- 1Department of Plant Protection, College of Agriculture, University of Kurdistan, Sanandaj, Iran
- 2Department of Horticultural Sciences, University of Kurdistan, Sanandaj, Iran
- 3Safiabad Agricultural Research and Education and Natural Resources Center, Agricultural Research, Education and Extension Organization (AREEO), Dezful, Iran
- 4Department of Sustainable Agro-Ecosystems and Bioresources, Research and Innovation Centre, Fondazione Edmund Mach (FEM), San Michele all’Adige, Italy
- 5Center Agriculture Food Environment (C3A), University of Trento, San Michele all’Adige, Italy
This study assessed the feasibility of using essential oils (EOs) against Colletotrichum nymphaeae inciting strawberry anthracnose. Two EOs, extracted from Allium sativum (garlic) and Rosmarinus officinalis (rosemary), were selected because their fungicide efficacy was already well characterized under laboratory and greenhouse conditions. We characterized both EOs in terms of efficacy and impact on qualitative traits and sensory quality of strawberry fruit. The gas chromatography–mass spectrometry analysis confirmed the Diallyl trisulfide (29.08%) and (α)-pinene (15.779%) as the main components of A. sativum and R. officinalis EOs, respectively. Both A. sativum and R. officinalis EOs significantly inhibited the mycelial growth and conidial germination of C. nymphaeae in contact and vapor assays compared with untreated control. However, EC50 assay indicated A. sativum EO was more effective than R. officinalis EO against the pathogen. Malformations of the vegetative structures of the pathogen exposed to both EOs were revealed as shriveled, collapsed, and swelling mycelia in the cultures. Both EOs confirmed their efficacy under in vivo and greenhouse conditions; in fact, they significantly reduced the development of fruit decay and anthracnose disease incidence and severity, compared with untreated controls. Both EOs preserved sensory attributes and quality parameters of strawberry fruit including firmness, total soluble solids, ascorbic acid, antioxidant activity, and anthocyanin, but may leave unwanted smells. These findings suggest that two EOs can potentially represent an alternative to synthetic chemical fungicides against C. nymphaeae preserving fruit quality factors, although their cost and the impact on the fruit odor must be carefully taken into consideration before developing a commercial product.
Introduction
Strawberry anthracnose caused by Colletotrichum nymphaeae (Pass.) Aa, is a major disease of strawberry (Fragaria × ananassa Duch.) limiting production worldwide by affecting fruits in preharvest and postharvest (Baroncelli et al., 2015; Karimi et al., 2017; Wang N.Y. et al., 2019). Although the pathogen causes symptoms on various parts of the plant, the most important damage is the fruit rot (Peres et al., 2005). In fact, large and sunken lesions on strawberry fruits make them unmarketable, leading to considerable production losses particularly under epidemic condition (Howard et al., 1992). Chemical fungicides, such as thiabendazole or azoxystrobin (Smith and Black, 1992; Wedge et al., 2007), are mainly used for the control of the disease, but their repeated application may result in serious problems including high residues on fruits, the development of fungicide resistance in pathogen populations, and environmental pollution (Karimi et al., 2016a). Furthermore, no suitable anthracnose-resistant strawberry cultivars have been obtained so far, mainly because resistant strawberry germplasms against the disease is not available. Considering the aforementioned concerns, a promising approach is the development of alternatives to synthetic chemical fungicides. In recent years, application of plant essential oils (EOs) received increasing attention as natural alternative to synthetic fungicides in the control of main postharvest diseases of different products (Sellamuthu et al., 2013; Fan et al., 2014; Ali et al., 2015; Shao et al., 2015; Vilaplana et al., 2018), including strawberries (Shao et al., 2013).
Essential oils are highly complex mixtures of volatile aromatic compounds synthesized by plants, which have diverse ecological functions, including the attraction of pollinators and defense mechanisms against harmful microorganisms (Bakkali et al., 2008; Medeiros et al., 2011; Li et al., 2013; Amaral et al., 2015; Costa et al., 2015; De Almeida et al., 2018). Monoterpenes, aldehydes, allyl phenols, alcohols, acids, and esters are the main components of plant EOs (Prakash et al., 2015; De Almeida et al., 2018). They are mainly produced and accumulated in undifferentiated cells, glandular trichomes, secretory ducts, and resin ducts (Nakatsu et al., 2000; Pimental et al., 2018).
Several studies have shown the inhibitory effect of plant EOs against phytopathogens (Bouchra et al., 2003; Daferera et al., 2003; Soylu et al., 2006; Bi et al., 2012; Ali et al., 2015; Amini et al., 2016). Some plant EOs have been evaluated against fruit rot of strawberry caused by Rhizopus stolonifer, Penicillium digitatum, Aspergillus niger, Botrytis cinerea, and C. nymphaeae (Reddy et al., 1998; Asghari et al., 2009; Nabigo and Morshedi, 2011; Zamani-Zadeh et al., 2013; Karimi et al., 2016a). Among the EOs studied so far, the antimicrobial activity of EOs from Allium sativum L. (garlic) (Corzomartinez et al., 2007; Mnayer et al., 2014; Satyal et al., 2017) and Rosmarinus officinalis L. (rosemary) (Mangena and Muyima, 1999; Soylu et al., 2006, 2010; Ozcan and Chalchat, 2008) has been proven against several plant pathogens and relatively well characterized. Some EOs may influence the quality of the treated products (Sivakumar and Bautista-Banos, 2014; Vilaplana et al., 2018). Although EOs may negatively affect the quality of fruits, for example, by giving a unwanted smell, there are no compressive studies on strawberry fruit rots, which take into consideration the fungicidal effect, their composition, and the side effect on fruit quality. In addition, to the best of our knowledge, inhibitory effects of A. sativum and R. officinalis EOs against C. nymphaeae were not yet assessed.
Therefore, the aim of this study was to evaluate the antifungal activity of two different EOs possessing a pungent odor, A. sativum and R. officinalis, against C. nymphaeae, under in vitro, in vivo, and greenhouse conditions and their effects on fruit quality parameters.
Materials and Methods
Pathogen
A highly pathogenic strain of C. nymphaeae (CCTUCCh32) provided from the culture collection of Tabriz University in Iran (Karimi et al., 2017) was used in the experiments. Fungal cultures were grown on potato dextrose agar (PDA; Merck Company, Germany) at 24°C ± 2°C for 10 days. Conidial suspension of C. nymphaeae was made by flooding fungal cultures with 15 mL of sterile distilled water containing Tween 80 (0.5%, vol/vol) and filtered through three layers of sterile cheesecloth to remove mycelial debris. The concentration of conidia was adjusted to 106 spores/mL using hemocytometer.
Fruits
Healthy and red ripened strawberry fruits (cv. Paros) having the same age and size were collected from an untreated strawberry farm in Kamyaran city, in Iran, at code 87 of BBCH (Biologische Bundesanstalt, Bundessortenamt und CHemische Industrie) scale (Meier, 2001). Fruits were transferred to laboratory, surface-disinfected with 70% ethanol for 30 s, rinsed with sterile distilled water (three times), and air-dried under a laminar flow hood for 10 min before further use.
Essential Oil Extraction and Gas Chromatography–Mass Spectrometry Analysis
Essential oil of A. sativum was purchased from Zardband Pharmaceuticals Company, Tehran, Iran (no. 17-350-003). Essential oil of R. officinalis was extracted from aerial parts collected from a farm located in the University of Kurdistan, Iran, at flowering stage. Plant samples were air-dried in the dark at room temperature for 2 weeks and grounded, and EO was obtained by hydrodistillation using Clevenger-type distillation apparatus for 3 h. The resulting oil (approximately 1 mL of EO per 100 g of dried raw plant materials) was dried with anhydrous sodium sulfate and stored at 4°C in the dark in a glass bottle. Both EOs were analyzed with an Agilent 7890 A gas chromatography (GC) coupled with Agilent 5975C mass spectrometry (MS) (Agilent Technologies, United States), using an HP-5 MS capillary column (30 m × 0.25 mm, film thickness 0.25 μm). The analytical was carried out as described by Amini et al. (2016). Identification of the EOs components was performed by comparison of their retention indexes with those reported in the literatures (Ozcan et al., 2006) and their mass spectra with those in the WILEY/NBS.
Minimal Inhibitory Concentration Using Contact and Vapor Assays
Six different concentrations of A. sativum (20, 155, 290, 430, 560, and 700 μL/L) and R. officinalis (100, 420, 740, 1,060, 1,380, and 1,700 μl/L) EOs were selected using a pretest analysis against C. nymphaeae (data not shown). The concentrations of EOs with 10 and 90% inhibition on the mycelial growth of the pathogen were subtracted and divided by five to determine other concentrations. The antifungal activity of the EOs was estimated as a reduction of the mycelial growth of C. nymphaeae under in vitro conditions using contact and vapor assays on PDA, described as follows. In contact assay, different amounts of EOs (μL/L) dissolved in 0.5% (vol/vol) Tween 80 were mixed to molten PDA to obtain the desired concentrations. The obtained solutions (60 mL) were added to each plate (9-cm diameter). Upon solidification, 5-day-old mycelial disk plug (5-mm diameter) of C. nymphaeae was placed in the center of each plate. Plates containing only freshly prepared PDA were used as control. The plates were incubated at 24°C ± 2°C for 10 days. Four plates were used per treatment. The percentage of inhibition was calculated using the following formula:
Inhibition percent = [(dc − dt)/dc] × 100, where dc is radial growth of the pathogen in control, and dt is radial growth of pathogen in treatments (Thomidis and Filotheou, 2016).
In vapor assay, a sterile filter paper disk (5.0-mm diameter, Whatman no.1) dipped into different concentrations of EOs (μL/L) dissolved in 0.5% (vol/vol) Tween 80 was placed in the inner space of the inverted lid of the plates. One mycelial disk plug of the pathogen (5-mm diameter) was taken from the margin of a 5-day-old culture and was placed in the center of plate containing PDA. The plates were sealed with parafilm to avoid any loss of volatile compounds. The filter paper disks loaded with the sterile distilled water were used as control. Each treatment included four replicated plates. The plates were incubated at 24°C ± 2°C for 10 days. The percentage of inhibition was calculated using aforementioned formula. Finally, the concentrations of each EO (μL/L) providing 10% (EC10), 50% (EC50), and 90% inhibition (EC90) against C. nymphaeae were measured by interpolation from linear regression analysis using the SPSS v16.0 software.
Inhibitory effect of A. sativum and R. officinalis EOs on conidial germination of C. nymphaeae at selected concentrations (μL/L) was evaluated according to the method as described by Hosseni et al. (2020). For this purpose, 1 mL of different concentrations of each EO (μL/L) containing Tween 80 (0.5%, vol/vol) was pipetted into microtube (5 mL) containing 1 mL of the pathogen’s suspension at concentration of 106 conidia/mL to obtain final concentrations. Microtubes were placed on the rotary shaker in 120 revolutions/min (rpm) at 25°C ± 2°C for 24 h. Afterward, 50 μL of resulting solutions was evenly spread on plates (9 cm) containing water agar medium (2% WA) using an L-shaped spreader. Essential oil-free tubes containing Tween 80 (0.5%, vol/vol) served as controls. Three replicates per treatment were prepared. Plates were incubated at 25°C ± 2°C for 48 h. For each replicate, 200 conidia were counted using an optical microscope (Olympus BX51, Japan; 40 × magnification using a micrometer), and the percentage of conidia germinated was calculated using the following formula:
Percentage of germinated conidia = number of conidia germinated/total number of conidia × 100.
A conidium was adverted to have germinated if the length of the germ tube was larger than or equal to the conidial diameter.
Scanning Electron Microscopy
Total mycelial growth inhibition of the pathogen was observed with A. sativum and R. officinalis EOs at concentrations of 700 and 1,700 μL/L, respectively; therefore, the effect of EOs on the fungal structures of C. nymphaeae CCTUCCh32 was studied at a lower concentration (560 and 1,380 μL/L, respectively) using scanning electron microscopy (SEM) in contact assay (TESCAN MIRA3, Czechia). After 7 days, fungal structures of C. nymphaeae were freeze-dried, and morphological changes were observed.
in vivo Antifungal Activity Assay
Effect of A. sativum and R. officinalis EOs on the development of fruit decay was studied using contact and vapor assays at concentrations of 700 and 1,700 μL/L, respectively. These selected concentrations were selected based on the total inhibitory effects under in vitro condition.
