- 1Postgraduate Program in Nutrition, Universidade Federal de São Paulo (UNIFESP), São Paulo, Brazil
- 2Postgraduate Program in Interdisciplinary Health Science, Universidade Federal de São Paulo (UNIFESP), São Paulo, Brazil
- 3Department of Chemical Engineering, Universidade Federal de São Paulo (UNIFESP), São Paulo, Brazil
- 4Nutrition and Food Service Research Center, Universidade Federal de São Paulo (UNIFESP), São Paulo, Brazil
Propolis is a resinous material rich in flavonoids and involved in several biological activities such as antimicrobial, fungicide, and antiparasitic functions. Conventionally, ethanolic solutions are used to obtain propolis phytochemicals, which restrict their use in some cultures. Given this, we developed an alcohol-free high-performance extractive approach to recover antibacterial and antioxidants phytochemicals from red propolis. Thus, aqueous-solutions of ionic liquids (IL) and eutectic solvents were used and then tested for their total flavonoids, antioxidant, and antimicrobial activities. The surface-responsive technique was applied regarding some variables, namely, the time of extraction, the number of extractions, and cavitation power (W), to optimize the process (in terms of higher yields of flavonoids and better antioxidant activity). After that, four extractions with the same biomass (repetitions) using 1-hexyl-3-methylimidazolium chloride [C6mim]Cl, under the operational conditions fixed at 3.3 min and 300 W, were able to recover 394.39 ± 36.30 mg RuE. g−1 of total flavonoids, with total antioxidant capacity evaluated up to 7595.77 ± 5.48 μmol TE. g−1dried biomass, besides inhibiting the growth of Staphylococcus aureus and Salmonella enteritidis bacteria (inhibition halo of 23.0 ± 1.0 and 15.7 ± 2.1, respectively). Aiming at the development of new technologies, the antimicrobial effect also presented by [C6mim]Cl may be appealing, and future studies are required to understand possible synergistic actions with propolis phytochemicals. Thereby, we successfully applied a completely alcohol-free method to obtain antimicrobials phytochemicals and highly antioxidants from red propolis, representing an optimized process to replace the conventional extracts produced until now.
Introduction
Propolis is associated broad spectrum of activities, namely, fungicide (Marques das Neves et al., 2016), antioxidant, and antiparasitic (do Nascimento et al., 2016; Dantas Silva et al., 2017). Moreover, the impact generated by the pandemic COVID-19 has motivated the studies of propolis in action against infection by SARS-CoV-2 (Berretta et al., 2020; Refaat et al., 2021), whereas this natural product has anti-inflammatory properties (Lima et al., 2014; Washio et al., 2015; Kitamura et al., 2018) and action against some virus species (Hazem et al., 2017; Kwon et al., 2020). Recently, a clinical trial conducted in Brazil (National Clinical Trial Number: NCT04480593) has shown the efficacy of green propolis in supporting treatment in patients hospitalized with COVID-19 (Silveira et al., 2021).
Brazilian red propolis demonstrated strong cytotoxic potential in vitro, suggesting a potential therapeutic alternative for treatment against Chagas disease (Dantas Silva et al., 2017), schistosomiasis (Silva et al., 2021), and some types of cancer (da Silva Frozza et al., 2013; Dantas Silva et al., 2017; Banzato et al., 2020). These properties are related to propolis complex chemical composition, including formononetin, isoliquiritigenin, liquiritigenin, and biochanin A, as described by da Silva Frozza et al. (2013).
Traditionally, ethanolic solutions are the most conventional solvent used to obtain phytochemicals from red propolis. However, hydroalcoholic extracts’ consumption has some restrictions in specific cultures (Muslim; Al-Ansari et al., 2019). Also, envisioning the purification of some specific molecules with different polarities, other volatile organic solvents (VOSs) are used such as hexane, methanol, and chloroform (Oldoni et al., 2011; Marques das Neves et al., 2016). These solvents are widely used in several industrial segments; however, beyond toxicity, there are risks for workers (high-volatility) and damage to the environment (environmental toxicity). Thus, alternative technologies have been required in order to replace these solvents with more sustainable alternatives (Choi and Verpoorte, 2019). In this scenario, the development of extraction methods using alternative solvents to replace VOS becomes a promising strategy for future commercial applications, which increases the number of applications in some industrial sectors, such as food, cosmetic, and pharmaceutic ones. Among the alternative solvents, ionic liquids (ILs) and eutectic solvents (ESs) stand out as promising candidates to replace VOS (Passos et al., 2014; Ventura et al., 2017). The non-volatile and flammability suggest that these compounds are an essential alternative in developing new extractive processes (Nam et al., 2015). Ionic liquid, for example, can assume several properties, it has been applied to obtain several molecules with different polarities, such as carotenoids (hydrophobic compounds; Xiao et al., 2018; de Souza Mesquita et al., 2019, 2020a,b; Murador et al., 2019a,b), as well as flavanones (hydrophilic compounds), depending on the ionic composition (Xiao et al., 2018). Another advantage of IL is the possibility to recycle and reuse, thus contributing to a low carbon footprint in the process (de Souza Mesquita et al., 2019, 2020a,b). The ES also has a high potential to extract bioactive compounds from natural products, including flavonoids and phenolic compounds, since they are considered as designer solvents and IL. In some cases, ES could improve the bioavailability and antioxidant activity of the extracts, which are considered as a promising characteristic for new applications, especially in the food sector (Meng et al., 2018; Funari et al., 2019; Liu et al., 2019; Murador et al., 2019a).
Using an alternative extraction strategy in terms of high-performance and transposing the limitations on the use of ethanol would make it possible to obtain a commercially differentiated red propolis extract obtained by alternative solvents. Thereby, this work aims to establish a new method of extracting antioxidant and antimicrobial phytochemicals from red propolis using alternative solvents, creating new possibilities for applying this biomass, which is considered as extremely important for different purposes.
Material and Methods
Materials
The following standards were used: rutin hydrate, formononetin, daidzein, and 6-hydroxy-2,5,7,8-tetramethylchroman-2-carboxylic acid (Trolox) from Sigma-Aldrich (Darmstadt, Germany). The reagents 1-methylimidazolium, 1-chlorobutane, and potassium hexafluorophosphate; IL 1-n-butyl-3-methylimidazolium tetrafluoroborate ([C4mim][BF4]) and 1-hexyl-3-methylimidazolium chloride ([C6mim]Cl); choline chloride, glycerol, 1,4-butanediol, and levulinic acid from Sigma-Aldrich (Darmstadt, Germany). Reagents sodium nitrite (Vetec®) and aluminum chloride (Proquímicos®); Fluorescein sodium salt and α, α′-Azodiisobutyramidine dihydrochloride (AAPH) from Sigma-Aldrich (Darmstadt, Germany). Culture mediums: Mueller Hinton Agar (Himedia®) and Nutrient broth (Himedia®). Salmonella enteritidis ATCC 13076 and Staphylococcus aureus ATCC 19095 were supplied by the Bacterial Culture Collection of the Oswaldo Cruz Institute – FIOCRUZ (Manguinhos, Rio de Janeiro, Brazil). Vancomycin (30 μg/disc) and Meropenem (10 μg/disc; DME®) antibiotic filter paper discs and inert filter paper discs (DME®).
Samples
The samples of red propolis were purchased from an apiary located in Porto de Pedras’ municipality, in the state of Alagoas (Latitude: 9° 09' 11" S, Longitude: 35° 17'19" 17'19" W) in April 2018, by donation. The sample was composed of a single batch consisting of 350 g of sample and was lyophilized for 48 h, processed using an analytical mill (Ika®, model A11 Basic), vacuum-packed in sealer (TECMAQ®, model TM250), and maintained frozen at −40°C.
Extraction of Flavonoids From Red Propolis
Convectional Extraction
Ethanol was applied as a control to obtain red propolis extract. Thus, results using ethanolic solutions (70 and 95% v:v) were compared and evaluated with the developed method’s extractive performance based on alternative solvents. The homogenization was performed in an ultrasonic probe (500 W, 20 kHz, 4 mm in diameter; Unique, model DES500, Brazil) at 400 W for 5 min, followed by centrifugation at 4700 rpm for 15 min (process repeated twice). The solid-liquid ratio (RS/L) was fixed at 1:3 (0.5 g of sample for 1.5 g of solvent). The procedure was performed in triplicate with three extractions for each sample. The supernatants were stored in a freezer at −40°C for further analysis.
Extraction of Flavonoids From Red Propolis With Ionic Liquids and Eutectic Solvents
Preliminary Tests
In total, four different IL were evaluated: 1-butyl-3-methylimidazolium tetrafluoroborate ([C4mim][BF4]) and 1-hexyl-3-methylimidazolium chloride ([C6mim]Cl) – which were obtained commercially, 1-butyl-3-methylimidazolium chloride ([C4mim]Cl) and 1-butyl-3-methylimidazolium hexafluorophosphate ([C4mim][PF6]) both synthesized in the laboratory, as described by Martins and de Rosso (2016). ([C4mim][BF4]) was synthesized in a round-bottom flask by mixing 0.10 mol of 1-methylimidazole and 0.10 mol of 1-chloro-butane. The mixture was stirred and refluxed (70–80°C) for 72 h. A viscous yellow liquid, which was washed twice with dichloromethane, was obtained. [C4mim]Cl was dried under vacuum at 100°C and crystallized at −40°C. [C4mim][PF6] was synthesized by a mixture containing 0.01 mol of [C4mim]Cl, and 0.01 mol of potassium hexafluorophosphate in distilled water was stirred vigorously for 45 min. The upper aqueous phase formed was separated and discarded; the remaining liquid was added to distilled water and stirred for 15 min. Then, 40 ml of chloroform was added. The solvent was evaporated under vacuum to yield a viscous liquid with a slightly yellow color. Besides, three ES were synthesized, associating choline chloride (CH) to three hydrogen donors: glycerol (CH–GLY), 1,4-butanediol (CH–BUT), and levulinic acid (CH–LEV), in a molar ratio of 1:2, as described by Liu et al. (2019). For each ES, 10% (g/g) water (co-solvent) was added and homogenized at 60°C for 10 min in a round-bottom flask.
