- 1Institute of Preventive Veterinary Medicine, Sichuan Agricultural University, Chengdu, China
- 2Key Laboratory of Animal Disease and Human Health of Sichuan Province, Sichuan Agricultural University, Chengdu, China
- 3Avian Disease Research Center, College of Veterinary Medicine, Sichuan Agricultural University, Chengdu, China
The protein encoded by the UL48 gene of alphaherpesviruses is named VP16 or alpha-gene-transactivating factor (α-TIF). In the early stage of viral replication, VP16 is an important transactivator that can activate the transcription of viral immediate-early genes, and in the late stage of viral replication, VP16, as a tegument, is involved in viral assembly. This review will explain the mechanism of VP16 acting as α-TIF to activate the transcription of viral immediate-early genes, its role in the transition from viral latency to reactivation, and its effects on viral assembly and maturation. In addition, this review also provides new insights for further research on the life cycle of alphaherpesviruses and the role of VP16 in the viral life cycle.
Introduction
The Herpesviridae family is classified into three subfamilies, alphaherpesvirinae, betaherpesvirinae, and gammaherpesvirinae. For all herpesviruses, a complete virion consists of four parts: a core that contains a double-stranded DNA genome, a capsid, a tegument, and an envelope (Guiping et al., 2007; Boštíková et al., 2014). The alphaherpesvirinae subfamily includes herpes simplex virus type-1/2 (HSV-1/2), pseudorabies virus (PRV), duck enteritis virus (DEV) (Qi et al., 2008; Zhao et al., 2008; Chang et al., 2009; Guo et al., 2009; Jia et al., 2009), varicella-zoster virus (VZV), equine herpesviruses (EHV), bovine herpesvirus (BHV), canine herpesvirus (CHV), and Marek’s disease virus (MDV) (Kobty, 2015; Smith, 2017; You et al., 2017; He et al., 2018; Lefkowitz et al., 2018).
The UL48 gene is conserved in most alphaherpesviruses, encoding the late tegument protein VP16, also known as alpha-gene-transactivating factor (α-TIF), but is not conserved in betaherpesvirinae and gammaherpesvirinae (Zhang et al., 2016). In the early stage of viral infection, VP16 released by invading virions binds to the immediate-early (IE) gene promoter to stimulate the transcription of IE genes as a transactivating factor that acts specifically on IE genes. In the late stage, VP16 assembles into the tegument to participate in the assembly of virions and promote their maturation (Jonker et al., 2005b; Zhang et al., 2016). In recent years, research on VP16 has found that its function is powerful and involves complex regulatory networks (Zhang et al., 2016; Sevin-Pujol et al., 2017). This article will explain the role of VP16 in promoting IE gene transcription, as well as its role in viral assembly and how VP16 functions when the virus reactivates from latency, providing new insights into the maturation of alphaherpesviruses and the role of VP16 in the viral life cycle.
The Life Cycle of Alphaherpesviruses
During herpesvirus infection, the virions adsorb to the plasma membrane through interactions between envelope glycoproteins and host cell-specific receptors, and the virions become engulfed in phagocytic vesicles, which derived from invaginating plasmalemma and enter host cells (Mettenleiter et al., 2006; Zhang et al., 2011). After entering the cell, the capsid and tegument gradually loosen and disintegrate, and the nucleocapsids are transported to the nuclear pore, releasing the viral DNA to the nucleus. Next, the DNA genome is circularized and replicated, and viral capsid proteins synthesized in the cytoplasm enter the nucleus to form capsids. Then, the viral genome is cleaved and packaged into the capsids to form nucleocapsids (Dai et al., 2013; Homa and Brown, 2015).
Nucleocapsids are translocated into the cytoplasm via a process of primary envelopment–deenvelopment: nucleocapsids bud into the inner nuclear membrane to obtain the primary envelope and enter the perinuclear space, then the primary envelope fuses with the outer nuclear membrane to deenvelop, and unenveloped capsids are released to the cytoplasm. Then, nucleocapsids can bind in an orderly manner to tegument proteins (mainly inner tegument proteins) and be transported through microtubules to trans-Golgi-derived vesicles that combine viral glycoproteins with outer tegument proteins, budding into vesicles via secondary envelopment to form complete virions. Eventually, the vesicles carry fully assembled virions to the plasma membrane for egress via the exocytosis pathway (Guo et al., 2010; Crump, 2018; Yang et al., 2019).
VP16 plays a role in mainly two phases of the viral life cycle. First, VP16 is a transcriptional activator that regulates viral gene transcription. Second, VP16 is a late tegument protein that further participates in the assembly and maturation of nucleocapsids in the cytoplasm.
VP16 Is a Transcriptional Activator of IE Genes
After alphaherpesvirus infects target cells, the viral genome that enters the nucleus is transcribed in a specific order, IE genes, then early (E) genes, and finally late (L) genes (Nishiyama, 2006; Liu et al., 2015), and this cascade of transcription is precisely initiated by VP16 (Dalrymple et al., 1985; Lu and Misra, 2000). Once the host cell is infected, VP16 is released by the virions and together with two cell factors, HCF-1 and Oct-1, to form a transcriptional regulatory complex through its conserved DNA-binding domain (DBD), also named the VP16-induced complex-forming domain (VIC), which binds to the promoter of IE genes stably. Then, through its unconserved transcriptional activation domain (TAD), VP16 can recruit numerous transcription factors to activate the transcription of IE genes (Laboissière et al., 1997; Simmen et al., 1997) (Figure 1).
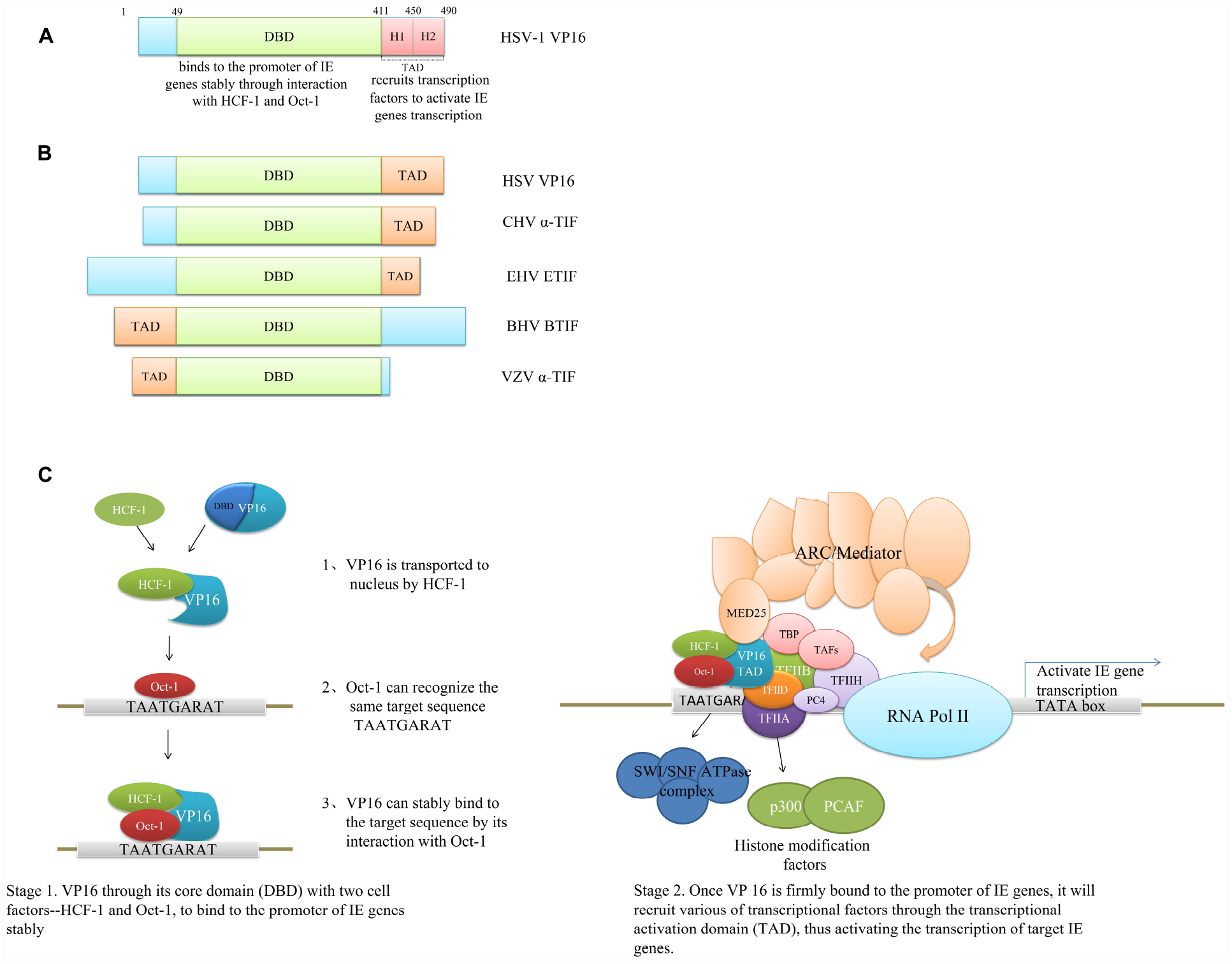
Figure 1. Domain structure and mechanism of VP16 to promote IE gene transcription. (A) Domain structure of HSV-1 VP16. The DBD can bind to the promoter of IE genes stably through interaction with HCF-1 and Oct-1, and the TAD of HSV VP16 can be divided into two regions, H1 and H2, which can bind to different transcriptional activators and also synergistically interact with some important transcriptional factors (Ikeda et al., 2002; Hirai et al., 2010). (B) The schematic of various VP16 homologs with their functional domains. The DBD is conserved in VP16 homologs, but the TADs of VP16 homologs are not conserved in position, sequence, or amino acid properties. The VP16 TAD of HSV and EHV is located at the C-terminus, but in BHV and VZV, the VP16 TAD is located at the N-terminus (Cohen and Seidel, 1994; Wysocka and Herr, 2003; Tyack et al., 2006). (C) Mechanism of VP16 to promote IE gene transcription. The DNA-binding ability of VP16 itself is weak, and the binding is unstable; VP16 mainly combines with HCF-1 and Oct-1 to form a complex through the unstructured region in its DBD, so as to bind stably to the specific site of the target gene promoter and then recruit transcriptional factors through the TAD to promote the transcription of the target gene. The formation of the complex by VP16, HCF-1, and Oct-1 is the most effective combination by VP16 dominated to activate viral gene cascade expression. The VP16-induced complex represents a regulatory switch for two modes of viral infection: lytic infection and latent infection. When it is “on,” it promotes the transcription of IE genes and thus lytic infection, and when it is “off,” limiting IE gene transcription, viruses can maintain established latent infections (Wysocka and Herr, 2003; Hirai et al., 2010; Milbradt et al., 2011).
The Formation of the VIC-Induced Transcriptional Regulatory Complex
The HSV VP16 VIC is located at residues 49–412, and the VIC contains both structural and non-structural regions (Liu et al., 1999). The structural region resembles a “seat-like” structure. A two-stranded antiparallel coiled coil formed by two long α-helixes is connected by a short six-amino-acid loop forming the back of the seat, two V-shaped spirals form the surface, and the seat bottom contains all of the strands (β1–β6) that form two β sheets: a two-stranded parallel β sheet (strands β5 and β2) and a four-stranded mixed antiparallel β sheet (strands β4, β3, β1, and β6) (Liu et al., 1999; Jonker et al., 2005a; Tyack et al., 2006). The seat surface can recognize and bind to the specific motif TAATGARAT on the IE gene promoter. Although VP16 can directly interact with the target sequence through the structured region, VP16 itself has weak DNA-binding ability; instead, it binds stably to DNA through the non-structural region (Lai and Herr, 1997; Babb et al., 2001; Tyack et al., 2006). The non-structural region is located mainly at residues 350–394 and 403–412, showing an irregular conformation. The residues 361–365 contain a specific motif (D/E)HXY(S/A) that can bind to HCF-1, and binding to Oct-1 is at mainly residues 373–378, but the formation of the transcriptional complex is also sensitive to changes in other parts of the non-structural region (Lai and Herr, 1997; Lu et al., 1998; Vogel and Kristie, 2013). VP16 itself does not possess a nuclear localization signal; it is transported to the nucleus by HCF-1 and then binds to Oct-1, which can also recognize the same target sequence. After binding, the irregular conformation of the non-structural region transforms into a stable and orderly structure, making VP16 firmly bind to the promoter of IE genes (Herr, 1998; Carroll et al., 2007; Liu et al., 2017) (Figure 1).
Serine at position 375 (Ser375) in VP16, also in the Oct-1-binding domain, resides in a CK2 site (S/TxxE/D) and can be phosphorylated by CK2. Both the CK2 site and Ser/Thr at position 375 are highly conserved among VP16 homologs (O’Reilly et al., 1997; Ottosen et al., 2006). Phosphorylation of Ser375 promotes the binding of VP16 to Oct-1, and replacing Ser375 with Thr (also phosphorylatable by CK2) but not Ala still results in Oct-1 binding to preserve the transcriptional activation by VP16. Other phosphorylation sites found in VP16 are Ser18, Ser353, and Ser452, and all of these sites are consistent with the target sequence for phosphorylation by kinases in the JNK families, but these sites are far less effective than Ser375 in VP16 transcriptional activation (Ottosen et al., 2006; Sawtell et al., 2011). This reflects the importance of the formation of a complex by VP16 and Oct-1 for transcriptional activation.
