- 1Department of Biomedical Engineering, Vanderbilt University, Nashville, TN, United States
- 2Department of Pediatrics, Division of Pediatric Infectious Diseases, Vanderbilt University Medical Center, Nashville, TN, United States
- 3Department of Pathology, Microbiology, and Immunology, Vanderbilt University Medical Center, Nashville, TN, United States
- 4Vanderbilt Center for Bone Biology, Vanderbilt University Medical Center, Nashville, TN, United States
- 5Vanderbilt Institute for Infection, Immunology, and Inflammation (VI4), Vanderbilt University Medical Center, Nashville, TN, United States
Staphylococcus aureus is a Gram-positive bacterium capable of infecting nearly all host tissues, causing severe morbidity and mortality. Widespread antimicrobial resistance has emerged among S. aureus clinical isolates, which are now the most frequent causes of nosocomial infection among drug-resistant pathogens. S. aureus produces an array of virulence factors that enhance in vivo fitness by liberating nutrients from the host or evading host immune responses. Staphylococcal virulence factors have been identified as viable therapeutic targets for treatment, as they contribute to disease pathogenesis, tissue injury, and treatment failure. Antivirulence strategies, or treatments targeting virulence without direct toxicity to the inciting pathogen, show promise as an adjunctive therapy to traditional antimicrobials. This Mini Review examines recent research on S. aureus antivirulence strategies, with an emphasis on translational studies. While many different virulence factors have been investigated as therapeutic targets, this review focuses on strategies targeting three virulence categories: pore-forming toxins, immune evasion mechanisms, and the S. aureus quorum sensing system. These major areas of S. aureus antivirulence research demonstrate broad principles that may apply to other human pathogens. Finally, challenges of antivirulence research are outlined including the potential for resistance, the need to investigate multiple infection models, and the importance of studying antivirulence in conjunction with traditional antimicrobial treatments.
Introduction
Staphylococcus aureus, a Gram-positive bacterium, asymptomatically colonizes approximately 30% of the population and can infect nearly every tissue in the body (Wertheim et al., 2005; Monaco et al., 2017). S. aureus readily adapts its metabolic and virulence responses in different tissues, causing superficial (e.g., folliculitis) and invasive infections (e.g., osteomyelitis; Balasubramanian et al., 2017; Potter et al., 2020). S. aureus biofilm formation, toxin production, and immune evasion strategies limit host antibacterial immune responses (Thammavongsa et al., 2015a; Muthukrishnan et al., 2019). Therefore, staphylococcal infections often necessitate long-term antibiotics (Lew and Waldvogel, 2004; Hatzenbuehler and Pulling, 2011). However, widespread antimicrobial resistance has highlighted the need to develop additional treatments (Ventola, 2015).
Staphylococcus aureus is the leading cause of nosocomial infections among antibiotic-resistant organisms, making staphylococcal infections a major target for investigation of antivirulence therapies (Sievert et al., 2013; Dickey et al., 2017). For the purposes of this review, antivirulence therapies are defined as those that do not inhibit bacterial growth in vitro but limit the production or function of virulence factors that promote infection or incite host damage in vivo. Antivirulence strategies aim to mitigate host tissue damage as host immune responses or conventional antimicrobials eradicate infection.
The number of published studies on antivirulence techniques has increased dramatically over the last decade (Maura et al., 2016; Dickey et al., 2017). While most antivirulence strategies for staphylococcal disease are currently preclinical, several antibody-based antivirulence approaches and one immunomodulatory peptide (NCT02469857, ClinicalTrials.gov [Internet], 2015) are in clinical trials (Huynh et al., 2016; Levy et al., 2016; François et al., 2018, 2019; Magyarics et al., 2019). This review will focus on recent investigations into antivirulence strategies targeting key virulence mechanisms of S. aureus as outlined in Figure 1 and listed in Table 1.
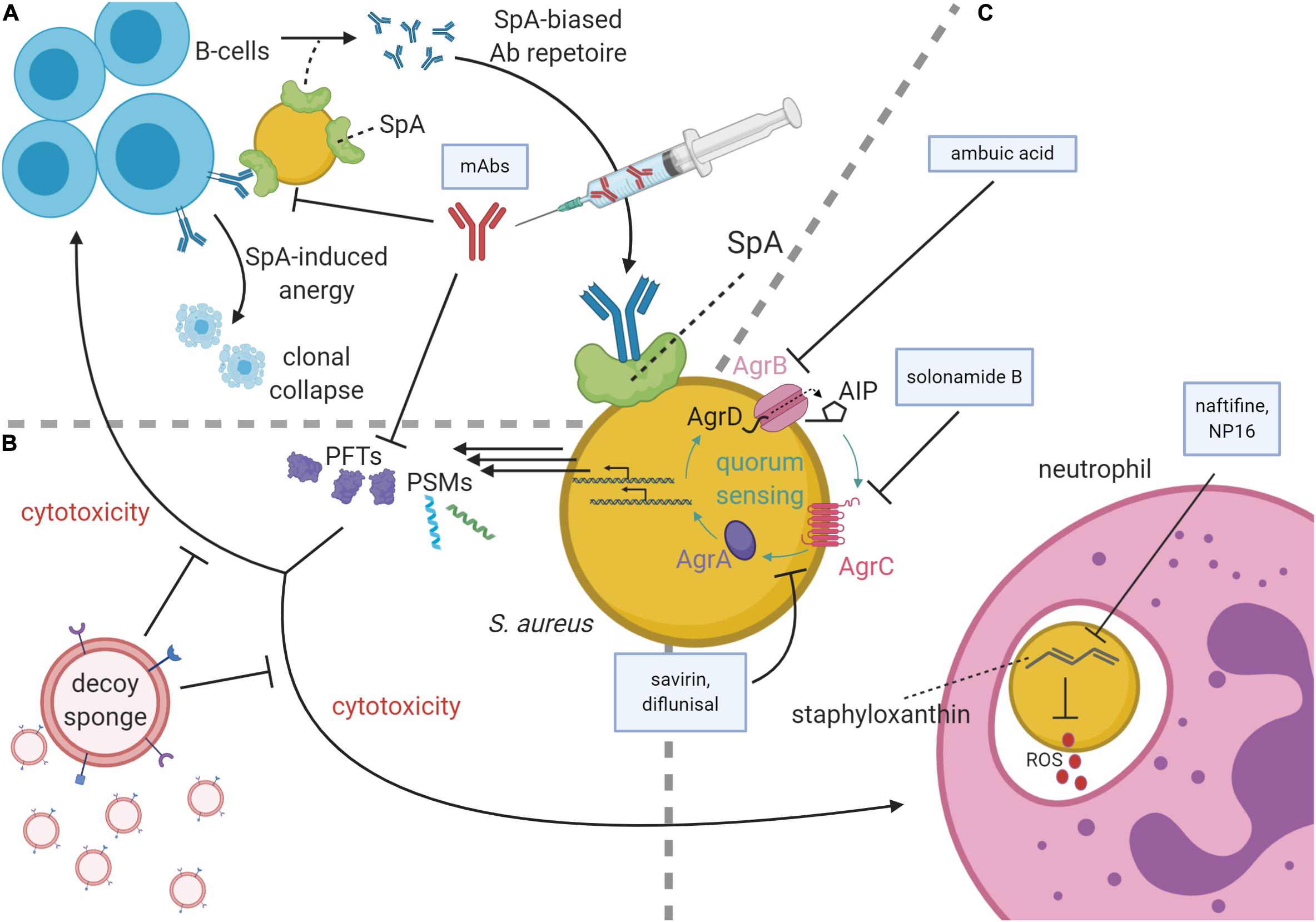
Figure 1. Staphylococcus aureus virulence pathways and antivirulence strategies. (A) At top left, B-cells secrete antibodies against S. aureus antigens, the Fc region of which may be bound by Staphylococcal protein A (SpA) on the S. aureus membrane, thereby subverting immune responses. SpA may also bind the Fab portion of VH3 family B-cell receptors, causing SpA-induced clonal expansion and anergic collapse. Superantigen activity of SpA also influences antibody (Ab) production by narrowing the breadth of anti-staphylococcal antibodies from VH3 family B-cells, creating a preference for poorly functioning anti-SpA clones. To inhibit SpA activity, therapeutic monoclonal antibodies (mAbs) raised against an attenuated SpA have been delivered parenterally. (B) mAbs can also inhibit pore-forming toxins (PFTs). PFTs and phenol-soluble modulins (PSMs) are cytolytic toxins regulated by the accessory gene regulator (agr) quorum sensing system. To diminish these toxins’ cytotoxicity, decoy sponges limit the activity of the PFTs by presenting a variety of decoy receptors on their surfaces. (C) Neutrophils use reactive oxygen species (ROS) to kill phagocytosed S. aureus. These ROS are inhibited by staphyloxanthin, which in turn can be inhibited by the drugs naftifine and NP16. At the image center, a schematic of the agr quorum sensing system is shown with color-coded protein labels. Beginning with transcription and translation of the agr operon, AgrB modifies and secretes AgrD to produce autoinducing peptide (AIP). Upon reaching quorum, AIP binds the receptor kinase, AgrC, which phosphorylates the response regulator, AgrA. AgrA activates the P2 and P3 promoters of the agr operon in a positive feedback loop and increases the production of many cytolytic virulence factors including PSMs and many PFTs. The Agr system may be targeted by several agents including ambuic acid (inhibition of AIP secretion), solonamide B (inhibition of AIP activation of AgrC), and savirin and diflunisal (inhibition of AgrC and AgrA downstream of AIP sensing).