The extractions were performed using a 1:3 solid-liquid ratio (RS/L; g/ml), as in conventional extraction. The samples were maintained in an ice bath, and the homogenization was performed in a 500 W, 4 mm in diameter, and 20 kHz ultrasonic probe (Unique, model DES500, Brazil) at 400 W for 5 min. Then, the samples were centrifuged at 4700 rpm for 15 min. This procedure was performed in triplicate with three extractions for each sample (using the same biomass). The supernatants were stored in a freezer at −40°C for further analysis.
Optimization of Extraction Conditions Using Response Surface Methodology
The most effective solvent for the extraction of flavonoids was submitted to a Central Composite Design (CCD) to optimize the process. The effects of the variables number of extractions, time of extraction (Tmin), and cavitation power (W) were assessed using the Central Composite Design Rotational (CCDR; 23), with six axial points (calculated by the interpolation considering the experimental values of factorial and central points) and three central points, totaling 17 trials. ILs, as well as ES, it has a high viscosity, and since propolis biomass is a very resinous substance, both factors difficult the extraction process; Thus, the variable (RS/L) was fixed at 1:3, as it was the minimum amount of solvent that enabled a less viscous liquid for cavitation. The response was measured by total flavonoid yield and antioxidant activity using the oxygen radical absorbance capacity (ORAC) method.
Model Validation
An experiment contemplating the best extraction conditions was carried out, in triplicate, to validate the obtained model and verify the reproducibility of the method and compare the average of the extracts with the predicted model obtained in the experimental design.
Quantification of Total Flavonoids
The total content of flavonoids present in red propolis extract was quantified according to the method exposed by Zhishen et al., 1999 colorimetric method, based on the reaction with aluminum chloride. The absorbance of the solution was determined at 510 nm in a Varian® spectrophotometer. Total flavonoid contents were calculated as mg of rutin equivalent (RuE). g−1 using a calibration curve ranging between 200 and 700 ppm (R2 = 0.998).
Analysis of Flavonoids in High Performance Liquid Chromatography
The flavonoids were separated and chromatographically identified by HPLC-DAD Shimadzu (Kyoto, Japan; Atlantis® C-18 column, size 4.6 mm × 250 mm) with quaternary pump (model LC-20AT), a DAD detector (Shimadzu, model SPD-M20A), a degassing unit (DGU-20A5), and a Rheodyne injection valve with a 20 μl loop. The extracts were filtered through a 0.22 μl filter (Millipore). The column was eluted using a linear gradient of acidified water (solvent A) and methanol (solvent B), starting with 80% solvent B up to 10 min, gradually reducing solvent B by 10% over time 15, 30, 45, 60, and 65 min, maintaining only solvent A, between 65 and 70 min, and increasing to 80% solvent B until 90 min (pump flow: 0.800 ml/min). The UV-visible spectra were obtained between 200 and 600 nm, and the chromatograms were processed at 280 and 355 nm. The red propolis flavonoids were tentatively identified based on the following information: elution order, retention time, and UV-visible spectral features compared to those of the standards analyzed under the same conditions and the available literature data. Co-chromatography was carried out with formononetin and daidzein analytical standards.
Evaluation to Deactivate Peroxyl Radicals by Hydrophilic Compounds in a Homogeneous System: Oxygen Radical Absorbance Capacity
The antioxidant activity against the peroxyl radical (ROO•) was measured by ORAC test, which is based on monitoring the effect of the hydrophilic or standard extract (Trolox) in the analysis of fluorescence resulting from the oxidation of fluorescein induced by ROO• radical, generated by decomposition of the 2,2′-azobis (2-methylpropionamidine) dihydrochloride (AAPH) at 37°C (Rodrigues et al., 2012).
The test was performed in 96-well microplates, where 150 μl of fluorescein (61 nm, prepared in 75 mm phosphate buffer pH 7.4) were transferred. Then, 25 μl of the propolis extracts were added in two different dilutions (1:1,000 and 1:10,000) in a phosphate buffer, or white (buffer solution only), or Trolox standard (control). The plate was incubated for 10 min at 37°C with intermittent shaking. After this time, 25 μl of AAPH solution (19 mm, prepared in phosphate buffer) was added to each well to generate radicals and microplate and inserted in the plate reader, where the fluorescence reading occurred at 538 nm after excitation at 485 nm, every minute, for 1 h and 30 min (Murador et al., 2016). The area under the fluorescence vs. time curve for the sample minus the area under the blank curve was compared with the standard Trolox curve. The results were expressed in μmol Trolox Equivalent (TE). g−1.
Antimicrobial Activity Test
The disc diffusion technique was used to assess the propolis extracts’ antimicrobial activity, in which the extracts were applied to filter paper discs, and these, placed on Mueller Hinton agar plates, previously sown with bacterial suspensions. The Gram-positive bacteria St. aureus ATCC 19095 and Gram-negative bacteria Sa. enteritidis ATCC 13076 were used for antimicrobial tests (CLSI/NCCLS, 2003; Winn, 2008). Salmonella enteritidis ATCC 13076 and St. aureus ATCC 19095 were supplied by the Collection of Bacterial Cultures of the Oswaldo Cruz Institute – FIOCRUZ (Manguinhos, Rio de Janeiro, Brazil), where they were grown on nutrient agar and incubated at 37°C for 24 h, then lyophilized and stored in vacuum sealed ampoules at a temperature between −10 and −20°C and transported to our laboratory in a thermic box. Analysis of purity, viability, morphology, and identity was carried out on the used batch.
Preparation of Discs
Inert, sterile Petri dishes were placed inert filter paper discs (DME®), and these were soaked with 10 μl of the optimized extracts in the dilutions 25 mg ml−1, 75 mg ml−1 (in sterile saline), and pure extract. Inert discs were soaked with IL in the same conditions to characterize the negative control. For positive control, Vancomycin (30 μg/disc) and Meropenem (10 μg/disc; DME®) antibiotic filter paper discs were used for Gram-positive and Gram-negative bacteria, respectively.
Disc Diffusion Technique
The bacterial strains were reactivated, sowed in nutrient broth (tryptone soy broth), and incubated in a bacteriological oven at 36 ± 1°C for 24 h. Sample’s inoculum was prepared according to document M2-A8 of the National Committee of Clinical and Laboratory Standardization/Clinical and Laboratory Standards Institute (NCCLS/CLSI) by carrying out a direct suspension, in sterile saline, from the growth in broth. The suspension’s turbidity was adjusted by comparing it with the 0.5 tubes of the McFarland scale, that is, an inoculum containing approximately 1.0 × 108 CFU/ml (CLSI/NCCLS, 2003; Winn, 2008).
The suspension was applied to Mueller Hinton agar Petri dishes, aided by a sterile swab, moving it in eight directions so that the plate was fully sown. After about 15 min, the disks impregnated with extracts were applied with the aid of flamed forceps. Then, Petri dishes were inverted and incubated in an oven at 36 ± 1°C for a period of between 16 and 18 h (CLSI/NCCLS, 2003; Winn, 2008).
After the incubation period, the antimicrobial action was read and assessed by the formation of a growth inhibition halo, which was measured using a millimeter rule (CLSI/NCCLS, 2003).
Statistical Analysis
The tests were conducted in triplicate, and data expressed as mean ± standard deviation (SD). The results were submitted to analysis of variance (ANOVA), the comparison between averages established by Tukey’s HSD post hoc test, adopting the 95% level of significance. All analyses were performed with StatSoft software STATISTICS® version 13.3 (Tulsa, United States).
Results and Discussion
Efficiency in the Extraction of Flavonoids: Preliminary Tests
The 16 results obtained from the preliminary tests are presented in Table 1 as well as conventional extraction and screening with IL and ES. Most of the tested solvents showed equivalent or superior efficacy, in terms of flavonoid concentration, compared to hydroalcoholic extracts used to relate the alternative solvents’ extraction power with the conventional method. The extract containing [C6mim]Cl/water in a 10:1 (g/g) ratio demonstrated twice the extractive potential of 95% ethanol. In contrast, ES CH-BUT and CH-LEV and IL [C4mim]Cl with water in the proportion 10:3 (g/g) were those with the lowest efficiency in extract flavonoids from red propolis; however, changing the ratio to 1:1 (g/g) of IL/water, the extraction efficiency doubled, equivalent to the yield obtained with 70% ethanol. In the literature, no studies of propolis extracts with IL were found, but comparing [C4mim]Cl extractor potential of flavonoids from heather, it showed superior performance compared to the 60% ethanol extract (Drozdz and Pyrzynska, 2019). The anion of IL acts in disrupting the structure of the natural products’ matrix. At the same time, the imidazolium cation plays an essential role due to the aromatic π-cloud, which strongly interacts with polar and aromatic analytes (Ventura et al., 2017).