The Formation of a VIC-Induced Transcriptional Regulatory Complex
VP16 effectively binds to HCF-1 and Oct-1 through the kelch domain of HCF-1 and the POU domain of Oct-1 (Herr and Cleary, 1995; Luciano and Wilson, 2002). The kelch domain of HCF-1 is composed of six kelch repeats, and four-stranded β sheets formed by these repeats assemble into a six-bladed propeller-like structure, which can bind VP16 and maintain the stability of the VIC-induced complex (Wilson et al., 1997; Luciano and Wilson, 2002). The POU domain of Oct-1 consists of two independent DNA-binding units, the POU-specific (POUS) domain and the POU-homeo (POUH) domain, which are connected by an unstructured linker (Herr and Cleary, 1995), and VP16 is connected only to the POUH domain (Chasman et al., 1999; Phillips and Luisi, 2000). The unstructured linker makes the combination of the Oct-1 POU subdomain with the DNA sequence very flexible, thus ensuring diversity of the DNA sequence recognition by Oct-1, which is why even IE-gene promoters of other alphaherpesviruses are not exactly TAATGARAT sequence but rather similar sequences, such as the TAATGAGCT motif in the BHV IE-1 promoter and the repeated TAATTACAC motifs in the CHV ICP4 promoter; Oct-1 can still bind to VP16 to form a VIC-induced complex on these promoters (Herr and Cleary, 1995; Phillips and Luisi, 2000; Wysocka and Herr, 2003; Tyack et al., 2006).
Forming the VIC-Induced Complex Is the Specific Combination to Activate IE Gene Transcription
The formation of the VIC-induced complex by VP16, HCF-1, and Oct-1 is the most effective combination of VP16 to activate the viral gene expression. In addition to HCF-1, the HCF family includes HCF-2, which can also stabilize the complex induced by VP16, but only the HCF-1-containing complex can effectively activate transcription (Johnson et al., 1999; Luciano and Wilson, 2002). In addition to the kelch domain, HCF-1 carboxy-terminal acidic region also contains a TAD, which cooperates with the activation domain of VP16 to activate transcription (Lee and Herr, 2001; Gudkova et al., 2019). Similarly, in addition to Oct-1, there are other proteins containing a POU domain. For example, in Oct-2, the protein most similar to Oct-1, only one amino acid is changed compared to Oct-1. Although they can recognize the same DNA sequence, Oct-2 cannot bind to VP16 (Cleary et al., 1993; Bentrari et al., 2015). The unique combination of VP16 with HCF-1 and Oct-1 provides evidence to explain why HSV can establish latent infection in sensory neurons: (1) as a cell proliferating factor, HCF-1 will change its status in non-proliferating cells to adapt to or even promote the cessation of cell proliferation. Moreover, HCF-1 exists in the cytoplasm of sensory neurons and is thus perhaps unable to transport VP16 to the nucleus and to play a role in transcription activation (Reilly and Herr, 2002; Wysocka and Herr, 2003). (2) There are many POU-domain proteins similar to Oct-1 in the nervous system, such as Oct-2, brn-1, and brn-2, that can effectively bind to the TAATGARAT site though they cannot interact with VP16 and may bind more tightly than Oct-1, thus perhaps inhibiting VP16-induced complex formation on the promoter and preventing gene transcription (Andersen and Rosenfeld, 2001; Wysocka and Herr, 2003). As a result, the VIC-induced complex represents a regulatory switch for two modes of viral infection: lytic infection and latent infection. When it is “on,” it promotes the transcription of IE genes and thus lytic infection, and when it is “off,” limiting IE gene transcription, viruses can maintain established latent infections (Wysocka and Herr, 2003; Thompson et al., 2009; Zhang et al., 2016).
VP16 Activates IE Gene Transcription via Its TAD
The VP16 TAD Recruits Different Types of Transcription Factors
Once VP 16 is firmly bound to the promoter of IE genes, it will recruit transcription factors through its TAD, thus activating the transcription of target genes (Hall and Struhl, 2002) (Figure 1).
The VP16 TAD can interact with many different types of transcription factors. (1) VP16 can directly bind to common transcription factors, such as TFIIB, TFIIH, TATA-binding protein (TBP), and TBP-related factors (TAFs) (Goodrich et al., 1993; Klemm et al., 1995; Hori et al., 2004; Langlois et al., 2008). The ternary complex formed by VP16, TFIIA, and TFIID is the initiation complex of RNA polymerase II, which promotes the recruitment of RNA polymerase II and the correct initiation location (Kobayashi et al., 1995; Hori et al., 2004). (2) VP16 can also interact with the activator-recruited cofactor (ARC)/mediator coactivator complex, promoting the binding of additional transcription factors and RNA polymerase II to the target gene promoter (Aguilar et al., 2014). There are more than 30 known subunits that make up the ARC/mediator coactivator complex (Harper and Taatjes, 2018). VP16 binds to the coactivator complex by mainly interacting with MED25 (also named ARC92 or ACID1) or MED17 (Aguilar et al., 2014; Lee et al., 2018). (3) VP16 can also recruit chromatin modification factors and promote chromatin remodeling, which is divided into mainly two types. The first are histone modification factors, such as the histone acetyltransferases p300/CBP and PCAF, histone methyltransferase SUVH39H1 (methylation of lysine 9 on histone H3), and histone demethylase JMJD2A (demethylation of lysines 9 and 36 on histone H3) (Wang et al., 2000; Kutluay et al., 2009). Through covalent modification of histones, such as acetylation, methylation, and ubiquitination, the deposition of histones on promoters is prevented, and the structure of chromatin becomes loose, which facilitates the binding of transcription regulators with cis-acting elements and the sliding of RNA polymerase on transcription templates to promote gene transcription; histone acetylation is a symbol of transcriptional activation (Cantin et al., 2003; Herrera and Triezenberg, 2004). The other type is ATP-dependent chromatin remodeling complexes, such as the SWI/SNF ATPase complex, which makes nucleosomes slide along DNA through ATP hydrolysis and disrupts the order of nucleosomes, participating in DNA double-strand fracture repair and nucleotide removal repair and promoting transcriptional extension (Cantin et al., 2003; Wu et al., 2017). In the absence of binding partners, TAD does not have a specific three-dimensional structure, and when bound to interacting transcriptional factors, the TAD will undergo conformational transformation from irregular crimping to an α-helix, providing a strong electrostatic driving force to enhance binding to transcriptional factors (Jonker et al., 2005a; Langlois et al., 2008). Although binding to some basic transcription factors, histone acetyltransferases, and the SWI/SNF complex is a common feature of transcriptional activators, the TAD of VP16 is highly efficient and widely used. After replacing the TAD of the host transcriptional activator with the TAD of VP16, the transcriptional activation ability of the activator was significantly improved, which provides a new method for improving the activation efficiency of inefficient transcriptional activators (Langlois et al., 2008; Sevin-Pujol et al., 2017; Mittal et al., 2018; Wang et al., 2019).
Structural Characteristics of Different TADs of VP16
In alphaherpesviruses, the TADs of VP16 homologs are not conserved in position, sequence, or amino acid properties, but the TADs of transcriptional activators have a general feature that is a short sequence pattern with no positive charge but with redundant negative charge residues and aromatic residues (Tyack et al., 2006; Ravarani et al., 2018). The TAD of HSV-1 VP16 is composed of 80 amino acids at the C-terminus, two hydrophobic amino acid clusters, and adjacent acidic residues (Regier et al., 1993), and it is a highly acidic sequence in which hydrophobic and aromatic residues are critical for transcriptional activation, such as phenylalanine at sites 442, 473, 475, and 479. The TAD of HSV-1 VP16 can be divided into two regions: H1 (amino acids at residues 411–456) and H2 (amino acids at residues 450–490), which can bind to different transcriptional activators and synergistically interact with some important transcriptional factors (Regier et al., 1993; Sullivan et al., 1998). For example, effective binding of the major transcriptional factor TFIIB requires the joint participation of H1 and H2 (Walker et al., 1993), and interaction with MED25 depends mainly on H1 (Aguilar et al., 2014), while the effective connection with TFIIA, TFIID, and TFIIH occurs through H2 (Johnson and Carey, 2003; Fukuda et al., 2004). In EHV-1, the TAD of ETIF (a homolog of VP16) is only a small segment of the C-terminus, especially the last 7 amino acids, which are necessary to activate the transcription of IE genes. The ETIF TAD is essentially the same as the core of H2 (H2. CCR) of the HSV VP16 TAD. However, the HSV VP16 TAD does not affect the binding of the VP16 core domain to HCF-1 and Oct-1 to form the transcriptional regulatory complex, while ETIF relies only on the conserved core domain and cannot bind to HCF-1 and Oct-1. The absence of the ETIF TAD could destroy the conformation of the core domain and inhibit the formation of the transcriptional regulatory complex (Grapes and O’Hare, 2000). Methionine residues at the C-terminus are important for transcriptional activation by ETIF, and they are also found at the C-terminus of HSV VP16 and CHV VP16, but not in BTIF (the homolog of VP16) (Grapes and O’Hare, 2000; Tyack et al., 2006).
In contrast, the TAD of BTIF is located at the N-terminus, and when the N-terminus of HSV VP16 is replaced with the N-terminus of BTIF, it results in a recombinant chimeric protein with higher transcriptional activation activity than any α-TIF (Misra et al., 1994, 1995; Grapes and O’Hare, 2000). Similar to BTIF, the α-TIF of VZV, a product encoded by the ORF10 gene, also lacks the acidic C-terminus of VP16, whose TAD is located at the N-terminus (Cohen and Seidel, 1994) (Figure 1). These results indicate that although the VP16 homologs have a relatively conserved core domain, the N-terminal and C-terminal domains are very different, and the location and structure of the TAD of VP16 homologs are different. Even though the TAD has a similar structure, such as the ETIF TAD being a small part of the HSV VP16 TAD, it plays a very different role. Core domain conservation may ensure the formation of a similar VP16-dominated transcriptional regulatory complex that binds stably to IE gene promoters. However, with such a large difference in the VP16 TADs, the impact on the transcriptional factors recruited by different VP16 proteins and the recruitment methods remains to be further researched.
The Transcriptional Activation Function of VP16 Is Influenced by Other Cellular or Viral Proteins
Some cellular proteins and viral proteins can regulate the transcription of IE genes mediated by VP16. Heat-shock protein 90α (Hsp90α) is a cellular molecular chaperone that not only promotes the nuclear transport of HSV-1 capsid proteins and the correct localization of DNA polymerase but also regulates the activity of the promoter of HSV IE genes and stimulates HSV-1 infection (Zhong et al., 2014). Hsp90α is not a direct activator of IE genes but can bind to the core domain of VP16, maintain the stability of VP16, and ensure that VP16 is not degraded by the autolysosomal degradation pathway, thus participating in the transcriptional activation of IE genes mediated by VP16 (Zhong et al., 2014; Wang et al., 2018). The viral tegument proteins pUL14, VP11/12 (encoded by UL46), and VP13/14 (encoded by UL47) can enhance the efficiency of IE gene transcription mediated by VP16, which may play the same role in promoting nuclear input of VP16 (Lee et al., 2008; Yamauchi et al., 2008; Hernández Durán et al., 2019). Another tegument protein, the protein kinase US3, can regulate the release of the virus and promote the dissociation of VP11/12, VP13/14, and VP16 from tegument by phosphorylation, allowing entry into the nucleus as early as possible to initiate the transcription of viral genes (Kato and Kawaguchi, 2018; Hernández Durán et al., 2019). The HSV-1IE protein ICP22 can inhibit the transcription of IE genes, but VP16 can relieve the inhibition. Although there is no direct interaction between VP16 and ICP22, ICP22 can interact with various transcription factors that also bind to VP16 (Cun et al., 2009; Guo et al., 2012). Therefore, the transcriptional regulation of IE genes by VP16 and ICP22 may be achieved through some unknown action of both and related transcription factors. What other cellular proteins or viral proteins are involved in regulating the transcriptional activation of IE genes mediated by VP16, and in addition to forming transcriptional regulatory complexes with HCF-1 and Oct-1, whether VP16 promotes the transcription of IE genes through other mechanisms are directions for future research on VP16.
The Role of VP16 in Viral Assembly
VP16 is not only an efficient transcriptional activator in the life cycle of alphaherpesvirus, promoting gene transcription, but also an important tegument protein that can regulate the assembly and maturation of viruses.
Effects of VP16 on the Proliferation and Replication of Different Alphaherpesviruses
Among alphaherpesviruses, VP16 has different effects on the effective proliferation and replication of the virus. In this regard, many studies have been performed by constructing different VP16-deleted mutants. The HSV strain SJO2 carries a mutation at Ser375 to Ala in VP16, which disrupts its interaction with Oct-1, leading to a decrease in the transcription level of the IE genes and a 10-fold reduction in viral titers (Ottosen et al., 2006; Sawtell et al., 2011). Another strain in 1,814, which inserts four amino acids after residue 379 of VP16, eliminates the transcriptional activation of the IE genes. At a low multiplicity of infection, the plaque-forming efficiency of the virions is reduced by 100–10,000-fold, and at high multiplicity, although it is not easy to form plaques, many virions can be detected (Preston et al., 1997; Smiley and Duncan, 1997). The whole TAD truncation mutation in strain RP5 also completely inhibits the transcriptional activation of VP16, but the viral titer decreases even more (Herrera and Triezenberg, 2004). These studies showed that the elimination of transcriptional activation of IE genes mediated by VP16 does not block production of mature virions but can greatly reduce the infectivity of generated virions. In addition to mediating IE gene expression, the VP16 TAD also has a non-transcriptional activation function that affects the production of virions. Another HSV mutant, 8MA, which lacks almost the entire VP16 ORF, could not produce detectable progeny viruses in non-complementary cells without VP16 and could not replicate, while 8MA-R (a revertant of 8MA) showed completely restored replication ability, and 8MA could replicate effectively only in complementary cell lines containing VP16 (Weinheimer et al., 1992; Mossman et al., 2000) (Table 1), indicating that VP16 is necessary for HSV proliferation and replication. Similarly, DNA transfection of an ETIF deletion mutant (vL11ΔETIF) resulted in only transient expression and no production of infectious recombinant virus, while transfection into ETIF-expressing cell lines resulted in the generation of progeny virus (von Einem et al., 2006), indicating that ETIF is also necessary for the growth and replication of EHV; similar results were observed with BTIF (Misra et al., 1995).
