- 1Key Laboratory of Coastal Biology and Biological Resources Utilization, Yantai Institute of Coastal Zone Research, Chinese Academy of Sciences, Yantai, China
- 2Key Laboratory of Coastal Environmental Processes and Ecological Remediation, Yantai Institute of Coastal Zone Research (YIC), Chinese Academy of Sciences (CAS), Yantai, China
- 3Department of Chemical Engineering, Sri Sivasubramaniya Nadar College of Engineering, Chennai, India
- 4Aix-Marseille Univ, CNRS, IRD, INRAE, Coll France, CEREGE, Aix en Provence, France
- 5State Key Laboratory of Multiphase Flow in Power Engineering, Xi’an Jiaotong University, Xi’an, China
- 6School of Resources and Environmental Engineering, Ludong University, Yantai, China
- 7College of Food Science and Engineering, Qingdao Agricultural University, Qingdao, China
- 8Shandong Key Laboratory of Biophysics, Shandong Engineering Laboratory of Swine Health Big Data and Intelligent Monitoring, Institute of Biophysics, Dezhou University, Dezhou, China
Recycling waste into new materials and energy is becoming a major challenge in the context of the future circular economy, calling for advanced methods of waste treatment. For instance, microbially-mediated anaerobic digestion is widely used for conversion of sewage sludge into biomethane, fertilizers and other products, yet the efficiency of microbial digestion is limited by the occurrence of antibiotics in sludges, originating from drug consumption for human and animal health. Here we present antibiotic levels in Chinese wastewater, then we review the effects of antibiotics on hydrolysis, acidogenesis and methanogenesis, with focus on macrolides, tetracyclines, β-lactams and antibiotic mixtures. We detail effects of antibiotics on fermentative bacteria and methanogenic archaea. Most results display adverse effects of antibiotics on anaerobic digestion, yet some antibiotics promote hydrolysis, acidogenesis and methanogenesis.
Introduction
Antibiotics are widely used to treat human and animal diseases, yet the overuse of antibiotics is inducing issues of pollution, development of antibiotic resistance by pathogenic bacteria, and inhibition of engineered microbial processes such as anaerobic digestion of wastewater. The global consumption of antimicrobials in livestock production was estimated at more than 63 thousand tons in 2010, and is projected to rise to 105 thousand tons by 2030 (Yadav and Kumar, 2020). In the 2010s, countries with the largest share of global antimicrobial consumption in livestock production were China (23%, in weight), United States of America (USA, 13%), Brazil (9%), India (3%), and Germany (3%). Projections show that by 2030 the main antibiotic-consuming nations should be China (30%), United States (10%), Brazil (8%), India (4%), and Mexico (2%) (Van Boeckel et al., 2015). Other reports estimate the annual consumption of antibiotics in China at about 150,000 tons to 200,000 tons in recent years, which is nearly ten times that of the United States and 150 times that of the United Kingdom (Larson, 2015; Zhang et al., 2015; Yadav and Kumar, 2020). The extensive use of antibiotics leads to their continuous enrichment through wastewater and sewage and eventual exposure to the natural environment (Chen et al., 2016; Liu et al., 2018; Qiao et al., 2018; Alonso et al., 2019). The strong adsorption capacity of sludge toward antibiotics enhances the accumulation of antibiotics in sludge. Therefore, antibiotic residues and their effects in sludge have attracted major attention (Ju et al., 2016; Shin et al., 2020; Yu et al., 2020; Xiao et al., 2021).
Anaerobic digestion involves processes during which microorganisms breakdown biodegradable organic material in the absence of oxygen (Chen et al., 2008). Digestion starts with bacterial hydrolysis of organic polymers such as proteins that are broken down into amino acids that feed bacteria. Sugars and amino acids are then converted by acidogenic bacteria into carbon dioxide, hydrogen, ammonia and organic acids. Acetogenetic bacteria convert these organic acids into acetate, and additional ammonia, hydrogen and carbon dioxide. Finally, these products are converted into methane and carbon dioxide by methanogens. Anaerobic digestion is widely used in the treatment of sewage sludges to reduce sludges volume and to produce methane (Mehariya et al., 2018; Xiao et al., 2019b).
The efficiency of anaerobic digestion is reduced by the presence of antibiotics and antibiotic residues, which inhibit the microbial community and activity, thus further limiting the efficiency of the entire anaerobic digestion system (Kovalakova et al., 2020; Rusanowska et al., 2020). Accordingly, existing pre-treatments are aimed to reduce levels of antibiotic residues (Gurmessa et al., 2020). For example, Pei et al. (2015) studied the effect of ultrasonic and ozone pre-treatments on pharmaceutical waste activated sludge’s solubilization and anaerobic biodegradability. Thermal hydrolysis pretreatment shows removal of fluoroquinones in sewage sludge during anaerobic digestion (Li et al., 2017). Research on the effects of antibiotics on anaerobic digestion has rapidly grown in the last decade (Walsh, 2000; Kohanski et al., 2007), yet comprehensive reviews are still rare (Cheng et al., 2018; Yang et al., 2019). Therefore, this paper takes China as an example to systematically summarize the general usage of antibiotics at present and reviews the main effects of antibiotics on anaerobic digestion with anaerobic biomethane production as the critical point.
Antibiotic Utilization Taking China as an Example
At present, antibiotics are mainly used in hospitals and livestock farms (Sim et al., 2011). Residual antibiotics are then transferred into wastewater where they are partly degraded and partly preserved. For instance, Table 1 shows that antibiotic concentrations range from 0.046 to 4.552 μg/L in medical wastewater and from 0 to 130.67 μg/L in livestock wastewater. Tetracyclines, β-lactams, sulfonamides and quinolones are found in medical and livestock wastewater (Wei et al., 2011; Zhang and Li, 2018). Medical wastewater also contains macrolides, which are mostly used in human clinical treatments (Wei et al., 2011; Li et al., 2013). However, compared with medical wastewater, animal wastewater contains higher levels of tetracyclines and sulfonamides, which are used to prevent and treat livestock diseases (Zhang and Li, 2018; Sun et al., 2019). The antibiotic contents in wastewater are variable in different regions (Table 1), such as 1.41 μg/L in Henan and less than 0.38 μg/L in Xinjiang for tetracycline. Wei et al. (2011) found that the antibiotic contents in wastewater are different within Jiangsu province, which is thought to be caused by the diverse utilization of antibiotics in different cities. The excessive use of antibiotics has received sufficient attention from the government and scientific researchers in China. As a consequence, a series of policies have been introduced, in aquaculture for instance (Broughton and Walker, 2010).
Effects of Antibiotics on Anaerobic Digestion
Microbes transform complex organic substances into methane through anaerobic digestion, which is the primary way of anaerobic mineralization of organics. The process of biomethane production from macromolecular organic carbon involves a variety of microbes, mainly fermenting bacteria and methanogenic archaea. The accumulation of antibiotics dissolved in wastewater into sludge can affect the microbial community associated with each stage of anaerobic digestion (Cheng et al., 2018; Zhi and Zhang, 2019; Luo et al., 2020). Specially, the influencing mechanisms of different antibiotics are various (Walsh, 2000; Kohanski et al., 2007). It is discussed in detail in the following parts.
Effects of Antibiotics on the Hydrolytic and Acidogenic Stages of Anaerobic Digestion
The hydrolysis stage is considered as the main rate-limiting step of anaerobic digestion (Mata-Alvarez et al., 2000; Carlsson et al., 2012; Gonzalez et al., 2018). During hydrolysis, partly insoluble sludge macromolecules such as proteins, carbohydrates, and lipids are converted into more soluble, smaller molecular substances. Then, during the acidification stage, the hydrolysates are further converted into volatile fatty acids (VFAs), including acetate, propionate, and butyrate.
