- 1Programa de Pós-graduação em Biotecnologia, Núcleo de Pesquisas em Ciências Biológicas, Universidade Federal de Ouro Preto, Ouro Preto, Brazil
- 2Laboratório Especial de Ciclo Celular, Instituto Butantan, São Paulo, Brazil
- 3Departamento de Tecnologia, Universidade Estadual Paulista, UNESP, Campus de Jaboticabal, Jaboticabal, Brazil
- 4Departamento de Fitopatologia e Nematologia, Escola Superior de Agricultura Luiz de Queiroz, Universidade de São Paulo, Piracicaba, Brazil
- 5Departamento de Bioquímica, Instituto de Química, Universidade de São Paulo, São Paulo, Brazil
- 6Fundo de Defesa da Citricultura (FUNDECITRUS), São Paulo, Brazil
- 7Faculdade de Computação, Universidade Federal de Mato Grosso do Sul, Campo Grande, Brazil
- 8Departamento de Ciências Biológicas, Instituto de Ciências Exatas e Biológicas, Universidade Federal de Ouro Preto, Ouro Preto, Brazil
Xanthomonas citri pv. aurantifolii pathotype B (XauB) and pathotype C (XauC) are the causative agents respectively of citrus canker B and C, diseases of citrus plants related to the better-known citrus canker A, caused by Xanthomonas citri pv. citri. The study of the genomes of strains of these related bacterial species has the potential to bring new understanding to the molecular basis of citrus canker as well as their evolutionary history. Up to now only one genome sequence of XauB and only one genome sequence of XauC have been available, both in draft status. Here we present two new genome sequences of XauB (both complete) and five new genome sequences of XauC (two complete). A phylogenomic analysis of these seven genome sequences along with 24 other related Xanthomonas genomes showed that there are two distinct and well-supported major clades, the XauB and XauC clade and the Xanthomonas citri pv. citri clade. An analysis of 62 Type III Secretion System effector genes showed that there are 42 effectors with variable presence/absence or pseudogene status among the 31 genomes analyzed. A comparative analysis of secretion-system and surface-structure genes showed that the XauB and XauC genomes lack several key genes in pathogenicity-related subsystems. These subsystems, the Types I and IV Secretion Systems, and the Type IV pilus, therefore emerge as important ones in helping explain the aggressiveness of the A type of citrus canker and the apparent dominance in the field of the corresponding strain over the B and C strains.
Introduction
Citrus is an important worldwide crop (FAS, 2019) that has been threatened by various diseases over many decades. Even though citrus Huanglongbin (greening) is today the major citrus threat (Wang et al., 2017), citrus canker (Goto, 1992; Schubert and Miller, 1996; Schubert et al., 2001) still is an important disease (CAB-International, 2019), especially in Brazil (Mendonça et al., 2017).
Three species of bacteria of the genus Xanthomonas are associated with citrus canker diseases in citrus: Xanthomonas citri subsp. citri (Xcc) pathotypes A, A∗ and Aw, X. citri subsp. aurantifolii pathotypes B and C (XauB and XauC), and X. alfalfae subsp. citrumelonis (Xacm). Xcc, XauB and XauC are respectively the causative agents of citrus canker A, B, and C, which cause small necrotic raised lesions surrounded by a water-soaked margin (Civerolo, 1984). Citrus canker A, the most aggressive, remains a concern in all citrus growing regions in Asia and South America (CAB-International, 2019). XauB strains are less aggressive, and XauC strains have a more restricted host range, when compared with symptoms and host range of Xcc, respectively. Canker B is currently known to be present only in Argentina, Paraguay, and Uruguay (Civerolo, 1984); moreover, XauB may have been eradicated even from this restricted region by competition from Xcc (Chiesa et al., 2013). Canker C is limited to the state of São Paulo, Brazil (Malavolta Júnior et al., 1984); the most recent field report dates to 2009 (Jaciani et al., 2009). Xacm is the causal agent of citrus bacterial spot, which induces symptoms very similar to those of canker, but the lesions are flat and not raised.
Sequencing of the X. citri susbsp. citri strain A306 genome (A306) allowed the characterization of important properties of this more aggressive pathotype (da Silva et al., 2002). Following that work, genomes of the other pathotypes were sequenced and compared with each other (Jalan et al., 2013; Bodnar et al., 2017).
Given the phylogenetic relatedness of the causal pathogens of cankers A, B, and C, the comparative study of XauB and XauC strains at the genomic level offers the opportunity of achieving a better understanding of citrus canker disease in general. Up to now, only one XauB and only one XauC strain genome have been sequenced (Moreira et al., 2010). We therefore decided to sequence the genomes of additional strains of XauB and XauC. The newly sequenced isolates were selected because they showed significant differences in pathogenicity and aggressiveness when inoculated in different citrus genotypes and/or had different genetic characteristics (Jaciani, 2012; Table 1). The isolates XauB 1561 and XauB 1566 showed less virulence with respect to the other isolates and absence of clear infection symptoms, suggesting a probable loss of pathogenicity, besides being genetically different by AFLP and ERIC-PCR (Jaciani, 2012). The selection of XauC strains was based on the ability of some isolates to produce dark pigment when cultivated in NB or NA culture media (NB: 0.5% peptone, 0.3% beef extract; NA: 0.5% peptone, 0.3% beef extract, 1.5% agar), also observed in X. citri pv. fuscans and X. campestris pv. vignicola (Schaad et al., 2005; Schaad et al., 2006). XauC 535 and XauC 1609 cause lesions only in Mexican lime [C. aurantifolia (Christm.) Swingle] and Swingle citrumelo [C. paradisi Macfad. × Poncirus trifoliata (L.) Raf.], and in both hosts with mild symptoms. XauC 1609 was found to infect Swingle citrumelo under natural conditions (Jaciani et al., 2009), despite the fact that prior work suggested that only Mexican lime was susceptible to XauC (Malavolta Júnior et al., 1984). Additionally, XauC 535 and XauC 1609 were also differentiated by AFLP and BOX-PCR (Jaciani, 2012). The isolates XauC 763, XauC 867, and XauC 1559, which do not produce pigment, were distinguished in terms of pathogenicity and aggressiveness. XauC 763 and XauC 1559, which are also Mexican lime pathogens, caused injuries in Swingle citrumelo and Cravo mandarin (C. reticulata Blanco), and when inoculated in high concentration they infected Rangpur lime (C. limonia Osbeck), Persian lime [C. latifolia (Yu. Tanaka) Tanaka], lemon [Citrus limon (L.) Burm. f.], Grapefruit (C. paradisi Macfad.), and Cleopatra mandarin (C. reshni hort. ex Tanaka) (Jaciani, 2012). Finally, XauC 1559 was slightly more aggressive than XauC 763 when inoculated in Cravo mandarin, and XauC 867 presented a slightly more restricted pathogenicity and lower aggressiveness in Mexican lime compared to XauC 763 and XauC 1559 (Jaciani, 2012).
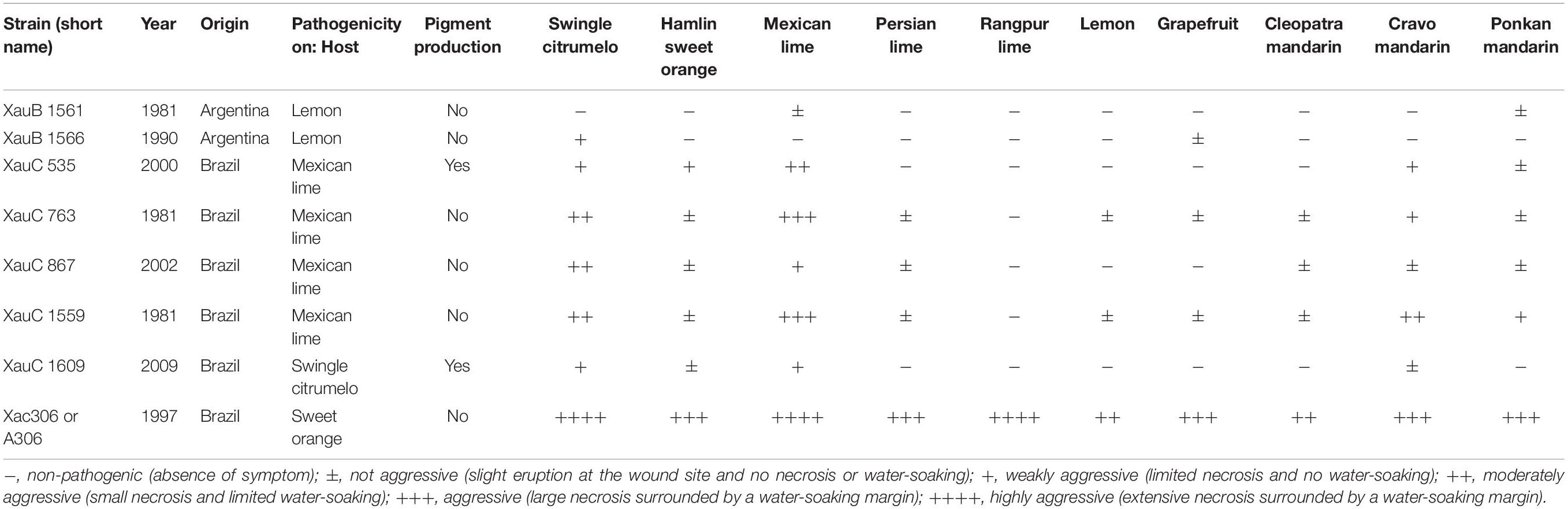
Table 1. Phenotype characteristics of eight Xanthomonas citri strains (Jaciani, 2012).
Altogether, based on the information above, we have sequenced two new XauB and five new XauC genomes, with the aim of achieving a better understanding of the genomic basis of citrus canker and the evolutionary history of these strains. Together with 24 other public and closely related genomes, this allowed us to carry out a phylogenomic analysis as well as an investigation of selected gene families relevant in bacteria-plant interactions in general and in citrus canker in particular (Ryan et al., 2011), which we present here.
A note on taxonomic nomenclature: Xanthomonas species that are pathogenic to citrus were described in this study using names as proposed by Schaad et al. (2006), since this classification is adopted for all cited references found until the present. The other Xanthomonas species were described as proposed by Bui Thi Ngoc et al. (2010) and Constantin et al. (2016).
Materials and Methods
Media and Culture Conditions
The new genomes presented here were sequenced from strains stored both in autoclaved tap water at room temperature and at −80°C in NB medium (3 g/L meat extract, 5 g/L peptone) containing 25% glycerol. Each one of the strains was recovered from a −80°C stock, streaked on solid NA medium (3 g/L meat extract, 5 g/L peptone and 15g/L agar) and cultivated for 48 h at 29°C. For each strain, colonies were inoculated into 10 mL of liquid NB medium in a sterile 50 mL Falcon conical centrifuge tube and incubated at 29°C in a rotary shaker at 180 rpm for 16 h (final OD600 nm ∼1.0).
DNA Extraction and Quantification
A volume of 2 mL of the culture was centrifuged at 16,000 g for 10 min at 4°C in a refrigerated benchtop microcentrifuge. The supernatant was discarded and the cell pellet was resuspended in 600 μL of Nuclei Lysis Solution supplied by Promega Wizard Genomic DNA purification kit (Promega Corporation, Madison, United States). Total DNA extraction was performed using Promega Wizard Genomic DNA purification kit according to manufacturer instructions. DNA quantity and quality were determined using NanoDrop ND-1000 spectrophotometer (NanoDrop Tech, Wilmington, DE, United States), Qubit 2.0 fluorometer (Invitrogen, Life Technologies, CA, United States) and 0.8% agarose gel electrophoresis. Each extraction yielded at least 5 μg of high-quality genomic DNA.
