- 1Laboratory of Research in Applied Microbiology (LAPEMA), University of Franca, Franca, Brazil
- 2Institute of Biomedical Sciences (ICBIM), Federal University of Uberlândia, Uberlândia, Brazil
Considering the impact of dental caries on public health and the wide biological potential described for species belonging to the genus Pinus, here we investigate the antibacterial activity of the P. elliottii and P. tropicalis resins and of the diterpene dehydroabietic acid (DHA) against cariogenic bacteria. For this purpose, we have determined the minimum inhibitory concentration (MIC) and the minimum bactericidal concentration (MBC) of the resins and of the diterpene. We have also investigated the biofilm inhibition ability (through MBIC50 determination), as well as the synergistic effect (through fractional inhibitory concentration index) and the cytotoxic potential (through XTT assay) of the P. elliottii and P. tropicalis resins. The resins gave promising MIC and MBC values, which ranged from 12.5 to 400 μg/mL; DHA provided MIC and MBC values ranging from 25 to 400 μg/mL. The MICB50 values ranged from 0.78 to 400 μg/mL for the resins. Neither additive nor synergistic effects emerged for the combinations of one of the resins with chlorhexidine. The cytotoxic activity was ≥312.5 and ≥156.3 μg/mL for the P. elliottii and P. tropicalis resins, respectively. The resins showed antibacterial activity against planktonic and sessile cariogenic bacteria. These data are relevant and encourage further research into these plants, which may contribute to the discovery of new substances that can inhibit the growth of cariogenic microorganisms and reduce the incidence of dental caries.
Introduction
The oral cavity is colonized by a group of intricate microorganisms that grow as a distinct oral biofilm, the dental plaque. This tooth-adhering film causes the most common oral microbial infection known to man, the so-called dental caries (Sunitha et al., 2017).
Dental caries is the most prevalent chronic disease worldwide and represents a costly burden to health care services especially in developing countries, where investments in prevention are minimal, and access to care is limited. Dental caries is a multifactorial disease that affects approximately 90% of the world population, with biological, environmental, and socio-behavioral risk factors contributing to its initiation and progression (Elamin et al., 2018). Microorganisms like Streptococcus mutans initiate the carious lesion on the tooth. Streptococcus sanguinis, Streptococcus salivarius, Streptococcus mitis, Streptococcus sobrinus, and Lactobacillus spp., among others, are the chief cariogenic microorganisms (Sunitha et al., 2017).
Given the current worldwide overuse of antibiotics in dentistry and the limited awareness of infection prevention guidelines, drug-resistant bacteria have become further hazards (Barenghi et al., 2018). Besides that, oral diseases can be so painful that they can obstruct food mastication and ingestion, thereby impairing the body nutritional status and immune function and negatively impacting the quality of life (Chinsembu, 2016; BaniHani et al., 2018). Against this backdrop, there is an urgent need to accelerate bioprospecting efforts and to isolate active compounds that may be used to manufacture new drugs and hygiene products for oral health.
In this context, plants stand out for having proven to be a promising source for the development of medicines to treat a broad spectrum of diseases (Cragg et al., 2014; Newman and Cragg, 2016). Plant oleoresins, which are substances containing resins in their composition (non-volatile principles), have raised interest due to their already reported antimicrobial potential (Caetano da Silva et al., 2014; Leandro et al., 2014; Fernández et al., 2018; Arruda et al., 2019).
The genus Pinus belongs to the family Pinaceae, includes many species, mainly trees, and is found mostly in the northern hemisphere. One of the features of Pinus species is that they exudate a resinous secretion, known as resin or colophony, which has several applications, including adhesives, soaps, and food additives (Beltran et al., 2016). The needles, leaves, and oils of plants belonging to the genus Pinus have been widely employed in folk medicine because of their numerous pharmacological properties, such as anti-aging and anti-inflammatory effects, and of the possibility of using them to treat liver diseases, skin diseases, and hypertension (Xie et al., 2015).
Pinus elliottii Engelm. and Pinus tropicalis Morelet are trees that can be as tall as 15–30 m, but which have been little studied as to their pharmacological properties. P. elliottii originates in southeastern United States, though it is widely cultivated as a subtropical crop in Brazil, India, and China. It is usually employed to fabricate oleoresin and furniture (Caetano da Silva et al., 2014), and its anthelmintic (Tóro et al., 2003) and antibacterial actions have been demonstrated (Caetano da Silva et al., 2014; Leandro et al., 2014). P. tropicalis is endemic to the island of Cuba and exhibits strong insecticidal (Leyva et al., 2009) and fungicidal (Andrade et al., 2014) effects.
Concerning resins obtained from Pinus species, phytochemical studies have shown that they consist mainly of diterpenes, which present countless known biological activities like anti-inflammatory, antifungal, antiparasite, and antibacterial actions (Caetano da Silva et al., 2014). DHA is an abietane diterpene that can be obtained from Pinus resin or commercial resin. It has already been described as the major compound in P. elliotti (Caetano da Silva et al., 2014). Researchers have investigated the DHA pharmacological potential and explored its ability to generate derivatives that can act as synthetic precursors of heteroaromatic compounds with promising biological properties. Indeed, gastroprotective, antiviral, antibacterial, and antiulcer activities have already been described for DHA or its derivative (Wada et al., 1985; Fonseca et al., 2004; Sepúlveda et al., 2005; Caetano da Silva et al., 2014; Leandro et al., 2014).
Considering the impact of dental caries on public health and the popular use and wide biological potential described for species belonging to the genus Pinus, here we investigate the antibacterial activity of P. elliottii and P. tropicalis resins and of the diterpene DHA, isolated from P. elliottii, against cariogenic bacteria. In addition, the lack of studies on the cytotoxicity of these resins has motivated us to address this issue along with the SI of the resins in relation to human cells.
Materials and Methods
Resin Acquisition and Tested Microorganisms
Authentic P. elliottii (batch number 21811-09) and P. tropicalis (batch number 20511-09) resin samples were obtained from the Brazilian Association of Resinators (ARESB), located in the city of Avaré – SP, Brazil. DHA was isolated from the P. elliottii resin and was obtained by the method previously described by Leandro et al. (2014) (Supplementary Figures S1, S2).
