- 1Laboratório de Taxonomia, Bioquímica e Bioprospecção de Fungos (LTBBF), Instituto Oswaldo Cruz (IOC), Fundação Oswaldo Cruz (FIOCRUZ), Rio de Janeiro, Brazil
- 2Laboratório de Estudos Avançados de Microrganismos Emergentes e Resistentes (LEAMER), Instituto de Microbiologia Paulo de Góes, Universidade Federal do Rio de Janeiro (UFRJ), Rio de Janeiro, Brazil
- 3Laboratório de Citotoxicidade e Genotoxicidade (LaCiGen), Instituto de Química, UFRJ, Rio de Janeiro, Brazil
- 4Laboratório de Microbiologia Celular, IOC/FIOCRUZ, Rio de Janeiro, Brazil
- 5Department of Chemistry, Maynooth University, National University of Ireland, Maynooth, Ireland
- 6Center for Biomimetic and Therapeutic Research, Focas Research Institute, Technological University Dublin, Dublin, Ireland
Phialophora verrucosa is a dematiaceous fungus that causes mainly chromoblastomycosis, but also disseminated infections such as phaeohyphomycosis and mycetoma. These diseases are extremely hard to treat and often refractory to current antifungal therapies. In this work, we have evaluated the effect of 1,10-phenanthroline-5,6-dione (phendione) and its metal-based complexes, [Ag (phendione)2]ClO4 and [Cu(phendione)3](ClO4)2.4H2O, against P. verrucosa, focusing on (i) conidial viability when combined with amphotericin B (AmB); (ii) biofilm formation and disarticulation events; (iii) in vitro interaction with human macrophages; and (iv) in vivo infection of Galleria mellonella larvae. The combination of AmB with each of the test compounds promoted the additive inhibition of P. verrucosa growth, as judged by the checkerboard assay. During the biofilm formation process over polystyrene surface, sub-minimum inhibitory concentrations (MIC) of phendione and its silver(I) and copper(II) complexes were able to reduce biomass and extracellular matrix production. Moreover, a mature biofilm treated with high concentrations of the test compounds diminished biofilm viability in a concentration-dependent manner. Pre-treatment of conidial cells with the test compounds did not alter the percentage of infected THP-1 macrophages; however, [Ag(phendione)2]ClO4 caused a significant reduction in the number of intracellular fungal cells compared to the untreated system. In addition, the killing process was significantly enhanced by post-treatment of infected macrophages with the test compounds. P. verrucosa induced a typically cell density-dependent effect on G. mellonella larvae death after 7 days of infection. Interestingly, exposure to the silver(I) complex protected the larvae from P. verrucosa infection. Collectively, the results corroborate the promising therapeutic potential of phendione-based drugs against fungal infections, including those caused by P. verrucosa.
Introduction
Phialophora verrucosa is a well-known chromoblastomycosis etiological agent that may also cause other cutaneous and subcutaneous diseases as well as disseminated infections, such as phaeohyphomycosis and mycetoma (Turiansky et al., 1995; Tong et al., 2013; Queiroz-Telles et al., 2017). P. verrucosa has been observed in patients suffering with distinct illnesses, including keratitis, endophthalmitis, sinusitis, osteomyelitis, and endocarditis (Lundstrom et al., 1997; Hofmann et al., 2005; Campos-Herrero et al., 2012). Infections caused by this melanized filamentous fungus can affect both immunocompetent and immunosuppressed individuals (Lundstrom et al., 1997; Qiu et al., 2019). To date, there is no gold standard therapy for these incapacitating and neglected diseases. Overall, the infections caused by P. verrucosa tend to chronicity and hinder treatments, leading to recurrence and resistance to available conventional therapies (Li et al., 2014; Brito and Bittencourt, 2018). This scenario has emphasized the necessity for the development of new drugs against P. verrucosa infections, to be administered either alone or in combination with established antifungal agents.
Metal-based drugs have attracted enormous attention due to their pharmacological effects, which includes anticancer, anti-inflammatory, antioxidant, and antimicrobial activities (Mjos and Orvig, 2014; Anthony et al., 2020). In fact, inorganic medicinal chemistry has advanced in recent years, providing an alternative therapeutic approach to the purely organic antimicrobial agents (Lemire et al., 2013; Viganor et al., 2017; Claudel et al., 2020). Several studies have shown that 1,10-phenanthroline (phen) and its derivatives, as ligands coordinated to transition metals, exhibit potent antifungal activity against Candida albicans and non-albicans Candida species, such as C. tropicalis, C. krusei, C. glabrata, and C. haemulonii (Geraghty et al., 2000; McCann et al., 2000, 2012; Coyle et al., 2003; Hoffman et al., 2015; Gandra et al., 2017, 2020). In a previous study, we showed that 1,10-phenanthroline-5,6-dione (phendione) and its silver(I) and copper(II) complexes, [Ag(phendione)2]ClO4 and [Cu(phendione)3](ClO4)2.4H2O (henceforth represented as their biologically active cations [Ag(phendione)2]+ and [Cu(phendione)3]2+), affected crucial physiological events of P. verrucosa (Granato et al., 2017). These three agents inhibited fungal proliferation, presenting minimum inhibitory concentration [MIC; mg/L (μM)] values of 2.5 (12.0), 2.5 (4.0), and 5.0 (5.0), respectively. Moreover, the compounds affected filamentation, sterol production, and the metallo-type peptidase activity of P. verrucosa (Granato et al., 2017).
Cytotoxicity assays revealed that phendione, [Ag(phendione)2]+, and [Cu(phendione)3]2+ were well tolerated in vitro by several mammalian tumor and non-tumor lines and also macrophages, and in vivo by Swiss mice and Galleria mellonella larvae (McCann et al., 2012; Gandra et al., 2020). This insect larva is a good and alternative in vivo experimental model due to the similarities exhibited with respect to the innate immune system of mammals, its ease of manipulation, low cost, and ethical acceptance (Jemel et al., 2020). Thus, G. mellonella larvae are widely used to assess the toxicity, efficacy, and safety of conventional antifungal agents and potential new drugs (Kavanagh and Sheehan, 2018; Maurer et al., 2019; Treviño-Rangel et al., 2019; Jemel et al., 2020).
The antimicrobial efficacy of phendione and its metal-based complexes has been shown against planktonic cells and also biofilm-growing cells (Viganor et al., 2016; Gandra et al., 2017; Ventura et al., 2020). Several studies have highlighted the biofilm relevance in the fungal pathogenesis, contextualizing the real issue of this structure formation in the medical settings (Vila and Rozental, 2016; Santos et al., 2018; Wall et al., 2019). This complex microbial community, which is adhered to a surface, is surrounded by an extracellular matrix that gives its cells several advantages, such as increased virulence, immune system, and environmental stress protection as well as resistance to antimicrobial agents (Costa-Orlandi et al., 2017; Santos et al., 2018). The biofilm extracellular matrix is formed mainly by polysaccharides, but also contains proteins, lipids, and DNA. This matrix is one of the most relevant structures of the biofilm and is directly associated with antimicrobial resistance (Ramage et al., 2012; Santos et al., 2018). In recent years, the capability of filamentous fungi (e.g., Aspergillus fumigatus, Paracoccidioides brasiliensis, Scedosporium spp., and Exophiala dermatitidis) to form a biofilm has been well documented (Kaur and Singh, 2014; Sardi et al., 2015; Mello et al., 2016; Kirchhoff et al., 2017). The ability of P. verrucosa to establish a biofilm on a polystyrene surface was previously investigated by our research group, and it was found that biofilm-growing P. verrucosa cells were more resistant to the action of conventional antifungal drugs than their planktonic counterparts (unpublished data).
The present study was designed to investigate the effects of phendione, [Ag(phendione)2]+, and [Cu(phendione)3]2+ on P. verrucosa, focusing on (i) the combination of these test agents with the classical antifungal drug, amphotericin B, (ii) biofilm formation and disarticulation, (iii) the in vitro interaction with human macrophages; and (iv) in vivo infection using G. mellonella larvae as a model.
Materials and Methods
Fungal Growth Conditions
P. verrucosa (strain FMC.2214 isolated from a human patient with chromoblastomycosis and used in our previous work; Granato et al., 2017) was maintained in Sabouraud dextrose agar (SDA) medium with mineral oil at 4°C. For all assays, fungal cells were cultivated for 7 days under constant agitation (130 rpm) at 26°C in 100 ml of Czapek-Dox broth medium (BD-Difco, United States). Conidia were obtained by gauze filtering followed by centrifugation at 4,000 × g for 10 min. The fungal cells were then washed three times with saline solution (0.85% NaCl), and the number of conidia cells was estimated using a Neubauer chamber (Granato et al., 2015).
Test Compounds
Phendione was purchased from Sigma-Aldrich. [Ag(phendione)2]+ and [Cu(phendione)3]2+ were prepared by reacting phendione with the appropriate metal perchlorate salts in accordance with procedures previously published by McCann et al. (2004). For all of the experiments, the compounds were dissolved in dimethyl sulfoxide (DMSO; Sigma-Aldrich).