Genome Sequencing and Assembly
The genomes of XauC 535, XauC 763, and XauC 867 strains were sequenced using the Illumina HiScanSQ platform at NGS Soluções Genômicas (Piracicaba, Brazil). An average of ∼20 M reads (2 × 100 bp) for each genome was generated. The raw reads were trimmed with seqyclean software1, using minimum phred value of 23, minimum read length of 30 bp, and removing custom Illumina TruSeq adapters. Genome assembly was carried out with SPAdes v3.8.1 (Bankevich et al., 2012) with default parameters. Contig sequences were assigned to plasmids using plasmidSPAdes v3.8.1 (Antipov et al., 2016).
The genomes of XauB1561, XauB1566, XauC1559, XauC1609 strains were sequenced using PacBio single molecule real-time (SMRT) technology at the Duke Center for Genomic and Computational Biology (United States). One SMRT library was sequenced for each sample using P6-C4 chemistry, generating reads with average length of 10–15 Kb, thus yielding ∼150X coverage of each genome. De novo assembly was conducted using SMRT® Analysis Server v2.3.02. Raw PacBio reads were mapped against the resulting contigs using the blasR aligner, and SNP corrections were conducted with variant-caller software using the quiver algorithm (both part of the Analysis Server).
The rationale for having some genomes sequenced using PacBio technology and some using Illumina technology was as follows. We wanted to ensure that we could provide complete genomes for both XauB and XauC, given that prior to this work only draft genomes were available for these pathotypes (Moreira et al., 2010). On the other hand our budget was limited, and we could afford PacBio sequencing for only four genomes. Under these constraints, the choice of which genomes to sequence by PacBio was arbitrary.
All assembled genomes were verified with CheckM (Parks et al., 2015), resulting in 100% completeness and 0% contamination for all of them.
Genome Selection
For the purposes of phylogenomic analyses, we searched for genomes in NCBI/GenBank using “Xanthomonas citri” as a keyword, then looked at the automatic dendrogram generated by genomic distances on the NCBI website3, which reveals all genomes within this group, including all subspecies/lineages/varieties available as reference sequences (RefSeq). After downloading this tree, we searched for all different lineages, and then downloaded up to three genomes from each such lineage, if available, and preferentially (if possible) drawing from separate clades where the lineage appears in NCBI’s dendrogram, to avoid pseudoreplication (i.e., avoiding picking two closely related genomes). This led to a final dataset of 31 genomes.
Phylogenomic Reconstruction
In order to generate comparable sets of gene families, Prokka (Seemann, 2014) (with default parameters) was employed for annotation of each genome. Get_Homologues (Contreras-Moreira and Vinuesa, 2013) was used for multiple local BLAST-directed comparisons among all genes (of all genomes), and these were further clustered by the OMCL method which drives the OrthoMCL algorithm (Li et al., 2003) within Get_Homologues. Subsequently compare_clusters.pl (a script within the same software) was used for retrieval of the set of orthologous genes uniquely present in all genomes (hereafter denominated the unicopy set). Mafft (Katoh and Standley, 2013) was used for multiple alignment of each unicopy gene, and then concatenation of all genes was done using FASconCAT (Kück and Meusemann, 2010). IQTree (Nguyen et al., 2015) was used for maximum likelihood (ML) estimation, with model choice employed before tree search, and branch support computed by UFBoot (Hoang et al., 2017).
Effector Analysis
The aminoacid sequences of 62 effectors were retrieved from the Xanthomonas.org site (AvrBs2, AvrXccA1, AvrXccA2, HpaA, HrpW, XopA, XopAA, XopAB, XopAC, XopAD, XopAE, XopAF1, XopAF2, XopAG, XopAH, XopAI, XopAJ, XopAK, XopAL1, XopAL2, XopAM, XopAP, XopAU, XopAV, XopAW, XopAX, XopAY, XopAZ, XopB, XopC1, XopC2, XopD, XopE1, XopE2, XopE3, XopF1, XopF2, XopG1, XopG2, XopH1, XopI1, XopJ1, XopJ2, XopJ3, XopJ4, XopJ5, XopK, XopL, XopM, XopN, XopO, XopP, XopQ, XopR, XopS, XopT, XopU, XopV, XopW, XopX, XopY, and XopZ1) (Supplementary Table S1), to infer their evolution across the 31 genomes. For each genome, we assessed whether each effector without a premature stop codon had a frameshift or not. In order to do so, we performed local tBLASTn searches within the Blast + suite (Camacho et al., 2009) with an e-value of 1e-50 (a threshold obtained by trial-and-error, that minimized the number of extra hits bearing indels and mismatches without compromising detection of supposedly functional copies), discarding alignments in which the subject sequences aligned to less than 60% of the query length or with less than 60% identity. After tBLASTn runs, multiple alignments were generated by an in-house python script for each effector, each of which was manually checked in Aliview (Larsson, 2014) for detection of frameshifts and premature stop codons. Optimization of character evolution for each effector along the ML tree (i.e., presence, frameshifts without premature stop codons, sequences with premature stop codons, and absence) was obtained by the ace function within the R library phytools (Revell, 2012).
Additional Gene Analyses
Additional gene families were investigated based on OrthoMCL clustering (Li et al., 2003) and STRING (Snel et al., 2000). OrthoMCL was run with default parameters, and results were then processed in the OrthologSorter pipeline (Setubal et al., 2018). Additionally, we created an Ortholog Alignment using gene families provided by OrthoMCL, with the A306 strain as anchor and all the XauB and XauC genomes, plus X. citri pv. fuscans 4384. This alignment is useful to visualize syntenic regions among genomes. The parts of this alignment that were used in reporting results in this work are shown in a simplified version in Supplementary Table S2. In the case of STRING, for each family of interest, the relevant genes as present in A306 were used as queries.
Gum Production Assay
The xanthan gum production assays were performed as described by Moreira et al. (2010), without modification.
Biofilm Production Assay
Biofilm production assays were performed following O’Toole (O’Toole, 2011), with a few modifications. The bacterial isolates were grown in liquid LB or XVM2 medium at 28°C. Bacterial density was standardized for all the isolates in OD600nm equal to 1.0. The samples were diluted 1:10 in liquid LB and 100 μL of each sample were placed in the 96-well plate for growth during 96 h at 28°C. After the incubation period, the plate was washed with distilled water to remove the cells and left drying for 2 h. Subsequently, 125 μL of crystal violet solution 0.1% (CV) were transferred to each well, which were left resting for 45 min. After the incubation period the plate was washed again with distilled water and left drying once more. Next, 125 μL of 95% ethanol were added to each well, which were left to rest for 45 min to complete CV dissolution. The absorbance reading was done at OD550nm. For each bacterial isolate 6 replicates were performed.
Autoaggregation Assay
The autoaggregation assay was adapted from Alamuri et al. (2010), with modifications. Cultures of different bacterial isolates were grown at 28°C in liquid LB medium or XVM2: 1.16 g/L NaCl, 1.32 g/L (NH4)2SO4, 0.021 g/L KH2PO4, 0.055 g/L K2HPO4, 0.0027 g/L FeSO4⋅7H2O, 1.8 g/L fructose, 3.423 g/L sucrose, 5 mMMgSO4, 1 mM CaCl2, 0.03% Casamino acid (pH 6.7), in triplicate. Samples with 10 mL of each culture were placed in a sterile 20 mL tube. Initially all cultures were vigorously shaken for 15 s and the tubes remained static throughout the experiment. Aliquots containing 100 μL were removed from approximately 1 cm of the top of the culture of each tube over time and optical density was measured at OD600 nm every hour.
Results
Information about the genomes that were sequenced for this work is given in Table 2. The additional genomes listed there were included in the analysis of pathogenicity-related genes.
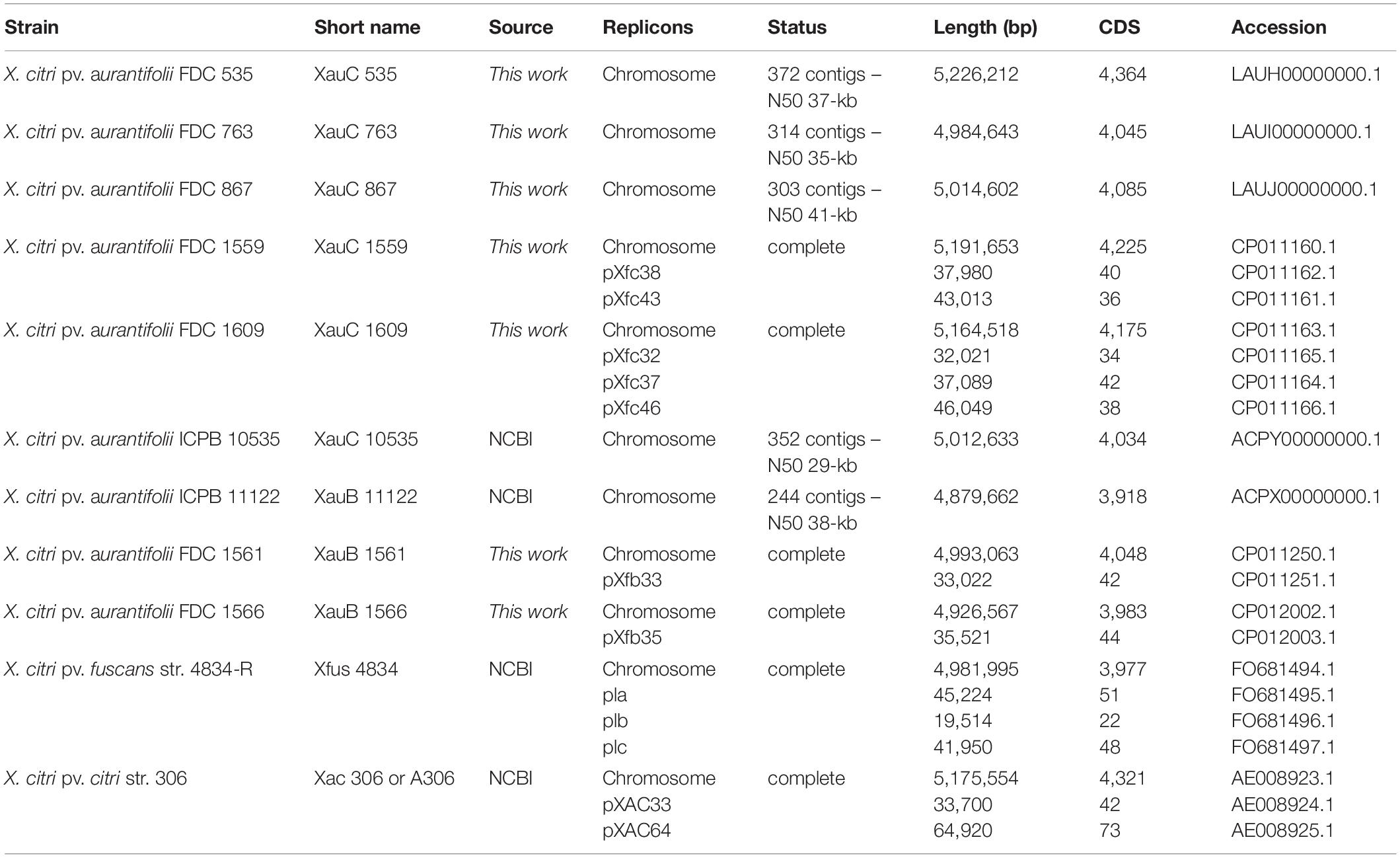
Table 2. List of genomes used in the comparative analysis section, including information about the seven newly sequenced XauB and XauC genomes.
