrichum isolates from Camellia sinensis. Bayesian posterior probabilities above 0.95 are shown at each node. C. xanthorrhoeae CBS 127831 was used as the outgroup. The scale bar indicates 0.004 expected changes per site. The branches crossed by diagonal lines are shortened by 50%. The ex-type strains are emphasized in bold: LG (Longguan), MZ (Maozhuyuan), and TJ (Tianjian) represent three different sampling points in Hangzhou. LS and SX represent Lishui and Shaoxin, respectively. (B) Morphological structures of C. camelliae (strain LS_19) and C. fructicola (strain SX_6). Scale bar = 10 μm.
The isolates were cultured on PDA plates and SNA culture medium (in triplicate) for the collection of conidia and appressoria, respectively. Three representative isolates of each species were selected for a survey of their morphological characteristics. On PDA, the CC colonies grew flat with an entire edge with white pigmentation becoming gray toward the center. The pigmentation of the center was gray-white and black on the reverse side; fewer acervuli developed, and these were hidden by the dense mycelia. The CC growth rate was 12.28–13.81 mm/d. On PDA, the CF colonies grew flat with white pigmentation, the reverse side was white, and orange conidial ooze was distributed over the colonies. The CF growth rate was 12.64–13.71 mm/d. The conidial ooze from CF was observed on PDA plates incubated for 3 days at 25°C. However, CC did not produce conidial ooze (Figure 1B and Table 3). We found that the isolates of CF were more capable of producing spores than were the CC isolates.
The appearance of the conidia and appressoria of these two species was similar. The conidia were hyaline, aseptate, smooth, and cylindrical with obtuse ends. For both CC and CF, the appressoria generated on SNA were irregularly shaped, crenate, brown to dark brown, and branched. The length-width ratio of the representative isolates LS_19 and SX_6 was the closest to that of their respective ex-type cultures (Liu et al., 2015). LS_19 (a strain of CC) produced conidia measuring 11.7–27.6 × 4.6–6.2 μm (average (av) ± SEM = 17.2 ± 0.6 × 5.0 ± 0.1 μm, n = 30) and appressoria measuring 7.2–11.3 × 6.2–8.1 μm (av ± SEM = 9.8 ± 0.2 × 7.1 ± 0.1 μm, n = 30). SX_6 (a strain of CF) produced conidia measuring 14.1–18.9 × 3.9–5.6 μm (av ± SEM = 16.5 ± 0.2 × 4.6 ± 0.1 μm, n = 30) and appressoria measuring 8.7–12.2 × 5.7–8.6 μm (av ± SEM = 10.0 ± 0.2 × 6.9 ± 0.1 μm, n = 30). Therefore, LS_19 and SX_6 were further used as the representatives CC and CF, respectively, for inoculation.
Pathogenicity Tests on Tea Plant Leaves in vitro
The pathogenicity of the Colletotrichum strains was tested on leaves of tea plants (LJ43) (Figure 2A). Based on pathogenicity studies with tea leaves, significant differences in virulence were observed between CC (LS_19) and CF (SX_6). The CC produced irregular, brown decay lesions around the wound areas. The necrotic lesions were first observed at 3 days postinoculation (dpi); these lesions enlarged over time, and sporogenous structures emerged at 7 dpi. Nonetheless, the SX_6 did not produce the typical lesions of anthracnose disease during the experiment, while LS_19 produced. Following Koch’s postulates, conidia from the lesions were recultivated on PDA medium, and characteristics of these conidia accorded with the original strains after identification. The results suggested that the interspecies virulence was distinct and that CC was more invasive than CF.
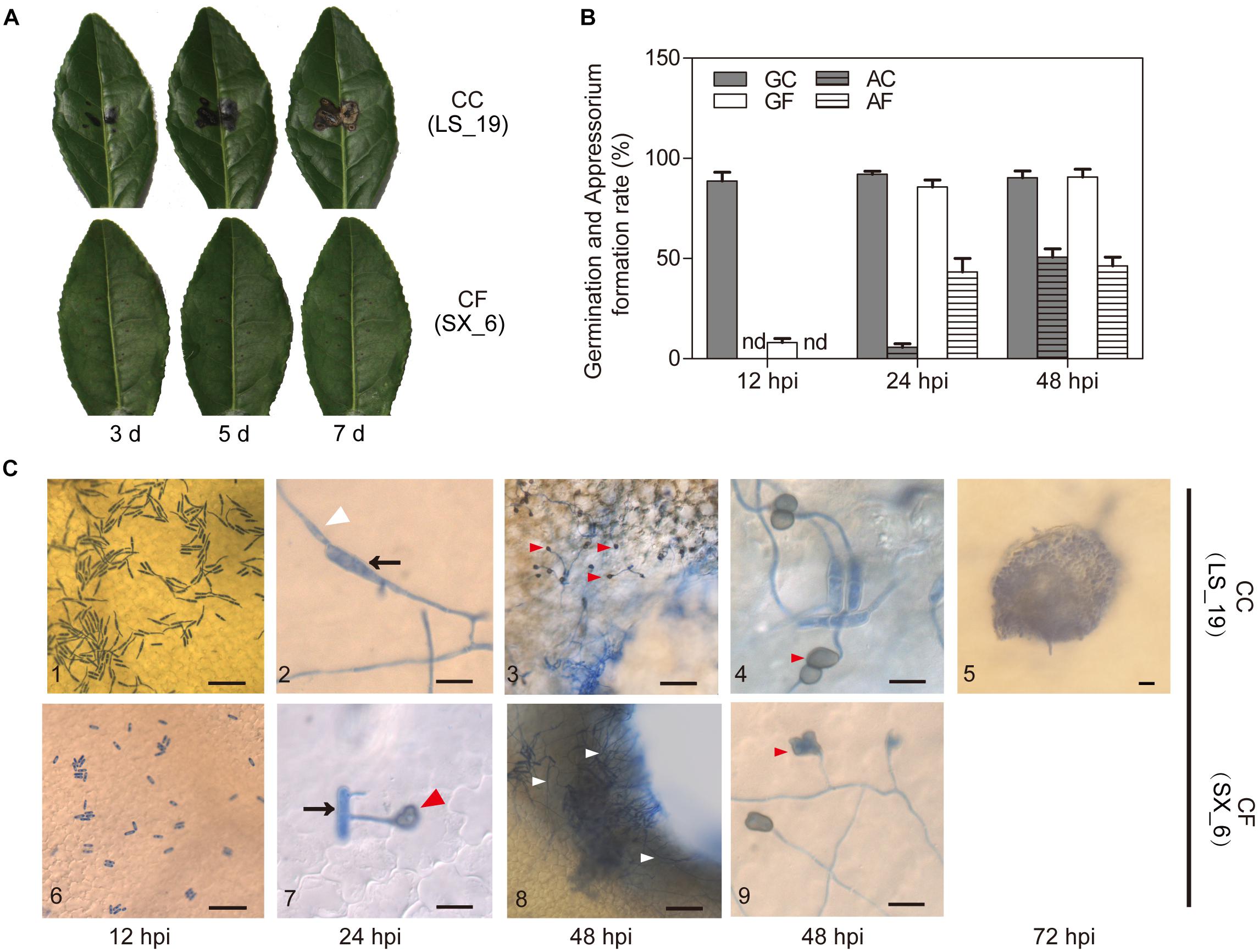
Figure 2. Pathogenicity and inoculation. (A) Pathogenicity tests of C. camelliae (strain LS_19) and C. fructicola (strain SX_6) on tea plant leaves after 3, 5, and 7 days. (B) Quantification of germinated conidia and appressoria per 100 conidia. GC, Germination of C. camelliae. AC, Appressoria of C. camelliae. GF, Germination of C. fructicola. AF, Appressoria of C. fructicola. nd, not detectable. The error bars represent the SEM; n = 100. (C) Microscopic examination of fungi stained with aniline blue. C3 and C8 show the wound areas; C4 and C9 show the nonwounded segment. C10 shows an acervul us at 72 hpi. The arrows indicate conidia, the white triangles indicate hyphae, and the red triangles indicate appressoria. Scale bar = 50 μm in C1, C3, C5, C6, and C8; scale bar = 10 μm in C2, C4, C7, and C9.