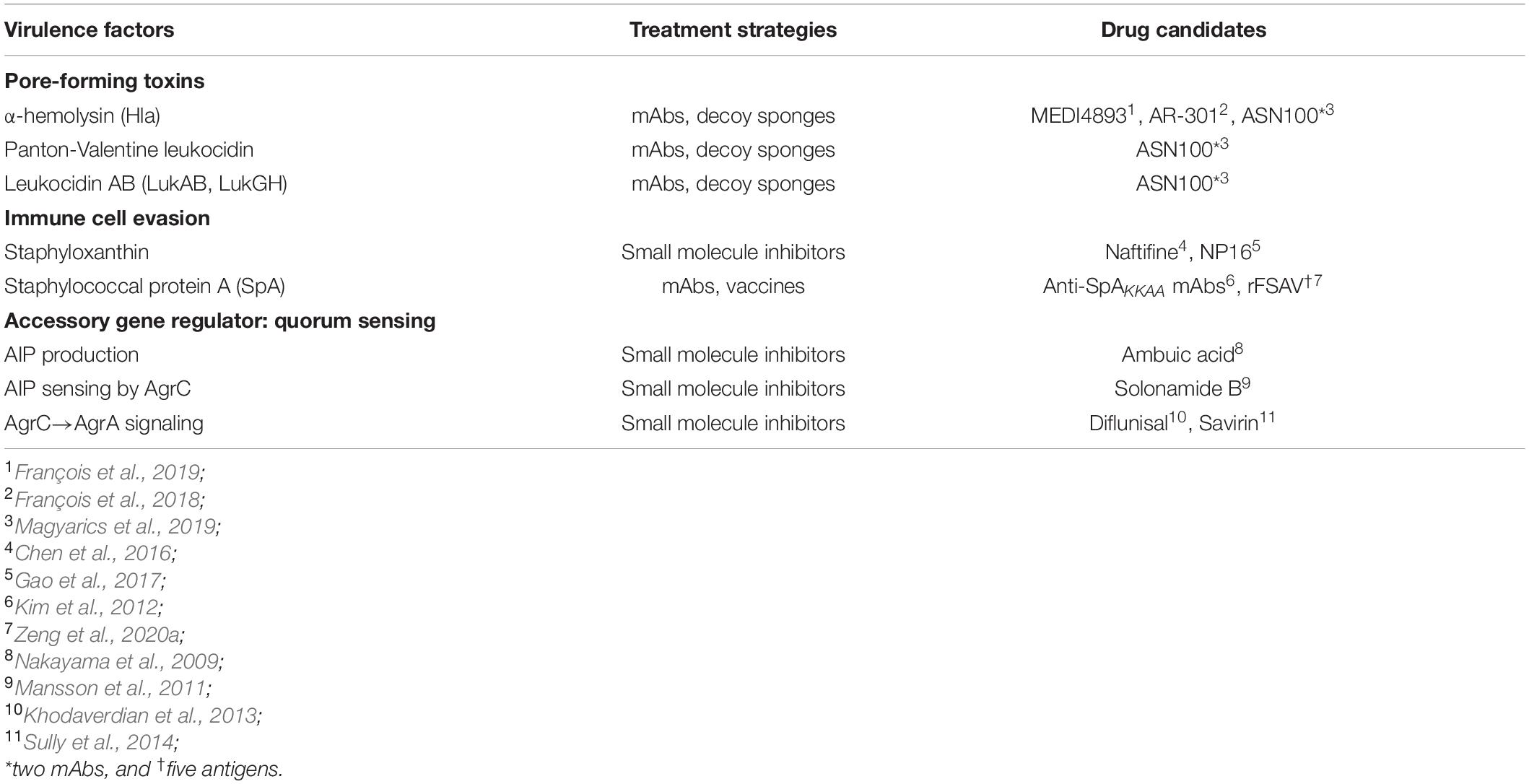
Table 1. Each of the virulence mechanisms of S. aureus discussed within the Mini Review is listed with accompanying drug candidates as shown.
Antivirulence Strategies
Antivirulence Targeting of Pore-Forming Toxins
Staphylococcus aureus produces an arsenal of pore-forming toxins (PFTs) that kill host cells, thereby combatting immune responses and liberating nutrients from the host. For more information regarding PFTs and their pharmacologic targeting, readers are directed to PFT-specific reviews (Reyes-Robles and Torres, 2017; Escajadillo and Nizet, 2018). Here, we highlight antivirulence approaches to three major PFTs: α-hemolysin (Hla, α-toxin), Panton-Valentine leukocidin (PVL), and leukocidin AB (LukAB, also known as LukGH). Hla was the first recognized PFT and is regarded as a key virulence factor of S. aureus (Bhakdi and Tranum-Jensen, 1991). PVL is highly cytotoxic, particularly when present during invasive infections such as necrotizing pneumonia (Panton and Valentine, 1932; Shallcross et al., 2013; Hu et al., 2015). LukAB is a recently identified bi-component leukocidin and one of the most immunogenic toxins in terms of neutralizing antibody induction (Thomsen et al., 2014). Expression of all three aforementioned PFTs is regulated by RNAIII, a major effector of the accessory gene regulator (agr) quorum sensing operon of S. aureus. Therefore, many strategies employed to inhibit these toxins target the agr system, which is discussed later (Le and Otto, 2015). Two primary approaches target PFTs directly: neutralizing antibodies and decoy receptors.
MEDI4893 (suvratoxumab), an Hla-neutralizing monoclonal antibody (mAb) previously known as LC10, is one of the most well-characterized antivirulence therapies for treating S. aureus infection. Hla binds the metalloprotease ADAM10 to promote oligomerization and pore formation (Wilke and Wardenburg, 2010). Exploiting this mechanism, MEDI4893 inhibits Hla interactions with ADAM10 and Hla self-oligomerization by binding a highly conserved region of Hla (Wilke and Wardenburg, 2010; Foletti et al., 2013; Oganesyan et al., 2014). Alanine scanning of this highly conserved region identified S. aureus mutants resistant to MEDI4893 neutralization. Importantly, mutants resistant to neutralization show reduced dermatonecrosis and mortality in murine models of S. aureus skin infection and pneumonia, respectively, likely reflecting impaired Hla function in the mutants (Tkaczyk et al., 2018). As of November 2020, MEDI4893 has completed Phase 2 clinical trials for prevention of S. aureus pneumonia in high-risk ICU patients. The data demonstrate a non-statistically significant trend toward prevention [relative risk reduction of 31.9% (90% CI, -7.5% to 56.8%)] and a favorable safety profile (Yu et al., 2017; François et al., 2019). Several other neutralizing antibodies against Hla have reached clinical trials, including AR-301 and ASN100. AR-301 is a mAb targeting Hla with previously published safety data that has now entered Phase 3 trials as an adjuvant therapy for S. aureus pneumonia (NCT03816956; François et al., 2018; ClinicalTrials.gov [Internet], 2019). ASN100 is a cocktail of two mAbs that together neutralize six cytolytic toxins of S. aureus including Hla, PVL, and LukAB, along with three other bicomponent toxins: γ-hemolysin AB (HlgAB), γ-hemolysin CB (HlgCB), and leukocidin ED (LukED). ASN100 limits tissue damage in a rabbit model of S. aureus pneumonia and completed Phase 1 clinical safety trials (Magyarics et al., 2019; Stulik et al., 2019). Notably, however, a Phase 2 trial for ASN100 was terminated due to futility (NCT02940626, ClinicalTrials.gov [Internet], 2016). mAbs targeting LukAB have been shown to improve bacterial burdens in murine models of systemic infection (Thomsen et al., 2017). Furthermore, inactivation of LukAB improves adaptive immune responses, as LukAB-mediated lysis of CD11b-positive antigen presenting cells attenuates adaptive responses (DuMont et al., 2013; Berends et al., 2019). LukAB does not readily target murine cells since LukAB, like many S. aureus toxins, demonstrates strong tropism for human cells, limiting its investigation in animal models (Spaan et al., 2017). Related bicomponent toxins, LukED and HlgAB, target similar cells in mice as LukAB does in humans, making LukED and HlgAB more impactful in preclinical mouse models (Spaan et al., 2017). In mice, immunization with LukED and HlgAB antigens induces stronger anti-LukED and anti-HlgAB antibody responses relative to infection with wild-type S. aureus and improves survival upon subsequent challenge with S. aureus (Tam et al., 2020).