Phylogenomic Analyses
For the phylogenomic analyses we used 31 genomes (Table 3). Gene family computation resulted in 2,449 single-copy shared families, leading to a concatenated alignment of 2,516,841 bp. The best ML model was GTR + G + R2 (where R2 means a mixed model of rate variation with two rate classes), with most nodes with support ≥ 95%. The resulting phylogeny is shown in Figure 1.
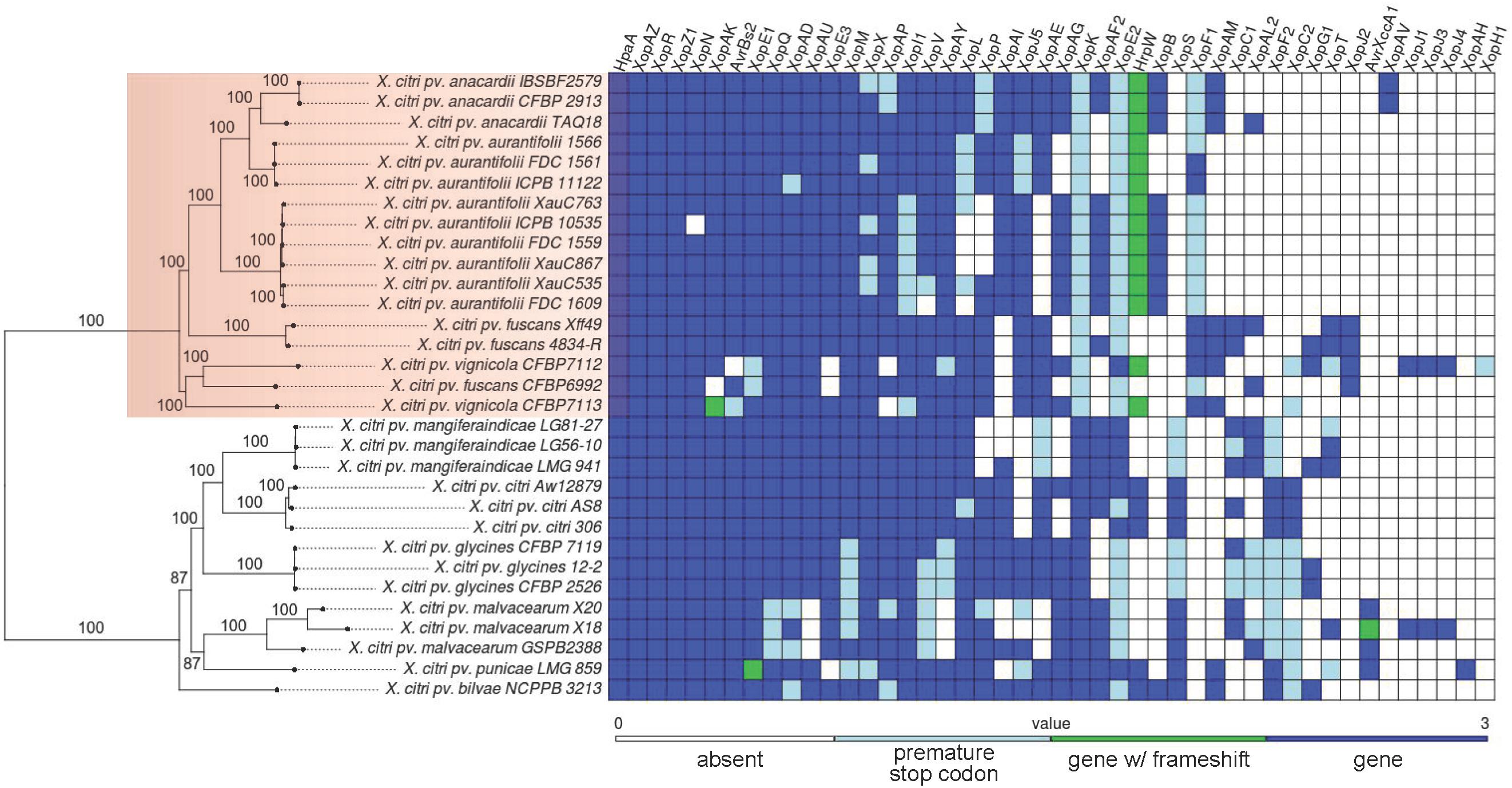
Figure 1. Maximum likelihood phylogeny of 31 Xanthomonas citri strains and table of presence, absence, and pseudogene status of 46 effector genes.
Xanthan Gum, Biofilm, and Autoaggregation Analysis
In an attempt to understand which physiological factors could contribute to the induction of the respective virulence phenotypes of the investigated strains, xanthan gum production, biofilm, and cell self-aggregation were analyzed (Supplementary Table S3). As expected, A306 is the strain with the highest production of xanthan gum by bacterial mass. On the other hand, XauC 535 and XauC 1609 showed respectively the highest biofilm production and self-aggregation capacity in virulence-inducing medium (XVM2).
Type III Secretion System Effector Analysis
Out of the 62 effectors investigated, four were present in all genomes, 16 were absent from all genomes, for a total of 42 effectors with variable presence/absence across lineages (Figure 1). For 11 effectors we observed interesting patterns of presence, absence, or pseudogenization. For these effectors we inferred their evolutionary history in terms of gains, losses or pseudogenization (Supplementary Figure S1).
Other Pathogenicity-Related Genes
Individual genes or genes that collectively encode proteins that compose cell complexes involved in virulence and adaptation were analyzed in all genomes listed in Table 2. The virulence and adaptation genes were grouped into two broad categories: (1) secretion systems (other than Type III effectors); (2) surface structure. The analysis framework we have adopted is as follows. The A306 strain has several genes in each of the categories analyzed (da Silva et al., 2002). On the other hand, as will be seen, the XauB and XauC genomes that we have analyzed lack many or all of the genes in some of these categories. In order to better understand the potential impact that the lack of these genes may have in the pathogenicity and/or survival capabilities of the XauB and XauC strains, for each category in which XauB and XauC lack genes we first describe the A306 gene content. We then note the differences exhibited by XauB and XauC (as given by the Ortholog Alignment of 11 genomes, with A306 as anchor, as described in section Materials and Methods), followed by a network analysis based on the A306 genes, using the tool STRING (Snel et al., 2000).
Secretion Systems
We verified that all the analyzed genomes retain all orthologous genes belonging to the two gene clusters associated with synthesis of the type II secretion system (T2SS, XAC0694-XAC0705, and XAC3534-3544), all the genes involved in structuring the type III secretion system apparatus (T3SS, XAC0393-XAC0417), all the genes associated with the type VI secretion system (T6SS, XAC4119-20-24, XAC4139-40-45), as well as complete Sec and Tat secretion systems. The main differences observed are related with the type I secretion system (T1SS), the type IV secretion system (T4SS) and effectors of the type III secretory system (T3SS). Results for T3SS effectors were already presented above.
XauB and XauC Lack Key Genes in the Type I Secretion System
The T1SS corresponds to an ABC transporter system and it is basically composed of two proteins, HlyD – an ABC transporter, and HlyB – a membrane fusion protein, whose main function, together with TolC, is to promote the secretion of toxins (Koronakis et al., 2004). In A306 two copies of the gene encoding the toxin presumably secreted by this system, hemolysin (type-calcium, XAC2197-98), are upstream of the genes hlyDB (XAC2201-02), separated by two hypothetical proteins (XAC2199-2200). These gene families (XAC2197-2202) were not found in the XauB genomes. The XauC genomes on the other hand do not have orthologs of XAC2197-98, but they do have hlyB and hlyD. Other genes associated with synthesis and regulation of hemolysin in these genomes were also analyzed. All genomes have orthologs of XAC4303 and XAC1668 (cryptic hemolysin transcriptional regulator), XAC3043 and XAC0079 (hemolysin III, hly3), and XAC1709 (hemolysin, tlyC). However, in XauB and XauC strains we did not find orthologs for the genes XAC1814 (outer membrane hemolysin activator protein) and XAC1918 (hemolysin-like protein).
Analysis of possible interactions of the products of genes hlyB and hlyD (Figure 2) revealed two well-defined interaction networks for the A306 hlyB gene used as query to STRING. One of these groups, in orange background, is the genes/proteins associated with T1SS composition and functionality. The other network (green background) is composed primarily of membrane genes/proteins, essentially ABC transporters. Eight genes/proteins represented by the nodes of the network composing the T1SS apparatus correspond to the same genes in the cluster discussed above, including the gene encoding the lytic enzyme (XAC0466) present in the XauC10535 genome. We observed that the genomes of XauB strains do not have any of these genes. However, the loss of a single gene of hemolysin in XauC strains would have a small effect, since this loss could be compensated by paralogous genes in their genomes. Concerning the cluster of membrane proteins, three of the ABC transporters are associated with resistance to acriflavin (XAC3994-95 and XAC3850) and two have the hlyD domain (PF00529), involved with secretion of toxins. Some of the genes in this network were not found in the genomes of strains XauC 1609 and XauC 535.
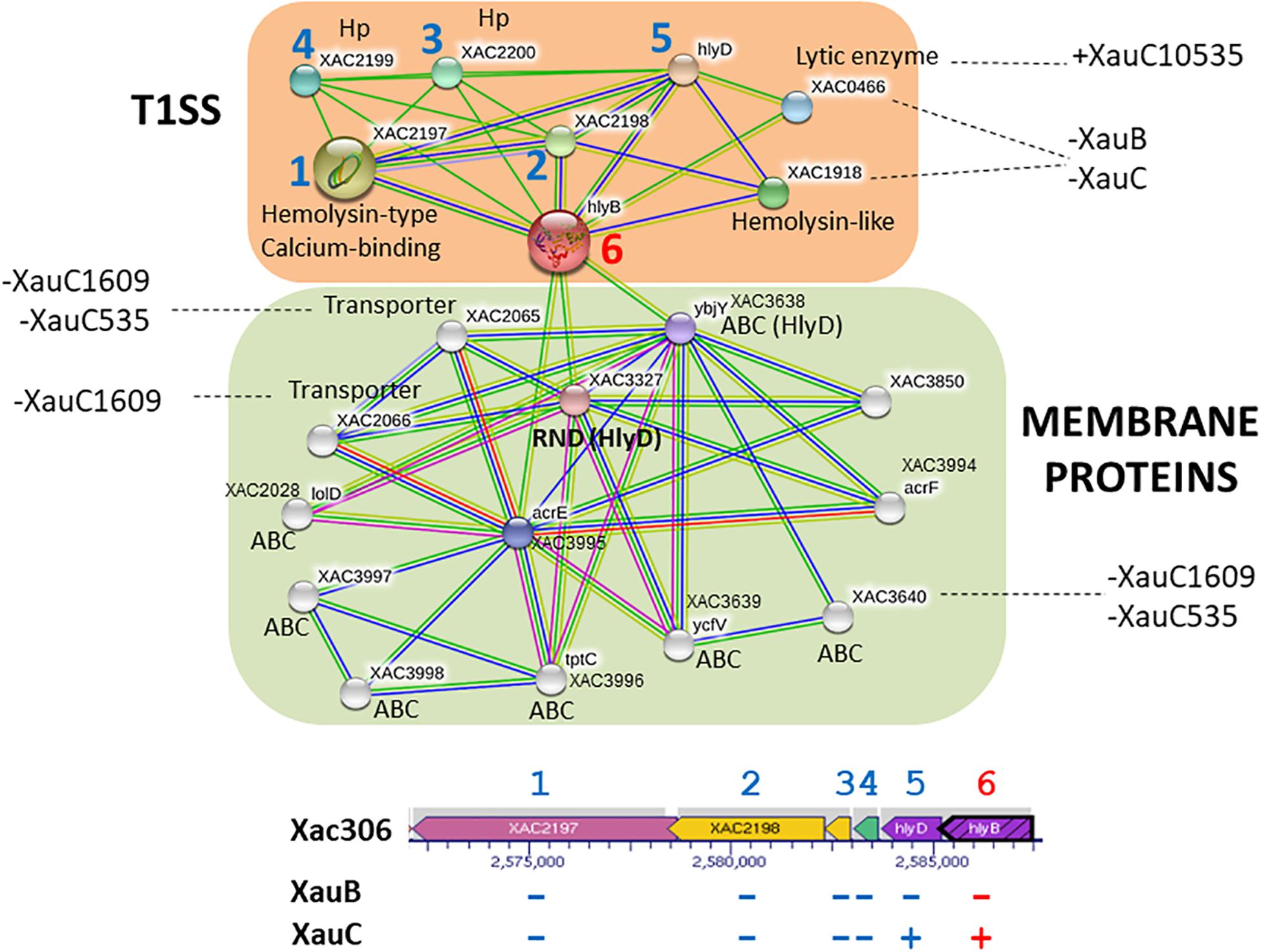
Figure 2. Network of putative interactions between genes/proteins using as query the hlyB gene from strain A306, a key gene in the Type 1 Secretion System. The nodes represent the genes/proteins present in the A306 genome. The color codes on the lines is: red – fusion evidence; green – neighborhood evidence; blue – co-occurrence evidence; purple – experimental evidence; yellow – text mining evidence; light blue – database evidence; black – co-expression evidence. The network part of the figure was generated by the program STRING (Snel et al., 2000). Genomic context is shown at the bottom, based on a figure generated by the BioCyc resource (Karp et al., 2017). The arrows represent the genes and the gray background the transcriptional units. The blue numbers correlate the position of a given gene in the genomic context and the same gene on the network. The number in red has a similar purpose and simply highlights the query gene in the network inference.