The following bacteria from the American Type Culture Collection (ATCC) collection were used to conduct the antibacterial assays: S. mutans (ATCC 25175), S. mitis (ATCC 49456), S. sanguinis (ATCC 10556), S. sobrinus (ATCC 33478), S. salivarius (ATCC 25975), Lactobacillus casei (ATCC 11578), and Enterococcus faecalis (ATCC 4082). These microorganisms were maintained in a freezer at −80°C in 20% glycerol solution in the Laboratory of Applied Microbiology Research (LaPeMA) – University of Franca (Unifran).
Minimum Inhibitory Concentration and Minimum Bactericidal Concentration
The MIC is defined as the lowest concentration of the sample that can inhibit bacterial growth. MIC was determined by microdilution in microplates. The experiments were accomplished in 96-well microplates and were repeated three times.
Briefly, 1.0 mg/mL resin solutions were prepared in DMSO (Merck, Darmstadt, Germany) and subsequently diluted in BHI broth (Difco, Detroit, MI, United States). Then, the resin samples were tested at concentrations ranging from 0.195 to 400 μg/mL. The final DMSO content in the samples was 5% (v/v); five inoculated wells containing DMSO at concentrations ranging from 5 to 1% were used as negative controls. The inoculum was adjusted for each organism at 625 nm in a spectrophotometer, to give a cell concentration of 5 × 105 CFU/mL, which corresponded to the final concentration in the well (CLSI, 2012). One inoculated well was included to control broth adequacy for organism growth. One non-inoculated well, free of antimicrobial agent, was also employed, to ensure medium sterility. The positive control was CHD (Sigma, St. Louis, MO, United States) at concentrations ranging from 0.115 to 59 μg/mL. Next, the microorganisms were incubated at 37°C for 24 h. S. mutans, S. mitis, S. sanguinis, S. sobrinus, and L. casei were incubated under microaerophilic conditions (atmosphere containing 10% CO2), while S. salivarius and E. faecalis were incubated under aerobic conditions.
After incubation, 30 μL of 0.02% resazurin (Sigma) aqueous solution was added to each well. Resazurin is an oxidation-reduction probe that allows microbial growth to be immediately detected. The blue and red colors represent absence and presence of microbial growth, respectively (Sarker et al., 2007).
To determine the MBC, a 10-μL aliquot of the inoculum was removed from each well before resazurin was added. The inoculum aliquot was then seeded on Blood Agar Base (Difco) supplemented with 5% defibrillation sheep blood for all strains. Next, the microplates were incubated as described above. MBC is defined as the lowest concentration of the sample where no bacterial growth occurs. A substance is considered to exert a bacteriostatic effect when its MBC value is higher than its MIC value. However, a substance is considered to exhibit a bactericidal effect when its MBC value is the same as its MIC value.
Minimum Inhibitory Concentration Against the Biofilm
Preliminary tests were performed to define the best conditions for the biofilm to form, such as the ideal inoculum concentration and the period that is necessary for the biofilm to grow. For this purpose, the biofilms were standardized in three repeated experiments; BHI (Difco) broth and 96-well flat-bottom microplates were used. This assay was carried out for the bacteria that afforded the most promising MIC and MBC results. The best inoculum concentration and the optimal biofilm formation time were 106 CFU/mL and 24 h, respectively (data not shown).
Minimum inhibitory concentration against the biofilm is defined as the minimum concentration of the P. elliottii or P. tropicalis resin that can inhibit biofilm formation by at least 50%. MICB50 was determined as described by Wei et al. (2006) with the modifications depicted below. Briefly, serial twofold resin dilutions were prepared in a 96-well polystyrene tissue culture plate (TPP, Trasadingen, Switzerland) containing BHI (Difco) broth for all the bacteria, as described previously. The final concentrations ranged from 0.195 to 400 μg/mL for the resins and between 0.115 and 59 μg/mL for the positive control (CHD; Sigma). Bacterial strains in the absence of antibacterial agent were employed as negative controls, and the inoculums were adjusted to give a cell concentration of 106 CFU/mL for all the bacteria. The well contents were discarded after incubation at 37°C for 24 h. Then, each well was washed three times with 150 μL of sterile Milli Q water and fixed with 150 μL of methanol for 20 min. The whole experiment was repeated three times.
By following the procedure reported by Stepanović et al. (2007), the biofilms were quantified by OD by adding 150 μL of crystal violet (2%) to the microplate wells. After 15 min at room temperature, excess dye was removed by rinsing with tap water, followed by air-drying at room temperature. Then, 150 μL of glacial acetic acid 33% was gently added to each well, to re-solubilize the dye bound to the cells. The microtiter plate was covered with the lid and kept at room temperature for at least 30 min to minimize evaporation. The OD of each well was measured at 595 nm with the aid of a microtiter plate reader (ASYS, Eugendorf, Salzburg, Austria). The percentage of inhibition was calculated by using the equation (1 - At595/Ac595) × 100, where At595 nm and Ac595 nm are the absorbance of the wells treated with the resins and the control, respectively (Wei et al., 2006).
Cell viability under antibiofilm activity was measured by counting the number of microorganisms; the same procedures described above were employed in another microplate. The assay was conducted according to Moreti et al. (2017) with the adaptations depicted below. Dilutions between 10−1 to 10−7 were prepared with BHI (Difco) broth, to allow microorganism counting. Subsequently, 50 μL of each dilution was transferred to BHI (Difco) agar plates supplemented with 5% defibrillation sheep blood, divided into eight parts, and incubated under the conditions described previously. After incubation, the colonies were counted. Results are expressed as CFU/mL. In addition, the IC50 (concentration of resins capable of inhibiting 50% of microbial growth) was calculated by using GraphPad Prism 6, and results are expressed in μg/mL.
Fractional Inhibitory Concentration Index (FICI)
The activities of combinations of one of the resins with CHD (Sigma) were evaluated by FICI for S. mutans, S. mitis, S. sanguinis, S. sobrinus, and L. casei, the bacteria that presented the lowest MIC values. Checkerboard assays were performed according to the protocol previously described by White et al. (1996) to investigate the in vitro antimicrobial efficacy of the combination of the P. elliottii (antimicrobial agent A) or P. tropicalis (antimicrobial agent B) resin with CHD (antimicrobial agent C; Sigma). The whole experiment was repeated three times, and concentrations of each compound were combined by using a standard MIC format.