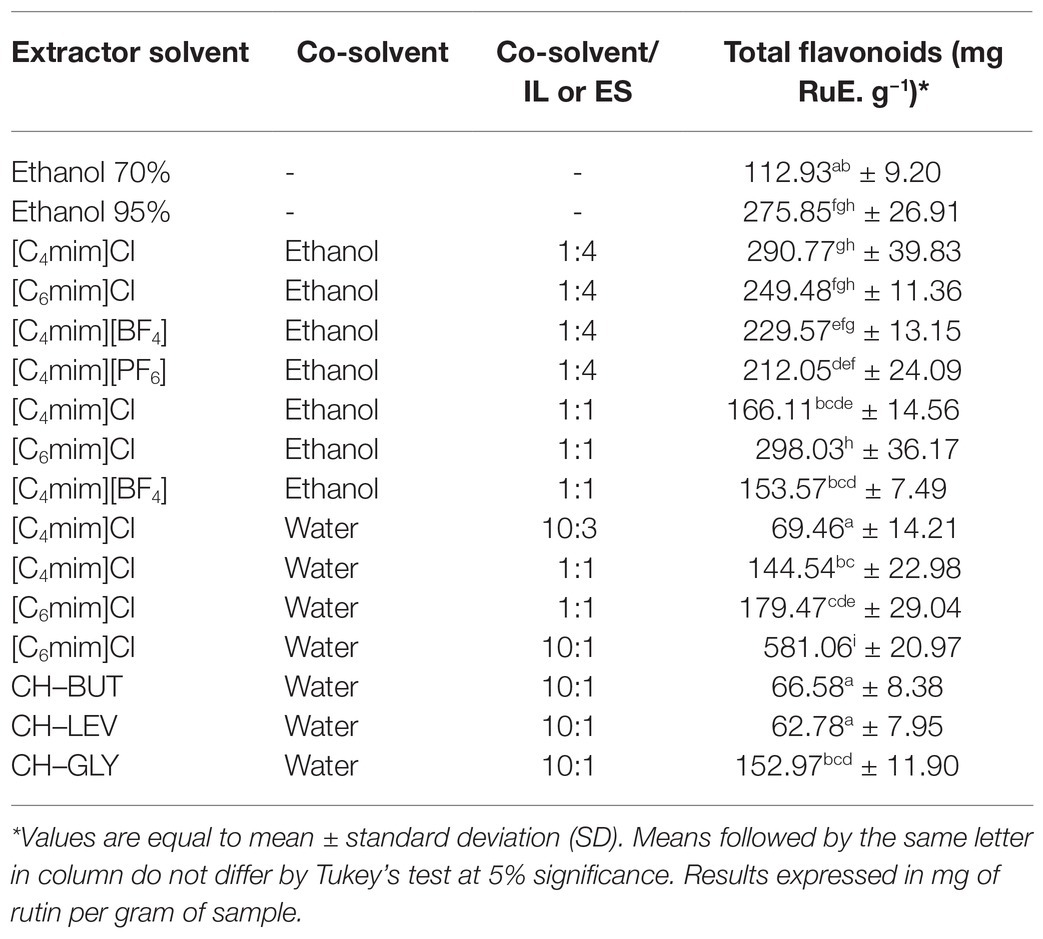
Table 1. Determination of total flavonoids in red propolis extracts made with different ionic liquids and eutectic solvents, compared with the conventional solvent.
Generally, extracts presented different characteristics, according to the solvent and co-solvent used, especially regarding color. The yield of flavonoids of most extracts with the solvents tested in this study showed higher values than conventional extracts found in the literature (Santana Andrade et al., 2017; Devequi-Nunes et al., 2018). The substitution of VOS by alternative solvents (non-volatile solvents) is a promising strategy increasingly addressed. While these alternatives can positively contribute to the environment, there may also be an improvement in terms of the yield of bioactive compounds. Some studies indicate the effectiveness of the use of some ES and IL in the extraction of flavonoids from several natural products, considering conventional solvents. However, no reports compare these solvents in samples of Brazilian red propolis, which have stood out for their biological activities (Koutsoukos et al., 2019; Trusheva et al., 2019).
From the preliminary tests, variables were selected for the experimental design (CCDR 23), analytical viability was considered, associated with the efficiency to extract flavonoids. The IL [C4mim][PF6] possesses some properties, such as low water solubility, which can cause some difficulties to dissolve with the tested co-solvents. On the other hand, [C4mim][BF4] is more soluble with the co-solvent; however, this IL’s extraction produced a heterogeneous extract.
Notably, propolis presents a natural resin with a complex composition. Thus, there is an inherent difficulty in standardizing the method for extract preparation with alternative solvents, which also have a high viscosity. In this study, all operational conditions were standardized and further optimized. However, some extracts presented different behaviors, forming multiphase systems, in addition to the formation of an emulsion, making them unfeasible for analysis, such as the case with extracts composed of [C4mim][PF6] and [C4mim][BF4], using water as a co-solvent. Those differences highlight the importance of evaluating different solvents and conditions to find the best manner to extract bioactive compounds. Those distinct characteristics may have occurred for fluoridated IL are unstable compounds in water since they hydrolyze, forming by-products that can produce specific toxicity (Plechkova and Seddon, 2008; Freire et al., 2010). For this reason, IL [C4mim][PF6] and [C4mim][BF4] were discarded from the screening of solvents. The extract with [C6mim]Cl in its largest proportion obtained an increase in flavonoids, about three times concerning the ratio 1:1 (with water).
Among the ES tested, extraction with choline chloride and glycerol did not significantly different compared to the extract with 70% ethanol in flavonoid concentrations. Since alcohol is used as the most common extractor among propolis extracts by several authors (Alencar et al., 2007; da Silva Frozza et al., 2013; Santana Andrade et al., 2017), equivalence in the extractive power of ES is crucial to obtain the exact yield using an alternative solvent. Trusheva et al. (2019) tested the extract with choline chloride and glycerol, and it showed 8.4% of total flavones and flavonols compared to 70% ethanol (11.3%) in propolis samples of poplar type. Funari et al. (2019) tested the same ES, resulting in a lower performance of phenolic compounds than 70% ethanol in green propolis samples. Some authors highlight the influence of water on the ES’s composition, showing that this may be the main factor that induces selectivity in extraction (Liu et al., 2018). The presence of water decreases the viscosity of ES, while it increases their polarity, interfering in the extractive power with low polarity (Funari et al., 2019). However, eutectic mixtures containing more than 30% water must be avoided, as this percentage results in the loss of existing hydrogen bonds, resulting in the de-characterization of the ES structure, which negatively influences its interaction with the flavonoid (Meng et al., 2018). Propolis is mainly composed of flavonoid aglycones and their derivatives, which are predominantly less polar than those glycosylated ones (Zhang et al., 2012; Huang et al., 2014). Thus, a higher percentage of water in ES would reduce the extractive potential of these compounds. On the other hand, ES presents high viscosity, reducing extraction efficiency by slow mass transfer (Fraige et al., 2019). This explains the fact that ES is not effective in extracting propolis flavonoids.
A global evaluation was executed to optimize the extraction methods using alternative solvents and, although the IL [C4mim]Cl has formed a homogeneous extract, with a high capacity to extract flavonoids, [C6mim]Cl performed better with water as a co-solvent, being this solvent chosen for the stage of extraction optimization, through the experimental design using response surface methodology (RSM).
Optimization of Ionic Liquid Extraction
Central Composition Design Rotational
As mentioned before, [C6mim]Cl showed better extraction yield in total flavonoids based on the preliminary test, and it was selected for the experimental design using a CCDR 23 (Table 2). Two responses were considered as results from the assays performed using the conditions specified by the experimental design, flavonoid yield, and antioxidant activity. All results are presented in Table 2.