Observation of CC and CF Infection Progress
To clarify the reasons what were the difference of infection progress of CC and CF, the wounded LJ43 leaves were sprayed with a spore suspension (106 spores/mL) for microscopic observation at 12, 24, 48, and 72 hpi. At 12 hpi, the germination rate of LS_19 and SX_6 was 88.7 and 12%, respectively. Appressoria of both species were first seen at 24 hpi, with an occurrence per 100 conidia of 5.7 for LS_19 and 43.3 for SX_6. Over time, the number of germinated conidia and appressoria in both species became no difference (Figure 2B).
Aniline blue was used to visualize the infection structures both species (Figure 2C). We found that both species could grow on the surface of tea leaves at early stages of inoculation (0–24 hpi). At 48 hpi, LS_19 and SX_6 developed filamentous mycelia. We found that a great quantity of hyphae appeared around the wound areas. Interestingly, SX_6 did not develop appressoria around the wounds. On the other hand, we observed appressoria of both species on intact parts of the tea leaves at 48 hpi. As time progressed, LS_19 developed acervuli, which are reproductive structures that are essential for pathophoresis, whereas SX_6 did not differentiate the analogous structures. As the above result showed, the times of germination and appressoria formation of the two species were clearly divergent at the early stage of inoculation. However, LS_19 developed acervuli, which contributed to lesion enlargement.
The thick cuticle of tea plants impedes penetration by pathogens. Therefore, we believed that infection of the necrotic tissues or wound areas, such as the fringe of the wound areas, was beneficial. This result suggested that LS_19 formed appressoria on wound areas and that this step was critical for its pathogenicity.
Effects of Compounds on Mycelial Growth in vitro
We focused on the antifungal activity of caffeine and catechins, which are the most important functional components in tea plants and play a role in plant defense, to evaluate the antifungal activity (Figures 3A,B). The results showed that the inhibition rates of CC and CF were elevated as the concentration of the two compounds increased. Compared with the inhibition rates after 1 day treatment, the antifungal activity decreased after 2 days of treatment. Inhibition rates of CC and CF reached to 100% under the 16 mg⋅mL−1 catechins treatment. Inhibition rates of CC and CF exhibit no significant difference at the higher concentrations. However, when the concentration of catechins decreased to 1 mg⋅mL−1, the mycelia growth of CF was inhibited to a greater extent than was that of CC. Compared with CC, CF had a higher inhibition rates when fungi were treated with caffeine from 0.0625 to 0.5 mg⋅mL−1. Therefore, CF was more vulnerable to catechins and caffeine.
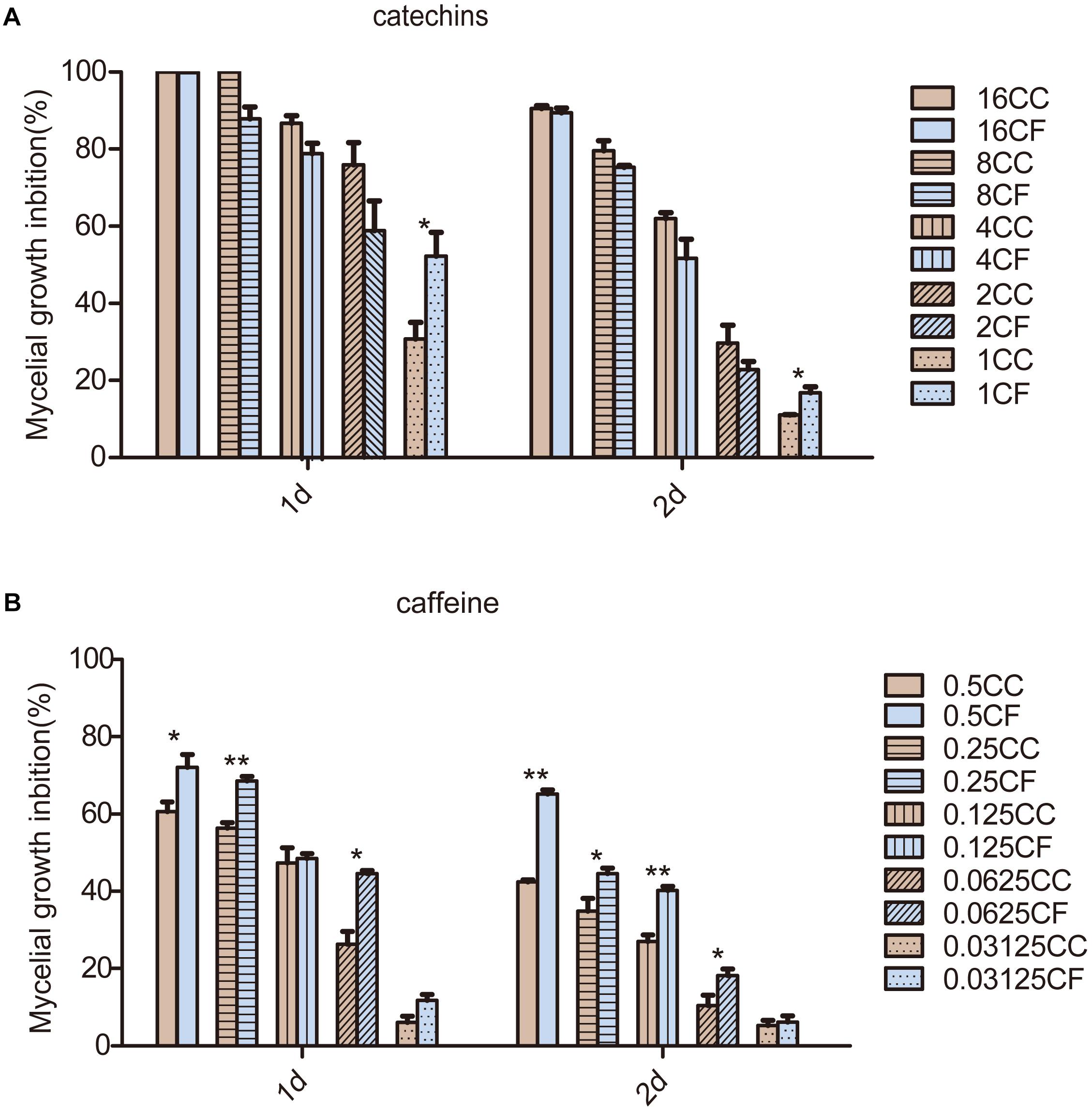
Figure 3. Effects of two compounds on mycelia growth of C. camelliae and C. fructicola in vitro. (A) The mycelial growth inhibition of CC and CF by catechins; (B) The mycelia growth inhibition of CC and CF by caffeine. The numbers in the legend represent treatment concentrations (mg⋅mL−1); ∗P < 0.05 and ∗∗P < 0.01 by the LSD test. The error bars represent SEM; n = 3.