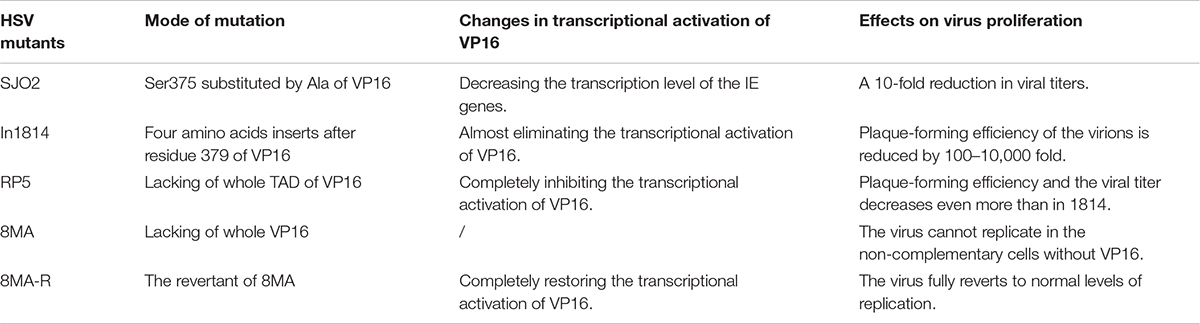
Table 1. Compare the transcriptional activation ability of different HSV VP16 mutants and effects on virus proliferation.
There are other new findings in the study of CHV and MDV. Infectious viruses cannot be produced after CHV genomic DNA is transfected into canine kidney cells unless the DNA is cotransfected with CHV VP16 (Tyack et al., 2006). MDV replicates in a highly cell-related manner in cell culture, and MDV can proliferate in specific feather follicle epithelium (FFE) cells of chickens but not in other cell cultures. Interestingly, VP16 is highly expressed in FFE cells (Dorange et al., 2002; Ponnuraj et al., 2019). These results show that VP16 is critical for the production of infectious virions by CHV and MDV. Moreover, exogenous VP16 is required to generate progeny viruses, which may be due to the inability of the cellular transcriptional activation system to activate viral genome transcription and its reliance on the transcriptional activation of IE genes mediated by the introduced VP16 to promote efficient genome expression in series. Contrary to the above studies, PRV VP16 is unnecessary for replication, and the VP16 null virus PRV-ΔVP16 can still proliferate in host cells. Although the virus grows slowly, the plaques are small, and the viral titer is significantly reduced, these defects can be fully recovered in PRV VP16-expressing cell lines (Fuchs et al., 2002, 2003). An ORF10 deletion mutant of VZV also proliferates normally in cell culture, and the viral titer is not decreased significantly, indicating that ORF10 is also unnecessary for VZV replication (Cohen and Seidel, 1994).
Influencing the Formation of Mature Virions by VP16
Effective Integration of VP16 Into the Tegument Requires VP1/2
In which stages does VP16 mainly participate in the virion assembly process? What interaction networks are involved? Observing PRV particles, such as most tegument proteins, VP16 can be detected only in nucleocapsids in the cytoplasm or extracellular mature virions, and it is integrated into nucleocapsids before secondary envelopment in the cytoplasm (Fuchs et al., 2002, 2003). However, in HSV-1, VP16 can be detected in the perinuclear space, indicating that it is integrated into nucleocapsids before primary envelopment (Mossman et al., 2000). HSV-1 VP16 can interact with some capsid proteins, such as pUL17, pUL19, pUL25, and the complex of pUL18–pUL38, so that VP16 can bind to the assembly site of immature capsids to promote the formation of assemblies (a nuclear structure formed by aggregation of immature capsids and some tegument proteins), thus promoting the maturation of nucleocapsids (Ward et al., 1996; Dai and Zhou, 2018). However, VP16 cannot bind to capsid alone, and the effective assembly of VP16 on the capsid is dependent on the largest tegument protein VP1/2 (encoded by UL36) (Coller et al., 2007; Svobodova et al., 2012) (Figure 2).
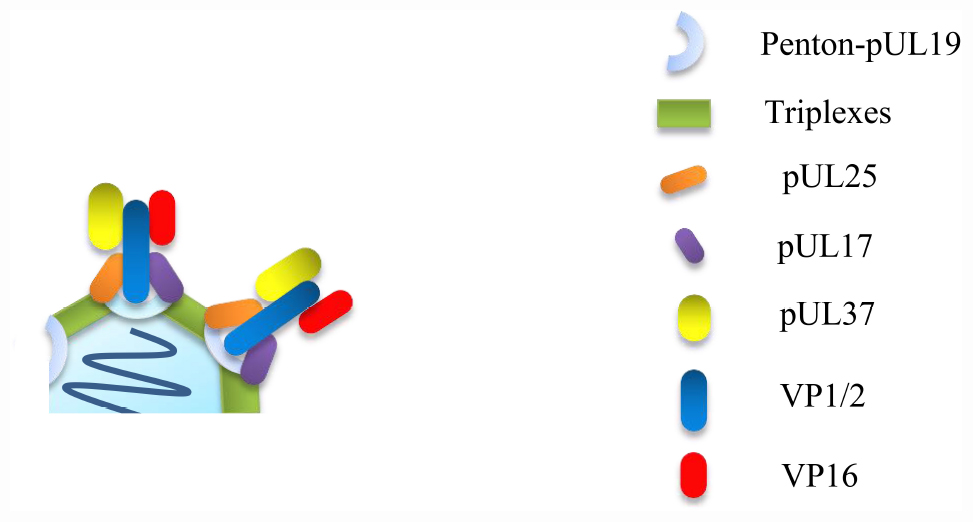
Figure 2. VP16 cannot be directly attached to the capsid alone, effective binding of VP16 to capsid requires VP1/2, and VP16 cannot only promote the formation of assemblies, so as to promote the maturation of nucleocapsids, but also promote the acquisition of outer tegument and envelope of nucleocapsids in the cytoplasm (Owen et al., 2015; Heming et al., 2017).
VP1/2 can bind to nucleocapsids in the nucleus, facilitating the primary envelopment and nuclear egress of the capsids (Schipke et al., 2012; Ivanova et al., 2016). VP1/2 can directly interact with VP16 to guide the capsids to the binding site of VP16; therefore, VP16 is assembled on the nucleocapsids. HSV VP16 begins to bind to capsids via VP1/2 in the nucleus (Ko et al., 2010; Svobodova et al., 2012). After the nucleocapsids leave the nucleus, additional VP16 can integrate into the tegument so that the number of VP16 molecules in the extracellular mature HSV virions is much higher than that in the enveloped primary virions in the perinuclear space. However, PRV VP16 binds to capsids only in the cytoplasm via VP1/2 (Naldinho-Souto et al., 2006). VP1/2, VP16, and another inner tegument protein, pUL37, can form a complex under the mediation of VP1/2 to promote the transport of capsids along microtubules in the cytoplasm, although pUL37 does not directly interact with VP16 (Sandbaumhüter et al., 2013; Laine et al., 2015) (Figure 3). Moreover, in HSV-1, the levels of VP1/2 and pUL37 seem to be strictly controlled and do not change even in the absence of VP16; however, there is a compensation relationship between VP16 and pUL37 in PRV, as a decreased quantity of VP16 will lead to an increase in the introduction of pUL37, and a lack of VP16 can also be compensated by the addition of other tegument proteins, such as VP11/12 and VP22 (encoded by UL49), or cellular proteins, such as dynein, which may be the main reason why VP16 is not an essential protein for PRV replication (Naldinho-Souto et al., 2006; Bucks et al., 2011; Diefenbach, 2015).
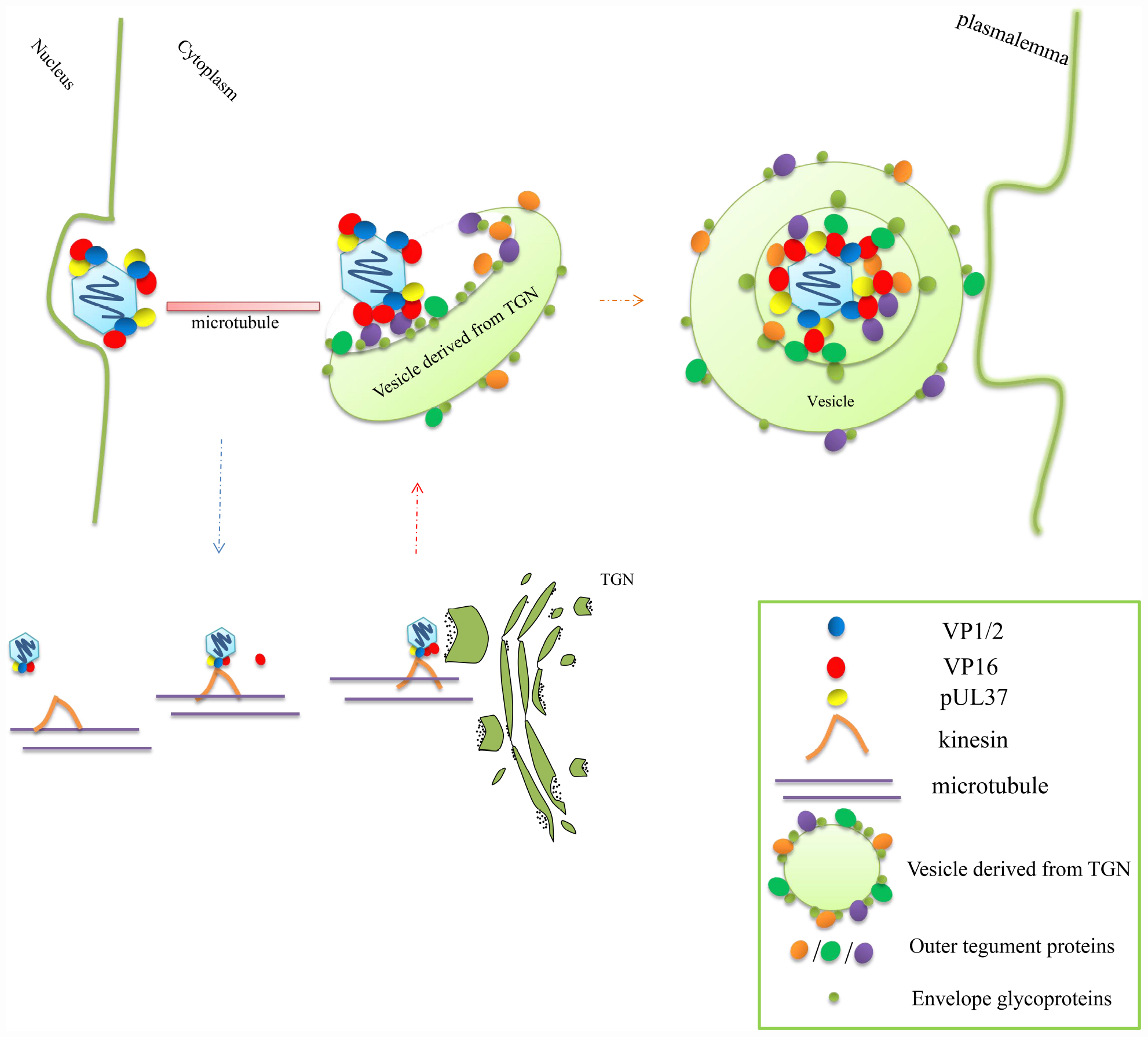
Figure 3. VP16 promotes nucleocapsid microtubule-transport and secondary envelopment in the cytoplasm. The complex composed of VP16, VP1/2, and pUL37 can bind kinesin on the microtubule to promote nucleocapsid transport; during transport, the capsid particle can bind to more VP16. When the nucleocapsid is transported to the specialized vesicles, which derived from the TGN, that are studded with various outer tegument proteins and glycoproteins, the nucleocapsid can effectively bud into the vesicle through the interaction between the tegument proteins and glycoproteins and thus obtain secondary envelopment. The secondary envelopment step also provides a transport vesicle that later fuses with the plasmalemma to release the mature enveloped virion out of the cell (Kelly et al., 2009; Owen et al., 2015).