XauB and XauC Genomes Lack the Chromosomal Copy of Type IV Secretion System Genes
The genes encoding the T4SS in A306 are found in two similar gene clusters, one in the chromosome (XAC2607-2623) and another in the plasmid (XACb0036-b0047) (da Silva et al., 2002). The genomes of XauB and XauC have only the plasmidial cluster (Supplementary Table S2). Note that in the cases of XauB 11122 and XauC 10535, whose contigs are not distinguished as belonging to the chromosome or to a plasmid, it is our inference based on synteny that the T4SS genes actually belong to a plasmid (Supplementary Table S2).
XauB and XauC Genomes Lack Key Genes in the Synthesis and Regulation of Type IV Pilus
A306 has at least four clusters of genes involved with synthesis and regulation of type IV pilus (T4p). One of these clusters, pilE-Y1-X-W-V-fimT (XAC2664-2669) is found between a set of prophage genes upstream and a transposase downstream, suggesting possible horizontal gene transfer. We observed that two of these genes (pilX-pilV) are missing in the XauB and XauC genomes (Supplementary Table S2). In the case of cluster pilS-R-B-A-A-C-D (XAC3237-3243) (Yang et al., 2004), the XauB and XauC genomes lack the two copies of pilA. PilA encodes pilin, an essential T4p component that contributes to twitching motility and biofilm development in A306 (Dunger et al., 2014; Petrocelli et al., 2016). Another gene whose product has a function related to T4p is pilL (XAC2253). In A306 this gene is found in a large genomic island (XAC2176 to XAC2286), but is absent from the XauB and XauC genomes.
We carried out an analysis of predicted interactions of pilA (XAC3240) (Figure 3). In orange background we observe that XAC3240 interconnects five other networks and that the pilin subunits (XAC3240 and XAC3241) are connected to one another, and connect to another pilA (XAC3805). As expected, one of the networks starting from pilins refers to genes/proteins associated with the pilus structure and with the T2SS (cyan background), as is known that both are evolutionarily related (Peabody et al., 2003). Moreover, three genes share the same genomic region of pilins in the chromosome of A306 (1, 4, and 5). Close to the pilin network (purple background) there is a network involving genes associated with quorum sensing (rpf), gum synthesis (gum) and the plant tissue degrading enzyme polygalacturonase (pglA), known to be virulence-related (Wang et al., 2008). Likewise, this network reflects the interaction profiles of DSF production mediated by rpf genes, which act as signaling molecules of gum synthesis and consequently of the production of plant cell wall degrading enzymes, as is the case for PglA mediated by T2SS (Vojnov et al., 2001; An et al., 2013). In addition, another network expands from rpfC. Indeed, this network (pale green background), associated with chemotaxis-related genes, includes phoB, which is involved in phosphate regulons, essential for adaptation and virulence induction in members of the genus Xanthomonas (Pegos et al., 2014; Moreira et al., 2015).
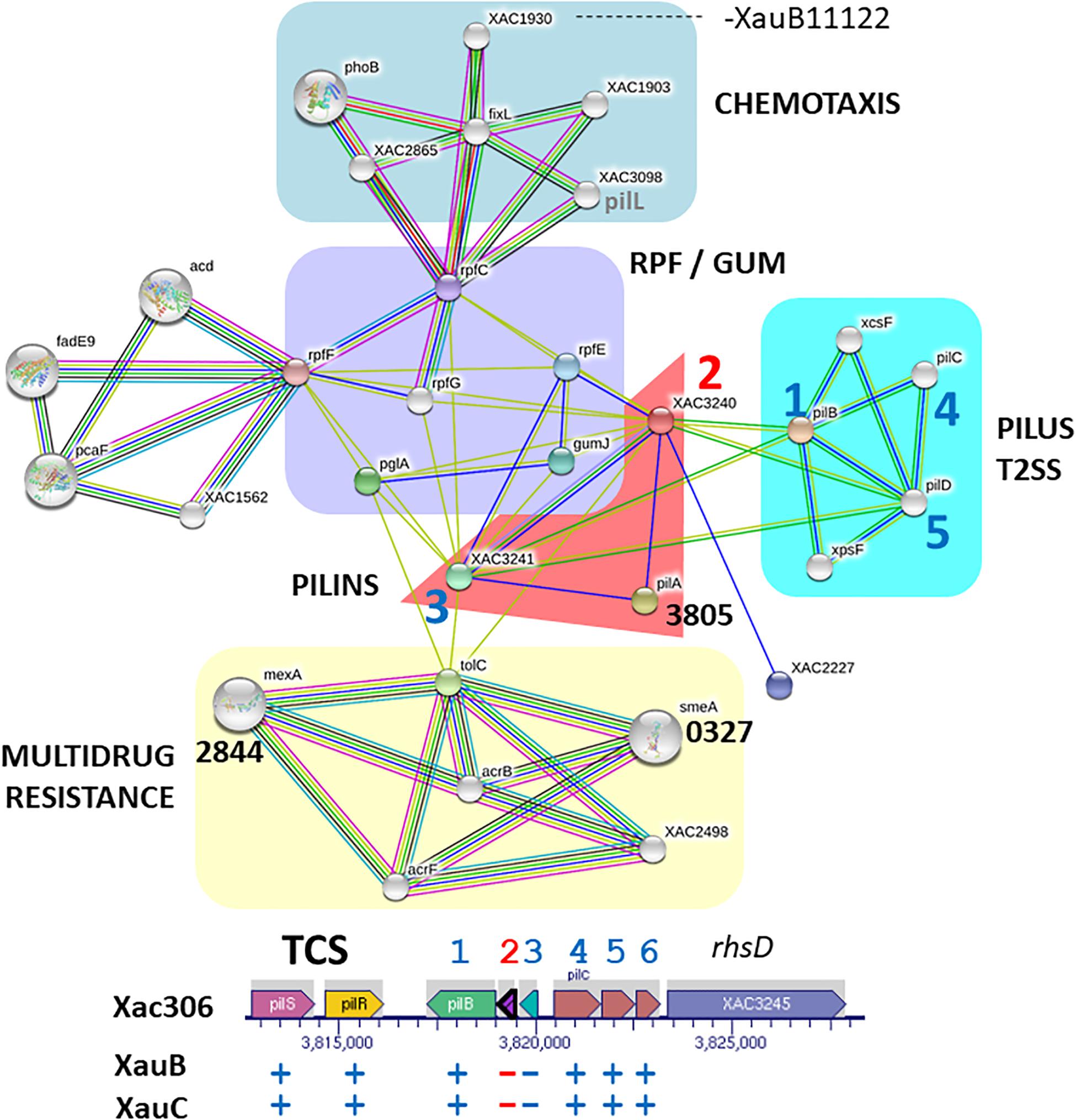
Figure 3. Network of putative interactions between genes/proteins using as query the pilin gene XAC3240 from the A306 strain. For additional details see legend of Figure 2. The network figure was generated by the program STRING (Snel et al., 2000) and the genomic context figure was based on a figure generated by the BioCyc resource (Karp et al., 2017).
XauB and XauC Genomes Lack an Alginate Biosynthesis Gene
In A306, the first gene downstream of pilE-Y1-X-W-V-FimT, XAC2670, encodes an alginate biosynthesis protein, which is absent from the XauB and XauC genomes (Supplementary Table S2). The A306, XauB, and XauC genomes encode another gene whose product is involved with the metabolism of alginate, alginate lyase: algL (XAC4349).
Analysis of predicted interactions of the gene XAC2670 with other genes/proteins revealed two distinct clusters (Figure 4). The first one on blue background contains seven nodes whose genes/proteins are directly related to synthesis and regulation of T4p (pil genes previously described). In this group, excepting pilO (XAC3383), all other genes are present in a cluster (XAC2664-XAC2669) downstream of a transposase and a phage insertion (numbers 1–6), and upstream of the gene XAC2670. The second cluster, on yellow background, contains 12 nodes, with most genes/proteins related to regulatory functions, especially algZR (XAC0620-21), encoding a two-component system, respectively for sensor and regulatory proteins (Okkotsu et al., 2014), and algC (phosphomannomutase) (Davies and Geesey, 1995), all described as essential to alginate synthesis. Another two-component system, lytST (XAC2142-2141, sensor-regulator), is also present in this network. LytT, as well as rpfD, also present in the yellow background network (XAC1874) and member of the rpf gene cluster, exhibit the lytR domain, also present in proteins such as AlgR with DNA binding function (Nikolskaya and Galperin, 2002). Finally, rpoE (XAC1319) connects the two clusters (Figure 4), and therefore may be directly associated with both by regulating the EPS synthesis and/or by modulating T4p expression.