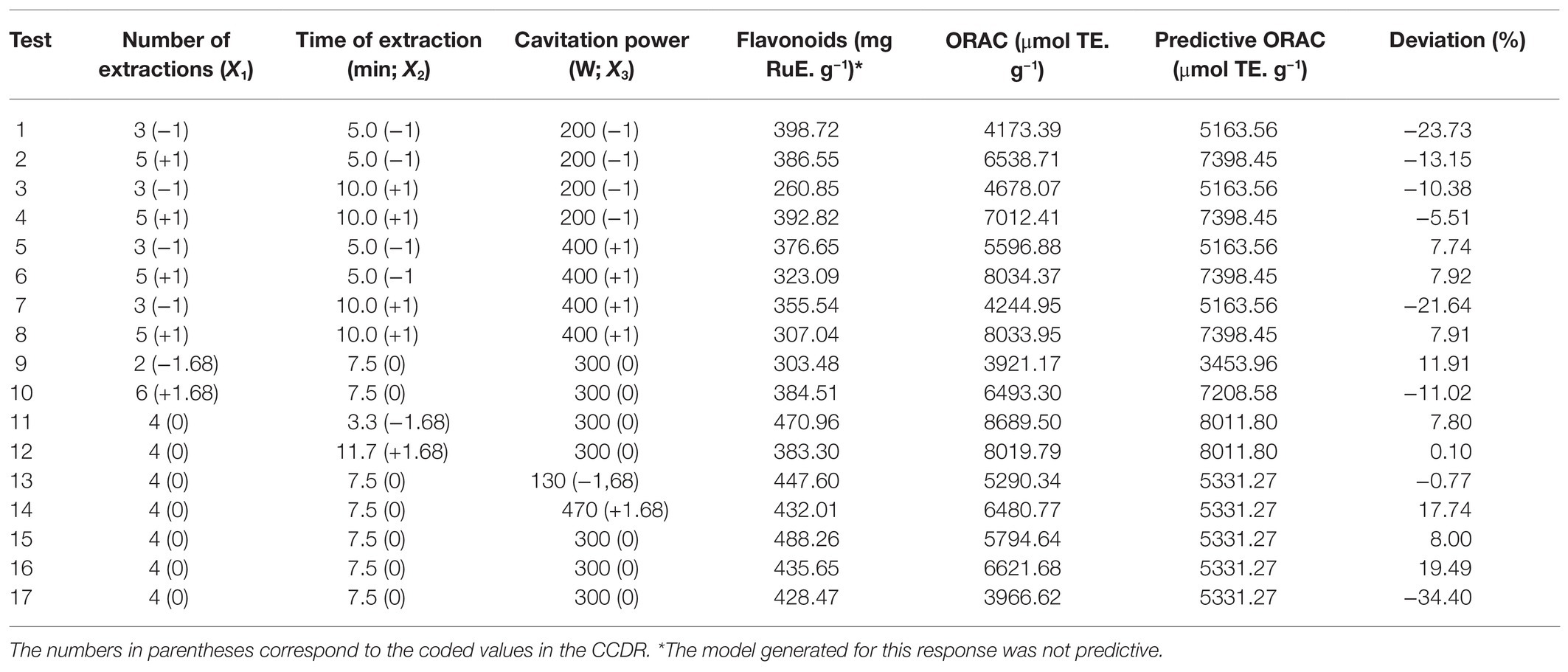
Table 2. Design of experiment 23 using 1-butyl-3-methylimidazolium chloride [C6mim][Cl] and 10% water as a co-solvent.
The flavonoid yield values obtained ranged between 260.85 and 470.96 mg RuE. g−1 of the sample. For the CCDR, three variables were considered: the number of extractions (X1), time of extraction (min; X2), and cavitation power (W; X3); however, after data evaluation using 95% of confidence, the effect of variables assessed was not statistically significant on the evaluated response. Also, no significant interaction between the variables used (R2 < 0.7) was observed. Therefore, data could not be adjusted, and it was not possible to generate a predictive model as well as an equation representing the conditions evaluated. It is difficult to indicate a reason for the lack of adjusting among the experimental data and the predictive values. Often this occurs due to several factors acting together.
Regarding the antioxidant activity against peroxyl radicals, the CCDR assays provided values ranging between 3921.17 and 8689.50 μmol TE. g−1 of extract, and two variables were considered to obtain Equation 1: number of extractions (X1) and time of extraction (X2). The equation is constructed considering only terms representing the variables with significative effects over the response (antioxidant activity). Thus, not statistically significant terms were incorporated into the lack of fit to calculate the R2 and F ratio.
The conditions provided the best antioxidant activity (8689.50 μmol TE. g−1 of the sample) from experiment 11 (Table 2), whose conditions were: four extractions with the same biomass, time of extraction at 3.3 min, and 300 W of cavitation power. The dependent (antioxidant activity) and independent variables were fitted to a second-order model, and they were examined in terms of goodness of fit. Therefore, ANOVA was used to evaluate the adequacy of the fitted model.
In this study, R2 was 0.70, and the estimated F value was approximately five times the F tabulated for antioxidant activity, showing that the proposed model was predictive, with a 95% confidence level. The obtained model for antioxidant activity was used to construct the response surface presented in Figure 1 to understand the interactions between the number of extractions (X1) and time of extraction (X2) required to maximize the antioxidant activity provided by the red propolis extracts.
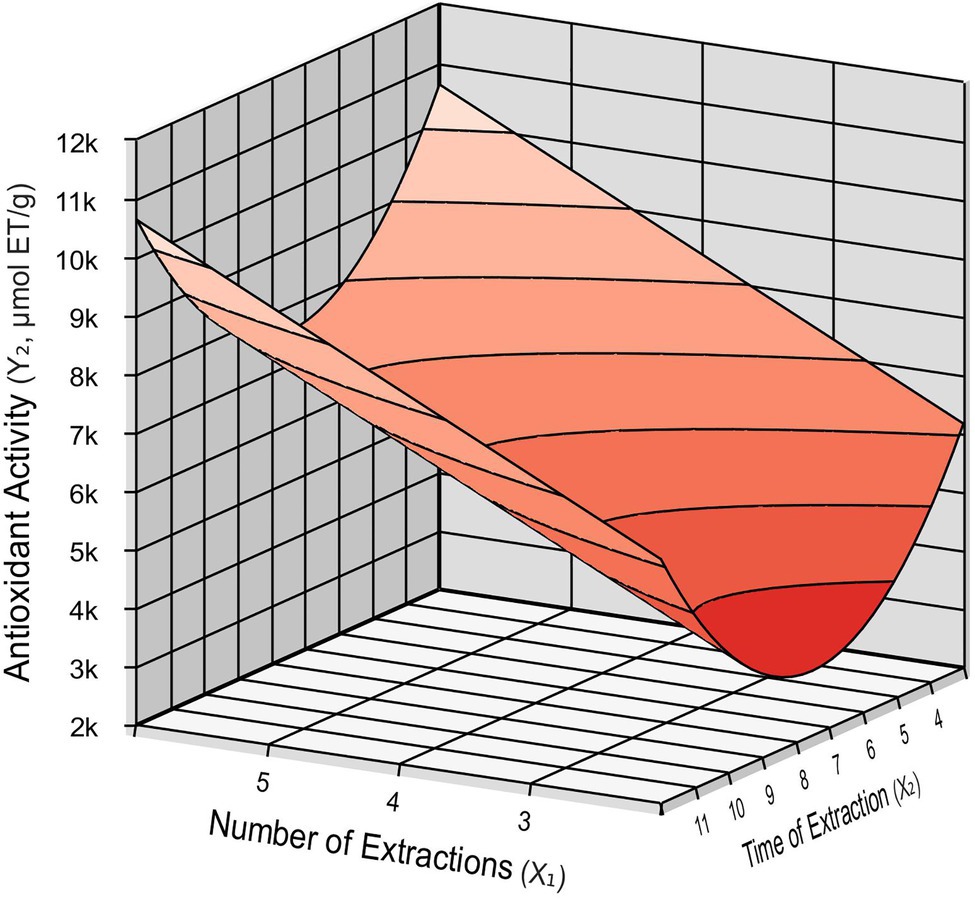
Figure 1. Response surface for antioxidant activity as a function of the number of extractions (X1) and time of extraction (X2).
Analyzing the response surface and the contour diagram presented in Figure 1, two work regions provide the optimal conditions for antioxidant activity, evaluating the number of extractions and time of extractions. Both areas are comprising a higher number of extractions. The minimum and maximum levels can be considered for variable extraction time since both presented high antioxidant activity values in propolis extracts. The results show that [C6mim]Cl has a strong extraction potential, intensified with each repetition of the process, even at the lowest extraction time tested, exhibiting affinity with the bioactive compounds contained in propolis, which represents an advantage in the extraction process, confirmed by the better performance in antioxidant activity assay.
Despite no model being obtained for flavonoids concentrations, the highest value obtained, considering the assays based on the CCDR, for the flavonoid yield was provided by the same conditions presented in the predictive model for antioxidant activity, showing a possible relationship between the flavonoids contained in red propolis in their antioxidant action.
Experimental Validation
Based on the response surface analysis (Figure 1), we determined the extraction condition in which higher antioxidant activity is obtained. Such conditions are shown in Table 3, which indicates separately the values in which variables X1, X2, and X3 provided the best values for antioxidant activity from the red propolis extracts.
The validation was carried out employing an experimental test under these conditions, presenting, by determining the extract antioxidant activity, 7595.77 μmol TE. g−1, a deviation of 5.48% concerning the result predicted by the experimental design. The results obtained confirm that the obtained model is predictive and reproducible for antioxidant activity, considering the assessed conditions in this work. Results presented related to antioxidant activity were superior to those obtained by some authors, such as Santana Andrade et al. (2017, 6,665 μmol TE. g−1). This optimized extract showed 394.39 ± 36.30 mg RuE. g−1 regarding total flavonoids, highlighting the close relationship between antioxidant activity and flavonoid concentration in the propolis extract, which has high levels of these polyphenols.
In the commercial context, the process of obtaining alcohol-free propolis extract is notably relevant. In countries, where most of the population is Muslim, less consumption of alcohol occurs, since it is forbidden to drink alcoholic beverages in the Islamic religion, requiring control in the production and import of alcohol in these countries (Al-ansari et al., 2015, 2019; Amin-Esmaeili et al., 2018). In addition to this group, abstainers and children are others who would be restricted from consuming alcoholic extracts. Although today various options for aqueous propolis extracts exist and studies related to them, hydroalcoholic extracts, in many cases, perform better in terms of biological activities (Hazem et al., 2017; Sayyadi et al., 2020). The proposal presented in this work represents an advantage concerning other processes in the yield of bioactive compounds.
