- 1College of Fisheries, Guangdong Ocean University, Zhanjiang, China
- 2Haimao Seed Technology Group Co., Ltd., Zhanjiang, China
- 3Aquatic Animals Precision Nutrition and High Efficiency Feed Engineering Research Center of Guangdong Province, Zhanjiang, China
- 4Guangdong Provincial Laboratory of Southern Marine Science and Engineering, Zhanjiang, China
- 5Guangdong Provincial Key Laboratory of Pathogenic Biology and Epidemiology for Aquatic Economic Animals, Zhanjiang, China
As a new type of shrimp lethal virus, decapod iridescent virus 1 (DIV1) has caused huge economic losses to shrimp farmers in China. Up to now, DIV1 has been detected in a variety of shrimps, but there is no report in Marsupenaeus japonicus. In the current study, we calculated the LC50 to evaluate the toxicity of DIV1 to M. japonicus and determined through nested PCR that M. japonicus can be the host of DIV1. Through enzyme activity study, it was found that DIV1 can inhibit the activities of superoxide dismutase, catalase, lysozyme, and phenoloxidase, which could be a way for DIV1 to achieve immune evasion. In a comprehensive study on the transcriptomic changes of M. japonicus in response to DIV1 infection, a total of 52,287 unigenes were de novo assembled, and 20,342 SSR markers associated with these unigenes were obtained. Through a comparative transcriptomic analysis, 6,900 differentially expressed genes were identified, including 3,882 upregulated genes and 3,018 downregulated genes. The Gene Ontology (GO) and Kyoto Encyclopedia of Genes and Genomes (KEGG) enrichment analysis showed that some GO terms related to virus invasion, replication, and host antiviral infection were promoted under DIV1 infection, such as carbohydrate binding, chitin binding, chitin metabolic process, and DNA replication initiation, and some KEGG pathways related to immune response were significantly influenced by DIV1 infection, including Toll and IMD signaling pathway, JAK-STAT signaling pathway, IL-17 signaling pathway, C-type lectin receptor signaling pathway, complement and coagulation cascades, antigen processing and presentation, necroptosis, apoptosis, NOD-like receptor signaling pathway, apoptosis—multiple species, and TNF signaling pathway. Further analysis showed that STAT, Dorsal, Relish, heat shock protein 70 (HSP70), C-type lectins, and caspase play an important role in DIV1 infection. This is the first detailed study of DIV1 infection in M. japonicus, which initially reveals the molecular mechanism of DIV1 infection in M. japonicus by using the transcriptome analysis of hemocytes combined with enzyme activity study.
Introduction
Iridoviridae is a member of a monophyletic clade of large, nucleocytoplasmic DNA viruses with double-stranded DNA genomes (İnce et al., 2018). According to their particle sizes, host range, DNA cross-hybridization, the presence of a methyltransferase, and the sequence of major capsid protein, family Iridoviridae is subclassified into five genera, including Iridovirus, Chloriridovirus, Ranavirus, Lymphocystivirus, and Megalocytivirus (Chinchar et al., 2017). The iridescent viruses mostly infect invertebrates and poikilothermic vertebrates, such as teleost fish, crustaceans, amphibians, insects, and reptiles (Matthews, 1979; Chinchar et al., 2011; Kurita and Nakajima, 2012; Xu et al., 2016). In recent years, Xu et al. (2016) and Qiu et al. (2017) respectively isolated and identified two iridescent viruses from red claw crayfish Cherax quadricarinatus and Litopenaeus vannamei and named them C. quadricarinatus iridovirus (CQIV CN01) and shrimp hemocyte iridescent virus (SHIV 20141215). Because the genome similarity between these two original isolations was 99%, the Executive Committee of the The International Committee on Taxonomy of Viruses (ICTV) (2019) identified SHIV 20141215 and CQIV CN01 as two virus isolates of decapod iridescent virus 1 (DIV1). This is the only species of the new genus Decapodiridovirus within the family Iridoviridae. As a new type of shrimp lethal virus, DIV1 has been detected in a variety of shrimps, such as L. vannamei, Fenneropenaeus merguiensis, C. quadricarinatus, Exopalaemon carinicauda, Macrobrachium rosenbergii, Penaeus monodon, and so on (Xu et al., 2016; Chen X. et al., 2019; Qiu et al., 2019; Liao X. et al., 2020; Liao X. Z. et al., 2020; He et al., 2021).
Shrimp farming is an important part of the crustacean farming industry. According to the latest worldwide statistics on aquaculture compiled by the Food and Agriculture Organization of the United Nations (FAO, 2020), the total crustacean production of the world in 2018 reached 9,386.5 thousand tonnes, with a total farmgate sale value of USD 69.3 billion. Among them, the five main cultured shrimps of L. vannamei, Procambarus clarkii, P. monodon, Macrobrachium nipponense, and M. rosenbergii accounted for 84.1% of the crustacean production. There is no doubt that shrimp farming had become an important source of income for crustacean farmers. However, with the rapidly increasing shrimp production, frequent outbreaks of various diseases followed. Virus pathogens, such as white spot syndrome virus (WSSV), Taura syndrome virus (TSV), and the infectious hypodermal and hematopoietic necrosis virus (IHHNV), still represent one of the major impediments to the development of shrimp culture (Thitamadee et al., 2016; Li C. et al., 2019). In recent years, the newly discovered shrimp lethal virus DIV1 has brought a huge challenge to the shrimp aquaculture industry due to its wide host spectrum and strong toxicity. On the one hand, the wide host spectrum makes DIV1 widely existent in natural and breeding environments, which makes it difficult for people to effectively control its spread. On the other hand, the strong toxicity of DIV1 can cause the large-scale deaths of farmed shrimps and cause huge economic losses to farmers. Kuruma shrimp Marsupenaeus japonicus is widely distributed in the Indo-Western Pacific region and the East and South China seas (Dall et al., 1990). Because of its high economic value, M. japonicus has become one of the main cultured shrimp species in China. Only in 2019, 55,228 tonnes of M. japonicus was harvested in China, which brought substantial economic benefits to the shrimp farmers (FBMA, 2020). To date, there are no detailed reports on DIV1 infection of M. japonicus.
Similar to other invertebrates, crustaceans mainly rely on their innate immune system, consisting of humoral immunity and cellular immunity, to defend against pathogens (Li and Xiang, 2013). Hemocytes are a major part of the shrimp innate immune system, which not only remove foreign substances through phagocytosis, encapsulation, and nodule formation but also resist pathogens and repair cell damage by producing reactive oxygen species (ROS), antimicrobial peptides, immune enzymes, and other immune factors (Jiravanichpaisal et al., 2006; Cerenius et al., 2010). Superoxide dismutase (SOD), catalase (CAT), lysozyme (LYZ), and phenoloxidase (PO) are four important immune enzymes, which have the function of anti-oxidant and anti-pathogen (Holmblad and Söderhäll, 1999; Cerenius and Söderhäll, 2004; Kaizu et al., 2011). The activity of these immune enzymes is usually used to reflect the impact of shrimp immunity after a pathogen infection (such as WSSV, IHHNV, Vibrio parahaemolyticus, and Spiroplasma eriocheiris) (Arockiaraj et al., 2012; Ren et al., 2020; Zhu et al., 2020; Jiao et al., 2021), harmful substance invasion (such as microcystin and tributyltin) (Wu et al., 2014; Chen et al., 2015), or dietary improvement (such as polypeptides and dietary hydrolyzable tannins) (Liao et al., 2019; Zhu et al., 2021). With the rapid development of high-throughput RNA sequencing technologies, including RNA sequencing (RNA-seq), more and more studies use RNA-seq to expose the immune molecular mechanism of shrimp against viral infections, bacterial infections, and environmental stress (Kurita and Nakajima, 2012; Li and Xiang, 2013; Wang et al., 2014; Zeng et al., 2014; Qin et al., 2018; Jin and Zhu, 2019; Nian et al., 2019; Duan et al., 2020). Among them, hemocytes were widely used in transcriptome studies of shrimp virus infections, such as WSSV, TSV, and DIV1 (Sookruksawong et al., 2013; Ghani and Bhassu, 2019; Wang et al., 2019; Liao X. et al., 2020). Therefore, the immune molecular mechanism of shrimp against a virus infection can be better understood by using the transcriptome analysis of hemocytes combined with enzyme activity study.