Combinatory Effects of Phendione and Its Metal Complexes With Amphotericin B on P. verrucosa Viability
The drug combinations were assessed using the checkerboard microdilution method as described by Chudzik et al. (2019). Briefly, initial concentrations equivalent to 8 × MIC of amphotericin B (AmB, MIC = 6.25 mg/L) were serially diluted in a cross fashion mode against 4 × MIC of each compound (phendione, [Ag(phendione)2]+, and [Cu(phendione)3]2+) in 96-well plates containing Roswell Park Memorial Institute (RPMI) 1640 medium (Sigma-Aldrich). Thus, the final concentrations range from 0.09 to 50 mg/L for AmB, 0.039 to 20 mg/L for [Cu(phendione)3]2+, and 0.019 to 10 mg/L for both phendione and [Ag(phendione)2]+. The inoculation and culture conditions followed the Clinical and Laboratory Standards Institute (CLSI, 2008) standard document M38-A2 for filamentous fungi with some minor modifications (Granato et al., 2017). Minimum inhibitory concentration values were established when the combination of AmB and each test compound inhibited 100% of the fungal growth as evidenced by visual inspection and the resazurin staining assay (Liu et al., 2007). The interaction type of two-drug combination was defined according to the fractional inhibitory concentration index (FICI) from the following formula: FICI = FICA + FICB, where FICA and FICB = MIC of drug A or B in combination divided by the MIC of drug A or B alone. Synergism was established when FICI value ≤ 0.5; additivity FICI > 0.5 to < 2.0; indifference FICI ≥ 2.0 to <4.0; and antagonism FICI ≥ 4.0 (Chudzik et al., 2019).
Effects of Phendione and Its Metal Complexes on P. verrucosa Biofilms
In this set of experiments, phendione, [Ag(phendione)2]+ and [Cu(phendione)3]2+ were tested on P. verrucosa biofilm using the broth microdilution assay in accordance with the document M38-A2 CLSI (2008) and Mello et al. (2016). Firstly, the compounds were examined for their ability to modulate biofilm formation. Conidia (1 × 106 cells) were placed in flat-bottom 96-well polystyrene microtiter plates containing RPMI medium supplemented or not with each test compound at concentrations varying from MIC to ⅛ × MIC values. After 72 h, non-adhered fungal cells were removed and the plates containing adhered biofilm-growing cells were subjected to colorimetric assays designed to measure the following biofilm parameters: biomass, viability, and extracellular matrix. The biomass quantification was evaluated after biofilm fixation with methanol (at 99%), followed by staining for 20 min with 0.3% crystal violet solution and the absorbance read at 590 nm. The determination of metabolic activity was conducted in non-fixed biofilms by the addition of 100 μl of a solution containing 2,3-bis (2-methoxy-4-nitro-5-sulfophenyl)-5-[(phenylamino) carbonyl]-2H-tetrazolium hydroxide (XTT, 0.04 mg) and menadione (0.0005 mg) into the plate wells, followed by incubation in the dark at 37°C for 4 h, and finally an absorbance reading at 490 nm. The assessment of the extracellular matrix was carried out using non-fixed biofilms, by staining with 0.1% safranin solution and the absorbance read at 530 nm (Mello et al., 2016). In order to investigate the effect of phendione and its metal complexes on mature biofilms, P. verrucosa conidia (1 × 106 cells) were added to 96-well polystyrene microplates and incubated for 72 h. Then, different concentrations of the test compounds, ranging from MIC to 64 × MIC values, were added to the plates and these were incubated for an additional 48 h. The biofilm MIC90 value (bMIC90) was determined by considering the lowest concentration of each test compound capable of causing a 90% reduction in biofilm viability (Romera et al., 2019). System controls were prepared with non-treated cells in RPMI medium supplemented or not with DMSO, and RPMI medium containing DMSO or not. All dyes and reagents used for the colorimetric assays were obtained from Sigma-Aldrich. The absorbance measurements were performed using a microplate reader (SpectraMax M3, Molecular Devices, United States). Biofilm formation was observed using an inverted microscope (Nikon TS100-F, Tokyo, Japan) with a ×40 objective lens.
Effects of Phendione and Its Metal Complexes on P. verrucosa-Macrophage Interaction
Cell Culture and Stimulation
Human monocytic leukemia THP-1 cells (ATCC TIB-202) were cultured in 25 cm3 culture flasks containing RPMI 1640 medium and 10% fetal bovine serum (Sigma-Aldrich) at 37°C in a 5% CO2 atmosphere. For experimental assays, 1 × 106 cells were added to each well of a 24-well cell culture plate and cultivated in the same conditions as detailed above, with the addition of phorbol myristate acetate (PMA, 80 nM, Sigma-Aldrich) to promote the differentiation of monocytic cells into macrophages. After 24 h, the THP-1 cells were washed three times with RPMI and incubated in fresh medium without PMA for an additional 24 h to allow for cell recovery. The differentiation of THP-1 monocytes into macrophages was confirmed by observing the cells adhesion capacity to culture plates and alteration of their morphology to a flat, amoeboid, and spreading shape using inverted microscope Nikon (Batista-Silva et al., 2016).
Fungi-Host Cells Interaction: Pre-treatment Assay
Conidia (2 × 106 cells) were treated with each test compound at concentrations varying from ¼ × MIC to 2 × MIC values, and after 20 h at 35°C, the fungal viability was assessed using the XTT assay (Mowat et al., 2007). For the interaction assay, P. verrucosa conidia were treated for 20 h with a non-cytotoxic concentration of each test compound, washed, and incubated for 30 min with the fluorescent dye PKH26 (2 μM; Sigma-Aldrich). After that, 250 μl of fetal bovine serum were added to interrupt the reaction, and the conidial cells were then washed and re-suspended in RPMI medium and incubated with macrophages in a 10:1 ratio (fungi:macrophage) for 3 h in a 5% CO2 atmosphere at 37°C. The interaction systems were washed three times with sterile phosphate-buffered saline, pH 7.2 (PBS) and then with 2 mM PBS-EDTA buffer for their transference to cytometry tubes (Ersland et al., 2010). The interaction was monitored by flow cytometry (BD FACSAria) using Flowjo software. Results were represented as percentage of infected macrophages, which indicates the percentage of macrophage infected with fluorescent-labeled conidial cells. Systems consisting of non-treated conidia (control) and unlabeled conidia were also examined. The fluorescence values were adjusted after subtracting the non-specific fluorescence values of unlabeled cells (autofluorescence). In parallel, macrophages infected with either untreated or treated conidial cells were lysed with sterile cold water, and the suspensions were plated onto SDA medium to count the number of colony forming units (CFU; Palmeira et al., 2008).
Fungi-Host Cells Interaction: Post-treatment (Killing) Assay
In this set of experiments, the effect of the test compounds on macrophage viability was initially investigated. THP-1 macrophages (1 × 106) were incubated in a 96-well cell culture plate for 20 h in the absence (control) or in the presence of each test compound at a concentration varying from 0.03 to 5 mg/L. Then, the viability of macrophages was assessed after addition of 3-(4,5-dimethylthiazol-2-yl)-2,5-diphenyl tetrazolium bromide (MTT, Sigma-Aldrich) at 0.5 mg/ml and incubation for 3 h at 37°C as described by Mosmann (1983). Subsequently, in order to investigate the killing capability of THP-1 macrophages against P. verrucosa, viable conidia were washed in PBS and transferred to a 24-well plate containing macrophages at 10:1 ratio (fungi:macrophage) and incubated for 1 h at 37°C. Non-adhered conidia were removed and the interaction systems were incubated for 20 h with RPMI medium supplemented or not (control) with a non-cytotoxic concentration of test compound. The cultures were washed with PBS, lysed with sterile cold water and the suspensions then plated onto SDA medium for establishing the CFU number (Palmeira et al., 2008).
Effect of [Ag(phendione)2]+ on G. mellonella Larvae Infected With P. verrucosa
G. mellonella Larvae
The larvae of G. mellonella were reared in the insectary of the Department of Biochemistry of the Institute of Chemistry at UFRJ in plastic boxes at 21°C in the dark. Healthy larvae from the last instar with similar size (15–20 mm) and weight (0.2 g), and without alterations in their color, were chosen for the experiments. In order to determine the in vivo cytotoxicity and the antifungal activity of each test compound, 10 larvae were used in each experimental system (McCann et al., 2012; Fernandes et al., 2017).
Larval Survival Assay
Firstly, the non-toxic concentrations of [Ag(phendione)2]+ and the appropriate fungal cellular density were determined as described by McCann et al. (2012). Briefly, the compound (10 μl) was administered by injection through the last left proleg directly into the larvae haemocoel, using a Hamilton syringe, resulting in the final concentrations of either 5 or 10 μg compound/larva. Simultaneously, P. verrucosa conidia were suspended in PBS and adjusted to final densities equal to 1 × 104, 1 × 105, 1 × 106, 4 × 106, or 1 × 107 and inoculated as described above into each group of G. mellonella larvae. Then, the larvae were incubated at 37°C for 7 days and survival monitored every 24 h. Larvae mortality was assessed by lack of movement in response to mechanical stimulus. Larvae inoculated with 10 μl of PBS or DMSO were used as control systems. Infected larvae images were obtained after 1 h of incubation. For antifungal assessment, each G. mellonella larvae group was infected with P. verrucosa conidia, at a concentration presenting an intermediate rate of larvae mortality, and after 2 h a non-cytotoxic concentration of the test compound was injected. After that, the experimental protocol and the survival rate determination were performed as detailed above.
Statistical Analysis
The experiments were performed in triplicate in three independent experimental sets. The graphics and data were constructed and analyzed statistically by means of the ANOVA-test. Survival curves of G. mellonella larvae experiments were constructed using the method of Kaplan-Meier and compared using the Log-Rank (Mantel-Cox) test. All statistical analyses were performed with GraphPad Prism 5.0 software. Values of p = 0.05 or less were assumed as significant.