VP16 Affects the Maturation of Virions Mainly by Promoting the Secondary Envelopment of Nucleocapsids
Although PRV-ΔVP16 can still produce a low level of infectious virions, the morphology of the virions is severely deficient in that the newly formed nucleocapsids are largely retained in the cytoplasm, while few enveloped virions are found in the cytoplasm or extracellular space; in contrast, a large number of capsid-free particles are produced and released (Fuchs et al., 2002, 2003). The morphology of EHV virions with ETIF deletion also has similar defects: The nucleocapsids can start budding on Golgi-derived vesicles but cannot completely enter the vesicles. There is a blurry mass around the nucleocapsids, which may block the secondary envelopment of the nucleocapsids (von Einem et al., 2006). After the HSV VP16 deletion strain infects cells, similarly, a large number of non-enveloped capsids accumulate around the vesicles, which are unable to enter the vesicles and produce extracellular enveloped particles. Enveloped virions can be observed only in the perinuclear space, and the quantity increases significantly; moreover, the level of DNA encapsidation decreases and the number of empty capsids increases significantly (Mossman et al., 2000; Knez et al., 2003). This also confirms that there are some interactions between VP16 and capsid proteins in HSV that can promote DNA encapsidation (Table 2). It also shows that VP16 has an impact on the egress of primary enveloped particles from the perinuclear space, but the effect is not significant. The most important factor causing the failure of virions to mature due to the absence of VP16 is that the secondary envelopment is severely blocked, and the nucleocapsids cannot enter the vesicles; as a result, they cannot be excreted from the cell through vesicle transport and fusion with cell membranes (Yuan et al., 2005; Diefenbach, 2015; Owen et al., 2015).
The Mechanism of VP16 Effects on Secondary Envelopment
How does VP16 affect the secondary envelopment of nucleocapsids? Through the analysis of the particle composition of nucleocapsids in the cytoplasm and extracellular capsid-free particles of VP16-null HSV and PRV, the tegument components of these two types of particles were found to be different. The tegument proteins on the nucleocapsids in the cytoplasm are mainly the inner proteins VP1/2 and pUL37, while the tegument components of extracellular capsid-free particles do not contain VP1/2 and pUL37 but mainly the outer tegument proteins VP11/12, VP13/14, and VP22 (Fuchs et al., 2002, 2007; Laine et al., 2015). This finding indicates that the connection between the inner tegument proteins and the outer tegument proteins is severed in the absence of VP16 and that VP16 acts as a bridge between the inner tegument and outer tegument. The large number of capsid-free particles indicates that the involvement of the outer tegument and Golgi-derived envelope in the maturation of virions is not affected by the nucleocapsid and proves that the formation of a whole virus particle is actually composed of two parts: the nucleocapsid and the outer tegument–envelope complex present on the vesicles derived from the trans-Golgi network (TGN) (Mettenleiter et al., 2006; Owen et al., 2015; Crump, 2018). VP16 is the key to the effective combination of these two parts (Figure 3). VP16 binds to the nucleocapsid through the action of VP1/2. When nucleocapsid is transported to the secondary envelope loci that associate with the Golgi apparatus, VP16 cannot only bind to outer tegument proteins of the outer tegument–envelope complex but also directly bind to some glycoproteins of the complex (Figure 4). After contact with the glycoproteins, the produced nucleocapsids can effectively bud in vesicles to complete secondary envelopment; this is because the glycoproteins can identify specific receptors on the membrane and promote the penetration of virions (Arii and Kawaguchi, 2018; White et al., 2020).
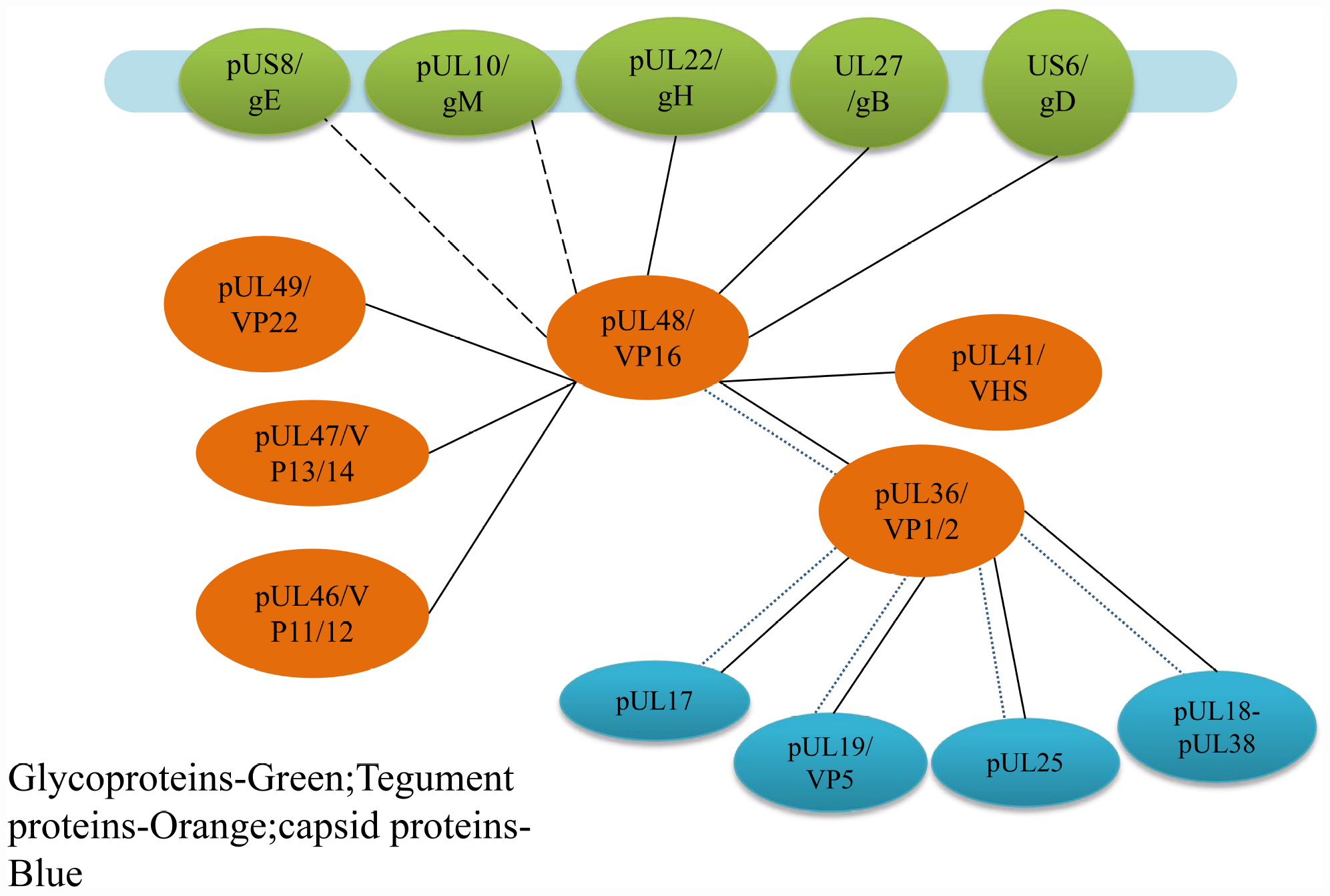
Figure 4. Protein interaction networks of VP16 that are involved in the assembly of alphaherpesvirus. It is the important linker from capsid-associated proteins to envelope-associated proteins. Solid lines indicate direct interactions, and short dashed lines show indirect interactions with capsid proteins by VP1/2 demonstrated in HSV, and the long dashed lines demonstrated in PRV (Owen et al., 2015).
Interactions of VP16 With Inner Tegument Proteins
The main outer tegument protein that interacts with VP16 at this stage is VP22. In HSV-1, VP22 effectively assembles into the nucleocapsid, which requires a collective effect by the VP16-binding region at residues 160–212 and the cytoplasmic complex-binding region at residues 213–301 at the C-terminus. Without the VP16-binding region, the amount of VP22 integrated into the tegument is significantly reduced, but VP22 does not inversely affect VP16 recruitment (Hafezi et al., 2005; O’Regan et al., 2007; Hew et al., 2015) (Figure 5). In addition to improving the efficiency of IE gene transcription mediated by VP16, the other two tegument proteins VP11/12 and VP13/14 can also promote secondary envelopment (Murphy et al., 2008; Guo et al., 2010; Owen et al., 2015). However, in this process, the relationship between VP11/12, VP13/14, and VP16 needs to be further explored.
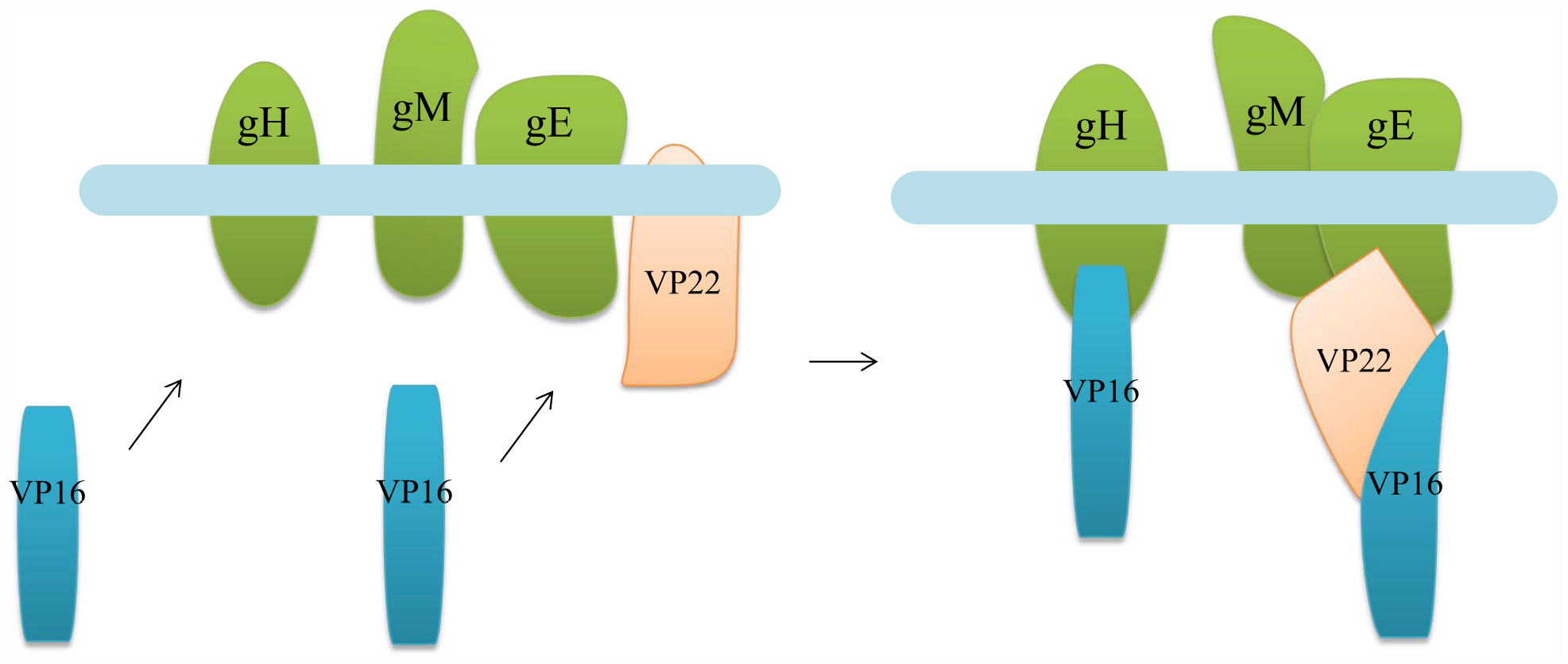
Figure 5. Diagram illustrating the binding of VP16 to gH and binding to the envelope through VP22. When the nucleocapsid is transported to the vesicle derived from the Golgi membrane, VP16 on the capsid cannot only directly bind to some glycoproteins on the envelope but also combine with the envelope through some tegument proteins to promote the secondary envelope of the nucleocapsid (Diefenbach, 2015; Owen et al., 2015).
Interaction of VP16 With Envelope Glycoproteins
The interaction between VP16 and envelope glycoproteins is not always the same in different alphaherpesviruses. In HSV-1, VP16 can bind to gH, and the reaction between the two belongs to the association between the tegument polypeptide and cytoplasmic tail of the envelope glycoprotein (Figure 5), providing the molecular driving force for nucleocapsid budding at the vesicle membrane (Gross et al., 2003). This association is temperature dependent, taking place at a physiological temperature of 37°C but not at low temperatures. NMR analysis shows that this effect is related to the conformation of the C-terminal peptide of gH. In a low-temperature environment, the gH peptide forms a specific and stable structure, while at physiological temperature, the peptide structure disintegrates and becomes irregular in order to bind to VP16. This effect raises the hypothesis of the “induced fit” mechanism of gH, that its tail is plastic and unstructured before binding, so that it can interact with VP16 and form a specific conformation, while another conformation may form when it binds to other tegument proteins (Gross et al., 2003; Kamen et al., 2005). In addition, in HSV-1, VP16 can also interact with gB and gD, but whether the interaction modes are the same as that of gH has not been studied (Johnson et al., 2011; You et al., 2018; Komala Sari et al., 2020). In PRV, VP16 interacts mainly with gM and gE but not with the cytoplasmic tail of gH (Chang et al., 2009; Omar et al., 2013). Although the HSV-1 and PRV gH proteins are homologous, the cytoplasmic tail of gH is extremely unconserved. It is possible that the cytoplasmic tail of PRV gH does not have a structural basis for binding to VP16 but can interact with other tegument proteins and participate in secondary envelopment.