Effect of Temperature on Mycelial Growth
To test the effect of temperature on the CC and CF mycelial growth, both three strains of these two fungi were grown on PDA medium at different temperatures (Figures 4A,B). The diameter of the CC colonies reached a maximum at 25°C and significantly exceeded that at all other temperatures. The minimum diameter resulted at 15°C. At 30°C, the intraspecific variations in the mycelial growth of the CC colonies were significant. The colony growth was arrested at temperatures higher than 35°C. The maximal diameter of CF occurred at 25 and 30°C. The CF colony diameters at 25 and 30°C were not significant difference, except for that of LG_5. Colony growth continued slowly at 35°C, at which temperature the minimum diameter was observed, and growth was arrested at 40°C. These results illustrated that CC was sensitive to temperature change and that CF grew in a wide permissive temperature range.
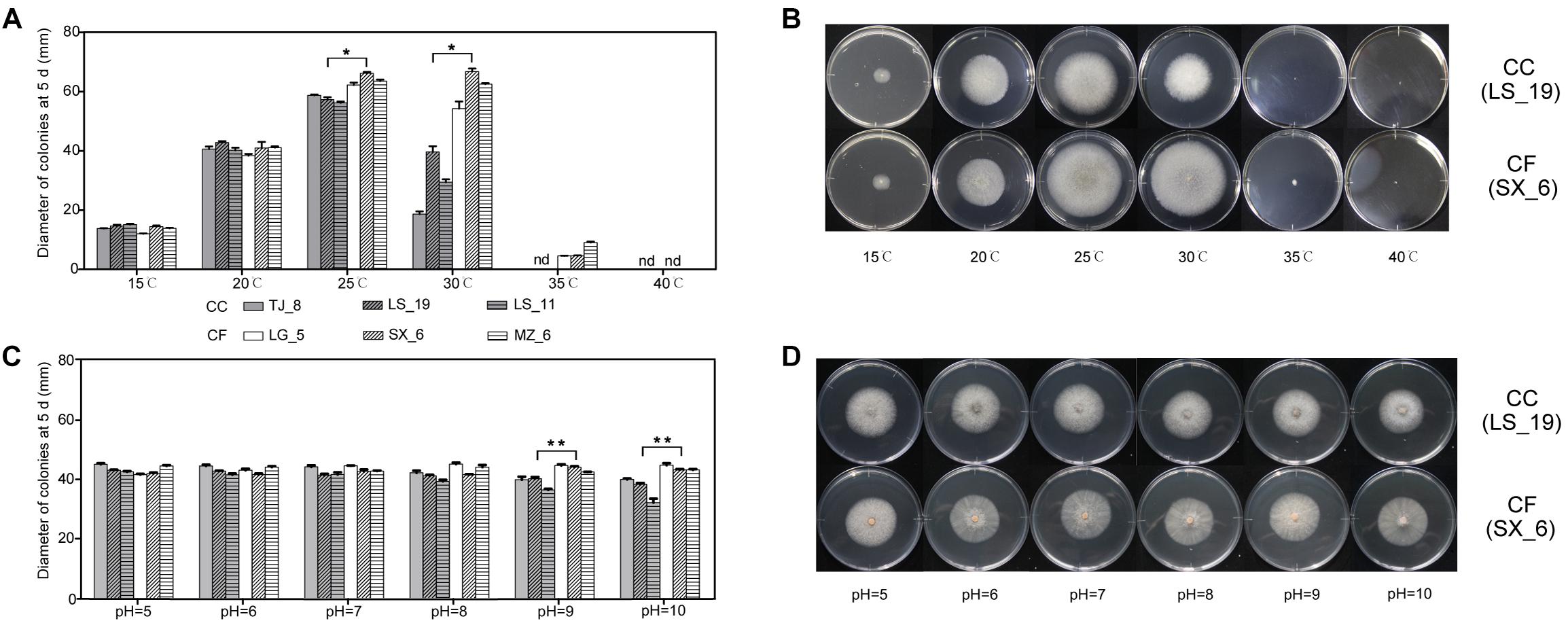
Figure 4. Effect of environmental factors on the growth of C. camelliae and C. fructicola. (A,B) Effect of temperature. (C,D) Effect of pH. ∗P < 0.05 and ∗∗P < 0.01 by the LSD test (comparing LS_19 and SX_6); nd, not detectable. The error bars represent SEM; n = 3.
The growth characteristics of the typical CC and CF strains LS_19 and SX_6 at different temperatures were compared. The growth rate was not significantly different between LS_19 and SX_6 at low temperatures (15 and 20°C). The SX_6 isolate was the fastest-growing isolate at 25 and 30°C, at which temperatures its diameter was significantly larger than that of LS_19.