Results and Discussion
Combinatory Effects of Phendione and Its Metal Complexes With Amphotericin B on P. verrucosa Viability
Using the checkerboard assay, we firstly investigated the effects of AmB combined with each of the test compounds (Table 1). The combinations, AmB-[Cu(phendione)3]2+ and AmB-[Ag(phendione)2]+, were both additive (FICI = 0.7 and 1.0, respectively). Although none of the drug combinations displayed synergism (FICI ≤ 0.5) in the present case, such an effect has been observed in previous studies involving combinations of the metal-phendione complexes with classical antifungal drugs. Eshwika et al. (2004) showed that C. albicans treated with non-cytotoxic concentrations of [Ag(phendione)2]+ became more susceptible to miconazole and AmB. In a later study on C. albicans, the combination of AmB with copper(II) ions (AmB-Cu(II) complex) was again found to be more effective than AmB alone (Gagoś et al., 2011; Chudzik et al., 2013). It has been suggested that drug combinations with an additive effect may enhance pharmaceutical efficacy and reduce toxicity when each compound targets distinct stages of the same biological pathway (Spitzer et al., 2017). Importantly, the combination of benzothioureas with caspofungin has been shown to function in an additive manner regarding anti-cryptococcal activity, even though caspofungin on its own is ineffective against Cryptococcus species (Hartland et al., 2016; Spitzer et al., 2017). Overall, metal-based drugs have different modes of action conventional organic antifungal agents, thus combination therapy could potentiate the effect as well as possibly minimizing both resistance and toxicity.
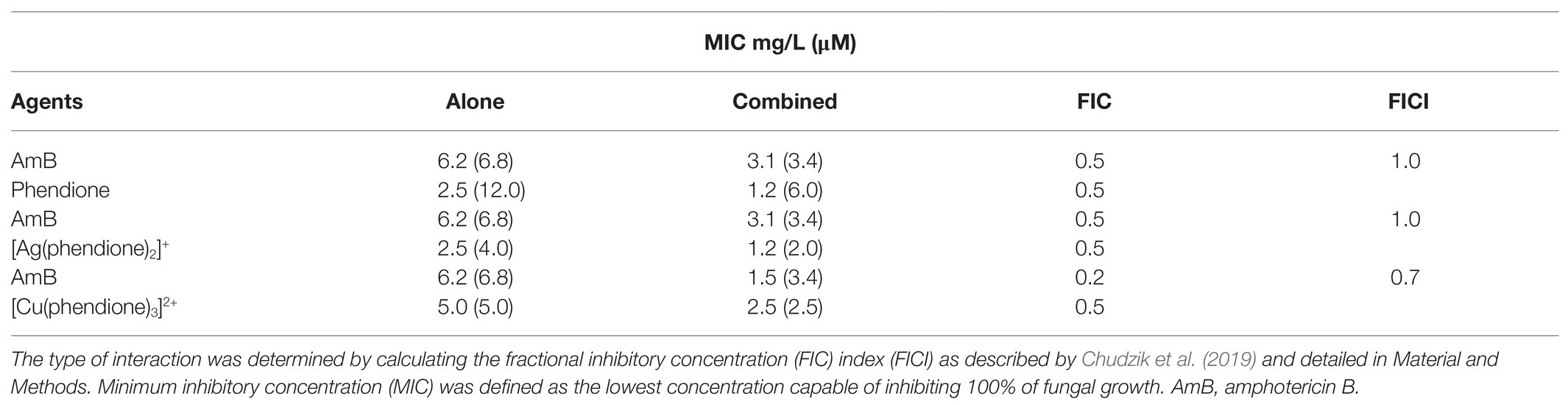
Table 1. Effects of phendione and its metal complexes combined with amphotericin B on Phialophora verrucosa growth.
Effects of Phendione and Its Metal Complexes on P. verrucosa Biofilms
The effectiveness of the phendione-based drugs to inhibit P. verrucosa conidial growth was previously described by our research group, with the following order: [Cu(phendione)3]2+ > [Ag(phendione)2]+ > phendione (Granato et al., 2017). Herein, we evaluated the effects of the test compounds upon biofilm formation by P. verrucosa on a polystyrene substrate. The fungal cells (1 × 106) incubated with sub-MIC concentrations preserved their metabolic viability along the process of biofilm formation, as shown by the XTT assay (Figure 1A). However, the biofilm biomass was affected by the incubation with the test compounds, showing a significant reduction when treated with phendione and [Cu(phendione)3]2+ at both ¼ × and ⅛ × MIC values, as well as with [Ag(phendione)2]+ at ½ × MIC value (Figure 1B). The extracellular matrix production was also affected by [Ag(phendione)2]+ at both ½ × and ¼ × MIC values, and by phendione at ¼ × MIC (Figure 1C).
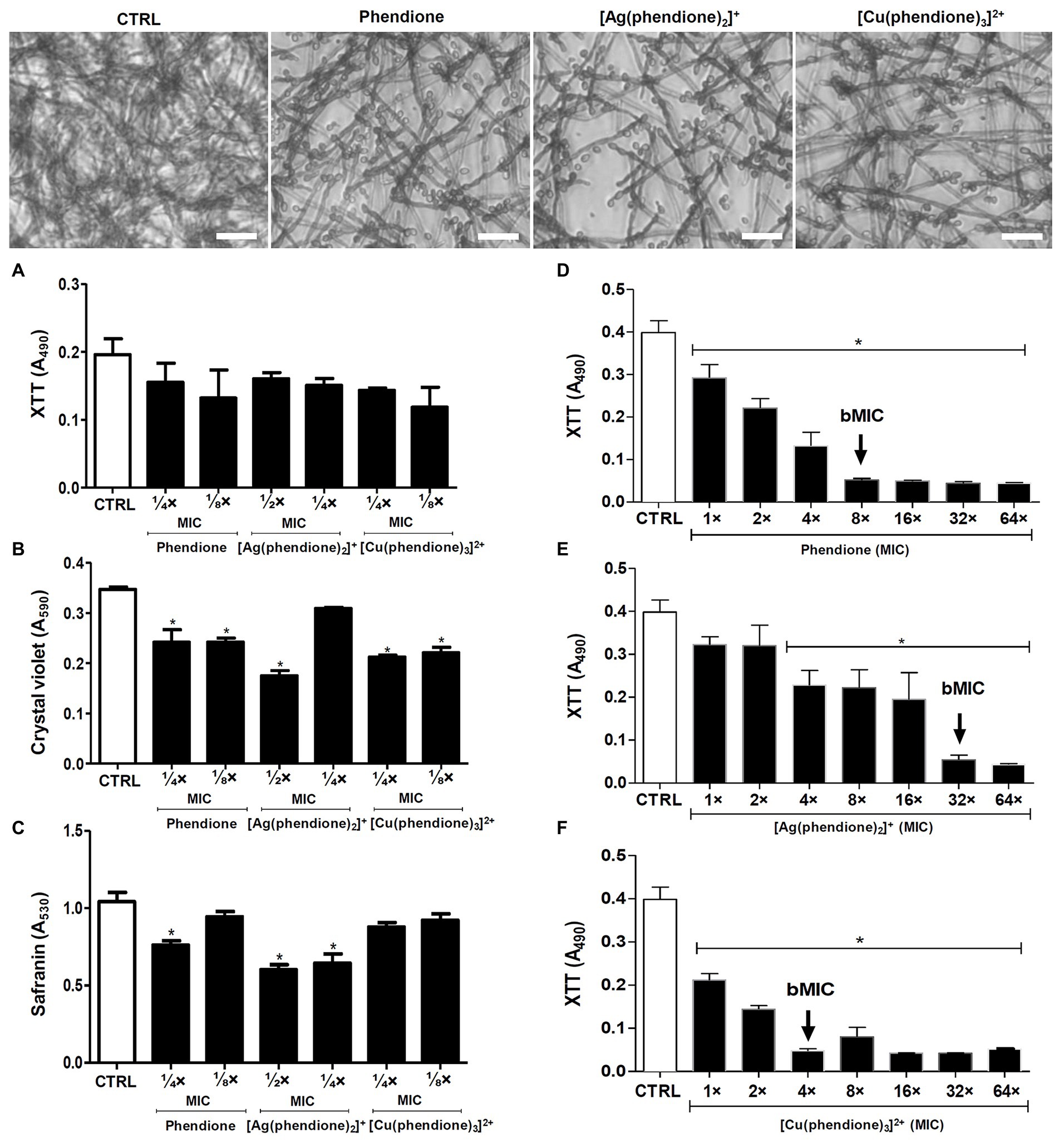
Figure 1. Effects of phendione and its metal complexes on P. verrucosa biofilm formation and maturation. Conidia (1 × 106) were added to 96-well plates containing Roswell Park Memorial Institute (RPMI) medium supplemented with sub-MIC concentrations of each test compound. After incubation for 72 h at 37°C, (A) cell viability, (B) quantification of biomass, and (C) extracellular matrix were evaluated, as described in Material and Methods. The same conidia density (D–F) was placed to interact with polystyrene for 72 h in RPMI medium. Then, concentrations varying from MIC to 64 × MIC of each test compound were added and the plates incubated for additional 48 h. The MIC90 values of the biofilm (bMIC90) were detected using the XTT reduction assay. Systems containing non-treated conidia were also prepared (CTRL, control). The eluent (dimethyl sulfoxide, DMSO) did not affect fungal growth (data not shown). The symbol (*) highlights the MIC values that caused a statistically significant reduction on each evaluated parameter in relation to the respective control (p < 0.05). Representative images of biomass (crystal violet-stained) formed by P. verrucosa non-treated (CTRL, control) and treated with phendione (¼ × MIC) and its silver(I) (½ × MIC) and copper(II) (¼ × MIC) complexes. Bar: 20 µm.
