- 1BIOMERIT Research Centre, School of Microbiology, University College Cork – National University of Ireland, Cork, Ireland
- 2School of Microbiology, University College Cork – National University of Ireland, Cork, Ireland
- 3Telethon Kids Institute, Perth, WA, Australia
- 4School of Biomedical Sciences, Curtin Health Innovation Research Institute, Curtin University, Perth, WA, Australia
The chronic colonization of the respiratory tract by the opportunistic pathogen Pseudomonas aeruginosa is the primary cause of morbidity and mortality in cystic fibrosis (CF) patients. P. aeruginosa has been shown to undergo extensive genomic adaptation facilitating its persistence within the CF lung allowing it to evade the host immune response and outcompete co-colonizing residents of the lung microbiota. However, whilst several studies have described the various mutations that frequently arise in clinical isolates of P. aeruginosa, the environmental factors governing the emergence of these genetic variants is less well characterized. Gastro-oesophageal reflux has recently emerged as a major co-morbidity in CF and is often associated with the presence of bile acids in the lungs most likely by (micro) aspiration. In order to investigate whether bile may select for genetic variants, P. aeruginosa was experimentally evolved in artificial sputum medium, a synthetic media resembling environmental conditions found within the CF lung. Pigmented derivatives of P. aeruginosa emerged exclusively in the presence of bile. Genome sequencing analysis identified single nucleotide polymorphisms (SNPs) in quorum sensing (lasR) and both the pyocyanin (phzS) and pyomelanin (hmgA) biosynthetic pathways. Phenotypic analysis revealed an altered bile response when compared to the ancestral P. aeruginosa progenitor strain. While the recovered pigmented derivatives retained the bile mediated suppression of swarming motility and enhanced antibiotic tolerance, the biofilm, and redox responses to bile were abolished in the adapted mutants. Though loss of pseudomonas quinolone signal (PQS) production in the pigmented isolates was not linked to the altered biofilm response, the loss of redox repression could be explained by defective alkyl-quinolone (AQ) production in the presence of bile. Collectively, these findings suggest that the adaptive variants of P. aeruginosa that arise following long term bile exposure enables the emergence of ecologically competitive sub-populations. Altered pigmentation and AQ signaling may contribute to an enhancement in fitness facilitating population survival within a bile positive environment.
Introduction
Chronic respiratory disease poses a major societal challenge, with the rapid onset of the post-antibiotic era representing a serious threat to the clinical management of infections associated with these conditions (Burney et al., 2015; GBD 2015 Chronic Respiratory Disease Collaborators, 2017). As is often the case, the development of chronic microbial infections results in a shift in clinical regimens wherein these infections are no longer treatable using conventional antibiotics. Therefore, understanding the factors that contribute to respiratory pathogen’s ability to shift from acute to chronic infections is of paramount importance in order to identify alternative interventions and reduce the global reliance on antibiotics.
Cystic fibrosis (CF), resulting from a mutation of the Cystic fibrosis transmembrane conductance regulator (CFTR) gene, is the most common inherited disease in Caucasians affecting over 70,000 people worldwide (Cutting, 2015). The prognosis for this life-limiting autosomal recessive disorder has improved considerably in recent years, largely due to advances in clinical management and the development of new therapeutic interventions. However, challenges remain for the successful implementation of these innovative therapies. Principal among these is maintaining health and lung function in pediatric patients. Lower respiratory infections, even from as early as the first weeks of life, are strongly associated with the development of pulmonary inflammation and bronchiectasis (Stick et al., 2009; Ramsey et al., 2014). Early acquisition of respiratory pathogens including Staphylococcus aureus and the subsequent colonization by Pseudomonas aeruginosa is associated with clinical decline and increased mortality (Pittman et al., 2011; Caudri et al., 2018). P. aeruginosa acquisition often occurs early in life with the median age of initial detection around 2 years of age. Reports of P. aeruginosa positive cultures in patients as young as 3 months of age have also emerged (Douglas et al., 2009; Mott et al., 2012). Early eradication has been associated with reduced prevalence of chronic P. aeruginosa infections in later life (Taccetti et al., 2005). Therefore, ensuring the successful eradication of P. aeruginosa is of paramount importance in CF care.
The pathogen-centric focus to respiratory infections has been challenged somewhat in recent years with the advent of next generation sequencing technologies. Genomics based studies have uncovered richly diverse microbial communities in the lungs of both healthy individuals and hospitalized patients, with perturbations in these populations implicated in many disease conditions (Douglas et al., 2009; Muhlebach et al., 2018). Micro-evolution of pathogens within these diverse communities has also received considerable attention with a growing appreciation for the extent of phenotypic heterogeneity present within these populations (Workentine et al., 2013; Clark et al., 2015; Darch et al., 2015; Grote et al., 2015; Jorth et al., 2015; Williams et al., 2015; Davies et al., 2017). The ability of pathogens to adapt and adopt multiple forms facilitates persistence and colonization in challenging niches such as the lungs of patients with respiratory disease. Mutations in key signaling pathways e.g., LasR are particularly prevalent among P. aeruginosa clinical isolates (Hoffman et al., 2009), with pigmented variants also reported in several studies (Mowat et al., 2011; Clark et al., 2015). Furthermore, re-wiring of regulatory networks in these clinical isolates has also been uncovered, suggesting a complex adaptive response to environmental challenges (Feltner et al., 2016; Wang et al., 2018). These changes can lead to phase dependent alterations in virulence (Cabeen, 2014). To enhance early treatment strategies of lung disease in CF, improvements in our understanding of factors that contribute to both pathogen acquisition and dysbiosis of the lower respiratory microbiota is essential.
Bile acids, one of the major components of bile, have been detected in the lungs of patients with respiratory disease, and have been shown to correlate directly with increased morbidity and colonization by P. aeruginosa (Zecca et al., 2008; Blondeau et al., 2010; Pauwels et al., 2012; Reen et al., 2014, 2016). This is further supported by several independent studies that show a correlation between bile reflux and pathogen colonization in patients with CF (van der Doef et al., 2009; Palm et al., 2012; Al-Momani et al., 2016), with evidence of increased inflammation and lung impairment also reported (Pauwels et al., 2012). Furthermore, both bile and bile acids have been shown to modulate the behavioral lifestyle of P. aeruginosa causing it to adopt a chronic biofilm lifestyle (Reen et al., 2012, 2016). This would appear to occur through transcriptional rewiring of the cell, with changes in virulence systems, signaling molecules, and key metabolic pathways including the glyoxylate shunt observed (Reen et al., 2016). While we have uncovered key insights into the mechanisms through which bile influences the behavior of P. aeruginosa, little is known about how the pathogen responds to long term exposure to bile challenge. This would be of particular interest considering the temporal nature of aspiration and its emerging role in shaping the microbiology of the lungs (Dickson et al., 2017).
This study was designed to assess the response of P. aeruginosa to long-term bile exposure. Increased production of pyocyanin (PYO) was initially observed and confirmed by transcriptional analysis. Over time, pigmented morphologies were observed exclusively in bile treated cultures. Characterization of these mutants revealed a loss of AQ signaling and PYO production with whole genome sequencing and single nucleotide polymorphism analysis revealing key genetic changes in the pigmented variants. These changes included point mutations in the quorum sensing regulator lasR. Although these pigmented mutants retained their response to bile with respect to antibiotic tolerance and swarming motility, they were notably unresponsive for biofilm formation and redox potential response, possibly due to defective AQ production. These findings implicate a role for AQs in the bile induced shift in redox potential with additional unidentified factors likely to be involved in the altered biofilm response to bile on the pigmented mutants.
Materials and Methods
Bacterial Culture
All cultures of P. aeruginosa were routinely grown in Tryptic soy broth (TSB) media or Luria-Bertani broth (LB) with shaking at 180 rpm at 37°C. PA14 and the transposon mutants described in this study were obtained from the PA14 non-redundant transposon insertion mutant library (Liberati et al., 2006). Strains were maintained on Tryptic Soy agar (TSA) or Luria-Bertani agar (LBA). For the purpose of the antibiotic tolerance assays Mueller-Hinton (MH) agar or broth was used. Bovine bile at a concentration of 0.3% (w/v) was added to media either prior to autoclaving at 105°C for 30 min or after autoclaving by filter sterilization with a 0.2 μm filter.
Pyocyanin Assay
Overnight cultures of P. aeruginosa were adjusted to an OD600nm 0.05 in artificial sputum media (ASM) with and without 3, 0.3, and 0.03% (w/v) bovine bile and incubated at 37°C with shaking at 180 rpm. After 24 and 96 h, test cultures (10 ml) were centrifuged at 5000 rpm for 15 min to obtain a cell free supernatant. Chloroform (3 ml) was added to the cell free supernatant, vortexed and centrifuged for 5 min at 5000 rpm. The bottom blue phase was transferred to a tube containing 2 ml of 0.2 M hydrochloric acid, vortexed, and centrifuged at 5000 rpm for 5 min. The absorbance of the top pink phase was read at OD520nm. Calculation of the concentration of pyocyanin (μg/mL) was undertaken according to the following: (OD520 nm × 17.072) × 1.5 to account for the dilution factor following transfer into the acidic phase.
Pyocyanin and Alkyl Quinolone Extraction
Overnight cultures of P. aeruginosa were adjusted to an OD600nm 0.02 in 20 ml TSB with or without 0.3% (w/v) bovine bile and incubated at 37°C with shaking at 180 rpm for 8 h. Culture was centrifuged at 5000 rpm for 15 min to obtain a cell free supernatant with resulting supernatant split into two 10 ml tubes. Pyocyanin was extracted as described above. Alkyl quinolones were extracted by addition of 10 ml of acidified ethyl acetate followed by vortexing and centrifugation for 5 min at 5000 rpm. The top clear phase was transferred to a fresh tube and stored at −20°C overnight. Rotary evaporation was completed to remove the solvent with extracts resuspended in 1 ml of methanol for analysis by thin layer chromatography.