In contact assay, the fruits were dipped in sodium hypochlorite 1% for 1 min and then rinsed three times in sterile distilled water and let to dry under laminar hood on sterile filter paper. Dried fruits were treated with each EO in above given concentrations containing 0.5% (vol/vol) Tween 80 for 10 s. Treated and untreated fruits were then wounded (one mm wide and deep) using a sterilized scalpel at the fruit equator zone before inoculation. Conidial suspensions of C. nymphaeae (106 conidia/mL, 10 μL) containing 0.5% (vol/vol) Tween 80 were then added to each wound on treated fruits. The wounded fruits inoculated with the pathogen’s inoculum and dipped only in sterile distilled water containing 0.5% (vol/vol) Tween 80 served as untreated inoculated and non-inoculated controls, respectively. Each treatment included four replicates containing five fruits each. The fruits were placed in plastic boxes under high relative humidity conditions (>80%) and incubated at 24°C ± 2°C with a 12-hour photoperiod. After 7 days, disease severity (DS) was measured using a scale of 0 to 5, where 0 represents healthy fruits, and 1, 2, 3, 4, and 5 represent <20%, 20.1% to 40%, 40.1% to 60%, 60.1% to 80%, and 80.1% to 100% of the fruit area rotted, respectively, as described by Huang et al. (2011).
In vapor assay, healthy strawberry fruits were disinfected as described above and dried under laminar hood. Each fruit was wounded (1 mm wide and deep) using a sterile scalpel. A conidial suspension of C. nymphaeae (10 μL) at concentration of 1.0 × 106 conidia/mL containing 0.5% (vol/vol) Tween 80 was then added to each wound. Inoculated fruits were placed in plastic boxes and 2 mL of each EO was added to a plate (2-cm diameter and 2-cm height) and placed next to the fruits in the center of plastic box. Inoculated and non-inoculated fruits into the boxes containing sterile distilled water were determined as positive and negative controls. To avoid any loss of volatile compounds, the plastic boxes were immediately sealed with layers of parafilm and maintained at 24°C ± 2°C for 7 days. Each treatment included three replicates containing five fruits each. DS was measured as mentioned previously (Huang et al., 2011). The percentage of disease incidence (DI), DS, and disease reduction (DR) were measured using the following formulas with some modifications (Nutter et al., 2006):
Where ni is the number of samples with the same score, vi is the scoring index of DS from 0 to 5 corresponding to each sample, N is the total number of the samples, and V is the highest DS index score.
Where is the mean of DS per treatment, and is the mean of DS in the untreated inoculated control.
Greenhouse Assay
In the greenhouse trial, healthy 5-week-old strawberry plants (cv. Paros, susceptible to anthracnose fruit rot) were initially purchased at the beginning of September from a local strawberry nursery (Sanandaj, Iran) and transplanted into 10-cm-diameter pots containing clay/sand/manure mix in a ratio of 2:1:1. The pots were kept in greenhouse at 25°C ± 2°C, 60% to 70% RH, with a 16:8-h light–dark photoperiod and irrigated manually every 2 to 3 days. The plants were treated with A. sativum and R. officinalis EOs at concentrations of 700 and 1,700 μL/L EOs, respectively, until runoff using a handheld atomizer. After 24 h, treated plants were sprayed with a 5 mL conidial suspension of C. nymphaeae containing Tween 80 (0.5%, vol/vol) at concentration of 1.0 × 106 conidia/mL and kept at 25°C ± 2°C, at high relative humidity (>80%) for 24 h covered with a 0.1-mm-thick transparent plastic film to preserve the moisture. The plants inoculated with fungal conidia and treated with distilled water containing Tween 80 (0.5%, vol/vol) were served as untreated non-inoculated and inoculated controls, respectively. Each treatment was composed of three replicates (three pots with one plant each), set up in a completely randomized block design. Disease severity was scored according to Delp and Milholland (1980), using a scale of 0 to 5, where 0 = “healthy petiole without lesions,” 1 = “petiole with lesions <3 mm in length,” 2 = “petiole with lesions from 3 to 10 mm,” 3 = “petiole with lesions from 10.1 to 20 mm,” 4 = “petiole with lesions from >20 mm,” and 5 = “entirely necrotic petiole and plant is dead.” Four randomly selected petioles per plant were assessed, and petiole infection by C. nymphaeae was confirmed by reisolation of the pathogen. The percentage of DI, DS, and DR were measured using aforementioned formula.
Effect of EOs on the Postharvest Physicochemical Quality of Strawberry Fruit
In order to measure the effect of the EOs on the postharvest quality parameters of strawberry fruit, healthy fruits having the same age and size were treated separately with EOs of A. sativum and R. officinalis in a contact assay setup, at the selected concentrations of 700 and 1,700 μL/L, respectively. Fruits treated with distilled water were used as untreated control. Treated fruits were air-dried under a laminar flow hood for 10 min and stored into polyethylene bags in cold storage (1°C and 80–85% RH) for 8 days. Three replicates per treatment containing five fruits were examined. The quality parameters including weight loss (WL), firmness, total soluble solid (TSS), titratable acidity (TA), pH, ascorbic acid (AA), total flavonoid (TF), total phenol (TP), total antioxidant activity (TAA), peroxidase activity (POD), and anthocyanin were measured at harvest time (before treatment, 0 days) and 8 days after cold storage.
The strawberry fruits were weighed before treatment (M1) and after 8 days of storage (M2). WL was measured as [(M1 − M2)/M1] × 100 and expressed as percentage (Sogvar et al., 2016).
Fruit firmness was measured after 8 days of storage at two points on the equatorial region using a TA-XT2 texture analyzer (Santam, STM-1, Iran) fitted with an 8-mm-diameter cylindrical probe. The probe descended toward the sample at the speed of 0.5 mm s–1. The firmness was defined as the maximum force (N).
Two pieces on two opposite sides of each fruit were isolated, pooled, and juiced for TSS and TA analyses. The TSS was recorded at harvest time and after 8 days of storage using a temperature-compensated refract meter (Atago ATC Co., Japan). The TA was recorded by titrating 3 mL of juice in 27 mL distilled water with 0.1 N NaOH solutions to pH 8.1. The TA content was defined as the percentage of citric acid (%). The pH of fruit juice was recorded using a pH meter (Motorhome, Switzerland).
AA was determined for each treatment according to the method described by Kevers et al. (2007). Two pieces on two opposite sides of each fruit were frozen in liquid nitrogen and conserved at −80°C until used for study. Frozen fruit sample (0.5 g) was added to 1 mL of 0.5% metaphosphoric acid (wt/vol) and was shaken at 12,000 rpm for 15 min at 4°C. The reaction mixture absorption was measured with spectrophotometer (Unico UV 2100, United States) at 510 nm. The results were expressed as mg AA per 100 g of fruit fresh weight (FW) using aqueous AA standard (Koushesh Saba and Moradi, 2016).
The TF in strawberry fruit was measured using the method described by Zhishen et al. (1999) with some modifications. Two hundred microliters of fruit extracts was dissolved in 1,280 μL distilled water and 60 μL 5% NaNO2 (wt/vol). After 5 min, 60 μL 10% AlCl3 (wt/vol) and 40 μL NaOH (1 mol L–1) were added to the mixture, respectively. The final mixture was stirred, and absorbance was recorded at 510 nm. The results were expressed as mg of rutin per 100 g of fruit FW.
The TP of fruit juice was evaluated using the method described by Singleton et al. (1999) with some modifications. Briefly, 20 μL fruit extracts was added to 250 μL distilled water, 750 μL solution of 10% Folin–Ciocâlteu (vol/vol), and 800 μL 7.5% Na2CO3 (wt/vol). Samples were placed in darkness for 1 h at 25°C. The absorbance of the samples was read at 765 nm using spectrophotometer. The TP was reported as mg gallic acid equivalents per 100 g fruit FW using aqueous gallic acid standard.
The TAA was measured using 2,2-diphenyl-1-picrylhydrazyl (DPPH) assay as described by Patras et al. (2009). For measurement of antioxidant activity, 200 μL of fruit crude extracts was mixed with 500 μL DPPH (0.238 mg per 1 mL methanol). After 30 min at 30°C, absorbance of the samples was recorded at 515 nm, and radical scavenging activity was calculated using the following formula:
Percentage of DPPH inhibition (IP) was measured as follows:
IP = [(Ab − As)/Ab) × 100, where Ab and As are the absorbance values of the control and treatment, respectively.
The POD activity was tested using the method described by Ghanati et al. (2002) with some modifications. Approximately 0.5 g frozen fruit powder was ground with 2 mL cold 100 mmol L–1 phosphate-buffered sodium (pH 7) containing 0.025 g polyvinylpolypyrrolidone (wt/vol). The homogenate was centrifuged at 15,000 rpm at 4°C for 15 min. One milliliter of resulting supernatants was used as crude enzyme extracts for assaying the enzyme activities. Four hundred microliters of crude enzyme extract was dissolved in 400 μL 50 mmol L–1 phosphate-buffered potassium (pH 6.6), 140 μL 0.03% H2O2, and 140 μL 1% guaiacol. The unit of enzyme activity was expressed as an increase in absorbance at 470 nm at 25°C for 2 min.
The anthocyanin content of fruit juice was measured using a pH difference as described by Cheng and Breen (1991). Using two buffers with different pHs including 400 mL 1 mol CH3COONa, 240 mL 1 N HCl, 360 mL distilled water, pH 4.5, and 125 mL 0.2 N KCl, 385 mL 0.2 N HCl, pH 1. In continue 1 g of strawberry frozen tissue was added to 8 mL buffers separately and homogenized. The homogenate was then centrifuged at 6,000 rpm at 4°C for 15 min. The absorbance of the supernatants was monitored at 520 and 700 nm at two pH values (1 and 4.5) using spectrophotometer (Unico UV 2100, United States). The following formula was used to calculate anthocyanin content based on pelargonidin triglycoside: A = (A520 nm − A700 nm) pH 1 − (A520 nm − A700 nm) pH 4.5.
Total anthocyanin (mg/L) = (A × 433.1 × 103 × DF)/ (22,400 × 1 × 1,000), where DF = dilution factor, 433.1 = molecular weight of pelargonidin, and 22,400 = molar extinction coefficient, L mol–1 cm–1.
Sensory Analysis
The sensory analysis was carried out according to Lu et al. (2017) with some modifications. It was carried out in a sensor panel room at the University of Kurdistan in three different days at 10:00 AM at 25 to 29°C. Eleven trained panelists (assessors, five males and six females, aged 20–30 years) on a 5-point scale (1 = very weak, 2 = weak, 3 = intermediate, 4 = good, and 5 = very good) for the sensory parameters including flavors, odor, taste (sweet, sour), and overall acceptance were studied. All parameters were measured at harvest time and 8 days of storage. There was 5-min interval between testing with providing water between assessors.
Statistical Analysis
Parametric data in this study were statistically analyzed as a completely randomized design by standard analysis of variance after verification of normal distribution and homoscedasticity using SAS version 8.2 software. The means were compared with Tukey test and presented as mean ± standard error.
The data for sensory evaluation were tested for normality, and because of lacking normality distribution, all data were subjected to non-parametric analysis using Kruskal–Wallis test by SPSS (Statistical Product and Service Solutions) version 16.0.
Results
Chemical Composition of the EOs
The chemical compositions of the A. sativum and R. officinalis EOs were revealed by GC-MS, and the results are presented in Tables 1, 2. A total of 8 and 19 compounds were identified in the A. sativum and R. officinalis EOs, representing 99.27 and 98.56% of the total oil compositions, respectively. The major components of A. sativum EO were allyl trisulfide (29.08%), diallyl disulfide (28.23%), and diallyl sulfide (16.106%), whereas α-pinene (15.779%), bornyl acetate (12.660%), camphor (11.998%), and 1,8-cineol (11.268%) constituted the most abundant compounds of R. officinalis EO.