The test compounds were also evaluated regarding their ability to disturb a mature biofilm formed by P. verrucosa. Phendione, [Ag(phendione)2]+, and [Cu(phendione)3]2+ inhibited fungal viability by approximately 90% (bMIC90) when treated with concentrations [mg/L (μM)] of 20 (96), 80 (128), and 20 (20), respectively (Figures 1D–F). Moreover, biofilm cells were more resistant to the test compounds, since their bMIC90 values were higher than those observed for the planktonic counterparts (Granato et al., 2017), increasing by about 4-, 8-, and 32-fold to [Cu(phendione)3]2+, phendione, and [Ag(phendione)2]+, respectively. Previous studies had shown that the biofilm of the C. haemulonii species complex was also disturbed by phen- and phendione-containing compounds, including copper(II) and silver(I) complexes (Gandra et al., 2017). In contrast to P. verrucosa, silver(I) chelates (16 mg/L, 25.5 μM) had a lower geometric mean of the bMIC values than their copper(II) counterparts (27.4 mg/L, 28.4 μM) for the C. haemulonii species complex. However, in comparison to the metal complexes containing perchlorate counter anions, the most active were manganese(II), silver(I), and copper(II) phen chelates containing coordinated dicarboxylate anions, which presented bMIC50 values below 10 μM for these opportunistic yeasts (Gandra et al., 2017).
Effects of Phendione and Its Metal Complexes on the Interaction Between P. verrucosa and Human Macrophages
Firstly, P. verrucosa conidial (2 × 106) viability was assessed after treatment with different concentrations of the test compounds. The XTT assay showed that only the highest concentration (2 × MIC) affected fungal viability after 20 h (Figure 2A). The pre-treatment of P. verrucosa conidia with the MIC value of each compound did not significantly affect the percentage of infected macrophages (Figure 2B). However, the number of intracellular viable conidia was significantly diminished (around 60%) when the fungal cells were pre-treated with [Ag(phendione)2]+, as judged by the CFU assay (Figure 2C).
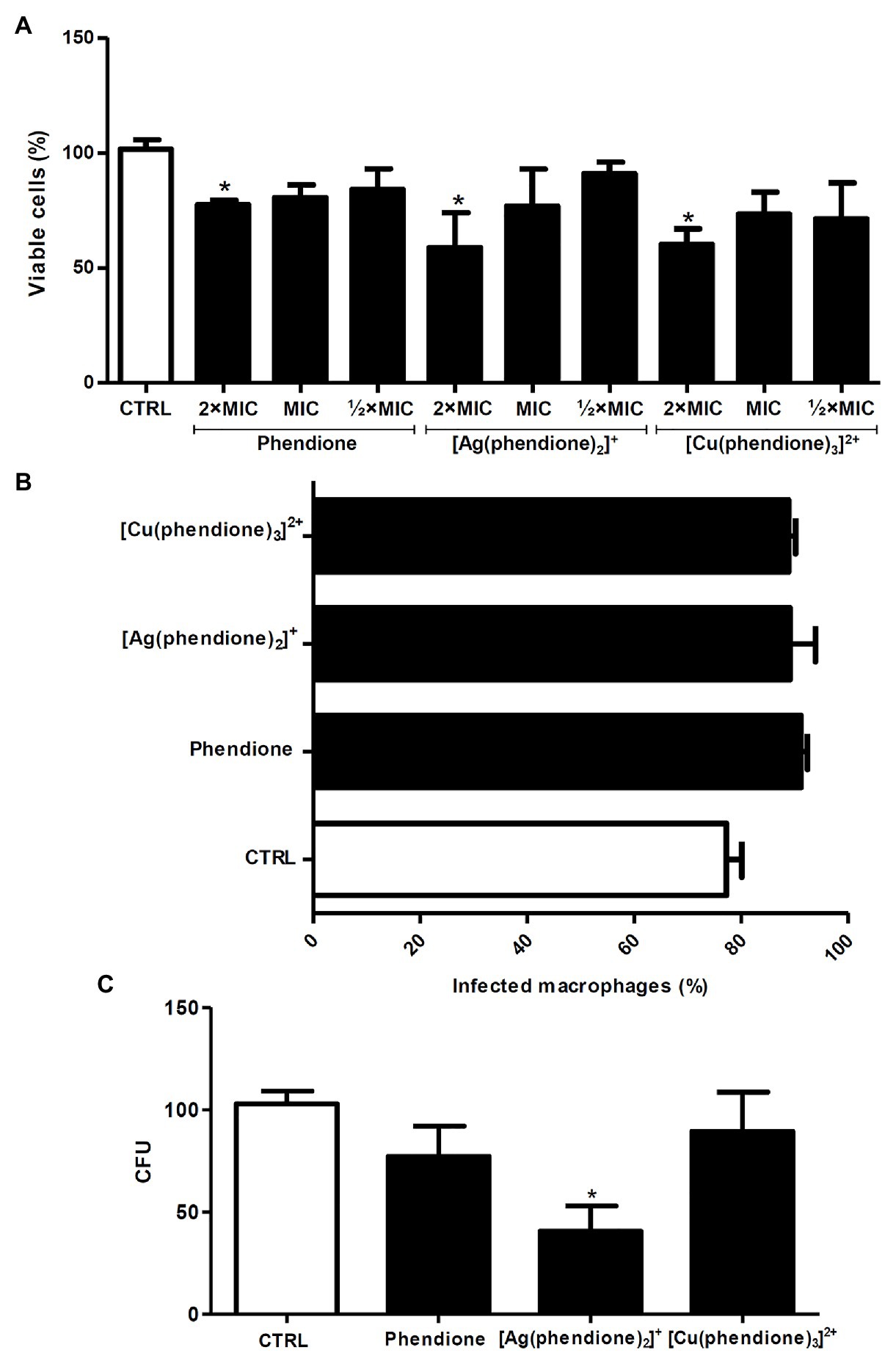
Figure 2. Effects of phendione and its metal complexes on P. verrucosa-macrophage interaction. (A) Conidia (2 × 106) were treated for 20 h with each test compound in order to establish non-cytotoxic concentrations using the XTT assay. Then, the fungal cells (B,C) were treated with the MIC values of each compound, washed, and added to 24-well plates containing macrophages, in a 1:10 ratio (macrophage:fungi). After 3 h at 37°C, (B) the cells were transferred to tubes and subjected to flow cytometry to determine the number of infected macrophages, (C) the cells were washed, lysed with sterile cold water and the colony forming units (CFU) counted. Systems containing non-treated conidia were also prepared (CTRL, control). The eluent (DMSO) did not affect fungal growth (data not shown). For the flow cytometry assay, conidia were stained with PKH26. The values represent the mean SD of three independent experiments performed in triplicate. Asterisks indicate values of p ≤ 0.05.
In order to investigate the killing capability of macrophages against P. verrucosa during the in vitro treatment, we firstly evaluated the effect of the test compounds on macrophage viability using the MTT assay. The results showed that phendione and its silver(I) complex were less toxic than the copper(II) complex, since around 90% of the macrophages remained viable following treatment with 1.25 (6 μM), 1.25 (2 μM), and 0.31 mg/L (0.31 μM) concentrations, respectively (Figure 3A). Similarly, previous cytotoxicity studies showed that animal cell lineages were more tolerant toward [Ag(phendione)2]+. McCann et al. (2012) showed that [Ag(phendione)2]+ (IC50 > 10 mg/L; 15.9 μM) was less toxic than [Cu(phendione)3]2+ (IC50 = 6.1 mg/L; 6.3 μM) when murine macrophages (RAW 264.7 lineage) were assayed. In addition, Gandra et al. (2017) assessed the toxicity of the same compounds against A549, a lung epithelial cell lineage, and showed that the CC50 value was significantly larger for the silver(I) complex (3.76 mg/L) than its copper(II) analogue (0.68 mg/L). Herein, we showed that the infected macrophages, subsequently treated for 20 h with non-toxic concentrations of phendione [1.25 mg/L (6 μM)], [Ag(phendione)2]+ [1.25 mg/L (2 μM)], and [Cu(phendione)3]2+ [0.31 mg/L (0.31 μM)], were able to reduce the viability of intracellular conidial cells by approximately 65, 60, and 70%, respectively (Figure 3B).