Since there is no detailed report on the DIV1 infection of M. japonicus, RNA-seq was applied in this study to explore the molecular mechanisms of M. japonicus hemocytes against DIV1 infection, based on the results of the LC50 test and the enzyme activity analysis. The result is of great value for diagnosing shrimp DIV1 infection and developing methods to prevent virus outbreaks.
Materials and Methods
Shrimp Culture
The study protocol was approved by the Ethics Review Board of the Institutional Animal Care and Use Committee in Guangdong Ocean University. Healthy juvenile M. japonicus were purchased from Suixi County Zhongli Aquatic Products Co., Ltd., and raised in the East Island Marine Biology Research Base, Guangdong Ocean University in Zhanjiang, Zhanjiang, China. Before the LC50 test, every 30 healthy M. japonicus (10.5 ± 1.6 g) were randomly selected to acclimatize for 1 week in a 0.3-m3 tanks with filtered seawater and continuous aeration. The salinity, pH, and temperature of the seawater were maintained at ∼30‰, ∼7.5, and ∼28 C, respectively. The shrimp were fed three times per day with commercial shrimp expanded pellets (Tongwei Co., Ltd., China), and the seawater was changed nearly 90% once a day. The shrimp were then randomly sampled and examined for potential pathogens by PCR to ensure that they were free from WSSV, IHHNV, and DIV1. The primers for pathogen detection are shown in Table 1.
LC50 Test
In the LC50 test, M. japonicus were randomly divided into seven groups; each group had three tanks. Among them, each healthy M. japonicus in six groups was intramuscularly injected with 50 μl of viral inoculum, and the concentrations were 3.95 × 109, 3.95 × 108, 3.95 × 107, 3.95 × 106, 3.95 × 105, and 3.95 × 104 copies/μg DNA, respectively. The other group was injected with 50 μl phosphate-buffered saline (PBS, pH 7.4) instead of the viral inoculum. The cumulative survival rate was counted every 4 h, and dead shrimp were picked up to avoid a secondary infection. The methods of viral inoculum preparation and quantification can be found in previous studies (Qiu et al., 2018; Chen X. et al., 2019). The DIV1 inoculum was tested by nest PCR to ensure that it was not contaminated with WSSV and IHHNV, and the nest PCR primers, specific qPCR primers, and TaqMan probe for DIV1 detection and quantification are shown in Table 1. The LC50 was calculated by probit analysis on SPSS 19.0 program (SPSS Inc., Chicago, IL, United States) using the Bliss method (Bliss, 1939).
Sample Collection
A total of 30 healthy M. japonicus injected with 50 μl of DIV1 inoculum of 3.95 × 109 copies/μg DNA were used as the DIV1-infected group, and the other 30 healthy M. japonicus injected with 50 μl of PBS were used as the negative control group. The weight of M. japonicus and the culture conditions were also kept the same as in the LC50 test (refer to the previous article; He et al., 2021). The M. japonicus at 24 h post-injection (hpi) were selected to make samples under an aseptic condition. The hemolymph was withdrawn into modified ACD anticoagulant solution, and the hemocytes and plasma were separated by centrifugation (3,000 × g for 5 min at 4 C). The plasma and hemocytes from three individuals in the same group were combined as one sample and immediately frozen in liquid nitrogen before storing at −80 C until enzyme activity analysis or RNA extraction.
Enzyme Activity Analysis
Prior to analysis, the homogenized solid samples were homogenized in pre-chilled PBS (1:9 dilution) and then centrifuged for 10 min (4 C and 5,000 × g) to obtain the supernatant for further use. SOD, CAT, LYZ, and PO in the plasma were measured using commercial detection kits (Jianglai Bioengineering Institute, Shanghai, China) according to the protocols of the manufacturer.
RNA Extraction and Transcriptome Sequencing
Total RNA was extracted separately from the hemocytes of three non-infected and three DIV1-infected samples using TranZol Up Plus RNA Kit (TransGen, Beijing, China) following the protocol of the manufacturer, and the RNA concentration was determined through SimpliNano (GE Healthcare, United States). Fragmentation buffer was used to break the mRNA into short fragments. Using mRNA as a template, the first-strand cDNA was synthesized using random hexamers, and then buffer, dNTPs, RNase H, and DNA polymerase I were added to synthesize the second-strand cDNA. After purification and elution with EB buffer, cDNA end-repair, and adenylation at the 3′ end, poly (A) was added and the sequencing adaptor was connected. Finally, transcriptome sequencing was performed by BGI (Shenzhen, China) with the Illumina Genome Analyzer technology.
De novo Assembly and Data Annotation
Raw reads were filtered to remove adaptor sequences, ambiguous “N” nucleotides (with a ratio of “N” more than 5%), and low-quality sequences (with a quality score less than 15) using trimmomatic software (v0.36) (Bolger et al., 2014). Meanwhile, the Q20, Q30, and GC contents of the clean reads were calculated. De novo assembly was accomplished using Trinity software (v2.0.6) (Haas et al., 2013). Based on the sequence similarity and length, TGICL (v2.1) was used to remove redundant sequences and generate unigenes (Pertea et al., 2003). The completeness of the assembly was assessed using BUSCO (v3.0.2) with the BUSCO arthropod dataset (Seppey et al., 2019). The assembled unigenes were annotated with five functional databases, including Kyoto Encyclopedia of Genes and Genomes (KEGG),1 Gene Ontology (GO),2 Nr,3 Swiss-Prot,4 and Clusters of Orthologous Genes.5
Analysis of Differentially Expressed Genes and Functional Enrichment
Fragments per kilobase of transcript per million reads method was used to measure the transcript expression levels of the unigenes. A differential expression analysis of the two groups was performed using the DESeq2 package (1.30.0). The p-value was adjusted using the q-value, and genes with q-value < 0.05 and | log2 (fold change)| > 1 found by DESeq2 were considered as differentially expressed genes (DEGs) (Love et al., 2014). All DEGs were further analyzed using GO and KEGG database to look for significantly enriched GO and KEGG terms.