Table 2 summarizes the effects of antibiotics on the successive steps of anaerobic digestion. Concerning hydrolysis and acidification, data shows the inhibition of the propionate metabolism by two antibiotics, of the butyrate metabolism by five antibiotics and of organic degradation by one antibiotic. Here, inhibiting antibiotics are macrolides and tetracyclines. For β-lactams, an absence of effect on organic degradation has been shown for cefalexin.
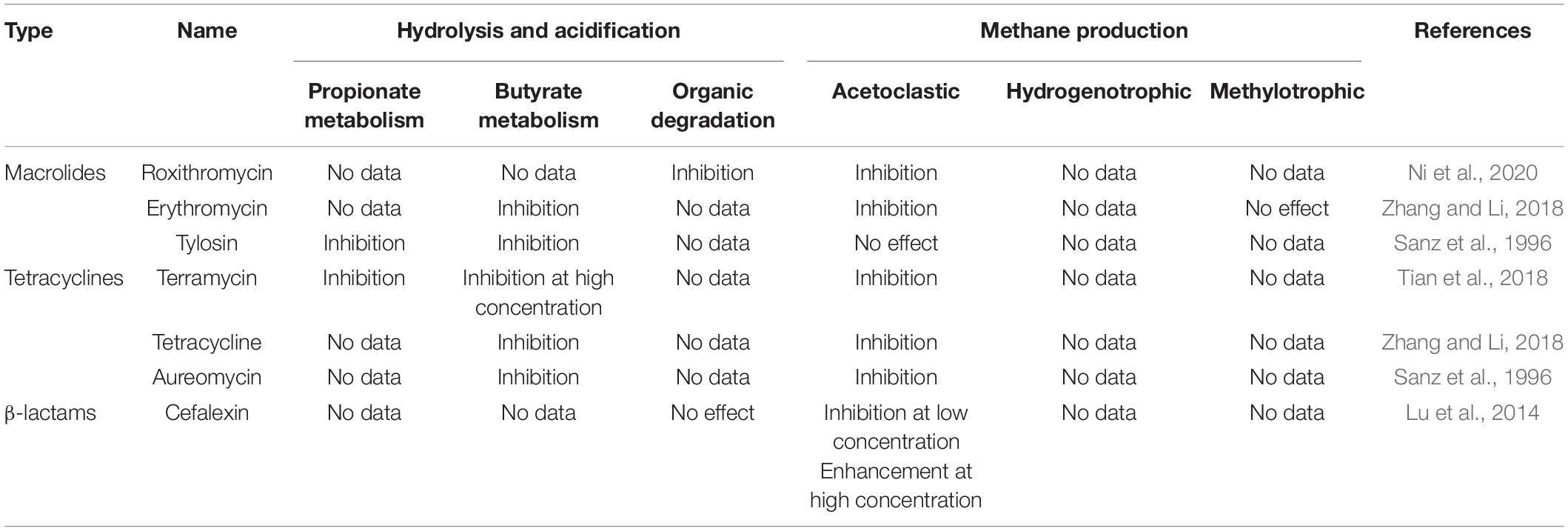
Table 2. Effects of antibiotics on the diverse stages of anaerobic digestion and biomethane production.
Effects of Antibiotics on the Hydrolytic Stage
Erythromycin’s long-term action inhibits microbial growth, reduces hydrolysis rate, blocks substrate storage, and accelerates endogenous respiration (Pala-Ozkok and Orhon, 2013). In contrast, the presence of azithromycin analogs in sludge leads to high methane production because of the increased hydrolysis efficiency that induces an increased proportion of fermentative bacteria and archae at the methanogenesis stage (Mustapha et al., 2018). Clarithromycin enhanced the production of VFAs during hydrolysis and acidogenesis of activated sludge, as a possible result of disruption and solubilization of extracellular polymeric substances (Huang et al., 2019). Yet clarithromycin inhibited acid consumption and, in turn, inhibited strongly hydrolysis and acidogenesis. In addition, species responsible for hydrolysis and acidogenesis were slightly more abundant with clarithromycin, whereas the abundance of acid-consuming microorganisms such as Gamma-proteobacteria and Rhodobacter declined. Overall, some macrolides and tetracyclines inhibit clearly hydrolysis and acidification, and more investigations are needed to confirm other isolated findings.
Effects of Antibiotics on the Acidogenic Stage
A study showed that roxithromycin macrolide and sulfamethoxazole increased the production of VFAs in anaerobic fermentation of waste activated sludge, which is explained by affecting microbial diversity and disruption of extracellular polymeric substances (Chen et al., 2020; Miritana et al., 2020). On the contrary, Ni et al. (2020) found that roxithromycin inhibits acidogenesis and methanogenesis in anaerobic digestion of activated sludge, leading to a decreased methane production. They also found that exposure to roxithromycin increased the abundance of antibiotic resistance genes (ARGs), esterases, methylases and phosphorylases in the digested sludge. Carneiro et al. (2020) suggest that the co-metabolic transformation of organic antibiotics is mainly achieved during acidogenesis. The influence of antibiotics on the acidogenic stage may also be closely related to the diversity, abundance, and activity of microorganisms.
The antibiotic concentration is a factor controlling effects on acidogenesis. For example, a high concentration of tylosin inhibited propionate and butyrate metabolisms during the anaerobic fermentation process of pig manure (Table 2; Stone et al., 2009). By contrast, a low tylosin concentration had no significant effect on the overall system performance, and, in particular, there was no change in the utilization of propionate, butyrate and acetate. In addition, some other studies presented similar trends (Cetecioglu et al., 2012; Tian et al., 2018). The mechanisms of inhibition may be explained by the accumulation of acidic intermediates that decrease the pH of the whole system. It was proposed that tylosin has negative effects on methanogenesis through its inhibition of propionate- and butyrate-oxidizing syntrophic bacteria and fermenting bacteria (Stone et al., 2009). Overall, there is convergent evidence that some macrolides and tetracyclines inhibit acidogenesis. Therefore, pretreatments should be performed to reduce antibiotic contents in order to improve further fermentation (Alamo et al., 2020; Gurmessa et al., 2020; Tao et al., 2020).
Effects of Antibiotics on Fermentative Bacteria
Bacteria having hydrolysis and acidification functions play an important role in the early stage of anaerobic digestion, in which these microbes convert larger molecules of organic matter in sludge into simple smaller molecules and then into VFAs, and consequently affect the overall efficiency of anaerobic digestion. In the last ten years, researchers focused on interaction between antibiotics and fermentative microbes. Some mechanisms of antibiotics affecting anaerobic fermentation have been elucidated. For example, macrolides and tetracyclines are inhibitors of protein synthesis in bacteria (Qiao et al., 2018), inhibiting bacteria’s normal growth. This may be the reason why these two types of antibiotics inhibit the bacteria having hydrolysis and acidification functions. The other mechanisms are concluded differently. Macrolides and tetracyclines bind to the 23S rRNA of the ribosomal large subunit and the 16S rRNA of the ribosomal small subunit, respectively (Vester and Douthwaite, 2001), which affect the function of ribosomes.
Different antibiotics of the same type also have diverse effects on fermentative bacteria. For example, both erythromycin and tylosin belonging to macrolides inhibit butyrate producing bacteria to a certain extent, leading to the accumulation of VFAs and the instability of the whole system (Amin et al., 2006). However, a recent study revealed that roxithromycin exposure affected the waste activated sludge anaerobic digestion and the change of ARGs in the anaerobic digestion, accompanied by the inhibition of acidogenesis and methanogenesis, leading to decreased methane production (Ni et al., 2020). Similarly, long-term exposure to tylosin directly inhibits the propionate-oxidizing syntrophic bacteria which closely relate to Syntrophobacter, thus indirectly inhibited Methanosaeta by high propionate concentrations and low pH, resulting in the long-term reactor failure (Shimada et al., 2011).