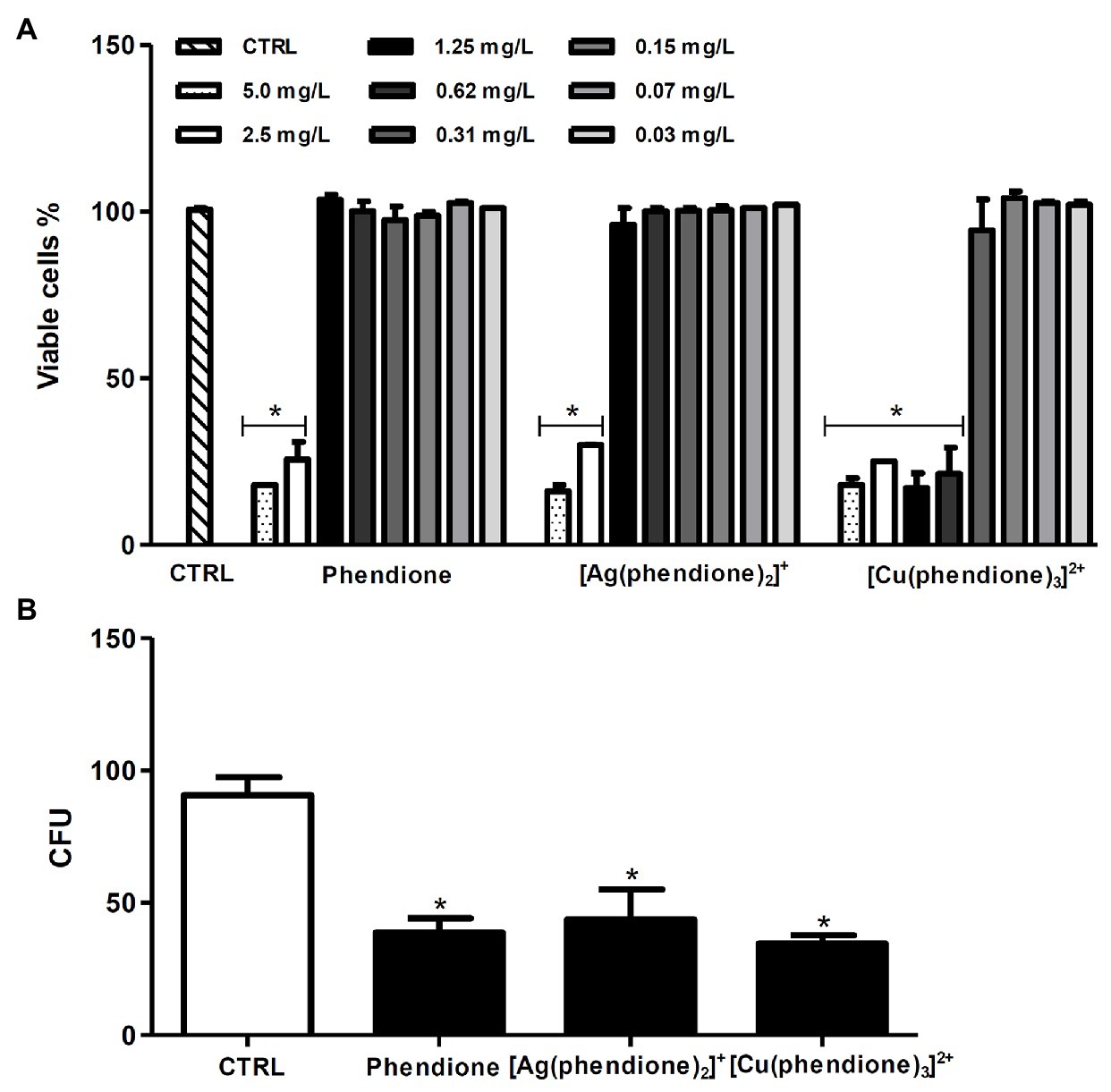
Figure 3. Effects of phendione and its metal complexes on THP-1 viability and the ability of macrophages to kill P. verrucosa conidia. (A) Macrophages (1 × 106) were incubated in the absence (CTRL, control) or in the presence of phendione and its silver(I) and copper(II) complexes (0.03–5 mg/L) for 20 h. After treatment, macrophage viability was determined using the MTT assay. (B) THP-1 cells were infected with P. verrucosa conidia in a ratio of 1:10 (macrophage:fungi) for 1 h. Then, non-adherent fungi were removed and the microplates incubated for an additional 20 h with non-cytotoxic concentrations [mg/L (μM)] of phendione [1.25 (6)], [Ag(phendione)2]+ [1.25 (2)], and [Cu(phendione)3]2+ [0.31 (0.31)]. Then, macrophages were washed and lysed with sterile cold water, and the suspensions were plated onto sabouraud dextrose agar (SDA) medium to count the number of colony forming units (CFU). The values represent the mean SD of three independent experiments performed in triplicate. Asterisks indicate values of p ≤ 0.05.
The mechanism of action of metal complexes of phen and phendione has not yet been fully established. However, the impact of these compounds on fungal cells can be attributed to their ability to induce different cellular changes, such as disruption of the cell membrane, rupture of internal organelles, nuclear fragmentation, sequestration of essential metals, and damage of the mitochondrial function (Coyle et al., 2003; McCann et al., 2012). In the previous study involving P. verrucosa conidia, we revealed some potential mechanisms of action of these test compounds (Granato et al., 2017). The phendione metal complexes were able to affect the production of fungal membrane ergosterol, thus possibly contributing to cell death. In addition, the complexes also inhibited the metallopeptidase activity of P. verrucosa conidia (Granato et al., 2017). Inhibition of this enzyme can prevent the fungal cells ability to access peptides and amino acids essential for their nutrition and thus restricting cell growth. From the current study, it is evident that addition of the test compounds improved the antifungal action of macrophages. The increase of compound-treated P. verrucosa susceptibility to macrophage killing could be related to an increase in the production of reactive oxygen species by these phagocytes since the respiratory burst is one of the most important mechanisms for the antimicrobial immunity of phagocytic cells (Herb and Schramm, 2021). Thus, the enhanced ability of macrophages to combat the fungal infection could be explained by the direct action of the test compounds on the intracellular conidia, and by the induction of intracellular macrophage mechanisms that mediate the antimicrobial immune responses, just as has previously been reported in regard to the anti-mycobacterial and antitumoral activities of phen- and phendione-based complexes (Kellett et al., 2011; McCarron et al., 2018). However, further studies are required in order to more fully understand the mechanisms involved in the anti- P. verrucosa activity of the test compounds.
Effects of [Ag(Phendione)2]+ on G. mellonella Infected With P. verrucosa
Although the copper(II) chelate was the most effective in disturbing a P. verrucosa mature biofilm, the silver(I) complex was less toxic toward THP-1 and it also affected the viability of conidial cells, at both pre- and post-treated proposals, in the in vitro interactions with macrophages. Thus, [Ag(phendione)2]+ was selected for the in vivo G. mellonella experiments. At dosages of 5 and 10 μg per larva, the silver(I) complex did not affect G. mellonella viability for a test period of up to 7 days (Figure 4A). This result corroborated the data obtained previously by McCann et al. (2012), who showed the low toxicity of phendione and its metal complexes for an exposure period of 72 h using the G. mellonella larvae as an experimental model. Similarly, the test compounds were also well tolerated by Swiss mice, as previously reported by McCann et al. (2012). In that study, phendione, [Ag(phendione)2]+, and [Cu(phendione)3]2+, at dosages up to 45 mg/kg, were injected into mice and after 7 days no deaths were recorded. Interestingly, only [Ag(phendione)2]+, administered at a concentration of 150 mg/kg over the same time period, did not cause mortality, indicating that this complex was the least toxic. Thus, mice tolerance was in the order [Ag(phendione)2]+ > phendione > [Cu(phendione)3]2+ (McCann et al., 2012).
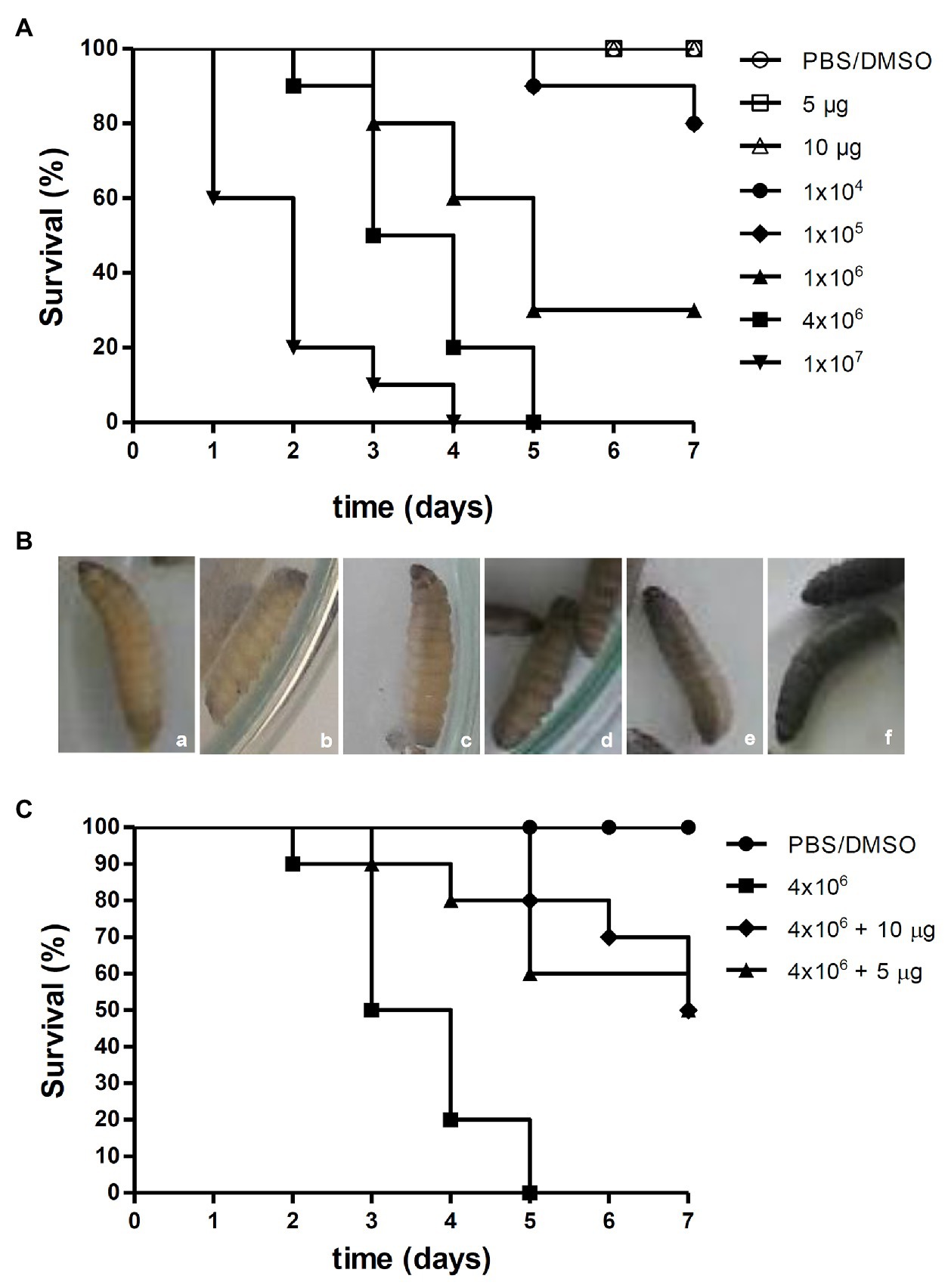
Figure 4. Effects of [Ag(phendione)2]+ on P. verrucosa infection of G. mellonella larvae. (A) Different cell densities of P. verrucosa conidia and [Ag(phendione)2]+, at concentrations of 5 and 10 μg/larva, were injected in G. mellonella larvae and the survival rate determined over a total period of 7 days. (B) Representative images of G. mellonella larvae 1 h post injection with (a) PBS/DMSO and different densities of P. verrucosa conidia (b) 1 × 104, (c) 1 × 105, (d) 1 × 106, (e) 4 × 106, and (f) 1 × 107. (C) Each larva was infected with P. verrucosa conidia (4 × 106), and after a period of 2 h was treated with the silver(I) complex at a concentration of either 5 or 10 μg/larva. Injection with only the PBS/DMSO eluent represents the control. The survival rate (10 larvae/systems) was monitored over 7 days, and death was assessed by the lack of larval movement in response to a stimulus.