Effect of pH Condition on Mycelial Growth
These six strains were grown on media at different pH values (pH = 5, 6, 7, 8, 9, and 10) at 20oC to explore the effects of the acid and base environments on colony growth (Figures 4C,D). The fungi could grow over the tested pH range. Each strain had a slightly different response to each pH except pH 9 and 10, at which the growth of CC was significantly slowed. Thus, the growth of CC was inhibited under a strong alkaline environment. The diameter of CF surpassed that of CC at pH 9. With increasing of pH, the difference in diameter between CC and CF increased. The diameter of LS_19 was lower under a strong alkaline environment. However, SX_6 responded satisfactorily to alternative pH conditions; this phenomenon was also observed for the other CC and CF strains. Both species grew normally in weakly alkaline environments. From the effects of these temperatures and pH conditions on growth, we suggested that CC was more sensitive to environmental change than was CF.
To confirm the effect of external pH on fungal growth, the PacC/RIM101 was identified based on comparative genome analysis. The reported 961 PacC genes in the Cg-14 genome (Alkan et al., 2013) were compared with those in the CC and CF genomes (unpublished data from our lab). The Venn diagram shows that 614 orthologs gene clusters were found among the three species, and one of the orthologs genes was annotated as a pH response regulator protein (Figure 5A). In order to verify the phylogenetic relationship of this obtained PacC/RIM101 gene in Colletotrichum genus, an neighbor-joining (NJ) tree was constructed using the protein sequences of 17 representative Colletotrichum species, the results showed that the CC, CF and C. gloeosporioides were clustered together, as well as belonged to C. gloeosporioides species complex (Figure 5B). Among them the identified sequence was translated into putative polypeptides of 585 and 584 amino acids in CC and CF, respectively, and the PacC/RIM101 amino acid sequences of CC and CF have very high homology (98.5% identity) and similar length (Figure 5C). The PacC/RIM101 gene associated with pathogenicity was identified from CC and CF genomes, The expression of this gene in vitro was downregulated in the hyphae of both species under pH value of 5 and 10, and lower transcript abundance was detected in CC than that in CF at pH 6 (Figure 5D). Totally, a homologous PacC/RIM101 gene were obtained from CC and CF based on comparative genomes, but the qRT-PCR results suggested that this gene was not associated with CC and CF response to acid base environment in vitro.
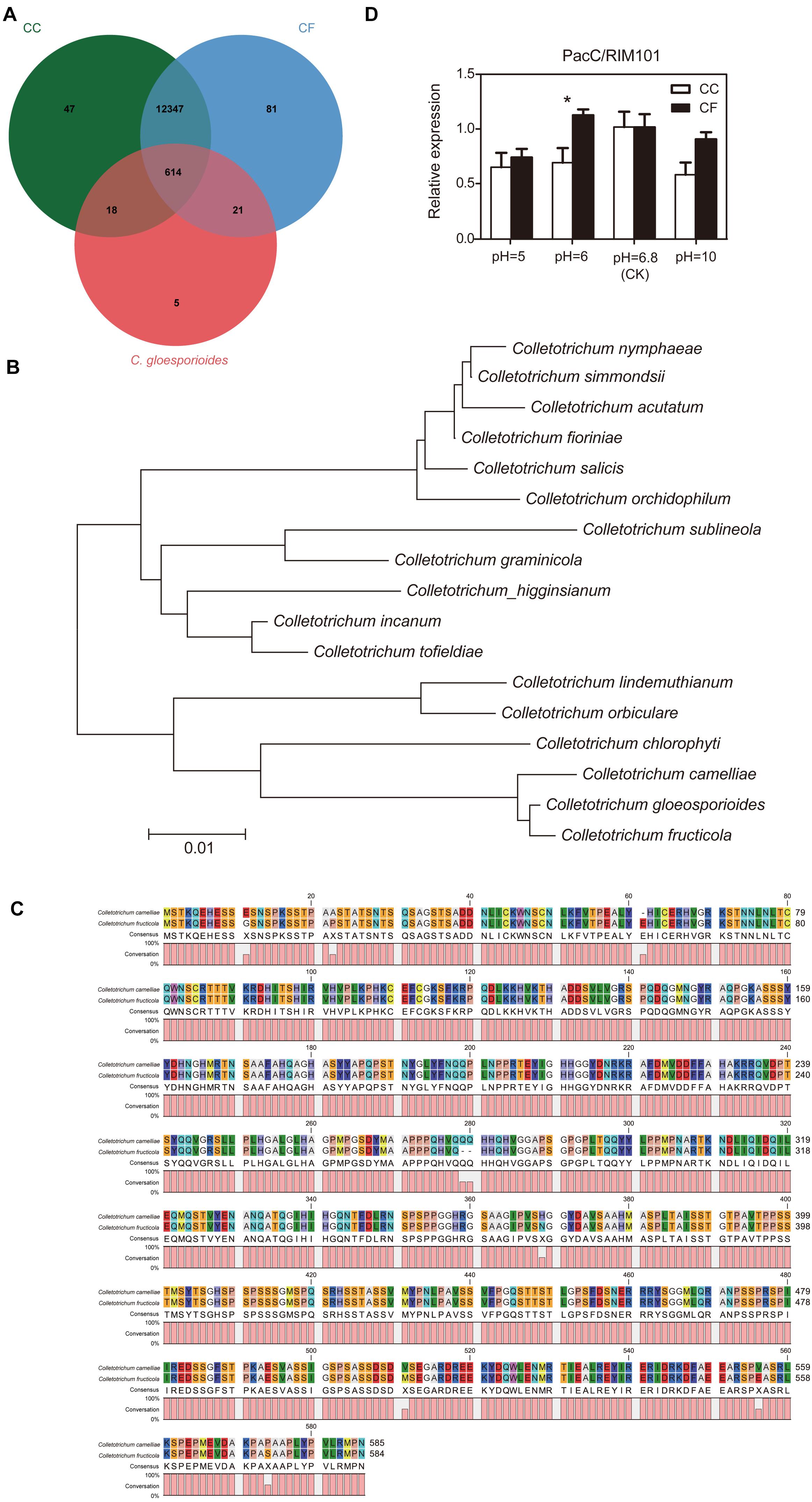
Figure 5. Analysis of PacC/RIM101 expression in C. camelliae and C. fructicola. (A) Venn diagram of the genome summarizing the numbers of sequences among the three species. CC, C. camelliae. CF, C. fructicola. (B) Phylogenetic tree relating PacC/RIM101 orthologs. This tree was constructed with MEGA v 5.0 using the neighbor-joining method. (C) Sequence alignment of PacC/RIM101 genes using Clustal Omega (https://www.ebi.ac.uk/Tools/msa/clustalo/) and CLC Sequence Viewer 8. (D) Quantitative real-time polymerase chain reaction (qRT-PCR) analysis showing the relative expression of the PacC/RIM101 gene presented as the average and SEM. CC was represented by the LS_19 strain; CF was represented by the SX_6 strain.