RNA Isolation and Transcriptional Analysis
Three independent cultures of P. aeruginosa strain PA14 were cultured in the presence and absence of 0.3% (w/v) bile (Sigma) in ASM. Untreated and treated samples were inoculated at a starting OD600nm 0.02 and left to grow for 72 h at 37°C. Cultures were centrifuged at 3000 rpm for 10 min with the subsequent pellet resuspended in 1 ml of RNAProtect bacteria reagent (Qiagen). Total RNA was extracted using the RNAeasy kit (Qiagen) according to the manufacturer’s instructions and DNase treated using TURBO DNase (Ambion). cDNA was synthesized using QuantiTect reverse transcription kit (QIAGEN). Real time primers were designed utilizing the Universal Probe Library Assay Design Center (UPL, Roche). RealTime PCR was conducted on a Chromo4 Continuous Fluorescence Detector (MJ Research) using FastStart TaqMAN Probe Master and probes from the Universal ProbeLibrary (UPL, Roche). All gene expression levels are presented relative to the housekeeping gene, proC.
Thin Layer Chromatography
A normal phase silica TLC plate was prepared by immersing in a solution of potassium phosphate monobasic for half an hour. The TLC plate was activated in hybridization oven for 1 h at 100°C. 20 μl of extract were spotted onto the prepared TLC plate with synthetic PQS/HHQ loaded as controls. The plate was developed in 95:5 dichloromethane: methanol and visualized under UV light.
Cycled Culture of P. aeruginosa in Artificial Sputum Media
Artificial sputum media was prepared according to the protocol described by Sriramulu et al. (2005), and consists of mucin, salmon sperm DNA, diethylene triamine pentaacetic acid, sodium chloride, potassium chloride, Tris base, egg yolk emulsion, and casamino acids (Siramulu, 2010). ASM cultures were initiated by inoculation of 2 × 106 cells from an overnight culture of a PA14 mutS transposon mutant into 20 ml ASM in the presence and absence of 3% (w/v) bovine bile. As described in Wassermann et al. (2016) the mutS transposon mutant was selected due to the mutation in its DNA repair system resulting in enhanced mutation frequency therefore speeding up the rate of mutation and experimental outcome. Cultures were incubated at 37°C static in order to mimic the microanaerobic conditions within CF lung environment for 96 h, with transfer (1:100) into fresh ASM media after mechanical homogenization. Transfers were repeated for 180 days (45 transfers and approximately 315 bacterial generations).
Colony Morphology Assay
A total of 3 μl of overnight TSB cultures were spotted on to TSA with or without 0.3% (w/v) bovine bile. The spot was allowed to dry at room temperature before incubation at 37°C overnight. Plates were transferred back to room temperature, with colony morphology monitored and recorded over a period of 7 days.
Pigment Extraction
Strains of interest were inoculated at an OD600nm 0.05 in 50 ml of MH in a 250 ml conical flask for 48 h with visual indication of pigment production. Pigments were successfully extracted by centrifugation of 40 ml of culture at 5000 rpm for 10 min. Ethanol (10 ml) was added to 2 ml of the cell free culture supernatant. The supernatant/ethanol mixture was centrifuged at max speed for a further 10 min. The resulting supernatant was rotary evaporated with compounds collected using methanol. A preparative TLC was undertaken.
Biofilm Assay
Overnight cultures were adjusted to an OD600nm 0.05 in TSB in the presence and absence of bile. Aliquots of 1 ml were transferred in to 24-well plates and incubated static at 37°C overnight. Biofilm formation was measured by removing spent culture by pipetting. Wells were washed with water by gentle pipetting to remove any unattached biofilm. Attached biofilm was measured by staining for 30 min with 1 ml of 0.1% (w/v) crystal violet. 100% (v/v) ethanol was used to solubilize the crystal violet followed by a measurement of the absorbance at a wavelength of 595 nm.
Antibiotic Tolerance Assay
Overnight cultures of P. aeruginosa were adjusted to 0.5 MacFarland units which is the standard recommendation of the Clinical Laboratory Standard Institute in MH broth. MH agar plates supplemented with or without 0.3% (w/v) bile were uniformly swabbed with culture. E-strips (Thermo Fisher scientific) were placed on to the surface of the agar manually. Plates were left to incubate at 37°C overnight after which the zone of inhibition was recorded.
Swarming Motility Assay
Swarming motility was measured on 0.6% (w/v) Eiken Agar (Eiken Chemical Tokyo) in the presence and absence of 0.3% (w/v) bile. Sterile toothpicks were used to gently inoculate a single colony onto the surface of the Eiken Agar with minimal pressure. Plates were incubated overnight for 1–2 days with degree of motility visualized and recorded.
Redox Assay
Overnight cultures of P. aeruginosa were adjusted to OD600nm 2.0 with 200 μl of adjusted cultured added to 25 ml of TSB supplemented with 0.01 mg/ml of tetrazolium violet. Cultures were incubated at 37°C shaking at 180 rpm for 24 h. Formazan production was measured by centrifuging 5 ml of culture at 5,000 g for 5 min. The supernatant was discarded with the pelleted cells re-suspended in 1.2 ml of dimethyl sulfoxide and centrifuging again at 5,000 g for 5 min. The OD510nm was recorded of the cell free supernatant.
Whole Genome Sequencing
Genomic DNA was extracted from bacterial strains of interest using a Gentra PureGene DNA extraction kit (QIAGEN) with isolated DNA re-suspended in sterile water. Paired end sequencing was conducted by Eurofins Genomics using Illumina MiSeq V3 with 2 × 300 bp reads. Reads were mapped to the P. aeruginosa UCBPP-PA14 NC_008463.1 reference genome and delivered as BAM and BAI files. Further sequence analysis for SNP identification was conducted using the Integrative Genome Viewer software platform using the reference strain UCBPP-PA14 and the more recent PA14 Or genome sequence (NZ_LT608330.1). This Whole Genome Shotgun project has been deposited at DDBJ/ENA/GenBank under the accession SZXO00000000 (red pigmented mutant), SZXP00000000 (brown pigmented mutant), and SZXQ00000000 (yellow pigmented mutant).
Results
Pyocyanin Production Is Elevated in the Presence of Bile in ASM
Bile has emerged as a key host factor capable of modulating behavioral changes in the life cycle of the opportunistic respiratory pathogen P. aeruginosa (Reen et al., 2012, 2016). Transcriptomic analysis conducted in the presence of bile has previously revealed changes in the expression of an array of quorum sensing and PYO related genes (Reen et al., 2016). Furthermore, the observed shift in the redox status to a more reduced level was also notable considering the inherent relationship between PYO (and other phenazine pigments) and the maintenance of redox homeostasis within the cell. Therefore, we investigated the impact of bile on PYO production in P. aeruginosa PA14 grown in ASM, a growth medium that is known to mimic conditions found within the CF lung environment.
Bile, at various sub-inhibitory concentrations was shown to significantly increase production of PYO in P. aeruginosa in ASM when compared to untreated cultures (Figure 1A). Induction was evident at 24 h incubation but was most pronounced at 96 h in the presence of 3% (w/v) bile. The range of bile concentrations tested are physiologically relevant, with 0.03–0.3% (w/v) bile previously shown to elicit a behavioral switch in P. aeruginosa (Reen et al., 2012). Higher concentrations of 3% would be encountered in the gastrointestinal tract where P. aeruginosa may possibly be conditioned prior to aspiration into the lungs of at risk patients (Reen et al., 2012). Induction was evident at 24 h incubation but was most pronounced at 96 h in the presence of 3% (w/v) bile. At 24 h, concentrations of pyocyanin went from 7.59 μg/mL in the absence of bile to 8.82, 9.85, and 9.01 μg/mL in the presence of 0.03, 0.3, and 3% (w/v) bile, respectively. At 96 h, concentrations of pyocyanin went from 3.31 μg/mL in the absence of bile to 4.22, 6.27, and 14.13 μg/mL in the presence of 0.03, 0.3, and 3% (w/v) bile, respectively. Gene expression analysis as measured by qRT-PCR on three branch point PYO biosynthetic genes (phzH, phzM, and phzS) revealed a significant increase in the expression of phzS in bile treated ASM cultures (Figure 1B). A trend toward increased expression of phzH and phzM was also observed although it did not reach statistical significance. phzS, encoding a flavin-containing monooxygenase, is the final gene of the PYO biosynthetic pathway responsible for the conversion of 5-methylphenazine-1-carboxylic acid betaine to PYO. The increased expression of this gene may underpin the overproduction of PYO following P. aeruginosa exposure to bile.