The Negative Regulation of VHS by VP16 Also Affects Viral Assembly
In addition to facilitating transcription of the viral genome and secondary envelopment of nucleocapsids, VP16 can also affect viral assembly through the negative regulation of host shutdown protein VHS (encoded by UL41) (Knez et al., 2003). VHS can non-selectively degrade host cellular and viral mRNA, and when the host cellular mRNA is degraded, VP16 can combine with VHS to inhibit its mRNA degradation activity and accumulate viral mRNA, which can stimulate viral gene expression (Knez et al., 2003; Strand and Leib, 2004). After HSV VP16-null strain 8MA infection of cells, VHS activity is unrestricted due to the inability to synthesize VP16 and results in excessive degradation of viral mRNA so that its translation is aborted halfway through the infection cycle, leading to the inhibition of synthesis of a variety of viral proteins. The failure of the normal synthesis of multiple structural proteins inevitably leads to a serious impact on viral assembly. For example, the expression of the capsid protein pUL19 is inhibited, which also leads to a reduction in the level of DNA encapsidation and an increase in the number of intracellular and cytoplasmic empty capsids (Heming et al., 2017). Therefore, it is reasonable to suspect that the unrestricted activity of VHS is the main reason why 8MA cannot replicate effectively. The study of the VP16 and VHS double-deleted strain 8MA/ΔSma answers this question. Compared with 8MA, 8MA/ΔSma shows no difference except increased encapsidation of DNA, and the virus can still not proliferate in cell lines without VP16 expression (Mossman et al., 2000). This finding indicates that the fatal defect of virus replication caused by VP16 deficiency cannot be overcome by inhibiting the expression of VHS; it is mainly because the connection between the nucleocapsid, outer tegument, and envelope is cut off, and effective secondary envelopment cannot be carried out. There is no significant difference in the morphology of nucleocapsids and mature virions of the ORF10-null VZV strain precisely because, in VZV, ORF10 is unnecessary to guide the secondary envelopment of the virions, so ORF10 is not important for VZV maturation, which may be dominated by other tegument proteins (Cohen and Seidel, 1994; Cai et al., 2012). Although the life cycles of different alphaherpesviruses all go through similar processes, the viral proteins involved or playing major roles at different stages and the specific molecular mechanisms may vary, and additional research is needed to elucidate the specific processes of the life cycles of different alphaherpesviruses.
De Novo Synthesis of VP16 Coordinates Reactivation From HSV Latency
Latent infection is a characteristic of herpesviruses and can help them evade the host immune response. Once the virus establishes latent infection, its genome can persist in the cell and may be activated by external factors to reenter the lytic period of replication and proliferation. Neurocytes are the primary site of latent herpesvirus infection (Kollias et al., 2015; Menendez and Carr, 2017). HSV establishes latent infection only in the postmitotic neurons of the host peripheral nervous system. Under the periodic stimulation of different stressors, the latent state of infected neurons is activated and then enters the lytic cycle to regenerate infectious virions (Kennedy et al., 2015; Whitley and Baines, 2018; Rübben, 2020). The establishment of latent infection is related to the interaction between the viral genome and the nuclear environment (Zhang et al., 2020). The viral genome and promyelocytic leukemia (PML) protein occupy the same nuclear region in infected neurons and eventually form nucleosome structures containing viral DNA (PML-NBs) (Cohen et al., 2018; Watson et al., 2018). ICP0 can induce degradation of a variety of cellular proteins through the proteasome pathway, including components of PML-NBs and centromeres. Thus, ICP0 can induce the destabilization of PML-NBs and centromeric chromatin, contributing to the establishment of a suitable nuclear environment for lytic infection (Lomonte and Morency, 2007; Gross et al., 2012; Wang et al., 2012), and ICP4 plays a major regulatory role in gene transcription and is necessary for HSV to undergo lytic infection (Hu et al., 2019). Thus, ICP0 and ICP4 are the two main proteins involved in the activation of HSV latent infection. Earlier studies suggested that VP16 has no effect on the exit of alphaherpesvirus from latency and the initiation of the lytic cycle because it is difficult to rely on structural proteins produced at the late stage of the infection cycle to initiate transcription of the viral genome during the lytic phase (Sears et al., 1991; Ecob-Prince et al., 1993). However, an increasing number of studies indicate that VP16 plays a significant role in HSV reactivation from latency (Thompson et al., 2009; Danaher et al., 2013; Sawtell and Thompson, 2016). VP16 may not be efficiently transported to the cell body through the axon, resulting in suppressed synthesis of ICP0 and ICP4, which promotes the entry of virus into latency (Thompson and Sawtell, 2010; Sawtell et al., 2011). Once VP16 is deficient, latently infected TG neurons may not detect the expression of viral proteins after stress stimulation. However, in the absence of other viral proteins, the VP16 promoter can be activated in neurons that are latently infected after stress stimulation (Thompson et al., 2009). When the virus changes from latency to the lytic cycle, VP16 does not act the same as the typical model of a late gene; it has the ability to be synthesized before IE genes in the initial stage of reactivation from latency and does not depend on ICP0 and viral DNA replication. This phenomenon is dependent on the Egr-1/Sp1 sites on the VP16 promoter near the VP16 TATA box, which is responsible for regulating de novo synthesis of VP16 in the initial stage of reactivation from latency. Viral DNA replication in infected neurons is initiated by de novo VP16 synthesis induced by the Egr-1/Sp1 sites (Diefenbach et al., 2008; Thompson et al., 2009; Thompson and Sawtell, 2010, 2019). Moreover, when VP16 can be effectively transported to the cell body of neurons, the expression of the IE genes ICP0 and ICP4 can be regulated by the TAATGARAT element by VP16, thereby causing lytic infection (Thompson and Sawtell, 2019). If the transcriptional activation function of VP16 is affected, such as when Ser375 is replaced by alanine, the number of neurons that can reexpress viral proteins in latently infected neurons during heat shock treatment is significantly reduced (Sawtell et al., 2011), indicating that the transcriptional activation function of VP16 is crucial for HSV reactivation from latency. However, VP16 is not unique to alphaherpesvirus reactivation from latency.
After stress stimulation, the latent infection established by BHV-1 can be reactivated even in the absence of VP16 (Kook et al., 2015). This phenomenon can occur because stress stimuli can increase the level of corticosteroids, such as glucocorticoid (GR), and there are glucocorticoid receptor response sites (GREs) upstream of the IE gene enhancer that can bind to GR. These sites are not related to the core motifs that are specifically recognized by VP16; moreover, in the absence of VP16, HCF-1 can combine with GR to form a complex that then acts on GREs to promote the transcription and expression of IE genes, thus promoting lytic infection (Frizzo da Silva et al., 2013; Kook et al., 2015; Sawant et al., 2018). Although no related reports were found in HSV, this observation proposed another mechanism for the reactivation of herpesviruses from latency, suggesting that VP16 is important for reactivation from latency but not unique. There may be other unknown mechanisms to promote herpesvirus reactivation from latency that require further investigation, especially cell factors such as HCF-1, which are particularly critical, requiring special attention.
Conclusion
In alphaherpesviruses, VP16 is a powerful transcriptional activator that specifically acts on IE genes, and the transcriptional activation mechanism of HSV VP16 has been studied most deeply. In addition to the formation of transcriptional regulatory complexes with HCF-1 and Oct-1, it is not clear whether VP16 has other mechanisms of regulating IE gene transcription. The current research focuses on the interaction of VP16 with other cellular or viral proteins and the mechanism and structure of the TAD associated with target proteins, which can help us understand how the TADs of other transcriptional activators work. Moreover, the transcriptional activation mediated by VP16 also plays an important role in the reactivation of alphaherpesviruses from latency. VP16 is also one of the main tegument components of alphaherpesviruses, and it is the interface between capsid-associated proteins (such as VP1/2) and membrane-associated proteins (such as VP22). In the secondary envelopment of nucleocapsids, VP16 plays the important role of “bridging,” effectively promoting capsid envelopment and mature virion formation. Although there have been many studies on the involvement of VP16 in the envelopment and assembly of virions, the interaction between VP16 and proteins involved in this process needs further exploration. Additionally, what different reactions will happen in alphaherpesviruses in which VP16 is not critical for assembly needs to be studied further, which will not only give us a clearer knowledge of VP16 but also be important for a deeper understanding of the alphaherpesvirus life cycle.
Author Contributions
All authors listed contributed to the completion of the article. DF and MW contributed to the design and wrote the article. RJ, QY, YW, DZ, XZ, SC, ML, SZ, XO, SM, QG, and DS provided ideas contributing to the conception of this article. XW, YL, YY, LZ, LP, and XC helped to create the tables and figures. AC modified the article. All authors reviewed and approved the final manuscript.
Funding
This work was supported by the National Natural Science Foundation of China (31872476), the China Agricultural Research System (CARS-42-17), the Sichuan Veterinary Medicine and Drug Innovation Group of China Agricultural Research System (SCCXTD-2020-18), and Integration and Demonstration of Key Technologies for Goose Industrial Chain in Sichuan Province (2018NZ0005).
Conflict of Interest
The authors declare that the research was conducted in the absence of any commercial or financial relationships that could be construed as a potential conflict of interest.
References
Aguilar, X., Blomberg, J., Brännström, K., Olofsson, A., Schleucher, J., and Björklund, S. (2014). Interaction studies of the human and Arabidopsis thaliana Med25-ACID proteins with the herpes simplex virus VP16- and plant-specific Dreb2a transcription factors. PLoS One 9:e98575. doi: 10.1371/journal.pone.0098575
Andersen, B., and Rosenfeld, M. G. (2001). POU domain factors in the neuroendocrine system: lessons from developmental biology provide insights into human disease. Endocr. Rev. 22, 2–35. doi: 10.1210/edrv.22.1.0421
Arii, J., and Kawaguchi, Y. (2018). The Role of HSV glycoproteins in mediating cell entry. Adv. Exp. Med. Biol. 1045, 3–21. doi: 10.1007/978-981-10-7230-7_1
Babb, R., Huang, C. C., Aufiero, D. J., and Herr, W. (2001). DNA recognition by the herpes simplex virus transactivator VP16: a novel DNA-binding structure. Mol. Cell. Biol. 21, 4700–4712. doi: 10.1128/mcb.21.14.4700-4712.2001
Bentrari, F., Chantôme, A., Knights, A., Jeannin, J. F., and Pance, A. (2015). Oct-2 forms a complex with Oct-1 on the iNOS promoter and represses transcription by interfering with recruitment of RNA PolII by Oct-1. Nucleic Acids Res. 43, 9757–9765. doi: 10.1093/nar/gkv829
Boštíková, V., Salavec, M., Smetana, J., Sleha, R., and Boštík, P. (2014). Infections caused by human alpha herpes viruses. Epidemiol. Mikrobiol. Imunol. 63, 205–212.
Bucks, M. A., Murphy, M. A., O’Regan, K. J., and Courtney, R. J. (2011). Identification of interaction domains within the UL37 tegument protein of herpes simplex virus type 1. Virology 416, 42–53. doi: 10.1016/j.virol.2011.04.018
Cai, M., Wang, S., Long, J., and Zheng, C. (2012). Probing of the nuclear import and export signals and subcellular transport mechanism of varicella-zoster virus tegument protein open reading frame 10. Med. Microbiol. Immunol. 201, 103–111. doi: 10.1007/s00430-011-0211-4
Cantin, G. T., Stevens, J. L., and Berk, A. J. (2003). Activation domain-mediator interactions promote transcription preinitiation complex assembly on promoter DNA. Proc. Natl. Acad. Sci. U.S.A. 100, 12003–12008. doi: 10.1073/pnas.2035253100
Carroll, K. D., Khadim, F., Spadavecchia, S., Palmeri, D., and Lukac, D. M. (2007). Direct interactions of Kaposi’s sarcoma-associated herpesvirus/human herpesvirus 8 ORF50/Rta protein with the cellular protein octamer-1 and DNA are critical for specifying transactivation of a delayed-early promoter and stimulating viral reactivation. J. Virol. 81, 8451–8467. doi: 10.1128/jvi.00265-07
Chang, H., Cheng, A., Wang, M., Guo, Y., Xie, W., and Lou, K. (2009). Complete nucleotide sequence of the duck plague virus gE gene. Arch. Virol. 154, 163–165. doi: 10.1007/s00705-008-0284-6
Chasman, D., Cepek, K., Sharp, P. A., and Pabo, C. O. (1999). Crystal structure of an OCA-B peptide bound to an Oct-1 POU domain/octamer DNA complex: specific recognition of a protein-DNA interface. Genes Dev. 13, 2650–2657. doi: 10.1101/gad.13.20.2650
Cleary, M. A., Stern, S., Tanaka, M., and Herr, W. (1993). Differential positive control by Oct-1 and Oct-2: activation of a transcriptionally silent motif through Oct-1 and VP16 corecruitment. Genes Dev. 7, 72–83. doi: 10.1101/gad.7.1.72
Cohen, C., Corpet, A., Roubille, S., Maroui, M. A., Poccardi, N., Rousseau, A., et al. (2018). Promyelocytic leukemia (PML) nuclear bodies (NBs) induce latent/quiescent HSV-1 genomes chromatinization through a PML NB/Histone H3.3/H3.3 Chaperone Axis. PLoS Pathog. 14:e1007313. doi: 10.1371/journal.ppat.1007313
Cohen, J. I., and Seidel, K. (1994). Varicella-zoster virus (VZV) open reading frame 10 protein, the homolog of the essential herpes simplex virus protein VP16, is dispensable for VZV replication in vitro. J. Virol. 68, 7850–7858. doi: 10.1128/jvi.68.12.7850-7858.1994
Coller, K. E., Lee, J. I., Ueda, A., and Smith, G. A. (2007). The capsid and tegument of the alphaherpesviruses are linked by an interaction between the UL25 and VP1/2 proteins. J. Virol. 81, 11790–11797. doi: 10.1128/jvi.01113-07
Crump, C. (2018). Virus Assembly and Egress of HSV. Adv. Exp. Med. Biol. 1045, 23–44. doi: 10.1007/978-981-10-7230-7_2
Cun, W., Guo, L., Zhang, Y., Liu, L., Wang, L., Li, J., et al. (2009). Transcriptional regulation of the Herpes Simplex Virus 1alpha-gene by the viral immediate-early protein ICP22 in association with VP16. Sci. China C Life Sci. 52, 344–351. doi: 10.1007/s11427-009-0051-2
Dai, B., Cheng, A., and Wang, M. (2013). Characteristics and functional roles of VP5 protein of herpesviruses. Rev. Med. Microbiol. 24, 35–40. doi: 10.1097/mrm.0b013e32835a1f1d
Dai, X., and Zhou, Z. H. (2018). Structure of the herpes simplex virus 1 capsid with associated tegument protein complexes. Science 360:aao7298. doi: 10.1126/science.aao7298
Dalrymple, M. A., McGeoch, D. J., Davison, A. J., and Preston, C. M. (1985). DNA sequence of the herpes simplex virus type 1 gene whose product is responsible for transcriptional activation of immediate early promoters. Nucleic Acids Res. 21:21.