In vitro Antifungal Activity
In this study, both EOs of A. sativum and R. officinalis significantly (p ≤ 0.01) inhibited the mycelial growth of C. nymphaeae in contact and vapor phases under in vitro compared with control. The inhibitory efficacy increased concomitantly with an increase in EOs concentration (Figure 1). Moreover, the vapor effect of both EOs was found to be more effective in the reduction of the mycelial growth of C. nymphaeae compared with contact effect (Table 3). Both EOs in this study exhibited varying degrees of antifungal activity against C. nymphaeae. However, the present data showed that A. sativum EO was more toxic than R. officinalis EO to C. nymphaeae at the same dosage (Table 3). Overall, the EO of A. sativum (EC5010.10 and 3.74 μL/L) exhibited significantly higher antifungal activity than R. officinalis EO (EC50 75.49 and 43.77 μL/L) against pathogen in both contact and vapor assays (Table 3).
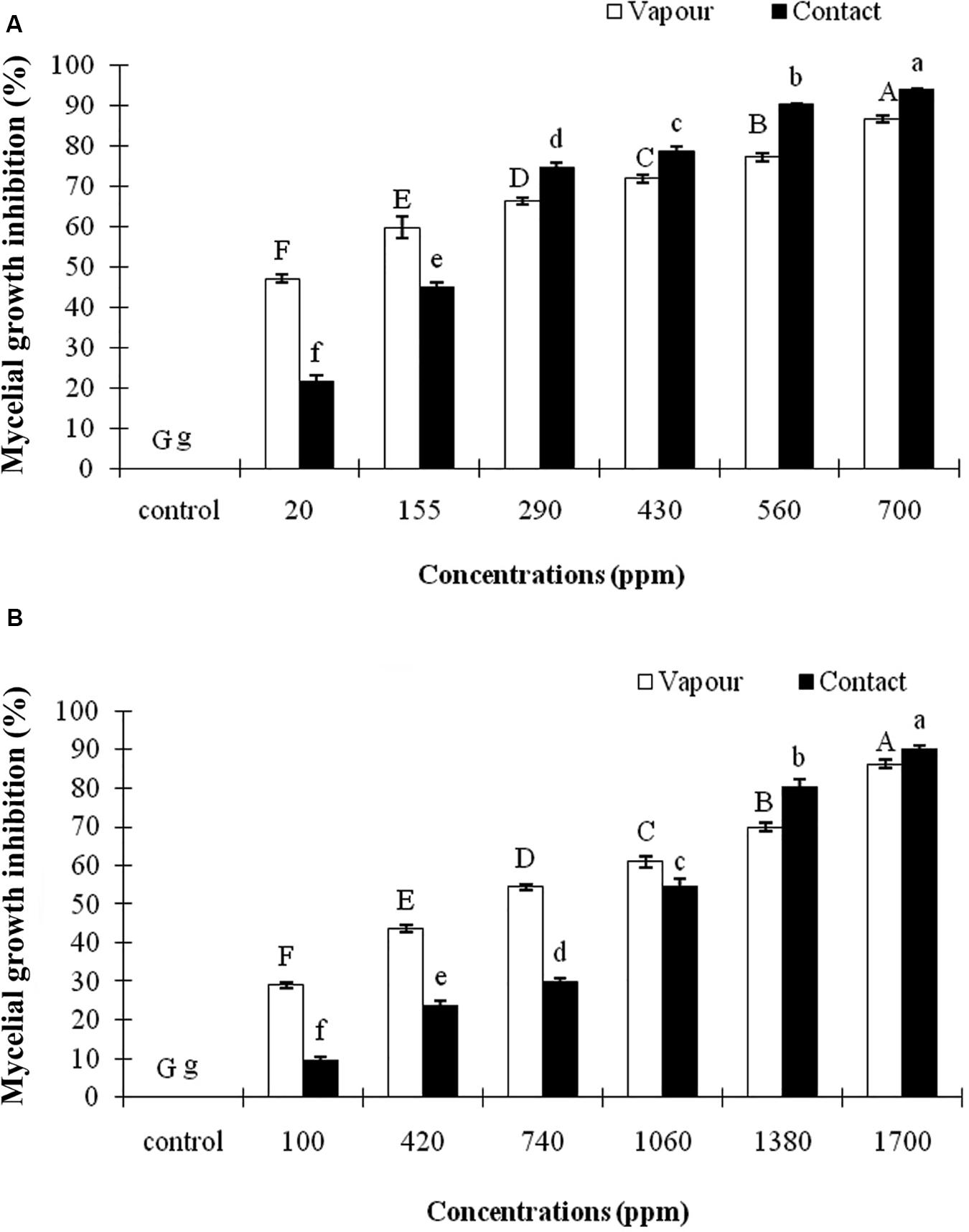
Figure 1. Inhibitory effect of different concentrations of Allium sativum L. (A) and Rosmarinus officinalis L. (B) essential oils on the mycelial growth of Colletotrichum nymphaeae CCTUCCh32 on potato dextrose agar based on contact and vapor assays. Different letters show significant difference (P ≤ 0.01) according to Tukey test.
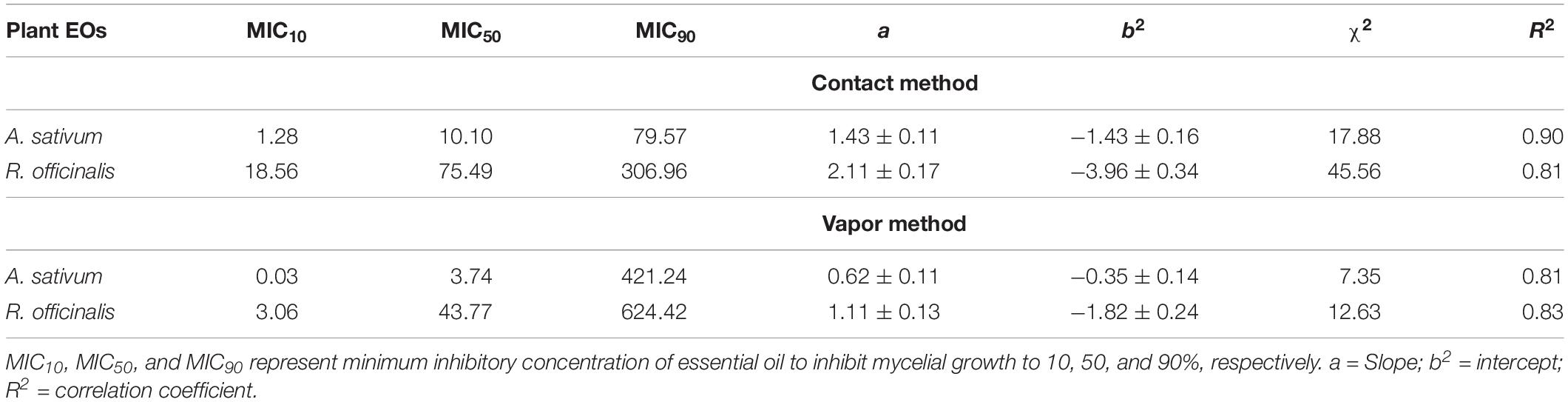
Table 3. Comparisons of MIC10, MIC50, and MIC90 of Allium sativum L. and Rosmarinus officinalis L. essential oil (μL/L) in reduction of the mycelial growth of Colletotrichum nymphaeae CCTUCCh32 (at confidence limit 95%) using contact and vapor assays on potato dextrose agar.
The effects of A. sativum and R. officinalis EOs on the conidial germination of C. nymphaeae are presented in Figure 2. The conidial germination of C. nymphaeae was significantly inhibited by both EOs at selected concentrations, and inhibition rate was increased concomitant with an increase in EOs concentration (Figure 2).
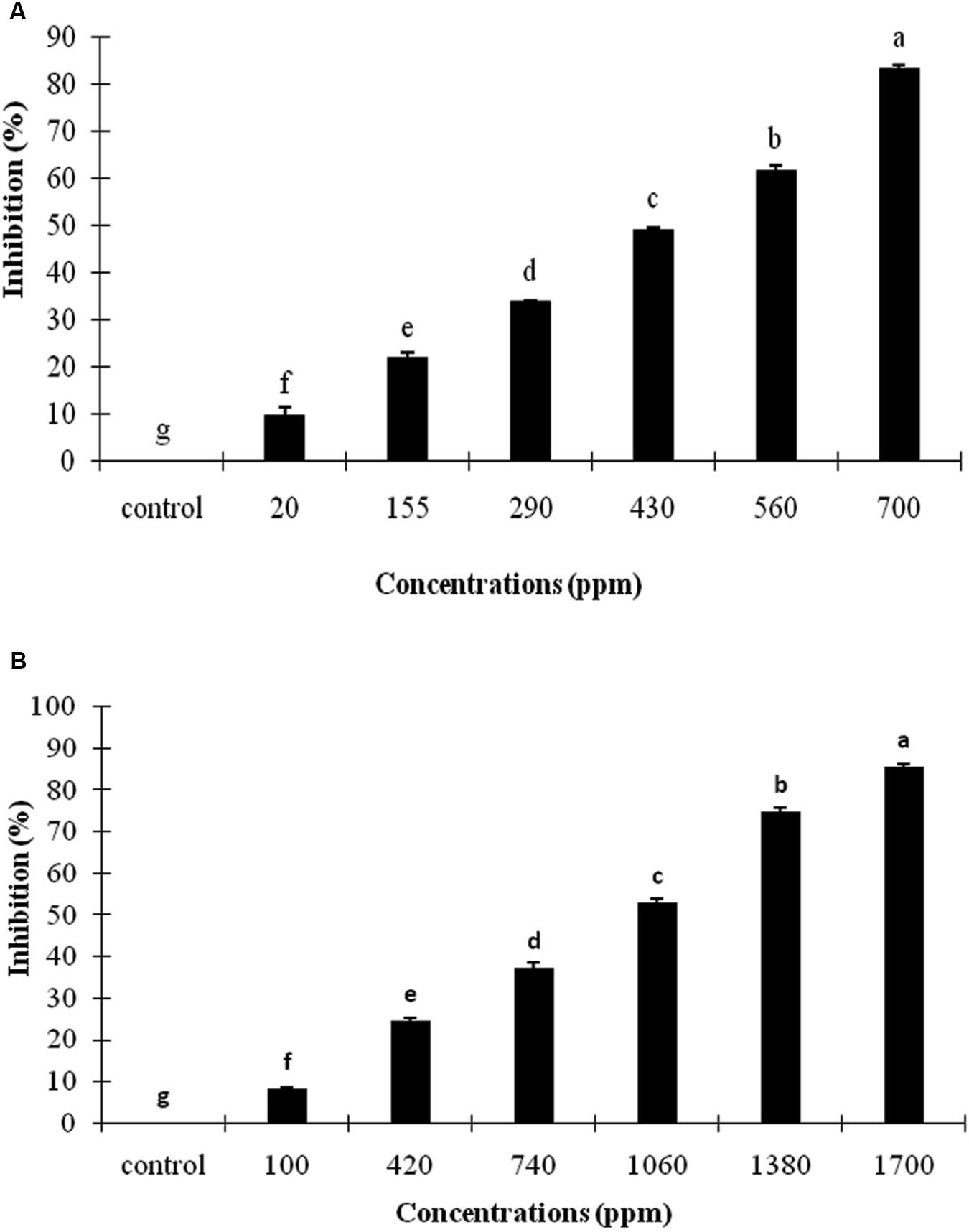
Figure 2. Inhibitory effect of Allium sativum L. (A) and Rosmarinus officinalis L. (B) essential oils on conidial germination of Colletotrichum nymphaeae CCTUC Ch32 under in vitro. Means with different letters show significant difference (P ≤ 0.01) according to Tukey test.
Effect of Plant EOs on the Hyphae Morphology
Morphological malformations in the fungal structures of C. nymphaeae exposed to A. sativum and R. officinalis EOs were observed using SEM (Figure 3). Fungal structures of C. nymphaeae showed classical degenerative changes as shriveled, collapsed, and swelling mycelia showing also cytoplasmic coagulation, which were not present in untreated control (Figure 3).