In addition to neutralizing antibodies, decoy receptors have been proposed as treatments for staphylococcal infection. Hla can self-oligomerize into an amphiphilic, pore-forming state in the presence of deoxycholate micelles (a bile salt related to cholesterol; Bhakdi et al., 1982). Sphingomyelin-cholesterol micelles were previously shown to act as decoy sponges that inhibit Hla toxicity in vitro and in a murine sepsis model (Henry et al., 2015). Recently, exosomes (termed “defensosomes”) with increased ADAM10 were found to be secreted from host cells in a TLR-dependent manner in response to S. aureus, resulting in Hla sequestration and a reduction in disease mortality (Keller et al., 2020). As a therapeutic, poly(lactic-co-glycolic acid) (PLGA)-based nanoparticles coated with natural membranes of human RBCs fulfill a similar decoy mechanism to combat infection (Chen et al., 2018). These “toxin nanosponges” are coated with human RBC membranes agnostic of intended receptors, and inhibit the activity of Hla and other toxins. In summary, therapeutics developed for direct inhibition of PFTs have thus far exploited two naturally occurring mechanisms: neutralizing antibodies and decoy membrane receptors.
Antivirulence Strategies Targeting Immune Evasion Mechanisms
Staphyloxanthin
Staphyloxanthin is an antioxidant that gives S. aureus its eponymous golden color and protects against reactive oxygen species (ROS) produced by innate immune cells in vivo (Marshall and Wilmoth, 1981; El-Agamey et al., 2004; Liu et al., 2005; Clauditz et al., 2006). The genes responsible for staphyloxanthin synthesis are in the crtOPQMN operon, which is highly conserved across S. aureus strains, making it an attractive antivirulence target (Pelz et al., 2005). Two commonly targeted enzymes are CrtM and CrtN (Wieland et al., 1994). CrtM is analogous to a squalene synthase in human cholesterol biosynthesis, and many compounds inhibit both enzymes (Liu et al., 2008; Song et al., 2009). Inhibition of S. aureus CrtM with repurposed lipid-lowering medications targeting squalene synthase may be favorable in infected patients with hyperlipidemia (Sharma et al., 1998; Liu et al., 2008; Song et al., 2009; Lin et al., 2010). However, due to the potential detrimental effects of human squalene synthase inhibition when targeting CrtM, CrtN may be a more attractive target for inhibiting staphyloxanthin biosynthesis (Gao et al., 2017). Naftifine, an FDA-approved antifungal, and its related analogs were the first identified inhibitors of CrtN (Mühlbacher, 1991). Naftifine inhibition of staphyloxanthin production sensitizes S. aureus to ROS and limits mortality in a murine sepsis model (Chen et al., 2016). Recent studies have also identified NP16 and naftifine derivatives that inhibit CrtN with improved potency and reduce S. aureus bacterial burdens during systemic infection in mice (Gao et al., 2017; Li et al., 2018).
Staphylococcal Protein A
Staphylococcal protein A (SpA) is a cell wall-anchored protein which contains immunoglobulin-binding domains that bind the Fcγ portion of human IgG antibodies and the Fab of some IgM subtypes (Kronvall and Williams, 1969; Sjödahl, 1977; Moks et al., 1986; Roben et al., 1995; Graille et al., 2000). SpA binding of the Fcγ portion of IgG limits antibody-mediated phagocytosis (Forsgren and Nordström, 1974; Forsgren and Quie, 1974; Thammavongsa et al., 2015a). SpA also has superantigen activity by crosslinking of VH3 B-cell receptors, thereby inducing B-cell clones of limited breadth that produce poorly effective anti-staphylococcal antibodies as well as anergic B-cell populations (Roben et al., 1995; Pauli et al., 2014; Thammavongsa et al., 2015a; Sun et al., 2018). SpA is almost universally present in clinical S. aureus strains and likely contributes to vaccine failure and attenuation of mAb-based treatments (Forsgren, 1970; Kim et al., 2010; Bagnoli et al., 2012).
Despite the subversion of antibody-mediated immune responses, antivirulence strategies targeting SpA have primarily focused on antibody-based treatments. By developing a mutant of SpA unable to bind immunoglobulins (SpAKKAA), Kim and colleagues demonstrated that, following exposure to the SpAKKAA mutant, mice develop opsonizing antibodies and reduced bacterial burdens upon rechallenge with wild-type S. aureus (Kim et al., 2010; Falugi et al., 2013). Passive immunization with anti-SpAKKAA mAbs neutralizes SpA activity in systemic S. aureus infection models and maintains efficacy when humanized by grafting the complementary determining region onto human IgG1 (Kim et al., 2012; Thammavongsa et al., 2015b). Additionally, passive neutralization of SpA encourages production of effective anti-staphylococcal antibodies that promote bacterial decolonization from nasal and gastrointestinal mucosa of mice (Chen et al., 2019). Furthermore, humanized anti-SpAKKAA antibodies promote bacterial clearance upon binding SpA through the induction of the complement cascade, and effective complement activation is dependent upon the glycosylation of SpA-binding mAbs (Chen et al., 2020). SpAKKAA has been proposed as part of a four-antigen S. aureus vaccine that demonstrates improved survival in systemic S. aureus infection in mice (Kim et al., 2011; Rauch et al., 2014). Moreover, a five-antigen vaccine (rFSAV), which includes SpAKKAA, has completed a Phase 1 trial and induces high antibody titers (Zeng et al., 2020a,b).
Antivirulence Strategies Targeting Quorum Sensing
The primary regulatory pathway studied as an antivirulence target in S. aureus has been the agr system. The proteins of the agr operon enable S. aureus quorum sensing and regulate many virulence factors including PFTs and phenol-soluble modulins (PSMs; Le and Otto, 2015). PSMs are a class of cytotoxic alpha-helical peptides and are one of the few toxins to be directly regulated by the system’s response regulator, AgrA (Queck et al., 2008; Cheung et al., 2014). Given its broad impact on virulence factor production, targeting the agr system as an antivirulence treatment has been reviewed extensively (reviewed in Khan et al., 2015, and Piewngam et al., 2020). The agr quorum sensing system requires production and sensing of autoinducing peptide (AIP). Factors that inhibit agr can inhibit any step in AIP production, sensing, and subsequent transcriptional activation of the agr P2 and P3 promoters, as well as the promoter for PSMs (Thoendel et al., 2011; Khan et al., 2015). For example, ambuic acid and solonamide B inhibit AIP production and sensing, respectively, while savirin inhibits downstream transcriptional activity of AgrA (Nakayama et al., 2009; Mansson et al., 2011; Sully et al., 2014). S. aureus contains four distinct AIP peptide sequences that exist in 3 cross-inhibition groups: I/IV, II, and III (Ji et al., 1997; Jarraud et al., 2000). I and IV differ by one amino acid and cross-activate (Jarraud et al., 2000). Otherwise, the presence of alternative AIP sequences from any other agr group competitively inhibits AIP autorecognition (Ji et al., 1997). This phenomenon has been observed for AIP peptides from many other staphylococcal species, which produce AIP molecules that cross-inhibit S. aureus agr signaling (Otto et al., 2001; Canovas et al., 2016, Canovas et al., 2017; Williams et al., 2019).
Treatment with AIP analogs has been investigated as an antivirulence mechanism using rational design and high-throughput screening mechanisms (reviewed in Horswill and Gordon, 2020). Changing native amino acid residues or the thiolactone ring of AIP can increase or decrease agr signaling (Mayville et al., 1999; Otto et al., 2001; Lyon et al., 2002). Substitution of the AIP-III thiolactone ring with a lactam was demonstrated to inhibit AIP binding for all four agr types of S. aureus in the nanomolar range in vitro but has not yet been tested in vivo (Tal-Gan et al., 2016). High-throughput screening also elucidated natural compounds that competitively inhibit AIP binding to its receptor kinase, AgrC. One of the first discovered natural inhibitors of agr signaling was solonamide B, a cyclodepsipeptide from Photobacterium halotolerans (Mansson et al., 2011). Solonamide B and its derivatives inhibit binding of AIP to AgrC, and through this mechanism, solonamide B mitigates toxin-mediated cytolysis of phagocytes and δ-toxin-mediated atopic dermatitis in mice (Nielsen et al., 2014; Baldry et al., 2016, 2018). Since the discovery of solonamide B, other compounds that inhibit AIP binding to AgrC have been discovered. For example, agr-inhibiting lipoproteins from Bacillus subtilis promote decolonization in mice, possibly through agr-regulated adhesins. Furthermore, colonization with probiotic Bacillus species is inversely related to S. aureus colonization in the gastrointestinal tract and nares in humans, corroborating the findings in mice (Piewngam et al., 2018).