Identification of Simple Sequence Repeat Loci in the Transcripts
SSR molecular marker technology has been wildly applied in the field of aquatic breeding, inheritance, and evolution (Andriantahina et al., 2013; Wang et al., 2018; Huang et al., 2020). To identify potential SSR loci from the transcriptome, sequences with six types of repeat unit, including mono-nucleotide, di-nucleotide, tri-nucleotide, tetra-nucleotide, penta-nucleotide, and hexa-nucleotide repeat SSR, were detected using the MISA software.6 The parameters were set for the detection of mono-nucleotide, di-nucleotide, tri-nucleotide, tetra-nucleotide, penta-nucleotide, and hexa-nucleotide repeat SSR with a minimum of 12, six, five, five, four, and four repetitive units, respectively (Wei et al., 2017).
Validation by qRT-PCR
To validate the gene expression profiles from Illumina sequencing results, five upregulated DEGs and seven downregulated DEGs were selected to perform qRT-PCR. Among them were STAT, Dorsal and Relish belonging to JAK-STAT, Toll, and IMD signaling pathway, which are very important for the host against viral infection. Primer 5.0 software was used to design the primers for these DEGs based on the sequences obtained by Illumina sequencing, and elongation factor-1 gene alpha (EF-1α) of M. japonicus was used as an internal reference gene. The specific primers are shown in Table 1. The qRT-PCR was performed using Bio-Rad CFX-96 real-time PCR system. The template cDNA was reverse-transcribed using the 5× All-in-One RT Master Mix (Applied Biological Materials, Vancouver, BC, Canada) according to the protocol of the manufacturer. The qRT-PCR reaction was performed with a 20-μl reaction mixture containing 10 μl of 2× ChamQ Universal SYBR qPCR Master Mix, 1 μl of each primer (10 μM), 2 μl of diluted cDNA template, and 7 μl of ultrapure water. The reaction cycle parameters were as follows: 95°C for 2 min, 40 cycles of 95°C for 15 s, and 60°C for 30 s. All reactions were performed with three technical replicates. The relative expression ratio of the target gene versus EF-1α was calculated by the 2–ΔΔCt method (Livak and Schmittgen, 2001).
Results
LC50 of DIV1 for M. japonicus
Marsupenaeus japonicus infected with DIV1 showed obvious clinical symptoms, including empty stomach and intestine, atrophy of the hepatopancreas with yellowing, red body, and soft shell (Figures 1A,B). As shown in Figures 1C,D, only DIV1 was detected in the diseased M. japonicus used for the DIV1 inoculum and the M. japonicus injected with DIV1. The survival rates of M. japonicus after exposure to DIV1 at different doses are shown in Figure 1E. The shrimp mortality rate increased as the virus dose increased. M. japonicus infected with 3.95 × 109 copies/μg DNA DIV1 inoculum had a mortality rate of 100% at 76 hpi, and the mortality rate of the other injection doses was stable at 80 hpi. Probit analysis showed that the LC50 of DIV1 infection in M. japonicus is 2.64 × 109, 3.61 × 106, 2.69 × 105, 1.05 × 105, and 6.24 × 104 copies/μg DNA at 36, 48, 60, 72, and 84 hpi, respectively (Figure 1E).
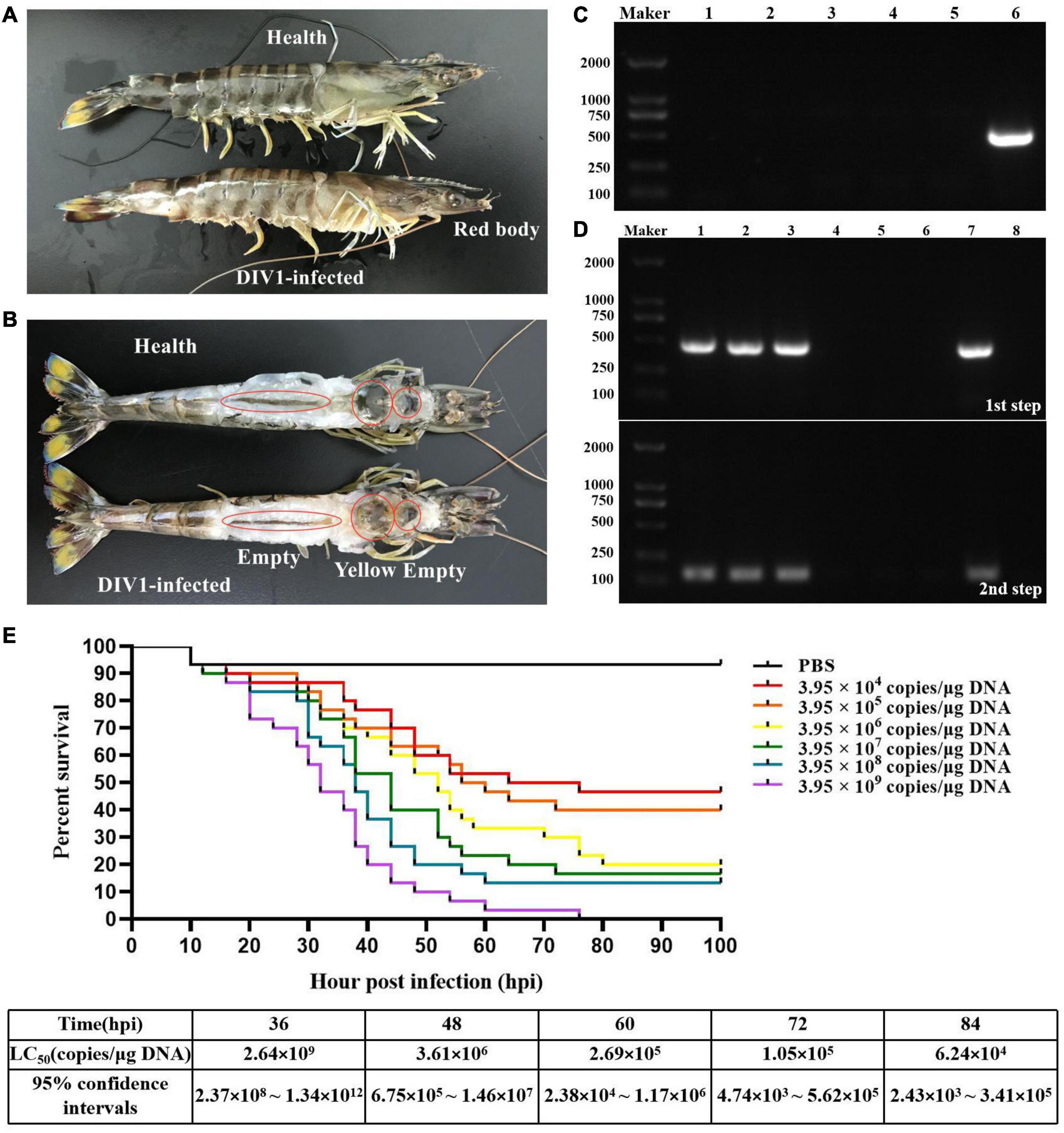
Figure 1. Clinical symptoms and LC50 test. (A,B) Clinical symptoms of DIV1-infected Marsupenaeus japonicus. (C) Virus detection of healthy M. japonicus and diseased shrimp used for LC50 test. Marker, DL2000 molecular mass marker; lanes 1–3: PCR-amplified products used for WSSV, IHHNV, and DIV1 detection in healthy M. japonicus; lanes 4–6, PCR-amplified products used for WSSV, IHHNV, and DIV1 detection in diseased shrimp. (D) DIV1 detection of M. japonicus hemocytes using nested PCR method. Marker: DL2000 molecular mass marker; lanes 1-3: PCR amplified products in DIV1-infected group; lanes 4-6: PCR amplified products in negative control group; lanes 7 and 8: PCR amplified products in positive and negative controls. (E) Seven groups of healthy M. japonicus were intramuscularly injected with 50 μl of DIV1 inoculum at six concentrations and phosphate-buffered saline as a control.