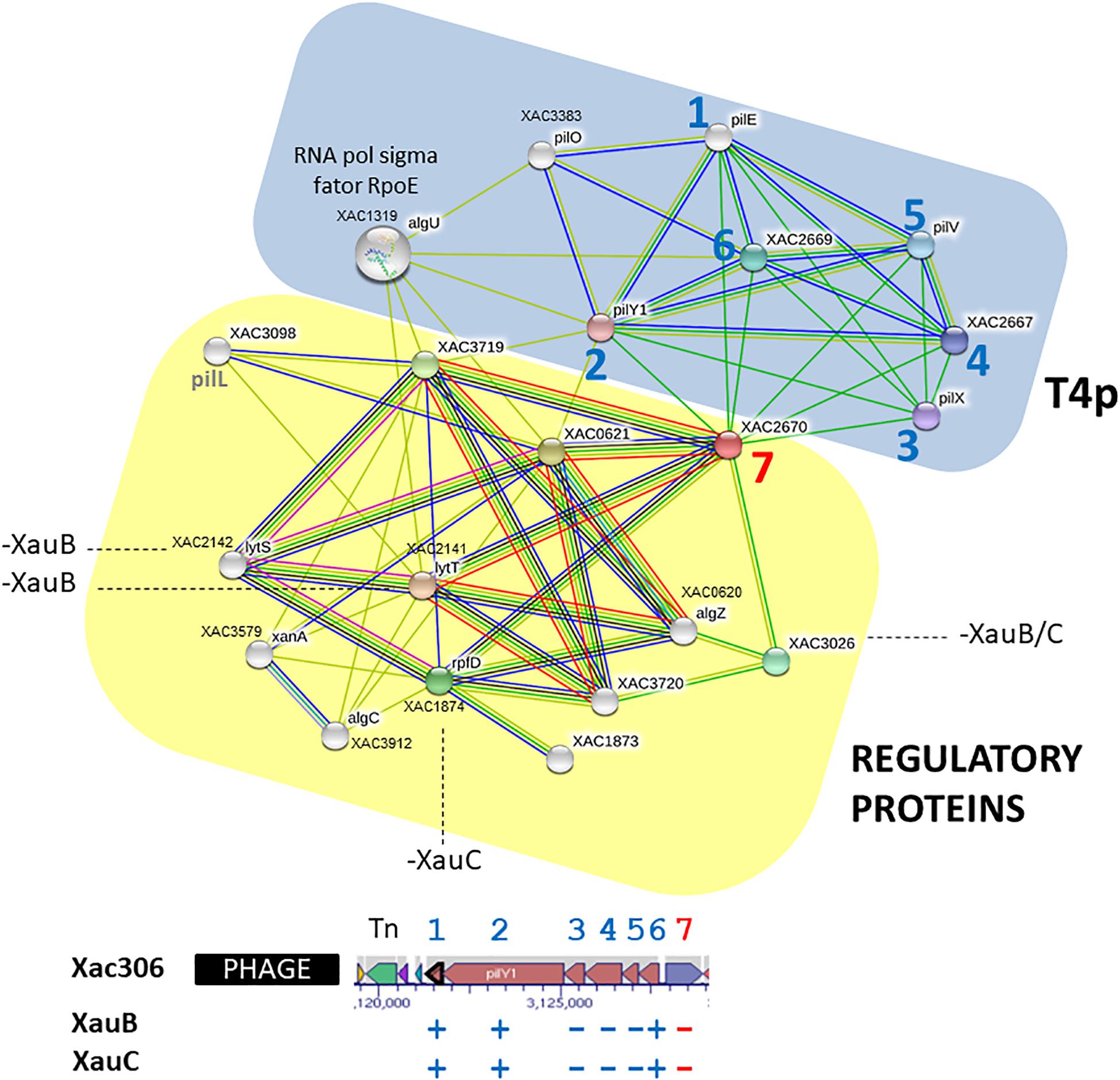
Figure 4. Network of putative interactions between genes/proteins using as query the A306 gene XAC2670, which encodes an alginate biosynthesis protein. For additional details see legend of Figure 2. The network figure was generated by the program STRING (Snel et al., 2000) and the genomic context figure was based on a figure generated by the BioCyc resource (Karp et al., 2017).
XauB and XauC Lack Several Genes Related to Hemagglutinin and Hemolysin Synthesis
A further set of genes with significant differences in terms of presence and absence in the analyzed genomes is related to hemagglutinin and hemolysin synthesis. These genes are located in two regions in the genome of A306 (XAC4112-XAC4125 and XAC1810-XAC1819). The first region is flanked by genes that are part of the T6SS, both downstream and upstream. All genes of this region are present in all genomes of XauB and XauC. However, the genes in the second region (XAC1810-XAC1819) are totally absent in the XauB and XauC genomes. Among these genes we highlight fhaC (XAC1814), which codes for an outer membrane hemolysin activator, fhaB (XAC1815), which codes for a filamentous hemagglutinin, XAC1816, which codes for a hemagglutinin/hemolysin-related protein, XAC1818, which codes for hemagglutinin, and the genes in the operon HmsHFR-hp (XAC1813-1810).
Analysis of predicted interactions of the gene fhaB (XAC1815) allowed the characterization of two major interaction networks (Figure 5). One of these networks (pink background) is associated with adhesion, whereas the other network (gray background) basically contains hypothetical genes/proteins. Furthermore, other genes/proteins in the adhesion network are located in the two regions related to hemagglutinin and hemolysin synthesis mentioned in the previous paragraph.
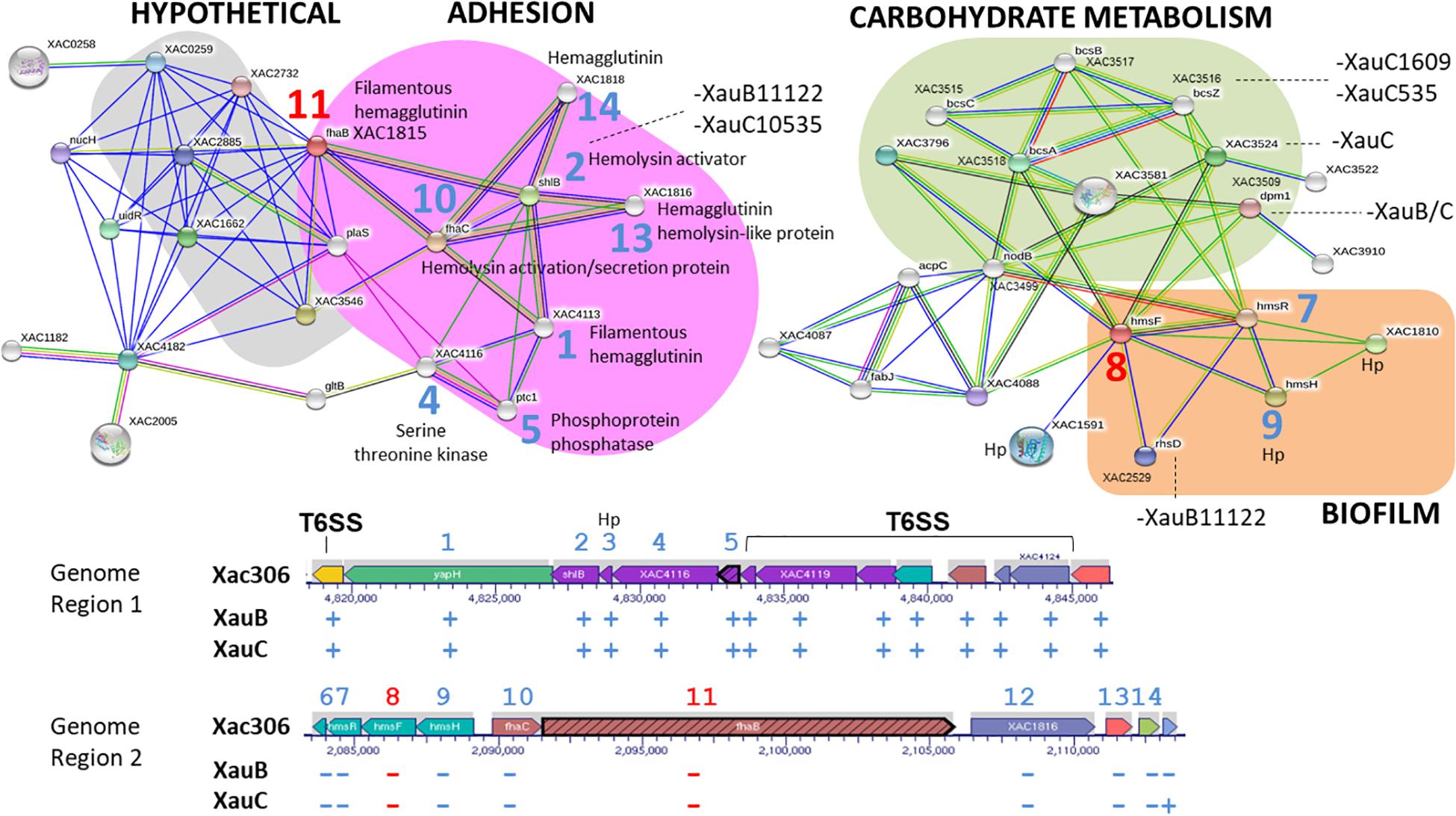
Figure 5. Networks of putative interactions between genes/proteins using as queries the A306 genes fhaB (XAC1815) and hmsF (XAC1812). For additional details see legend of Figure 2. The network figure was generated by the program STRING (Snel et al., 2000) and the genomic context figure was based on a figure generated by the BioCyc resource (Karp et al., 2017).
Using hmsF (XAC1812), we obtained an interaction network made of three clusters, two of which seem to be functionally related (Figure 5). One of the clusters (green background) contains genes/proteins associated with carbohydrate metabolism. The other cluster (orange background) contains the hmsFHR genes, related to biofilm formation.
A summary of these results is presented in Figure 6, which includes some additional pathogenicity-related genes also lacking in the XauB and XauC genomes: vapBC, a toxin-antitoxin module in Acidovorax citrulli (Shavit et al., 2015); and tspO, which encodes a protein with a potential role in the oxidative stress response, iron homeostasis, and virulence expression in Pseudomonas (Leneveu-Jenvrin et al., 2014).
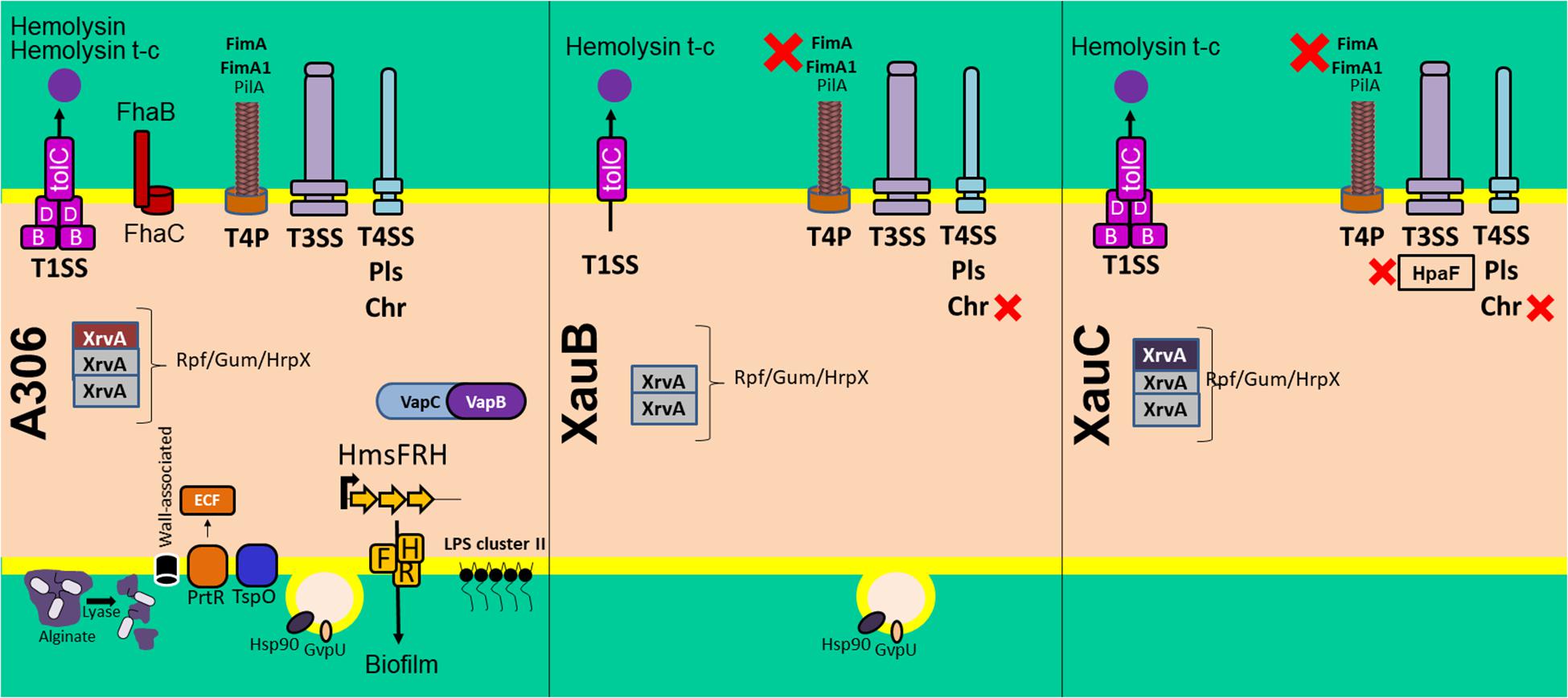
Figure 6. Summary of the comparative analyses of 11 Xanthomonas citri genomes listed in Table 2 in terms of the pathogenicity-related genes and systems discussed in the text. The red X symbol denotes lack of the genes or system next to it.