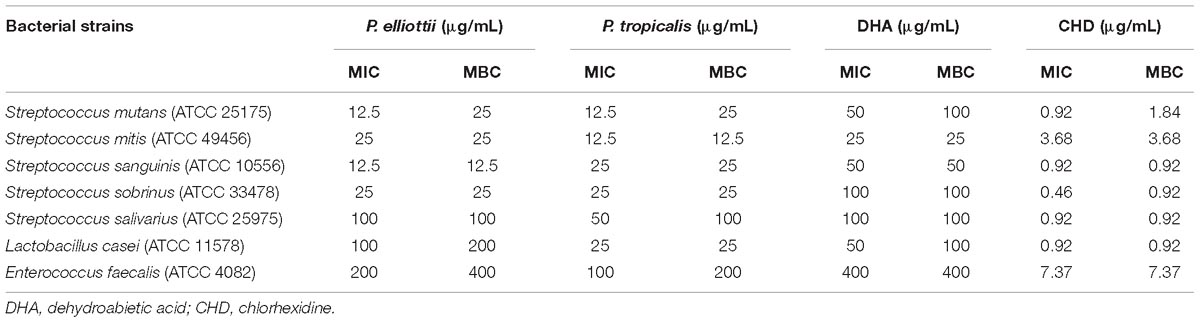
Table 1. Minimum inhibitory concentration (MIC) and minimum bactericidal concentration (MBC) in μg/mL of the P. elliottii and P. tropicalis resins and of dehydroabietic acid (DHA) against bacteria that cause caries.
Serial twofold dilutions of resins and CHD (Sigma) were mixed in each well of a 96-well microtiter plate. Fifty-microliter aliquots of the first and the second antimicrobial agents were added in vertical and horizontal orientation, respectively. A volume of 100 mL of fresh bacterial suspension (1 × 106 CFU/mL) was added to each well and incubated as mentioned previously. FICIs were calculated according to the following formula: FICI = (MIC of antimicrobial agent A or B in combination / MIC of antimicrobial agent A or B alone) + (MIC of antimicrobial agent C in combination / MIC of antimicrobial agent C alone). FICI ≤ 0.5, >0.5–1.0, 1–4.0, and >4.0 was interpreted as synergistic, additive, indifferent, and antagonistic, respectively (Lewis et al., 2002).
Cell Line and Culture Conditions and XTT-Based Cytotoxicity Assay
Human gingival fibroblasts (HGF cells) were employed to assess the cytotoxic potential of the P. tropicalis and P. elliottii resins. The cell line was maintained as monolayers in plastic culture flasks (25 cm2) in HAM-F10/DMEM (1:1, Sigma) culture medium supplemented with 10% fetal bovine serum (Nutricell), antibiotics (0.01 mg/mL streptomycin and 0.005 mg/mL penicillin; Sigma), and 2.38 mg/mL Hepes (Sigma), at 37°C with 5% CO2.
The cytotoxic activity was measured with the in vitro Toxicology Colorimetric Assay Kit (XTT; Roche Diagnostics) according to the manufacturer’s instructions. Extracellular XTT reduction occurs on the plasma membrane surface via transmembrane electron transport. This assay is widely used to determine cell proliferation or the cytotoxic effects of chemicals (Bernas and Dobrucki, 1999; Ferreira et al., 2016). For these experiments, the cells (104 cells/well) were plated onto 96-well microplates. Each well received 100 μL of HAM F10/DMEM medium containing P. tropicalis or P. elliottii. The tested concentrations ranged from 1.2 to 2,500 μg/mL. The negative (without treatment), solvent (DMSO 1%; Sigma), and positive (DMSO 25%) controls were included. After incubation at 37°C for 24 h, the medium was removed; the cells were washed with 100 μL of PBS and exposed to 100 μL of HAM-F10 medium without phenol red. Then, 25 μL of XTT was added to each well. The microplates were covered and incubated at 37°C for 17 h. The samples had their absorbance determined by using a multiplate reader (ELISA – Asys – UVM 340/Microwin 2000) operating at a test wavelength of 450 nm and a reference wavelength of 620 nm. Cell viability is expressed as percentage of untreated cells, which served as the negative control group and was designated as 100%; hence, results are expressed as a percentage of the negative control. The antiproliferative activity was assessed by using the parameter of 50% inhibition of cell line growth (IC50). The experiments were repeated three times.
The SI of the samples was calculated by using the formula SI = IC50/MIC; the concentrations that reduced cell viability in HGF cells by 50% (IC50) and the MIC against the tested bacteria were taken into account (Elisha et al., 2017).
Results
Table 1 lists the MIC and MBC values determined for the P. elliottii and P. tropicalis resins and for DHA against seven cariogenic bacteria. The values ranged from 12.5 to 400 μg/mL for the resins and from 25 to 400 for DHA. DMSO alone (5% concentration; negative control) had no effect on the tested bacteria.
The resins and DHA had the same MBC and MIC values against most of the tested bacteria, which meant that they exerted a bactericidal effect in these cases. The P. elliottii resin exhibited bacteriostatic action against S. mutans, L. casei, and E. faecalis, whereas the P. tropicalis resin showed this same effect on S. mutans, S. salivarius, and E. faecalis. DHA displayed bacteriostatic activity against S. mutans and L. casei (Table 1).
The P. elliottii resin presented promising results against S. mutans, S. mitis, S. sanguinis, and S. sobrinus, while P. tropicalis showed promising activity against all the investigated bacteria except E. faecalis (≥100 μg/mL; Table 1). DHA did not provide promising MIC results against any of the evaluated bacteria (≥10 μg/mL; Table 1).
The remaining experiments only involved the bacteria for which the resins yielded the best MIC results.
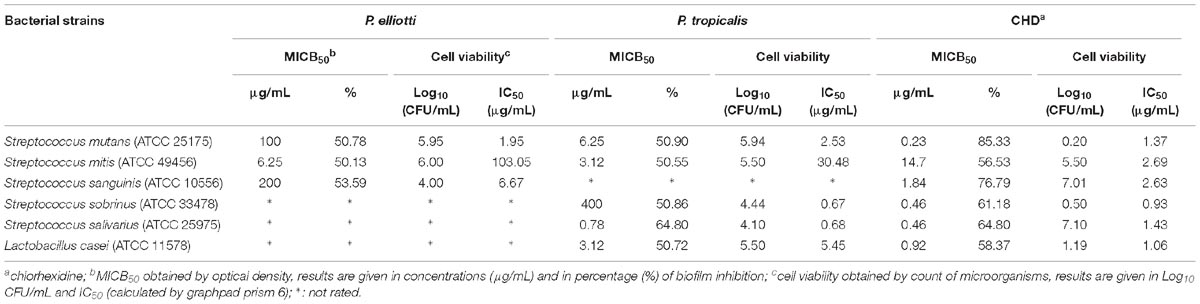
Table 2. Minimum inhibitory concentration against the biofilm and cell viability determined for the P. elliottii and P. tropicalis resins against cariogenic bacteria.