Immune Enzyme Activities in the Tissues of M. japonicus
After infection with DIV1 at 24 hpi, the activities of SOD, CAT, LYZ, and PO in the plasma were detected to evaluate the effect of DIV1 infection on the immune enzymes of M. japonicus (Figure 2). The results showed that the activity of SOD in the plasma was the highest, followed by PO, CAT, and LYZ. It was worth noting that, after infection with DIV1, the activities of the four immune enzymes were all significantly decreased in the plasma (p < 0.05).
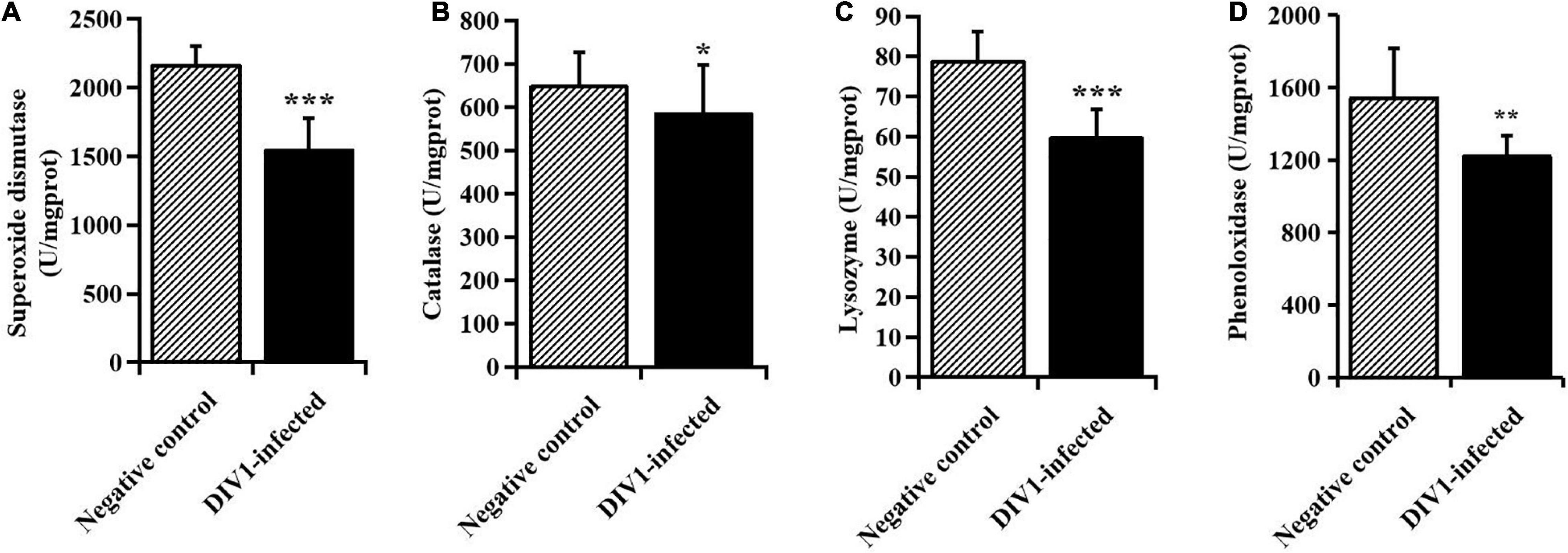
Figure 2. The activities of immune enzymes in the plasma of Marsupenaeus japonicus (mean ± SD). (A) Superoxide dismutase, (B) catalase, (C) lysozyme, and (D) phenoloxidase (PO). The statistically significant differences between the two groups were calculated by Student’s t-test (*p < 0.05, **p < 0.01, ***p < 0.001).
Statistics of Transcriptome Sequencing and de novo Assembly
To identify immune-related genes which are vital for M. japonicus defense against DIV1 infection, six cDNA libraries were prepared from hemocyte samples collected from DIV1-infected and non-infected M. japonicus with three biological replicates. After the removal of the adapter sequence and low-quality reads, a total of 127,748,656 and 128,230,224 clean reads that represent a total of 19.16 and 19.24 Gb nucleotides were generated for the negative control and DIV1-infected group, respectively. The CG content of the clean reads was 39.63% in the negative control group and 40.04% in the DIV1-infected group, respectively (Table 2). All sequencing reads were deposited into the Sequence Read Archive of the National Center for Biotechnology Information and are available with the accession number PRJNA720475.7 After removing redundancy of the assembled contigs, a total of 52,287 unigenes were recovered. The size and the length distribution of the negative control group and the DIV1-inected group unigenes are shown in Figure 3A. Among them, most of the unigenes (14,968, 28.63%) were 200–300 nt in length, followed by 300–400 nt (6,984, 13.36%), and 2,773 unigenes (8.66%) were ≥ 3,000 nt.
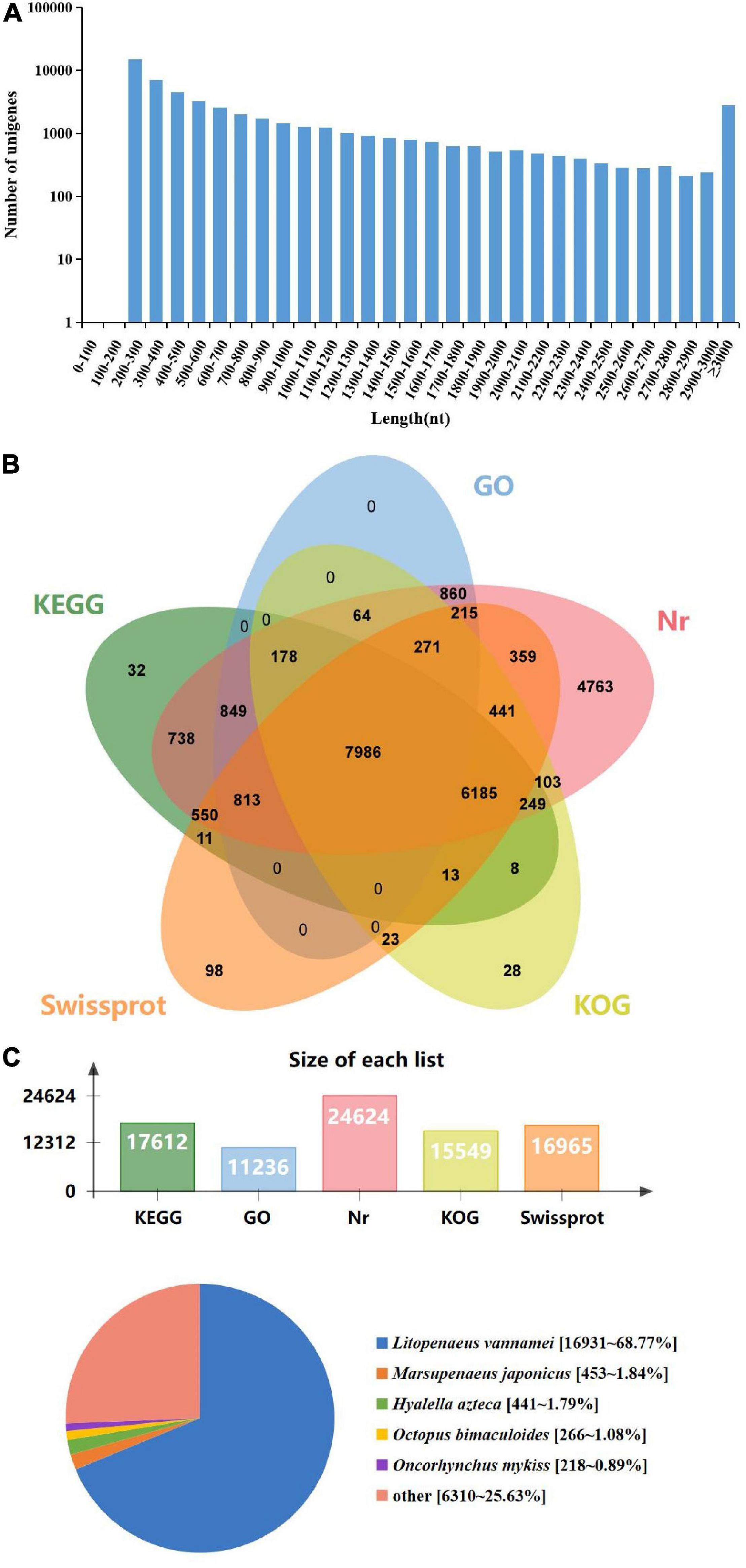
Figure 3. Length distribution, annotation, and species distribution of transcriptome unigenes. (A) The X-axis indicates the length of the unigenes, while the Y-axis indicates the number of unigenes. (B) The Venn diagram shows the annotation of the unigenes from the Marsupenaeus japonicus transcriptome. (C) The species distribution of unigene BLASTx matches against the Nr protein database and the proportions for each species.