Discussion
Our results show that the three lineages inflicting citrus canker (A strains and XauB and XauC strains) can be robustly separated into two well defined clades, with A strains in one clade, which we call the Citri-citri (C-c) clade, and XauB and XauC in another clade, which we call the aurantifolii clade (Figure 1); furthermore, XauB and XauC were shown to be in a paraphyletic clade, with X. citri pv. anacardii being closer to XauB (Figure 1). It is noteworthy to observe that the C-c and aurantifolii clades contain strains that are pathogenic in taxonomically disparate plant hosts, such as citrus (X. citri pv. citri and X. citri pv. aurantifolii), leguminosae (X. citri pv. glycines and X. citri pv. fuscans emerging from more basal nodes), cashew (X. citri pv. anacardii), mango (X. citri pv. mangiferaindicae), and cotton (X. citri pv. glycines). Curiously, X. citri pv. anacardii (infecting cashew) apparently evolved within a citrus-associated clade, suggesting a possible host jump.
We have made an extensive analysis of the presence and absence of effectors in the 31 genomes we sampled to reconstruct the phylogeny. We now discuss the main results of this analysis. XopS was shown to be the only effector among the 62 investigated that is present in the C-c clade (although in some cases as a pseudogene) but absent in the aurantifolii clade (Figure 1). XopS is completely dependent on HpaB to be translocated; it contributes to disease symptoms and bacterial growth and suppresses pathogen-associated molecular pattern (PAMP)-triggered plant defense gene expression (Schulze et al., 2012). XopF1 was found to have the opposite pattern compared to xopS: present in the aurantifolii clade (in some cases as a pseudogene) and absent in the C-c clade. In Xanthomonas oryzae pv. oryzae, XopF1 has been shown to repress basal PAMP-triggered immunity response in rice (Mondal et al., 2015). A third interesting case is xopK, which is present in the C-c clade, but was found to be a pseudogene in all genomes of the aurantifolii clade. XopK has been shown to inhibit PAMP-triggered immunity upstream of mitogen-activated protein kinase cascades in Xanthomonas oryzae pv. oryzae (Qin et al., 2018). Figure 1 makes clear that there are many other differences in effector repertoires among the 31 genomes analyzed; 11 of these other effectors have been studied in terms of their gains and losses across the evolution of the 31 strains (Supplementary Figure S1). Because the pattern of gains, losses and pseudogenization is more intricate, additional studies are required to correlate these inferred histories to known phenotypic traits of the affected strains.
In addition to effectors, we have carefully analyzed the gene content in the broad categories of secretion systems-related genes and surface structure-related genes. Our main tool in this analysis, in addition to presence/absence results, was the prediction of possible interactions. These analyses resulted in several noteworthy differences of XauB and XauC strains when compared to the A306 genome.
Type I Secretion System
The T1SS is responsible for secreting toxins, such as hemolysins in E. coli (Thomas et al., 2014). In the three XauB strains investigated, both genes coding for apparatus secretory proteins (hlyB and hlyD) and genes coding for hemolysins (hlyA) were not found. This absence might contribute to a decrease in the elicitation of the plant immune response as well as to decreased competitive capability with other organisms due to the inability to secrete these toxins.
Type IV Secretion System
The T4SS has multiple functions, including transport of a variety of substrates from DNA and protein-DNA complexes to proteins, and it plays fundamental roles in both bacterial pathogenesis and adaptation to the cellular milieu in which bacteria live (Darbari and Waksman, 2015). Jacob et al. (Jacob et al., 2014), reported that the T4SS in A306, unlike the T3SS, is not associated with virulence induction, but rather in cell-cell interactions. This finding was confirmed by Souza et al. (2015), who demonstrated the involvement of the chromosomal T4SS in bacterial killing, showing that this special class of T4SS is a mediator of both antagonistic and cooperative interbacterial interactions. We speculate that the lack of the T4SS chromosomal gene cluster in XauB and XauC genomes may have a consequence in the ability of these strains to compete with other bacteria, in particular with A306 itself. If this speculation is correct, this may be an explanation for the apparent disappearance of XauB strains from the field (Chiesa et al., 2013).
Synthesis and Regulation of Type IV Pilus
Among the protein complexes involved in biofilm formation is the type IV pilus (T4p) (Dunger et al., 2014). Besides actively participating in this matrix, the T4p is of fundamental importance in the adhesion process to the host tissue in the early stages of infection and independent flagellum displacement, called twitching motility (Mattick, 2002). The orthologous gene cluster pilE-Y1-X-W-V-FimT (XAC2664-2669) of A306 in P. aeruginosa has been shown to be involved in negative regulation of swarming motility (Giltner et al., 2010; Kuchma et al., 2012). In this same context, inactivation of pilA inserted in the pilS-R-B-A-A-C-D cluster (XAC3238-3243) interfered with twitching motility, biofilm development, and adherence of XAC (Dunger et al., 2014). Thus, the lack of genes pilX, pilV, pilA, pill, and fimT genes in the XauB and XauC genomes, all involved with T4p apparatus structuring, seem to explain at least in part the decreased production of biofilm and self-aggregation capability in some XauB and XauC strains (Supplementary Table S3). On the other hand, these same results show that XauC 535 and XauC 1609 presented, respectively, the highest biofilm production and self-aggregation capability in virulence-inducing media (XVM2) among all strains, even in the absence of the genes listed; this result requires further investigation.
Alginate Biosynthesis
Alginate is an EPS related to biofilm formation and produced by bacteria of the genus Pseudomonas (Baker and Svanborg-Eden, 1989; Orgad et al., 2011). The function of alginate lyase is to hydrolyze bonds that hold the structured polymer, thereby enabling the bacterium to leave the biofilm structure, allowing its spreading by the colonized tissue (Boyd and Chakrabarty, 1994).
The intricate network we inferred for XAC2670 (which codes for an alginate biosynthesis protein in A306) may be depleted in XauB and XauC due to the lack of key genes/proteins in the composition of these clusters, as it is the case of pilX and pilV, and XAC2670 itself, which could impair the synthesis and regulation of T4p apparatus and EPS production. Importantly, there are no reports in the literature mentioning any Xanthomonas species as an alginate producer. However, it is interesting to notice the presence of at least nine genes in A306 that may be involved with synthesis and regulation of this polymer, from which four are present in the interaction networks described above.
Hemagglutinin and Hemolysin Synthesis
The hemagglutinin gene (XAC1818) has been described as fundamental to the virulence process in many organisms, including Xylella fastidiosa (Caserta et al., 2010; Voegel et al., 2010), another plant pathogen of the Xanthomonadaceae family, and in A306 (Gottig et al., 2009). The hmsHFR-hp genes (XAC1813-1810) are involved in adaptation and virulence, and have been reported respectively to be homologous to E. coli genes pgaABCD (Wang et al., 2004). Mutations in genes from this operon in members of the genera Chromobacterium, Yersinia, and Xanthomonas have resulted in reduction of biofilm formation and consequent reduction in virulence induction (Becker et al., 2009; Abu Khweek et al., 2010; Wang et al., 2012). Therefore, the absence of XAC1810-XAC1819 in the genomes of strains XauB and XauC might contribute to less efficient tissue adhesion processes and biofilm formation, and reduce cell-to-cell aggregation dependent of adhesin and exopolysaccharides molecules; this in turn would lead to reduction in tissue colonization capabilities in these strains.
Conclusion
Taken together, our results show that the XauB and XauC genomes lack many genes that are known to play a role in host infection, either in A306 or in other pathosystems. This result is consistent with the attenuated citrus canker phenotypes of the XauB and XauC strains. In addition, the lack of recent reports about the presence of XauB and XauC strains in the field suggests a scenario in which A306 or other A strains may have outcompeted the XauB and XauC strains, possibly leading to their eradication. If so, this would be a process similar to what has taken place with Candidatus Liberibacter americanus, a causative agent of citrus huanglongbin, which has reportedly been eradicated, in South America, by Candidatus Liberibacter asiaticus (Wulff et al., 2014). It is to be hoped that such knowledge can be put to practical use in the efforts to eradicate from the field the A strains as well.
Data Availability Statement
Raw reads are available at the Short Read Archive at NCBI at the following URLs: https://www.ncbi.nlm.nih.gov/Traces/study/?acc=PRJNA273983; https://www.ncbi.nlm.nih.gov/sra/?term=PRJNA273983.
Author Contributions
JS, LM, JF, JB, and FJ conceived the study. JB and FJ selected and prepared strains for sequencing. AF, RF, and JF did the genome sequencing. AV and NA assembled the genomes. NF, ÉF, WC, AS, IC, CL, RA, CG, JM, JP, LM, and JS analyzed the data and interpreted the results. JS, LM, and JP wrote the manuscript.
Funding
This study was financed in part by the Coordenação de Aperfeiçoamento de Pessoal de Nível Superior – Brasil (CAPES) – Finance Code 001 (the BIGA project). NF was funded in part by grant Fundect-MS (007/2015 SIAFEM 025139). JS, LM, NF, and AV were funded in part by Researcher Fellowships from CNPq.
Conflict of Interest
The authors declare that the research was conducted in the absence of any commercial or financial relationships that could be construed as a potential conflict of interest.
Acknowledgments
We thank Carlos Morais Piroupo for providing computational assistance.
Supplementary Material
The Supplementary Material for this article can be found online at: https://www.frontiersin.org/articles/10.3389/fmicb.2019.02361/full#supplementary-material
FIGURE S1 | Trees with the reconstruction of gains, losses, and pseudogenization events for 11 effector genes. The effector name is shown at the top of each tree frame.
TABLE S1 | Type III Secretion System Effectors investigated.
TABLE S2 | Representation of an alignment of ortholog genes having as anchor the Xac306 genome.
TABLE S3 | Results of biochemical assays related to xanthan gum and biofilm production, and aggregation (left-had side).