Inhibitors of agr also target signaling downstream of the receptor kinase (AgrC) and response regulator (AgrA). Savirin is a well-characterized antivirulence compound that targets AgrA by interfering with its transcriptional regulation (Sully et al., 2014). In an air-pouch infection model, mice treated with savirin exhibit reduced dermatonecrosis. While most tested agr inhibitors are administered at the time of bacterial inoculation, savirin was tested in a delayed treatment model and still showed efficacy, though the effect size was reduced compared to immediate treatment (Sully et al., 2014). An important future direction is therefore to evaluate the efficacy of antivirulence compounds in delayed treatment models, which perhaps more accurately reflect the clinical scenario in humans. In addition to novel small molecules, existing drugs have been identified that target AgrA and are amenable to drug repurposing. For example, diflunisal is an FDA-approved non-steroidal anti-inflammatory drug (NSAID) predicted to inhibit phosphorylation of AgrA by AgrC (Hannah et al., 1977; Khodaverdian et al., 2013). Diflunisal is a derivative of salicylic acid, which also has antivirulence properties through effects on Sigma Factor B (SigB) activity (Kupferwasser et al., 2003). In a murine model of osteomyelitis, delivery of diflunisal mitigates infection-mediated bone loss without impacting S. aureus burden in the infected femur (Hendrix et al., 2016; Ford et al., 2020; Spoonmore et al., 2020).
Targeting of agr quorum sensing is controversial in part because of its role in regulating biofilm dispersal (Boles and Horswill, 2008). Inactivation of agr in S. aureus promotes biofilm formation in vitro and impairs biofilm dispersal during osteomyelitis (Vuong et al., 2000; Nishitani et al., 2015). Biofilms are often associated with chronic infections, and agr-deficient mutants have emerged during the course of biofilm-associated infections (Brady et al., 2008; Traber et al., 2008; Suligoy et al., 2018; He et al., 2019). Therefore, it is important to test antivirulence compounds targeting agr signaling in the context of non-biofilm and biofilm infections as agr inhibition may lessen toxin-mediated virulence at the risk of promoting infection persistence (He et al., 2019). Additionally, it is important to recognize the natural occurrence of agr-deficient isolates, for which agr inhibition is inherently ineffective.
Conclusion
Antivirulence strategies are actively under preclinical investigation as therapies for staphylococcal disease. Several mAbs have advanced to clinical trials (e.g., AR-301 in phase 3, NCT03816956, ClinicalTrials.gov [Internet], 2019) and may soon be used as adjunctive therapies with conventional antimicrobials. We are unaware of any clinical trials in the United States that have investigated the use of small molecule inhibitors as antivirulence agents for S. aureus infection as of November 2020. However, several investigational antivirulence compounds are FDA-approved for alternative purposes. For example, diflunisal, an NSAID, inhibits the agr system, and terbinafine, an antifungal, inhibits staphyloxanthin production (Khodaverdian et al., 2013; Chen et al., 2016). Drug repurposing may therefore offer an attractive timeline for translation compared to the timeline for developing novel compounds.
Some traditional antibiotics also exhibit antivirulence properties. At sub-inhibitory concentrations, azithromycin inhibits toxin-mediated hemolysis in vitro and limits tissue destruction in a model of S. aureus keratitis (Ikemoto et al., 2020). Additionally, the bacteriostatic antimicrobial clindamycin is a protein synthesis inhibitor that suppresses toxin production in vitro and is now recommended for treatment of toxin-mediated diseases (e.g., toxic shock syndrome; Schlievert and Kelly, 1984; Ohlsen et al., 1998; Stevens et al., 2007; Nathwani et al., 2008). However, different antibiotic classes alter virulence factor production in distinct ways. Treatment of S. aureus with β-lactam antibiotics is thought to increase virulence factor production, including that of Hla and PVL (Ohlsen et al., 1998; Stevens et al., 2007). Recognizing that treatment with some antibiotics may increase virulence underscores the importance of investigating antivirulence strategies and how these will partner with traditional treatment regimens.
Unlike traditional antimicrobials, antivirulence therapies, strictly defined, do not inhibit bacterial growth in vitro and likely have decreased risk of resistance development. However, in vitro environments do not recapitulate elements of the host immune response found in vivo. Since virulence factors promote bacterial survival in the host, some selective pressure for resistance likely exists (Maura et al., 2016). Emergent resistance to antivirulence therapeutics has been observed (Maeda et al., 2012). However, not all antivirulence strategies carry the same risk of resistance. Targeting individual virulence mechanisms, such as PFTs, may have more limited risk of resistance compared to regulatory targets like agr that have the potential to impact large sets of virulence factors. Moreover, S. aureus mutants with emergent resistance to some antivirulence treatments may exhibit reduced overall fitness, as has been observed with mutants resistant to MEDI4893 (Tkaczyk et al., 2018). Targeting individual PFTs in S. aureus may lack efficacy due to functional redundancy of cytolytic toxins. Unfortunately, the influence of such redundancy in preclinical investigations may be missed due to the species tropism of many S. aureus toxins. For example, unlike LukED and HlgAB, PVL has no cytotoxic effect on murine cells but impacts invasive infection clinically (Spaan et al., 2017). Animal models continue to elucidate fundamental host-pathogen interactions; however, many otherwise promising S. aureus therapies demonstrate strong preclinical data without success in clinical trials (Bagnoli et al., 2012). Because of the high costs and risks of clinical trials, improved animal models offer an attractive compromise. Humanized mice with engrafted human immune cells may advance the translatability of mouse models of S. aureus infection (Allen et al., 2019). Virulence of human-targeted toxins (such as PVL) is more likely to be revealed in mice with human hematopoietic cells (Mrochen et al., 2020). Improved animal models may help determine which therapeutics should advance to clinical trials as antivirulence therapies continue to be explored.
Author Contributions
CF, IH, and JC contributed to initial conceptualization of the review. CF and IH performed initial literature reviews and manuscript drafting. JC contributed to literature review and extensive manuscript editing. All authors contributed to the article and approved the submitted version.
Funding
JC is supported by R01AI132560 (NIAID), R01AI145992 (NIAID), and a Career Award for Medical Scientists from the Burroughs Wellcome Fund. CF was supported through T32GM007347 (NIGMS) and is supported by F30AI138424 (NIAID).
Conflict of Interest
The authors declare that the research was conducted in the absence of any commercial or financial relationships that could be construed as a potential conflict of interest.
Acknowledgments
Members of the Cassat Laboratory (Casey Butrico, Virginia Cruz Victorio, Kara Eichelberger, Brittney Gimza, Christopher Peek, and Jenna Petronglo) provided criticism and feedback for development of the manuscript. Figure 1 was created with BioRender.com software.