The survival of G. mellonella larvae following infection with P. verrucosa was also studied (Figure 4A). After inoculation of larvae with the lowest fungal densities (1 × 104 and 1 × 105), 80% survived up to the last day of monitoring. However, 1 × 106 conidia induced larvae death gradually until the fifth day, and at the end of the incubation period only 30% survived. Administering a cell density of 4 × 106 conidia caused a reduction of 50% of viable larvae by the third day and 100% mortality by the fifth day. In addition, it was found that injection of 1 × 107 conidia triggered 80% of larval death by the second day and 100% by the fourth day (Figure 4A). These experiments demonstrated that the mortality of G. mellonella infected with P. verrucosa was dependent upon the fungal inoculum density. It is well known that disease outcomes are influenced by the quantity of the infective particles. However, tests using the same invertebrate model infected with Paracoccidioides lutzii and Histoplasma capsulatum revealed that these fungi were lethal to the larvae and without any correlation with cellular density (Thomaz et al., 2013). With the current infection assay using P. verrucosa, it was observed that the melanization of the larvae increased in accordance with dispensed cell density. In fact, larvae inoculated with the highest P. verrucosa cell density (1 × 107) immediately became melanized, whereas with cell densities of 1 × 106 and 4 × 106, the pigmentation was observed only after 1 h (Figure 4B). Larvae melanization, when exposed to other fungi, has been well documented (Li et al., 2013; Thomaz et al., 2013; Gandra et al., 2020). Like P. verrucosa, other non-albicans Candida species induced early larvae melanization and its progression was dependent upon inoculum density (Silva et al., 2018). However, premature larvae melanization was not observed following infection with Cryptococcus neoformans (Trevijano-Contador et al., 2015). The G. mellonella model was also used for studying the virulence of other fungi that cause chromoblastomycosis and phaeohyphomycosis, such as Fonsecaea spp. and Exophiala jeanselmei (Vicente et al., 2017; Huang et al., 2018). Huang et al. (2018) showed that the increase in fungal inoculum resulted in greater larval mortality. In contrast to P. verrucosa, the authors observed that F. monophora (1 × 106 conidia/larva) infection induced G. mellonella melanization only on the second day post injection. G. mellonella larvae melanization process occurs in response to trauma or microbial cell invasion through the activation of phenoloxidase (Trevijano-Contador and Zaragoza, 2019). The insect enzymatic system can be activated during the immune response after recognition of specific components, such as β-glucans in the fungal cell wall (Pereira et al., 2018; Trevijano-Contador and Zaragoza, 2019).
Addition of [Ag(phendione)2]+ at two non-toxic concentrations (5 and 10 μg/larva) to G. mellonella larvae which were pre-infected with P. verrucosa (inoculum density of 4 × 106 conidia), resulted in an intermediate rate of larval mortality. The results showed that by the third day of incubation, 50% of untreated larvae had perished, while 90% of larvae treated with the same two concentrations of the silver(I) complex remained alive (Figure 4C). On the fifth day, 100% mortality was recorded for the untreated larvae. However, 80% of larvae survived after [Ag(phendione)2]+ treatment at 10 μg per larva. At the lower concentration of added [Ag(phendione)2]+ (5 μg per larva) there was a 60% survival rate, suggesting a dose-dependent effect. It was also found that, following treatment with [Ag(phendione)2]+, 50% of larvae survived until the last day of monitoring (Figure 4C), thus clearly demonstrating a protective effect by the silver(I) complex. In their study of G. mellonella infected with Candida spp., Rowan et al. (2009) found that the larvae survival rate was increased after treatment with the silver(I) complex, [Ag2(mal)(phen)3] (mal H2 = malonic acid). Gandra et al. (2020) showed that different phen and phendione metal complexes, including [Ag(phendione)2]+, were able to inhibit C. haemulonii proliferation in G. mellonella infected larvae. In that study, the manganese(II) phen chelate containing the 3,6,9-trioxaundecanedioate dianion was the most effective. The antifungal efficacy against other fungi, such as Candida spp., Cryptococcus spp., Aspergillus spp., and Trichosporon spp. has also been assessed using the G. mellonella model (Kavanagh and Sheehan, 2018; Jemel et al., 2020). Thus, our present work has demonstrated that the G. mellonella in vivo model can be employed successfully to evaluate the antifungal effectiveness of phendione complexes presenting in vitro anti-P. verrucosa activity.
Conclusion
The silver(I) phendione complex, [Ag(phendione)2]+, is capable of reducing the viability of P. verrucosa conidia following interaction with human macrophages in vitro, and also conferring protection, in vivo, to G. mellonella larvae infected with the fungus. Transition metal complexes represent a novel group of antifungal agents due to their multi-modal activity, low cost, ease of synthesis, and their relatively high tolerance in in vitro animal cells as well as in lower mammals and insects used routinely as in vivo experimental models. Further studies are ongoing in an effort to understand the mechanisms of action of these metal complexes against P. verrucosa and other fungi that also cause chromoblastomycosis and phaeohyphomycosis. This advancement will further inform and facilitate the development of more effective compounds with reduced toxicity and enhanced potency against the microbes responsible for these debilitating diseases.
Data Availability Statement
All datasets generated for this study are included in the article/supplementary material.
Author Contributions
MG, MDP, MCP, MM, MD, MB, AS, and LK conceived and designed the study and wrote and revised the paper. MG, TM, RN, and TR performed the experiments. All authors analyzed the data. MDP, MCP, MM, MD, MB, AS, and LK contributed the reagents, materials, and/or analysis tools. All authors contributed to the research, approved the final version of the manuscript, and agree to be accountable for all aspects of the work.
Funding
This study was supported by grants from the Brazilian agencies: Conselho Nacional de Desenvolvimento Científico e Tecnológico (CNPq), Fundação de Amparo à Pesquisa no Estado do Rio de Janeiro (FAPERJ), Coordenação de Aperfeiçoamento de Pessoal de Nível Superior (CAPES, financial code 001), and Fundação Oswaldo Cruz (FIOCRUZ).
Conflict of Interest
The authors declare that the research was conducted in the absence of any commercial or financial relationships that could be construed as a potential conflict of interest.
References
Anthony, E. J., Bolitho, E. M., Bridgewater, H. E., Carter, O. W., Donnelly, J. M., Imberti, C., et al. (2020). Metallodrugs are unique: opportunities and challenges of discovery and development. Chem. Sci. 11:12888. doi: 10.1039/D0SC04082G
Batista-Silva, L. R., Rodrigues, L. S., Vivarini, A. C., Costa, F. M. R., Mattos, K. A., Costa, M. R. S. N., et al. (2016). Mycobacterium leprae-induced insulin-like growth factor I attenuates antimicrobial mechanisms, promoting bacterial survival in macrophages. Sci. Rep. 6:27632. doi: 10.1038/srep27632
Brito, A. C., and Bittencourt, M. J. S. (2018). Chromoblastomycosis: an etiological, epidemiological, clinical, diagnostic, and treatment update. An. Bras. Dermatol. 93, 495–506. doi: 10.1590/abd1806-4841.20187321
Campos-Herrero, M. I., Tandón, L., Horcajada, I., and Medina-Rivero, F. (2012). Endophthalmitis caused by Phialophora verrucosa: A case report and literature review of Phialophora ocular infections. Enferm. Infecc. Microbiol. Clin. 30, 163–166. doi: 10.1016/j.eimc.2011.10.012
Chudzik, B., Bonio, K., Dabrowski, W., Pietrzak, D., Niewiadomy, A., Olender, A., et al. (2019). Synergistic antifungal interactions of amphotericin B with 4-(5-methyl-1,3,4-thiadiazole-2-yl) benzene-1,3-diol. Sci. Rep. 9:12945. doi: 10.1038/s41598-019-49425-1
Chudzik, B., Tracz, I. B., Czernel, G., Fiołka, M. J., Borsuk, G., and Gagoś, M. (2013). Amphotericin B-copper(II) complex as a potential agent with higher antifungal activity against Candida albicans. Eur. J. Pharm. Sci. 49, 850–857. doi: 10.1016/j.ejps.2013.06.007
Claudel, M., Schwarte, J. V., and Fromm, K. M. (2020). New antimicrobial strategies based on metal complexes. Chemistry 2, 849–899. doi: 10.3390/chemistry2040056
CLSI (2008). Clinical Laboratory Standards Institute: Reference Method for Broth Dilution Antifungal Susceptibility Testing of Filamentous Fungi, 2nd Edn., Approved Standard M38-A2. Wayne, PA: CLSI.