Footnotes
- ^ https://bitbucket.org/izhbannikov/seqyclean
- ^ https://www.pacb.com/documentation/smrt-analysis-software-installation-v2-3-0/
- ^ https://www.ncbi.nlm.nih.gov/projects/treeview/
References
Abu Khweek, A., Fetherston, J. D., and Perry, R. D. (2010). Analysis of HmsH and its role in plague biofilm formation. Microbiology 156, 1424–1438. doi: 10.1099/mic.0.036640-0
Alamuri, P., Lower, M., Hiss, J. A., Himpsl, S. D., Schneider, G., and Mobley, H. L. T. (2010). Adhesion, invasion, and agglutination mediated by two trimeric autotransporters in the human uropathogen Proteus mirabilis. Infect. Immun. 78, 4882–4894. doi: 10.1128/IAI.00718-10
An, S. Q., Febrer, M., McCarthy, Y., Tang, D. J., Clissold, L., Kaithakottil, G., et al. (2013). High-resolution transcriptional analysis of the regulatory influence of cell-to-cell signalling reveals novel genes that contribute to Xanthomonas phytopathogenesis. Mol. Microbiol. 88, 1058–1069. doi: 10.1111/mmi.12229
Antipov, D., Hartwick, N., Shen, M., Raiko, M., Lapidus, A., and Pevzner, P. A. (2016). plasmidSPAdes: assembling plasmids from whole genome sequencing data. Bioinformatics 32, 3380–3387.
Baker, N. R., and Svanborg-Eden, C. (1989). Role of alginate in the adherence of Pseudomonas aeruginosa. Antibiot. Chemother. 42, 72–79. doi: 10.1159/000417607
Bankevich, A., Nurk, S., Antipov, D., Gurevich, A. A., Dvorkin, M., Kulikov, A. S., et al. (2012). SPAdes: a new genome assembly algorithm and its applications to single-cell sequencing. J. Comput. Biol. 19, 455–477. doi: 10.1089/cmb.2012.0021
Becker, S., Soares, C., and Porto, L. M. (2009). Computational analysis suggests that virulence of Chromobacterium violaceum might be linked to biofilm formation and poly-NAG biosynthesis. Genet. Mol. Biol. 32, 640–644. doi: 10.1590/S1415-47572009000300031
Bodnar, A. M., Santillana, G., Mavrodieva, V. A., Liu, Z., Nakhla, M. K., and Gabriel, D. W. (2017). Three complete genome sequences of novel Xanthomonas citri strains from Texas carry atypical PthA alleles and unusual large plasmids. Phytopathology 107:11.
Boyd, A., and Chakrabarty, A. M. (1994). Role of alginate lyase in cell detachment of Pseudomonas aeruginosa. Appl. Environ. Microbiol. 60, 2355–2359.
Bui Thi Ngoc, L., Vernière, C., Jouen, E., Ah-You, N., Lefeuvre, P., Chiroleu, F., et al. (2010). Amplified fragment length polymorphism and multilocus sequence analysis-based genotypic relatedness among pathogenic variants of Xanthomonas citri pv. citri and Xanthomonas campestris pv. bilvae. Int. J. Syst. Evol. Microbiol. 60, 515–525. doi: 10.1099/ijs.0.009514-0
CAB-International, (2019). Xanthomonas citri (citrus canker). Wallingford: Invasive Species Compendium.
Camacho, C., Coulouris, G., Avagyan, V., Ma, N., Papadopoulos, J., Bealer, K., et al. (2009). BLAST+: architecture and applications. BMC Bioinformatics 10:421. doi: 10.1186/1471-2105-10-421
Caserta, R., Takita, M. A., Targon, M. L., Rosselli-Murai, L. K., de Souza, A. P., Peroni, L., et al. (2010). Expression of Xylella fastidiosa fimbrial and afimbrial proteins during biofilm formation. Appl. Environ. Microbiol. 76, 4250–4259. doi: 10.1128/AEM.02114-09
Chiesa, M. A., Siciliano, M. F., Ornella, L., Roeschlin, R. A., Favaro, M. A., Delgado, N. P., et al. (2013). Characterization of a variant of Xanthomonas citri subsp citri that triggers a host-specific defense response. Phytopathology 103, 555–564. doi: 10.1094/PHYTO-11-12-0287-R
Civerolo, E. L. (1984). Bacterial canker disease of citrus. J. Rio Grande Valley Hortic. Soc. 37, 127–145.
Constantin, E. C., Cleenwerck, I., Maes, M., Baeyen, S., Van Malderghem, C., De Vos, P., et al. (2016). Genetic characterization of strains named as Xanthomonas axonopodis pv. dieffenbachiae leads to a taxonomic revision of the X-axonopodis species complex. Plant Pathol. 65, 792–806.
Contreras-Moreira, B., and Vinuesa, P. (2013). GET_HOMOLOGUES, a versatile software package for scalable and robust microbial pangenome analysis. Appl. Environ. Microbiol. 79, 7696–7701. doi: 10.1128/AEM.02411-13
da Silva, A. C., Ferro, J. A., Reinach, F. C., Farah, C. S., Furlan, L. R., Quaggio, R. B., et al. (2002). Comparison of the genomes of two Xanthomonas pathogens with differing host specificities. Nature 417, 459–463.
Darbari, V. C., and Waksman, G. (2015). Structural biology of bacterial Type IV secretion systems. Annu. Rev. Biochem. 84, 603–629. doi: 10.1146/annurev-biochem-062911-102821
Davies, D. G., and Geesey, G. G. (1995). Regulation of the alginate biosynthesis gene algc in pseudomonas-aeruginosa during biofilm development in continuous-culture. Appl. Environ. Microb. 61, 860–867.
Dunger, G., Guzzo, C. R., Andrade, M. O., Jones, J. B., and Farah, C. S. (2014). Xanthomonas citri subsp citri Type IV pilus is required for twitching motility, biofilm development, and adherence. Mol. Plant Microbe Int. 27, 1132–1147. doi: 10.1094/MPMI-06-14-0184-R
Giltner, C. L., Habash, M., and Burrows, L. L. (2010). Pseudomonas aeruginosa minor pilins are incorporated into type IV pili. J. Mol. Biol. 398, 444–461. doi: 10.1016/j.jmb.2010.03.028
Goto, M. (1992). “Citrus canker,” in Plant Diseases of International Importance, Vol III, eds J. Kumer, et al. (Upper Saddle River NJ: Prentice Hall).
Gottig, N., Garavaglia, B. S., Garofalo, C. G., Orellano, E. G., and Ottado, J. (2009). A filamentous hemagglutinin-like protein of Xanthomonas axonopodis pv. citri, the phytopathogen responsible for citrus canker, is involved in bacterial virulence. PLoS One 4:e4358. doi: 10.1371/journal.pone.0004358
Hoang, V., Medrek, S. K., Pendurthi, M., Kao, C., and Parulekar, A. D. (2017). Improving donor lung management and recipient selection in lung transplantation. Am. J. Respir. Crit. Care Med. 196, 782–784.
Jaciani, F. J. (2012). Diversidade Genética de Xanthomonas citri subsp. Citri, Caracterizaçãomolecular e patogênica. (de)Xanthomonas fuscans subsp. aurantifolii e detecção deXanthomonas alfalfae em. (citrumelo)‘Swingle’ (Citrus paradisi Macf. x Poncirus trifoliataL. Raf. (.))no Brasil. Ph.D thesis, Universidade Estadual Paulista Julio de Mesquita Filho, Jaboticabal, SP, Brazil.
Jaciani, F. J., Destefano, S. A. L., Neto, J. R., and Belasque, J. (2009). Detection of a new bacterium related to Xanthomonas fuscans subsp aurantifolii Infecting Swingle Citrumelo in Brazil. Plant Dis. 93, 1074–1074. doi: 10.1094/PDIS-93-10-1074B
Jacob, T. R., de Laia, M. L., Moreira, L. M., Goncalves, J. F., Carvalho, F. M., Ferro, M. I., et al. (2014). Type IV secretion system is not involved in infection process in citrus. Int. J. Microbiol. 2014:763575. doi: 10.1155/2014/763575
Jalan, N., Kumar, D., Andrade, M. O., Yu, F., Jones, J. B., Graham, J. H., et al. (2013). Comparative genomic and transcriptome analyses of pathotypes of Xanthomonas citri subsp. citri provide insights into mechanisms of bacterial virulence and host range. BMC Genomics 14:551. doi: 10.1186/1471-2164-14-551
Karp, P. D., Billington, R., Caspi, R., Fulcher, C. A., Latendresse, M., Kothari, A., et al. (2017). The BioCyc collection of microbial genomes and metabolic pathways. Brief. Bioinform. doi: 10.1093/bib/bbx085 [Epub ahead of print].
Katoh, K., and Standley, D. M. (2013). MAFFT multiple sequence alignment software version 7: improvements in performance and usability. Mol. Biol. Evol. 30, 772–780. doi: 10.1093/molbev/mst010
Koronakis, V., Eswaran, J., and Hughes, C. (2004). Structure and function of TolC: the bacterial exit duct for proteins and drugs. Annu. Rev. Biochem. 73, 467–489. doi: 10.1146/annurev.biochem.73.011303.074104
Kuchma, S. L., Griffin, E. F., and O’Toole, G. A. (2012). Minor pilins of the type IV pilus system participate in the negative regulation of swarming motility. J. Bacteriol. 194, 5388–5403. doi: 10.1128/JB.00899-12
Kück, P., and Meusemann, K. (2010). FASconCAT: convenient handling of data matrices. Mol. Phylogenet. Evol. 56, 1115–1118. doi: 10.1016/j.ympev.2010.04.024
Larsson, A. (2014). AliView: a fast and lightweight alignment viewer and editor for large datasets. Bioinformatics 30, 3276–3278. doi: 10.1093/bioinformatics/btu531
Leneveu-Jenvrin, C., Connil, N., Bouffartigues, E., Papadopoulos, V., Feuilloley, M. G., and Chevalier, S. (2014). Structure-to-function relationships of bacterial translocator protein (TSPO): a focus on Pseudomonas. Front. Microbiol. 5:631. doi: 10.3389/fmicb.2014.00631
Li, L., Stoeckert, C. J., and Roos, D. S. (2003). OrthoMCL: identification of ortholog groups for eukaryotic genomes. Genome Res. 13, 2178–2189. doi: 10.1101/gr.1224503
Malavolta Júnior, V. A., Yamashiro, T., Nogueira, E. M. C., and Feichtenberger, E. (1984). Distribuição do tipo C de Xanthomonas campestris pv. citri no Estado de São Paulo. Summa Phytopathol. 10:11.
Mattick, J. S. (2002). Type IV pili and twitching motility. Annu. Rev. Microbiol. 56, 289–314. doi: 10.1146/annurev.micro.56.012302.160938
Mendonça, L. B. P., Zambolim, L., and Badel, J. L. (2017). Bacterial citrus diseases: major threats and recent progress. J. Bacteriol. Mycol. 5, 340–350.