References
Allen, T. M., Brehm, M. A., Bridges, S., Ferguson, S., Kumar, P., Mirochnitchenko, O., et al. (2019). Humanized immune system mouse models: progress, challenges and opportunities. Nat. Immunol. 20, 770–774. doi: 10.1038/s41590-019-0416-z
Bagnoli, F., Bertholet, S., and Grandi, G. (2012). Inferring reasons for the failure of Staphylococcus aureus vaccines in clinical trials. Front. Cell. Infect. Microbiol. 2:16. doi: 10.3389/fcimb.2012.00016
Balasubramanian, D., Harper, L., Shopsin, B., and Torres, V. J. (2017). Staphylococcus aureus pathogenesis in diverse host environments. Pathog. Dis. 75:ftx005. doi: 10.1093/FEMSPD/FTX005
Baldry, M., Kitir, B., Frøkiær, H., Christensen, S. B., Taverne, N., Meijerink, M., et al. (2016). The agr Inhibitors Solonamide B and Analogues Alter Immune Responses to Staphylococcus aureus but Do Not Exhibit Adverse Effects on Immune Cell Functions. PLoS One 11:e0145618. doi: 10.1371/journal.pone.0145618
Baldry, M., Nakamura, Y., Nakagawa, S., Frees, D., Matsue, H., Núñez, G., et al. (2018). Application of an agr-Specific Antivirulence Compound as Therapy for Staphylococcus aureus-Induced Inflammatory Skin Disease. J. Infect. Dis. 218, 1009–1013. doi: 10.1093/infdis/jiy259
Berends, E. T. M., Zheng, X., Zwack, E. E., Ménager, M. M., Cammer, M., Shopsin, B., et al. (2019). Staphylococcus aureus impairs the function of and kills human dendritic cells via the LukAB toxin. MBio 10:e01918-18. doi: 10.1128/mBio.01918-18
Bhakdi, S., Fuessle, R., and Tranum-Jensen, J. (1982). Staphylococcal α-toxin: Oligomerization of hydrophilic monomers to form amphiphilic hexamers induced through contact with deoxycholate detergent micelles. Proc. Natl. Acad. Sci. U. S. A. 78, 5475–5479. doi: 10.1073/pnas.78.9.5475
Bhakdi, S., and Tranum-Jensen, J. (1991). Alpha-toxin of Staphylococcus aureus. Microbiol. Rev. 55, 733–751. doi: 10.1128/mmbr.55.4.733-751.1991
Boles, B. R., and Horswill, A. R. (2008). agr-Mediated Dispersal of Staphylococcus aureus Biofilms. PLoS Pathog. 4:e1000052. doi: 10.1371/journal.ppat.1000052
Brady, R. A., Leid, J. G., Calhoun, J. H., Costerton, J. W., and Shirtliff, M. E. (2008). Osteomyelitis and the role of biofilms in chronic infection. FEMS Immunol. Med. Microbiol. 52, 13–22. doi: 10.1111/j.1574-695X.2007.00357.x
Canovas, J., Baldry, M., Bojer, M. S., Andersen, P. S., Gless, B. H., Grzeskowiak, P. K., et al. (2016). Cross-Talk between Staphylococcus aureus and Other Staphylococcal Species via the agr Quorum Sensing System. Front. Microbiol. 7:1733. doi: 10.3389/fmicb.2016.01733
Canovas, J., Mara Baldry, Martin, S., Bojer, Paal, S., Andersen, et al. (2017). Corrigendum: Cross-Talk between Staphylococcus aureus and Other Staphylococcal Species via the agr Quorum Sensing System. Front. Microbiol. 8:1075. doi: 10.3389/fmicb.2017.01075
Chen, F., Di, H., Wang, Y., Cao, Q., Xu, B., Zhang, X., et al. (2016). Small-molecule targeting of a diapophytoene desaturase inhibits S. aureus virulence. Nat. Chem. Biol. 12, 174–179. doi: 10.1038/nchembio.2003
Chen, Y., Chen, M., Zhang, Y., Lee, J. H., Escajadillo, T., Gong, H., et al. (2018). Broad-Spectrum Neutralization of Pore-Forming Toxins with Human Erythrocyte Membrane-Coated Nanosponges. Adv. Healthc. Mater. 7:e1701366. doi: 10.1002/adhm.201701366
Chen, X., Sun, Y., Missiakas, D., and Schneewind, O. (2019). Staphylococcus aureus decolonization of mice with monoclonal antibody neutralizing protein A. J. Infect. Dis. 219, 884–888. doi: 10.1093/infdis/jiy597
Chen, X., Shi, M., Tong, X., Kim, H. K., Wang, L. X., Schneewind, O., et al. (2020). Glycosylation-dependent opsonophagocytic activity of staphylococcal protein A antibodies. Proc. Natl. Acad. Sci. U. S. A. 117, 22992–23000. doi: 10.1073/pnas.2003621117
Cheung, G. Y. C., Joo, H. S., Chatterjee, S. S., and Otto, M. (2014). Phenol-soluble modulins - critical determinants of staphylococcal virulence. FEMS Microbiol. Rev. 38, 698–719. doi: 10.1111/1574-6976.12057
Clauditz, A., Resch, A., Wieland, K. P., Peschel, A., and Götz, F. (2006). Staphyloxanthin plays a role in the fitness of Staphylococcus aureus and its ability to cope with oxidative stress. Infect. Immun. 74, 4950–4953. doi: 10.1128/IAI.00204-06
ClinicalTrials.gov [Internet] (2015). Phase III Efficacy and Safety Study of AB103 in the Treatment of Patients With Necrotizing Soft Tissue Infections (ACCUTE); 2015 Jun 12. 2000 Feb 29. Identifier NCT02469857 Bethesda (MD): National Library of Medicine.
ClinicalTrials.gov [Internet] (2016). Prevention of S. aureus Pneumonia Study in Mechanically Ventilated Subjects Who Are Heavily Colonized With S. aureus; 2016 Oct 21. 2000 Feb 29. Identifier NCT02940626 Bethesda (MD): National Library of Medicine. doi: 10.1128/iai.00204-06
ClinicalTrials.gov [Internet] (2019). Adjunctive Therapy to Antibiotics in the Treatment of S. aureus Ventilator-Associated Pneumonia With AR-301; 2019 Jan 25. 2000 Feb 29. Identifier NCT03816956 Bethesda (MD): National Library of Medicine. doi: 10.1128/iai.00204-06
Dickey, S. W., Cheung, G. Y. C., and Otto, M. (2017). Different drugs for bad bugs: antivirulence strategies in the age of antibiotic resistance. Nat. Rev. Drug Discov. 16, 457–471. doi: 10.1038/nrd.2017.23
DuMont, A. L., Yoong, P., Day, C. J., Alonzo, F., McDonald, W. H., Jennings, M. P., et al. (2013). Staphylococcus aureus LukAB cytotoxin kills human neutrophils by targeting the CD11b subunit of the integrin Mac-1. Proc. Natl. Acad. Sci. U. S. A. 110, 10794–10799. doi: 10.1073/pnas.1305121110
El-Agamey, A., Lowe, G. M., McGarvey, D. J., Mortensen, A., Phillip, D. M., Truscott, T. G., et al. (2004). Carotenoid radical chemistry and antioxidant/pro-oxidant properties. Arch. Biochem. Biophys. 430, 37–48. doi: 10.1016/j.abb.2004.03.007
Escajadillo, T., and Nizet, V. (2018). Pharmacological targeting of pore-forming toxins as adjunctive therapy for invasive bacterial infection. Toxins 10:542. doi: 10.3390/toxins10120542
Falugi, F., Kim, H. K., Missiakas, D. M., and Schneewind, O. (2013). Role of protein A in the evasion of host adaptive immune responses by Staphylococcus aureus. MBio 4:00575-13. doi: 10.1128/mBio.00575-13
Foletti, D., Strop, P., Shaughnessy, L., Hasa-Moreno, A., Casas, M. G., Russell, M., et al. (2013). Mechanism of action and in vivo efficacy of a human-derived antibody against Staphylococcus aureus α-hemolysin. J. Mol. Biol. 425, 1641–1654. doi: 10.1016/j.jmb.2013.02.008
Ford, C. A., Spoonmore, T. J., Gupta, M. K., Duvall, C. L., Guelcher, S. A., and Cassat, J. E. (2020). Diflunisal−loaded poly(propylene sulfide) nanoparticles decrease S. aureus−mediated bone destruction during osteomyelitis. J. Orthop. Res. 2020:24948. doi: 10.1002/jor.24948
Forsgren, A. (1970). Significance of Protein A Production by Staphylococci. Infect. Immun. 2, 672–673. doi: 10.1128/iai.2.5.672-673.1970
Forsgren, A., and Nordström, K. (1974). Protein A From Staphylococcus aureus: The Biological Significance of Its Reaction With IgG. Ann. N. Y. Acad. Sci. 236, 252–266. doi: 10.1111/j.1749-6632.1974.tb41496.x
Forsgren, A., and Quie, P. G. (1974). Effects of Staphylococcal Protein A on Heat Labile Opsonins. J. Immunol. 112:1177-80.
François, B., Garcia Sanchez, M., Eggimann, P., Dequin, P.-F., Laterre, P.-F., Huberlant, V., et al. (2019). “Suvratoxumab Reduces Staphylococcus aureus Pneumonia in High-Risk ICU Patients: Results of the SAATELLITE Study,” in proceedings of the American Thoracic Society International Conference Meetings Abstracts American Thoracic Society International Conference Meetings Abstracts, (American Thoracic Society), A7358–A7358. doi: 10.1164/ajrccm-conference.2019.199.1_meetingabstracts.a7358
François, B., Mercier, E., Gonzalez, C., Asehnoune, K., Nseir, S., Fiancette, M., et al. (2018). Safety and tolerability of a single administration of AR-301, a human monoclonal antibody, in ICU patients with severe pneumonia caused by Staphylococcus aureus: first-in-human trial. Intensive Care Med. 44, 1787–1796. doi: 10.1007/s00134-018-5229-2
Gao, P., Davies, J., and Kao, R. Y. T. (2017). Dehydrosqualene desaturase as a novel target for anti-virulence therapy against Staphylococcus aureus. MBio 8:01224-17. doi: 10.1128/mBio.01224-17
Graille, M., Stura, E. A., Corper, A. L., Sutton, B. J., Taussig, M. J., Charbonnier, J. B., et al. (2000). Crystal structure of a Staphylococcus aureus protein a domain complexed with the Fab fragment of a human IgM antibody: Structural basis for recognition of B-cell receptors and superantigen activity. Proc. Natl. Acad. Sci. U. S. A. 97, 5399–5404. doi: 10.1073/pnas.97.10.5399
Hannah, J., Ruyle, W., Jones, H., Matzuk, A., Kelly, K., Witzel, B., et al. (1977). Discovery of diflunisal. Br. J. Clin. Pharmacol. 4, 7S–13S. doi: 10.1111/j.1365-2125.1977.tb04508.x
Hatzenbuehler, J., and Pulling, T. J. (2011). Diagnosis and Management of Osteomyelitis. Am. Fam. Physician 84:1027-33.