The MICB50 values ranged from 6.25 to 200 μg/mL for the P. elliottii resin against S. mutans, S. mitis, and S. sanguinis, and from 0.78 to 400 μg/ mL for the P. tropicalis resin against S. mutans, S. mitis, S. sobrinus, S. salivarius, and L. casei. The IC50 values for the microorganism count ranged from 1.95 to 103.05 μg/mL for the P. elliottii resin and from 0.68 to 30.48 μg/mL for the P. tropicalis resin (Table 2).
The FICI obtained for the resins combined with CHD (Sigma) revealed that the combination between the P. elliottii resin and CHD (Sigma) exerted an antagonistic effect on S. mutans and S. sanguinis and an indifferent effect on S. mitis. The combination between the P. tropicalis resin and CHD (Sigma) had an indifferent effect on the three bacteria (Table 3).

Table 3. Fraction Inhibitory Concentration Index (FICI) of the P. elliottii (PE) or the P. tropicalis (PT) resin in combination with chlorhexidine (CHD) against cariogenic bacteria.
Figure 1 represents the mean of three independent cell viability experiments, and the bars indicate the standard error of the mean. Cell viability reduced significantly at P. elliottii and P. tropicalis resin concentrations above and equal to 312.5 μg/mL (IC50 331.37 ± 10.55 μg/mL) and 156.3 μg/mL (IC50 167.37 ± 22.96), respectively, indicating that the resins at these concentrations had a cytotoxic effect on the cells. Except for P. tropicalis for E. faecalis, SI demonstrated that both resins were at least once more selective toward bacterial cells than toward normal human cells (Table 4).
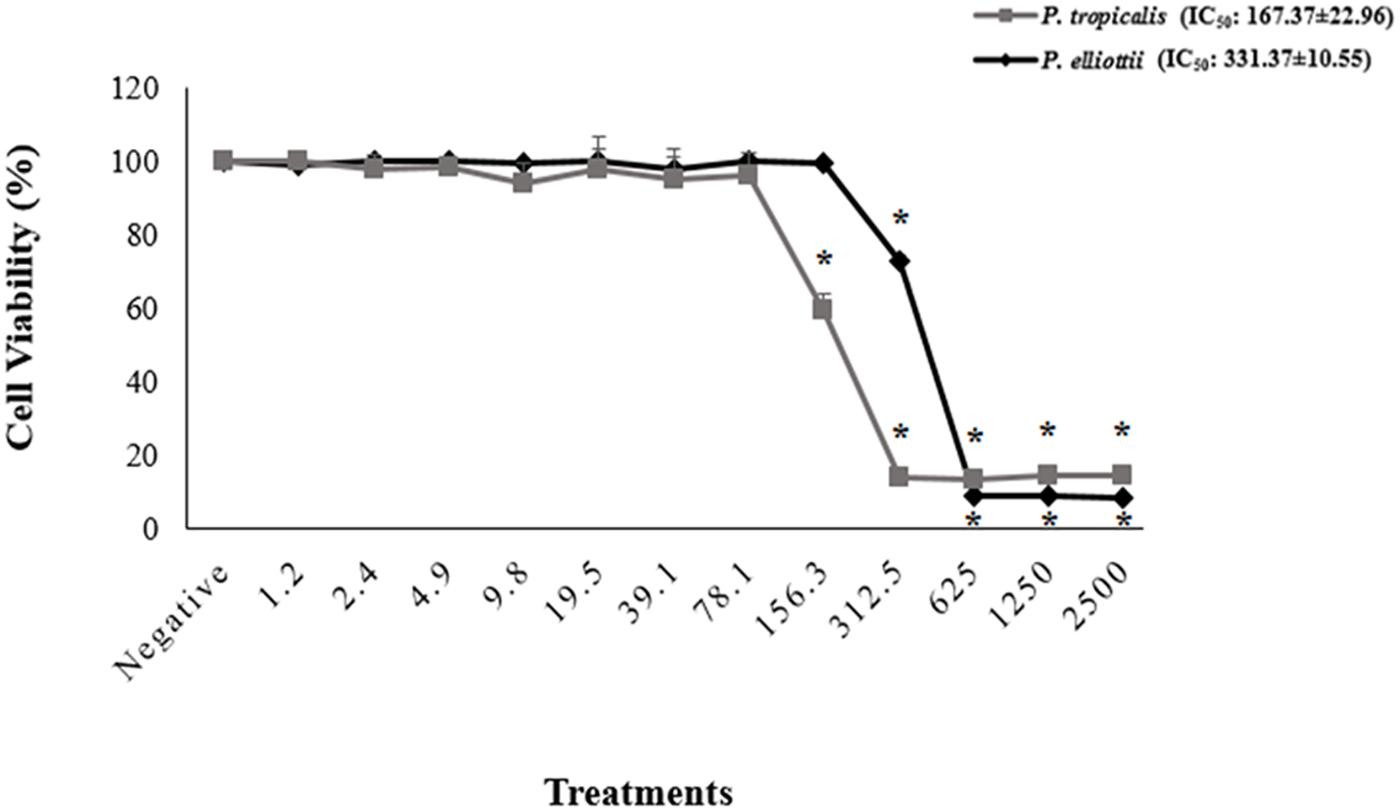
Figure 1. Cell viability (%) of HGF cells after treatment with the P. tropicalis and the P. elliottii extracts. Negative control (no treatment); the solvent (DMSO 1%) group showed cell viability of 99.84%; the positive control (DMSO 25%) showed cell viability of 9.27%. Values are means ± standard deviations. ∗Significantly different from the negative group (P < 0.05).
Discussion
Biofilms can naturally emerge in dental enamel, but poor oral hygiene can lead to biofilm accumulation, which is not compatible with an individual’s health (Marsh, 1992). With this concern in mind, we decided to investigate the antibacterial activity of two resins of the genus Pinus and of an isolated compound, the diterpene DHA, against cariogenic bacteria in the sessile and in the planktonic mode.