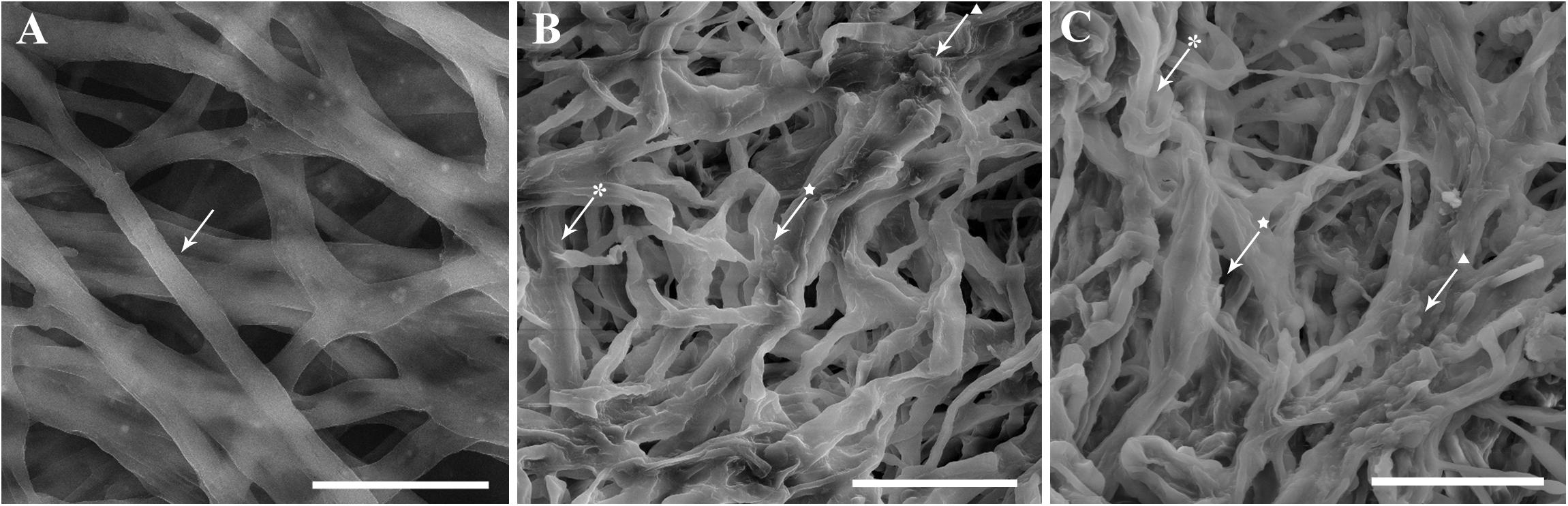
Figure 3. Morphological responses of Colletotrichum nymphaeae CCTUCCh32 to the effects of Allium sativum L. (B) and Rosmarinus officinalis L. (C) essential oils compared with untreated control (A) revealed by SEM images. Arrows show obvious malformations as shriveled (), collapsed (★), and swelling (▲) mycelia of C. nymphaeae exposed to A. sativum and R. officinalis essential oils compared with intact mycelial structures in untreated control. Scale bars: A = 10 μm, B and C = 20 μm.
Efficacy of EOs on Fruit Decay Development Under in vivo
The efficacy of the EOs to reduce fruit decay development in both contact and vapor assays is presented in Table 4. The DI was 100% in the untreated inoculated control, in both contact and vapor assays. Anthracnose symptoms in untreated inoculated fruits were observed as black, sunken necrotic lesions containing conidial mass on fruits (data not shown). On the contrary, the percentage of DI in the inoculated fruits treated with EOs of A. sativum and R. officinalis in contact phase was 37.5 and 62.5%, respectively, whereas the corresponding results in the vapor assay were 12.5 and 6.25%, respectively. Moreover, both EOs significantly (p ≤ 0.01) reduced the severity of fruit decay development compared with untreated inoculated control in both contact and vapor assays. In contact phase, A. sativum and R. officinalis EOs reduced the fruit decay development by 90.62 and 84.37%, respectively, whereas the corresponding results were 97.36% (A. sativum) and 98.68% (R. officinalis) in the vapor assay without any significant difference with untreated non-inoculated control (Table 4).
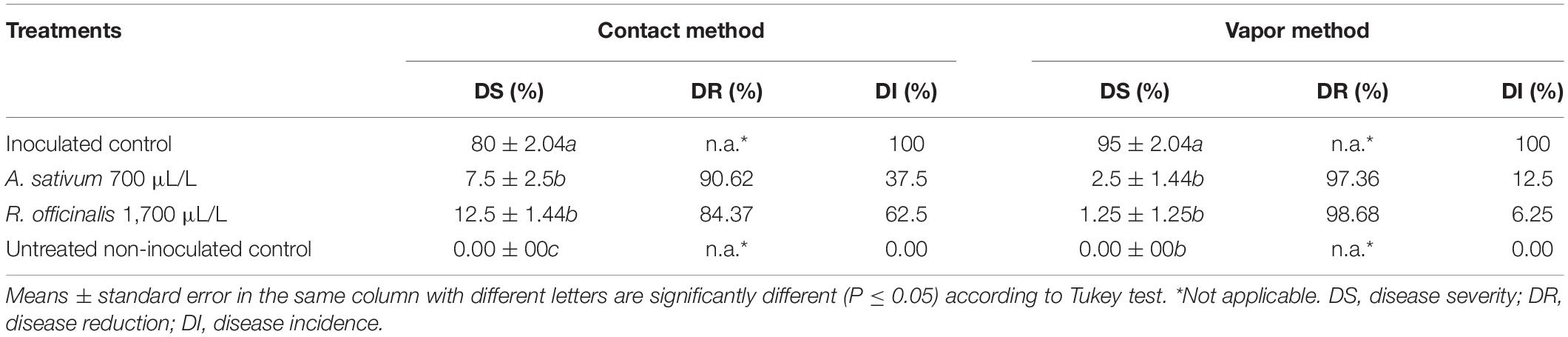
Table 4. Effect of Allium sativum L. and Rosmarinus officinalis L. essential oils in reduction of fruit decay development on strawberry fruits (cv. Paros) caused by Colletotrichum nymphaeae CCTUCCh32, 7 days after inoculation under in vivo condition.
Efficacy of EOs on Disease Severity of Strawberry Anthracnose in Greenhouse
Disease symptoms were first observed on petioles in the untreated non-inoculated treatment 35 days after inoculation under greenhouse condition. Disease severity was scored 60 days after inoculation with the pathogen, corresponding to the highest level of DS in the untreated non-inoculated control. DI and DS scoring indicates that inoculated strawberry plants treated with A. sativum and R. officinalis EOs had highly significantly (p ≤ 0.01) lower disease compared to untreated non-inoculated control (Table 5). Allium sativum EO showed the highest efficacy in DS reduction (96.15%) at the concentration of 700 μL/L followed by R. officinalis EO with 76.92% at the concentration of 1,700 μL/L compared with untreated inoculated control (Table 5). Disease severity in treatments treated with A. sativum EO was not significantly different to untreated non-inoculated control (Table 5).
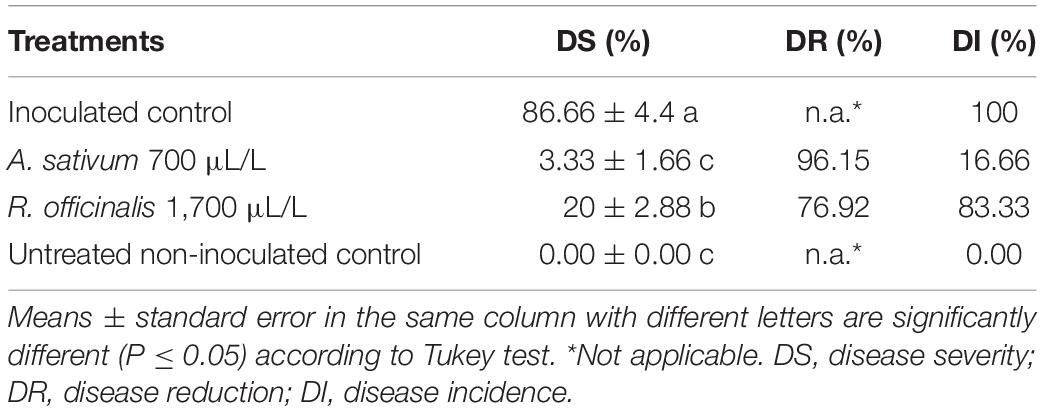
Table 5. Effect of Allium sativum L. and Rosmarinus officinalis L. essential oils on the reduction of anthracnose symptoms on strawberry plants (cv. Paros) in greenhouse conditions after 2 months of inoculation with Colletotrichum nymphaeae CCTUCCh32.
Efficacy of EOs on Fruit Quality
No difference was detected between untreated control and the fruit treated with EOs in terms of WL. The WL rate of R. officinalis and A. sativum EOs after 8 days of storage was 0.50 and 0.32%, respectively, whereas WL for untreated control was 0.41% (Table 6).
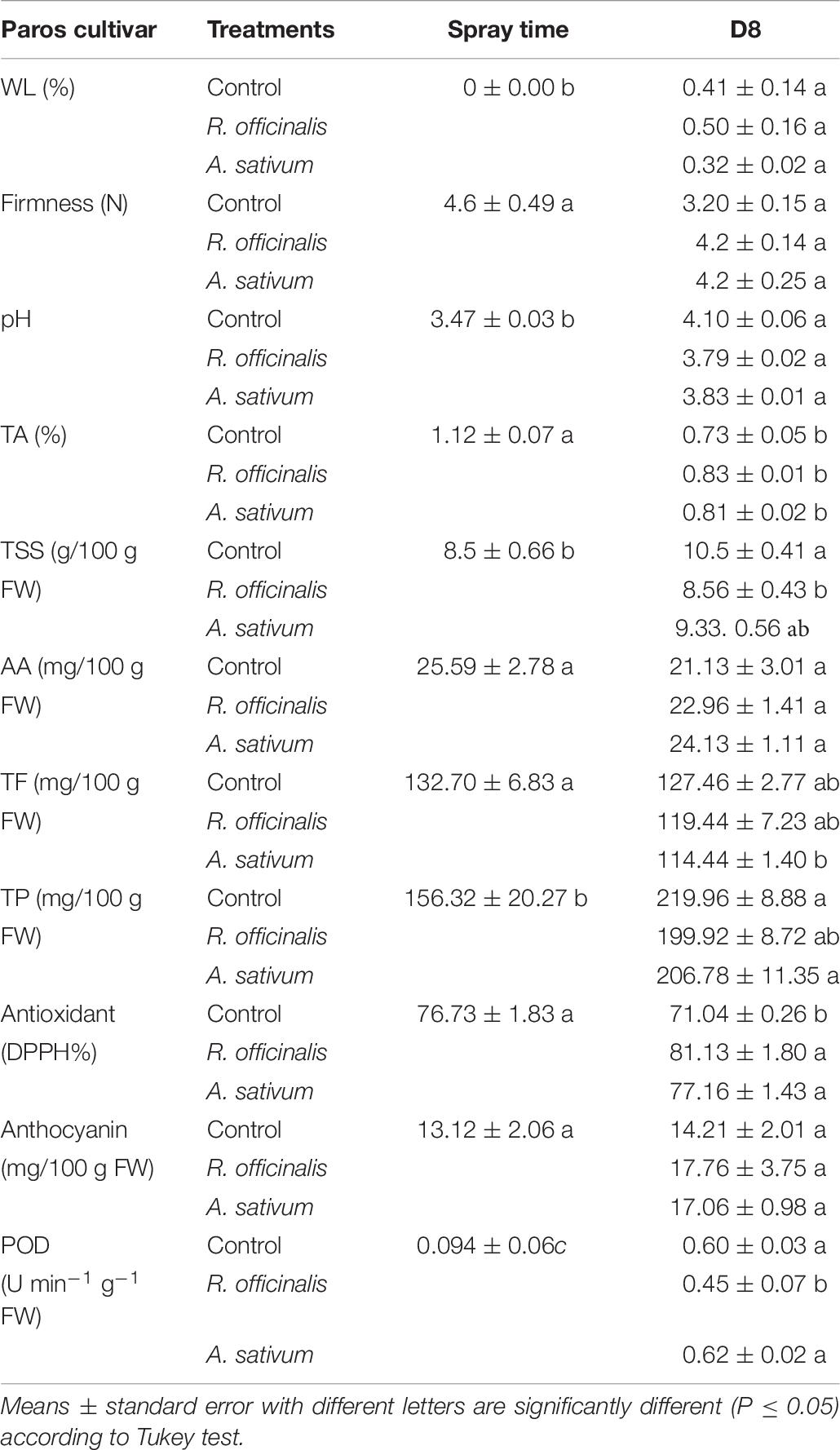
Table 6. Effect of Allium sativum L. and Rosmarinus officinalis L. essential oils at concentrations of 1,700 and 700 μL/L, respectively on strawberry fruit quality after 8 days’ storage.