Compared to macrolides, fewer studies were conducted about the effects of tetracyclines and β-lactam on anaerobic fermentation. Though terramycin and aureomycin are both tetracyclines, they have different effects on anaerobic fermenting bacteria. Specially, the mechanisms ruling the effects of different concentrations of antibiotics on fermenting bacteria are also distinct. At a low concentration (50 mg/L), oxyterramycin significantly inhibited propionate oxidizing bacteria, and shown significantly lower degradation rate of propionate than that of the control group without antibiotics (Arikan et al., 2006). However, the metabolic activity of butyrate oxidizing bacteria was little affected, so that the butyrate content in VFAs was extremely low. At a high concentration (500 mg/L), terramycin can further enhance the inhibition of propionate oxidizing bacteria and, at the same time, inhibit butyrate oxidizing bacteria (Arikan et al., 2006).
β-lactam inhibitors can inhibit the synthesis of cell wall murein by inhibiting penicillin-binding protein, which can cause bacterial cell wall defects (Sanz et al., 1996). β-lactams can stimulate the synthesis of autolytic enzymes, thus accelerating bacterial death. Cefalexin belonging to β-lactams can stimulate the secretion of exopolysaccharides, which act as a protective layer of microbial cells and create a suitable environment for microbial growth and methanogenesis. Studies have shown that bacteria in urban sludge currently have higher drug resistance to β-lactams and lower resistance to tetracyclines. For example, the antibiotic resistance to ampicillin, cefalotin, and cefotaxime could be elevated through anaerobic digestion (LaPara et al., 2011).
Effects of Antibiotics on the Methanogenic Stage of Anaerobic Digestion
The methanogenic stage, as the final step of anaerobic digestion, is the key step of substrate fermentation. Three processes are actually known to contribute to methane production: CO2 reduction, acetate dismutation, and methylotrophic methanogenesis. First, about the two-thirds of biomethane production is explained by acetoclastic methanogenesis in natural environments. However, in H2 upgrading/ammonia inhibited AD system, methane production is mainly from hydrogenotrophic methanogenesis pathway. For acetoclastic methanogenesis, methanogens oxidize carboxyl groups to CO2 and generate reducing forces to reduce methyl carbon to methane (Welte and Deppenmeier, 2014; Xiao et al., 2019b, 2020a). At present, only Methanosarcina and Methanosaeta have been found to produce methane by acetoclastic methanogenesis (Holmes and Smith, 2016; Li et al., 2018; Xiao et al., 2019a).
The second pathway for biomethane production is CO2 reduction using hydrogen/formic acid as indirect electron carriers. With the discovery of methane production through direct electron reduction, the theory of “electromethanogenesis” has been gradually recognized and named as direct interspecies electron transfer (DIET) (Morita et al., 2011; Xiao et al., 2018). Overall, direct electron transfer by conductive pili/protein and indirect electron transfer by H2/formate work together for CO2 reduction to generate biomethane. Third, methylotrophic methanogenesis reduces the methyl group of, e.g., methanol and methylamine, to methane (Yuan et al., 2019; Conrad, 2020); this process contributes only a small amount of methane production in bioengineering. Mustapha et al. (2016) showed the simultaneous functions and interactions of diverse bacteria and methanogenic archaea at different stages of the anaerobic digestion of waste-activated sludge. They found that the proportion of Caldilinea, Methanosarcina, and Clostridium is related to methane production trends after the exposure of azithromycin, chloramphenicol, and kanamycin. The effects on antibiotics are detailed in the next subsections and Table 3.
Macrolides
Macrolides mainly include roxithromycin, erythromycin and tylosin. Cetecioglu et al. (2012) showed that increasing erythromycin concentrations decreased methane production, possibly caused by reduced acetate utilization. Another study of metabolic transformations of VFAs showed that inhibition depended on the concentration of erythromycin (Cetecioglu et al., 2015). The inhibitory impact was variable with the initial erythromycin dose: at lower doses, the VFA mixture was removed entirely but partially utilized, leading to reduced biogas and methane generation, suggesting the analogy of uncompetitive inhibition.
Chen et al. (2020) showed that roxithromycin inhibits more methanogens than hydrolytic bacteria, thus resulting in an accumulation of VFAs. Exposure of roxithromycin was found to reduce the abundance of methanogenic archaea such as Methanoseata, Methanofastidiosum, and Methanolinea (Ni et al., 2020). Some studies demonstrated that methane production could be restored by prolonged duration of antibiotic action, e.g., using roxithromycin and erythromycin (Table 3). Tylosin has a high inhibitory effect on methane production after long-term action, and this inhibitory effect rises with tylosin concentration. Adaptation may also occur. Indeed, a low concentration of tylosin, 0.01 mg/L was enough to inhibit methane production for biomass that has not been previously in contact with tylosin; whereas no inhibition of methanogenesis was observed in digesters acclimated with 0.01–0.065 mg/L of tylosin (Garcia-Sanchez et al., 2016). Overall, macrolides decrease methane production by about 10–100% depending on the antibiotic concentration (Table 3).
Tetracyclines
Compared to macrolides, terramycin, tetracycline, and aureomycin belonging to tetracyclines showed similar tendency to suppress methane production. Terramycin, tetracycline and aureomycin tetracyclines do not inhibit methane production below 25 mg/L but inhibit methane production above 500 mg/L (Arikan et al., 2006; Tian et al., 2018). The inhibition of methane production by terramycin decreases with longer exposure time, even at higher concentrations, which is explained by the development of antibiotic resistance by the microbial community.
Temperature is controlling the impact of tetracyclines. For instance, terramycin reduced the cumulative methane production by 23.75% at 35°C (Arikan et al., 2006), while at 55°C, the same concentration of terramycin did not inhibit anaerobic digestion (Alonso et al., 2019). On the contrary, the inhibition of anaerobic digestion by tetracycline increases with temperature (Diehl and LaPara, 2010; Yi et al., 2016). Here, it is speculated that terramycin is likely to be hydrolyzed at higher temperature, thus in turn losing its inhibitory capacity. By compararison, tetracycline displays better thermal stability and is relatively more difficult to be hydrolyzed (Yi et al., 2016).
The type of tetracycline is also controlling the accumulation of VFAs. For instance, tetracycline induces acetate and butyrate accumulation during thermophilic anaerobic digestion, whereas terramycin does not (Alonso et al., 2019). This is important because many studies reveal that the accumulation of acids inhibit methanogenesis (Lee et al., 2017). Herein, VFAs as non-antibiotic factors may also lead to reduced methane accumulation in the presence of tetracycline. A possible reason for accumulated VFAs may be that methanogens are more vulnerable to tetracycline than acetogens and other bacteria. Thus inhibited methanogens may explain the under-performing AD process. Overall, tetracyclines decrease methane production by 0–90% depending on concentration, temperature and compound type (Table 3), and the development of antibiotic resistance previously observed for macrolides is confirmed.
β-Lactams
Several investigation show that β-lactams display inhibitory effects on anaerobic digestion (Guerra et al., 2014; Huang et al., 2018). Lu et al. (2014) showed that a lower concentration of cefalexin inhibits methane production during anaerobic digestion of activated sludge, whereas higher concentration had no significant effect on methane production. This behavior was explained by the fact that cefalexin addition induces the excretion of extracellular polymeric substances that form microbial protecting layers, thus providing a suitable environment for microbes’ growth and fermentation. Moreover, the long-term observation of the impact of cefalexin on organic substrate degradation and microbial community structure in a granular sludge bed system showed that the presence of cefalexin increased the soluble chemical oxygen demand (COD) and accumulated VFAs in the effluent of the system (Meng et al., 2017). Here, cefalexin also increased the proportion of Gelria and Syntrophorhabdus bacteria and fungi, and the functional diversity of archaea.