Discussion
Identification and Characterization of the Isolates
Anthracnose, caused by species of the genus Colletotrichum, is one of the most important diseases affecting tea plants and leads to economic losses (Guo et al., 2014; Liu et al., 2015). According to previous studies, 17 species of Colletotrichum have been reported from tea plants (Liu, 2013; Liu et al., 2015; Wang et al., 2016a). CC and CF were previously proposed to be the dominant species causing anthracnose in tea plants in China (Cai et al., 2009; Guo et al., 2014; Wang et al., 2016a; Liu et al., 2017). In our present research, we isolated 38 Colletotrichum strains that belonged to the CC and CF species. In our combined analysis of morphological features and multigene molecular phylogeny, 25 of these isolates clustered with the ex-type culture of CC, and 13 clustered with the type strain of CF. These strains were isolated from three different cities in Zhejiang Province. We obtained only CC in Lishui but found both species in Hangzhou and Shaoxin. Twenty-two of these isolates were collected from Hangzhou. We found only CF in LG region and the majority of isolates in MZ region were CC. The dominant species varied among sampling locations. All isolates had obvious interspecific differences. CC displayed dense mycelia and little sporulation. In contrast, CF, for which orange conidial ooze was observed, possessed a substantial capability to produce spores on PDA culture medium.
CC Was More Virulent Than CF
Pathogenicity is the potential capacity of a certain species to cause a disease. The primary symptom of Colletotrichum infection on tea plants is an irregular dark brown lesion. Initially, the affected tea leaves will develop circular lesions that subsequently continue to expand. Finally, the junction of diseased and healthy tissue forms a shape resembling water spots, and sporadic black specks appear on the surface of the scab (Luo et al., 2010). Previous researchers used various standards to measure the pathogenicity of pathogens. The time to the appearance of the lesion or acervuli is one of the standards for evaluating the virulence of the pathogen (Sangdee et al., 2011). The earlier a fungus generates acervuli, the higher its virulence. In our research, leaves inoculated with CC began to develop lesions 3 dpi, and these spots continued to grow over time. CC first developed acervuli at 72 hpi in our inoculation experiment in vitro. However, CF did not develop obvious lesions or acervuli during the experiment. These results showed that CC is highly virulent, and are consistent with those of previous studies (Sharma et al., 2015; Wang et al., 2016a).
Over its long evolutionary history, Colletotrichum forms some specialized infection structures. The appressorium is an important factor in the fungus’ ability to invade the host plant (Perfect et al., 1999; O’Connell et al., 2004). In our inoculation experiments in vitro, we found that both species formed appressoria on undamaged areas. However, CF did not develop appressoria on wound areas. in the previous reports considered hydrophobicity and surface hardness to be crucial for the initiation of appressoria and that wax monomers could induce appressorium development (Wilson and Talbot, 2009; Ryder and Talbot, 2015). Therefore, we speculated that CC and CF activated discrepant response mechanisms to develop appressoria in wound areas where leaf structures, metabolites and other properties have been changed. Appressoria not only exert physical forces to pierce the host surface but also secrete effectors from the appressorial penetration pores (Irieda and Takano, 2014; Ryder and Talbot, 2015). Virulence effectors in Colletotrichum species likely have critical roles in the manipulation of the host plant (Kleemann et al., 2008). In our study, we suggested that the difference in appressorium development between CC and CF leaded to pathogenic variation between these two species.
Secondary Metabolites Play the Important in Inhibition
During the tea plant – Colletotrichum interaction, abundant secondary metabolites in tea plants play a role in plant defense. In the previous study, caffeine provided tea plants significant resistance to anthracnose by impairing mycelia cell walls and plasma membranes (Wang et al., 2016b). Epicatechin can increase the disease resistance of avocado fruit by decreasing the activity of PG, PL and lipoxygenase of C. gleosporioides (Prusky et al., 1988; Guetsky et al., 2005). Our results indicated that catechins and caffeine affected the mycelia growth of CC and CF (Figures 3A,B). A difference in inhibition rate between CC and CF was detected only in PDA culture with 1 mg⋅mL−1 catechins. Under caffeine treatment, the inhibition rate of CF was significantly higher than that of CC under concentrations of 0.0625–0.5 mg⋅mL−1. The inhibition rate of CF was significantly greater than that of CC under the caffeine treatment. Taken together, our results revealed that the growth of CF was more vulnerable that was that of CC to catechins and caffeine. According to above studies, secondary metabolites play the important role in tea plant defense to different Colletotrichum species through inhibiting the growth of pathogen.
Environment Conditions Affect Growth of CC and CF
Environmental condition is one of the vital factors that affect fungal growth. Temperature plays an important role in the development of anthracnose disease through its effects on conidial germination and appressorium development. In previous studies, the optimum temperature of C. truncatum isolated from soybean was 25°C, whereas that of C. siamense, C. fioriniae, and C. karstii collected from tea plants was 26–28°C (Liu, 2013; Chavan and Dhutraj, 2017). In the present study, the range of temperatures at which CC could grow and its optimum growth temperature were 15–30°C and 25°C, respectively. The optimal temperature for CF growth is 25–30°C; when temperatures exceed 35°C, mycelium stopped developing. From the effects of temperature, we found that isolates of CC were sensitive to environmental changes, whereas isolates of CF had a wider range of adaptation. Although the CF has stronger adaptability than CC, its pathogenicity was weaker. Hence, we will further clarify the role of temperature on the pathogenesis of these two pathogens.
The pH is another environmental factor that influences fungal growth and infection capacity. In our study, the results showed that both CC and CF can grow normally in environments ranging from acidic to weak alkaline environments. No notable differences in growth were seen between CC and CF between pH 5 and pH 8, but a downward trend in CC was observed when the pH reached 9. When Colletotrichum colonizes the surface of the host plant, the alkalization of the surroundings by ammonia is critical for conidial differentiation (You et al., 2007; Miyara et al., 2010; Alkan et al., 2015). When host was attacked by the fungus, the pH of infection site increased to 8 (Yakoby et al., 2000; Prusky et al., 2001). Under pH 8, both of species have no significant difference in growth rate. PacC/Rim101 gene can mediate pH regulation and regulate growth, differentiation, and virulence in some fungi by transcriptional activation or suppression (Peñalva and Arst, H. N. Jr., 2004; You et al., 2007). In our study, we identified PacC/Rim101 gene from Cg-14, CC, and CF genome in order to explore its effects. We found that CF is more closely related to C. gloeosporioides than to CC. The relative expression of PacC/Rim101gene in CC and CF had significant difference at 6. With the increasing the pH value, their expression tended to no obvious difference. Therefore, we suggested that the pH value is not the major reason causing the pathogenicity difference between the two pathogens. PacC/Rim101 may be associated with pathogenicity in interaction of tea plant against Colletotrichum, but it still needs further study, such as screening for mutants, to proving genes’ function.