Red Propolis Extract Flavonoid Composition
The optimized and validated extracts were analyzed by high performance liquid chromatography (HPLC; Figure 2) and chromatogram, as shown in Figure 3. The characteristics of the flavonoids separated in red propolis extract are shown in Table 4. Medicarpin (peak 3) and isoliquiritigenin (peak 5) were the major constituents in an extract with [C6mim]Cl, representing 24 and 18% of total flavonoids content, respectively, followed by hesperetin derivative (peak 4) and formononetin (peak 6), which contributed 17 and 12%, respectively. The extraction processes using hydrophobic IL [C6mim]Cl proved to be selective for more hydrophobic flavonoids (medicarpin). Thus, the higher hydrophobicity of the IL implies the extraction of the most hydrophobic components of the biomass because of the π–π, n–π, and hydrophobic bonds (de Souza Mesquita et al., 2019; Murador et al., 2019b).
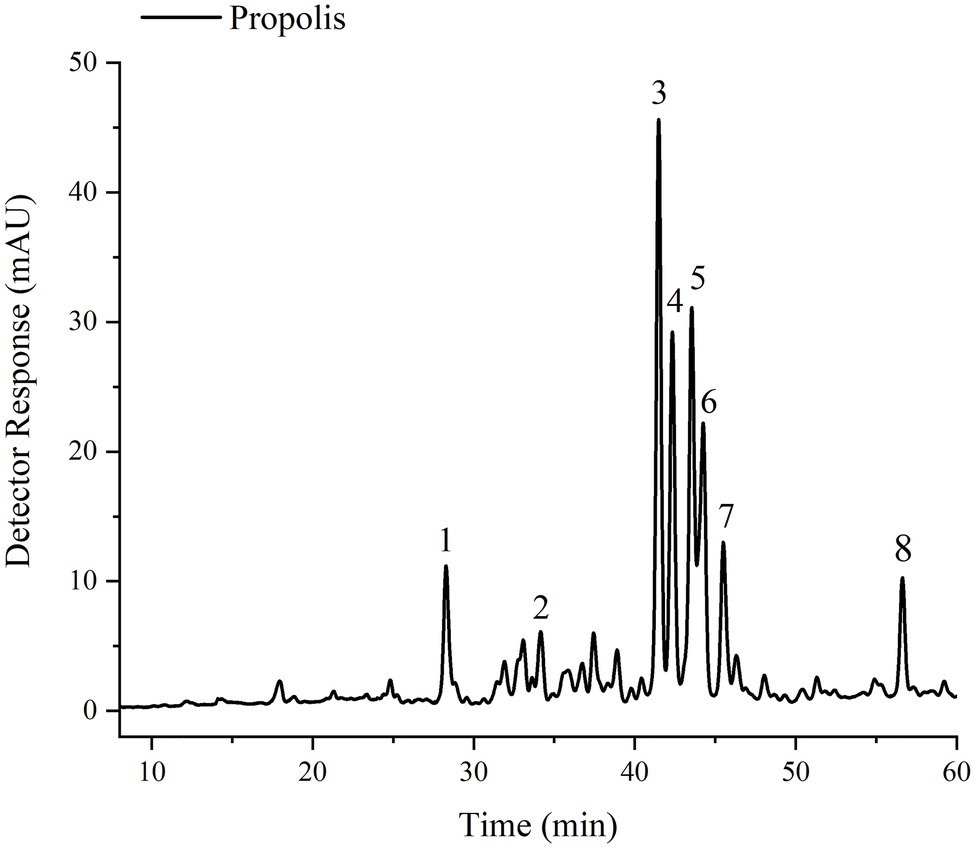
Figure 3. Typical chromatogram of red propolis flavonoids extract obtained from [C6mim]Cl. Chromatograms were processed at 280 nm.
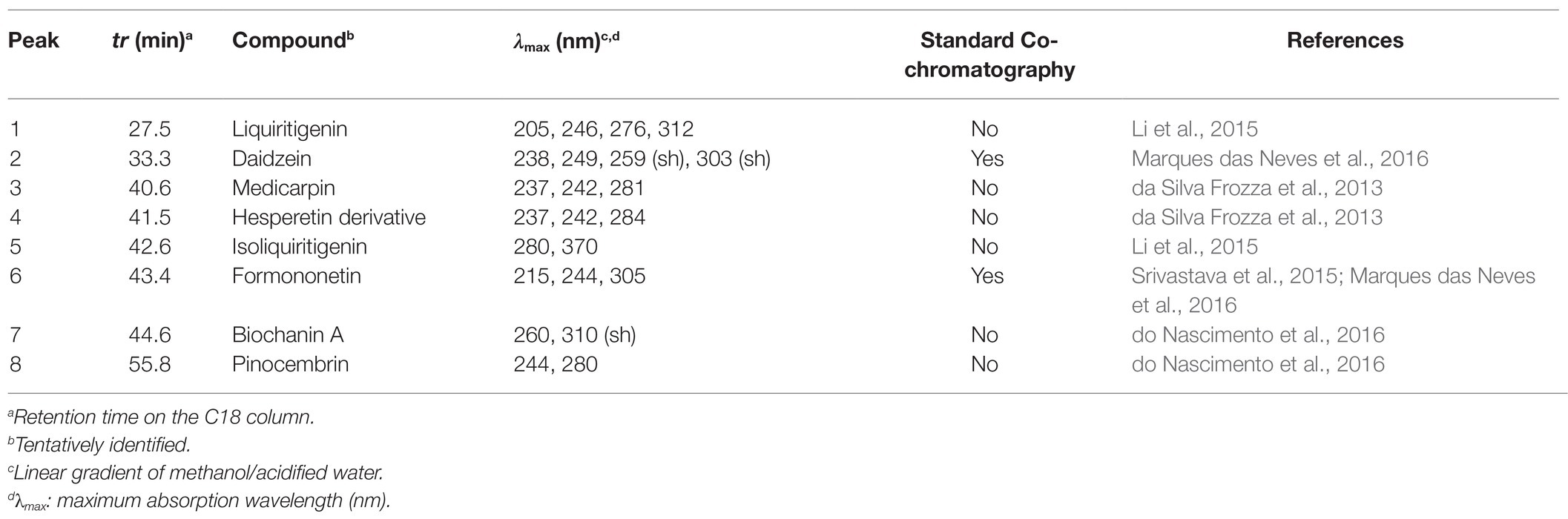
Table 4. Chromatographic and UV-visible (UV-vis) characteristics of the flavonoids identified in the red propolis extract obtained from [C6mim]Cl.
Red propolis can be found in tropical countries like Brazil, Cuba, Venezuela, and Mexico (Alencar et al., 2007; Cuesta-Rubio et al., 2007; Lotti et al., 2010; López et al., 2014). In research on the phytochemical markers of red propolis, daidzein was not found in all samples from Alagoas, the same geographical origin as the sample in this study. At the same time, formononetin and biochanin A were found in all studied samples (López et al., 2014).
This result demonstrates the variety in propolis chemical composition, although some authors have found these compounds in samples of red propolis. It is known that propolis chemical composition varies according to original geographic region, and propolis phytochemical profile can vary due to several factors such as local flora, season, and extraction methods, resulting in different extracts (Alencar et al., 2007; Cavalcanti de Pontes et al., 2018; do Nascimento et al., 2019). da Silva Frozza et al. (2017) identified some compounds of biological interest, such as isoflavones formononetin, and biochanin A besides isoliquiritigenin in samples of red propolis from Alagoas. These compounds were found in this study, but others flavonoids demonstrating once again the variability of this type of extract (Bankova, 2005; Escriche and Juan-Borrás, 2018; do Nascimento et al., 2019).
Antimicrobial Activity Test
The extract containing IL presented antimicrobial activity against the two bacteria tested, as described in Table 5. The optimized extract pure with IL (394.39 mg RuE. g−1) and the negative control with IL [C6mim]Cl presented no significant difference in the antimicrobial action against both tested bacteria, indicating that this solvent contributed to the antimicrobial effect. Some review articles report the antimicrobial activity of imidazolium-based IL (Reddy and Nancharaiah, 2020; Sivapragasam et al., 2020). The mechanism of IL antimicrobial activity is not known. Still, there is evidence that the long alkyl chain linear of the cation positively influences this activity, which may disrupt membrane proteins (Docherty and Kulpa., 2005; Duman et al., 2019; Florio et al., 2019).
The optimized process is presented in Figure 2. The disc with the extract in the concentration of 75 mg ml−1, which contains 23.8 μg RuE/disc, showed antimicrobial action against Sa. enteritidis. The extract in the concentration of 25 mg ml−1 (7,9 μg RuE/disc) presented no antimicrobial activity for these bacteria. There were no comparative studies in the literature regarding the effect of the red propolis against this bacterium. Still, it is known that Gram-negative bacteria tend to better resist these compounds due to the intense lipid layer, making the cell wall impermeable for several macromolecules (Efenberger-Szmechtyk et al., 2021). Despite this, with approximately twice the extract concerning the amount of antibiotics, it was possible to verify antimicrobial activity against this Gram-negative bacterium.
The antimicrobial action against St. aureus from the extract with IL (394 mg RuE. g−1) was statistically higher concerning the positive control for this bacterium. The extracts in all dilutions showed antimicrobial activity against this Gram-positive bacterium, including negative control. Despite this, the extract diluted to a concentration of 75 mg. 10−1 showed more significant inhibition compared to IL in the same conditions.