Danaher, R. J., Cook, R. K., Wang, C., Triezenberg, S. J., Jacob, R. J., and Miller, C. S. (2013). C-terminal trans-activation sub-region of VP16 is uniquely required for forskolin-induced herpes simplex virus type 1 reactivation from quiescently infected-PC12 cells but not for replication in neuronally differentiated-PC12 cells. J. Neurovirol. 19, 32–41. doi: 10.1007/s13365-012-0137-7
Diefenbach, R. J. (2015). Conserved tegument protein complexes: Essential components in the assembly of herpesviruses. Virus Res. 210, 308–317. doi: 10.1016/j.virusres.2015.09.007
Diefenbach, R. J., Miranda-Saksena, M., Douglas, M. W., and Cunningham, A. L. (2008). Transport and egress of herpes simplex virus in neurons. Rev. Med. Virol. 18, 35–51. doi: 10.1002/rmv.560
Dorange, F., Tischer, B. K., Vautherot, J. F., and Osterrieder, N. (2002). Characterization of Marek’s disease virus serotype 1 (MDV-1) deletion mutants that lack UL46 to UL49 genes: MDV-1 UL49, encoding VP22, is indispensable for virus growth. J. Virol. 76, 1959–1970. doi: 10.1128/jvi.76.4.1959-1970.2002
Ecob-Prince, M. S., Rixon, F. J., Preston, C. M., Hassan, K., and Kennedy, P. G. (1993). Reactivation in vivo and in vitro of herpes simplex virus from mouse dorsal root ganglia which contain different levels of latency-associated transcripts. J. Gen. Virol. 74(Pt 6), 995–1002. doi: 10.1099/0022-1317-74-6-995
Frizzo da Silva, L., Kook, I., Doster, A., and Jones, C. (2013). Bovine herpesvirus 1 regulatory proteins bICP0 and VP16 are readily detected in trigeminal ganglionic neurons expressing the glucocorticoid receptor during the early stages of reactivation from latency. J. Virol. 87, 11214–11222. doi: 10.1128/jvi.01737-13
Fuchs, W., Granzow, H., Klupp, B. G., Karger, A., Michael, K., Maresch, C., et al. (2007). Relevance of the interaction between alphaherpesvirus UL3.5 and UL48 proteins for virion maturation and neuroinvasion. J. Virol. 81, 9307–9318. doi: 10.1128/jvi.00900-07
Fuchs, W., Granzow, H., Klupp, B. G., Kopp, M., and Mettenleiter, T. C. (2002). The UL48 tegument protein of pseudorabies virus is critical for intracytoplasmic assembly of infectious virions. J. Virol. 76, 6729–6742. doi: 10.1128/jvi.76.13.6729-6742.2002
Fuchs, W., Granzow, H., and Mettenleiter, T. C. (2003). A pseudorabies virus recombinant simultaneously lacking the major tegument proteins encoded by the UL46, UL47, UL48, and UL49 genes is viable in cultured cells. J. Virol. 77, 12891–12900. doi: 10.1128/jvi.77.23.12891-12900.2003
Fukuda, A., Nakadai, T., Shimada, M., Tsukui, T., Matsumoto, M., Nogi, Y., et al. (2004). Transcriptional coactivator PC4 stimulates promoter escape and facilitates transcriptional synergy by GAL4-VP16. Mol. Cell. Biol. 24, 6525–6535. doi: 10.1128/mcb.24.14.6525-6535.2004
Goodrich, J. A., Hoey, T., Thut, C. J., Admon, A., and Tjian, R. (1993). Drosophila TAFII40 interacts with both a VP16 activation domain and the basal transcription factor TFIIB. Cell 75, 519–530. doi: 10.1016/0092-8674(93)90386-5
Grapes, M., and O’Hare, P. (2000). Differences in determinants required for complex formation and transactivation in related VP16 proteins. J. Virol. 74, 10112–10121. doi: 10.1128/jvi.74.21.10112-10121.2000
Gross, S., Catez, F., Masumoto, H., and Lomonte, P. (2012). Centromere architecture breakdown induced by the viral E3 ubiquitin ligase ICP0 protein of herpes simplex virus type 1. PLoS One 7:e44227. doi: 10.1371/journal.pone.0044227
Gross, S. T., Harley, C. A., and Wilson, D. W. (2003). The cytoplasmic tail of Herpes simplex virus glycoprotein H binds to the tegument protein VP16 in vitro and in vivo. Virology 317, 1–12. doi: 10.1016/j.virol.2003.08.023
Gudkova, D., Dergai, O., Praz, V., and Herr, W. (2019). HCF-2 inhibits cell proliferation and activates differentiation-gene expression programs. Nucleic Acids Res. 47, 5792–5808. doi: 10.1093/nar/gkz307
Guiping, Y., Anchun, C., Mingshu, W., Xiaoying, H., Yi, Z., and Fei, L. (2007). Preliminary study on duck enteritis virus-induced lymphocyte apoptosis in vivo. Avian. Dis. 51, 546–549. doi: 10.1637/0005-2086(2007)51[546:psodev]2.0.co;2
Guo, H., Shen, S., Wang, L., and Deng, H. (2010). Role of tegument proteins in herpesvirus assembly and egress. Protein Cell 1, 987–998. doi: 10.1007/s13238-010-0120-0
Guo, L., Wu, W. J., Liu, L. D., Wang, L. C., Zhang, Y., Wu, L. Q., et al. (2012). Herpes simplex virus 1 ICP22 inhibits the transcription of viral gene promoters by binding to and blocking the recruitment of P-TEFb. PLoS One 7:e45749. doi: 10.1371/journal.pone.0045749
Guo, Y., Cheng, A., Wang, M., and Zhou, Y. (2009). Purification of anatid herpesvirus 1 particles by tangential-flow ultrafiltration and sucrose gradient ultracentrifugation. J. Virol. Methods 161, 1–6. doi: 10.1016/j.jviromet.2008.12.017
Hafezi, W., Bernard, E., Cook, R., and Elliott, G. (2005). Herpes simplex virus tegument protein VP22 contains an internal VP16 interaction domain and a C-terminal domain that are both required for VP22 assembly into the virus particle. J. Virol. 79, 13082–13093. doi: 10.1128/jvi.79.20.13082-13093.2005
Hall, D. B., and Struhl, K. (2002). The VP16 activation domain interacts with multiple transcriptional components as determined by protein-protein cross-linking in vivo. J. Biol. Chem. 277, 46043–46050. doi: 10.1074/jbc.M208911200
Harper, T. M., and Taatjes, D. J. (2018). The complex structure and function of mediator. J. Biol. Chem. 293, 13778–13785. doi: 10.1074/jbc.R117.794438
He, T., Wang, M., Cao, X., Cheng, A., Wu, Y., Yang, Q., et al. (2018). Molecular characterization of duck enteritis virus UL41 protein. Virol. J. 15:12. doi: 10.1186/s12985-018-0928-4
Heming, J. D., Conway, J. F., and Homa, F. L. (2017). Herpesvirus Capsid Assembly and DNA Packaging. Adv. Anat. Embryol. Cell Biol. 223, 119–142. doi: 10.1007/978-3-319-53168-7_6
Hernández Durán, A., Greco, T. M., Vollmer, B., Cristea, I. M., Grünewald, K., and Topf, M. (2019). Protein interactions and consensus clustering analysis uncover insights into herpesvirus virion structure and function relationships. PLoS Biol. 17:e3000316. doi: 10.1371/journal.pbio.3000316
Herr, W. (1998). The herpes simplex virus VP16-induced complex: mechanisms of combinatorial transcriptional regulation. Cold. Spring Harb. Symp. Quant. Biol. 63, 599–607. doi: 10.1101/sqb.1998.63.599
Herr, W., and Cleary, M. A. (1995). The POU domain: versatility in transcriptional regulation by a flexible two-in-one DNA-binding domain. Genes Dev. 9, 1679–1693. doi: 10.1101/gad.9.14.1679
Herrera, F. J., and Triezenberg, S. J. (2004). VP16-dependent association of chromatin-modifying coactivators and underrepresentation of histones at immediate-early gene promoters during herpes simplex virus infection. J. Virol. 78, 9689–9696. doi: 10.1128/jvi.78.18.9689-9696.2004
Hew, K., Dahlroth, S. L., Pan, L. X., Cornvik, T., and Nordlund, P. (2015). VP22 core domain from Herpes simplex virus 1 reveals a surprising structural conservation in both the Alpha- and Gammaherpesvirinae subfamilies. J. Gen. Virol. 96(Pt 6), 1436–1445. doi: 10.1099/vir.0.000078
Hirai, H., Tani, T., and Kikyo, N. (2010). Structure and functions of powerful transactivators: VP16. MyoD and FoxA. Int. J. Dev. Biol. 54, 1589–1596. doi: 10.1387/ijdb.103194hh
Homa, F. L., and Brown, J. C. (2015). Capsid assembly and DNA packaging in herpes simplex virus. Rev. Med. Virol. 7, 107–122. doi: 10.1002/(sici)1099-1654(199707)7:2<107::aid-rmv191>3.0.co;2-m
Hori, R. T., Xu, S., Hu, X., and Pyo, S. (2004). TFIIB-facilitated recruitment of preinitiation complexes by a TAF-independent mechanism. Nucleic Acids Res. 32, 3856–3863. doi: 10.1093/nar/gkh711
Hu, M., Depledge, D. P., Flores Cortes, E., Breuer, J., and Schang, L. M. (2019). Chromatin dynamics and the transcriptional competence of HSV-1 genomes during lytic infections. PLoS Pathog. 15:e1008076. doi: 10.1371/journal.ppat.1008076
Ikeda, K., Stuehler, T., and Meisterernst, M. (2002). The H1 and H2 regions of the activation domain of herpes simplex virion protein 16 stimulate transcription through distinct molecular mechanisms. Genes Cells 7, 49–58. doi: 10.1046/j.1356-9597.2001.00492.x
Ivanova, L., Buch, A., Döhner, K., Pohlmann, A., Binz, A., Prank, U., et al. (2016). Conserved Tryptophan Motifs in the Large Tegument Protein pUL36 are required for efficient secondary envelopment of herpes simplex virus capsids. J. Virol. 90, 5368–5383. doi: 10.1128/jvi.03167-15
Jia, R., Cheng, A., Wang, M., Qi, X., Zhu, D., Ge, H., et al. (2009). Development and evaluation of an antigen-capture ELISA for detection of the UL24 antigen of the duck enteritis virus, based on a polyclonal antibody against the UL24 expression protein. J. Virol. Methods 161, 38–43. doi: 10.1016/j.jviromet.2009.05.011
Johnson, D. C., Wisner, T. W., and Wright, C. C. (2011). Herpes simplex virus glycoproteins gB and gD function in a redundant fashion to promote secondary envelopment. J. Virol. 85, 4910–4926. doi: 10.1128/jvi.00011-11
Johnson, K. M., and Carey, M. (2003). Assembly of a mediator/TFIID/TFIIA complex bypasses the need for an activator. Curr. Biol. 13, 772–777. doi: 10.1016/s0960-9822(03)00283-5
Johnson, K. M., Mahajan, S. S., and Wilson, A. C. (1999). Herpes simplex virus transactivator VP16 discriminates between HCF-1 and a novel family member. HCF-2. J. Virol. 73, 3930–3940. doi: 10.1128/jvi.73.5.3930-3940.1999
Jonker, H. R., Wechselberger, R. W., Boelens, R., Folkers, G. E., and Kaptein, R. (2005a). Structural properties of the promiscuous VP16 activation domain. Biochemistry 44, 827–839.
Jonker, H. R. A., Wechselberger, R. W., Rolf, B., Folkers, G. E., and Rob, K. (2005b). Structural properties of the promiscuous VP16 activation domain. Biochemistry 44:827. doi: 10.1021/bi0482912
Kamen, D. E., Gross, S. T., Girvin, M. E., and Wilson, D. W. (2005). Structural basis for the physiological temperature dependence of the association of VP16 with the cytoplasmic tail of herpes simplex virus glycoprotein H. J. Virol. 79, 6134–6141. doi: 10.1128/jvi.79.10.6134-6141.2005
Kato, A., and Kawaguchi, Y. (2018). Us3 protein kinase encoded by HSV: the precise function and mechanism on viral life cycle. Adv. Exp. Med. Biol. 1045, 45–62. doi: 10.1007/978-981-10-7230-7_3
Kelly, B. J., Fraefel, C., Cunningham, A. L., and Diefenbach, R. J. (2009). Functional roles of the tegument proteins of herpes simplex virus type 1. Virus Res. 145, 0–186.