He, L., Le, K. Y., Khan, B. A., Nguyen, T. H., Hunt, R. L., Bae, J. S., et al. (2019). Resistance to leukocytes ties benefits of quorum sensing dysfunctionality to biofilm infection. Nat. Microbiol. 4, 1114–1119. doi: 10.1038/s41564-019-0413-x
Hendrix, A. S., Spoonmore, T. J., Wilde, A. D., Putnam, N. E., Hammer, N. D., Snyder, D. J., et al. (2016). Repurposing the Nonsteroidal Anti-inflammatory Drug Diflunisal as an Osteoprotective, Antivirulence Therapy for Staphylococcus aureus Osteomyelitis. Antimicrob. Agents Chemother. 60, 5322–5330. doi: 10.1128/AAC.00834-16
Henry, B. D., Neill, D. R., Becker, K. A., Gore, S., Bricio-Moreno, L., Ziobro, R., et al. (2015). Engineered liposomes sequester bacterial exotoxins and protect from severe invasive infections in mice. Nat. Biotechnol. 33, 81–88. doi: 10.1038/nbt.3037
Horswill, A. R., and Gordon, C. P. (2020). Structure-Activity Relationship Studies of Small Molecule Modulators of the Staphylococcal Accessory Gene Regulator. J. Med. Chem. 63, 2705–2730. doi: 10.1021/acs.jmedchem.9b00798
Hu, Q., Cheng, H., Yuan, W., Zeng, F., Shang, W., Tang, D., et al. (2015). Panton-Valentine Leukocidin (PVL)-Positive health care-associated methicillin-resistant Staphylococcus aureus isolates are associated with skin and soft tissue infections and colonized mainly by infective PVL-encoding bacteriophages. J. Clin. Microbiol. 53, 67–72. doi: 10.1128/JCM.01722-14
Huynh, T., Stecher, M., Mckinnon, J., Jung, N., and Rupp, M. E. (2016). Safety and Tolerability of 514G3, a True Human Anti-Protein A Monoclonal Antibody for the Treatment of S. aureus Bacteremia. Open Forum Infect. Dis. 3:1354.
Ikemoto, K., Kobayashi, S., Haranosono, Y., Kozai, S., Wada, T., Tokushige, H., et al. (2020). Contribution of anti-inflammatory and anti-virulence effects of azithromycin in the treatment of experimental Staphylococcus aureus keratitis. BMC Ophthalmol. 20:89. doi: 10.1186/s12886-020-01358-4
Jarraud, S., Lyon, G. J., Figueiredo, A. M. S., Gerard, L., Vandenesch, F., Etienne, J., et al. (2000). Exfoliatin-producing strains define a fourth agr specificity group in Staphylococcus aureus. J. Bacteriol. 182, 6517–6522. doi: 10.1128/JB.182.22.6517-6522.2000
Ji, G., Beavis, R., and Novick, R. P. (1997). Bacterial interference caused by autoinducing peptide variants. Science 276, 2027–2030. doi: 10.1126/science.276.5321.2027
Keller, M. D., Ching, K. L., Liang, F.-X., Dhabaria, A., Tam, K., Ueberheide, B. M., et al. (2020). Decoy exosomes provide protection against bacterial toxins. Nature 579:260–264. doi: 10.1038/s41586-020-2066-6
Khan, B. A., Yeh, A. J., Cheung, G. Y., and Otto, M. (2015). Investigational therapies targeting quorum-sensing for the treatment of Staphylococcus aureus infections. Exp. Opin. Investig. Drugs 24, 689–704. doi: 10.1517/13543784.2015.1019062
Khodaverdian, V., Pesho, M., Truitt, B., Bollinger, L., Patel, P., Nithianantham, S., et al. (2013). Discovery of antivirulence agents against methicillin-resistant Staphylococcus aureus. Antimicrob. Agents Chemother. 57, 3645–3652. doi: 10.1128/AAC.00269-13
Kim, H. K., Cheng, A. G., Kim, H. Y., Missiakas, D. M., and Schneewind, O. (2010). Nontoxigenic protein A vaccine for methicillin-resistant Staphylococcus aureus infections in mice. J. Exp. Med. 207, 1863–1870. doi: 10.1084/jem.20092514
Kim, H. K., Emolo, C., DeDent, A. C., Falugi, F., Missiakas, D. M., and Schneewind, O. (2012). Protein A-specific monoclonal antibodies and prevention of Staphylococcus aureus disease in mice. Infect. Immun. 80, 3460–3470. doi: 10.1128/IAI.00230-12
Kim, H. K. H., Kim, H. K. H., Schneewind, O., and Missiakas, D. (2011). Identifying protective antigens of Staphylococcus aureus, a pathogen that suppresses host immune responses. FASEB J. 25, 3605–3612. doi: 10.1096/fj.11-187963
Kronvall, G., and Williams, R. C. (1969). Differences in Anti-Protein a Activity among IgG Subgroups. J. Immunol. 103, 828–833.
Kupferwasser, L. I., Yeaman, M. R., Nast, C. C., Kupferwasser, D., Xiong, Y.-Q., Palma, M., et al. (2003). Salicylic acid attenuates virulence in endovascular infections by targeting global regulatory pathways in Staphylococcus aureus. J. Clin. Invest. 112, 222–233. doi: 10.1172/jci16876
Le, K. Y., and Otto, M. (2015). Quorum-sensing regulation in staphylococci-an overview. Front. Microbiol. 6:01174. doi: 10.3389/fmicb.2015.01174
Levy, R., Rotfogel, Z., Hillman, D., Popugailo, A., Arad, G., Supper, E., et al. (2016). Superantigens hyperinduce inflammatory cytokines by enhancing the B7-2/CD28 costimulatory receptor interaction. Proc. Natl. Acad. Sci. U. S. A. 113, E6437–E6446. doi: 10.1073/pnas.1603321113
Lew, D. P., and Waldvogel, F. A. (2004). Osteomyelitis. Lancet 364, 369–379. doi: 10.1016/S0140-6736(04)16727-5
Li, B., Ni, S., Chen, F., Mao, F., Wei, H., Liu, Y., et al. (2018). Discovery of Potent Benzocycloalkane Derived Diapophytoene Desaturase Inhibitors with an Enhanced Safety Profile for the Treatment of MRSA. VISA, and LRSA Infections. ACS Infect. Dis. 4, 208–217. doi: 10.1021/acsinfecdis.7b00259
Lin, F. Y., Liu, C. I., Liu, Y. L., Zhang, Y., Wang, K., Jeng, W. Y., et al. (2010). Mechanism of action and inhibition of dehydrosqualene synthase. Proc. Natl. Acad. Sci. U. S. A. 107, 21337–21342. doi: 10.1073/pnas.1010907107
Liu, C. I., Liu, G. Y., Song, Y., Yin, F., Hensler, M. E., Jeng, W. Y., et al. (2008). A cholesterol biosynthesis inhibitor blocks Staphylococcus aureus virulence. Science 319, 1391–1394. doi: 10.1126/science.1153018
Liu, G. Y., Essex, A., Buchanan, J. T., Datta, V., Hoffman, H. M., Bastian, J. F., et al. (2005). Staphylococcus aureus golden pigment impairs neutrophil killing and promotes virulence through its antioxidant activity. J. Exp. Med. 202, 209–215. doi: 10.1084/jem.20050846
Lyon, G. J., Wright, J. S., Christopoulos, A., Novick, R. P., and Muir, T. W. (2002). Reversible and specific extracellular antagonism of receptor-histidine kinase signaling. J. Biol. Chem. 277, 6247–6253. doi: 10.1074/jbc.M109989200
Maeda, T., García-Contreras, R., Pu, M., Sheng, L., Garcia, L. R., Tomás, M., et al. (2012). Quorum quenching quandary: Resistance to antivirulence compounds. ISME J. 6, 493–501. doi: 10.1038/ismej.2011.122
Magyarics, Z., Leslie, F., Bartko, J., Rouha, H., Luperchio, S., Schörgenhofer, C., et al. (2019). Randomized, double-blind, placebo-controlled, single-ascending-dose study of the penetration of a monoclonal antibody combination (ASN100) targeting Staphylococcus aureus cytotoxins in the lung epithelial lining fluid of healthy volunteers. Antimicrob. Agents Chemother. 63:e00350-19. doi: 10.1128/AAC.00350-19