Fruit firmness gradually decreased during storage, and no significant differences were observed between treatments. However, the fruits treated with both EOs showed higher firmness than untreated fruits after storage. Firmness of the fruits treated with both EOs and untreated control was 4.20 and 3.20 at the end of storage (Table 6).
During storage, the pH of strawberry fruits increased, whereas TA decreased. However, no significant difference was observed between treated and untreated fruits in terms of pH and TA (Table 6).
The TSS content in untreated fruit was 8.5% at harvest time and increased to 10.5% at the end of storage, whereas the fruits treated with R. officinalis EO significantly (p ≤ 0.05) preserved the TSS content during storage compared with untreated control (Table 6).
The AA rate was reduced in all treatments after 8 days of the storage, but there was no significant difference between the treatments. The AA content of strawberry fruits in untreated control at harvest time and end of storage was 25.59 and 21.13 mg/100 g FW, respectively (Table 6).
In this study, the TF was reduced in all treatments at the end of storage. Allium sativum EO (114 mg/100 g FW) showed the lower TF than untreated control (127.46 mg/100 g FW) at the end of storage (Table 6). The TP rate of fruits in all treatments increased during storage, but no significant differences were detected between treatments after storage. The TP of fruits in untreated control was 156.32 and 219.96 mg/100 g FW at harvest time and end of storage, respectively (Table 6). The results of the antioxidant assays indicated that DPPH values of fruits treated with A. sativum EO (77.16%) and R. officinalis (81.13%) were significantly (p ≤ 0.05) higher than untreated control (71.04%) at the end of storage (Table 6). The DPPH of the fruits in untreated control was decreased at the end of storage (Table 6).
Anthocyanin rate increased in all treatments treated with A. sativum and R. officinalis EOs during storage, but without any significant difference with untreated control. However, the anthocyanin rate of fruit treated with A. sativum EO (17.06 mg/100 g FW) and R. officinalis EO (17.76 mg/100 g FW) was higher than untreated control (14.21 mg/100 g FW) (Table 6).
The POD in all treatments increased from the beginning until the eighth day of cold storage. The POD of untreated control and the fruits treated with R. officinalis and A. sativum EOs was 0.6, 0.45, and 0.62 U min–1 g–1 FW, respectively, at the end of storage (Table 6). Our results showed that POD activity of strawberry fruits treated with of R. officinalis EO was significantly (p ≤ 0.05) less than untreated control at the end of storage.
Sensory Evaluation
The sensory analysis of the fruits treated with EOs did not reveal any adverse effect on fruit taste, flavor and overall evaluation significantly, but the odor was altered. The odor of the fruits treated with A. sativum EO was significantly (p ≤ 0.05) affected and categorized as the lowest rank based on sensory evaluation, whereas no significant difference was found between R. officinalis EO and untreated control (Figure 4).
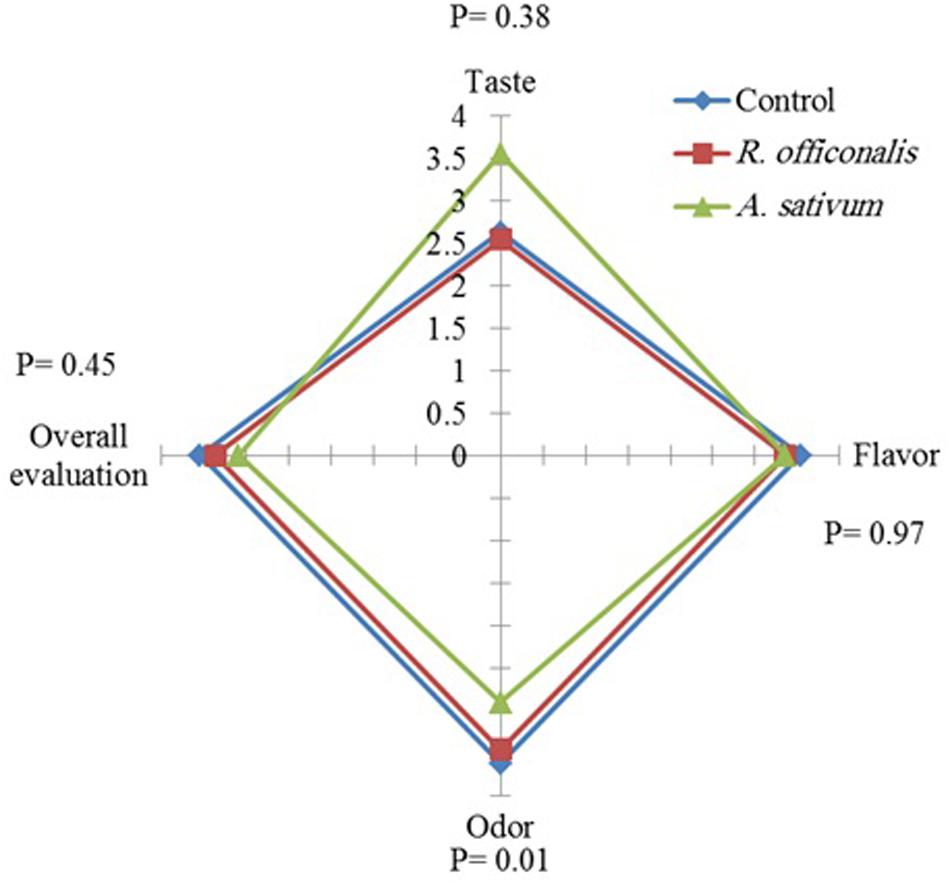
Figure 4. Influence of Allium sativum L. and Rosmarinus officinalis L. essential oils on sensory quality attributes of strawberry fruit (cv. Paros) after 8 days’ storage at 0°C.
Discussion
During the past decades, many efforts have been made to find eco-friendly alternatives, such as EOs derived from medicinal plants, to substitute synthetic chemical fungicides for the management of postharvest pathogens. Colletotrichum nymphaeae that causes strawberry anthracnose is, together with B. cinerea, among the most dangerous postharvest pathogens of strawberry (Reddy et al., 1998; Huang et al., 2011; Karimi et al., 2017; Wang N.Y. et al., 2019). The feasibility of using EOs as fungicides to control C. nymphaeae is poorly studied. Among the most studied and effective EOs, we selected A. sativum and R. officinalis EOs, and we analyzed chemical composition, antifungal activities, and their effect on the postharvest quality of strawberry fruits during storage period, with the aim of assessing the feasibility of using EOs as eco-friendly disease control measure.
We confirmed that the EO chemical composition is in line with what was already reported in literature. In fact, the analysis of A. sativum EOs revealed the sulfur-containing compounds including diallyl disulfide, allyl trisulfide, and diallyl tetrasulfide as the major compounds of A. sativum EO, and the related concentration within the range reported in previous studies (Kim et al., 2004; Corzomartinez et al., 2007; Mnayer et al., 2014; Satyal et al., 2017; Wang Y. et al., 2019). The A. sativum EO is, therefore dominated by allyl polysulfides. These compounds are well known for their antifungal, antibacterial, acaricidal, antiparasitic, nematicidal, antiviral, and insecticidal properties (Kim et al., 2004; Amini et al., 2015; Satyal et al., 2017; Wang Y. et al., 2019). On the contrary, monoterpenes and oxygenated monoterpenoids constituted the most abundant compounds of R. officinalis EO (Table 2). The abundance of monoterpenes and monoterpenoid compounds obtained from R. officinalis EO in this study was in the range reported by previous investigations (Bouchra et al., 2003; Ozcan and Chalchat, 2008; Pitarokili et al., 2008; Laborda et al., 2013; Barreto et al., 2014; da Silva Bomfim et al., 2015; Bajalan et al., 2017; Abada et al., 2020). However, the range of relative concentrations of each single component is quite variable in literature. The observed differences might be due to several factors, such as differences in place and cultivation conditions, plant species, soil type, harvest time, climatic and seasonal factors, and geographical origins (Hajlaoui et al., 2010; Rahimi-Nasrabadi et al., 2013; Bitu et al., 2015; Grulova et al., 2015; Lee et al., 2015; Alencar-Filho et al., 2017). The high variability poses an important question on how to standardize the composition of the EOs for the use as plant protection products, in particular under regulations, as in the European Union, where the consistency in the composition is a prerequisite in the registration process (EU Regulation 1107/2009). The high variability in the composition makes it also very difficult to understand which component(s) and/or their relative concentration in the mixture is responsible for the fungicide efficacy. In addition, most of the studies on the single components focus on the major ones, and the role in the antifungal activity of the minor components in the EO composition is, consequently, most of the time neglected.
Antifungal activity of R. officinalis EO and its monoterpenes compounds has been well documented against different phytopathogens including Alternaria alternata, B. cinerea, Sclerotinia sclerotiorum, Phytophthora nicotianae, Sclerotium cepivorum, Fusarium oxysporum f. sp. dianthi, and F. proliferatum (Bouchra et al., 2003; Ozcan and Chalchat, 2008; Pitarokili et al., 2008), although efficacy is noticed also against insects (i.e., Ectomyelois ceratoniae), mites (i.e., Tetranychus urticae), bacteria (i.e., Streptococcus agalactiae, Staphylococcus aureus, Escherichia coli, Klebsiella pneumoniae, Listeria monocytogenes, and Bacillus subtilis), and yeasts (i.e., Candida strains) (Laborda et al., 2013; Barreto et al., 2014; da Silva Bomfim et al., 2015; Bajalan et al., 2017; Abada et al., 2020).
As expected from the results reported in aforementioned studies, A. sativum and R. officinalis EOs could inhibit the mycelial growth of the C. nymphaeae in contact and vapor phases under in vitro conditions, although the highest inhibitory activity was observed in vapor phase compared with contact phase (Table 3). This result was also in line with the study of Soylu et al. (2010), where the effect of the EOs of Origanum syriacum, Lavandula stoechas, and R. officinalis against B. cinerea in vapor phase was higher than contact phase. It could be due to the better attachment of EO molecules to the lipophilic fungal mycelia in vapor phase, exerting antifungal effect directly on the mycelia (Ali et al., 2015). Moreover, these EOs could inhibit the conidial germination of C. nymphaeae at selected concentrations, although inhibition potential of A. sativum EO appeared at lower concentrations compared with R. officinalis EO (Figure 2). In some studies, the influence of dill, lemongrass, and cinnamon EOs on mycelial growth and conidial germination reduction of C. nymphaeae, Colletotrichum gloeosporioides, and Colletotrichum musae has been well shown (Maqbool et al., 2011; Karimi et al., 2016b). However, suppression of conidial germination is most probably related to destructive effects of EOs presented here on cell wall and permeability of plasma membrane of the conidia as observed for vegetative structures of the pathogen using SEM observations. Distinctive changes in vegetative structures of C. nymphaeae cultures exposed to both A. sativum and R. officinalis EOs were revealed as shriveled, collapsed, and swelling mycelia together with cytoplasmic coagulation (Figure 3); these observations were in line with other studies, where the mycelium of C. nymphaeae and B. cinerea exposed to dill and Syrian oregano EOs showed considerable morphological alterations in their vegetative structures compared with untreated control (Soylu et al., 2010; Karimi et al., 2016a). Morphological damage to fungal hyphae in this study might be due to leakage of ions and metabolites caused by altered membrane permeability and the breaking of fungal cell wall (Bordoh et al., 2020).