According to Ríos and Recio (2005) and Gibbons (2008), plant extracts (as well as essential oils) and isolated compounds with demonstrated activity at concentrations below 100 and 10 μg/mL, respectively, have promising biological action. Here, the P. elliottii and P. tropicalis resins proved to be promising against most of the tested bacteria, while DHA did not yield satisfactory MIC results. In fact, the literature asserts that plant resins and extracts often exhibit more pronounced biological activities than their isolated constituents. This is usually due to synergistic, additive, or potentiating interactions between the constituents, which generally involve action on multiple targets, resulting in significant biological potential (Raskin et al., 2002; Schmidt et al., 2008).
We did not achieve any promising results against E. faecalis for any of the tested resins or DHA. This could be due to the high levels of resistance that have already been described for this microorganism. Barbosa-Ribeiro et al. (2016) reported that, because of their morphologic and genetic characteristics, E. faecalis might be resistant to intracanal procedures and even to systemic antibiotics. The E. faecalis resistance mechanisms result from physiological or structural changes in the bacterial cell, which is a survival strategy against attack by antimicrobial agents (Barbosa-Ribeiro et al., 2016). Our results corroborate with the data published by Leandro et al. (2014), who used multiresistant strains of this microorganism and obtained MIC values of 100 and 50 μg/mL against E. faecalis (NCTC 14990) and 100 and 25 μg/mL against E. faecalis (clinical isolate) for the P. elliottii resin and DHA, respectively. On the other hand, in the same study, the authors found promising results against Staphylococcus epidermidis (ATCC 14990): MIC values were 25 μg/mL and 6.25 μg/mL for the P. elliottii resin and DHA, respectively.
Caetano da Silva et al. (2014) evaluated the antibacterial potential of the P. elliottii resin and DHA against endodontic anaerobic bacteria. As in the case of our study, the resin afforded promising MIC values, which ranged from 0.4 to 50 μg/mL. In contrast, DHA only showed promising antibacterial activity against P. gingivalis (ATCC 33277), with MIC of 6.2 μg/mL. Together with our results, the results of Caetano da Silva et al. (2014) revealed that the P. elliottii resin is promising against different microorganisms, whereas DHA, despite its already demonstrated antimicrobial potential, seems to rely on a synergistic action among the resin constituents to act against cariogenic bacteria.
The antibacterial activity of the genus Pinus has been explored over the years (Kim and Shin, 2005; Feng et al., 2010; Merah et al., 2018). However, the antibacterial potential of P. tropicalis has not been assessed yet, so we had difficulty comparing our data with literature results. Nevertheless, the present study will aid later works that address this issue and is the first study that has verified that the P. tropicalis resins exhibit significant antibacterial activity against cariogenic bacteria.
Stoddart (2011) reported that it is essential to conduct other assays when we evaluate the efficacy of a possible antimicrobial agent. For this reason, we also assessed the antibacterial potential of the Pinus resins by other techniques. We determined MICB50 to examine whether the P. elliotti and P. tropicalis resins can prevent the target bacteria from forming biofilm. We performed this assay, as well as FICI experiments with the bacteria for which the resins gave the best MIC results.
The resins tested herein provided promising MICB50 values against the studied bacteria as demonstrated by both OD reading and IC50 given by microorganism counts. Our results were even better than the results obtained by MIC. For some bacteria (S. mitis for both resins and S. salivarius for the P. tropicalis resin), the results were even better than the results achieved with the positive control, CHD (Sigma). Biofilms respond differently from planktonic cells depending on the growth phase, period of exposure to the antibacterial agent, and antimicrobial concentration (Gursoy et al., 2009). In other words, the same bacterium displays distinct behavior when it is in the biofilm mode and in the planktonic state, a fact that must be considered during all laboratory tests (Signoretto et al., 2012). Wei et al. (2006) highlighted that preventing biofilm formation is extremely relevant because inhibiting biofilm growth also avoids its maturing, which underscores the importance of the results obtained in our study.
This is the first work that has evaluated the MICB50 of species belonging to the genus Pinus. However, assessment of the antibiofilm activity of DHA (extracted from the P. elliottii resin) against endodontic bacteria revealed significant results, and MICB50 lay between 7.8 and 62.5 μg/mL and prevented all the bacteria from forming a biofilm. For some bacteria, the results were lower as compared to the values determined for the reference drug, CHD (Sigma).
It is unusual for bacterial inhibition values of bacteria in the sessile mode to be lower than or equal to bacterial inhibition values of bacteria in the planktonic mode because biofilms are considered to be more resistant to antimicrobial agents as compared to planktonic cells (Stewart and Costerton, 2001). However, this is not unprecedented in the literature (Al-Shuneigat et al., 2005; Karpanen et al., 2008; Fernández et al., 2018). According to Karpanen et al. (2008), unexpected results such as this suggest that the extracellular matrix of the bacterial biofilm may alter the activity of the tested substances, or that substances consisting of several heterogeneous, hydrophilic, and hydrophobic properties may alter ionic interactions in the biofilm extracellular matrix and act on the same target on the bacterial cell. Therefore, further studies are necessary to establish the modes of action of the Pinus resins investigated herein. We emphasize that spectrophotometric readings (OD) and microorganism count (log10 CFU/mL) are recommended to show the ability of antimicrobial agents to inhibit biofilm formation (antibiofilm activity). Together, the techniques applied here can provide reliable results concerning biofilm activity (Kharazmi et al., 1999; Pettit et al., 2005; Caetano da Silva et al., 2014).
The literature has recorded the increased resistance of microorganisms to antiseptics, such as CHD, in addition to their potential cytotoxicity to normal cells (Faria et al., 2007; Lessa et al., 2010; Cieplik et al., 2018). Therefore, the MICB50 values found here are interesting, demonstrating that these Pinus resins have potential action against biofilms formed by microorganisms that participate in the formation of dental caries. In other words, these resins deserve to be explored since they may represent an alternative to the antibacterial chemical agents that have been traditionally employed.
Regarding cytotoxicity, all the promising concentrations of the resins were below the cytotoxic concentrations. The SI demonstrated that both resins are more selective toward bacterial cells than toward normal human cells for most of the tested bacteria. This selectivity is even more evident if we consider the activity of the P. elliottii resin against S. mutans and S. sanguinis and the action of P. tropicalis against S. mutans, S. mitis, S. sobrinus, and S. sanguinis – in these cases, selectivity toward bacterial cells is at least ten times higher than selectivity toward normal human cells. This is important because it indicates that the resins can be used at concentrations up to 10-fold or higher than their MIC without being cytotoxic to normal cells.