Kennedy, P. G., Rovnak, J., Badani, H., and Cohrs, R. J. (2015). A comparison of herpes simplex virus type 1 and varicella-zoster virus latency and reactivation. J. Gen. Virol. 96(Pt 7), 1581–1602. doi: 10.1099/vir.0.000128
Klemm, R. D., Goodrich, J. A., Zhou, S., and Tjian, R. (1995). Molecular cloning and expression of the 32-kDa subunit of human TFIID reveals interactions with VP16 and TFIIB that mediate transcriptional activation. Proc. Natl. Acad. Sci. U.S.A. 92, 5788–5792. doi: 10.1073/pnas.92.13.5788
Knez, J., Bilan, P. T., and Capone, J. P. (2003). A single amino acid substitution in herpes simplex virus type 1 VP16 inhibits binding to the virion host shutoff protein and is incompatible with virus growth. J. Virol. 77, 2892–2902. doi: 10.1128/jvi.77.5.2892-2902.2003
Ko, D. H., Cunningham, A. L., and Diefenbach, R. J. (2010). The major determinant for addition of tegument protein pUL48 (VP16) to capsids in herpes simplex virus type 1 is the presence of the major tegument protein pUL36 (VP1/2). J. Virol. 84, 1397–1405. doi: 10.1128/jvi.01721-09
Kobayashi, N., Boyer, T. G., and Berk, A. J. (1995). A class of activation domains interacts directly with TFIIA and stimulates TFIIA-TFIID-promoter complex assembly. Mol. Cell. Biol. 15, 6465–6473. doi: 10.1128/mcb.15.11.6465
Kobty, M. (2015). Herpes simplex virus: beyond the basics. Neonatal Netw. 34, 279–283. doi: 10.1891/0730-0832.34.5.279
Kollias, C. M., Huneke, R. B., Wigdahl, B., and Jennings, S. R. (2015). Animal models of herpes simplex virus immunity and pathogenesis. J. Neurovirol. 21, 8–23. doi: 10.1007/s13365-014-0302-2
Komala Sari, T., Gianopulos, K. A., and Nicola, A. V. (2020). Glycoprotein C of Herpes Simplex Virus 1 Shields Glycoprotein B from antibody neutralization. J. Virol. 94:e01852-19. doi: 10.1128/jvi.01852-19
Kook, I., Doster, A., and Jones, C. (2015). Bovine herpesvirus 1 regulatory proteins are detected in trigeminal ganglionic neurons during the early stages of stress-induced escape from latency. J. Neurovirol. 21, 585–591. doi: 10.1007/s13365-015-0339-x
Kutluay, S. B., DeVos, S. L., Klomp, J. E., and Triezenberg, S. J. (2009). Transcriptional coactivators are not required for herpes simplex virus type 1 immediate-early gene expression in vitro. J. Virol. 83, 3436–3449. doi: 10.1128/jvi.02349-08
Laboissière, S., Walker, S., and O’Hare, P. (1997). Concerted activity of host cell factor subregions in promoting stable VP16 complex assembly and preventing interference by the acidic activation domain. Mol. Cell. Biol. 17, 7108–7118. doi: 10.1128/mcb.17.12.7108
Lai, J. S., and Herr, W. (1997). Interdigitated residues within a small region of VP16 interact with Oct-1. HCF, and DNA. Mol. Cell. Biol. 17, 3937–3946. doi: 10.1128/mcb.17.7.3937
Laine, R. F., Albecka, A., van de Linde, S., Rees, E. J., Crump, C. M., and Kaminski, C. F. (2015). Structural analysis of herpes simplex virus by optical super-resolution imaging. Nat. Commun. 6:5980. doi: 10.1038/ncomms6980
Langlois, C., Mas, C., Di Lello, P., Jenkins, L. M., Legault, P., and Omichinski, J. G. (2008). NMR structure of the complex between the Tfb1 subunit of TFIIH and the activation domain of VP16: structural similarities between VP16 and p53. J. Am. Chem. Soc. 130, 10596–10604. doi: 10.1021/ja800975h
Lee, J. H., Vittone, V., Diefenbach, E., Cunningham, A. L., and Diefenbach, R. J. (2008). Identification of structural protein-protein interactions of herpes simplex virus type 1. Virology 378, 347–354. doi: 10.1016/j.virol.2008.05.035
Lee, M. S., Lim, K., Lee, M. K., and Chi, S. W. (2018). Structural Basis for the Interaction between p53 Transactivation Domain and the Mediator Subunit MED25. Molecules 23:2726. doi: 10.3390/molecules23102726
Lee, S., and Herr, W. (2001). Stabilization but not the transcriptional activity of herpes simplex virus VP16-induced complexes is evolutionarily conserved among HCF family members. J. Virol. 75, 12402–12411. doi: 10.1128/jvi.75.24.12402-12411.2001
Lefkowitz, E. J., Dempsey, D. M., Hendrickson, R. C., Orton, R. J., Siddell, S. G., and Smith, D. B. (2018). Virus taxonomy: the database of the International Committee on Taxonomy of Viruses (ICTV). Nucleic Acids Res. 46, D708–D717. doi: 10.1093/nar/gkx932
Liu, C., Cheng, A., Wang, M., Chen, S., Jia, R., Zhu, D., et al. (2015). Duck enteritis virus UL54 is an IE protein primarily located in the nucleus. Virol. J. 12:198. doi: 10.1186/s12985-015-0424-z
Liu, P., Chen, S., Wang, M., and Cheng, A. (2017). The role of nuclear localization signal in parvovirus life cycle. Virol. J. 14:80. doi: 10.1186/s12985-017-0745-1
Liu, Y., Gong, W., Huang, C. C., Herr, W., and Cheng, X. (1999). Crystal structure of the conserved core of the herpes simplex virus transcriptional regulatory protein VP16. Genes Dev. 13, 1692–1703. doi: 10.1101/gad.13.13.1692
Lomonte, P., and Morency, E. (2007). Centromeric protein CENP-B proteasomal degradation induced by the viral protein ICP0. FEBS Lett. 581, 658–662. doi: 10.1016/j.febslet.2007.01.027
Lu, R., and Misra, V. (2000). Potential Role for Luman, the Cellular Homologue of Herpes Simplex Virus VP16 (alpha Gene trans-Inducing Factor), in Herpesvirus Latency. J. Virol. 74, 934–943. doi: 10.1128/jvi.74.2.934-943.2000
Lu, R., Yang, P., Padmakumar, S., and Misra, V. (1998). The herpesvirus transactivator VP16 mimics a human basic domain leucine zipper protein, luman, in its interaction with HCF. J. Virol. 72, 6291–6297. doi: 10.1128/jvi.72.8.6291-6297.1998
Luciano, R. L., and Wilson, A. C. (2002). An activation domain in the C-terminal subunit of HCF-1 is important for transactivation by VP16 and LZIP. Proc. Natl. Acad. Sci. U.S.A. 99, 13403–13408. doi: 10.1073/pnas.202200399
Menendez, C. M., and Carr, D. J. J. (2017). Defining nervous system susceptibility during acute and latent herpes simplex virus-1 infection. J. Neuroimmunol. 308, 43–49. doi: 10.1016/j.jneuroim.2017.02.020
Mettenleiter, T. C., Klupp, B. G., and Granzow, H. (2006). Herpesvirus assembly: a tale of two membranes. Curr. Opin. Microbiol. 9, 423–429. doi: 10.1016/j.mib.2006.06.013
Milbradt, A. G., Kulkarni, M., Yi, T., Takeuchi, K., Sun, Z. Y., Luna, R. E., et al. (2011). Structure of the VP16 transactivator target in the Mediator. Nat. Struct. Mol. Biol. 18:410. doi: 10.1038/nsmb.1999
Misra, V., Bratanich, A. C., Carpenter, D., and O’Hare, P. (1994). Protein and DNA elements involved in transactivation of the promoter of the bovine herpesvirus (BHV) 1 IE-1 transcription unit by the BHV alpha gene trans-inducing factor. J. Virol. 68, 4898–4909. doi: 10.1128/jvi.68.8.4898-4909.1994
Misra, V., Walker, S., Hayes, S., and O’Hare, P. (1995). The bovine herpesvirus alpha gene trans-inducing factor activates transcription by mechanisms different from those of its herpes simplex virus type 1 counterpart VP16. J. Virol. 69, 5209–5216. doi: 10.1128/jvi.69.9.5209-5216.1995
Mittal, C., Culbertson, S. J., and Shogren-Knaak, M. A. (2018). Distinct requirements of linker DNA and transcriptional activators in promoting SAGA-mediated nucleosome acetylation. J. Biol. Chem. 293, 13736–13749. doi: 10.1074/jbc.RA118.004487
Mossman, K. L., Sherburne, R., Lavery, C., Duncan, J., and Smiley, J. R. (2000). Evidence that herpes simplex virus VP16 is required for viral egress downstream of the initial envelopment event. J. Virol. 74, 6287–6299. doi: 10.1128/jvi.74.14.6287-6299.2000
Murphy, M. A., Bucks, M. A., O’Regan, K. J., and Courtney, R. J. (2008). The HSV-1 tegument protein pUL46 associates with cellular membranes and viral capsids. Virology 376, 279–289. doi: 10.1016/j.virol.2008.03.018
Naldinho-Souto, R., Browne, H., and Minson, T. (2006). Herpes simplex virus tegument protein VP16 is a component of primary enveloped virions. J. Virol. 80, 2582–2584. doi: 10.1128/jvi.80.5.2582-2584.2006
Omar, O. S., Simmons, A. J., Andre, N. M., Wilson, D. W., and Gross, S. T. (2013). Pseudorabies virus and herpes simplex virus type 1 utilize different tegument-glycoprotein interactions to mediate the process of envelopment. Intervirology 56, 50–54. doi: 10.1159/000339467
O’Regan, K. J., Murphy, M. A., Bucks, M. A., Wills, J. W., and Courtney, R. J. (2007). Incorporation of the herpes simplex virus type 1 tegument protein VP22 into the virus particle is independent of interaction with VP16. Virology 369, 263–280. doi: 10.1016/j.virol.2007.07.020
O’Reilly, D., Hanscombe, O., and O’Hare, P. (1997). A single serine residue at position 375 of VP16 is critical for complex assembly with Oct-1 and HCF and is a target of phosphorylation by casein kinase II. EMBO J. 16, 2420–2430. doi: 10.1093/emboj/16.9.2420
Ottosen, S., Herrera, F. J., Doroghazi, J. R., Hull, A., Mittal, S., Lane, W. S., et al. (2006). Phosphorylation of the VP16 transcriptional activator protein during herpes simplex virus infection and mutational analysis of putative phosphorylation sites. Virology 345, 468–481. doi: 10.1016/j.virol.2005.10.011
Owen, D. J., Crump, C. M., and Graham, S. C. (2015). Tegument Assembly and Secondary Envelopment of Alphaherpesviruses. Viruses 7, 5084–5114. doi: 10.3390/v7092861
Phillips, K., and Luisi, B. (2000). The virtuoso of versatility: POU proteins that flex to fit. J. Mol. Biol. 302, 1023–1039. doi: 10.1006/jmbi.2000.4107
Ponnuraj, N., Tien, Y. T., Vega-Rodriguez, W., Krieter, A., and Jarosinski, K. W. (2019). The Herpesviridae Conserved Multifunctional Infected-Cell Protein 27 (ICP27) Is Important but Not Required for Replication and Oncogenicity of Marek’s Disease Alphaherpesvirus. J. Virol. 93:e01903-18. doi: 10.1128/jvi.01903-18
Preston, C. M., Mabbs, R., and Nicholl, M. J. (1997). Construction and characterization of herpes simplex virus type 1 mutants with conditional defects in immediate early gene expression. Virology 229, 228–239. doi: 10.1006/viro.1996.8424
Qi, X., Yang, X., Cheng, A., Wang, M., Zhu, D., and Jia, R. (2008). Quantitative analysis of virulent duck enteritis virus loads in experimentally infected ducklings. Avian. Dis. 52, 338–344. doi: 10.1637/8120-100207-ResNote.1
Ravarani, C. N., Erkina, T. Y., De Baets, G., Dudman, D. C., Erkine, A. M., and Babu, M. M. (2018). High-throughput discovery of functional disordered regions: investigation of transactivation domains. Mol. Syst. Biol. 14:e8190. doi: 10.15252/msb.20188190
Regier, J. L., Shen, F., and Triezenberg, S. J. (1993). Pattern of aromatic and hydrophobic amino acids critical for one of two subdomains of the VP16 transcriptional activator. Proc. Natl. Acad. Sci. U.S.A. 90, 883–887. doi: 10.1073/pnas.90.3.883
Reilly, P. T., and Herr, W. (2002). Spontaneous reversion of tsBN67 cell proliferation and cytokinesis defects in the absence of HCF-1 function. Exp. Cell Res. 277, 119–130. doi: 10.1006/excr.2002.5551
Rübben, A. (2020). [Anal herpes simplex virus infections]. Hautarzt 71, 293–297. doi: 10.1007/s00105-019-04539-5