The antimicrobial action of the red propolis extract in Gram-positive bacteria has already been evidenced by some authors, who have also found promising results on the activity against the bacterium St. aureus (Souza Machado et al., 2016; Dantas Silva et al., 2017; Regueira-Neto et al., 2018; Silva et al., 2019).
The excellent performance of the red propolis extract with [C6mim]Cl can be attributed to the increase in phytochemicals achieved by the extraction. At the same time, regarding negative control, the IL, as it is non-volatile, remained impregnated in the disc. Thus, tests involving other microbiological techniques are necessary to compare with the results obtained. Besides, methods for separating the IL from the extract – which is one of the advantages of using IL, as it can be recycled and reused – must be carried out to enhance the extract viability and to minimize possible toxic effects originated at the IL (do Nascimento et al., 2019).
The determination of toxicity and antimicrobial activity is crucial to release an IL to the industry. Although these compounds are considered sustainable due to their possibility of being recycled and reused, non-flammability and non-volatility are characteristics that associate them with “green” chemistry. Some articles have shown some IL based on imidazolium that are associated with toxicity. Therefore, it was impossible to consider IL as genuinely green compounds (Plechkova and Seddon, 2008). Cationic elements of IL play an essential role in microorganisms, depending on the length of the alkyl chain, contributing to toxicity (Fister et al., 2017) with significant antimicrobial activity against Gram-positive and Gram-negative bacteria (Docherty and Kulpa., 2005; Patil et al., 2020; Sivapragasam et al., 2020). This may explain the action of the IL against the tested bacteria. Thus, it is necessary to understand the behavior of both IL and red propolis individually to verify if, in fact, the sample showed antimicrobial activity or if the effect shown was overestimated by the action of the IL on bacteria.
On the other hand, the antimicrobial activity of [C6mim]Cl can be an advantage to enable the use of the extract in several applications, such as food packaging incorporated into biodegradable films (de Souza Mesquita et al., 2020a,b). The optimized extracts supported the antimicrobial activity, inhibiting the growth of both St. aureus and Sa. enteritidis, the latter being a Gram-negative bacterium, a type known to obtain a more complex cell wall, rich in lipopolysaccharides. An interesting point was the antimicrobial action of pure [C6mim]Cl, suggesting that this solvent interacts with the cellular components of the bacterial wall, generating the need to know these mechanisms, in addition to also evaluating the toxicity in human cells.
Conclusion
Propolis is known for thousands of years for its diverse biological actions. However, its use is limited in certain groups. The study of alternative solvents, moreover, to be an appealing commercial strategy from an economic point of view, enables expanded research on propolis chemical composition. [C6mim]Cl was able to extract total flavonoid contents better than ethanol in the two concentrations tested in the screening tests. Furthermore, the optimization of the process determined the best conditions for preparing highly antioxidant extracts. Thus, it was possible to obtain a high-performance extract regarding flavonoids, with antioxidant and antimicrobial activity, as to expose in Figure 3, we developed a complete and optimized process designed for high recovery of phytochemicals from red propolis, compared to the methods previously developed in the literature mediated by VOS or ES.
In summary, we developed an ultrasound-assisted extraction under the operational conditions optimized at three cycles of extractions with the same biomass, up to 3.3 min at 300 W of cavitation, which was possible to recover 470.96 mg of phytochemicals with high antioxidant activity (7595.77 μmol TE. g−1). Besides, the biomass residues can be used for other applications, applying the biorefinery concepts, which are extremely important in a sustainable society. In the end, the extracts were evaluated by microbiological assays, showing that the use of IL displays positive effects against Sa. enteritidis and St. aureus.
Nevertheless, some steps are still necessary in order to promote the characterization of this complex biomass and spur future investigations to minimize the effects inherent to the process, including the recycling and reuse of the [C6mim]Cl, to make the method more sustainable. Besides, we suggest that future studies focus on understanding IL’s interaction with the phytochemicals extracted from red propolis as a way to use it more effectively, better using these benefits from its rich composition in phytochemicals, also assuring the sustainable use of the raw material.
Data Availability Statement
The raw data supporting the conclusions of this article will be made available by the authors, without undue reservation.
Author Contributions
CS contributed to the execution and analysis of all experiments. VR contributed to the planning of experiments. AB contributed to the analysis and interpretation of the data included. All authors wrote and reviewed the results and approved the final version of the manuscript.
Conflict of Interest
The authors declare that the research was conducted in the absence of any commercial or financial relationships that could be construed as a potential conflict of interest.
Acknowledgments
The authors are grateful to Fernando Barbalho from the apiary localized in Porto das Pedras, Alagoas, Brazil, for donating the sample of Brazilian propolis and Professor Severino Matias de Alencar, da ESALQ/USP, for donating the analytical standards.
References
Al-Ansari, B., Thow, A.-M., Day, C. A., and Conigrave, K. M. (2015). Extent of alcohol prohibition in civil policy in Muslim majority countries: the impact of globalization. Addiction 111, 1703–1713. doi: 10.1111/add.13159
Al-Ansari, B., Thow, A.-M., Mirzaie, M., Day, C. A., and Conigrave, K. M. (2019). Alcohol policy in Iran: policy content analysis. Int. J. Drug Policy 73, 185–198. doi: 10.1016/j.drugpo.2019.07.032
Alencar, S. M., Oldoni, T. L. C., Castro, M. L., Cabral, I. S. R., Costa-Neto, C. M., Cury, J. A., et al. (2007). Chemical composition and biological activity of a new type of Brazilian propolis: red propolis. J. Ethnopharmacol. 113, 278–283. doi: 10.1016/j.jep.2007.06.005
Amin-Esmaeili, M., Motevalian, A., Hajebi, A., Sharifi, V., Stockwell, T., and Rahimi-Movaghar, A. (2018). Methods for calculation of per capita alcohol consumption in a Muslim majority country with a very low drinking level: findings from the 2011 Iranian mental health survey. Drug Alcohol Rev. 37, 874–878. doi: 10.1111/dar.12847
Bankova, V. (2005). Chemical diversity of propolis and the problem of standardization. J. Ethnopharmacol. 100, 114–117. doi: 10.1016/j.jep.2005.05.004
Banzato, T. P., Gubiani, J. R., Bernardi, D. I., Nogueira, C. R., Monteiro, A. F., Juliano, F. F., et al. (2020). Antiproliferative Flavanoid dimers isolated from Brazilian red Propolis. J. Nat. Prod. 83, 1784–1793. doi: 10.1021/acs.jnatprod.9b01136
Berretta, A. A., Silveira, M. A. D., Cóndor Capcha, J. M., and de Jong, D. (2020). Propolis and its potential against SARS-CoV-2 infection mechanisms and COVID-19 disease. Biomed. Pharmacother. 131:110622. doi: 10.1016/j.biopha.2020.110622
Cavalcanti de Pontes, M. L., Vasconcelos, I. R. A., de Melo Diniz, M. D. F. F., and Freire Pessôa, H. D. L. (2018). Chemical characterization and pharmacological action of Brazilian red propolis. Acta Brasiliensis 2:34. doi: 10.22571/2526-433868
Choi, Y. H., and Verpoorte, R. (2019). Green solvents for the extraction of bioactive compounds from natural products using ionic liquids and deep eutectic solvents. Curr. Opin. Food Sci. 26, 87–93. doi: 10.1016/j.cofs.2019.04.003
CLSI/NCCLS (2003). Performance Standards for Antimicrobial Disk Susceptibility Tests; Approved Standard – Eighth Edition. 2003; Wayne, Pennsylvania (USA: Clinical and Laboratory Standards Institute).