Combinations of one of the resins with CHD (Sigma) do not exhibit any additive effects. A search of the scientific literature did not retrieve any articles on the synergism of P. elliottii, P. tropicalis, or other Pinus species, which made comparison of our results with data by other authors impossible. Despite the absence of synergistic effects when the resins are combined with CHD (Sigma), the P. elliottii and P. tropicalis resins showed promising results during the other antimicrobial tests performed herein. In this sense, this is the first study that has revealed that an association of the resins tested here with CHD (Sigma) is not recommended. On the other hand, the use of natural products may be an economical approach to prevent and to treat dental caries (Wassel and Khattab, 2017). Considering that phytotherapy constitutes a form of medicinal therapy that has notably grown in recent years (Silva et al., 2015), additional research involving the use of P. elliottii and P. tropicalis as possible phytotherapics against cariogenic bacteria is encouraged.
Conclusion
The results of the present study do not demonstrate any activity of DHA against the tested bacteria. On the other hand, our data reveal the in vitro antibacterial activity of the P. elliottii and P. tropicalis resins against cariogenic bacteria in the planktonic and in the sessile modes. The promising MIC/MBC values are lower than the resin concentrations that provide 50% viability for human gingival fibroblast cells. Additionally, on the basis of the SI, the resins are more selective toward the bacterial cells than toward the normal cells. As for the evaluation of the combinations of one of the resins with CHD, results point to antagonism or indifference. Judging from the values of MIC of biofilm obtained herein, the resins can inhibit all the assayed bacteria by at least 50%. These results encourage further studies to unveil the action mechanisms of these resins and contribute to the quest for new effective and less toxic alternatives that can inhibit bacterial growth and reduce the incidence of dental caries.
Author Contributions
CM, DT, RV, and SA conceived the idea for this study. KS, JD, FA, and CM participated in the study design, organized the data, and evaluated their quality. MOI and KS conducted the antibacterial assays. NF conducted the cytotoxicity assay. CM, RV, SA, and DT critically reviewed the manuscript. All authors have read and approved the final manuscript.
Funding
The authors thank National Council for Scientific and Technological Development (CNPq) and Coordination for the Improvement of Higher Education Personnel (CAPES) for financial support.
Conflict of Interest Statement
The authors declare that the research was conducted in the absence of any commercial or financial relationships that could be construed as a potential conflict of interest.
Supplementary Material
The Supplementary Material for this article can be found online at: https://www.frontiersin.org/articles/10.3389/fmicb.2019.00987/full#supplementary-material
Abbreviations
BHI, brain heart infusion; CHD, chlorhexidine; DHA, dehydroabietic acid; DMSO, dimethylsulfoxide; FICI, fractional inhibitory concentration index; HGF, human gingival fibroblasts; IC50, 50% inhibitory concentration; MBC, minimum bactericidal concentration; MIC, minimum inhibitory concentration; MICB50, minimum inhibitory concentration against the biofilm; OD, optical density; PBS, phosphate buffered saline; SI, selectivity index.
References
Al-Shuneigat, J., Cox, S. D., and Markham, J. L. (2005). Effects of a topical essential oil-containing formulation on biofilm-forming coagulase-negative staphylococci. Lett. Appl. Microbiol. 41, 52–55. doi: 10.1111/j.1472-765X.2005.01699.x
Andrade, G., Abrão, F., Silva, P., Ambrósio, S. R., Veneziani, R. C. S., Cunha, W. R., et al. (2014). Antifungal activity of oleoresin and fractions of Pinus elliottii Engelm and Pinus tropicalis against phytopathogens. Am. J. Plant Sci. 5, 3899–3903. doi: 10.4236/ajps.2014.526408
Arruda, C., Aldana Mejía, J. A., Ribeiro, V. P., Gambeta Borges, C. H., Martins, C. H. G., Sola Veneziani, R. C., et al. (2019). Occurrence, chemical composition, biological activities and analytical methods on Copaifera genus-A review. Biomed. Pharmacother. 109, 1–20. doi: 10.1016/j.biopha.2018.10.030
BaniHani, A., Deery, C., Toumba, J., Munyombwe, T., and Duggal, M. (2018). The impact of dental caries and its treatment by conventional or biological approaches on the oral health-related quality of life of children and carers. Int. J. Paediatr. Dent. 28, 266–276. doi: 10.1111/ipd.12350
Barbosa-Ribeiro, M., De-Jesus-Soares, A., Zaia, A. A., Ferraz, C. C., Almeida, J. F., and Gomes, B. P. (2016). Antimicrobial susceptibility and characterization of virulence genes of Enterococcus faecalis isolates from teeth with failure of the endodontic treatment. J. Endod. 42, 1022–1028. doi: 10.1016/j.joen.2016.03.015
Barenghi, L., Barenghi, A., and Di Blasio, A. (2018). Infection Control in Dentistry and Drug-Resistant Infectious Agents: A Burning Issue. Part 1. London: IntechOpen.
Beltran, V., Salvadó, N., Butí, S., and Pradell, T. (2016). Ageing of resin from Pinus species assessed by infrared spectroscopy. Anal. Bioanal. Chem. 408, 4073–4082. doi: 10.1007/s00216-016-9496-x
Bernas, T., and Dobrucki, J. (1999). Reduction of a tetrazolium salt, CTC, by intact HepG2 human hepatoma cells: subcellular localization of reducing systems. Biochim. Biophys. Acta 1451, 73–81. doi: 10.1016/S0167-4889(99)00140-8
Caetano da Silva, S. D., Mendes de Souza, M. G., Oliveira Cardoso, M. J., da Silva Moraes, T., Ambrósio, S. R., Sola Veneziani, R. C., et al. (2014). Antibacterial activity of Pinus elliottii against anaerobic bacteria present in primary endodontic infections. Anaerobe 30, 146–152. doi: 10.1016/j.anaerobe.2014.09.013
Chinsembu, K. C. (2016). Plants and other natural products used in the management of oral infections and improvement of oral health. Acta Trop. 154, 6–18. doi: 10.1016/j.actatropica.2015.10.019
Cieplik, F., Kara, E., Muehler, D., Enax, J., Hiller, K. A., Maisch, T., et al. (2018). Antimicrobial efficacy of alternative compounds for use in oral care toward biofilms from caries-associated bacteria in vitro. Microbiologyopen 26:e00695. doi: 10.1002/mbo3.695
CLSI (2012). Clinical and Laboratory Standards Institute. Methods for Dilution Antimicrobial Susceptibility Tests for Bacteria That Grow Aerobically. Approved standard M7-A9. Wayne, PA: CLSI.