Annotation of the Assembled Sequences
To obtain comprehensive function information of unigenes, the unigenes were annotated in five major databases (Figure 3B). These databases included KEGG (17,612 unigenes), GO (11,236 unigenes), Nr (24,624 unigenes), Eukaryotic Orthologous Groups (KOG; 15,549 unigenes), and Swiss-Prot (16,965 unigenes). The analysis showed that among the 52,287 unigenes, most of the unigenes were annotated in Nr. The species distribution of the most significant hits in the Nr database was examined to learn the sequence conservation of M. japonicus compared with other species (Figure 3C). Over 74% of the total unigenes matched with the sequences from five top-hit species: L. vannamei (68.77%), M. japonicus (1.84%), Hyalella azteca (1.79%), Octopus bimaculoides (1.08%), and Oncorhynchus mykiss (0.89%), all of which belong to aquatic organisms.
The unigenes were aligned to GO terms; 28,444 unigenes were mainly divided into three categories with 42 functional groups: biological process (17 functional groups), cellular component (12 functional groups), and molecular function (13 functional groups). In the biological process category, most unigenes were involved in the “cellular process” (9.40%), “biological regulation” (5.70%), and “cellular component organization or biogenesis” (3.80%). In the category of cellular component, “membrane part” (15.56%) and “cell” (8.59%) were the most represented. As for the molecular function category, “binding” (18.92%) and “catalytic activity” (15.77%) were the dominant groups (Figure 4).
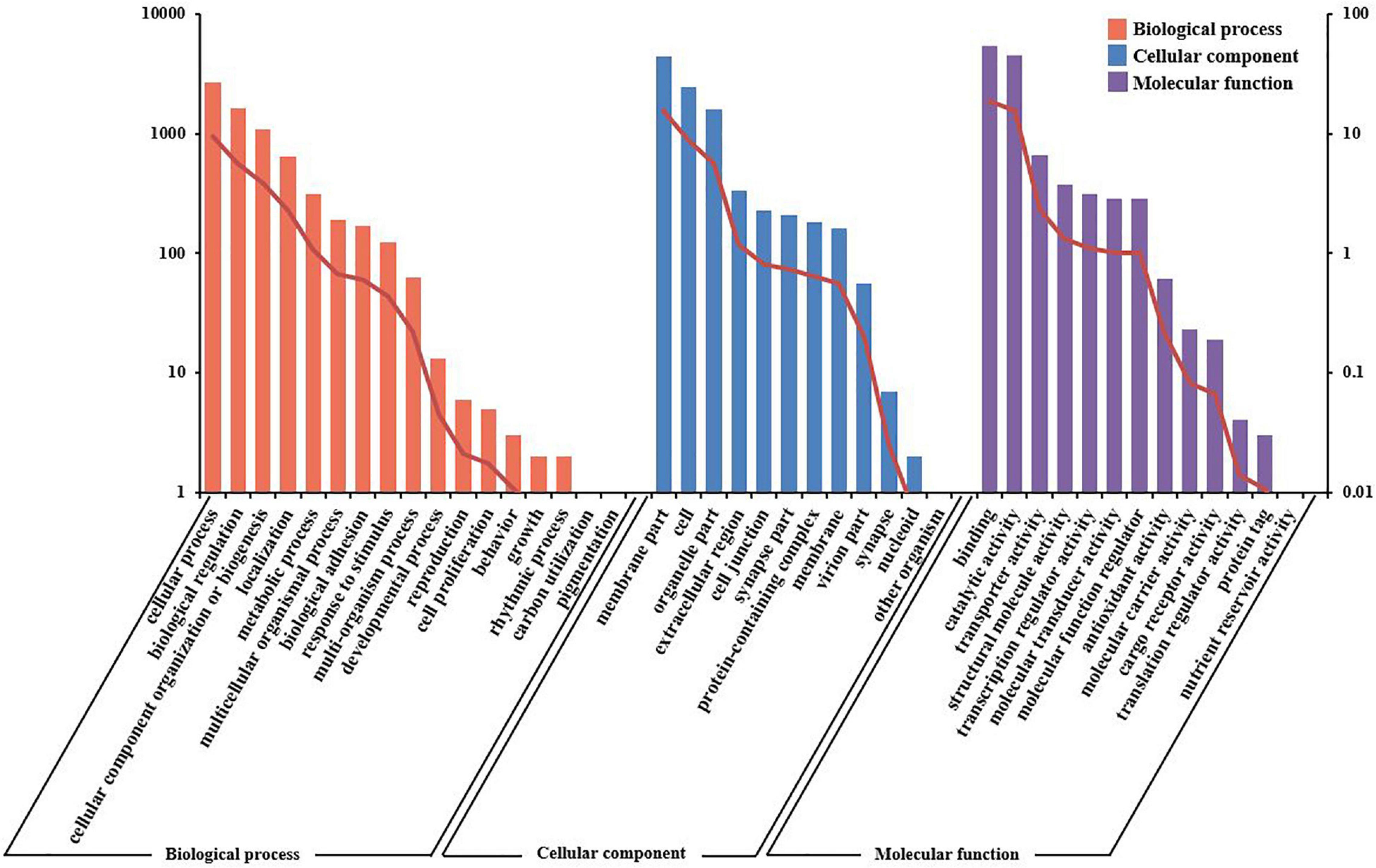
Figure 4. Gene ontology (GO) terms and annotation of the integrated transcriptome assembly. Three main GO categories: cellular component, molecular function, and biological process. The X-axis indicates GO categories, while the Y-axis indicates the number of genes and percentage of genes.