The results of this study are important because the widespread persistence of CC and CF on tea plant causes anthracnose to influence plant health. Both these species had prominent interspecific differences. The strains of CC were virulent and were the primary cause of anthracnose. Catechins and caffeine can inhibit CF growth more effectively. CF rarely formed lesions in the pathogenicity test and the in vitro inoculation experiments, whereas it had adapted well to varying temperature and acid-base conditions. According to the effect of pH and expression of related gene, infection ability of CC and CF may be less affected by pH changes. In the future, further study is required to explain the mechanism underlying pathogenicity differences between CC and CF.
Author Contributions
QL, XW, and YY designed the study. QL, YW, and NL performed the experiments. QL, YW, and DN analyzed the data. QL, YW, and XW wrote and revised the manuscript. QL, DN, XW, and YY revised the final version to be published. All authors read the manuscript.
Funding
The study was funded by the Earmarked Fund for China Agriculture Research System (CARS-19), the Major Project of Agricultural Science and Technology in Breeding of Tea Plant Varity in Zhejiang Province (2016C02053-4), the Chinese Academy of Agricultural Sciences through an Innovation Project for Agricultural Sciences and Technology (CAAS-ASTIP-2017-TRICAAS), China Postdoctoral Science Foundation Funded Project (2017M620970), and Zhejiang Province Postdoctoral Science Foundation.
Conflict of Interest Statement
The authors declare that the research was conducted in the absence of any commercial or financial relationships that could be construed as a potential conflict of interest.
Acknowledgments
We thank the Tea Experimental Stations of China Agriculture Research System in Lishui and Shaoxing.
References
Ahuja, I., Kissen, R., and Bones, A. M. (2012). Phytoalexins in defense against pathogens. Trends Plant Sci. 17, 73–90. doi: 10.1016/j.tplants.2011.11.002
Alkan, N., Friedlander, G., Ment, D., Prusky, D., and Fluhr, R. (2015). Simultaneous transcriptome analysis of Colletotrichum gloeosporioides and tomato fruit pathosystem reveals novel fungal pathogenicity and fruit defense strategies. New Phytol. 205, 801–815. doi: 10.1111/nph.13087
Alkan, N., Meng, X., Friedlander, G., Reuveni, E., Sukno, S., Sherman, A., et al. (2013). Global aspects of pacC regulation of pathogenicity genes in Colletotrichum gloeosporioides as revealed by transcriptome analysis. Mol. Plant Microbe Interact. 26, 1345–1358. doi: 10.1094/MPMI-03-13-0080-R
Antonin, C. C. J., Lucila, O. C., Miriam, T. S., Scott, G., and Jose, R. H. (2010). Functional analysis of the pH responsive pathway Pal/Rim in the phytopathogenic basidiomycete Ustilago maydis. Fungal Genet. Biol. 47, 446–457. doi: 10.1016/j.fgb.2010.02.004
Araujo, L., Bispo, W. M. S., Rios, J. A., Fernandes, S. A., and Rodrigues, F. D. Á (2016). Alkaloids and phenolics biosynthesis increases mango resistance to infection by Ceratocystis fimbriata. Bragantia 75, 199–211. doi: 10.1590/1678-4499.261
Asakura, M., Okuno, T., and Takano, Y. (2006). Multiple contributions of peroxisomal metabolic function to fungal pathogenicity in Colletotrichum lagenarium. Appl. Environ. Microbiol. 72, 6345–6354. doi: 10.1128/AEM.00988-06
Carbone, I., and Kohn, L. M. (1999). A method for designing primer sets for speciation studies in filamentous ascomycetes. Mycologia 91, 553–556. doi: 10.2307/3761358
Cai, L., Hyde, K. D., Taylor, P., Weir, B. S., Waller, J. M., Abang, M. M., et al. (2009). A polyphasic approach for studying Colletotrichum. Fungal Divers. 39, 183–204.
Cannon, P. F., Damm, U., Johnston, P. R., and Weir, B. S. (2012). Colletotrichum – current status and future directions. Stud. Mycol. 73, 181–213. doi: 10.3114/sim0014
Chavan, S., and Dhutraj, D. N. (2017). Effect of cultural media and temperature on growth and sporulation of Colletotrichum truncatum of soybean in vitro. Int. J. Appl. Pure Sci. Agric. 3, 25–30.
Damm, U., Woudenberg, J. H. C., Cannon, P. F., and Crous, P. W. (2009). Colletotrichum species with curved conidia from herbaceous hosts. Fungal Divers. 39, 45–87.
Deising, H. B., Werner, S., and Wernitz, M. (2000). The role of fungal appressoria in plant infection. Microbes Infect. 2, 1631–1641. doi: 10.1016/S1286-4579(00)01319-8
Emmett, R. W., and Parbery, D. G. (1975). Appressoria. Annu. Rev. Phytopathol. 13, 147–165. doi: 10.1146/annurev.py.13.090175.001051
Gardes, M., and Bruns, T. D. (1993). ITS primers with enhanced specificity for basidiomycetes - application to the identification of mycorrhizae and rusts. Mol. Ecol. 2, 113–118. doi: 10.1111/j.1365-294X.1993.tb00005.x
Glass, N. L., and Donaldson, G. C. (1995). Development of primer sets designed for use with the PCR to amplify conserved genes from filamentous ascomycetes. Appl. Environ. Microbiol. 61, 1323–1330.
Guetsky, R., Kobiler, I., Wang, X., Perlman, N., Gollop, N., Avila-Quezada, G., et al. (2005). Metabolism of the flavonoid epicatechin by laccase of Colletotrichum gloeosporioidesand its effect on pathogenicity on avocado fruits. Phytopathology 95, 1341–1348. doi: 10.1094/phyto-95-1341
Guo, M., Pan, Y. M., Dai, Y. L., and Gao, Z. M. (2014). First report of brown blight disease caused by Colletotrichum gloeosporioides on Camellia sinensis in Anhui Province, China. Plant Dis. 98, 284–284. doi: 10.1094/PDIS-03-13-0276-PDN
Hyde, K. D., Cai, L., McKenzie, E. H. C., Yang, Y. L., Zhang, J. Z., and Prihastuti, H. (2009). Colletotrichum: a catalogue of confusion. Fungal Divers. 39, 1–17.