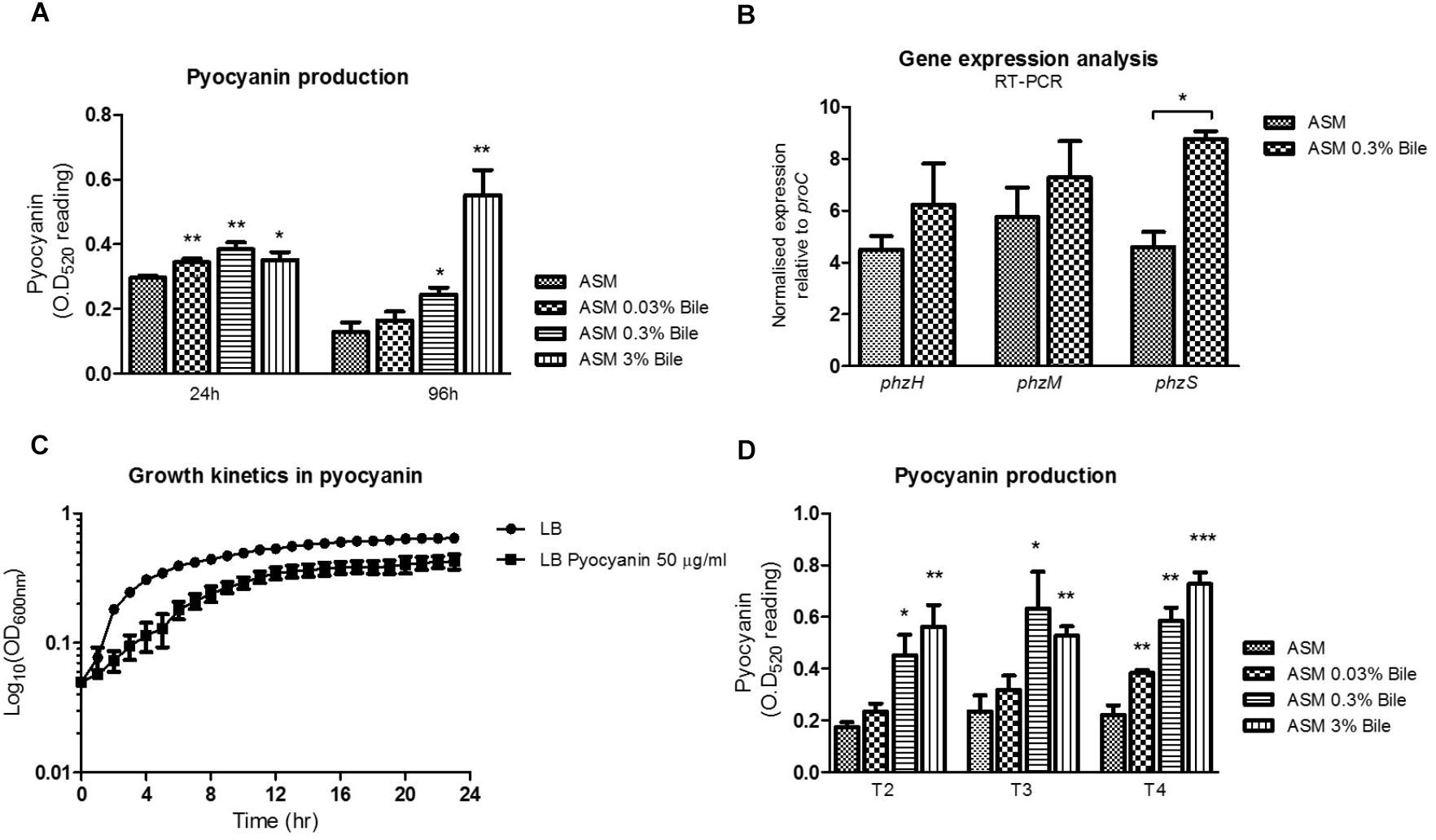
Figure 1. (A) The phenazine PYO is elevated in ASM supplemented with a range of bile concentrations. (B) Enhanced PYO production is underpinned by increased phzS expression, the final step of the PYO biosynthetic pathway, as measured by RT-PCR analysis. No significant change in gene expression was observed for phzH or phzM, the two other central genes in PYO biosynthesis. (C) PYO, at a concentration of 50 μg/mL reduces the growth rate and biomass of Pseudomonas aeruginosa. (D) Serially cycling P. aeruginosa in ASM supplemented with bile confirms the consistent up-regulation of PYO production over time. Data is the mean of at least three independent biological replicates. Statistical analysis was performed by Student’s t-test (∗p ≤ 0.05; ∗∗p ≤ 0.01; ∗∗∗p ≤ 0.001).
Growth curve analysis was undertaken to investigate the impact that the accumulation of PYO within the microbial community may have on growth rate. An upper range concentration of 50 μg/mL, which may be representative of the accumulation of elevated levels of pyocyanin observed in bile treated cultures, was chosen for these experiments. A recent study by Meirelles et al. has shown that elevated levels of PYO can have both beneficial and detrimental effects on P. aeruginosa populations (Meirelles and Newman, 2018). Consistent with these findings we found that a concentration of 50 μg/mL of PYO reduced the growth rate and resulting biomass of P. aeruginosa (Figure 1C). To investigate the effects of longer-term bile exposure on PYO production, P. aeruginosa was serially transferred into fresh ASM at 96 h intervals. Subsequent PYO analysis following three rounds of 96 h growth cycles confirmed that PYO levels remain elevated following the prolonged exposure to bile (Figure 1D). Therefore, it was essential to understand the mechanism(s) by which P. aeruginosa might adapt to these elevated, toxic levels of PYO which would potentially represent a selective pressure for the evolution of P. aeruginosa within a bile positive lung environment.
Hyper-Biofilm Forming Pigmented Variants of Pseudomonas aeruginosa Emerge Exclusively in the Presence of Bile
Upon confirmation that PYO levels remained elevated upon sequential transfer of P. aeruginosa in ASM supplemented with bile, serial transfers were performed for a duration of 6 months encompassing approximately 45 transfer events (n = 3). Biofilm analysis of 9 randomly selected end-point isolates from untreated ASM and 9 randomly selected end-point isolates from bile treated ASM isolated from 3 independent biological replicates revealed that bile treated ASM isolates displayed elevated biofilm levels relative to untreated ASM isolates (Figure 2). Consistent with the findings of Davies et al. (2017), swarming motility appeared to be dramatically reduced in the untreated ASM colonies. However, there was an apparent selection for the maintenance of swarming motility in several of the bile treated ASM colonies. These findings were further confirmed by larger scale analysis of randomly selected isolates taken from the final replicate of the adaptive experiment encompassing 48 colonies from bile treated ASM and 48 colonies from untreated ASM. The same pattern of enhanced biofilm and retention of swarming motility was observed in colonies selected from the bile treated ASM samples when compared with those selected from ASM alone (Supplementary Figures 1, 2).
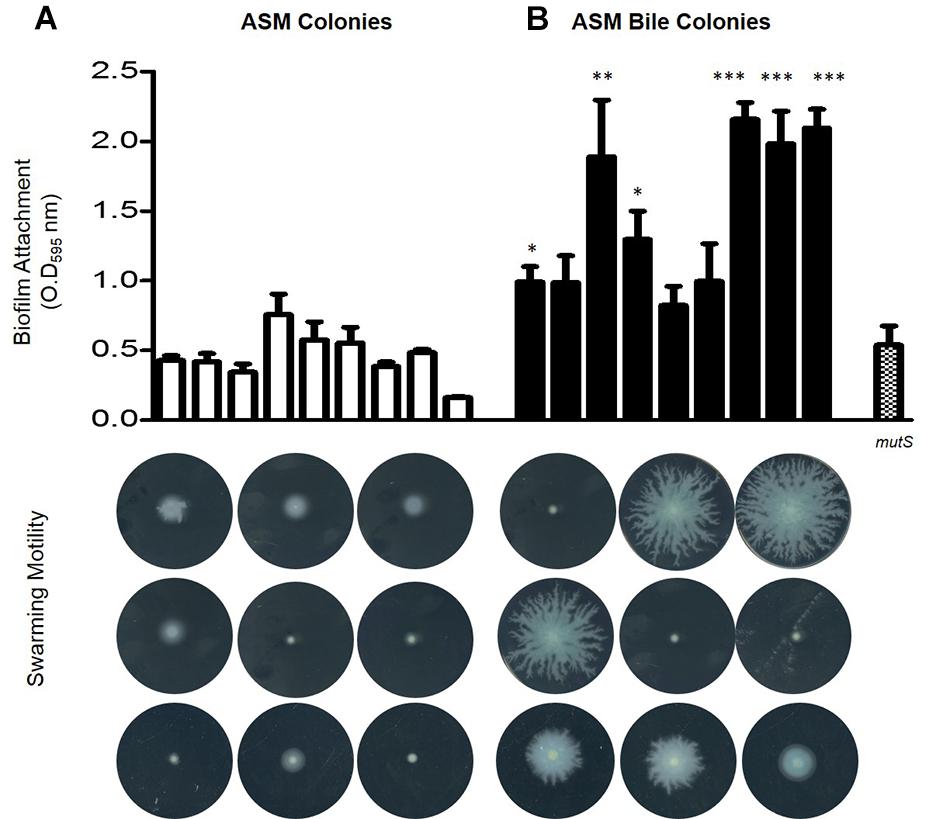
Figure 2. Phenotypic analysis encompassing biofilm formation and swarming motility in 9 colonies from three independent biological replicates isolated from (A) untreated ASM and (B) bile treated ASM. Data is the mean of at least three independent biological replicates. Statistical analysis was performed by Student’s t-test (∗p ≤ 0.05; ∗∗p ≤ 0.01; ∗∗∗p ≤ 0.001).
Colony morphology analysis revealed the emergence of uniquely pigmented variants of P. aeruginosa derived from ASM in the presence of bile, with yellow, red and brown derivatives observed (Supplementary Figures 3A–D). The yellow pigmented variant was the first to emerge, followed by the brown and red pigmented variants, respectively. The yellow pigmented variant became the most dominant member of the community representing approximately 60% of the population with the brown and the red variants comprising approximately 10 and 5% of the population, respectively, upon completion of the experiment. Interestingly, though the brown and red derivatives were maintained in the community, they did not significantly increase in abundance with time. This suggests that there may be some fitness cost preventing them from outcompeting the progenitor wild-type phenazine producing genotype within the population. In contrast to the bile treated ASM cultures, the majority of colonies obtained from untreated ASM appear to either retain green pigmentation, most likely a result of intact phenazine production, or lose pigmentation completely (Supplementary Figure 3A). Furthermore, colony morphologies of the untreated ASM cells appeared larger and more rugose than their bile treated counterparts, which were smaller and more smooth (Supplementary Figure 3A).
Genotypic Profiling of the Pigmented Variants
As the pigmented derivatives emerged exclusively in the presence of bile, red pigmented variants (n = 3), brown pigmented variants (n = 3), and yellow pigmented variants (n = 3) were selected for further analysis in order to elucidate their functional importance in the presence of bile. Colony morphology analysis confirmed the stable production of the brown/red/yellow pigment which appears to be extruded from the colony (Figure 3A). However, in the presence of bile there appears to be a further increase in the production of these pigments. Therefore, though a mutational event likely underpins the production of these alternative pigments, the intact regulatory pathway governing the regulation of pigment production appears to still be responsive to bile (Figure 3B).