Using the KOG database to further explore the protein orthologs of the assembled unigenes, 15,549 unigenes were successfully annotated with 25 specific protein function definitions or orthologous categories. The largest three categories were “general function prediction only” (2,661, 17.11%), “signal transduction mechanisms” (1,538, 9.89%), and “function unknown” (1,430, 9.20%). The two smallest categories were “coenzyme transport and metabolism” (106, 0.68%) and “cell motility” (37, 0.24%) (Figure 5).
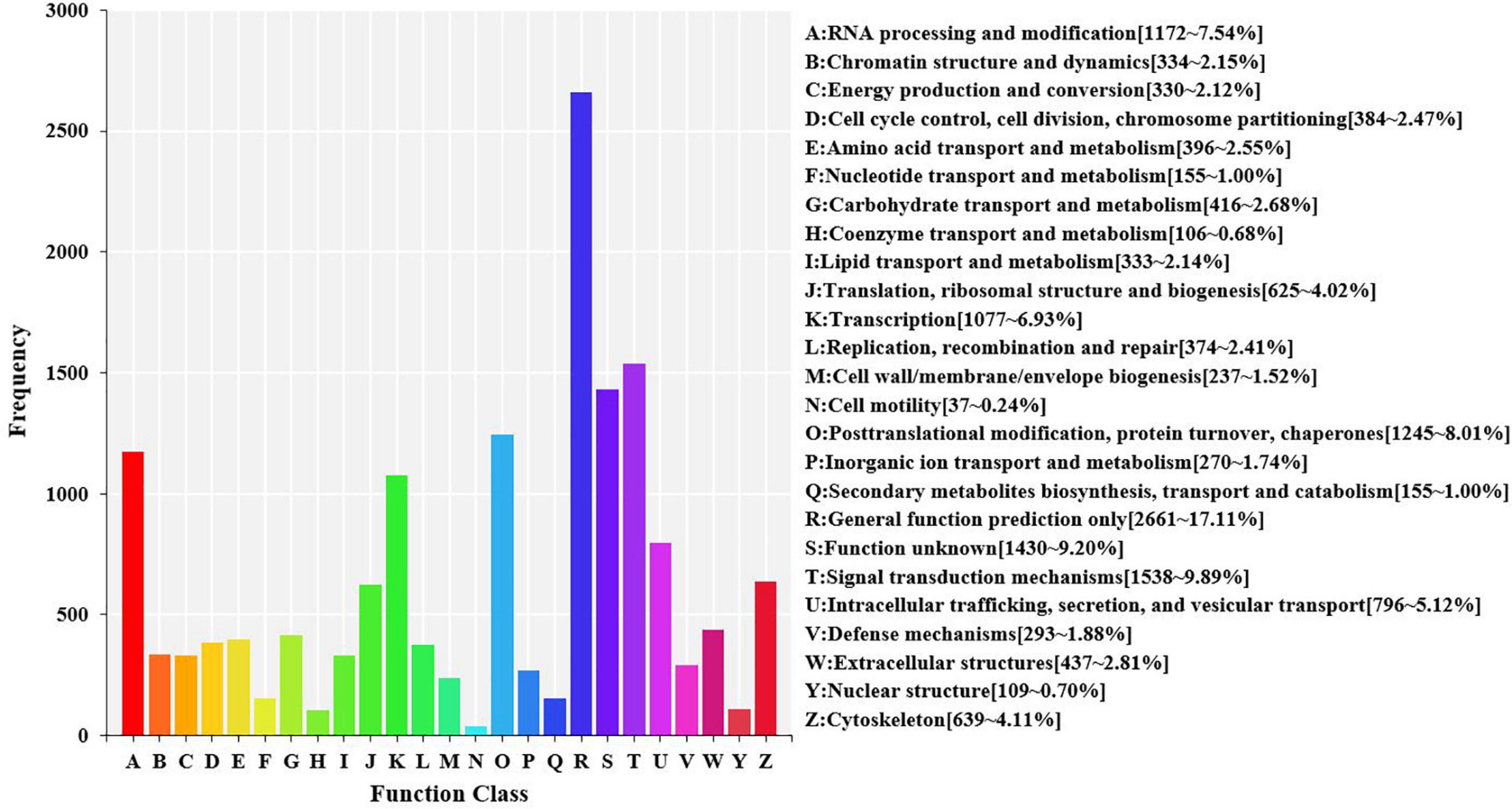
Figure 5. Eukaryotic orthologous groups (KOG) function classification in the transcriptome of hemocytes in Marsupenaeus japonicus hemocytes. Each bar represents the number of unigenes classified into each of the 25 KOG functional categories. The X-axis stands for the functional categories, and the Y-axis indicates the frequency.
To identify the biological processes of the unigenes, 32,094 unigenes that were assigned were annotated using the KEGG database and assigned to different pathways in six major groups of the KEGG pathways, including cellular processes, environmental information processing, genetic information processing, human diseases, metabolism, and organismal systems. These annotated unigenes were further divided into 44 levels and two subcategories. The largest subcategory group was signal transduction (2,585 unigenes), followed by global and overview maps (1,888 unigenes) and infectious diseases: viral (2,304 unigenes) (Figure 6).