Cuesta-Rubio, O., Piccinelli, A. L., Campo Fernandez, M., Márquez Hernández, I., Rosado, A., and Rastrelli, L. (2007). Chemical characterization of Cuban Propolis by HPLC−PDA, HPLC−MS, and NMR: the brown, red, and yellow Cuban varieties of propolis. J. Agric. Food Chem. 55, 7502–7509. doi: 10.1021/jf071296w
Dantas Silva, R. P., Machado, B. A. S., Barreto, G. da A., Costa, S. S., Andrade, L. N., Amaral, R. G., et al. (2017). Antioxidant, antimicrobial, antiparasitic, and cytotoxic properties of various Brazilian propolis extracts. PLoS One 12:e0172585. doi: 10.1371/journal.pone.0172585
da Silva Frozza, C. O., Garcia, C. S. C., Gambato, G., de Souza, M. D. O., Salvador, M., Moura, S., et al. (2013). Chemical characterization, antioxidant and cytotoxic activities of Brazilian red propolis. Food Chem. Toxicol. 52, 137–142. doi: 10.1016/j.fct.2012.11.013
da Silva Frozza, C. O., Santos, D. A., Rufatto, L. C., Minetto, L., Scariot, F. J., Echeverrigaray, S., et al. (2017). Antitumor activity of Brazilian red propolis fractions against Hep-2 cancer cell line. Biomed. Pharmacother. 91, 951–963. doi: 10.1016/j.biopha.2017.05.027
de Souza Mesquita, L. M., Martins, M., Maricato, É., Nunes, C., Quinteiro, P. S. G. N., Dias, A. C. R. V., et al. (2020a). Ionic liquid-mediated recovery of carotenoids from the Bactris gasipaes fruit waste and their application in food-packaging chitosan films. ACS Sustain. Chem. Eng. 8, 4085–4095. doi: 10.1021/acssuschemeng.9b06424
de Souza Mesquita, L. M., Neves, B. V., Pisani, L. P., and de Rosso, V. V. (2020b). Mayonnaise as a model food for improving the bioaccessibility of carotenoids from Bactris gasipaes fruits. LWT 122:109022. doi: 10.1016/j.lwt.2020.109022
de Souza Mesquita, L. M., Ventura, S. P. M., Braga, A. R. C., Pisani, L. P., Dias, A. C. R. V., and de Rosso, V. V. (2019). Ionic liquid-high performance extractive approach to recover carotenoids from Bactris gasipaes fruits. Green Chem. 21, 2380–2391. doi: 10.1039/C8GC03283A
Devequi-Nunes, D., Machado, B. A. S., Barreto, G. da A., Rebouças Silva, J., da Silva, D. F., da Rocha, J. L. C., et al. (2018). Chemical characterization and biological activity of six different extracts of propolis through conventional methods and supercritical extraction. PLoS One 13:e0207676. doi: 10.1371/journal.pone.0207676
Docherty, K. M., and Kulpa, C. F. Jr. (2005). Toxicity and antimicrobial activity of imidazolium and pyridinium ionic liquids. Green Chem. 7:185. doi: 10.1039/b419172b
do Nascimento, T. G., da Silva, P. F., Azevedo, L. F., da Rocha, L. G., de Moraes Porto, I. C. C., Lima e Moura, T. F. A., et al. (2016). Polymeric nanoparticles of Brazilian red propolis extract: preparation, characterization, antioxidant and leishmanicidal activity. Nanoscale Res. Lett. 11:301. doi: 10.1186/s11671-016-1517-3
do Nascimento, T. G., dos Santos Arruda, R. E., da Cruz Almeida, E. T., dos Santos Oliveira, J. M., Basílio-Júnior, I. D., Celerino da Moraes Porto, I. C., et al. (2019). Comprehensive multivariate correlations between climatic effect, metabolite-profile, antioxidant capacity and antibacterial activity of Brazilian red propolis metabolites during seasonal study. Sci. Rep. 9:18293. doi: 10.1038/s41598-019-54591-3
Dróżdż, P., and Pyrzynska, K. (2019). Screening of ionic liquids for extraction of flavonoids from heather. Nat. Prod. Res. 33, 148–151. doi: 10.1080/14786419.2018.1437441
Duman, A. N., Ozturk, I., Tunçel, A., Ocakoglu, K., Colak, S. G., Hoşgör-Limoncu, M., et al. (2019). Synthesis of new water-soluble ionic liquids and their antibacterial profile against Gram-positive and Gram-negative bacteria. Heliyon 5:e02607. doi: 10.1016/j.heliyon.2019.e02607
Efenberger-Szmechtyk, M., Nowak, A., and Czyzowska, A. (2021). Plant extracts rich in polyphenols: antibacterial agents and natural preservatives for meat and meat products. Crit. Rev. Food Sci. Nutr. 61, 149–178. doi: 10.1080/10408398.2020.1722060
Escriche, I., and Juan-Borrás, M. (2018). Standardizing the analysis of phenolic profile in propolis. Food Res. Int. 106, 834–841. doi: 10.1016/j.foodres.2018.01.055
Fister, S., Mester, P., Sommer, J., Witte, A. K., Kalb, R., Wagner, M., et al. (2017). Virucidal influence of ionic liquids on phages P100 and MS2. Front. Microbiol. 8:1608. doi: 10.3389/fmicb.2017.01608
Florio, W., Becherini, S., D’Andrea, F., Lupetti, A., Chiappe, C., and Guazzelli, L. (2019). Comparative evaluation of antimicrobial activity of different types of ionic liquids. Mater. Sci. Eng. C 104:109907. doi: 10.1016/j.msec.2019.109907
Fraige, K., Arrua, R. D., Sutton, A. T., Funari, C. S., Cavalheiro, A. J., Hilder, E. F., et al. (2019). Using natural deep eutectic solvents for the extraction of metabolites in Byrsonima intermedia leaves. J. Sep. Sci. 42, 591–597. doi: 10.1002/jssc.201800905
Freire, M. G., Neves, C. M. S. S., Marrucho, I. M., Coutinho, J. A. P., and Fernandes, A. M. (2010). Hydrolysis of tetrafluoroborate and hexafluorophosphate counter ions in imidazolium-based ionic liquids. Chem. Eur. J. 114, 3744–3749. doi: 10.1021/jp903292n
Funari, C. S., Sutton, A. T., Carneiro, R. L., Fraige, K., Cavalheiro, A. J., da Silva Bolzani, V., et al. (2019). Natural deep eutectic solvents and aqueous solutions as an alternative extraction media for propolis. Food Res. Int. 125:108559. doi: 10.1016/j.foodres.2019.108559
Hazem, A., Pitica-Aldea, I. M., Popescu, C., Matei, L., Dragu, D., Economescu, M., et al. (2017). The antiviral/virucidal effects of alchoolic and aqueous extracts with propolis. Farmacia 65, 868–876.
Huang, S., Zhang, C.-P., Wang, K., Li, G., and Hu, F.-L. (2014). Recent advances in the chemical composition of propolis. Molecules 19, 19610–19632. doi: 10.3390/molecules191219610
Kitamura, H., Saito, N., Fujimoto, J., Nakashima, K., and Fujikura, D. (2018). Brazilian propolis ethanol extract and its component kaempferol induce myeloid-derived suppressor cells from macrophages of mice in vivo and in vitro. BMC Complement. Altern. Med. 18:138. doi: 10.1186/s12906-018-2198-5
Koutsoukos, S., Tsiaka, T., Tzani, A., Zoumpoulakis, P., and Detsi, A. (2019). Choline chloride and tartaric acid, a natural deep eutectic solvent for the efficient extraction of phenolic and carotenoid compounds. J. Clean. Prod. 241:118384. doi: 10.1016/j.jclepro.2019.118384
Kwon, M. J., Shin, H. M., Perumalsamy, H., Wang, X., and Ahn, Y.-J. (2020). Antiviral effects and possible mechanisms of action of constituents from Brazilian propolis and related compounds. J. Apic. Res. 59, 413–425. doi: 10.1080/00218839.2019.1695715
Li, B., Liu, B., Li, J., Xiao, H., Wang, J., and Liang, G. (2015). Experimental and theoretical investigations on the supermolecular structure of Isoliquiritigenin and 6-O-α-D-Maltosyl-β-cyclodextrin inclusion complex. Int. J. Mol. Sci. 16, 17999–18017. doi: 10.3390/ijms160817999
Lima, L. D., Andrade, S. P., Campos, P. P., Barcelos, L. S., Soriani, F. M., and Moura, S., et al., (2014). Brazilian green propolis modulates inflammation, angiogenesis and fibrogenesis in intraperitoneal implant in mice. BMC Complement. Altern. Med. 14:177. doi: 10.1186/1472-6882-14-177
Liu, X., Ahlgren, S., Korthout, H. A. A. J., Salomé-Abarca, L. F., Bayona, L. M., Verpoorte, R., et al. (2018). Broad range chemical profiling of natural deep eutectic solvent extracts using a high performance thin layer chromatography–based method. J. Chromatogr. A 1532, 198–207. doi: 10.1016/j.chroma.2017.12.009
Liu, Y., Zhang, H., Yu, H., Guo, S., and Chen, D. (2019). Deep eutectic solvent as a green solvent for enhanced extraction of narirutin, naringin, hesperidin and neohesperidin from Aurantii Fructus. Phytochem. Anal. 30, 156–163. doi: 10.1002/pca.2801
López, B. G.-C., Schmidt, E. M., Eberlin, M. N., and Sawaya, A. C. H. F. (2014). Phytochemical markers of different types of red propolis. Food Chem. 146, 174–180. doi: 10.1016/j.foodchem.2013.09.063
Lotti, C., Campo Fernandez, M., Piccinelli, A. L., Cuesta-Rubio, O., Márquez Hernández, I., and Rastrelli, L. (2010). Chemical constituents of red Mexican propolis. J. Agric. Food Chem. 58, 2209–2213. doi: 10.1021/jf100070w
Souza Machado, B. A., Silva, R. P. D., Barreto, G. da A., Costa, S. S., da Silva, D. F., Brandão, H. N., et al. (2016). Chemical composition and biological activity of extracts obtained by supercritical extraction and ethanolic extraction of brown, green and red propolis derived from different geographic regions in Brazil. PLoS One 11:e0145954. doi: 10.1371/journal.pone.0145954