Mansson, M., Nielsen, A., Kjærulff, L., Gotfredsen, C. H., Wietz, M., Ingmer, H., et al. (2011). Inhibition of virulence gene expression in Staphylococcus aureus by novel depsipeptides from a marine Photobacterium. Mar. Drugs 9, 2537–2552. doi: 10.3390/md9122537
Marshall, J. H., and Wilmoth, G. J. (1981). Pigments of Staphylococcus aureus, a series of triterpenoid carotenoids. J. Bacteriol. 147, 900–913. doi: 10.1128/jb.147.3.900-913.1981
Maura, D., Ballok, A. E., and Rahme, L. G. (2016). Considerations and caveats in anti-virulence drug development. Curr. Opin. Microbiol. 33, 41–46. doi: 10.1016/j.mib.2016.06.001
Mayville, P., Ji, G., Beavis, R., Yang, H., Goger, M., Novick, R. P., et al. (1999). Structure-activity analysis of synthetic autoinducing thiolactone peptides from Staphylococcus aureus responsible for virulence. Proc. Natl. Acad. Sci. U. S. A. 96, 1218–1223. doi: 10.1073/pnas.96.4.1218
Moks, T., Abrahmsén, L., Nilsson, B., Hellman, U., Sjöquist, J., and Uhlén, M. (1986). Staphylococcal protein A consists of five IgG-binding domains. Eur. J. Biochem. 156, 637–643. doi: 10.1111/j.1432-1033.1986.tb09625.x
Monaco, M., de Araujo, F., Cruciani, M., Coccia, E. M., and Pantosti, A. (2017). “Worldwide Epidemiology and Antibiotic Resistance of Staphylococcus aureus,” in Staphylococcus aureus: Microbiology, Pathology, Immunology, Therapy and Prophylaxis, eds F. Bagnoli, R. Rappuoli, and G. Grandi (Cham: Springer International Publishing), 21–56. doi: 10.1007/82_2016_3
Mrochen, D. M., Fernandes, de Oliveira, L. M., Raafat, D., and Holtfreter, S. (2020). Staphylococcus aureus host tropism and its implications for murine infection models. Int. J. Mol. Sci. 21, 1–35. doi: 10.3390/ijms21197061
Mühlbacher, J. M. (1991). Naftifine: A topical allylamine antifungal agent. Clin. Dermatol. 9, 479–485. doi: 10.1016/0738-081X(91)90076-W
Muthukrishnan, G., Masters, E. A., Daiss, J. L., and Schwarz, E. M. (2019). Mechanisms of Immune Evasion and Bone Tissue Colonization That Make Staphylococcus aureus the Primary Pathogen in Osteomyelitis. Curr. Osteoporos. Rep. 17, 395–404. doi: 10.1007/s11914-019-00548-4
Nakayama, J., Uemura, Y., Nishiguchi, K., Yoshimura, N., Igarashi, Y., and Sonomoto, K. (2009). Ambuic acid inhibits the biosynthesis of cyclic peptide quormones in gram-positive bacteria. Antimicrob. Agents Chemother. 53, 580–586. doi: 10.1128/AAC.00995-08
Nathwani, D., Morgan, M., Masterton, R. G., Dryden, M., Cookson, B. D., French, G., et al. (2008). Guidelines for UK practice for the diagnosis and management of methicillin-resistant Staphylococcus aureus (MRSA) infections presenting in the community. J. Antimicrob. Chemother. 61, 976–994. doi: 10.1093/jac/dkn096
Nielsen, A., Man̊sson, M., Bojer, M. S., Gram, L., Larsen, T. O., Novick, R. P., et al. (2014). Solonamide B inhibits quorum sensing and reduces Staphylococcus aureus mediated killing of human neutrophils. PLoS One 9:e84992 doi: 10.1371/journal.pone.0084992
Nishitani, K., Sutipornpalangkul, W., de Mesy Bentley, K. L., Varrone, J. J., Bello-Irizarry, S. N., Ito, H., et al. (2015). Quantifying the natural history of biofilm formation in vivo during the establishment of chronic implant-associated Staphylococcus aureus osteomyelitis in mice to identify critical pathogen and host factors. J. Orthop. Res. 33, 1311–1319. doi: 10.1002/jor.22907
Oganesyan, V., Peng, L., Damschroder, M. M., Cheng, L., Sadowska, A., Tkaczyk, C., et al. (2014). Mechanisms of neutralization of a human anti-α-toxin antibody. J. Biol. Chem. 289, 29874–29880. doi: 10.1074/jbc.M114.601328
Ohlsen, K., Ziebuhr, W., Koller, K. P., Hell, W., Wichelhaus, T. A., and Hacker, J. (1998). Effects of subinhibitory concentrations of antibiotics on alpha-toxin (hla) gene expression of methicillin-sensitive and methicillin-resistant Staphylococcus aureus isolates. Antimicrob. Agents Chemother. 42, 2817–2823. doi: 10.1128/aac.42.11.2817
Otto, M., Echner, H., Voelter, W., Goẗz, F., Goẗz, G., and Genetik, M. (2001). Pheromone Cross-Inhibition between Staphylococcus aureus and Staphylococcus epidermidis. Infect. Immun. 69:1957. doi: 10.1128/IAI.69.3.1957-1960.2001
Panton, P. N., and Valentine, F. C. O. (1932). STAPHYLOCOCCAL TOXIN. Lancet 219, 506–508. doi: 10.1016/S0140-6736(01)24468-7
Pauli, N. T., Kim, H. K., Falugi, F., Huang, M., Dulac, J., Dunand, C. H., et al. (2014). Staphylococcus aureus infection induces protein A-mediated immune evasion in humans. J. Exp. Med. 211, 2331–2339. doi: 10.1084/jem.20141404
Pelz, A., Wieland, K.-P., Putzbach, K., Hentschel, P., Albert, K., and Götz, F. (2005). Structure and Biosynthesis of Staphyloxanthin from Staphylococcus aureus. J. Biol. Chem. 280, 32493–32498. doi: 10.1074/jbc.M505070200
Piewngam, P., Chiou, J., Chatterjee, P., and Otto, M. (2020). Alternative approaches to treat bacterial infections: targeting quorum-sensing. Expert Rev. Anti. Infect. Ther. 18, 499–510. doi: 10.1080/14787210.2020.1750951
Piewngam, P., Zheng, Y., Nguyen, T. H., Dickey, S. W., Joo, H. S., Villaruz, A. E., et al. (2018). Pathogen elimination by probiotic Bacillus via signalling interference. Nature 562, 532–537. doi: 10.1038/s41586-018-0616-y
Potter, A. D., Butrico, C. E., Ford, C. A., Curry, J. M., Trenary, I. A., Tummarakota, S. S., et al. (2020). Host nutrient milieu drives an essential role for aspartate biosynthesis during invasive Staphylococcus aureus infection. Proc. Natl. Acad. Sci. U. S. A. 117, 12394–12401. doi: 10.1073/pnas.1922211117
Queck, S. Y., Jameson-Lee, M., Villaruz, A. E., Bach, T. H. L., Khan, B. A., Sturdevant, D. E., et al. (2008). RNAIII-Independent Target Gene Control by the agr Quorum-Sensing System: Insight into the Evolution of Virulence Regulation in Staphylococcus aureus. Mol. Cell 32, 150–158. doi: 10.1016/j.molcel.2008.08.005
Rauch, S., Gough, P., Kim, H. K., Schneewind, O., and Missiakas, D. (2014). Vaccine protection of leukopenic mice against Staphylococcus aureus bloodstream infection. Infect. Immun. 82, 4889–4898. doi: 10.1128/IAI.02328-14
Reyes-Robles, T., and Torres, V. J. (2017). “Staphylococcus aureus pore-forming toxins,” in Staphylococcus aureus: Microbiology, Pathology, Immunology, Therapy and Prophylaxis, eds F. Bagnoli, R. Rappuoli, and G. Grandi (Cham: Springer International Publishing), 121–144. doi: 10.1007/82_2016_16
Roben, P. W., Salem, A. N., and Silverman, G. J. (1995). VH3 family antibodies bind domain D of staphylococcal protein A. J. Immunol. 154, 6437–6445.