The effects of cefazolin on methane production are variable (Beneragama et al., 2013; Alonso et al., 2019). On one hand, the addition of cefazolin reduced methane production by 39.8–68.3% during the anaerobic digestion of cow manure (Lateef et al., 2018). On the other hand, cefazolin did not inhibit methane production using the same digestion substrate and similar treatment duration (Beneragama et al., 2013). Effects are also variable for penicillin. For instance, Masse et al. (2000) showed that methane production of anaerobic digestion of pig feces is reduced by 35% due to the presence of penicillin at a concentration of 16 mg/kg in the feed. Whereas, penicillin was found to stabilize the anaerobic digestion process of rain tree kernels and thus to facilitate the methane production (Viswanath and Nand, 1989). Overall, the addition of β-lactams modifies microbial communities and induce variable effects on methane production.
Antibiotic Mixtures
Antibiotics are usually occurring as mixtures in waste and contaminated ecosystems, thus calling for the study of the effect of antibiotic mixtures on microbial processes. For instance, Ozbayram et al. (2015) tested the effects of sulfamethoxazole-tetracycline, erythromycin-sulfamethoxazole, and erythromycin-tetracycline on methane production. They found that methane production is inhibited in reactors fed with erythromycin-sulfamethoxazole and sulfamethoxazole-tetracycline, while the mixture of erythromycin-tetracycline showed only a weak inhibition. Inhibition on acetate utilization and methane production followed similar trend, which suggested the potential effect of antibiotic combinations on acetate digestion.
Inhibition of methane production with three antibiotics together, e.g., erythromycin-sulfamethoxazole-tetracycline, was higher than that with erythromycin-sulfamethoxazole; but lower than that of sulfamethoxazole-tetracycline, and erythromycin-tetracycline (Aydin et al., 2015a,b; Ozbayram et al., 2015). As a consequence, tetracycline may have a synergistic effect with erythromycin and sulfamethoxazole, respectively, while the presence of erythromycin with the other two antibiotics may produce an antagonistic effect. In the same investigations, similar trends were observed for the effect of antibiotic mixtures on acetate consumption, implying that inhibition of methanogenesis is due to a reduction of acetate consumption.
A mixture of oxytetracycline and chlortetracycline reduced methane production by more than half, indicating a much stronger inhibition effect than a single antibiotic (Alvarez et al., 2010). Here, authors proposed that the stability of oxytetracycline and chlortetracycline was favored by their strong adsorption to solid matter. Christensen et al. (2006) also observed a major synergic effect of erythromycin and terramycin on methane production in activated sludge samples. Furthermore, a study reveals that the inhibition effect of terramycin and cefazolin-terramycin mixture on methane production follows nearly same inhibition pattern during the thermophilic anaerobic digestion of cow manure (Beneragama et al., 2013). Overall, experiments on antibiotic mixtures show both synergetic and antagonistic effects, depending on the type of antibiotics.
Effects of Antibiotics on Methanogenic Archaea
Methanogens are a class of exclusive anaerobic archaea that are widely distributed and can survive in lakes, marshes, sludges, wetlands, rice paddy soils, inside organisms, and even extreme environments (Xiao et al., 2017, 2019b,c; Conrad, 2020). The blocking of the methanogenic reaction leads to the accumulation of organic acids, and the upstream acidification reaction will be slowed down by product inhibition.
Lamberti et al. (2011) suggested that bacteria and archaea have similar ribosome structures, because of which the macrolides and tetracyclines can inhibit methanogenic archaea. Roxithromycin made a positive contribution to the production of VFAs (Ni et al., 2020). The potential mechanism is presented as follows: acetate kinase activity was increased while the activities of alpha-glucosidase and coenzyme F-420 were decreased by the addition of roxithromycin; methane production was significantly inhibited than the hydrolysis process, which heavily accumulated VFAs content in the system. Moreover, inhibition of the acidogenesis and methanogenesis in waste activated sludge anaerobic digestion was observed for roxithromycin, resulting in decreased methane production. Methanoseata, Methanofastidiosum, and Methanolinea were key methanogenic archaea members in these systems but their proportion decreased in the presence of roxithromycin (Ni et al., 2020).
Interestingly, the impact of tylosin on methanogens and/or methanogenic progress seems to be slight compared to other macrolides antibiotics (Chelliapan et al., 2011, 2014). Chelliapan et al. (2011) showed an adaption of methanogens to tylosin, where archaeal cells were not inhibited by tylosin at the concentrations between 100 and 400 mg/L and dominated the reactor. Especially, methanogenesis was not inhibited in the digesters acclimated with 0.01 to 0.065 mg/L tylosin, and methane production was increased (Garcia-Sanchez et al., 2016). It could be inferred that not only the resistance but also the metabolizing ability to antibiotics were developed by such microorganisms. Tylosin effects on manure degradation were limited as well (Stone et al., 2009). Thus the consumption of acetate and other C-1 VFA compounds, such as formate, during methanogenesis was sufficient even with tylosin in the system.
Like the inhibition mechanism on bacteria, terramycin significantly inhibits acetoclastic methanogenic archaea at low concentration, and further enhances the inhibition ability at high concentration (Arikan et al., 2006). In contrast to terramycin, at a low concentration of aureomycin (25 mg/L), acetoclastic methanogens are not affected. However, at concentrations higher than 200 mg/L, the consumption of acetate is halted, and the whole methanogenic progress is inhibited (Sanz et al., 1996). Some studies showed that tylosin has no noticeable effect on acetoclastic methanogens, but only inhibits the oxidation of propionate and butyrate at high concentration. Unlike tylosin, erythromycin directly inhibits acetate-based methane metabolism (Sanz et al., 1996; Zhang and Li, 2018).
Cefalexin, one of the β-lactams, mainly affects methane production by inhibiting acetoclastic methanogens, thus accumulating VFAs (Lu et al., 2014). With the extension of cefalexin action duration, methanogenic archaea and anaerobic fermenting bacteria can adapt, and methane production tends to be restored. However, there is only a partial recovery in the methane production due to excessive cefalexin (Lu et al., 2014). The decline in the inhibitory effect of cefazolin in the later stage of the reaction might be due to the cefazolin which is easy to degrade under thermophilic environments (Fabre et al., 1994).
Perspective and Conclusion
Antibiotic residues in sludge treatment systems often adversely affect the anaerobic digestion process, depending on conditions, antibiotic nature and antibiotic concentration. Research progress and knowledge in this field have been significantly enriched in the past ten years. Based on the current progress, some rules of antibiotics’ impacts on anaerobic digestion are summarized as follows:
(1) The presence of antibiotics generally lead to the accumulation of VFAs in the anaerobic fermentation system. However, different types of antibiotics or even different antibiotics of the same type have different influences on the anaerobic digestion process, the methane-production capacity, and the related microbial community.
(2) Besides the type of antibiotics, the concentrations, the duration of action, and the temperature of the anaerobic digestion system altogether considered to be the key factors affecting the effects of antibiotics on anaerobic digestion and methane production.
(3) The short-term and long-term effects of antibiotics show certain differences. Short-term experiments may be difficult to accurately reflect the potential effects of antibiotics on complex microbial consortia due to the lack of microbial adaptation. Short-term experiments ignore the long-term effects on the growth of anaerobic microbes and the adaptability of microbes to antibiotics. Thus, long-term observation and comprehensive analysis are to be given topmost priority. In the later stage of the anaerobic digestion reaction, some antibiotics lose their bacteriostatic effects. The possible reasons for such happenings are as follows: (1). the hydrolysis reaction of antibiotics is natural to occur; (2). bacteria and methanogens have developed tolerance to specific concentrations of antibiotics.
Antibiotics in sludge are generally not present alone, they are usually accompanied by other antibiotics. However, current research mainly focuses on a single antibiotic and its inhibition effect on anaerobic digestion. The lack of exploration and summary of mixed antibiotics’ impact on the anaerobic digestion system results in an incomprehensive knowledge on the joint effect of multiple antibiotics. Thus, it may not be instructive to practice under anaerobic conditions for wastewater and/or diverse antibiotic-enriched sludge treatment.