Mondal, K. K., Verma, G., Junaid, M. A., and Mani, C. (2015). Rice pathogen Xanthomonas oryzae pv. oryzae employs inducible hrp-dependent XopF type III effector protein for its growth, pathogenicity and for suppression of PTI response to induce blight disease. Eur. J. Plant Pathol. 144, 311–323. doi: 10.1007/s10658-015-0768-7
Moreira, L. M., Almeida, N. F., Potnis, N., Digiampietri, L. A., Adi, S. S., Bortolossi, J. C., et al. (2010). Novel insights into the genomic basis of citrus canker based on the genome sequences of two strains of Xanthomonas fuscans subsp. aurantifolii. BMC Genomics 11:238. doi: 10.1186/1471-2164-11-238
Moreira, L. M., Facincani, A. P., Ferreira, C. B., Ferreira, R. M., Ferro, M. I., Gozzo, F. C., et al. (2015). Chemotactic signal transduction and phosphate metabolism as adaptive strategies during citrus canker induction by Xanthomonas citri. Funct. Integr. Genomics 15, 197–210. doi: 10.1007/s10142-014-0414-z
Nguyen, L.-T., Schmidt, H. A., von Haeseler, A., and Minh, B. Q. (2015). IQ-TREE: a fast and effective stochastic algorithm for estimating maximum-likelihood phylogenies. Mol. Biol. Evol. 32, 268–274. doi: 10.1093/molbev/msu300
Nikolskaya, A. N., and Galperin, M. Y. (2002). A novel type of conserved DNA-binding domain in the transcriptional regulators of the AlgR/AgrA/LytR family. Nucleic Acids Res. 30, 2453–2459. doi: 10.1093/nar/30.11.2453
Okkotsu, Y., Little, A. S., and Schurr, M. J. (2014). The Pseudomonas aeruginosa AlgZR two-component system coordinates multiple phenotypes. Front. Cell Infect. Microbiol. 4:82. doi: 10.3389/fcimb.2014.00082
Orgad, O., Oren, Y., Walker, S. L., and Herzberg, M. (2011). The role of alginate in Pseudomonas aeruginosa EPS adherence, viscoelastic properties and cell attachment. Biofouling 27, 787–798. doi: 10.1080/08927014.2011.603145
O’Toole, G. A. (2011). Microtiter dish biofilm formation assay. J. Vis. Exp. 47:2437. doi: 10.3791/2437
Parks, D. H., Imelfort, M., Skennerton, C. T., Hugenholtz, P., and Tyson, G. W. (2015). CheckM: assessing the quality of microbial genomes recovered from isolates, single cells, and metagenomes. Genome Res. 25, 1043–1055. doi: 10.1101/gr.186072.114
Peabody, C. R., Chung, Y. J., Yen, M. R., Vidal-Ingigliardi, D., Pugsley, A. P., and Saier, M. H. Jr. (2003). Type II protein secretion and its relationship to bacterial type IV pili and archaeal flagella. Microbiology 149, 3051–3072. doi: 10.1099/mic.0.26364-0
Pegos, V. R., Nascimento, J. F., Sobreira, T. J., Pauletti, B. A., Paes-Leme, A., and Balan, A. (2014). Phosphate regulated proteins of Xanthomonas citri subsp. citri: a proteomic approach. J. Proteomics 108, 78–88. doi: 10.1016/j.jprot.2014.05.005
Petrocelli, S., Arana, M. R., Cabrini, M. N., Casabuono, A. C., Moyano, L., Beltramino, M., et al. (2016). Deletion of pilA, a minor pilin-like gene, from Xanthomonas citri subsp citri influences bacterial physiology and pathogenesis. Curr. Microbiol. 73, 904–914. doi: 10.1007/s00284-016-1138-1
Qin, J., Zhou, X., Sun, L., Wang, K., Yang, F., Liao, H., et al. (2018). The Xanthomonas effector XopK harbours E3 ubiquitin-ligase activity that is required for virulence. New Phytol. 220, 219–231. doi: 10.1111/nph.15287
Revell, L. J. (2012). phytools: an R package for phylogenetic comparative biology (and other things). Methods Ecol. Evol. 3, 217–223. doi: 10.1111/j.2041-210x.2011.00169.x
Ryan, R. P., Vorholter, F. J., Potnis, N., Jones, J. B., Van Sluys, M. A., Bogdanove, A. J., et al. (2011). Pathogenomics of Xanthomonas: understanding bacterium-plant interactions. Nat. Rev. Microbiol. 9, 344–355. doi: 10.1038/nrmicro2558
Schaad, N. W., Postnikova, E., Lacy, G., Sechler, A., Agarkova, I., Stromberg, P. E., et al. (2006). Emended classification of xanthomonad pathogens on citrus. Syst. Appl. Microbiol. 29, 690–695. doi: 10.1016/j.syapm.2006.08.001
Schaad, N. W., Postnikova, E., Lacy, G. H., Sechler, A., Agarkova, I., Stromberg, P. E., et al. (2005). Reclassification of Xanthomonas campestris pv. citri (ex Hasse 1915) Dye 1978 forms A, B/C/D, and E as X. smithii subsp. citri (ex Hasse) sp. nov. nom. rev. comb. nov., X. fuscans subsp. aurantifolii (ex Gabriel 1989) sp. nov. nom. rev. comb. nov., and X. alfalfae subsp. citrumelo (ex Riker and Jones) Gabriel et al., 1989 sp. nov. nom. rev. comb. nov.; X. campestris pv malvacearum (ex smith 1901) Dye 1978 as X. smithii subsp. smithii nov. comb. nov. nom. nov.; X. campestris pv. alfalfae (ex Riker and Jones, 1935) dye 1978 as X. alfalfae subsp. alfalfae (ex Riker et al., 1935) sp. nov. nom. rev.; and "var. fuscans" of X. campestris pv. phaseoli (ex Smith, 1987) Dye 1978 as X. fuscans subsp. fuscans sp. nov. Syst. Appl. Microbiol. 28, 494–518. doi: 10.1016/j.syapm.2005.03.017
Schubert, T. S., and Miller, J. W. (1996). Bacterial Citrus Canker. Gainesville, FL: Florida Department of Agriculture and Consumer Services, Division of Plant Industry.
Schubert, T. S., Rizvi, S. A., Sun, X. A., Gottwald, T. R., Graham, J. H., and Dixon, W. N. (2001). Meeting the challenge of eradicating citrus canker in Florida - Again. Plant Dis. 85, 340–356. doi: 10.1094/pdis.2001.85.4.340
Schulze, S., Kay, S., Buttner, D., Egler, M., Eschen-Lippold, L., Hause, G., et al. (2012). Analysis of new type III effectors from Xanthomonas uncovers XopB and XopS as suppressors of plant immunity. New Phytol. 195, 894–911. doi: 10.1111/j.1469-8137.2012.04210.x
Seemann, T. (2014). Prokka: rapid prokaryotic genome annotation. Bioinformatics 30, 2068–2069. doi: 10.1093/bioinformatics/btu153
Setubal, J. C., Almeida, N. F., and Wattam, A. R. (2018). Comparative genomics for prokaryotes. Methods Mol. Biol. 1704, 55–78. doi: 10.1007/978-1-4939-7463-4_3
Shavit, R., Lebendiker, M., Pasternak, Z., Burdman, S., and Helman, Y. (2015). The vapB-vapC operon of acidovorax citrulli functions as a bona-fide toxin-antitoxin module. Front. Microbiol. 6:1499. doi: 10.3389/fmicb.2015.01499
Snel, B., Lehmann, G., Bork, P., and Huynen, M. A. (2000). STRING: a web-server to retrieve and display the repeatedly occurring neighbourhood of a gene. Nucleic Acids Res. 28, 3442–3444. doi: 10.1093/nar/28.18.3442
Souza, D. P., Oka, G. U., Alvarez-Martinez, C. E., Bisson-Filho, A. W., Dunger, G., Hobeika, L., et al. (2015). Bacterial killing via a type IV secretion system. Nat. Commun. 6:6453. doi: 10.1038/ncomms7453
Thomas, S., Holland, I. B., and Schmitt, L. (2014). The Type 1 secretion pathway - the hemolysin system and beyond. Biochim. Biophys. Acta 1843, 1629–1641. doi: 10.1016/j.bbamcr.2013.09.017
Voegel, T. M., Warren, J. G., Matsumoto, A., Igo, M. M., and Kirkpatrick, B. C. (2010). Localization and characterization of Xylella fastidiosa haemagglutinin adhesins. Microbiology 156, 2172–2179. doi: 10.1099/mic.0.037564-0
Vojnov, A. A., Slater, H., Daniels, M. J., and Dow, J. M. (2001). Expression of the gum operon directing xanthan biosynthesis in Xanthomonas campestris and its regulation in planta. Mol. Plant Microbe Interact. 14, 768–774. doi: 10.1094/mpmi.2001.14.6.768
Wang, J., Yan, Q., and Wang, N. (2012). “hmsF Is a virulence factor of the citrus canker pathogen Xanthomonas citri subsp. citri 306,” in Proceedings of the 2012 APS annual meeting, (Providebce, RI: APS), 546.
Wang, L. F., Rong, W., and He, C. Z. (2008). Two Xanthomonas extracellular polygalacturonases, PghAxc and PghBxc, are regulated by type III secretion regulators HrpX and HrpG and are required for virulence. Mol. Plant Microbe Int. 21, 555–563. doi: 10.1094/MPMI-21-5-0555
Wang, N., Pierson, E. A., Setubal, J. C., Xu, J., Levy, J. G., Zhang, Y., et al. (2017). The candidatus liberibacter-host interface: insights into pathogenesis mechanisms and disease control. Annu. Rev. Phytopathol. 55, 451–482. doi: 10.1146/annurev-phyto-080516-035513
Wang, X., Preston, J. F. III, and Romeo, T. (2004). The pgaABCD locus of Escherichia coli promotes the synthesis of a polysaccharide adhesin required for biofilm formation. J. Bacteriol. 186, 2724–2734. doi: 10.1128/jb.186.9.2724-2734.2004
Wulff, N. A., Zhang, S., Setubal, J. C., Almeida, N. F., Martins, E. C., Harakava, R., et al. (2014). The complete genome sequence of ‘Candidatus Liberibacter americanus’, associated with Citrus huanglongbing. Mol. Plant Microbe Interact. 27, 163–176. doi: 10.1094/MPMI-09-13-0292-R
Keywords: effectors, adaptation, virulence, Xanthomonas evolution, genome sequencing
Citation: Fonseca NP, Patané JSL, Varani AM, Felestrino ÉB, Caneschi WL, Sanchez AB, Cordeiro IF, Lemes CGdC, Assis RdAB, Garcia CCM, Belasque J Jr, Martins J Jr, Facincani AP, Ferreira RM, Jaciani FJ, Almeida NFd, Ferro JA, Moreira LM and Setubal JC (2019) Analyses of Seven New Genomes of Xanthomonas citri pv. aurantifolii Strains, Causative Agents of Citrus Canker B and C, Show a Reduced Repertoire of Pathogenicity-Related Genes. Front. Microbiol. 10:2361. doi: 10.3389/fmicb.2019.02361
Received: 01 March 2019; Accepted: 27 September 2019;
Published: 11 October 2019.
Edited by:
Dawn Arnold, University of the West of England, United KingdomReviewed by:
Jeffrey Jones, University of Florida, United StatesDavid J. Studholme, University of Exeter, United Kingdom
Copyright © 2019 Fonseca, Patané, Varani, Felestrino, Caneschi, Sanchez, Cordeiro, Lemes, Assis, Garcia, Belasque, Martins, Facincani, Ferreira, Jaciani, Almeida, Ferro, Moreira and Setubal. This is an open-access article distributed under the terms of the Creative Commons Attribution License (CC BY). The use, distribution or reproduction in other forums is permitted, provided the original author(s) and the copyright owner(s) are credited and that the original publication in this journal is cited, in accordance with accepted academic practice. No use, distribution or reproduction is permitted which does not comply with these terms.
*Correspondence: Leandro Marcio Moreira, lmmorei@gmail.com; João C. Setubal, setubal@iq.usp.br
†These authors have contributed equally to this work