Similarly to in vitro tests, the strawberry fruits treated with both A. sativum and R. officinalis EOs in volatile phase exhibited the less DS compared with contact phase (Table 4). This result highlights the more toxicity of volatile compounds of both A. sativum and R. officinalis EOs than those that are toxic in contact phase. In an accordant study, strawberry fruits treated with Anethum graveolens EO showed the less DS caused by C. nymphaeae compared with untreated control (Karimi et al., 2016b). In other studies, the R. officinalis EOs have been shown to be effective in reducing postharvest decay of table grapes, stone, and pome fruit (Lopez-Reyes et al., 2010, 2013; Servili et al., 2017). Furthermore, our findings confirmed the results of previous studies describing the antifungal activity of A. sativum against B. cinerea and Penicillium expansum as the causal agent of fruit decay in citrus and apples (Obagwu and Korsten, 2003; Daniel et al., 2015).
Under greenhouse condition, at the tested dosages, A. sativum EO had higher efficacy in the reduction of anthracnose DI and severity than R. officinalis EO without any significant difference with untreated non-inoculated control (Table 5). This result was in line with in vivo and in vitro tests. In general and unlike in vitro assays, very few studies have been conducted under greenhouse condition to assess antifungal activity of EOs against plant pathogens (Amini et al., 2015, 2016; La Torre et al., 2016; Hosseni et al., 2020). The effect of plant EOs as volatile compounds on the surface of strawberry fruits and leaves may have induced a stressful environment, which could trigger phenolic compounds and peroxidase synthesis, creating a defense system in strawberry plant against phytopathogens (Ben-Jabeur et al., 2015). Furthermore, the phenolic compounds in the EOs have been proven to prevent the mycelial growth of Alternaria citri as the causal agent of orange black rot and reduce the severity of decay in orange as well (Ramezanian et al., 2016). Application of plant EOs against soil-borne pathogens (Amini et al., 2015, 2016; La Torre et al., 2016), foliar diseases (Hong et al., 2015; Jing et al., 2018), and postharvest diseases (Thomidis and Filotheou, 2016; Servili et al., 2017) has been studied.
In this study, A. sativum and R. officinalis EOs did not reduce water loss and delayed the qualitative changes in fruit firmness, PH, AA, TA, TP, TF, and anthocyanin, compared with untreated control (Table 6). On the contrary, a significant difference was found between the strawberry fruits treated with A. sativum and R. officinalis EOs in preservation of TSS content and increase of TAA and POD compared with untreated control (Table 6). The lower TSS content in this study indicated that EOs especially R. officinalis EO could postpone the process of strawberry fruit senescence during storage at 1°C. A similar result to Abdolahi et al. (2010), where the decrease in TSS content was attributed to reduce water loss in the fruits treated with EOs as observed in this study. Increase of TSS content during fruit storage might be occurred by the breakdown of the carbohydrates to simple sugars and degradation of polysaccharides in the fruit cell wall (Ben and Gaweda, 1985; Wills and Rigney, 2007; Ali et al., 2016) or organic acid consumption for respiration (Pelayo et al., 2003; Valero et al., 2006).
Generally, antioxidant activity in the fruits during postharvest periods may be reduced because of the degradation of cell structure, a consequence of fruit senescence (Macheix et al., 1990). In this study, the strawberry fruits treated with A. sativum and R. officinalis EOs significantly showed the higher TAA including POD compared with untreated control (Table 6). The protection of fruit cells from oxidative injury depends on the level of antioxidant enzymes (Petriccione et al., 2015). For example, antioxidant activity in plant cells can reduce membrane lipids peroxidation and protect cellular membranes of plant against plant pathogens (Franck et al., 2007). Peroxidase as one of the most important antioxidant enzymes regulates the metabolism of reactive oxygen species catalyzed by H2O2, a prerequisite for lignin synthesis to reinforce the cell wall and may also alter the antioxidant ability of fruit to cope with pathogens (Passardi et al., 2004). Both antioxidant and phenolic compounds in plant EOs have direct relationship in reduction of decay development in fruits during storage (Shen et al., 2013). It has been previously proven that phenolic compounds in plants treated with Ocimum basilicum increased the antioxidant activity and induced the defense system of plant against plant pathogens (Tzortzakis et al., 2011). Other studies indicated that EOs can induce the defense system of plants, through increasing the activity of defense-related enzymes and at least enhancing antioxidant capacity in plants (Ben-Jabeur et al., 2015; Khumalo et al., 2017).
Although a large number of studies are published on the composition of A. sativum and R. officinalis oils and their efficacy as pesticides, meta-analysis of these results is impossible because of inconsistency of methods and conditions used in the analysis (contact, vapor, concentration, time, environmental conditions at the application, etc.). Both A. sativum and R. officinalis EOs demonstrate in literature a wide spectrum of activity (insects, mites, fungi, bacteria), which can be considered an advantage in term of multiple-pest activity, but raises also the question of possible negative side effect on beneficial organisms and/or on human microbiota, which is for the two tested EOs still unknown.
Another important aspect in the practical feasibility of using EOs as alternatives to chemical pesticides is the availability of sufficient quantities at a reasonable cost for field application. The minimal inhibitory concentration or the effective concentrations found in literature and confirmed by our study indicate that they are in the order of few mg/L, which means an application dosage corresponding to several g/ha. In the case of R. officinalis EO in our study, the quantity of EO to be used per hectare is 680 mL, at a volume of application of 400 L/ha, and it corresponds to 68 kg of R. officinalis per hectare of treated strawberry field.
In current study, sensory evaluation revealed that EOs had no adverse effects on taste, odor, and flavors of fruit except those fruits, whereas their odor was affected by A. sativum EO. This result showed that apart from the positive effects of A. sativum on the reduction of the disease development, reduction or elimination of its negative effects on the fruit odor should be addressed in further studies. Sensory parameters such as taste, odor, and flavors of fruit are important factors, which can be changed by EOs and during storage time. Therefore, application of plant EOs against plant pathogens as biofungicide must not interfere with fruit sensorial quality and has to ensure their acceptability by consumers (Antunes et al., 2012; Sivakumar and Bautista-Banos, 2014; Vilaplana et al., 2018).
Conclusion
In this study, the EOs of A. sativum and R. officinalis confirmed an expected promising fungicidal activity against C. nymphaeae constituting a potential source for the development of biofungicide. Moreover, EOs preserved the quality parameters in treated strawberry fruits. TP and POD of fruits increased in the fruit treated with A. sativum and R. officinalis EOs. Reduction of fruit decay development and DS in the fruits treated with the EOs of A. sativum and R. officinalis might be associated with an increase in phenol content and the activity of defense-related enzymes such as peroxidase. In addition, results showed that EOs were capable of maintaining the sensory quality such as fruit taste, flavor, and overall evaluation (except fruit odor) during the postharvest period. These results confirmed that both A. sativum and R. officinalis EOs can be utilized as biofungicides in the protection of strawberry instead of chemical fungicides against C. nymphaeae, with the lowest negative effects on the physicochemical, qualitative, and sensory properties of fresh strawberry fruits. However, the feasibility of use as fungicide must be discussed in a wider view before reaching the conclusion of having identified a promising alternative to chemical pesticides. In particular, the availability of sufficient quantities at a reasonable cost and the absence of negative effect on the quality of the treated fruits must be carefully considered in the development of a botanical fungicide.
Data Availability Statement
The raw data supporting the conclusion of the results will be made available on request.
Ethics Statement
The studies involving human participants were reviewed and approved by Research Ethics Committee of the University of Kurdistan in Iran. All participants provided written informed consent to participate in this study. The patients/participants provided their written informed consent to participate in this study.
Author Contributions
SH performed the experiments and analyzed the data. JA and MS designed, analyzed, discussed the results, and wrote the manuscript. KK and IP discussed the results and wrote the manuscript. All authors contributed to the article and approved the submitted version.
Funding
This research received grant (No. 96/19/29435) from Plant Protection Department, the University of Kurdistan in Iran.
Conflict of Interest
The authors declare that the research was conducted in the absence of any commercial or financial relationships that could be construed as a potential conflict of interest.
Acknowledgments
We acknowledge the Analysis Laboratory of Horticultural Science Department of the University of Kurdistan in Iran.
References
Abada, M. B., Hamdi, S. H., Masseoud, C., Jroud, H., Bousshih, E., and Jemâa, J. M. B. (2020). Variations in chemotypes patterns of Tunisian Rosmarinus officinalis essential oils and applications for controlling the date moth Ectomyelois ceratoniae (Pyralidae). S. Afr. J. Bot. 128, 18–27. doi: 10.1016/j.sajb.2019.10.010
Abdolahi, A., Hassani, A., Ghosta, Y., Bernousi, I., and Meshkatalsadat, M. H. (2010). Study on the potential use of essential oils for decay control and quality preservation of Tabarzeh table grapes. J. Plant Prot. Res. 50, 45–52.
Alencar-Filho, J. M. T., Araujo, L. C., Oliveira, A. P., Guemaraes, A. L., Pacheco, A. G. M., Silva, F. S., et al. (2017). Chemical composition and antibacterial activity of essential oil from leaves of Croton heliotropiifolius in different season of the year. Rev. Bras. Farmacogn. 27, 440–444. doi: 10.1016/j.bjp.2017.02.004
Ali, A., Hei, G. K., and Keat, Y. W. (2016). Efficacy of ginger oil and extract combined with gum arabic on anthracnose and quality of papaya fruit during cold storage. J. Food Sci. Technol. 53, 1435–1444. doi: 10.1007/s13197-015-2124-5
Ali, A., Wee Pheng, T., and Mustafa, M. A. (2015). Application of lemongrass oil in vapour phase for the effective control of anthracnose of ‘S ekaki’papaya. J. Appl. Microbiol. 118, 1456–1464. doi: 10.1111/jam.12782
Amaral, L. D. P., Tondolo, J. S. M., Schindler, B., da Silva, D. T., Pinheiri, C. G., Longhi, S. J., et al. (2015). Seasonal influence on the essential oil production of Nectandra megapotamica (Spreng.) Mez. Braz. Arch. Biol. Technol. 58, 12–21. doi: 10.1590/s1516-8913201502462
Amini, J., Farhang, V., and Javadi, T. (2015). The effect of garlic essential oil plants, Mancozeb and Metalaxyl-Mancozeb on damping-off diseases caused by Phytophthora spp. Biol. Control Pests Dis. 4, 47–56.
Amini, J., Farhang, V., Javadi, T., and Nazemi, J. (2016). Antifungal effect of plant essential oils on controlling Phytophthora species. Plant Pathol. J. 32, 16–24. doi: 10.5423/ppj.oa.05.2015.0091
Antunes, M. D., Gago, C. M., Cavaco, A. M., and Miguel, M. G. (2012). Edible coating enriched with essential oils and their compounds for fresh and fresh-cut fruit. Recent. Pat. Food Nurr. Agric. 4, 114–122. doi: 10.2174/2212798411204020114
Asghari, M., Mostofi, A., Shoeibi, Y., and Fattahi, M. (2009). Effect of cumin essential oil on postharvest decay and some quality factors of strawberry. J. Med. Plants. 3, 25–43.
Bajalan, I., Rouzbahani, R., Pirbalouti, A. G., and Maggi, F. (2017). Antioxidant and antibacterial activities of the essential oils obtained from seven Iranian populations of Rosmarinus officinalis. Ind. Crops Prod. 107, 305–311. doi: 10.1016/j.indcrop.2017.05.063
Bakkali, F., Averbeck, S., Assverbeck, D., and Idaomar, M. (2008). Biological effects of essential oils-a review. Food Chem. Toxicol. 46, 446–475.