The effects of single or multiple antibiotics on anaerobic digestion of sludge depend not only on the antibiotics themselves but also on the sludge substrates, the microbial community composition, the biological and non-biological degradation of antibiotics, and the adsorption of antibiotics. These factors need to be further evaluated and will be the focus of future research. As environmental pollutants, ARGs require to be comprehensively studied in anaerobic fermentative environments, which is indispensable to characterize the impact of antibiotics. In contrast, the current techniques can only cultivate a small proportion of the anaerobic fermentative system members, which severely restrained our knowledge of the bacterial meta-resistome in various environments. Moreover, it wasn’t yet well documented the distribution of ARGs and antibiotic-producers and the effects of antibiotics on anaerobic digestion.
As a non-culture-based method, metagenomics becomes a vital tool to comprehend the bacterial communities sufficiently. Various omics techniques are more and more applied in understanding the effect of antibiotics on microbial communities, synthesizing new antimicrobial compounds, and analyzing the antibiotic resistance genes’ distribution in different anaerobic systems. For example, functional metagenomics can be applied in identifying novel antibiotic resistance genes, and descriptive metagenomics can be used for analyzing the composition of the microbial communities and catching the proportion of known antibiotic resistance genes (Garmendia et al., 2012; Gupta et al., 2020). Furthermore, metatranscriptome becomes other powerful analyzing tools for identifying ARGs and assessing the effects of antibiotics on the environment (Asante and Sekyere, 2019). Consequently, the application of omics methods will bring revolutionary improvements to the study of anaerobic digestion and methanogenic performance affected by antibiotics.
Author Contributions
LX, YW, ZL, PK, JL, DF, QY, and FL collected the data. LX, EL, PK, and DF analyzed the data and wrote this manuscript. All authors contributed to the article and approved the submitted version.
Funding
This research was financially supported by the National Natural Science Foundation of China (no. 42077025), Research project of ecological environment protection and restoration of Yangtze River in Zhoushan (SZGXZS2020068), and the Key Laboratory of Wet Subtropical Geographical Process, Ministry of Education, Fujian Normal University.
Conflict of Interest
The authors declare that the research was conducted in the absence of any commercial or financial relationships that could be construed as a potential conflict of interest.
Acknowledgments
We are very grateful to the three reviewers for their contributions to improving the quality of this paper.
References
Alamo, A. C., Gonzalez, C., Pariente, M. I., Molina, R., and Martinez, F. (2020). Fenton-like catalyst based on a reticulated porous perovskite material: activity and stability for the on-site removal of pharmaceutical micropollutans in a hospital wastewater. Chem. Eng. J. 401:126113. doi: 10.1016/j.cej.2020.126113
Alonso, L. L., Demetrio, P. M., Capparelli, A. L., and Marino, D. J. G. (2019). Behavior of ionophore antibiotics in aquatic environments in Argentina: the distribution on different scales in water courses and the role of wetlands in depuration. Environ. Int. 133:105144. doi: 10.1016/j.envint.2019.105144
Alvarez, J. A., Otero, L., Lema, J. M., and Omil, F. (2010). The effect and fate of antibiotics during the anaerobic digestion of pig manure. Bioresour. Technol. 101, 8581–8586. doi: 10.1016/j.biortech.2010.06.075
Amin, M. M., Zilles, J. L., Greiner, J., Charbonneau, S., Raskin, L., and Morgenroth, E. (2006). Influence of the antibiotic erythromycin on anaerobic treatment of a pharmaceutical wastewater. Environ. Sci. Technol. 40, 3971–3977. doi: 10.1021/es060428j
Arikan, O. A., Sikora, L. J., Mulbry, W., Khan, S. U., Rice, C., and Foster, G. D. (2006). The fate and effect of oxytetracycline during the anaerobic digestion of manure from therapeutically treated calves. Process Biochem. 41, 1637–1643. doi: 10.1016/j.procbio.2006.03.010
Asante, J., and Sekyere, J. O. (2019). Understanding antimicrobial discovery and resistance from a metagenomic and metatranscriptomic perspective: advances and applications. Environ. Microbiol. Rep. 11, 62–86. doi: 10.1111/1758-2229.12735
Aydin, S., Cetecioglu, Z., Arikan, O., Ince, B., Ozbayram, E. G., and Ince, O. (2015a). Inhibitory effects of antibiotic combinations on syntrophic bacteria, homoacetogens and methanogens. Chemosphere 120, 515–520. doi: 10.1016/j.chemosphere.2014.09.045
Aydin, S., Ince, B., and Ince, O. (2015b). Application of real-time PCR to determination of combined effect of antibiotics on Bacteria. Methanogenic Archaea. Archaea in anaerobic sequencing batch reactors. Water Res. 76, 88–98. doi: 10.1016/j.watres.2015.02.043
Beneragama, N., Lateef, S. A., Iwasaki, M., Yamashiro, T., and Umetsu, K. (2013). The combined effect of cefazolin and oxytertracycline on biogas production from thermophilic anaerobic digestion of dairy manure. Bioresour. Technol. 133, 23–30. doi: 10.1016/j.biortech.2013.01.032
Broughton, E. I., and Walker, D. G. (2010). Policies and practices for aquaculture food safety in China. Food Policy 35, 471–478. doi: 10.1016/j.foodpol.2010.05.007
Carlsson, M., Lagerkvist, A., and Morgan-Sagastume, F. (2012). The effects of substrate pre-treatment on anaerobic digestion systems: a review. Waste Manage. 32, 1634–1650. doi: 10.1016/j.wasman.2012.04.016
Carneiro, R. B., Gonzalez-Gil, L., Londono, Y. A., Zaiat, M., Carballa, M., and Lema, J. M. (2020). Acidogenesis is a key step in the anaerobic biotransformation of organic micropollutants. J. Hazard. Mater. 389:121888. doi: 10.1016/j.jhazmat.2019.121888
Cetecioglu, Z., Ince, B., Ince, O., and Orhon, D. (2015). Acute effect of erythromycin on metabolic transformations of volatile fatty acid mixture under anaerobic conditions. Chemosphere 124, 129–135. doi: 10.1016/j.chemosphere.2014.12.004
Cetecioglu, Z., Ince, B., Orhon, D., and Ince, O. (2012). Acute inhibitory impact of antimicrobials on acetoclastic methanogenic activity. Bioresour. Technol. 114, 109–116. doi: 10.1016/j.biortech.2012.03.020
Chelliapan, S., Wilby, T., Sallis, P. J., and Yuzir, A. (2011). Tolerance of the antibiotic Tylosin on treatment performance of an Up-flow Anaerobic Stage Reactor (UASR). Water Sci. Technol. 63, 1599–1606. doi: 10.2166/wst.2011.222
Chelliapan, S., Yuzir, A., Din, M. F. M., Anuar, A. N., Ponraj, M., and Sallis, P. J. (2014). Impact of hydraulic retention time on the performance and archaea populations of an anaerobic reactor treating synthetic Tylosin wastewater.Desalin. Water Treat. 52, 3647–3653. doi: 10.1080/19443994.2013.854037