Schlievert, P. M., and Kelly, J. A. (1984). Clindamycin-induced suppression of toxic-shock syndrome-associated exotoxin production. J. Infect. Dis. 149:471. doi: 10.1093/infdis/149.3.471
Shallcross, L. J., Fragaszy, E., Johnson, A. M., and Hayward, A. C. (2013). The role of the Panton-Valentine leucocidin toxin in staphylococcal disease: A systematic review and meta-analysis. Lancet Infect. Dis. 13, 43–54. doi: 10.1016/S1473-3099(12)70238-4
Sharma, A., Slugg, P. H., Hammett, J. L., and Jusko, W. J. (1998). Clinical pharmacokinetics and pharmacodynamics of a new squalene synthase inhibitor, BMS-188494, in healthy volunteers. J. Clin. Pharmacol. 38, 1116–1121. doi: 10.1177/009127009803801205
Sievert, D. M., Ricks, P., Edwards, J. R., Schneider, A., Patel, J., Srinivasan, A., et al. (2013). Antimicrobial-Resistant Pathogens Associated with Healthcare-Associated Infections Summary of Data Reported to the National Healthcare Safety Network at the Centers for Disease Control and Prevention, 2009–2010. Infect. Control Hosp. Epidemiol. 34, 1–14. doi: 10.1086/668770
Sjödahl, J. (1977). Repetitive sequences in protein A from Staphylococcus aureus: Arrangement of Five Regions within the Protein, Four being Highly Homologous and Fc-Binding. Eur. J. Biochem. 351, 343–351. doi: 10.1111/j.1432-1033.1977.tb11324.x
Song, Y., Lin, F. Y., Yin, F., Hensler, M., Poveda, C. A. R., Mukkamala, D., et al. (2009). Phosphonosulfonates are potent, selective inhibitors of dehydrosqualene synthase and staphyloxanthin biosynthesis in Staphylococcus aureus. J. Med. Chem. 52, 976–988. doi: 10.1021/jm801023u
Spaan, A. N., Van Strijp, J. A. G., and Torres, V. J. (2017). Leukocidins: Staphylococcal bi-component pore-forming toxins find their receptors. Nat. Rev. Microbiol. 15, 435–447. doi: 10.1038/nrmicro.2017.27
Spoonmore, T. J., Ford, C. A., Curry, J. M., Guelcher, S. A., and Cassat, J. E. (2020). Concurrent local delivery of diflunisal limits bone destruction but fails to improve systemic vancomycin efficacy during Staphylococcus aureus osteomyelitis. Antimicrob. Agents Chemother. 64:e00182-20. doi: 10.1128/aac.00182-20
Stevens, D. L., Ma, Y., Salmi, D. B., McIndoo, E., Wallace, R. J., and Bryant, A. E. (2007). Impact of antibiotics on expression of virulence-associated exotoxin genes in methicillin-sensitive and methicillin-resistant Staphylococcus aureus. J. Infect. Dis. 195, 202–211. doi: 10.1086/510396
Stulik, L., Rouha, H., Labrousse, D., Visram, Z. C., Badarau, A., Maierhofer, B., et al. (2019). Preventing lung pathology and mortality in rabbit Staphylococcus aureus pneumonia models with cytotoxin-neutralizing monoclonal IgGs penetrating the epithelial lining fluid. Sci. Rep. 9, 1–11. doi: 10.1038/s41598-019-41826-6
Suligoy, C. M., Lattar, S. M., Llana, M. N., González, C. D., Alvarez, L. P., Robinson, D. A., et al. (2018). Mutation of Agr is associated with the adaptation of Staphylococcus aureus to the host during chronic osteomyelitis. Front. Cell. Infect. Microbiol. 8:18. doi: 10.3389/fcimb.2018.00018
Sully, E. K., Malachowa, N., Elmore, B. O., Alexander, S. M., Femling, J. K., Gray, B. M., et al. (2014). Selective Chemical Inhibition of agr Quorum Sensing in Staphylococcus aureus Promotes Host Defense with Minimal Impact on Resistance. PLoS Pathog. 10:e1004174. doi: 10.1371/journal.ppat.1004174
Sun, Y., Emolo, C., Holtfreter, S., Wiles, S., Kreiswirth, B., Missiakas, D., et al. (2018). Staphylococcal protein A contributes to persistent colonization of mice with Staphylococcus aureus. J. Bacteriol. 200:JB.00735-17. doi: 10.1128/JB.00735-17
Tal-Gan, Y., Ivancic, M., Cornilescu, G., Yang, T., and Blackwell, H. E. (2016). Highly Stable, Amide-Bridged Autoinducing Peptide Analogues that Strongly Inhibit the AgrC Quorum Sensing Receptor in Staphylococcus aureus. Angew. Chemie 128, 9059–9063. doi: 10.1002/ange.201602974
Tam, K., Lacey, K. A., Devlin, J. C., Coffre, M., Sommerfield, A., Chan, R., et al. (2020). Targeting leukocidin-mediated immune evasion protects mice from Staphylococcus aureus bacteremia. J. Exp. Med. 217:e20190541. doi: 10.1084/JEM.20190541
Thammavongsa, V., Kim, H. K., Missiakas, D., and Schneewind, O. (2015a). Staphylococcal manipulation of host immune responses. Nat. Rev. Microbiol. 13, 529–543. doi: 10.1038/nrmicro3521
Thammavongsa, V., Rauch, S., Kim, H. K., Missiakas, D. M., and Schneewind, O. (2015b). Protein A-neutralizing monoclonal antibody protects neonatal mice against Staphylococcus aureus. Vaccine 33, 523–526. doi: 10.1016/j.vaccine.2014.11.051
Thoendel, M., Kavanaugh, J. S., Caralyn, E., Flack, A., and Horswill, A. R. (2011). Peptide signaling in the Staphylococci. Chem. Rev. 111, 117–151. doi: 10.1021/cr100370n.Peptide
Thomsen, I. P., DuMont, A. L., James, D. B. A., Yoong, P., Saville, B. R., Soper, N., et al. (2014). Children with invasive Staphylococcus aureus disease exhibit a potently neutralizing antibody response to the cytotoxin LukAB. Infect. Immun. 82, 1234–1242. doi: 10.1128/IAI.01558-13
Thomsen, I. P., Sapparapu, G., James, D. B. A., Cassat, J. E., Nagarsheth, M., Kose, N., et al. (2017). Monoclonal Antibodies Against the Staphylococcus aureus Bicomponent Leukotoxin AB Isolated Following Invasive Human Infection Reveal Diverse Binding and Modes of Action. J. Infect. Dis. 215, 1124–1155. doi: 10.1093/infdis/jix071
Tkaczyk, C., Semenova, E., Shi, Y. Y., Rosenthal, K., Oganesyan, V., Warrener, P., et al. (2018). Alanine scanning mutagenesis of the MEDI4893 (Suvratoxumab) epitope reduces alpha toxin lytic activity in vitro and Staphylococcus aureus fitness in infection models. Antimicrob. Agents Chemother. 62:e01033-18. doi: 10.1128/AAC.01033-18
Traber, K. E., Lee, E., Benson, S., Corrigan, R., Cantera, M., Shopsin, B., et al. (2008). agr function in clinical Staphylococcus aureus isolates. Microbiology 154, 2265–2274. doi: 10.1099/mic.0.2007/011874-0
Vuong, C., Saenz, H. L., Götz, F., and Otto, M. (2000). Impact of the agr Quorum−Sensing System on Adherence to Polystyrene in Staphylococcus aureus. J. Infect. Dis. 182, 1688–1693. doi: 10.1086/317606
Wertheim, H. F. L., Melles, D. C., Vos, M. C., Van Leeuwen, W., Van Belkum, A., Verbrugh, H. A., et al. (2005). The role of nasal carriage in Staphylococcus aureus infections. Lancet Infect. Dis. 5, 751–762. doi: 10.1016/S1473-3099(05)70295-4
Wieland, B., Feil, C., Gloria-Maercker, E., Thumm, G., Lechner, M., Bravo, J. M., et al. (1994). Genetic and biochemical analyses of the biosynthesis of the yellow carotenoid 4,4’-diaponeurosporene of Staphylococcus aureus. J. Bacteriol. 176, 7719–7726. doi: 10.1128/jb.176.24.7719-7726.1994
Wilke, G. A., and Wardenburg, J. B. (2010). Role of a disintegrin and metalloprotease 10 in Staphylococcus aureus α-hemolysin-mediated cellular injury. Proc. Natl. Acad. Sci. U. S. A. 107, 13473–13478. doi: 10.1073/pnas.1001815107
Williams, M. R., Costa, S. K., Zaramela, L. S., Khalil, S., Todd, D. A., Winter, H. L., et al. (2019). Quorum sensing between bacterial species on the skin protects against epidermal injury in atopic dermatitis. Sci. Transl. Med. 11, 8329. doi: 10.1126/scitranslmed.aat8329
Yu, X. Q., Robbie, G. J., Wu, Y., Esser, M. T., Jensen, K., Schwartz, H. I., et al. (2017). Safety, tolerability, and pharmacokinetics of MEDI4893, an investigational, extended-half-life, anti-Staphylococcus aureus alpha-toxin human monoclonal antibody, in healthy adults. Antimicrob. Agents Chemother. 61:e01020-16. doi: 10.1128/AAC.01020-16
Zeng, H., Yang, F., Feng, Q., Zhang, J., Gu, J., Jing, H., et al. (2020a). Rapid and broad immune efficacy of a recombinant five-antigen vaccine against Staphylococcus aureus infection in animal models. Vaccines 8:134. doi: 10.3390/vaccines8010134
Keywords: Staphylococcus aureus – bacteria, antivirulence, antimicrobial resistance, virulence, infection, quorum sensing, accessory gene regulator, toxin
Citation: Ford CA, Hurford IM and Cassat JE (2021) Antivirulence Strategies for the Treatment of Staphylococcus aureus Infections: A Mini Review. Front. Microbiol. 11:632706. doi: 10.3389/fmicb.2020.632706
Received: 23 November 2020; Accepted: 22 December 2020;
Published: 14 January 2021.
Edited by:
Francesco Imperi, Roma Tre University, ItalyReviewed by:
Hanne Ingmer, University of Copenhagen, DenmarkMichael Otto, National Institute of Allergy and Infectious Diseases, National Institutes of Health (NIH), United States
Copyright © 2021 Ford, Hurford and Cassat. This is an open-access article distributed under the terms of the Creative Commons Attribution License (CC BY). The use, distribution or reproduction in other forums is permitted, provided the original author(s) and the copyright owner(s) are credited and that the original publication in this journal is cited, in accordance with accepted academic practice. No use, distribution or reproduction is permitted which does not comply with these terms.
*Correspondence: James E. Cassat, amltLmNhc3NhdEB2dW1jLm9yZw==