Baroncelli, R., Zapparata, A., Sarrocco, S., Sukno, S. A., Lane, C. R., Thon, M. R., et al. (2015). Molecular diversity of anthracnose pathogen populations associated with UK strawberry production suggests multiple introductions of three different Colletotrichum species. PLoS ONE 10:e0129140. doi: 10.1371/journal.pone.0129140
Barreto, H. M., Silva Filho, E. C., Lima, E. D. O., Coutinho, H. D., Morais-Braga, M. F., Tavares, C. C., et al. (2014). Chemical composition and possible use as adjuvant of the antibiotic therapy of the essential oil of Rosmarinus officinalis L. Ind. Crops Prod. 59, 290–294. doi: 10.1016/j.indcrop.2014.05.026
Ben, J., and Gaweda, M. (1985). Changes of pectic compounds in Jonathan apples under various storage conditions. Acta Physiol. Plant. 7, 45–54.
Ben-Jabeur, M., Ghabri, E., Myriam, M., and Hamada, W. (2015). Thyme essential oil as a defense inducer of tomato against gray mold and Fusarium wilt. Plant Physiol. Biochem. 94, 35–40. doi: 10.1016/j.plaphy.2015.05.006
Bi, Y., Jiang, H., Hausbeck, M. K., and Hao, J. J. (2012). Inhibitory effects of essential oils for controlling Phytophthora capsici. Plant Dis. 96, 797–803. doi: 10.1094/pdis-11-11-0933
Bitu, V. C. N., Costa, J. G. M., Rodrigues, F. F. G., Colares, A. V., Coutinho, H. D. M., Botelho, M. A., et al. (2015). Effect of collection time on composition of essential oil of Lippia gracilis Schaure (Verbenaceae) growing in Northeast Brazil. J. Essent. Oil Bear Plants 18, 674–653.
Bordoh, P. K., Ali, A., Dickinson, M., and Siddiqui, Y. (2020). Antimicrobial effect of rhizome and medicinal herb extract in controlling postharvest anthracnose of dragon fruit and their possible phytotoxicity. Sci. Hortic. 265, 109–249.
Bouchra, C., Achouri, M., Idrissi Hassani, L. M., and Hmamouchi, M. (2003). Chemical composition and antifungal activity of essential oils of seven Moroccan Labiatae against Botrytis cinerea Pers: Fr. J. Ethnopharmacol. 89, 165–169. doi: 10.1016/s0378-8741(03)00275-7
Cheng, G. W., and Breen, P. J. (1991). Activity of phenylalanine ammonia-lyase (PAL) and concentrations of anthocyanins and phenolics in developing strawberry fruit. J. Am. Soc. Hortic. Sci. 116, 865–869. doi: 10.21273/jashs.116.5.865
Corzomartinez, M., Corzo, N., and Villamiel, M. (2007). Biological properties of onions and garlic. Trends Food Sci. Technol. 18, 609–625. doi: 10.1016/j.tifs.2007.07.011
Costa, L. C. B., Pinto, J. E. B. P., Bertolucci, S. K. V., Costa, J. C. B., Alves, P. B., and Niculau, E. S. (2015). In vitro antifungal activity of Ocimum selloi essential oil and methylchavicol against phytopathogenic fungi. Cienc. Agron. 2, 428–435.
da Silva, Bomfim, N., Nakassugi, L. P., Oliveira, J. F. P., Kohiyama, C. Y., Mossini, S. A. G., et al. (2015). Antifungal activity and inhibition of fumonisin production by Rosmarinus officinalis L. essential oil in Fusarium verticillioides (Sacc.) Nirenberg. Food Chem. 166, 330–336. doi: 10.1016/j.foodchem.2014.06.019
Daferera, D. J., Ziogas, B. N., and Polissiou, M. G. (2003). The effectiveness of plant essential oils on the growth of Botrytis cinerea, Fusarium sp. and Clavibacter michiganensis subsp. michiganensis. Crop protect. 22, 39–44. doi: 10.1016/S0261-2194(02)00095-9
Daniel, C. K., Lennox, C. L., and Vries, F. A. (2015). In vivo application of garlic extracts in combination with clove oil to prevent postharvest decay caused by Botrytis cinerea, Penicillium expansum and Neofabraes alba on apples. Postharvest Biol. Technol. 99, 88–92. doi: 10.1016/j.postharvbio.2014.08.006
De Almeida, W. S., De Lima, S. G., Barreto, H. M., De Sousa Andrade, L. M., Fonseca, L., Sobrinho, C. A., et al. (2018). Chemical composition and antimicrobial activity of the essential oil of Lippia Lasiocalycina Cham. (Verbenaceae). Ind. Crops Prod. 125, 236–240. doi: 10.1016/j.indcrop.2018.09.007
Delp, B., and Milholland, R. (1980). Evaluation strawberry plants for resistance to Colletotrichum fragariae. Plant Dis. 64, 1071–1073.
Fan, F., Tao, N., Jia, L., and He, X. (2014). Use of citral incorporated in postharvest wax of citrus fruit as botanical fungicide against Penicillium digitatum. Postharvest Biol. Technol. 90, 52–55. doi: 10.1016/j.postharvbio.2013.12.005
Franck, C., Lammertyn, J., Ho, Q. T., Verboven, P., Verlinden, B., and Nicolai, B. M. (2007). Browning disorders in pear fruit. Postharvest Biol. Technol. 43, 1–13. doi: 10.1016/j.postharvbio.2006.08.008
Ghanati, F., Morita, A., and Yokota, H. (2002). Induction of suberin and increase of lignin content by excess boron in tobacco cells. Soil. Sci. Plant. Nutr. 48, 357–364. doi: 10.1080/00380768.2002.10409212
Grulova, D., De Martino, I., Mancini, E., Salamon, I., and De Feo, V. (2015). Seasonal variability of the main components in essential oil of Mentha × piperita L. J. Sci. Food Agric. 95, 621–527.
Hajlaoui, H., Snoussi, M., Noumi, E., Zanetti, S., Ksouri, R., and Bakhrouf, A. (2010). Chemical composition, antioxidant and antibacterial activities of the essential oil of five Tunisian aromatic plants. Ital. J. Food Sci. 22, 320–329.
Hong, J. K., Yang, H. J., Jung, H., Yoon, D. J., Sang, M. K., and Jeun, Y. C. (2015). Application of volatile antifungal plant essential oils for controlling pepper fruit anthracnose by Colletotrichum gloeosporioides. Plant Pathol. J. 31, 269–277. doi: 10.5423/ppj.oa.03.2015.0027
Hosseni, S., Amini, J., Rafei, J. N., and Khorshidi, J. (2020). Management of strawberry anthracnose using plant essential oils as bio-fungicides, and evaluation of their effects on quality of strawberry fruit. J. Oleo Sci. 69, 377–390. doi: 10.5650/jos.ess19119
Howard, C. M., Mass, J. L., Chandler, C. K., and Albregts, E. E. (1992). Anthracnose of strawberry caused by the Colletotrichum complex in Florida. Plant. Dis. 76, 976–981.
Huang, R., Li, G. Q., Zhang, J., Yang, L., Che, H. J., Jiang, D., et al. (2011). Control of postharvest Botrytis fruit rot of strawberry by volatile organic compound of Candida intermedia. Phytopathol. 101, 859–869. doi: 10.1094/phyto-09-10-0255
Jing, C., Zhao, J., Han, X., Huang, R., and Cai, D. (2018). Essential oil Syringa oblata Lindl. As a potential biocontrol agent against tobacco brown spot caused by Alternaria alternate. Crop Prot. 104, 41–46. doi: 10.1016/j.cropro.2017.10.002
Karimi, K., Ahari, A. B., Weisany, W., Pertot, I., Vrhovsek, U., and Arzanlou, M. (2016a). Funneliformis mosseae root colonization affects Anethum graveolens essential oil composition and its efficacy against Colletotrichum nymphaeae. Ind. Crops Prod. 90, 126–134. doi: 10.1016/j.indcrop.2016.06.024
Karimi, K., Arzanlou, M., and Pertot, I. (2016b). Antifungal activity of the dill (Anethum graveolens L.) seed essential oil against strawberry anthracnose under in vitro and in vivo conditions. Arch. Phytopathol. Plant Protect. 49, 554–566. doi: 10.1080/03235408.2016.1243999
Karimi, K., Babai Ahari, A., Arzanlou, M., Amini, J., Pertot, I., and Rota-Stabelli, O. (2017). Application of the consolidated species concept to identify the causal agent of strawberry anthracnose in Iran and initial molecular dating of the Colletotrichum acutatum species complex. Eur. J. Plant Pathol. 147, 375–387. doi: 10.1007/s10658-016-1009-4
Kevers, C., Falkowski, M., Tabart, J., Defraigne, J. O., Dommes, J., and Pincemail, J. (2007). Evolution of antioxidant capacity during storage of selected fruits and vegetables. J. Agric. Food Chem. 55, 8596–8603. doi: 10.1021/jf071736j
Khumalo, K. N., Tinyane, P., Soundy, P., Romanazzi, G., Glowacz, M., and Sivakumar, D. (2017). Effect of thyme oil vapor exposure on the brown rot infection, phenylalanine ammonia-layse (PAL) activity, phenolic content and antioxidant activity in red and yellow skin peach cultivars. Sci. Hortic. 214, 195–199. doi: 10.1016/j.scienta.2016.11.044
Kim, J. W., Kim, Y. S., and Kyung, K. H. (2004). Inhibitory activity of essential oils of garlic and onion against bacteria and yeasts. J. Food Prot. 67, 499–504. doi: 10.4315/0362-028x-67.3.499
Koushesh Saba, M., and Moradi, S. (2016). Internal browning disorder of eight pear cultivars affected by bioactive constituents and enzyme activity. Food Chem. 205, 257–263. doi: 10.1016/j.foodchem.2016.03.022
La Torre, A., Caradonia, F., Matere, A., and Battaglia, V. (2016). Using plant oils to control Fusarium wilt in tomato plants. Eur. J. Plant Pathol. 144, 487–495.
Laborda, R., Manzano, I., Gamón, M., Gavidia, I., Pérez-Bermúdez, P., and Boluda, R. (2013). Effects of Rosmarinus officinalis and Salvia officinalis essential oils on Tetranychus urticae Koch (Acari: Tetranychidae). Ind. Crops Prod. 48, 106–110. doi: 10.1016/j.indcrop.2013.04.011
Lee, J. E., Lee, B. J., Chung, J. O., Kim, H. N., Jung, S., Lee, H., et al. (2015). Metabolomic unveiling of a diverse range of green tea (Camellia sinensis) metabolites dependent on geography. Food Chem. 174, 452–459. doi: 10.1016/j.foodchem.2014.11.086
Li, Z.J., Njateng, G.S.S., He, W.J., Zhang, H.-X., Gu, J.L., Chen, S., and Du, Z.Z. (2013). Chemical composition and antimicrobial activity of the essential oil from the edible aromatic plant Aristolochia delavayi. Chem. Biodivers. 10, 2032–2041.
Lopez-Reyes, J. C., Spadaro, D., Guillino, M. I., and Garibaldi, A. (2010). Efficacy of plant essential oils on postharvest control of rot caused by fungi on four cultivar of apples in vivo. Flav. Fragr. J. 25, 171–177. doi: 10.1002/ffj.1989
Lopez-Reyes, J. C., Spadaro, D., Prelle, A., Garibaldi, A., and Gullino, M. I. (2013). Efficacy of plant essential oils on postharvest control of rots caused by fungi on different stone fruits in vivo. J. Food Prot. 76, 631–639. doi: 10.4315/0362-028x.jfp-12-342
Lu, H., Ban, Z., Wang, K., Li, D., Poverenov, E., Li, I., et al. (2017). Aroma volatiles sensory and chemical attributes of strawberry (Fragaria × ananasa Duch.) achenes and receptacle. Int. J. Food Sci. Technol. 52, 2614–2622. doi: 10.1111/ijfs.13548
Macheix, J. J., Fleuriet, A., and Bilot, J. (1990). Fruit Phenolics. Boca Raton, FL: CRC press, Inc.