Costa-Orlandi, C. B., Sardi, J. C. O., Pitangui, N. S., Oliveira, H. C., Scorzoni, L., Galeane, M. C., et al. (2017). Fungal biofilms and polymicrobial diseases. J. Fungi 3:22. doi: 10.3390/jof3020022
Coyle, B., Kavanagh, K., McCann, M., Devereux, M., and Geraghty, M. (2003). Mode of anti-fungal activity of 1, 10-phenanthroline and its Cu(II), Mn(II) and Ag(I) complexes. Biometals 16, 321–329. doi: 10.1023/A:1020695923788
Ersland, K., Wüthrich, M., and Klein, B. S. (2010). Dynamic interplay among monocyte derived, dermal, and resident lymph node dendritic cells during the generation of vaccine immunity to fungi. Cell Host Microbe 7, 474–487. doi: 10.1016/j.chom.2010.05.010
Eshwika, A., Coyle, B., Devereux, M., McCann, M., and Kavanagh, K. (2004). Metal complexes of 1,10-phenanthroline-5,6-dione alter the susceptibility of the yeast Candida albicans to amphotericin B and miconazole. Biometals 17, 415–422. doi: 10.1023/B:BIOM.0000029438.97990.c6
Fernandes, C. M., Fonseca, F. L., Goldman, G. H., Pereira, M. D., and Kurtenbach, E. (2017). A reliable assay to evaluate the virulence of Aspergillus nidulans using the alternative animal model Galleria mellonella (Lepidoptera). Bio-Protocol 7:e2329. doi: 10.21769/BioProtoc.2329
Gagoś, M., Czernel, G., Kamiński, D. M., and Kostro, K. (2011). Spectroscopic studies of amphotericin B-Cu2+ complexes. Biometals 24:915. doi: 10.1007/s10534-011-9445-2
Gandra, R. M., McCarron, P., Fernandes, M. F., Ramos, L. S., Mello, T. P., Aor, A. C., et al. (2017). Antifungal potential of copper(II), manganese(II) and silver(I) 1,10-phenanthroline chelates against multidrug-resistant fungal species forming the Candida haemulonii complex: impact on the planktonic and biofilm lifestyles. Front. Microbiol. 8:1257. doi: 10.3389/fmicb.2017.01257
Gandra, R. M., McCarron, P., Viganor, L., Fernandes, M. F., Kavanagh, K., McCann, M., et al. (2020). In vivo activity of copper(II), manganese(II), and silver(I) 1,10-phenanthroline chelates against Candida haemulonii using the Galleria mellonella model. Front. Microbiol. 11:470. doi: 10.3389/fmicb.2020.00470
Geraghty, M., Cronin, J. F., Devereux, M., and McCann, M. (2000). Synthesis and antimicrobial activity of copper (II) and manganese (II) α,ω-dicarboxylate complexes. Biometals 13, 1–8. doi: 10.1023/A:1009271221684
Granato, M. Q., Gonçalves, D. S., Seabra, S. H., McCann, M., Devereux, M., Santos, A. L. S., et al. (2017). 1,10-phenanthroline-5,6-dione-based compounds are effective in disturbing crucial physiological events of Phialophora verrucosa. Front. Microbiol. 8:76. doi: 10.3389/fmicb.2017.00076
Granato, M. Q., Massapust, P. A., Rozental, S., Alviano, C. S., Santos, A. L. S., and Kneipp, L. F. (2015). 1,10-phenanthroline inhibits the metallopeptidase secreted by Phialophora verrucosa and modulates its growth, morphology and differentiation. Mycopathologia 179, 231–242. doi: 10.1007/s11046-014-9832-7
Hartland, K., Pu, J., Palmer, M., Dandapani, S., Moquist, P. N., Munoz, B., et al. (2016). Highthroughput screen in identifies a novel molecular scaffold that inhibits cell wall integrity pathway signaling. ACS Infect. Dis. 2, 93–102. doi: 10.1021/acsinfecdis.5b00111
Herb, M., and Schramm, M. (2021). Functions of ROS in macrophages and antimicrobial immunity. Antioxidants 10:313. doi: 10.3390/antiox10020313
Hoffman, A. E., Miles, L., Greenfield, T. J., Shoen, C., DeStefano, M., Cynamon, M., et al. (2015). Clinical isolates of Candida albicans, Candida tropicalis, and Candida krusei have different susceptibilities to Co(II) and Cu(II) complexes of 1,10-phenanthroline. Biometals 28, 415–423. doi: 10.1007/s10534-015-9825-0
Hofmann, H., Choi, S. M., Wilsmann-Theis, D., Horré, R., de Hoog, G. S., and Bieber, T. (2005). Invasive chromoblastomycosis and sinusitis due to Phialophora verrucosa in a child from northern Africa. Mycoses 48, 456–461. doi: 10.1111/j.1439-0507.2005.01150.x
Huang, X., Liu, Y., Xi, L., Zeng, K., and Mylonakis, E. (2018). Galleria mellonella as a model invertebrate host for the study of muriform cells of dematiaceous fungi. Future Microbiol. 13, 1021–1028. doi: 10.2217/fmb-2018-0036
Jemel, S., Guillot, J., Kallel, K., Botterel, F., and Dannaoui, E. (2020). Galleria mellonella for the evaluation of antifungal efficacy against medically important fungi, a narrative review. Microorganisms 8:390. doi: 10.3390/microorganisms8030390
Kaur, S., and Singh, S. (2014). Biofilm formation by Aspergillus fumigatus. Med. Mycol. 52, 2–9. doi: 10.3109/13693786.2013.819592
Kavanagh, K., and Sheehan, G. (2018). The use of Galleria mellonella larvae to identify novel antimicrobial agents against fungal species of medical interest. J. Fungi 4:E113. doi: 10.3390/jof4030113
Kellett, A., O’Connor, M., McCann, M., Howe, O., Casey, A., McCarron, P., et al. (2011). Water-soluble bis(1,10-phenanthroline) octanedioate Cu2+ and Mn2+ complexes with unprecedented nano and picomolar in vitro cytotoxicity: promising leads for chemotherapeutic drug development. Med. Chem. Commun. 2, 579–584. doi: 10.1039/c0md00266f
Kirchhoff, L., Olsowski, M., Zilmans, K., Dittmer, S., Haase, G., Sedlacek, L., et al. (2017). Biofilm formation of the black yeast-like fungus Exophiala dermatitidis and its susceptibility to antiinfective agents. Sci. Rep. 7:42886. doi: 10.1038/srep42886
Lemire, J., Harrison, J., and Turner, R. (2013). Antimicrobial activity of metals: mechanisms, molecular targets and applications. Nat. Rev. Microbiol. 11, 371–384. doi: 10.1038/nrmicro3028
Li, D.-D., Deng, L., Hu, G.-H., Zhao, L. X., Hu, D.-D., Jiang, Y.-Y., et al. (2013). Using Galleria mellonella-Candida albicans infection model to evaluate antifungal agents. Biol. Pharm. Bull. 36, 1482–1487. doi: 10.1248/bpb.b13-00270
Li, Y., Wan, Z., and Li, R. (2014). In vitro activities of nine antifungal drugs and their combinations against Phialophora verrucosa. Antimicrob. Agents Chemother. 58, 5609–5612. doi: 10.1128/AAC.02875-14
Liu, M., Seidel, V., Katerere, D. R., and Gray, A. I. (2007). Colorimetric broth microdilution method for the antifungal screening of plant extracts against yeasts. Methods 42, 325–329. doi: 10.1016/j.ymeth.2007.02.013
Lundstrom, T. S., Fairfax, M. R., Dugan, M. C., Vazquez, J. A., Chandrasekar, P. H., Abella, E., et al. (1997). Phialophora verrucosa infection in a BMT patient. Bone Marrow Transplant. 20, 789–791. doi: 10.1038/sj.bmt.1700969
Maurer, E., Hörtnagl, C., Lackner, M., Grässle, D., Naschberger, V., Moser, P., et al. (2019). Galleria mellonella as a model system to study virulence potential of mucormycetes and evaluation of antifungal treatment. Med. Mycol. 57, 351–362. doi: 10.1093/mmy/myy042
McCann, M., Coyle, B., McKay, S., McCormack, P., Kavanagh, K., Devereux, M., et al. (2004). Synthesis and X-ray crystal structure of [Ag(phendio)2]ClO4 (phendio = 1,10-phenanthroline-5,6-dione) and its effects on fungal and mammalian cells. Biometals 17, 635–645. doi: 10.1007/s10534-004-1229-5
McCann, M., Geraghty, M., Devereux, M., O’Shea, D., Mason, J., and O’Sullivan, L. (2000). Insights into the mode of action of the anti-Candida activity of 1,10-phenanthroline and its metal chelates. Met. Based Drugs 7, 185–193. doi: 10.1155/MBD.2000.185
McCann, M., Santos, A. L. S., Silva, B. A., Romanos, M. T. V., Pyrrho, A. S., Devereux, M., et al. (2012). In vitro and in vivo studies into the biological activities of 1,10-phenanthroline, 1,10-phenanthroline-5, 6-dione and its copper(II) and silver(I) complexes. Toxicol. Res. 1, 47–54. doi: 10.1039/c2tx00010e
McCarron, P., McCann, M., Devereux, M., Kavanagh, K., Skerry, C., Karakousis, P. C., et al. (2018). Unprecedented in vitro antitubercular activitiy of manganese(II) complexes containing 1,10-phenanthroline and dicarboxylate ligands: increased activity, superior selectivity, and lower toxicity in comparison to their copper(II) analogs. Front. Microbiol. 9:1432. doi: 10.3389/fmicb.2018.01432