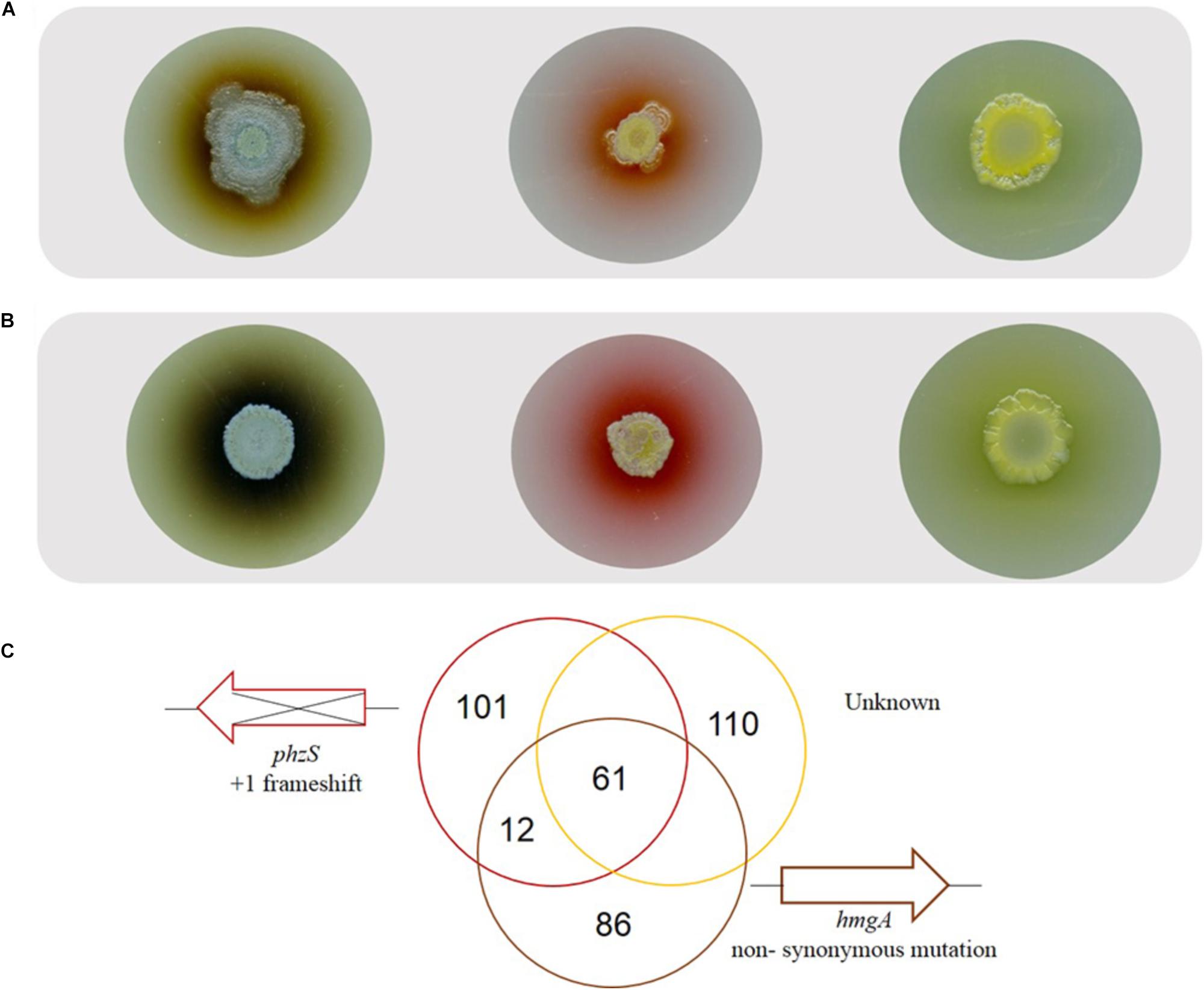
Figure 3. (A) Representative colony morphology analysis of the brown, red, and yellow pigmented variants on TSB agar and (B) TSB agar supplemented with bile highlighting pigment production with further induction in the presence of bile. (C) Whole genome sequence analysis on representative pigmented isolates with sequence comparison analysis undertaken with PA14 or as the reference strain.
Whole genome sequencing analysis was conducted on a representative red, brown and yellow pigmented isolate. Sequence validation following PCR amplification using gene-specific primers was carried out on selected target genes in the mutS ancestral strain. While all three isolates were found to have 281 SNP’s in common when using the UCBPP-PA14 reference strain, this number was reduced down to 61 SNP’s when using PA14 Or as a reference strain (Figure 3C). This finding is important when considering the use of these reference genomes for SNP analysis of P. aeruginosa clinical isolates and highlights the potential importance of using multiple reference genomes in clinical genome analysis. The genetic events within each of the individual isolates described below with indels in coding regions representing the most important category as these will definitively alter the protein (Table 1). These indels are further outlined in Supplementary Figure 4.
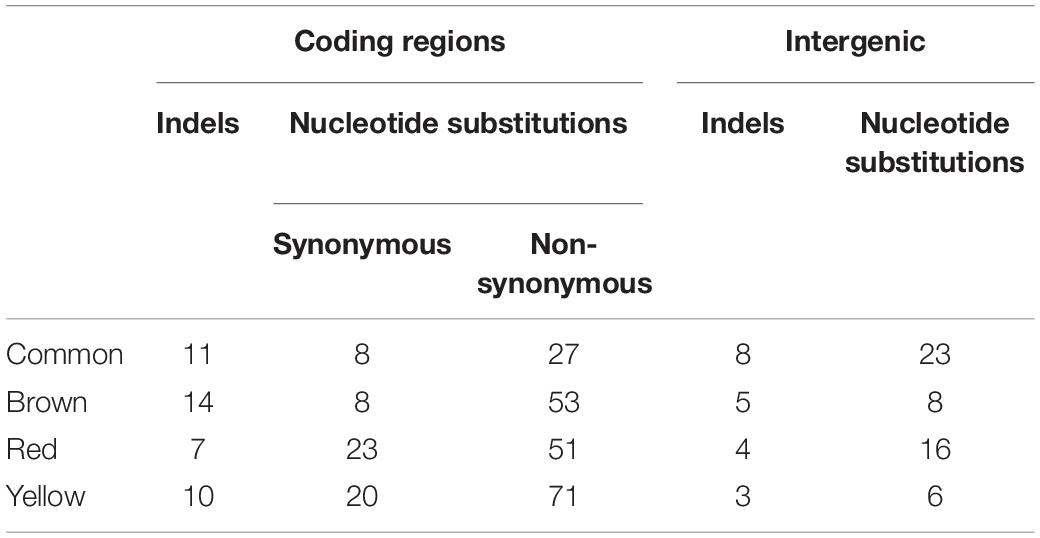
Table 1. Genetic events present in all three pigmented variants, the brown pigmented variant alone, the red pigmented variant alone, and the yellow pigmented variant alone.
Within the genome of the brown pigmented variant a single base pair substitution at nucleotide 984 of a T to a C was identified in the hmgA gene. This non-synonymous substitution results in a change of the amino acid phenylalanine (TTC) to leucine (CTC). This single base pair mutation was also located in the hmgA gene of the two other brown pigmented variants tested, as confirmed by sequencing of PCR amplicons (Supplementary Figure 5). Loss of function mutations in hmgA have previously been shown to result in production of the brown polymeric pigment pyomelanin by P. aeruginosa (Rodriguez-Rojas et al., 2009). However, further characterization will be required to definitively attribute production of the brown pigment to the SNP identified in this study.
Within the genome of the red pigmented variant sequence analysis revealed the insertion of a C nucleotide at position 125 of the phzS encoded protein. The production of a red pigment in P. aeruginosa has previously been reported for phzS mutants, suggesting that this insertion underpins the pigmentation the red variant (Gibson et al., 2009). The insertion of a C nucleotide at position 125 resulted in a+1 frameshift mutation which was confirmed by PCR sequencing and extended to all other red variants tested (Supplementary Figure 5). Interestingly, subsequent plating of red pigmented variants consistently led to loss of pigmentation in a subset of colonies. Sequencing of these colonies revealed reversion back to the original ancestral sequence. This is consistent with previous studies describing a high frequency of reversion for +1 frameshift mutations in a mutS mutant (Smania et al., 2004). This finding validated that insertion of the single C nucleotide into the phzS sequence was responsible for production of the red pigment in these mutants. Additionally, pigment extraction and preparative TLC analysis from a phzS transposon mutant and the red derivative further reinforced these findings with an RF value of 0.92 obtained for both the phzS mutant and the red pigmented isolate. While the potential causative mutations could be identified for the red and brown pigmented variants, no likely mutation could be located that could underpin production of the yellow pigment. The phzM gene and upstream regions including the promoter region were unchanged in all strains. It is possible that another mutation within the genome has affected the expression of phzM and therefore production of the yellow pigment could still be attributed to altered phzM expression. However, previous studies have also shown that insertional mutagenesis of rhlI results in yellow pigmentation, possibly as a consequence of increased pyoverdine production (Orlandi et al., 2015). Therefore, elucidating the origin of the yellow pigment identified in this study will require further mechanistic investigation.
Phenotypic Profiling of Pigmented Mutant Reveals Altered Biofilm Response to Bile
As the strongest evidence of a causative mutation was found in the red pigmented variant, three representative isolates of this variant were phenotypically profiled in tandem with a phzS transposon mutant, the ancestral progenitor mutS transposon mutant and the PA14 WT strain. Key phenotypes associated with the chronic lifestyle, including biofilm formation, swarming motility, and antibiotic tolerance, were tested (Figure 4). Importantly, all three phenotypes were previously shown to be modulated in response to bile where biofilm and antibiotic tolerance were both increased, while swarming motility was repressed (Reen et al., 2012, 2016). As the red pigmented mutants arose solely in the presence of bile supplemented ASM, we reasoned that these mutants may respond differently to the wild-type strain when challenged with this important host factor.