Mangena, T., and Muyima, N. Y. O. (1999). Comparative evaluation of the antimicrobial activity of essential oils of Artemisia afra, Pteronia incana and Rosmarinus officinalis on selected bacteria and yeast strains. Lett. Appl. Microbiol. 28, 291–296. doi: 10.1046/j.1365-2672.1999.00525.x
Maqbool, M., Ali, A., Alderson, P. G., Mohamed, M. T. M., Siddiqui, Y., and Zahid, N. (2011). Postharvest application of gum arabic and essential oils for controlling anthracnose and quality of banana and papaya during cold storage. Postharvest Biol. Technol. 62, 71–76. doi: 10.1016/j.postharvbio.2011.04.002
Medeiros, R. T. S., Goncalez, E., Felicio, R. C., and Felicio, J. D. (2011). Evaluation of antifungal activity of Pittosporum undulatum L. Essential oil against Aspergillus flavus and aflatoxin production. Cienc. Agrotec. 35, 71–76. doi: 10.1590/s1413-70542011000100008
Meier, U. (2001). Growth Stages of Mono- and Dicotyledonous Plants. Berlin: Federal Biological Research Centre for Agriculture and Forestry.
Mnayer, D., Fabiano-Tixier, A.-S., Petitcolas, E., Hamieh, T., Nehme, N., Ferrant, C., et al. (2014). Chemical composition, antibacterial and antioxidant activities of six essentials oils from the Alliaceae family. Molecules 19, 20034–20053. doi: 10.3390/molecules191220034
Nabigo, A., and Morshedi, H. (2011). Evaluation of the antifungal activity of the Iranian thyme essential oils on the postharvest pathogens of strawberry fruits. Afr. J. Biotech. 10, 9864–9869. doi: 10.5897/ajb10.2018
Nakatsu, T., Lupo, A. T. Jr., Chinn, J. W. Jr., and Kang, R. K. (2000). Biological activity of essential oils and their constituents. Stud. Nat. Prod. Chem. 21, 571–631. doi: 10.1016/s1572-5995(00)80014-9
Nutter, F. W., Esker, P. D., and Netto, R. A. C. (2006). Disease assessment concepts and the advancements made in improving the accuracy and precision of plant disease data. Eur. J. Plant Pathol. 115, 95–103. doi: 10.1007/1-4020-5020-8_7
Obagwu, J., and Korsten, L. (2003). Control of citrus green and blue mould with garlic extracts. Eur. J. Plant Pathol. 109, 221–225.
Ozcan, M. M., and Chalchat, J. C. (2008). Chemical composition and antifungal activity of rosemary (Rosmarinus officinalis L.) oil from Turkey. Int. J. Food Sci. Nutr. 59, 691–698. doi: 10.1080/09637480701777944
Ozcan, M. M., Chalchat, J.-C., Arslan, D., Ateş, A., and Unever, A. (2006). Comparative essential oil composition and antifungal effect of bitter fennel (Foenichlum vulgare ssp. piperitum) fruit oils obtained during different vegetation. J. Med. Food. 9, 552–561. doi: 10.1089/jmf.2006.9.552
Passardi, F., Penel, C., and Dunand, C. (2004). Performing the paradoxical: how plant peroxidase modify the cell wall. Trends. Plant Sci. 9, 534–540. doi: 10.1016/j.tplants.2004.09.002
Patras, A., Brunton, N. P., Da Pieve, S., and Butler, F. (2009). Impact of high pressure processing on total antioxidant activity, phenolic, ascorbic acid, anthocyanin content and color of strawberry and blackberry purées. Innov. Food Sci. Emerg. Technol. 10, 308–313. doi: 10.1016/j.ifset.2008.12.004
Pelayo, C., Ebeler, S. E., and Kader, A. A. (2003). Postharvest life and flavor quality of three strawberry cultivars Kept at 5°C in air or air +20 kPa CO2. Postharvest Biol. Technol. 27, 171–183. doi: 10.1016/s0925-5214(02)00059-5
Peres, N. A., Timmer, L. W., Adaskaveg, J. E., and Correll, J. E. (2005). Lifestyle of Colletotrichum acutatum. Plant Dis. 89, 784–796.
Petriccione, M., Mastrobuoni, F., Pasquariello, M. S., Zampella, L., Nobis, E., Capriolo, G., et al. (2015). Effect of chitosan coating on the postharvest quality and antioxidant enzyme system response of strawberry fruit during cold storage. Foods 4, 501–523.
Pimental, R. B. Q., Souza, D. P., Albuquerque, P. M., Fernandes, A. V., Santos, A. S., Duvoisin, S., et al. (2018). Variability and antifungal activity of volatile compounds from Aniba rosaeodora Ducke, harvested from Central Amazonia in two different seasons. Ind. Crops Prod. 123, 1–9.
Pitarokili, D., Tzakou, O., and Loukis, A. (2008). Composition of the essential oil of spontaneous Rosmarinus officinalis from Greece and antifungal activity against phytopathogenic fungi. J. Essent. Oil Res. 20, 457–459.
Prakash, B., Kedia, A., Mishra, P. K., and Dubey, N. K. (2015). Plant essential oils as food preservative to control moulds, mycotoxin contamination and oxidative deterioration of agri-food commodities – potentials and challenges. Food Control. 47, 381–391.
Rahimi-Nasrabadi, M., Nazarian, S. H., Farahani, H., Fallah- Koohbijari, G. R., Ahmadi, F., and Batooli, H. (2013). Chemical composition, antioxidant, and antibacterial activities of the essential oil methanol extracts of Eucalyptus largiflorens. Int. J. Food Proper. 16, 369–381.
Ramezanian, A., Azadi, M., Mostowizadeh-Ghalamfarsa, R., and Saharkhiz, M. J. (2016). Effect of Zataria multiflora Bioss and Thymus vulgaris L. essential oils on black rot of Washington Navel orange fruit. Postharvest Biol. Technol. 112, 152–158.
Reddy, M. V. B., Angers, P., Andre, G., and Arul, J. (1998). Characterization and use of essential oil from Thymus vulgaris against Botrytis cinerea and Rhizopus stolonifer in strawberry fruits. Phytochemistry 47, 1515–1520.
Satyal, P., Craft, J. D., Dosoky, N. S., and Setzer, W. (2017). The chemical compositions of the volatile oils of garlic (Allium sativum) and wild garlic (Allium vineale). Food 6, 1–10.
Sellamuthu, P. S., Sivakumar, D., Soundy, P., and Korsten, L. (2013). Essential oil vapors suppress the development of anthracnose and enhance defense related and antioxidant enzyme activity in avocado fruit. Postharvest Biol. Technol. 81, 66–72.
Servili, A., Feliziani, E., and Romanazzi, G. (2017). Exposure to volatiles of essential oils alone or under hypobaric treatment to control postharvest gray mold of table grapes. Postharvest Biol. Technol. 133, 36–40.
Shao, X., Cao, B., Xu, F., Xie, S., Yu, D., and Wang, H. (2015). Effect of postharvest application of chitosan combined with clove oil against citrus green mold. Postharvest Biol. Technol. 99, 37–43.
Shao, X., Wang, H., Xu, F., and Cheng, S. (2013). Effects and possible mechanisms of tea tree oil vapor treatment on the main disease in postharvest strawberry fruit. Postharvest Biol. Technol. 77, 94–101.
Shen, Y., Yang, H., Chen, J., Liu, D., and Ye, X. (2013). Effect of waxing and wrapping on phenolic content and antioxidant activity of citrus during storage. J. Food Process Preserve. 37, 222–231.
Singleton, V. L., Orthofer, R., and Lamuela-Raventos, R. M. (1999). Analysis of total phenols and other oxidation substrates and antioxidants by means of folin-ciocalteu reagent. Methods Enzymol. 299, 152–178.
Sivakumar, D., and Bautista-Banos, S. (2014). A review on the use of essential oils for postharvest decay control and maintenance of fruit quality during storage. Crop Prot. 64, 27–23.
Smith, B. J., and Black, L. L. (1992). In vitro activity of fungicides against Colletotrichum fragariae. Acta Hortic. 348, 509–512.
Sogvar, O. B., Saba, M. K., and Emamifar, A. (2016). Aloe vera and ascorbic acid coatings maintain postharvest quality and reduce microbial load of strawberry fruit. Postharvest Biol. Technol. 114, 29–35.
Soylu, E. M., Kurt, S., and Soylu, S. (2010). In vitro and In vivo antifungal activities of the essential oils of various plants against tomato grey mould disease agent Botrytis cinerea. Int. J. Food Microbiol. 143, 183–189.
Soylu, E. M., Soylu, S., and Kurt, S. (2006). Antimicrobial activity of the essential oils of various plants against tomato late blight disease agent Phytophthora infestans. Mycopathologia 161, 119–128.
Thomidis, T., and Filotheou, A. (2016). Evaluation of five essential oils as bio-fungicides on the control of Pilidiella granati rot in pomegranate. CropPro 89, 66–71.
Tzortzakis, N. G., Tzanakaki, K., and Economaki, C. D. (2011). Effect of origanum oil and vinegar on the maintenance of postharvest quality of tomato. Food. Nutr. Sci. 2, 974–982.
Valero, D., Valverde, J., Martinez-Romero, D., Guillen, F., Castillo, S., and Serrano, M. (2006). The combination of modified atmosphere packaging with eugenol or thymol to maintain quality, safety and functional properties of table grapes. Postharvest Biol. Technol. 41, 317–327.
Vilaplana, R., Pazmino, L., and Valencia-Chamorro, S. (2018). Control of anthracnose, caused by Colletotrichum musae, on postharvest organic banana by thyme oil. Postharvest Biol. Technol. 138, 56–63.
Wang, N. Y., Forcelini, B. B., and Peres, N. A. (2019). Anthracnose fruit and root necrosis of strawberry are caused by a dominant species within the Colletotrichum acutatum species complex in the United States. Phytopathology 109, 1293–1301.
Wang, Y., Wei, K., Han, X., Zhao, D., Zheng, Y., Chao, J., et al. (2019). The antifungal effect of garlic essential oil on Phytophthora nicotianae and the inhibitory component Involved. Biomolecules 9:632.
Wedge, D. E., Smith, B. J., Quebedeaux, J. P., and Constantin, R. J. (2007). Fungicide management strategies for control of strawberry fruit rot diseases in Louisiana and Mississippi. Crop Prot. 26, 1449–1458.
Wills, R., and Rigney, C. (2007). Effect of calcium on activity of mitochondria and peptic enzymes isolated from tomato fruits. J. Food. Biochem. 3, 103–110.
Zamani-Zadeh, M., Soleimanian-Zad, S., and Sheikh-Zeinoddin, M. (2013). Biocontrol of gray mold disease on strawberry fruit by integration of Lactobacillius plantarum A7 with ajwain and cinnamon essential oils. J. Food Sci. 78, 1582–1588.
Keywords: biofungicide, black spot, Fragaria × ananassa Duch, fruit quality parameters, volatile oils
Citation: Hosseini S, Amini J, Saba MK, Karimi K and Pertot I (2020) Preharvest and Postharvest Application of Garlic and Rosemary Essential Oils for Controlling Anthracnose and Quality Assessment of Strawberry Fruit During Cold Storage. Front. Microbiol. 11:1855. doi: 10.3389/fmicb.2020.01855
Received: 26 April 2020; Accepted: 15 July 2020;
Published: 14 August 2020.
Edited by:
Laurent Dufossé, Université de la Réunion, FranceReviewed by:
Yasmeen Siddiqui, Putra Malaysia University, MalaysiaAsgar Ali, University of Nottingham Malaysia Campus, Malaysia
Copyright © 2020 Hosseini, Amini, Saba, Karimi and Pertot. This is an open-access article distributed under the terms of the Creative Commons Attribution License (CC BY). The use, distribution or reproduction in other forums is permitted, provided the original author(s) and the copyright owner(s) are credited and that the original publication in this journal is cited, in accordance with accepted academic practice. No use, distribution or reproduction is permitted which does not comply with these terms.
*Correspondence: Jahanshir Amini, amFtaW5pQHVvay5hYy5pcg==; YW1pbmlqYWhhbjUxQHlhaG9vLmNvbQ==