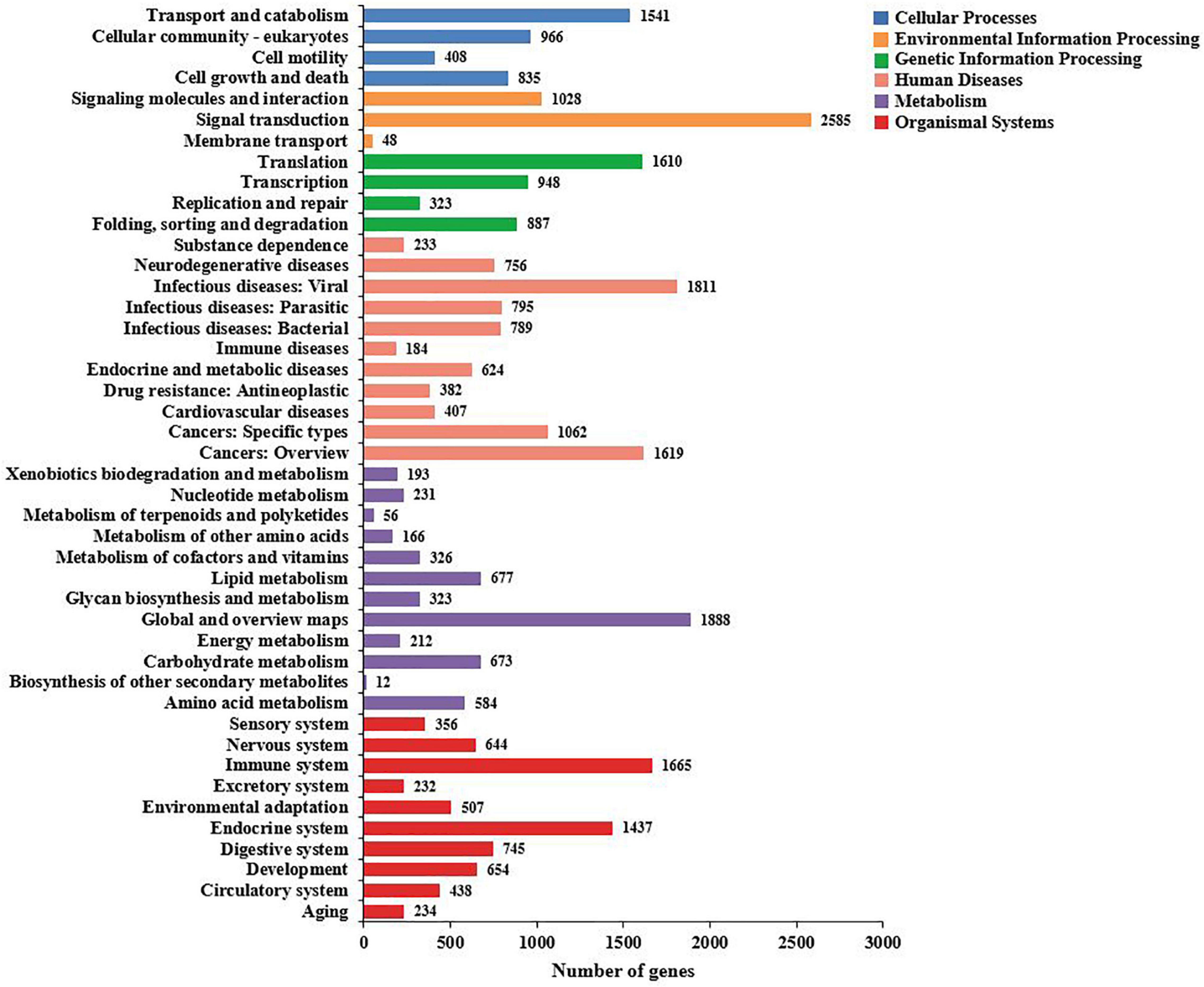
Figure 6. The Kyoto Encyclopedia of Genes and Genomes (KEGG) biological pathway classification histograms for annotated unigenes identified in the hemocytes of Marsupenaeus japonicus. Each bar represents the number of unigenes classified into different biological processes. The X-axis shows the number of matched unigenes, while the Y-axis shows the pathways from the KEGG classification.
Identification and Functional Characterization of DEGs
To identify the DEGs between the DIV1-infected group and the negative control group, the transcript abundance in each unigene was compared between the two groups using the FRKM approach. A total of 6,900 DEGs were screened, including 3,882 upregulated genes and 3,018 downregulated genes, with a cut-off q-value < 0.05 and | log2 (fold change)| > 1 (Figure 7). The DEGs were found to have a variety of biological functions according to the Nr annotation. Among them, some well-characterized genes implicated in innate immune defense, such as Dorsal, Relish, C-type lectin 1 (CTL1), caspase 1, inhibitor of apoptosis protein, heat shock protein 70 (HSP70), heat shock protein 90, and prophenoloxidase activating enzyme 2 (PPAE2) (Table 3).
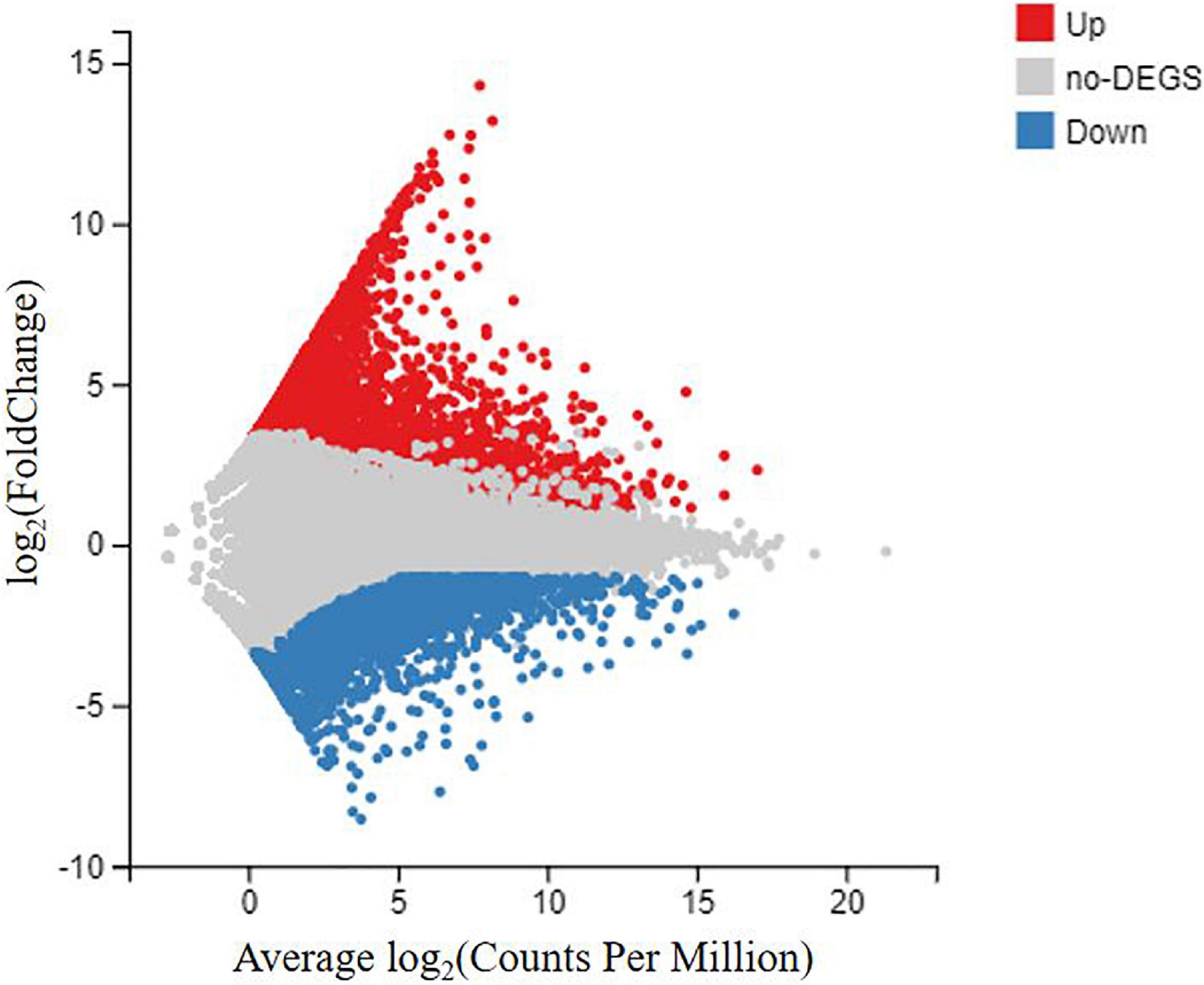
Figure 7. MA plot between differentially expressed genes (DEGs) of the DIV1-infected group and the negative control group of Marsupenaeus japonicus hemocytes. The X-axis indicates the average expression level, and the Y-axis indicates the fold change. Red dots represent the significantly upregulated DEGs, while blue dots represent the significantly downregulated DEGs [q-value < 0.05 and | log2 (fold change)| > 1]. Gray dots represent the DEGs which were not significantly different.