Mello, T. P., Aor, A. C., Gonçalves, D. S., Seabra, S. H., Branquinha, M. H., and Santos, A. L. S. (2016). Assessment of biofilm formation by Scedosporium apiospermum, S. aurantiacum, S. minutisporum and Lomentospora prolificans. Biofouling 32, 737–749. doi: 10.1080/08927014.2016.1192610
Mjos, K. D., and Orvig, C. (2014). Metallodrugs in medicinal inorganic. Chem. Rev. 114, 4540–4563. doi: 10.1021/cr400460s
Mosmann, T. (1983). Rapid colorimetric assay for cellular growth and survival: application to proliferation and cytotoxicity assays. J. Immunol. Methods 65, 55–63. doi: 10.1016/0022-1759(83)90303-4
Mowat, E., Butcher, J., Lang, S., Williams, C., and Ramage, G. (2007). Development of a simple model for studying the effects of antifungal agents on multicellular communities of Aspergillus fumigatus. J. Med. Microbiol. 56, 1205–1212. doi: 10.1099/jmm.0.47247-0
Palmeira, V. F., Kneipp, L. F., Rozental, S., Alviano, C. S., and Santos, A. L. S. (2008). Beneficial effects of HIV peptidase inhibitors on Fonsecaea pedrosoi: promising compounds to arrest key fungal biological processes and virulence. PLoS One 3:e3382. doi: 10.1371/journal.pone.0003382
Pereira, T. C., Barros, P. P., Fugisaki, L. R. O., Rossoni, R. D., Ribeiro, F. C., Menezes, R. T., et al. (2018). Recent advances in the use of Galleria mellonella model to study immune responses against human pathogens. J. Fungi 4:128. doi: 10.3390/jof4040128
Qiu, Y., Zhang, J., Tang, Y., Zhong, X., and Deng, J. (2019). Case report: fever- pneumonia- lymphadenectasis- osteolytic- subcutaneous nodule: disseminated chromoblastomycosis caused by Phialophora. J. Infect. Chemother. 25, 1031–1036. doi: 10.1016/j.jiac.2019.05.002
Queiroz-Telles, F., de Hoog, S., Santos, D. W. C. L., Salgado, C. G., Vicente, V. A., Bonifaz, A., et al. (2017). Chromoblastomycosis. Clin. Microbiol. Rev. 30, 233–276. doi: 10.1128/CMR.00032-16
Ramage, G., Rajendran, R., Sherry, L., and Williams, C. (2012). Fungal biofilm resistance. Int. J. Microbiol. 2012:528521. doi: 10.1155/2012/528521
Romera, D., Aguilera-Correa, J. J., Gadea, I., Vinuela-Sandoval, L., Garcia-Rodriguez, J., and Esteban, J. (2019). Candida auris: a comparison between planktonic and biofilm susceptibility to antifungal drugs. J. Med. Microbiol. 68, 1353–1358. doi: 10.1099/jmm.0.001036
Rowan, R., Moran, C., McCann, M., and Kavanagh, K. (2009). Use of Galleria mellonella larvae to evaluate the in vivo anti-fungal activity of [Ag2(mal)(phen)3]. Biometals 22, 461–467. doi: 10.1007/s10534-008-9182-3
Santos, A. L. S., Galdino, A. C. M., Mello, T. P., Ramos, L. S., Branquinha, M. H., Bolognese, A. M., et al. (2018). What are the advantages of living in a community? A microbial biofilm perspective! Mem. Inst. Oswaldo Cruz 113:e180212. doi: 10.1590/0074-02760180212
Sardi, J. C. O., Pitangui, N. S., Voltan, A. R., Braz, J. D., Machado, M. P., Fusco-Almeida, A. M., et al. (2015). In vitro Paracoccidioides brasiliensis biofilm and gene expression of adhesins and hydrolytic enzymes. Virulence 6, 642–651. doi: 10.1080/21505594.2015.1031437
Silva, L. N., Campos-Silva, R., Ramos, L. S., Trentin, D. S., Macedo, A. J., Branquinha, M. H., et al. (2018). Virulence of Candida haemulonii complex in Galleria mellonella and efficacy of classical antifungal drugs: A comparative study with other clinically relevant non-albicans Candida species. FEMS Yeast Res. 18:foy082. doi: 10.1093/femsyr/foy082
Spitzer, M., Robbins, N., and Wright, G. D. (2017). Combinatorial strategies for combating invasive fungal infections. Virulence 8, 169–185. doi: 10.1080/21505594.2016.1196300
Thomaz, L., García-Rodas, R., Guimarães, A. J., Taborda, C. P., Zaragoza, O., and Nosanchuk, J. D. (2013). Galleria mellonella as a model host to study Paracoccidioides lutzii and Histoplasma capsulatum. Virulence 4, 139–146. doi: 10.4161/viru.23047
Tong, Z., Chen, S. C., Chen, L., Dong, B., Li, R., Hu, Z., et al. (2013). Generalized subcutaneous phaeohyphomycosis caused by Phialophora verrucosa: report of a case and review of literature. Mycopathologia 175, 301–306. doi: 10.1007/s11046-013-9626-3
Trevijano-Contador, N., Herrero-Fernández, I., García-Barbazán, I., Scorzoni, L., Rueda, C., Rossi, S. A., et al. (2015). Cryptococcus neoformans induces antimicrobial responses and behaves as a facultative intracellular pathogen in the non mammalian model Galleria mellonella. Virulence 6, 66–74. doi: 10.4161/21505594.2014.986412
Trevijano-Contador, N., and Zaragoza, O. (2019). Immune response of Galleria mellonella against human fungal pathogens. J. Fungi 5:3. doi: 10.3390/jof5010003
Treviño-Rangel, R. J., Villanueva-Lozano, H., Méndez-Galomo, K. S., Solís-Villegas, E. M., Becerril-García, M. A., Montoya, A. M., et al. (2019). In vivo evaluation of the antifungal activity of sertraline against Aspergillus fumigatus. J. Antimicrob. Chemother. 74, 663–666. doi: 10.1093/jac/dky455
Turiansky, G. W., Benson, P. M., Sperling, L. C., Sau, P., Salkin, I. F., McGinnis, M. R., et al. (1995). Phialophora verrucosa: a new cause of mycetoma. J. Am. Acad. Dermatol. 32, 311–315. doi: 10.1016/0190-9622(95)90393-3
Ventura, R. F., Galdino, A. C. M., Viganor, L., Schuenck, R. P., Devereux, M., McCann, M., et al. (2020). Antimicrobial action of 1,10-phenanthroline-based compounds on carbapenemase-producing Acinetobacter baumannii clinical strains: efficacy against planktonic- and biofilm-growing cells. Braz. J. Microbiol. 51, 1703–1710. doi: 10.1007/s42770-020-00351-9
Vicente, V. A., Weiss, V. A., Bombassaro, A., Moreno, L. F., Costa, F. F., Raittz, R. T., et al. (2017). Comparative genomics of sibling species of Fonsecaea associated with human chromoblastomycosis. Front. Microbiol. 8:1924. doi: 10.3389/fmicb.2017.01924
Viganor, L., Galdino, A. C. M., Nunes, A. P. F., Santos, K. R. N., Branquinha, M. H., Devereux, M., et al. (2016). Anti-Pseudomonas aeruginosa activity of 1,10-phenanthroline-based drugs against both planktonic- and biofilm-growing cells. J. Antimicrob. Chemother. 71, 128–134. doi: 10.1093/jac/dkv292
Viganor, L., Howe, O., McCarron, P., McCann, M., and Devereux, M. (2017). The antibacterial activity of metal complexes containing 1,10-phenanthroline: potential as alternative therapeutics in the era of antibiotic resistance. Curr. Top. Med. Chem. 17, 1280–1302. doi: 10.2174/1568026616666161003143333
Vila, T. V. M., and Rozental, S. (2016). “Biofilm Formation as a Pathogenicity Factor of Medically Important Fungi,” in Fungal Pathogenicity. ed. S. Sultan (London: InTech), 978–953.
Keywords: 1,10-phenanthroline-5,6-dione, chromoblastomycosis, dematiaceous fungi, antifungal activity, cellular interaction
Citation: Granato MQ, Mello TP, Nascimento RS, Pereira MD, Rosa TLSA, Pessolani MCV, McCann M, Devereux M, Branquinha MH, Santos ALS and Kneipp LF (2021) Silver(I) and Copper(II) Complexes of 1,10-Phenanthroline-5,6-Dione Against Phialophora verrucosa: A Focus on the Interaction With Human Macrophages and Galleria mellonella Larvae. Front. Microbiol. 12:641258. doi: 10.3389/fmicb.2021.641258
Edited by:
Ruoyu Li, Peking University First Hospital, ChinaReviewed by:
Erin E. McClelland, Independent Researcher, Murfreesboro, TN, United StatesMarta Palusinska-Szysz, Marie Curie-Sklodowska University, Poland
Copyright © 2021 Granato, Mello, Nascimento, Pereira, Rosa, Pessolani, McCann, Devereux, Branquinha, Santos and Kneipp. This is an open-access article distributed under the terms of the Creative Commons Attribution License (CC BY). The use, distribution or reproduction in other forums is permitted, provided the original author(s) and the copyright owner(s) are credited and that the original publication in this journal is cited, in accordance with accepted academic practice. No use, distribution or reproduction is permitted which does not comply with these terms.
*Correspondence: Lucimar F. Kneipp, bHVjaW1hckBpb2MuZmlvY3J1ei5icg==