Irieda, H., and Takano, Y. (2014). Colletotrichum orbiculare secretes virulence effectors to a biotrophic interface at the primary hyphal neck via exocytosis coupled with SEC22-mediated traffic. Plant Cell 26:2265. doi: 10.1105/tpc.113.120600
Kim, Y.-S., Choi, Y.-E., and Sano, H. (2010). Plant vaccination: stimulation of defense system by caffeine production in planta. Plant Signal. Behav. 5, 489–493. doi: 10.4161/psb.11087
Kleemann, J., Rincon-Rivera, L. J., Takahara, H., Neumann, U., Themaat, E. V. L. V., van der Dose, H. C., et al. (2012). Correction: sequential delivery of host-induced virulence effectors by appressoria and intracellular hyphae of the phytopathogen Colletotrichum higginsianum. PLoS Pathog. 8:e1002643. doi: 10.1371/annotation/0f398a0c-dfda-4277-b172-4ff9cb31aec3
Kleemann, J., Takahara, H., Stüber, K., and O’Connell, R. (2008). Identification of soluble secreted proteins from appressoria of Colletotrichum higginsianum by analysis of expressed sequence tags. Microbiology 154, 1204–1217. doi: 10.1099/mic.0.2007/014944-0
Ko, M., Cho, J. H., Seo, H. H., Lee, H. H., Kang, H. Y., Nguyen, T. S., et al. (2016). Constitutive expression of a fungus-inducible carboxylesterase improves disease resistance in transgenic pepper plants. Planta 244, 1–14. doi: 10.1007/s00425-016-2514-6
Kuc, J. (1995). Phytoalexins, stress metabolism, and disease resistance in plants. Annu. Rev. Phytopathol. 33, 275–297. doi: 10.1146/annurev.py.33.090195.001423
Li, M., Martin, S. J., Bruno, V. M., Mitchell, A. P., and Davis, D. A. (2004). Candida albicans Rim13p, a protease required for Rim101p processing at acidic and alkaline pHs. Eukaryot. Cell 3, 741–751. doi: 10.1128/EC.3.3.741-751.2004
Liu, F., Weir, B. S., Damm, U., Crous, P. W., Wang, Y., Liu, B., et al. (2015). UnravellingColletotrichumspecies associated with Camellia: employing ApMat and GS loci to resolve species in the C. gloeosporioides complex. Persoonia 35, 63–86. doi: 10.3767/003158515X687597
Liu, W. (2013). Anthracnose Pathogens Identification and the Genetic Diversity of Tea Plant Master. Fuzhou: Fujian Agriculture and Forestry University.
Liu, W., Yuan, D., Guo, G. Y., Yang, G. Y., and Ye, N. X. (2017). Identification of anthracnose pathogen in tea plant. J. Southern Agric. 48, 448–453.
Liu, W., Yuan, D., Yin, P., Wang, Z., and Guo, G. (2016). Research Progress on Anthracnose of Tea Plant. Chin. J. Trop. Agric. 36, 20–26.
Luo, Y., Wu, J., and Chen, J. (2010). Identification and prevention of main diseases of tea tree. Plant Doct. 23:17. doi: 10.13718/j.cnki.zwys.2010.01.012
Mackenzie, D. A., Jeenes, D. J., Belshaw, N. J., and Archer, D. B. (1993). Regulation of secreted protein production by filamentous fungi: recent developments and perspectives. J. Gen. Microbiol. 139:2295. doi: 10.1099/00221287-139-10-2295
Ment, D., Alkan, N., Luria, N., Bi, F. C., Reuveni, E., Fluhr, R., et al. (2014). A role of AREB in the regulation of PACC-dependent acid-expressed-genes and pathogenicity of Colletotrichum gloeosporioides. Mol. Plant Microbe Interact. 28:154. doi: 10.1094/MPMI-09-14-0252-R
Miyara, I., Shafran, H., Davidzon, M., Sherman, A., and Prusky, D. (2010). pH Regulation of ammonia secretion by Colletotrichum gloeosporioides and its effect on appressorium formation and pathogenicity. Mol. Plant Microbe Interact. 23, 304–316. doi: 10.1094/MPMI-23-3-0304
O’Connell, R., Herbert, C., Sreenivasaprasad, S., Khatib, M., Esquerré-Tugayé, M.-T., and Dumas, B. (2004). A novel Arabidopsis-Colletotrichum pathosystem for the molecular dissection of plant-fungal interactions. Mol. Plant Microbe Interact. 17, 272–282. doi: 10.1094/MPMI.2004.17.3.272
O’Connell, R. J., Bailey, J. A., and Richmond, D. V. (1985). Cytology and physiology of infection of Phaseolus vulgaris by Colletotrichum lindemuthianum. Physiol. Plant Pathol. 27, 75–98. doi: 10.1016/0048-4059(85)90058-X
O’Connell, R. J., Thon, M. R., Hacquard, S., Amyotte, S. G., Kleemann, J., Torres, M. F., et al. (2012). Lifestyle transitions in plant pathogenic Colletotrichum fungi deciphered by genome and transcriptome analyses. Nat. Genet. 44, 1060–1065. doi: 10.1038/ng.2372
O’Donnell, K., and Cigelnik, E. (1997). Two divergent intragenomic rDNA ITS2 types within a monophyletic lineage of the fungusfusariumare nonorthologous. Mol. Phylogenet. Evol. 7, 103–116. doi: 10.1006/mpev.1996.0376
Pasanen, A. L., Kalliokoski, P., Pasanen, P., Jantunen, M. J., and Nevalainen, A. (1991). Laboratory studies on the relationship between fungal growth and atmospheric temperature and humidity. Environ. Int. 17, 225–228. doi: 10.1016/0160-4120(91)90006-C
Peñalva, M. A., and Arst, H. N. Jr. (2004). Recent advances in the characterization of ambient pH regulation of gene expression in filamentous fungi and yeasts. Annu. Rev. Microbiol. 58, 425–451. doi: 10.1146/annurev.micro.58.030603.123715
Perfect, S. E., Hughes, H. B., O’Connell, R. J., and Green, J. R. (1999). Colletotrichum: a model genus for studies on pathology and fungal–plant interactions. Fungal Genet. Biol. 27, 186–198. doi: 10.1006/fgbi.1999.1143
Prusky, D., Kobiler, I., and Jacoby, K. (1988). Involvement of epicatechin in cultivar susceptibility of avocado fruits to Colletotrichum gloeosporioides after harvest. J. Phytopathol. 123, 140–146. doi: 10.1111/j.1439-0434.1988.tb04461.x
Prusky, D., Mcevoy, J. L., Leverentz, B., and Conway, W. S. (2001). Local modulation of host pH by Colletotrichum species as a mechanism to increase virulence. Mol. Plant Microbe Interact. 14, 1105–1113. doi: 10.1094/MPMI.2001.14.9.1105
Rao, S., and Nandineni, M. R. (2017). Genome sequencing and comparative genomics reveal a repertoire of putative pathogenicity genes in chilli anthracnose fungus Colletotrichum truncatum. PLoS One 12:e0183567. doi: 10.1371/journal.pone.0183567
Ryder, L. S., and Talbot, N. J. (2015). Regulation of appressorium development in pathogenic fungi. Curr. Opin. Plant Biol. 26, 8–13. doi: 10.1016/j.pbi.2015.05.013
Sangdee, A., Sachan, S., and Khankhum, S. (2011). Morphological, pathological and molecular variability of Colletotrichum capsici causing anthracnose of chilli in the North-east of Thailand. Afr. J. Microbiol. Res. 525, 4368–4372.