Chen, H. B., Zeng, X. N., Zhou, Y. Y., Yang, X., Lam, S. S., and Wang, D. B. (2020). Influence of roxithromycin as antibiotic residue on volatile fatty acids recovery in anaerobic fermentation of waste activated sludge. J. Hazard. Mater. 394:122570. doi: 10.1016/j.jhazmat.2020.122570
Chen, Q. L., An, X. L., Li, H., Su, J. Q., Ma, Y. B., and Zhu, Y. G. (2016). Long-term field application of sewage sludge increases the abundance of antibiotic resistance genes in soil. Environ. Int. 9, 1–10. doi: 10.1016/j.envint.2016.03.026
Chen, Y., Cheng, J. J., and Creamer, K. S. (2008). Inhibition of anaerobic digestion process: a review. Bioresour. Technol. 99, 4044–4064. doi: 10.1016/j.biortech.2007.01.057
Cheng, D. L., Ngo, H. H., Guo, W. S., Chang, S. W., Nguyen, D. D., Kumar, S. M., et al. (2018). Problematic effects of antibiotics on anaerobic treatment of swine wastewater. Bioresour. Technol. 263, 642–653. doi: 10.1016/j.biortech.2018.05.010
Christensen, A. M., Ingerslev, F., and Baun, A. (2006). Ecotoxicity of mixtures of antibiotics used in aquacultures. Environ. Toxicol. Chem. 25, 2208–2215. doi: 10.1897/05-415r.1
Conrad, R. (2020). Importance of hydrogenotrophic, aceticlastic and methylotrophic methanogenesis for methane production in terrestrial, aquatic and other anoxic environments: a mini review. Pedosphere. 30, 25–39. doi: 10.1016/s1002-0160(18)60052-9
Diehl, D. L., and LaPara, T. M. (2010). Effect of temperature on the fate of genes encoding tetracycline resistance and the integrase of class 1 integrons within anaerobic and aerobic digesters treating municipal wastewater solids. Environ. Sci. Technol. 44, 9128–9133. doi: 10.1021/es102765a
Fabre, H., Ibork, H., and Lerner, D. A. (1994). Photoisomerization kinetics of cefuroxime axetil and related-compounds. J. Pharm. Sci. 83, 553–558. doi: 10.1002/jps.2600830422
Garcia-Sanchez, L., Garzon-Zuniga, M. A., Buelna, G., and Estrada-Arriaga, E. B. (2016). Tylosin effect on methanogenesis in an anaerobic biomass from swine wastewater treatment. Water Sci. Technol. 73, 445–452. doi: 10.2166/wst.2015.507
Garmendia, L., Hernandez, A., Sanchez, M. B., and Martinez, J. L. (2012). Metagenomics and antibiotics. Clin. Microbiol. Infect. 18, 27–31. doi: 10.1111/j.1469-0691.2012.03868.x
Gonzalez, A., Hendriks, A. T. W. M., van Lier, J. B., and de Kreuk, M. (2018). Pre-treatments to enhance the biodegradability of waste activated sludge: elucidating the rate limiting step. Biotechnol. Adv. 36, 1434–1469. doi: 10.1016/j.biotechadv.2018.06.001
Guerra, P., Kim, M., Shah, A., Alaee, M., and Smyth, S. A. (2014). Occurrence and fate of antibiotic, analgesic/anti-inflammatory, and antifungal compounds in five wastewater treatment processes. Sci. Total Environ. 473, 235–243. doi: 10.1016/j.scitotenv.2013.12.008
Gupta, C. L., Tiwari, R. K., and Cytryn, E. (2020). Platforms for elucidating antibiotic resistance in single genomes and complex metagenomes. Environ. Int. 138:105667. doi: 10.1016/j.envint.2020.105667
Gurmessa, B., Pedretti, E. F., Cocco, S., Cardelli, V., and Corti, G. (2020). Manure anaerobic digestion effects and the role of pre- and post-treatments on veterinary antibiotics and antibiotic resistance genes removal efficiency. Sci. Total Environ. 721:137532. doi: 10.1016/j.scitotenv.2020.137532
Holmes, D. E., and Smith, J. A. (2016). Biologically produced methane as a renewable energy source. Adv. Appl. Microbiol. 97, 1–61. doi: 10.1016/bs.aambs.2016.09.001
Huang, B., Wang, H. C., Cui, D., Zhang, B., Chen, Z. B., and Wang, A. J. (2018). Treatment of pharmaceutical wastewater containing beta-lactams antibiotics by a pilot-scale anaerobic membrane bioreactor (AnMBR). Chem. Eng. J. 341, 238–247. doi: 10.1016/j.cej.2018.01.149
Huang, X. D., Xu, Q. X., Wu, Y. X., Wang, D. B., Yang, Q., Chen, F., et al. (2019). Effect of clarithromycin on the production of volatile fatty acids from waste activated sludge anaerobic fermentation. Bioresour. Technol. 288:121598. doi: 10.1016/j.biortech.2019.121598
Ju, F., Li, B., Ma, L. P., Wang, Y. B., Huang, D. P., and Zhang, T. (2016). Antibiotic resistance genes and human bacterial pathogens: Co-occurrence, removal, and enrichment in municipal sewage sludge digesters. Water Res. 91, 1–10. doi: 10.1016/j.watres.2015.11.071
Kohanski, M. A., Dwyer, D. J., Hayete, B., Lawrence, C. A., and Collins, J. J. (2007). A common mechanism of cellular death induced by bactericidal antibiotics. Cell 130, 797–810. doi: 10.1016/j.cell.2007.06.049
Kovalakova, P., Cizmas, L., McDonald, T. J., Marsalek, B., Feng, M. B., and Sharma, V. K. (2020). Occurrence and toxicity of antibiotics in the aquatic environment: a review. Chemosphere 251:126351. doi: 10.1016/j.chemosphere.2020.126351
Lamberti, A., Martucci, N. M., Ruggiero, I., Arcari, P., and Masullo, M. (2011). Interaction between the antibiotic tetracycline and the elongation factor 1 alpha from the archaeon Sulfolobus solfataricus. Chem. Biol. Drug Des. 78, 260–268. doi: 10.1111/j.1747-0285.2011.01142.x
LaPara, T. M., Burch, T. R., McNamara, P. J., Tan, D. T., Yan, M., Eichmiller, J. J., et al. (2011). Tertiary-treated municipal wastewater is a significant point source of antibiotic resistance genes into duluth-superior harbor. Environ. Sci. Technol. 45, 9543–9549. doi: 10.1021/es202775r
Larson, C. (2015). Pharmaceuticals China’s lakes of pig manure spawn antibiotic resistance. Science 347:704. doi: 10.1126/science.347.6223.704
Lateef, S. A., Iwasaki, M., Yamashiro, T., and Umetsu, K. (2018). Influence of cefazolin contamination on performance of two-stage and single stage anaerobic batch digesters. Energy Sustain. Dev. 44, 117–124. doi: 10.1016/j.esd.2018.03.009
Lee, L. J., Lee, Y. S., and Han, G. B. (2017). Effect of two-step thermo-disintegration on the enhancing solubilization and anaerobic biodegradability of excess waste sludge. Chem. Eng. J. 317, 742–750. doi: 10.1016/j.cej.2017.02.123
Li, J. J., Xiao, L. L., Zheng, S. L., Zhang, Y. C., Luoc, M., Tong, C., et al. (2018). A new insight into the strategy for methane production affected by conductive carbon cloth in wetland soil: Beneficial to acetoclastic methanogenesis instead of CO2 reduction. Sci. Total Environ. 643, 1024–1030. doi: 10.1016/j.scitotenv.2018.06.271
Li, N., Liu, H. J., Xue, Y. G., Wang, H. Y., and Dai, X. H. (2017). Partition and fate analysis of fluoroquinolones in sewage sludge during anaerobic digestion with thermal hydrolysis pretreatment. Sci. Total Environ. 581, 715–721. doi: 10.1016/j.scitotenv.2016.12.188
Li, W. H., Shi, Y. L., Gao, L. H., Liu, J. M., and Cai, Y. Q. (2013). Occurrence, distribution and potential affecting factors of antibiotics in sewage sludge of wastewater treatment plants in China. Sci. Total Environ. 445, 306–313. doi: 10.1016/j.scitotenv.2012.12.050