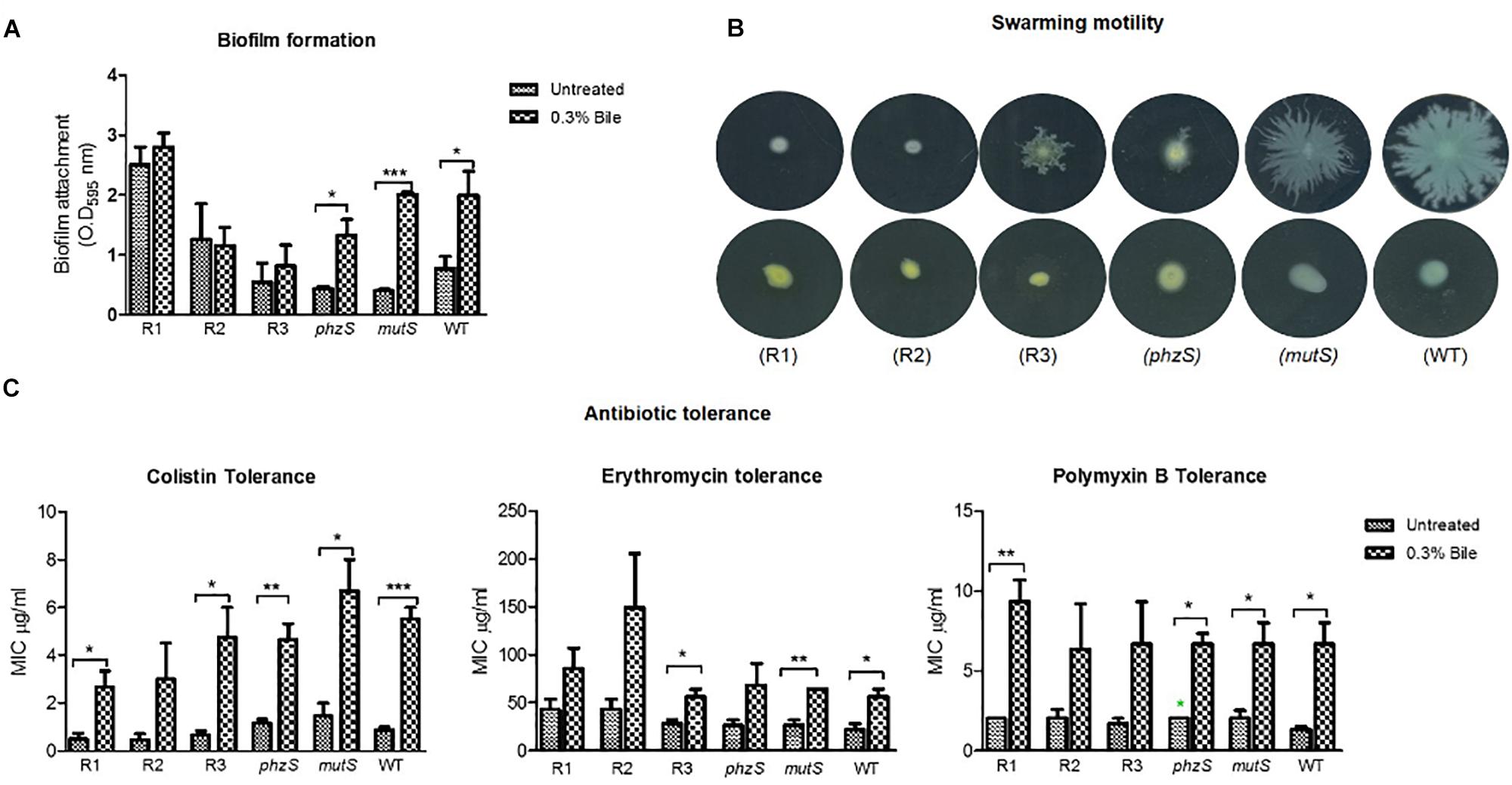
Figure 4. Phenotypic profiling in the presence and absence of bile of the red pigmented derivatives, phzS and mutS transposon mutants and the PA14 WT strain for (A) biofilm formation. (B) Swarming motility. (C) Antibiotic tolerance. Data is the mean of at least three independent biological replicates. Statistical analysis was performed by Student’s t-test (∗p ≤ 0.05; ∗∗p ≤ 0.01; ∗∗∗p ≤ 0.001).
In respect of biofilm formation, the bile-induced biofilm formation evident in the wild-type and mutS transposon mutant was not observed in the red pigmented isolates. One of the three red pigmented mutants tested exhibited a higher level of biofilm formation when compared with the wild-type and mutS mutant. This is expected on the basis of the high levels of biofilm formation in the bile treated ASM colonies. However, there was no significant increase in biofilm formation in any of the red variants in the presence of bile (Figure 4A). The phzS mutant exhibited a comparable increase in biofilm to the wild-type and mutS mutant, suggesting that pigment production alone is not responsible for the loss of enhanced biofilm formation in the presence of bile. All three red pigmented variants were found to be swarming mutants, as was the case for the phzS transposon mutant (Figure 4B). In contrast, the mutS transposon mutant exhibited wild-type swarming motility which was suppressed in the presence of bile. The increase in tolerance to macrolide and polymyxin antibiotics was also apparent in the red pigmented variants while also being retained in the phzS mutant (Figure 4C). However, the trend toward increased antibiotic tolerance did not reach significance in the R2 red pigmented variant. Notwithstanding their increased tolerance to colistin in the presence of bile, it was notable that the red pigmented mutants were themselves more susceptible to colistin when compared directly with the mutS mutant and wild-type strain. The differential biofilm response between the red derivatives and the phzS transposon mutant further suggests that other factors may be involved in mediating enhanced biofilm in response to bile in P. aeruginosa.
The perturbations of redox homeostasis appear intricately connected to the ability of P. aeruginosa to thrive in bile. Transcriptional profiling has previously shown changes in the expression of genes encoding metabolic pathways, while the redox profile was shown to be repressed in the presence of bile (Reen et al., 2016). Furthermore, the functional role of pigments within P. aeruginosa is linked to the maintenance of redox homeostasis within the cell (Dietrich et al., 2006; Price-Whelan et al., 2007). Therefore, the redox status of the pigmented variants was investigated and compared to the original ancestral isolate. The phzS transposon mutant exhibited a similar response to the WT and mutS mutant, with a repression of redox in the presence of bile (Figure 5). However, while redox potential in the pigmented variants was comparable to wild-type, mutS, and phzS transposon mutants in the absence of bile, no reduction in potential was evident upon challenge with bile. This indicates that redox potential is “locked in” in these variants and the metabolic changes that underpin a reduction in redox potential in response to bile may not occur in these strains.
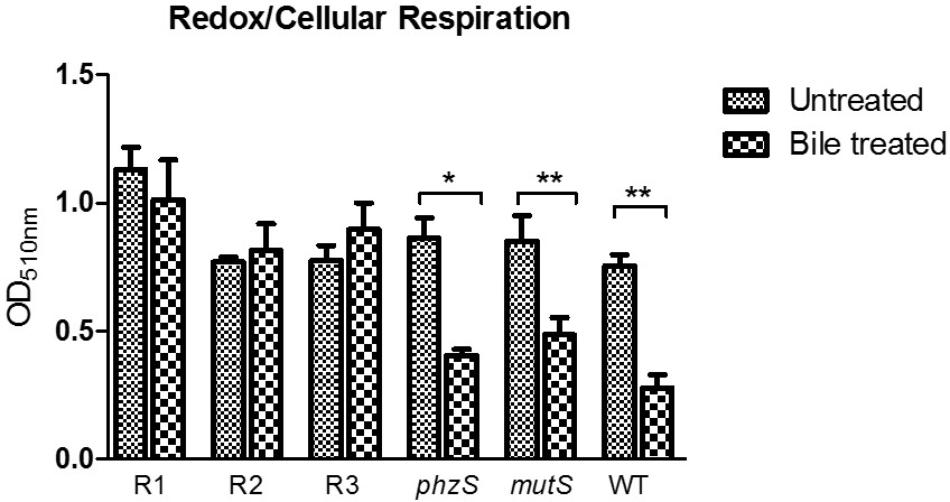
Figure 5. Tetrazolium violet redox assay revealing the lack of repression of redox in the presence of bile in the red pigmented variants with a repression of redox evident in the phzS mutant. Data is the mean of at least three independent biological replicates. Statistical analysis was performed by Student’s t-test (∗p ≤ 0.05; ∗∗p ≤ 0.01).
Akyl Quinolone Signaling Is Central to an Effective Bile Response in P. aeruginosa
The observation that pigmented variants no longer exhibited increased biofilm formation or reduced redox potential in the presence of bile led us to further investigate the possible mechanisms involved. Our finding that a phzS transposon mutant still retained an intact biofilm response to bile suggested that neither pigment production alone, nor loss of PYO production through mutation of phzS, were solely responsible. This raised the intriguing possibility that another signaling system was involved, potentially upstream of PYO, with the AHL-PQS axis considered a likely target. Evidence supporting this hypothesis was provided through SNP analysis whereby LasR was found to exhibit a single amino acid change in both the red and brown mutants from independent replicates of the serial cycling experiment (Supplementary Table 1). Loss of LasR functionality has been previously shown to result in decreased levels of PQS within the cell, with lasR mutations described in clinical isolates of P. aeruginosa isolated from the lungs of CF patients (Hoffman et al., 2009). Interestingly, the yellow pigmented mutants did not show any change in lasR sequence suggesting that mutation of lasR occurred after or independent of the emergence of the yellow variant.
Analysis of AQ signal and PYO production by Thin Layer Chromatography revealed altered profiles in the red pigmented mutants, consistent with their genotypic profile as putative lasR mutants. PQS production was abolished in these strains, while its biological precursor HHQ was retained, comparable to the profile of a pqsH mutant (Figure 6A). Interestingly, increased HHQ production was observed in the presence of bile in these strains, indicating that autoinduction by PQS is not required to elicit increased activation of the biosynthetic operon in the presence of bile. It was also notable that both PQS and HHQ production was retained in the phzS mutant. As expected, PYO production was also abolished in the red pigmented mutants, unsurprising given the loss of phzS activity (data not shown).
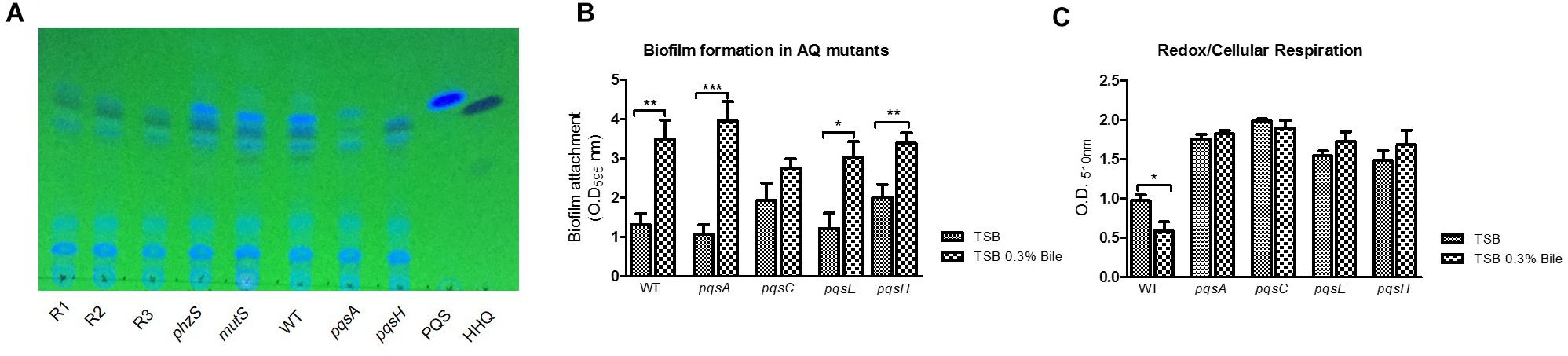
Figure 6. (A) TLC analysis indicates loss of PQS production in pigmented mutants, consistent with the possible loss of lasR identified in the SNP comparisons. A phzS mutant retains PQS production, indicating loss of PQS occurs independent of pigment production. (B) Mutation of the biosynthetic genes for PQS did not significantly alter bile induced hyper-biofilm formation. (C) Mutation of the biosynthetic genes for PQS resulted in a loss of bile induced redox repression. Data is the mean of at least three independent biological replicates. Statistical analysis was performed by Student’s t-test (∗p ≤ 0.05; ∗∗p ≤ 0.01; ∗∗∗p ≤ 0.001).