Cragg, G. M., Grothaus, P. G., and Newman, D. J. (2014). New horizons for old drugs and drug leads. J. Nat. Prod. 77, 703–723. doi: 10.1021/np5000796
Elamin, A., Garemo, M., and Gardner, A. (2018). Dental caries and their association with socioeconomic characteristics, oral hygiene practices and eating habits among preschool children in Abu Dhabi, United Arab Emirates - the NOPLAS project. BMC Oral Health 18:104. doi: 10.1186/s12903-018-0557-8
Elisha, I. L., Jambalang, A. R., Botha, F. S., Buys, E. M., McGaw, L. J., and Eloff, J. N. (2017). Potency and selectivity indices of acetone leaf extracts of nine selected South African trees against six opportunistic Enterobacteriaceae isolates from commercial chicken eggs. BMC Compl. Altern. Med. 17:90. doi: 10.1186/s12906-017-1597-3
Faria, G., Celes, M. R., De Rossi, A., Silva, L. A., Silva, J. S., and Rossi, M. A. (2007). Evaluation of chlorhexidine toxicity injected in the paw of mice and added to cultured l929 fibroblasts. J. Endod. 33, 715–722. doi: 10.1016/j.joen.2006.12.023
Feng, S., Zeng, W., Fuo, F., Zhao, L., Yang, Z., and Sun, Q. (2010). Antibacterial activity of organic acids in aqueous extracts from pine needles (Pinus massoniana Lamb.). Food Sci. Biotechnol. 19, 35–41. doi: 10.1007/s10068-010-0005-2
Fernández, Y. A., Damasceno, J. L., Abrão, F., Silva, T. S., Cândido, A. L. P., Fregonezi, N. F., et al. (2018). Antibacterial, preservative, and mutagenic potential of Copaifera spp. oleoresins against causative agents of foodborne diseases. Foodborne Pathog. Dis. 15, 790–797. doi: 10.1089/fpd.2018.2478
Ferreira, N. H., de Andrade, K. J., Leandro, L. F., Acésio, N. O., Mendes, S. A., Cintra, L. S., et al. (2016). Testing of Schefflera vinosa extract in mammalian cells in vitro and in vivo for potential toxicity, genetic damage, and role of oxidation. J. Toxicol. Environ. Health. Part A 79, 1201–1210. doi: 10.1080/15287394.2016.1229238
Fonseca, T., Gigante, B., Marques, M. M., Gilchrist, T. L., and De Clercq, E. (2004). Synthesis and antiviral evaluation of benzimidazoles, quinoxalines and indoles from dehydroabietic acid. Bioorg. Med. Chem. 12, 103–112. doi: 10.1016/j.bmc.2003.10.013
Gibbons, S. (2008). Phytochemicals for bacterial resistance–strengths, weaknesses and opportunities. Planta Med. 74, 594–602. doi: 10.1055/s-2008-1074518
Gursoy, U. K., Gursoy, M., Gursoy, O. V., Cakmakci, L., Könönen, E., and Uitto, V. J. (2009). Antibiofilm properties of Satureja hortensis L. essential oil against periodontal pathogens. Anaerobe 15, 164–167. doi: 10.1016/j.anaerobe.2009.02.004
Karpanen, T. J., Worthington, T., Hendry, E. R., Conway, B. R., and Lambert, P. A. (2008). Antimicrobial efficacy of chlorhexidine digluconate alone and in combination with eucalyptus oil, tea tree oil and thymol against planktonic and biofilm cultures of Staphylococcus epidermidis. J. Antimicrob. Chemother. 62:1031e6. doi: 10.1093/jac/dkn325
Kharazmi, A., Giwercman, B., and Holby, N. (1999). Robbins device in biofilm research. Methods Enzymol. 310:207e15. doi: 10.1016/S0076-6879(99)10018-1
Kim, Y. S., and Shin, D. H. (2005). Volatile components and antibacterial effects of pine needle (Pinus densiflora S. and Z.) extracts. Food Microbiol. 22, 37–45. doi: 10.1016/j.fm.2004.05.002
Leandro, L. F., Cardoso, M. J., Silva, S. D., Souza, M. G., Veneziani, R. C., Ambrosio, S. R., et al. (2014). Antibacterial activity of Pinus elliottii and its major compound, dehydroabietic acid, against multidrug-resistant strains. J. Med. Microbiol. 63, 1649–1653. doi: 10.1099/jmm.0.081711-0
Lessa, F. C. R., Aranha, A. M. F., Nogueira, I., Giro, E. M. A., Hebling, J., and De Souza Costa, C. A. (2010). Toxicity of chlorhexidine on odontoblast-like cells. J. Appl. Oral Sci. 18, 50–58. doi: 10.1590/S1678-77572010000100010
Lewis, R. E., Diekema, D. J., Messer, S. A., Pfaller, M. A., and Klepser, M. E. (2002). Comparison of Etest, chequerboard dilution and time-kill studies for the detection of synergy or antagonism between antifungal agents tested against Candida species. J. Antimicrob. Chemother. 49, 345–351. doi: 10.1093/jac/49.2.345
Leyva, M., Acoronte, J. E., Marquetti, M. D. C., Scull, R., Tiomno, O., Mesa, A., et al. (2009). Utilización de aceites esenciales de pinaceas endémicas como una alternativa en el control del Aedes aegypti. Rev. Cuba Med. Trop. 61, 239–243.