Martins, P. L. G., and de Rosso, V. V. (2016). Thermal and light stabilities and antioxidant activity of carotenoids from tomatoes extracted using an ultrasound-assisted completely solvent-free method. Food Res. Int. 82, 156–164. doi: 10.1016/j.foodres.2016.01.015
Meng, Z., Zhao, J., Duan, H., Guan, Y., and Zhao, L. (2018). Green and efficient extraction of four bioactive flavonoids from pollen typhae by ultrasound-assisted deep eutectic solvents extraction. J. Pharm. Biomed. Anal. 161, 246–253. doi: 10.1016/j.jpba.2018.08.048
Murador, D. C., de Souza Mesquita, L. M., Vannuchi, N., Braga, A. R. C., and de Rosso, V. V. (2019a). Bioavailability and biological effects of bioactive compounds extracted with natural deep eutectic solvents and ionic liquids: advantages over conventional organic solvents. Curr. Opin. Food Sci. 26, 25–34. doi: 10.1016/j.cofs.2019.03.002
Murador, D. C., Mercadante, A. Z., and de Rosso, V. V. (2016). Cooking techniques improve the levels of bioactive compounds and antioxidant activity in kale and red cabbage. Food Chem. 196, 1101–1107. doi: 10.1016/j.foodchem.2015.10.037
Murador, D. C., Salafia, F., Zoccali, M., Martins, P. L. G., Ferreira, A. G., Dugo, P., et al. (2019b). Green extraction approaches for carotenoids and esters: characterization of native composition from orange peel. Antioxidants 8:613. doi: 10.3390/antiox8120613
Nam, M. W., Zhao, J., Lee, M. S., Jeong, J. H., and Lee, J. (2015). Enhanced extraction of bioactive natural products using tailor-made deep eutectic solvents: application to flavonoid extraction from Flos sophorae. Green Chem. 17, 1718–1727. doi: 10.1039/C4GC01556H
Marques das Neves, M. V., Silva, T. M. S., Lima, E. D. O., Cunha, E. V. L., and Oliveira, E. D. J. (2016). Isoflavone formononetin from red propolis acts as a fungicide against Candida sp. Braz. J. Microbiol. 47, 159–166. doi: 10.1016/j.bjm.2015.11.009
Oldoni, T. L. C., Cabral, I. S. R., D’Arce, M. A. B. R., Rosalen, P. L., Ikegaki, M., Nascimento, A. M., et al. (2011). Isolation and analysis of bioactive isoflavonoids and chalcone from a new type of Brazilian propolis. Sep. Purif. Technol. 77, 208–213. doi: 10.1016/j.seppur.2010.12.007
Passos, H., Freire, M. G., and Coutinho, J. A. P. P. (2014). Ionic liquid solutions as extractive solvents for value-added compounds from biomass. Green Chem. 16, 4786–4815. doi: 10.1039/c4gc00236a
Patil, V., Mahajan, S., Kulkarni, M., Patil, K., Rode, C., Coronas, A., et al. (2020). Synthesis of silver nanoparticles colloids in imidazolium halide ionic liquids and their antibacterial activities for Gram-positive and Gram-negative bacteria. Chemosphere 243:125302. doi: 10.1016/j.chemosphere.2019.125302
Plechkova, N. V., and Seddon, K. R. (2008). Applications of ionic liquids in the chemical industry. Chem. Soc. Rev. 37, 123–150. doi: 10.1039/B006677J
Reddy, G. K. K., and Nancharaiah, Y. V. (2020). Alkylimidazolium ionic liquids as antifungal alternatives: antibiofilm activity against Candida albicans and anderlying mechanism of action. Front. Microbiol. 11:730. doi: 10.3389/fmicb.2020.00730
Refaat, H., Mady, F. M., Sarhan, H. A., Rateb, H. S., and Alaaeldin, E. (2021). Optimization and evaluation of propolis liposomes as a promising therapeutic approach for COVID-19. Int. J. Pharm. 592:120028. doi: 10.1016/j.ijpharm.2020.120028
Regueira-Neto, M. D. S., Tintino, S. R., Rolón, M., Coronal, C., Vega, M. C., de Queiroz Balbino, V., et al. (2018). Antitrypanosomal, antileishmanial and cytotoxic activities of Brazilian red propolis and plant resin of Dalbergia ecastaphyllum (L) Taub. Food Chem. Toxicol. 119, 215–221. doi: 10.1016/j.fct.2018.04.029
Rodrigues, E., Mariutti, L. R. B., Faria, A. F., and Mercadante, A. Z. (2012). Microcapsules containing antioxidant molecules as scavengers of reactive oxygen and nitrogen species. Food Chem. 134, 704–711. doi: 10.1016/j.foodchem.2012.02.163
Santana Andrade, J. K., Denadai, M., de Oliveira, C. S., Nunes, M. L., and Narain, N. (2017). Evaluation of bioactive compounds potential and antioxidant activity of brown, green and red propolis from Brazilian northeast region. Food Res. Int. 101, 129–138. doi: 10.1016/j.foodres.2017.08.066
Sayyadi, F., Mahdavi, S., Moghadamnia, A. A., Moslemi, D., Shirzad, A., and Motallebnejad, M. (2020). The effect of aqueous and ethanolic extract of Iranian propolis on Candida albicans isolated from the mouth of patients with colorectal malignancy undergone chemotherapy: an in-vitro study. Caspian J. Intern. Med. 11, 62–66. doi: 10.22088/cjim.11.1.62
Silva, F. R. G., Matias, T. M. S., Souza, L. I. O., Matos-Rocha, T. J., Fonseca, S. A., Mousinho, K. C., et al. (2019). Phytochemical screening and in vitro antibacterial, antifungal, antioxidant and antitumor activities of the red propolis Alagoas. Braz. J. Biol. 79, 452–459. doi: 10.1590/1519-6984.182959
Silva, M. P., Silva, T. M., Mengarda, A. C., Salvadori, M. C., Teixeira, F. S., Alencar, S. M., et al. (2021). Brazilian red propolis exhibits antiparasitic properties in vitro and reduces worm burden and egg production in an mouse model harboring either early or chronic Schistosoma mansoni infection. J. Ethnopharmacol. 264:113387. doi: 10.1016/j.jep.2020.113387
Silveira, M. A. D., de Jong, D., Berretta, A. A., Galvão, E. B. D. S., Ribeiro, J. C., Cerqueira-Silva, T., et al. (2021). Efficacy of Brazilian green propolis (EPP-AF®) as an adjunct treatment for hospitalized COVID-19 patients: a randomized, controlled clinical trial. Biomed. Pharmacother. 138:111526. doi: 10.1016/j.biopha.2021.111526
Sivapragasam, M., Moniruzzaman, M., and Goto, M. (2020). An overview on the toxicological properties of ionic liquids toward microorganisms. Biotechnol. J. 15:1900073. doi: 10.1002/biot.201900073
Srivastava, A., Mishra, R., Kumar, S., Dev, K., Tandon, P., and Maurya, R. (2015). Molecular structure, spectral investigation (1H NMR, 13C NMR, UV-visible, FT-IR, FT-Raman), NBO, intramolecular hydrogen bonding, chemical reactivity and first hyperpolarizability analysis of formononetin [7-hydroxy-3(4-methoxyphenyl)chromone]: a quantum. J. Mol. Struct. 1084, 55–73. doi: 10.1016/j.molstruc.2014.11.070
Trusheva, B., Petkov, H., Popova, M., Dimitrova, L., Zaharieva, M., Tsvetkova, I., et al. (2019). ‘GREEN’ approach to propolis extraction: natural deep eutectic solvents. C. R. Acad. Bulg. Sci. 72, 897–905. doi: 10.7546/CRABS.2019.07.06
Ventura, S. P. M., e Silva, F. A., Quental, M. V., Mondal, D., Freire, M. G., and Coutinho, J. A. P. (2017). Ionic-liquid-mediated extraction and separation processes for bioactive compounds: past, present, and future trends. Chem. Rev. 117, 6984–7052. doi: 10.1021/acs.chemrev.6b00550
Washio, K., Kobayashi, M., Saito, N., Amagasa, M., and Kitamura, H. (2015). Propolis ethanol extract stimulates cytokine and chemokine production through NF- κB activation in C2C12 myoblasts. Evid. Based Complement. Alternat. Med. 2015, 1–7. doi: 10.1155/2015/349751
Winn, W. (2008). Diagnóstico Microbiológico: Texto e Atlas Colorido. 6a Edn. Rio de Janeiro: Guanabara Koogan.
Xiao, J., Chen, G., and Li, N. (2018). Ionic liquid solutions as a green tool for the extraction and isolation of natural products. Molecules 23:1765. doi: 10.3390/molecules23071765
Zhang, C.-P., Liu, G., and Hu, F.-L. (2012). Hydrolysis of flavonoid glycosides by propolis β-glycosidase. Nat. Prod. Res. 26, 270–273. doi: 10.1080/14786419.2010.541877
Keywords: Brazilian propolis, red propolis, flavonoids, ionic liquids, eutectic solvents
Citation: dos Santos CM, de Souza Mesquita LM, Braga ARC and de Rosso VV (2021) Red Propolis as a Source of Antimicrobial Phytochemicals: Extraction Using High-Performance Alternative Solvents. Front. Microbiol. 12:659911. doi: 10.3389/fmicb.2021.659911
Edited by:
Fabricio Luiz Tulini, Federal University of Western Bahia, BrazilReviewed by:
Piotr Okińczyc, Wroclaw Medical University, PolandMilena Popova, Institute of Organic Chemistry With Centre of Phytochemistry (BAS), Bulgaria
Salwa Abu El Kassem, Al-Azhar University, Egypt
Copyright © 2021 dos Santos, de Souza Mesquita, Braga and de Rosso. This is an open-access article distributed under the terms of the Creative Commons Attribution License (CC BY). The use, distribution or reproduction in other forums is permitted, provided the original author(s) and the copyright owner(s) are credited and that the original publication in this journal is cited, in accordance with accepted academic practice. No use, distribution or reproduction is permitted which does not comply with these terms.
*Correspondence: Anna Rafaela C. Braga, YW5uYS5icmFnYUB1bmlmZXNwLmJy