From the community perspective, loss of PQS signaling might be expected to result in a less competitive sub-population of P. aeruginosa. This is in light of its central role in virulence regulation and its emerging role as an interkingdom effector (Reen et al., 2011; Toyofuku et al., 2017). While loss of PQS would result in the reduction of potentially toxic PYO levels within the cell, this would already be achieved by mutation of the phzS gene. Therefore, the role of PQS in response to bile may be a more central mechanism to the adaptation of the global population, within what would appear to be an enhanced biofilm community.
To investigate this, several mutants affected for AQ signaling were tested for their biofilm and redox response to bile. Loss of the PQS biosynthetic genes did not result in the loss of bile induced biofilm formation. This suggested that HHQ and other AQs produced by the pqsA-E operon were not required for a functional bile-induced biofilm response (Figure 6B). Interestingly, the loss of the PQS biosynthetic genes resulted in a loss of redox repression in the presence of bile with redox potential considerably higher than the WT counterpart in these mutants (Figure 6C). This finding would strongly implicate a role for alkyl-quinolones in the perturbations of redox potential observed in the presence of bile with the red and brown pigmented derivatives representing bile-adapted redox isolates.
Discussion/Conclusion
The clinical treatment of respiratory disease is faced with several challenges, not least the rapid decline in novel antibiotic discovery (Cooper and Shlaes, 2011). Innovative approaches to pathogen control have been the focus of intensive research efforts, yet resistance continues to increase globally in spite of improved stewardship and hygiene control (Van Puyvelde et al., 2018). Studies into host factors capable of modulating bacterial behavior are important initiatives facilitating the development of these innovative intervention strategies. There is increasing evidence implicating a role for gastro-oesophageal reflux and subsequent pulmonary aspiration in the progression of CF and other chronic respiratory diseases (van der Doef et al., 2009; Palm et al., 2012; Pauwels et al., 2012; Reen et al., 2014, 2016; Dickson et al., 2017). To date, experimental analysis investigating bile/bacterial interactions has focused on phenotypic responses linked to the acute-chronic switch in P. aeruginosa and pathogenesis in general in gastrointestinal pathogens. However, little is known about the consequences for longer term exposure to bile in these organisms and the nature of the adaptation that might occur. Therefore, in this study we performed a growth cycling experiment with P. aeruginosa cultured in ASM in the presence and absence of bile, a synthetic media designed to more closely mimic conditions encountered within the sputum rich CF lung. This revealed that global adaptation to bile resulted in enhanced biofilm formation with mutations occurring in signaling systems and lasR genes underpinning the emergence of pigmented derivatives.
Phenazine production has been shown to enhance anaerobic survival in P. aeruginosa (Glasser et al., 2014), while phenazines have also been shown to influence the physiology of host cells and competing organisms. Production of the phenazine pigment PYO was significantly increased in response to bile in ASM cultures after 24 and 96 h. The increased levels of PYO in the cultures were found to be toxic to the growth of P. aeruginosa. This key virulence factor contributes to the pathogenicity of P. aeruginosa with significant quantities of this redox-active molecule recovered from the lungs of infected CF patients (Caldwell et al., 2009). The production of PYO is under control of the quorum sensing signaling system and its production involves two distantly encoded biosynthetic operons (phzA-G), as well as the modification genes phzH/M/S. Several studies have linked production of PYO to redox and virulence processes in P. aeruginosa (Dietrich et al., 2006; Price-Whelan et al., 2007; Meirelles and Newman, 2018). PYO has been shown to cause direct damage to human host cells, inhibiting cellular respiration and inducing neutrophil apoptosis (Allen et al., 2005; Manago et al., 2015; Heirali et al., 2016). The importance of PYO was demonstrated in both acute and chronic mouse models, where PYO mutants were less competitive than their wild-type counterparts. PYO is known to play an important role in the establishment of biofilms in P. aeruginosa. It is responsible for extracellular DNA release resulting from auto-poisoning and cell death (Das and Manefield, 2012), while also promoting survival of oxidant limited cells within biofilms (Meirelles and Newman, 2018). PYO has also been shown to enhance biofilm formation through second messenger c-di-GMP signaling and its ability to serve as an alternative terminal electron acceptor within the hypoxic biofilm. A recent study by Meirelles et al. has shown that PYO production may have both toxic and beneficial effects (Meirelles and Newman, 2018), analogous in some ways to the role proposed for PQS by Haussler and Becker (2008). PYO-tolerant sub-populations were shown to emerge from nutrient-limited biofilms. In contrast, a susceptible population was sacrificed for eDNA release and structural support in a “net-benefit” arrangement for the population. It is possible therefore that the emergence of pigmented variants that no longer produce PYO following long term exposure to bile, is an adaptation mechanism within the population to the elevated “toxic” levels encountered in the bile positive artificial sputum environment. Elevated toxic levels of PYO may contribute to the selection of a sub-population of PYO defective mutants within the ASM bile microenvironment. Further study is required to elucidate the impact of enhanced pyocyanin production within a bile positive CF lung on the evolutionary trajectory of residing P. aeruginosa populations.
The occurrence of pigmented variants within the lungs of patients with respiratory disease has been reported for decades (Meader et al., 1925; Wahba, 1965; Clark et al., 2015; Hocquet et al., 2016; Ketelboeter and Bardy, 2017). Distinction between red and brown pigmented clinical isolates was complicated further by the observation of some isolates that turned from “yellowish to red” (Gessard, 1920). Advances in sequencing and high throughput screening technologies have reinforced the finding that P. aeruginosa populations within the lungs of patients with CF are not uniform, but rather display a remarkable level of genotypic and phenotypic heterogeneity (O’Brien et al., 2017). The rationale underpinning the function of these pigmented mutants within lung populations remains to be understood. In our study, while maintained within the growth cycled populations in bile treated ASM, neither the brown nor the red pigmented mutants ever exceeded more than 10% of the population. This would suggest that their function may be crucial in maintaining a PYO positive population of P. aeruginosa. Reversion of some colonies following subsequent culturing of red pigmented variants would support this hypothesis. Indeed, the frequency of red pigmented variants in clinical samples is low, ranging from 3.5–6% (Ogunnariwo and Hamilton-Miller, 1975). Brown pyomelanin pigmented variants have been reported at higher frequencies of up to 13% in chronically infected CF patients (Mayer-Hamblett et al., 2014). The selective pressure underpinning the emergence of these pigmented variants in the lungs, urinary tract, and wounds of patients’ remains to be determined. Increased persistence through maintenance of redox-balanced populations within biofilms (Meirelles and Newman, 2018), intraspecific competition through production of pyocins (Hocquet et al., 2016), and tolerance to oxidative stress (Rodriguez-Rojas et al., 2009), may all contribute to the necessity for these pigmented sub-populations.
The quorum sensing AQ signaling system is an effective strategy utilized by P. aeruginosa allowing a coordinated gene expression response at the population level. This level of regulation serves as an additional global mechanism of adaptation to external stimuli and has been demonstrated to play a role in both virulence factor production and biofilm formation (Yang et al., 2007; Dubern and Diggle, 2008). The pseudomonas quinolone signal (PQS) has been shown to exert differential effects on individual members of communities of P. aeruginosa. It is capable of both sensitizing cells to external stresses and also inducing effective stress responses (Haussler and Becker, 2008). The presence of high levels of PQS has been shown to induce cellular autolysis whilst remaining unaffected cells are triggered to transition to a reduced metabolic and increased PQS tolerant state. The synergy between this selective impact on a population and the recent findings related to the action of PYO on P. aeruginosa populations is intriguing and points to a concerted control of populations. As with PYO, PQS represents a central factor in the modulation of population structure and adaptation. The red pigmented derivative was shown to be defective in the production of PQS but not its precursor HHQ, possibly as a consequence of changes in lasR sequence. As with the loss of PYO production in a subpopulation of cells, the global reduction of the PQS signaling molecule may represent a successful strategy offsetting any negative effects resulting from over production in a sputum rich environment. The proposed concentration dependent “housekeeping” function of PQS and pyocyanin, whereby both positive and detrimental effects on P. aeruginosa communities have been reported are consistent with the emergence of sub-populations in response to extended bile exposure. The molecular mechanisms underlying the emergence of these variants and the collective contribution of each pigmented strain on the persistence of the community as a whole will be the focus of further study to better understand the pathogenesis of P. aeruginosa in the lungs of patients with respiratory disease. Furthermore, taking cognizance of the phenotypic and genotypic heterogeneity that exists within P. aeruginosa communities in the lung, extension of this study to model (e.g., wild-type PAO1 and PA14) and clinical strains will inform whether the adaptive trajectories described here will also be observed within natural populations.
Data Availability
The datasets generated for this study can be found in NCBI, PRJNA535434.
Author Contributions
FR and FO’G conceived the study and supervised the research. SF, FR, and FO’G designed the study. SF performed the laboratory experiments and wrote the manuscript with FR. FO’G provided the critical feedback on the manuscript.