Marsh, P. D. (1992). Microbiological aspects of the chemical control of plaque and gingivitis. J. Dent. Res. 71, 1431–1438. doi: 10.1177/00220345920710071501
Merah, S., Dahmane, D., Krimat, S., Metidji, H., Nouasri, A., Lamari, L., et al. (2018). Chemical analysis of phenolic compounds and determination of anti-oxidant, antimicrobial and cytotoxic activities of organic extracts of Pinus coulteri. Bangladesh J. Pharmacol. 13, 120–129. doi: 10.3329/bjp.v13i2.35479
Moreti, D. L. C., Leandro, L. F., da Silva Moraes, T., Moreira, M. R., Sola Veneziani, R. C., Ambrosio, S. R., et al. (2017). Mikania glomerata sprengel extract and its major compound ent-kaurenoic acid display activity against bacteria present in endodontic infections. Anaerobe 47, 201–208. doi: 10.1016/j.anaerobe.2017.06.008
Newman, D. J., and Cragg, G. M. (2016). Natural products as sources of new drugs from 1981 to 2014. J. Nat. Prod. 79, 629–661. doi: 10.1021/acs.jnatprod.5b01055
Pettit, R. K., Weber, C. A., Kean, M. J., Hoffmann, H., Pettit, G. R., Tan, R., et al. (2005). Microplate alamar blue assay for Staphylococcus epidermidis biofilm susceptibility testing. Antimicrob. Agents Chemother. 49:2612e7. doi: 10.1128/AAC.49.7.2612-2617.2005
Raskin, I., Ribnicky, D. M., Komarnytsky, S., Ilic, N., Poulev, A., Borisjuk, N., et al. (2002). Plants and human health in the twenty-first century. Trends Biotechnol. 20, 522–531. doi: 10.1016/S0167-7799(02)02080-2
Ríos, J. L., and Recio, M. C. (2005). Medicinal plants and antimicrobial activity. J. Ethnopharmacol. 100, 80–84. doi: 10.1016/j.jep.2005.04.025
Sarker, S. D., Nahar, L., and Kumarasamy, Y. (2007). Microtitre platebased antibacterial assay incorporating resazurin as an indicator of cell growth, and its application in the in vitro antibacterial screening of phytochemicals. Methods 42, 321–324. doi: 10.1016/j.ymeth.2007.01.006
Schmidt, B., Ribnicky, D. M., Poulev, A., Logendra, S., Cefalu, W. T., and Raskin, I. (2008). A natural history of botanical therapeutics. Metabolism 57, S3–S9. doi: 10.1016/j.metabol.2008.03.001
Sepúlveda, B., Astudillo, L., Rodríguez, J. A., Yáñez, T., Theoduloz, C., and Schmeda-Hirschmann, G. (2005). Gastroprotective and cytotoxic effect of dehydroabietic acid derivatives. Pharmacol. Res. 52, 429–437. doi: 10.1016/j.phrs.2005.06.004
Signoretto, C., Canepari, P., Stauder, M., Vezzulli, L., and Pruzzo, C. (2012). Functional foods and strategies contrasting bacterial adhesion. Curr. Opin. Biotechnol. 23, 160–167. doi: 10.1016/j.copbio.2011.08.006
Silva, N. B., Valença, A. M. G., Costa, A. C., Castro, R. D., and Cavalcanti, A. L. (2015). Atividade antiaderente de produtos fitoterápicos sobre Streptococcus mutans. Rev. Bras. Ciên. Saúde 19, 49–54. doi: 10.4034/RBCS.2015.19.01.08
Stepanović, S., Vuković, D., Hola, V., Di Bonaventura, G., Djukić, S., Cirković, I., et al. (2007). Quantification of biofilm in microtiter plates: overview of testing conditions and practical recommendations for assessment of biofilm production by Staphylococci. APMIS 115, 891–899. doi: 10.1111/j.1600-0463.2007.apm_630.x
Stewart, O. S., and Costerton, J. W. (2001). Antibiotic resistance of bacteria in biofilms. Lancet 11:559e62. doi: 10.1016/S0140-6736(01)05321-1
Stoddart, M. J. (2011). Cell viability assays: introduction. Methods Mol. Biol. 740:741. doi: 10.1007/978-1-61779-108-6_1
Sunitha, J., Krishna, S., Ananthalakshmi, R., Jeeva, J. S., Girija, A. S., and Jeddy, N. (2017). Antimicrobial effect of leaves of Phyllanthus niruri and Solanum nigrum on caries causing bacteria: an in vitro study. J. Clin. Diagn. Res. 11, KC01–KC04. doi: 10.7860/JCDR/2017/23602
Tóro, R. M., Gessner, A. A., Furtado, N. A., Ceccarelli, P. S., de Albuquerque, S., and Bastos, J. K. (2003). Activity of the Pinus elliottii resin compounds against Lernaea cyprinacea in vitro. Vet. Parasitol. 118, 143–149. doi: 10.1016/j.vetpar.2003.08.008
Wada, H., Kodato, S., Kawamori, M., Morikawa, T., Nakai, H., Takeda, M., et al. (1985). Antiulcer activity of dehydroabietic acid derivatives. Chem. Pharm. Bull. 33, 1472–1487. doi: 10.1248/cpb.33.1472
Wassel, M. O., and Khattab, M. A. (2017). Antibacterial activity against Streptococcus mutans and inhibition of bacterial induced enamel demineralization of propolis, miswak, and chitosan nanoparticles based dental varnishes. J. Adv. Res. 8, 387–392. doi: 10.1016/j.jare.2017.05.006
Wei, G. X., Campagna, A. N., and Bobek, L. A. (2006). Effect of MUC7 peptides on the growth of bacteria and on Streptococcus mutans biofilm. J. Antimicrob. Chemother. 57, 1100–1109. doi: 10.1093/jac/dkl120
White, R. L., Burgess, D. S., Manduru, M., and Bosso, J. A. (1996). Comparison of three different in vitro methods of detecting synergy: time-kill, checkerboard, and E test. Antimicrob. Agents Chemother. 40, 1914–1918. doi: 10.1128/AAC.40.8.1914
Keywords: dental caries, cariogenic bacteria, antibacterial activity, cytotoxic activity, Pinus
Citation: Silva KR, Damasceno JL, Inácio MO, Abrão F, Ferreira NH, Tavares DC, Ambrosio SR, Veneziani RCS and Martins CHG (2019) Antibacterial and Cytotoxic Activities of Pinus tropicalis and Pinus elliottii Resins and of the Diterpene Dehydroabietic Acid Against Bacteria That Cause Dental Caries. Front. Microbiol. 10:987. doi: 10.3389/fmicb.2019.00987
Received: 05 February 2019; Accepted: 18 April 2019;
Published: 07 May 2019.
Edited by:
Kin Weng Kong, University of Malaya, MalaysiaReviewed by:
Susan Semple, University of South Australia, AustraliaTamás Papp, University of Szeged, Hungary
Copyright © 2019 Silva, Damasceno, Inácio, Abrão, Ferreira, Tavares, Ambrosio, Veneziani and Martins. This is an open-access article distributed under the terms of the Creative Commons Attribution License (CC BY). The use, distribution or reproduction in other forums is permitted, provided the original author(s) and the copyright owner(s) are credited and that the original publication in this journal is cited, in accordance with accepted academic practice. No use, distribution or reproduction is permitted which does not comply with these terms.
*Correspondence: Carlos Henrique Gomes Martins, Y2FybG9zLm1hcnRpbnMyQHVmdS5icg==