Liu, L. M., Su, J. Q., Guo, Y. Y., Wilkinson, D. M., Liu, Z. W., Zhu, Y. G., et al. (2018). Large-scale biogeographical patterns of bacterial antibiotic resistome in the waterbodies of China. Environ. Int. 117, 292–299. doi: 10.1016/j.envint.2018.05.023
Lu, X. Q., Zhen, G. Y., Liu, Y., Hojo, T., Estrada, A. L., Li, Y. Y., et al. (2014). Long-term effect of the antibiotic cefalexin on methane production during waste activated sludge anaerobic digestion. Bioresour. Technol. 169, 644–651. doi: 10.1016/j.biortech.2014.07.056
Luo, L. Y., Zhang, C. J., Zhang, Z., Peng, J., Han, Y. Q., Wang, P., et al. (2020). Differences in tetracycline antibiotic resistance genes and microbial community structure during aerobic composting and anaerobic digestion. Front. Microbiol. 11:583995. doi: 10.3389/fmicb.2020.583995
Masse, D. I., Lu, D., Masse, L., and Droste, R. L. (2000). Effect of antibiotics on psychrophilic anaerobic digestion of swine manure slurry in sequencing batch reactors. Bioresour. Technol. 75, 205–211. doi: 10.1016/s0960-8524(00)00046-8
Mata-Alvarez, J., Mace, S., and Llabres, P. (2000). Anaerobic digestion of organic solid wastes, An overview of research achievements and perspectives. Bioresour. Technol. 74, 3–16. doi: 10.1016/s0960-8524(00)00023-7
Mehariya, S., Patel, A. K., Obulisamy, P. K., Punniyakotti, E., and Wong, J. W. C. (2018). Co-digestion of food waste and sewage sludge for methane production: Current status and perspective. Bioresour. Technol. 265, 519–531. doi: 10.1016/j.biortech.2018.04.030
Meng, L. W., Li, X. K., Wang, S. T., Liu, L. L., Ma, K. L., and Zhang, J. (2017). The long-term impact of cefalexin on organic substrate degradation and microbial community structure in EGSB system. Chemosphere 184, 215–223. doi: 10.1016/j.chemosphere.2017.05.171
Miritana, V. M., Massini, G., Visca, A., Grenni, P., Patrolecco, L., Spataro, F., et al. (2020). Effects of sulfamethoxazole on the microbial community dynamics during the anaerobic digestion process. Front. Microbiol. 11:537783. doi: 10.3389/fmicb.2020.537783
Morita, M., Malvankar, N. S., Franks, A. E., Summers, Z. M., Giloteaux, L., Rotaru, A. E., et al. (2011). Potential for direct interspecies electron transfer in methanogenic wastewater digester aggregates. mBio 2:e00159-11.
Mustapha, N. A., Hu, A. Y., Yu, C. P., Sharuddin, S. S., Ramli, N., Shirai, Y., et al. (2018). Seeking key microorganisms for enhancing methane production in anaerobic digestion of waste sewage sludge. Appl. Microbiol. Biotechnol. 102, 5323–5334. doi: 10.1007/s00253-018-9003-8
Mustapha, N. A., Sakai, K., Shirai, Y., and Maeda, T. (2016). Impact of different antibiotics on methane production using waste-activated sludge: mechanisms and microbial community dynamics. Appl. Microbiol. Biotechnol. 100, 9355–9364. doi: 10.1007/s00253-016-7767-2
Ni, B. J., Zeng, S. T., Wei, W., Dai, X. H., and Sun, J. (2020). Impact of roxithromycin on waste activated sludge anaerobic digestion: methane production, carbon transformation and antibiotic resistance genes. Sci. Total Environ. 703:134899. doi: 10.1016/j.scitotenv.2019.134899
Ozbayram, E. G., Arikan, O., Ince, B., Cetecioglu, Z., Aydin, S., and Ince, O. (2015). Acute effects of various antibiotic combinations on acetoclastic methanogenic activity. Environ. Sci. Pollut. Res. 22, 6230–6235. doi: 10.1007/s11356-014-3841-4
Pala-Ozkok, I., and Orhon, D. (2013). Chronic effect of erythromycin on substrate biodegradation kinetics of activated sludge. Biochem. Eng. J. 81, 29–39. doi: 10.1016/j.bej.2013.10.002
Pei, J., Yao, H., Wang, H., Shan, D., Jiang, Y. C., Ma, L. Q. Y., et al. (2015). Effect of ultrasonic and ozone pre-treatments on pharmaceutical waste activated sludge’s solubilisation, reduction, anaerobic biodegradability and acute biological toxicity. Bioresour. Technol. 192, 418–423. doi: 10.1016/j.biortech.2015.05.079
Qiao, M., Ying, G. G., Singer, A. C., and Zhu, Y. G. (2018). Review of antibiotic resistance in China and its environment. Environ. Int. 110, 160–172. doi: 10.1016/j.envint.2017.10.016
Rusanowska, P., Harnisz, M., Zielinski, M., Debowski, M., Korzeniewska, E., Kisielewska, M., et al. (2020). Individual and synergistic effects of metronidazole, amoxicillin, and ciprofloxacin on methane fermentation with sewage sludge. Clean Soil Air Water. 48:1900281. doi: 10.1002/clen.201900281
Sanz, J. L., Rodriguez, N., and Amils, R. (1996). The action of antibiotics on the anaerobic digestion process. Appl. Microbiol. Biotechnol. 46, 587–592. doi: 10.1007/s002530050865
Shimada, T., Li, X., Zilles, J. L., Morgenroth, E., and Raskin, L. (2011). Effects of the antimicrobial tylosin on the microbial community structure of an anaerobic sequencing batch reactor. Biotechnol. Bioeng. 108, 296–305. doi: 10.1002/bit.22934
Shin, J., Rhee, C., Shin, J., Jang, H. M., Shin, S. G., and Kim, Y. M. (2020). Determining the composition of bacterial community and relative abundance of specific antibiotics resistance genes via thermophilic anaerobic digestion of sewage sludge. Bioresour. Technol. 311:123510. doi: 10.1016/j.biortech.2020.123510
Sim, W. J., Lee, J. W., Lee, E. S., Shin, S. K., Hwang, S. R., and Oh, J. E. (2011). Occurrence and distribution of pharmaceuticals in wastewater from households, livestock farms, hospitals and pharmaceutical manufactures. Chemosphere 82, 179–186. doi: 10.1016/j.chemosphere.2010.10.026
Stone, J. J., Clay, S. A., Zhu, Z. W., Wong, K. L., Porath, L. R., and Spellman, G. M. (2009). Effect of antimicrobial compounds tylosin and chlortetracycline during batch anaerobic swine manure digestion. Water Res. 43, 4740–4750. doi: 10.1016/j.watres.2009.08.005
Sun, C. X., Li, W., Chen, Z., Qin, W. T., and Wen, X. H. (2019). Responses of antibiotics, antibiotic resistance genes, and mobile genetic elements in sewage sludge to thermal hydrolysis pre-treatment and various anaerobic digestion conditions. Environ. Int. 133:105156. doi: 10.1016/j.envint.2019.105156
Tao, Z. T., Wang, D. B., Yao, F. B., Huang, X. D., Wu, Y., Du, M. T., et al. (2020). The effects of thiosulfinates on methane production from anaerobic co-digestion of waste activated sludge and food waste and mitigate method. J. Hazard. Mater 384:121363. doi: 10.1016/j.jhazmat.2019.121363
Tian, Z., Zhang, Y., and Yang, M. (2018). Chronic impacts of oxytetracycline on mesophilic anaerobic digestion of excess sludge: Inhibition of hydrolytic acidification and enrichment of antibiotic resistome. Environ. Pollut. 238, 1017–1026. doi: 10.1016/j.envpol.2018.02.023
Van Boeckel, T. P., Brower, C., Gilbert, M., Grenfell, B. T., Levin, S. A., Robinson, T. P., et al. (2015). Global trends in antimicrobial use in food animals. Proc. Natl. Acad. Sci. U.S.A. 112, 5649–5654.