Funding
This research was supported in part by grants from the Enterprise Ireland (CF-2017-0757-P) and Health Research Board/Irish Thoracic Society (MRCG-2018-16) to FR and FO’G and Enterprise Ireland (IP-2015-0390) to FO’G, the European Commission (EU2020-634486-2015), the Science Foundation Ireland (SSPC-2, 15/TIDA/2977 and SFI09/RFP/BMT2350), the Irish Research Council for Science, Engineering and Technology (GOIPG/2014/647), the Health Research Board/Irish Thoracic Society (MRCG-2014-6), and the Cystic Fibrosis Foundation, United States (OG1710).
Conflict of Interest Statement
The authors declare that the research was conducted in the absence of any commercial or financial relationships that could be construed as a potential conflict of interest.
Acknowledgments
The authors thank Niall Dunphy for performing the qRT-PCR experiments.
Supplementary Material
The Supplementary Material for this article can be found online at: https://www.frontiersin.org/articles/10.3389/fmicb.2019.02013/full#supplementary-material
References
Allen, L., Dockrell, D. H., Pattery, T., Lee, D. G., Cornelis, P., and Hellewell, P. G. (2005). Pyocyanin production by Pseudomonas aeruginosa induces neutrophil apoptosis and impairs neutrophil-mediated host defenses in vivo. J. Immunol. 174, 3643–3649. doi: 10.4049/jimmunol.174.6.3643
Al-Momani, H., Perry, A., Stewart, C. J., Jones, R., Krishnan, A., Robertson, A. G., et al. (2016). Microbiological profiles of sputum and gastric juice aspirates in cystic fibrosis patients. Sci. Rep. 6:26985. doi: 10.1038/srep26985
Blondeau, K., Pauwels, A., Dupont, L., Mertens, V., Proesmans, M., Orel, R., et al. (2010). Characteristics of gastroesophageal reflux and potential risk of gastric content aspiration in children with cystic fibrosis. J. Pediatr. Gastroenterol. Nutr. 50, 161–166. doi: 10.1097/MPG.0b013e3181acae98
Burney, P., Jarvis, D., and Perez-Padilla, R. (2015). The global burden of chronic respiratory disease in adults. Int. J. Tuberc. Lung Dis. 19, 10–20. doi: 10.5588/ijtld.14.0446
Cabeen, M. T. (2014). Stationary phase-specific virulence factor overproduction by a lasR mutant of Pseudomonas aeruginosa. PLoS One 9:e88743. doi: 10.1371/journal.pone.0088743
Caldwell, C. C., Chen, Y., Goetzmann, H. S., Hao, Y., Borchers, M. T., Hassett, D. J., et al. (2009). Pseudomonas aeruginosa exotoxin pyocyanin causes cystic fibrosis airway pathogenesis. Am. J. Pathol. 175, 2473–2488. doi: 10.2353/ajpath.2009.090166
Caudri, D., Turkovic, L., Ng, J., de Klerk, N. H., Rosenow, T., Hall, G. L., et al. (2018). The association between Staphylococcus aureus and subsequent bronchiectasis in children with cystic fibrosis. J. Cyst. Fibros. 17, 462–469. doi: 10.1016/j.jcf.2017.12.002
Clark, S. T., Caballero, J. D., Cheang, M., Coburn, B., Wang, P. W., Donaldson, S. L., et al. (2015). Phenotypic diversity within a Pseudomonas aeruginosa population infecting an adult with cystic fibrosis. Sci. Rep. 5:10932.
Cooper, M. A., and Shlaes, D. (2011). Fix the antibiotics pipeline. Nature 472:32. doi: 10.1038/472032a
Cutting, G. R. (2015). Cystic fibrosis genetics: from molecular understanding to clinical application. Nat. Rev. Genet. 16, 45–56. doi: 10.1038/nrg3849
Darch, S. E., McNally, A., Harrison, F., Corander, J., Barr, H. L., Paszkiewicz, K., et al. (2015). Recombination is a key driver of genomic and phenotypic diversity in a Pseudomonas aeruginosa population during cystic fibrosis infection. Sci. Rep. 5:7649. doi: 10.1038/srep07649
Das, T., and Manefield, M. (2012). Pyocyanin promotes extracellular DNA release in Pseudomonas aeruginosa. PLoS One 7:e46718. doi: 10.1371/journal.pone.0046718
Davies, E. V., James, C. E., Brockhurst, M. A., and Winstanley, C. (2017). Evolutionary diversification of Pseudomonas aeruginosa in an artificial sputum model. BMC Microbiol. 17:3. doi: 10.1186/s12866-016-0916-z
Dickson, R. P., Erb-Downward, J. R., Freeman, C. M., McCloskey, L., Falkowski, N. R., and Huffnagle, G. B. (2017). Bacterial topography of the healthy human lower respiratory tract. mBio 8, e2287–e2216. doi: 10.1128/mBio.02287-16
Dietrich, L. E. P., Price-Whelan, A., Petersen, A., Whiteley, M., and Newman, D. K. (2006). The phenazine pyocyanin is a terminal signalling factor in the quorum sensing network of Pseudomonas aeruginosa. Mol. Microbiol. 61, 1308–1321. doi: 10.1111/j.1365-2958.2006.05306.x
Douglas, T. A., Brennan, S., Gard, S., Berry, L., Gangell, C., Stick, S. M., et al. (2009). Acquisition and eradication of P. aeruginosa in young children with cystic fibrosis. Eur. Respir. J. 33, 305–311. doi: 10.1183/09031936.00043108
Dubern, J. F., and Diggle, S. P. (2008). Quorum sensing by 2-alkyl-4-quinolones in Pseudomonas aeruginosa and other bacterial species. Mol. Biosyst. 4, 882–888. doi: 10.1039/b803796p
Feltner, J. B., Wolter, D. J., Pope, C. E., Groleau, M. C., Smalley, N. E., Greenberg, E. P., et al. (2016). LasR variant cystic fibrosis isolates reveal an adaptable quorum-sensing hierarchy in Pseudomonas aeruginosa. mBio 7:e1513. doi: 10.1128/mBio.01513-16
GBD 2015 Chronic Respiratory Disease Collaborators (2017). Global, regional, and national deaths, prevalence, disability-adjusted life years, and years lived with disability for chronic obstructive pulmonary disease and asthma, 1990-2015: a systematic analysis for the global burden of disease study 2015. Lancet Respir. Med. 5, 691–706. doi: 10.1016/S2213-2600(17)30293-X
Gibson, J., Sood, A., and Hogan, D. A. (2009). Pseudomonas aeruginosa-Candida albicans interactions: localization and fungal toxicity of a phenazine derivative. Appl. Environ. Microbiol. 75, 504–513. doi: 10.1128/AEM.01037-08
Glasser, N. R., Kern, S. E., and Newman, D. K. (2014). Phenazine redox cycling enhances anaerobic survival in Pseudomonas aeruginosa by facilitating generation of ATP and a proton-motive force. Mol. Microbiol. 92, 399–412. doi: 10.1111/mmi.12566
Grote, J., Krysciak, D., and Streit, W. R. (2015). Phenotypic heterogeneity, a phenomenon that may explain why quorum sensing does not always result in truly homogenous cell behavior. J. Appl. Environ. Microbiol. 81, 5280–5289. doi: 10.1128/AEM.00900-15
Haussler, S., and Becker, T. (2008). The pseudomonas quinolone signal (PQS) balances life and death in Pseudomonas aeruginosa populations. PLoS Pathog. 4:e1000166. doi: 10.1371/journal.ppat.1000166
Heirali, A., McKeon, S., Purighalla, S., Storey, D. G., Rossi, L., Costilhes, G., et al. (2016). Assessment of the microbial constituents of the home environment of individuals with cystic fibrosis (CF) and their association with lower airways infections. PLoS One 11:e0148534. doi: 10.1371/journal.pone.0148534
Hocquet, D., Petitjean, M., Rohmer, L., Valot, B., Kulasekara, H. D., Bedel, E., et al. (2016). Pyomelanin-producing Pseudomonas aeruginosa selected during chronic infections have a large chromosomal deletion which confers resistance to pyocins. Environ. Microbiol. 18, 3482–3493. doi: 10.1111/1462-2920.13336
Hoffman, L. R., Kulasekara, H. D., Emerson, J., Houston, L. S., Burns, J. L., Ramsey, B. W., et al. (2009). Pseudomonas aeruginosa lasR mutants are associated with cystic fibrosis lung disease progression. J. Cyst. Fibros. 8, 66–70. doi: 10.1016/j.jcf.2008.09.006
Jorth, P., Staudinger, B. J., Wu, X., Hisert, K., Hayden, H., Garudathri, J., et al. (2015). Regional isolation drives bacterial diversification within cystic fibrosis lungs. Cell Host Microbe 18, 307–319. doi: 10.1016/j.chom.2015.07.006
Ketelboeter, L. M., and Bardy, S. L. (2017). Characterization of 2-(2-nitro-4-trifluoromethylbenzoyl)-1,3-cyclohexanedione resistance in pyomelanogenic Pseudomonas aeruginosa DKN343. PLoS One 12:e0178084. doi: 10.1371/journal.pone.0178084
Liberati, N., Urbach, J. M., Miyata, S., Lee, D. G., Drenkard, E., Wu, G., et al. (2006). An ordered, nonredundant library of Pseudomonas aeruginosa strain PA14 transposon insertion mutants. Proc. Natl. Acad. Sci. U.S.A. 103, 2833–2838. doi: 10.1073/pnas.0511100103
Manago, A., Becker, K. A., Carpinteiro, A., Wilker, B., Soddemann, M., Seitz, A. P., et al. (2015). Pseudomonas aeruginosa pyocyanin induces neutrophil death via mitochondrial reactive oxygen species and mitochondrial acid sphingomyelinase. Antioxid. Redox. Signal. 22, 1097–1110. doi: 10.1089/ars.2014.5979
Mayer-Hamblett, N., Rosenfeld, M., Gibson, R. L., Ramsey, B. W., Kulasekara, H. D., Retsch-Bogart, G. Z., et al. (2014). Pseudomonas aeruginosa in vitro phenotypes distinguish cystic fibrosis infection stages and outcomes. Am. J. Respir. Crit. Care Med. 190, 289–297. doi: 10.1164/rccm.201404-0681OC
Meader, P., Robinson, G. H., and Leonard, V. (1925). Pyorubrin, a red water-soluble pigment characteristic of B. pyocyaneus. Am. J. Hyg. 5:682. doi: 10.1093/oxfordjournals.aje.a119690
Meirelles, L. A., and Newman, D. K. (2018). Both toxic and beneficial effects of pyocyanin contribute to the lifecycle of Pseudomonas aeruginosa. Mol. Microbiol. 110, 995–1010. doi: 10.1111/mmi.14132
Mott, L. S., Park, J., Murray, C. P., Gangell, C. L., de Klerk, N. H., Robinson, P. J., et al. (2012). Progression of early structural lung disease in young children with cystic fibrosis assessed using CT. Thorax 67, 509–516. doi: 10.1136/thoraxjnl-2011-200912
Mowat, E., Paterson, S., Fothergill, J. L., Wright, E. A., Ledson, M. J., Walshaw, M. J., et al. (2011). Pseudomonas aeruginosa population diversity and turnover in cystic fibrosis chronic infections. Am. J. Respir. Crit. Care Med. 183, 1674–1679. doi: 10.1164/rccm.201009-1430OC
Muhlebach, M. S., Zorn, B. T., Esther, C. R., Hatch, J. E., Murray, C. P., Turkovic, L., et al. (2018). Initial acquisition and succession of the cystic fibrosis lung microbiome is associated with disease progression in infants and preschool children. PLoS Pathog. 14:e1006798. doi: 10.1371/journal.ppat.1006798
O’Brien, S., Williams, D., Fothergill, J. L., Paterson, S., Winstanley, C., and Brockhurst, M. A. (2017). High virulence sub-populations in Pseudomonas aeruginosa long-term cystic fibrosis airway infections. BMC Microbiol. 17:30. doi: 10.1186/s12866-017-0941-6
Ogunnariwo, J., and Hamilton-Miller, J. M. (1975). Brown- and red-pigmented Pseudomonas aeruginosa: differentiation between melanin and pyorubrin. J. Med. Microbiol. 8, 199–203. doi: 10.1099/00222615-8-1-199
Orlandi, V. T., Bolognese, F., Chiodaroli, L., Tolker-Nielsen, T., and Barbieri, P. (2015). Pigments influence the tolerance of Pseudomonas aeruginosa PAO1 to photodynamically induced oxidative stress. Microbiol 161, 2298–2309. doi: 10.1099/mic.0.000193
Palm, K., Sawicki, G., and Rosen, R. (2012). The impact of reflux burden on Pseudomonas positivity in children with cystic fibrosis. Pediatr. Pulmonol. 47, 582–587. doi: 10.1002/ppul.21598
Pauwels, A., Decraene, A., Blondeau, K., Mertens, V., Farre, R., Proesmans, M., et al. (2012). Bile acids in sputum and increased airway inflammation in patients with cystic fibrosis. Chest 141, 1568–1574. doi: 10.1378/chest.11-1573
Pittman, J. E., Calloway, E. H., Kiser, M., Yeatts, J., Davis, S. D., Drumm, M. L., et al. (2011). Age of Pseudomonas aeruginosa acquisition and subsequent severity of cystic fibrosis lung disease. Ped. Pulmonol. 46, 497–504.