Sharma, G., Pinnaka, A. K., and Shenoy, B. D. (2015). Resolving the Colletotrichum siamense species complex using ApMat marker. Fungal Divers. 71, 247–264. doi: 10.1007/s13225-014-0312-7
Stephenson, S. A., Green, J. R., Manners, J. M., and Maclean, D. J. (1997). Cloning and characterisation of glutamine synthetase from Colletotrichum gloeosporioides and demonstration of elevated expression during pathogenesis on Stylosanthes guianensis. Curr. Genet. 31, 447–454. doi: 10.1007/s002940050228
Takahara, H., Hacquard, S., Kombrink, A., Hughes, H. B., Halder, V., Robin, G. P., et al. (2016). Colletotrichum higginsianum extracellular LysM proteins play dual roles in appressorial function and suppression of chitin-triggered plant immunity. New Phytol. 211, 1323–1337. doi: 10.1111/nph.13994
Templeton, M. D., Rikkerink, E. H. A., Solon, S. L., and Crowhurst, R. N. (1992). Cloning and molecular characterization of the glyceraldehyde-3-phosphate dehydrogenase-encoding gene and cDNA from the plant pathogenic fungus Glomerella cingulata. Gene 122, 225–230. doi: 10.1016/0378-1119(92)90055-T
Wang, Y., Coleman-Derr, D., Chen, G., and Gu, Y. (2015). OrthoVenn: a web server for genome wide comparison and annotation of orthologous clusters across multiple species. Nucleic Acids Res. 43, W78–W84. doi: 10.1093/nar/gkv487
Wang, Y. C., Hao, X. Y., Wang, L., Xiao, B., Wang, X. C., and Yang, Y. J. (2016a). Diverse Colletotrichum species cause anthracnose of tea plants (Camellia sinensis (L.) O. Kuntze) in China. Sci. Rep. 6:35287. doi: 10.1038/srep35287
Wang, Y. C., Qian, W. J., Li, N. N., Hao, X. Y., Lu, W., Xiao, B., et al. (2016b). Metabolic changes of caffeine in tea plant (Camellia sinensis (L.) O. Kuntze) as defense response to Colletotrichum fructicola. J. Agric. Food Chem. 64:6685. doi: 10.1021/acs.jafc.6b02044
Weir, B. S., Johnston, P. R., and Damm, U. (2012). The Colletotrichum gloeosporioides species complex. Stud. Mycol. 73:115. doi: 10.3114/sim0011
White, T. J., Bruns, T., Lee, S., and Taylor, J. W. (1990). “Amplification and direct sequencing of fungal ribosomal RNA genes for phylogenetics,” in PCR Protocols: A Guide to Methods and Applications, eds M. A. Innis, D. H. Gelfand, J. J. Sninsky, and T. J. (New York, NY: White Academic Press, Inc.), 315–322.
Wilson, R. A., and Talbot, N. J. (2009). Under pressure: investigating the biology of plant infection by Magnaporthe oryzae. Nat. Rev. Microbiol. 7:185. doi: 10.1038/nrmicro2032
Yakoby, N., Kobiler, I., Dinoor, A., and Prusky, D. (2000). pH regulation of pectate lyase secretion modulates the attack of Colletotrichum gloeosporioides on avocado fruits. Appl. Environ. Microbiol. 66, 1026–1030. doi: 10.1128/AEM.66.3.1026-1030.2000
Yan, J. Y., Jayawardena, M. M. R. S., Goonasekara, I. D., Yong, W., Wei, Z., Mei, L., et al. (2015). Diverse species of Colletotrichum associated with grapevine anthracnose in China. Fungal Divers. 71, 233–246. doi: 10.1007/s13225-014-0310-9
You, B. J., Choquer, M., and Chung, K. R. (2007). The Colletotrichum acutatum gene encoding a putative pH-responsive transcription regulator is a key virulence determinant during fungal pathogenesis on citrus. Mol. Plant Microbe Interact. 20, 1149–1160. doi: 10.1094/MPMI-20-9-1149
Keywords: Colletotrichum camelliae, Colletotrichum fructicola, pathogenicity, inoculation, PacC/Rim101
Citation: Lu Q, Wang Y, Li N, Ni D, Yang Y and Wang X (2018) Differences in the Characteristics and Pathogenicity of Colletotrichum camelliae and C. fructicola Isolated From the Tea Plant [Camellia sinensis (L.) O. Kuntze]. Front. Microbiol. 9:3060. doi: 10.3389/fmicb.2018.03060
Received: 22 June 2018; Accepted: 27 November 2018;
Published: 11 December 2018.
Edited by:
Raffaella Balestrini, Istituto per la Protezione Sostenibile delle Piante (IPSP), ItalyReviewed by:
Shouan Liu, Jilin University, ChinaLuca Nerva, Council for Agricultural and Economics Research, Italy
Copyright © 2018 Lu, Wang, Li, Ni, Yang and Wang. This is an open-access article distributed under the terms of the Creative Commons Attribution License (CC BY). The use, distribution or reproduction in other forums is permitted, provided the original author(s) and the copyright owner(s) are credited and that the original publication in this journal is cited, in accordance with accepted academic practice. No use, distribution or reproduction is permitted which does not comply with these terms.
*Correspondence: Dejiang Ni, bmlkakBtYWlsLmh6YXUuZWR1LmNu Yajun Yang, eWp5YW5nQHRyaWNhYXMuY29t Xinchao Wang, eGN3NzVAdHJpY2Fhcy5jb20=
†These authors have contributed equally to this work