Vester, B., and Douthwaite, S. (2001). Macrolide resistance conferred by base substitutions in 23S rRNA. Antimicrob. Agents Chemother. 45, 1–12. doi: 10.1128/aac.45.1.1-12.2001
Viswanath, P., and Nand, K. (1989). Influence of penicillin on the stabilization of anaerobic-digestion of rain tree kernels for methane generation. J. Ferment. Bioeng. 68, 154–156. doi: 10.1016/0922-338x(89)90067-6
Walsh, C. (2000). Molecular mechanisms that confer antibacterial drug resistance. Nature 406, 775–781. doi: 10.1038/35021219
Wang, Q., Wang, P. L., and Yang, Q. X. (2018). Occurrence and diversity of antibiotic resistance in untreated hospital wastewater. Sci. Total Environ. 621, 990–999. doi: 10.1016/j.scitotenv.2017.10.128
Wei, R. C., Ge, F., Huang, S. Y., Chen, M., and Wang, R. (2011). Occurrence of veterinary antibiotics in animal wastewater and surface water around farms in Jiangsu Province, China. Chemosphere 82, 1408–1414. doi: 10.1016/j.chemosphere.2010.11.067
Welte, C., and Deppenmeier, U. (2014). Bioenergetics and anaerobic respiratory chains of aceticlastic methanogens. Bioenergetics 1837, 1130–1147. doi: 10.1016/j.bbabio.2013.12.002
Xiao, L., Li, J., Lichtfouse, E., Li, Z., Wang, Q., Liu, F., et al. (2021). Augmentation of chloramphenicol degradation by Geobacter-based biocatalysis and electric field. J. Hazard. Mater. 409:124977. doi: 10.1016/j.jhazmat.2020.124977
Xiao, L., Zheng, S., Lichtfouse, E., Luo, M., Tan, Y., and Liu, F. (2020b). Carbon nanotubes accelerate acetoclastic methanogenesis: From pure cultures to anaerobic soils. Soil Biol. Biochem. 150:107938. doi: 10.1016/j.soilbio.2020.107938
Xiao, L., Liu, F. H., Lichtfouse, E., Zhang, P., Feng, D. W., and Li, F. B. (2020a). Methane production by acetate dismutation stimulated by Shewanella oneidensis and carbon materials: an alternative to classical CO2 reduction. Chem. Eng. J. 389:124469. doi: 10.1016/j.cej.2020.124469
Xiao, L. L., Liu, F. H., Liu, J. C., Li, J. J., Zhang, Y. C., Yu, J. F., et al. (2018). Nano-Fe3O4 particles accelerating electromethanogenesis on an hour-long timescale in wetland soil. Environ. Sci. Nano 5, 436–445. doi: 10.1039/c7en00577f
Xiao, L. L., Liu, F. H., Xu, H. D., Feng, D. W., Liu, J. C., Han, G., et al. (2019a). Biochar promotes methane production at high acetate concentrations in anaerobic soils. Environ. Chem. Lett. 17, 1347–1352. doi: 10.1007/s10311-019-00863-3
Xiao, L. L., Sun, R., Zhang, P., Zheng, S. L., Tan, Y., Li, J. J., et al. (2019b). Simultaneous intensification of direct acetate cleavage and CO2 reduction to generate methane by bioaugmentation and increased electron transfer. Chem. Eng. J. 378:122229. doi: 10.1016/j.cej.2019.122229
Xiao, L. L., Wei, W. C., Luo, M., Xu, H. D., Feng, D. W., Yu, J. F., et al. (2019c). A potential contribution of a Fe(III)-rich red clay horizon to methane release: biogenetic magnetite-mediated methanogenesis. Catena 181:104081. doi: 10.1016/j.catena.2019.104081
Xiao, L. L., Xie, B. H., Liu, J. C., Zhang, H. X., Han, G. X., Wang, O. M., et al. (2017). Stimulation of long-term ammonium nitrogen deposition on methanogenesis by Methanocellaceae in a coastal wetland. Sci. Total Environ. 595, 337–343. doi: 10.1016/j.scitotenv.2017.03.279
Yadav, V., and Kumar, T. S. (2020). Present scenario of antibiotic use in veterinary practice: importance of wastewater microbiology. Curr. Dev. Biotechnol. Bioeng. 9, 279–325. doi: 10.1016/b978-0-12-819722-6.00009-2
Yang, Y., Huang, W. L., and Huang, W. W. (2019). Antibiotic inhibition on anaerobic digestion of animal manure and controlling strategies: a short review. Clean Soil Air Water. 47:201700653.
Yi, Q. Z., Gao, Y. X., Zhang, H., Zhang, H. F., Zhang, Y., and Yang, M. (2016). Establishment of a pretreatment method for tetracycline production wastewater using enhanced hydrolysis. Chem. Eng. J. 300, 139–145. doi: 10.1016/j.cej.2016.04.120
Yu, H., Zhao, Q. L., Meng, F. C., Ruan, L. Y., Sun, T. T., Liu, X. N., et al. (2020). Deciphering the role of calcium peroxide on the fate of antibiotic resistance genes and mobile genetic elements during bioelectrochemically-assisted anaerobic composting of excess dewatered sludge. Chem. Eng. J. 397:125355. doi: 10.1016/j.cej.2020.125355
Yuan, J. J., Liu, D. Y., Ji, Y., Xiang, J., Lin, Y. X., Wu, M., et al. (2019). Spartina alterniflora invasion drastically increases methane production potential by shifting methanogenesis from hydrogenotrophic to methylotrophic pathway in a coastal marsh. J. Ecol. 107, 2436–2450. doi: 10.1111/1365-2745.13164
Zhang, Q. Q., Ying, G. G., Pan, C. G., Liu, Y. S., and Zhao, J. L. (2015). Comprehensive evaluation of antibiotics emission and fate in the river basins of China: source Analysis, multimedia modeling, and linkage to bacterial resistance. Environ. Sci. Technol. 49, 6772–6782. doi: 10.1021/acs.est.5b00729
Zhang, X. Y., and Li, R. Y. (2018). Variation of antibiotics in sludge pretreatment and anaerobic digestion processes: degradation and solid-liquid distribution. Bioresour. Technol. 255, 266–272. doi: 10.1016/j.biortech.2018.01.100
Keywords: antibiotics, anaerobic digestion, biomethane, fermentative bacteria, methanogenic archaea
Citation: Xiao L, Wang Y, Lichtfouse E, Li Z, Kumar PS, Liu J, Feng D, Yang Q and Liu F (2021) Effect of Antibiotics on the Microbial Efficiency of Anaerobic Digestion of Wastewater: A Review. Front. Microbiol. 11:611613. doi: 10.3389/fmicb.2020.611613
Received: 07 October 2020; Accepted: 16 December 2020;
Published: 28 January 2021.
Edited by:
Stefano Campanaro, University of Padua, ItalyReviewed by:
Miao Yan, Technical University of Denmark, DenmarkAlessandra Fontana, Catholic University of the Sacred Heart, Piacenza, Italy
Xinyu Zhu, Technical University of Denmark, Denmark
Copyright © 2021 Xiao, Wang, Lichtfouse, Li, Kumar, Liu, Feng, Yang and Liu. This is an open-access article distributed under the terms of the Creative Commons Attribution License (CC BY). The use, distribution or reproduction in other forums is permitted, provided the original author(s) and the copyright owner(s) are credited and that the original publication in this journal is cited, in accordance with accepted academic practice. No use, distribution or reproduction is permitted which does not comply with these terms.
*Correspondence: P. Senthil Kumar, c2VudGhpbGNoZW04NTgyQGdtYWlsLmNvbQ==; Dawei Feng, ZHdmZW5nQHlpYy5hYy5jbg==
†ORCID: Eric Lichtfouse, orcid.org/0000-0002-8535-8073