Price-Whelan, A., Dietrich, L. E. P., and Newman, D. K. (2007). Pyocyanin alters redox Homeostasis and carbon flux through central metabolic pathways in Pseudomonas aeruginosa PA14. J. Bacteriol. 189, 6372–6381. doi: 10.1128/jb.00505-07
Ramsey, K. A., Ranganathan, S., Park, J., Skoric, B., Adams, A.-M., Simpson, S. J., et al. (2014). Early respiratory infection is associated with reduced spirometry in children with cystic fibrosis. Am. J. Respir. Crit. Care Med. 190, 1111–1116. doi: 10.1164/rccm.201407-1277OC
Reen, F. J., Flynn, S., Woods, D. F., Dunphy, N., Chroinin, M. N., and Mullane, D. (2016). Bile signalling promotes chronic respiratory infections and antibiotic tolerance. Sci. Rep. 6:29768. doi: 10.1038/srep29768
Reen, F. J., Mooij, M. J., Holcombe, L. J., McSweeney, C. M., McGlacken, G. P., Morrissey, J. P., et al. (2011). The Pseudomonas quinolone signal (PQS), and its precursor HHQ, modulate interspecies and interkingdom behaviour. FEMS Microbiol. Ecol. 77, 413–428. doi: 10.1111/j.1574-6941.2011.01121.x
Reen, F. J., Woods, D. F., Mooij, M. J., Adams, C., and O’Gara, F. (2012). Respiratory pathogens adopt a chronic lifestyle in response to bile. PLoS One 7:e45978. doi: 10.1371/journal.pone.0045978
Reen, F. J., Woods, D. F., Mooij, M. J., Chroinin, M. N., Mullane, D., Zhou, L., et al. (2014). Aspirated bile: a major host trigger modulating respiratory pathogen colonisation in cystic fibrosis patients. Eur. J. Clin. Microbiol. Infect. Dis. 33, 1763–1771. doi: 10.1007/s10096-014-2133-8
Rodriguez-Rojas, A., Mena, A., Martin, S., Borrell, N., Oliver, A., and Blazquez, J. (2009). Inactivation of the hmgA gene of Pseudomonas aeruginosa leads to pyomelanin hyperproduction, stress resistance and increased persistence in chronic lung infection. Microbiology 155(Pt 4), 1050–1057. doi: 10.1099/mic.0.024745-0
Smania, A. M., Segura, I., Pezza, R. J., Becerra, C., Albesa, I., and Argaraña, C. E. (2004). Emergence of phenotypic variants upon mismatch repair disruption in Pseudomonas aeruginosa. Microbiology 150, 1327–1338. doi: 10.1099/mic.0.26751-0
Sriramulu, D. D., Lünsdorf, H., Lam, J. S., and Römling, U. (2005). Microcolony formation: a novel biofilm model of Pseudomonas aeruginosa for the cystic fibrosis lung. J. Med. Microbiol. 54, 667–676. doi: 10.1099/jmm.0.45969-0
Stick, S. M., Brennan, S., Murray, C., Douglas, T., von Ungern-Sternberg, B. S., Garratt, L. W., et al. (2009). Bronchiectasis in infants and preschool children diagnosed with cystic fibrosis after newborn screening. J. Pediatr. 155, 623–628. doi: 10.1016/j.jpeds.2009.05.005
Taccetti, G., Campana, S., Festini, F., Mascherini, M., and Doring, G. (2005). Early eradication therapy against Pseudomonas aeruginosa in cystic fibrosis patients. Eur. Respir. J. 26, 458–461.
Toyofuku, M., Morinaga, K., Hashimoto, Y., Uhl, J., Shimamura, H., Inaba, H., et al. (2017). Membrane vesicle-mediated bacterial communication. ISME J. 11, 1504–1509. doi: 10.1038/ismej.2017.13
van der Doef, H. P., Arets, H. G., Froeling, S. P., Westers, P., and Houwen, R. H. (2009). Gastric acid inhibition for fat malabsorption or gastroesophageal reflux disease in cystic fibrosis: longitudinal effect on bacterial colonization and pulmonary function. J. Pediatr. 155, 629–633. doi: 10.1016/j.jpeds.2009.06.040
Van Puyvelde, S., Deborggraeve, S., and Jacobs, J. (2018). Why the antibiotic resistance crisis requires a one health approach. Lancet Infect. 18, 132–134. doi: 10.1016/s1473-3099(17)30704-1
Wang, Y., Gao, L., Rao, X., Wang, J., Yu, H., Jiang, J., et al. (2018). Characterization of lasR-deficient clinical isolates of Pseudomonas aeruginosa. Sci. Rep. 8:13344. doi: 10.1038/s41598-018-30813-y
Wassermann, T., Meinike Jorgensen, K., Ivanyshyn, K., Bjarnsholt, T., Khademi, S. M., Jelsbak, L., et al. (2016). The phenotypic evolution of Pseudomonas aeruginosa populations changes in the presence of subinhibitory concentrations of ciprofloxacin. Microbiology 162, 865–875. doi: 10.1099/mic.0.000273
Williams, D., Evans, B., Haldenby, S., Walshaw, M. J., Brockhurst, M. A., Winstanley, C., et al. (2015). Divergent, coexisting Pseudomonas aeruginosa lineages in chronic cystic fibrosis lung infections. Am. J. Respir. Crit. Care Med. 191, 775–785. doi: 10.1164/rccm.201409-1646OC
Workentine, M. L., Sibley, C. D., Glezerson, B., Purighalla, S., Norgaard-Gron, J. C., Parkins, M. D., et al. (2013). Phenotypic heterogeneity of Pseudomonas aeruginosa populations in a cystic fibrosis patient. PLoS One 8:e60225. doi: 10.1371/journal.pone.0060225
Yang, L., Barken, K. B., Skindersoe, M. E., Christensen, A. B., Givskov, M., and Tolker-Nielsen, T. (2007). Effects of iron on DNA release and biofilm development by Pseudomonas aeruginosa. Microbiology 153(Pt 5), 1318–1328. doi: 10.1099/mic.0.2006/004911-0
Keywords: Pseudomonas aeruginosa, evolution, adaptation, pigmented, chronic, quorum sensing, bile, artificial sputum media
Citation: Flynn S, Reen FJ and O’Gara F (2019) Exposure to Bile Leads to the Emergence of Adaptive Signaling Variants in the Opportunistic Pathogen Pseudomonas aeruginosa. Front. Microbiol. 10:2013. doi: 10.3389/fmicb.2019.02013
Received: 25 February 2019; Accepted: 16 August 2019;
Published: 29 August 2019.
Edited by:
Martin G. Klotz, Washington State University, United StatesReviewed by:
Pierre Cornelis, Vrije University Brussel, BelgiumSheyda Azimi, Georgia Institute of Technology, United States
Copyright © 2019 Flynn, Reen and O’Gara. This is an open-access article distributed under the terms of the Creative Commons Attribution License (CC BY). The use, distribution or reproduction in other forums is permitted, provided the original author(s) and the copyright owner(s) are credited and that the original publication in this journal is cited, in accordance with accepted academic practice. No use, distribution or reproduction is permitted which does not comply with these terms.
*Correspondence: F. Jerry Reen, ai5yZWVuQHVjYy5pZQ==; Fergal O’Gara, Zi5vZ2FyYUB1Y2MuaWU=