Sandbaumhüter, M., Döhner, K., Schipke, J., Binz, A., Pohlmann, A., Sodeik, B., et al. (2013). Cytosolic herpes simplex virus capsids not only require binding inner tegument protein pUL36 but also pUL37 for active transport prior to secondary envelopment. Cell Microbiol. 15, 248–269. doi: 10.1111/cmi.12075
Sawant, L., Kook, I., Vogel, J. L., Kristie, T. M., and Jones, C. (2018). The Cellular Coactivator HCF-1 Is Required for Glucocorticoid Receptor-Mediated Transcription of Bovine Herpesvirus 1 Immediate Early Genes. J. Virol. 92:e00987-18. doi: 10.1128/jvi.00987-18
Sawtell, N. M., and Thompson, R. L. (2016). De Novo Herpes Simplex Virus VP16 Expression Gates a Dynamic Programmatic Transition and Sets the Latent/Lytic Balance during Acute Infection in Trigeminal Ganglia. PLoS Pathog. 12:e1005877. doi: 10.1371/journal.ppat.1005877
Sawtell, N. M., Triezenberg, S. J., and Thompson, R. L. (2011). VP16 serine 375 is a critical determinant of herpes simplex virus exit from latency in vivo. J. Neurovirol. 17, 546–551. doi: 10.1007/s13365-011-0065-y
Schipke, J., Pohlmann, A., Diestel, R., Binz, A., Rudolph, K., Nagel, C. H., et al. (2012). The C terminus of the large tegument protein pUL36 contains multiple capsid binding sites that function differently during assembly and cell entry of herpes simplex virus. J. Virol. 86, 3682–3700. doi: 10.1128/jvi.06432-11
Sears, A. E., Hukkanen, V., Labow, M. A., Levine, A. J., and Roizman, B. (1991). Expression of the herpes simplex virus 1 alpha transinducing factor (VP16) does not induce reactivation of latent virus or prevent the establishment of latency in mice. J. Virol. 65, 2929–2935. doi: 10.1128/jvi.65.6.2929-2935.1991
Sevin-Pujol, A., Sicard, M., Rosenberg, C., Auriac, M. C., Lepage, A., Niebel, A., et al. (2017). Development of a GAL4-VP16/UAS trans-activation system for tissue specific expression in Medicago truncatula. PLoS One 12:e0188923. doi: 10.1371/journal.pone.0188923
Simmen, K. A., Newell, A., Robinson, M., Mills, J. S., Canning, G., Handa, R., et al. (1997). Protein interactions in the herpes simplex virus type 1 VP16-induced complex: VP16 peptide inhibition and mutational analysis of host cell factor requirements. J. Virol. 71, 3886–3894. doi: 10.1128/jvi.71.5.3886-3894.1997
Smiley, J. R., and Duncan, J. (1997). Truncation of the C-terminal acidic transcriptional activation domain of herpes simplex virus VP16 produces a phenotype similar to that of the in1814 linker insertion mutation. J. Virol. 71, 6191–6193. doi: 10.1128/jvi.71.8.6191-6193.1997
Smith, G. A. (2017). Assembly and Egress of an Alphaherpesvirus Clockwork. Adv. Anat. Embryol. Cell Biol. 223:171. doi: 10.1007/978-3-319-53168-7_8
Strand, S. S., and Leib, D. A. (2004). Role of the VP16-binding domain of vhs in viral growth, host shutoff activity, and pathogenesis. J. Virol. 78, 13562–13572. doi: 10.1128/jvi.78.24.13562-13572.2004
Sullivan, S. M., Horn, P. J., Olson, V. A., Koop, A. H., Niu, W., Ebright, R. H., et al. (1998). Mutational analysis of a transcriptional activation region of the VP16 protein of herpes simplex virus. Nucleic Acids Res. 26, 4487–4496. doi: 10.1093/nar/26.19.4487
Svobodova, S., Bell, S., and Crump, C. M. (2012). Analysis of the interaction between the essential herpes simplex virus 1 tegument proteins VP16 and VP1/2. J. Virol. 86, 473–483. doi: 10.1128/jvi.05981-11
Thompson, R. L., Preston, C. M., and Sawtell, N. M. (2009). De novo synthesis of VP16 coordinates the exit from HSV latency in vivo. PLoS Pathog. 5:e1000352. doi: 10.1371/journal.ppat.1000352
Thompson, R. L., and Sawtell, N. M. (2010). Therapeutic implications of new insights into the critical role of VP16 in initiating the earliest stages of HSV reactivation from latency. Future Med. Chem. 2, 1099–1105. doi: 10.4155/fmc.10.197
Thompson, R. L., and Sawtell, N. M. (2019). Targeted Promoter Replacement Reveals That Herpes Simplex Virus Type-1 and 2 Specific VP16 Promoters Direct Distinct Rates of Entry Into the Lytic Program in Sensory Neurons in vivo. Front. Microbiol. 10:1624. doi: 10.3389/fmicb.2019.01624
Tyack, S. G., Studdert, M. J., and Johnson, M. A. (2006). Sequence and function of canine herpesvirus alpha-transinducing factor and its interaction with an immediate early promoter. Virus Genes 33, 299–307. doi: 10.1007/s11262-006-0069-5
Vogel, J. L., and Kristie, T. M. (2013). The dynamics of HCF-1 modulation of herpes simplex virus chromatin during initiation of infection. Viruses 5, 1272–1291. doi: 10.3390/v5051272
von Einem, J., Schumacher, D., O’Callaghan, D. J., and Osterrieder, N. (2006). The alpha-TIF (VP16) homologue (ETIF) of equine herpesvirus 1 is essential for secondary envelopment and virus egress. J. Virol. 80, 2609–2620. doi: 10.1128/jvi.80.6.2609-2620.2006
Walker, S., Greaves, R., and O’Hare, P. (1993). Transcriptional activation by the acidic domain of Vmw65 requires the integrity of the domain and involves additional determinants distinct from those necessary for TFIIB binding. Mol. Cell. Biol. 13, 5233–5244. doi: 10.1128/mcb.13.9.5233
Wang, L., Grossman, S. R., and Kieff, E. (2000). Epstein-Barr virus nuclear protein 2 interacts with p300, CBP, and PCAF histone acetyltransferases in activation of the LMP1 promoter. Proc. Natl. Acad. Sci. U.S.A. 97, 430–435. doi: 10.1073/pnas.97.1.430
Wang, S., Long, J., and Zheng, C. F. (2012). The potential link between PML NBs and ICP0 in regulating lytic and latent infection of HSV-1. Protein Cell 3, 372–382. doi: 10.1007/s13238-012-2021-x
Wang, X. G., Ma, S. Y., Chang, J. S., Shi, R., Wang, R. L., Zhao, P., et al. (2019). Programmable activation of Bombyx gene expression using CRISPR/dCas9 fusion systems. Insect. Sci. 26, 983–990. doi: 10.1111/1744-7917.12634
Wang, Y., Wang, R., Li, F., Wang, Y., Zhang, Z., Wang, Q., et al. (2018). Heat-shock protein 90α is involved in maintaining the stability of VP16 and VP16-mediated transactivation of α genes from herpes simplex virus-1. Mol. Med. 24:65. doi: 10.1186/s10020-018-0066-x
Ward, P. L., Ogle, W. O., and Roizman, B. (1996). Assemblons: nuclear structures defined by aggregation of immature capsids and some tegument proteins of herpes simplex virus 1. J. Virol. 70, 4623–4631. doi: 10.1128/jvi.70.7.4623-4631.1996
Watson, Z. L., Washington, S. D., Phelan, D. M., Lewin, A. S., Tuli, S. S., Schultz, G. S., et al. (2018). In Vivo Knockdown of the Herpes Simplex Virus 1 Latency-Associated Transcript Reduces Reactivation from Latency. J. Virol. 92:e00812-18. doi: 10.1128/jvi.00812-18
Weinheimer, S. P., Boyd, B. A., Durham, S. K., Resnick, J. L., and O’Boyle, D. R. (1992). Deletion of the VP16 open reading frame of herpes simplex virus type 1. J. Virol. 66, 258–269. doi: 10.1128/jvi.66.1.258-269.1992
White, E. M., Stampfer, S. D., and Heldwein, E. E. (2020). Expression, Purification, and Crystallization of HSV-1 Glycoproteins for Structure Determination. Methods Mol. Biol. 2060, 377–393. doi: 10.1007/978-1-4939-9814-2_23
Whitley, R., and Baines, J. (2018). Clinical management of herpes simplex virus infections: past, present, and future. F1000Res 7:F1000 Faculty Rev-1726. doi: 10.12688/f1000research.16157.1
Wilson, A. C., Freiman, R. N., Goto, H., Nishimoto, T., and Herr, W. (1997). VP16 targets an amino-terminal domain of HCF involved in cell cycle progression. Mol. Cell. Biol. 17, 6139–6146. doi: 10.1128/mcb.17.10.6139
Wu, Q., Lian, J. B., Stein, J. L., Stein, G. S., Nickerson, J. A., and Imbalzano, A. N. (2017). The BRG1 ATPase of human SWI/SNF chromatin remodeling enzymes as a driver of cancer. Epigenomics 9, 919–931. doi: 10.2217/epi-2017-0034
Wysocka, J., and Herr, W. (2003). The herpes simplex virus VP16-induced complex: the makings of a regulatory switch. Trends Biochem. Sci. 28, 294–304. doi: 10.1016/s0968-0004(03)00088-4
Yamauchi, Y., Kiriyama, K., Kubota, N., Kimura, H., Usukura, J., and Nishiyama, Y. (2008). The UL14 tegument protein of herpes simplex virus type 1 is required for efficient nuclear transport of the alpha transinducing factor VP16 and viral capsids. J. Virol. 82, 1094–1106. doi: 10.1128/jvi.01226-07
Yang, L., Wang, M., Cheng, A., Yang, Q., Wu, Y., Jia, R., et al. (2019). Innate immune evasion of alphaherpesvirus tegument proteins. Front. Immunol. 10:2196. doi: 10.3389/fimmu.2019.02196
You, Y., Cheng, A. C., Wang, M. S., Jia, R. Y., Sun, K. F., Yang, Q., et al. (2017). The suppression of apoptosis by α-herpesvirus. Cell Death Dis. 8:e2749. doi: 10.1038/cddis.2017.139
You, Y., Liu, T., Wang, M., Cheng, A., Jia, R., Yang, Q., et al. (2018). Duck plague virus Glycoprotein J is functional but slightly impaired in viral replication and cell-to-cell spread. Sci. Rep. 8:4069. doi: 10.1038/s41598-018-22447-x
Yuan, G. P., Cheng, A. C., Wang, M. S., Liu, F., Han, X. Y., Liao, Y. H., et al. (2005). Electron microscopic studies of the morphogenesis of duck enteritis virus. Avian. Dis. 49, 50–55. doi: 10.1637/7237-071004r
Zhang, S., Cheng, A., and Wang, M. (2011). Characteristics and functional roles of glycoprotein K of herpesviruses. Rev. Med. Microbiol. 22, 90–95. doi: 10.1097/mrm.0b013e3283494765
Zhang, Y., Chen, A., and Wang, M. (2016). Research Advances in VP16 of the herpes virus. Chin. J. Virol. 32, 817–824.
Zhang, Y., Xin, Q., Zhang, J. Y., Wang, Y. Y., Cheng, J. T., Cai, W. Q., et al. (2020). Transcriptional Regulation of Latency-Associated Transcripts (LATs) of Herpes Simplex Viruses. J. Cancer 11, 3387–3399. doi: 10.7150/jca.40186
Zhao, L., Cheng, A., Wang, M., Yuan, G., and Cai, M. (2008). Characterization of codon usage bias in the dUTPase gene of duck enteritis virus. Progr. Nat. Sci. 18, 1069–1076. doi: 10.1016/j.pnsc.2008.03.009
Keywords: alphaherpesvirus, VP16, transcriptional activation, secondary envelopment, reactivation from latency
Citation: Fan D, Wang M, Cheng A, Jia R, Yang Q, Wu Y, Zhu D, Zhao X, Chen S, Liu M, Zhang S, Ou X, Mao S, Gao Q, Sun D, Wen X, Liu Y, Yu Y, Zhang L, Tian B, Pan L and Chen X (2020) The Role of VP16 in the Life Cycle of Alphaherpesviruses. Front. Microbiol. 11:1910. doi: 10.3389/fmicb.2020.01910
Received: 09 May 2020; Accepted: 21 July 2020;
Published: 18 August 2020.
Edited by:
Akio Adachi, Kansai Medical University, JapanReviewed by:
Takayuki Murata, Fujita Health University, JapanEvelyne Manet, UMR 5308 Centre International de Recherche en Infectiologie (CIRI), France
Copyright © 2020 Fan, Wang, Cheng, Jia, Yang, Wu, Zhu, Zhao, Chen, Liu, Zhang, Ou, Mao, Gao, Sun, Wen, Liu, Yu, Zhang, Tian, Pan and Chen. This is an open-access article distributed under the terms of the Creative Commons Attribution License (CC BY). The use, distribution or reproduction in other forums is permitted, provided the original author(s) and the copyright owner(s) are credited and that the original publication in this journal is cited, in accordance with accepted academic practice. No use, distribution or reproduction is permitted which does not comply with these terms.
*Correspondence: Mingshu Wang, mshwang@163.com; Anchun Cheng, chenganchun@vip.163.com
†These authors have contributed equally to this work and share first authorship