To further evaluate the biological function, all the DEGs were mapped to the term in the GO and KEGG databases. In the GO enrichment analysis, 1,237 upregulated genes and 788 downregulated genes expressed in the DIV1-infected group were divided into three categories with 55 functional groups, consisting of biological progress (24 functional groups), cellular component (19 functional groups), and molecular function (12 functional groups). The top 20 GO terms influenced by DIV1 infection are shown in Figure 8A. Compared to the healthy group, DIV1 infection promoted carbohydrate binding (32 upregulated genes and three downregulated genes), chitin binding (26 upregulated genes and five downregulated genes), chitin metabolic process (26 upregulated genes and four downregulated genes), and DNA replication initiation (11 upregulated genes). These GO terms may be related to virus invasion, replication, and host antiviral infection.
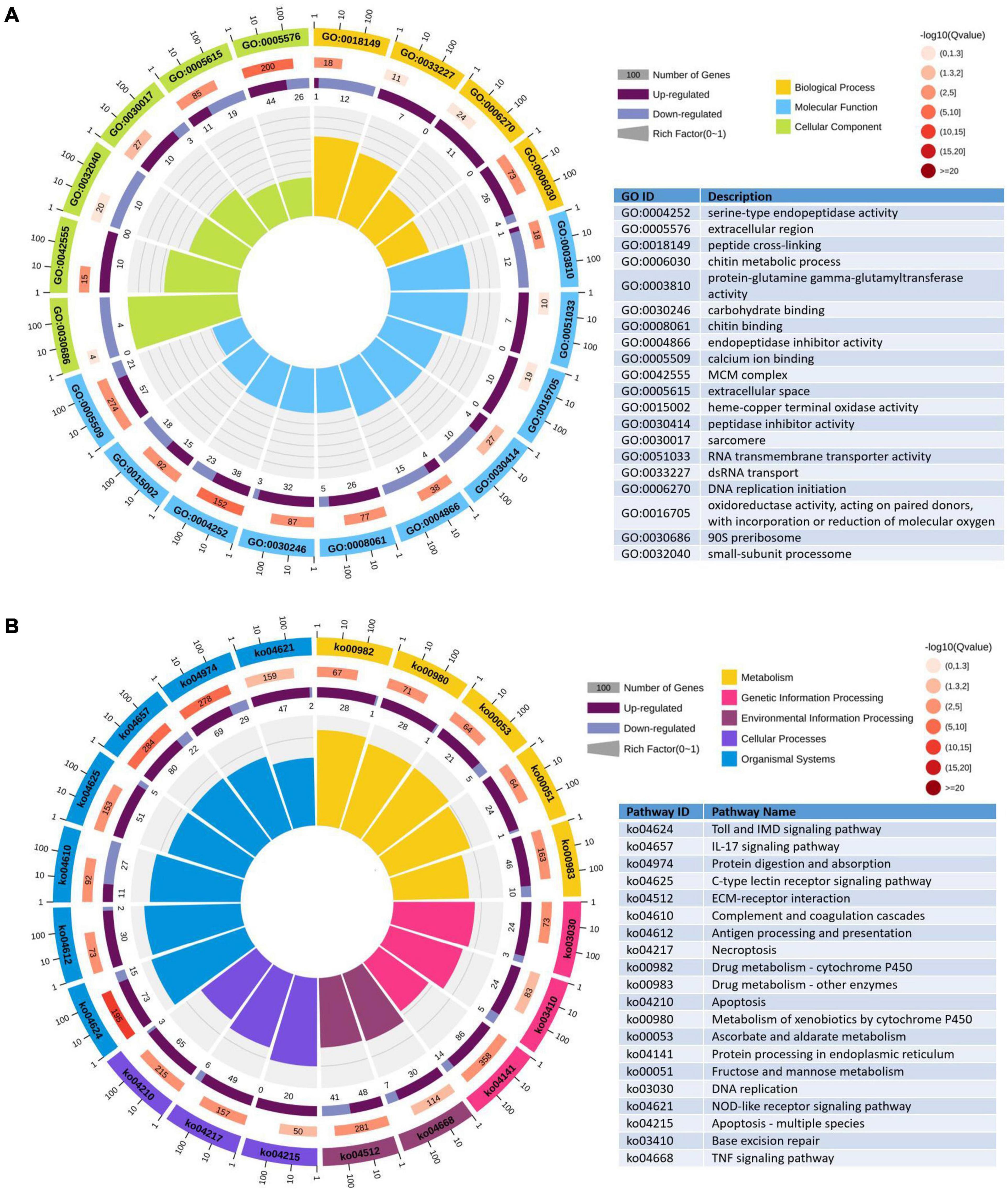
Figure 8. Gene Ontology (GO) terms and Kyoto Encyclopedia of Genes and Genomes (KEGG) pathway enrichment analysis of differentially expressed genes (DEGs). (A) The first lap indicates the top 20 GO terms and the number of genes that correspond to the outer lap. The second lap indicates the number of genes in the genome background and the q-values for enrichment of the DEGs for the specified biological process. The third lap indicates the ratio of upregulated genes (deep purple) and downregulated genes (light purple). The fourth lap indicates the enrichment factor of each GO term. (B) The first lap indicates the top 20 KEGG terms and the number of genes that correspond to the outer lap. The second lap indicates the number of genes in the genome background and q-values for enrichment of the DEGs for the specified biological process. The third lap indicates the ratio of upregulated genes (deep purple) and downregulated genes (light purple). The fourth lap indicates the enrichment factor of each KEGG term.
For the KEGG pathway enrichment analysis, 2,140 DEGs were annotated into 249 pathways. Among them, 1,396 upregulated genes were annotated into 247 pathways, and 744 downregulated genes were annotated into 217 pathways. The top 20 KEGG pathway enrichments influenced by DIV1 infection are shown in Figure 8B. It was worth noting that half of them are related to immune response, such as Toll and IMD signaling pathway (73 upregulated genes and 15 downregulated genes), IL-17 signaling pathway (80 upregulated genes and 22 downregulated genes), C-type lectin receptor signaling pathway (51 upregulated genes and five downregulated genes), complement and coagulation cascades (11 upregulated genes and 27 downregulated genes), antigen processing and presentation (30 upregulated genes and two downregulated genes), necroptosis (49 upregulated genes and six downregulated genes), apoptosis (65 upregulated genes and three downregulated genes), NOD-like receptor signaling pathway (47 upregulated genes and two downregulated genes), apoptosis—multiple species (20 upregulated genes), and TNF signaling pathway (30 upregulated genes and seven downregulated genes). Furthermore, almost all of these immune-related pathways were activated after DIV1 infection, except of complement and coagulation cascades.
Identification of SSR in the Transcriptome
To date, only a few SSR markers are available for M. japonicus. In order to diagnose the disease caused by DIV1 and to lay the foundation for the following genetic breeding studies, SSRs were identified in the M. japonicus hemocyte transcriptome. As shown in Figure 9, a total of 20,342 SSRs were obtained, including 8,825 mono-nucleotide repeats, 11,806 di-nucleotide repeats, 5,269 tri-nucleotide repeats, 1,433 quad-nucleotide repeats, 403 penta-nucleotide repeats, and 805 hexa-nucleotide repeats. Among the di-nucleotide repeats, AT/AT (5,117, 18.75%) was the most dominant motif, and the tri-nucleotide repeats was AAT/ATT (2,402, 8.80%) (Figure 9).
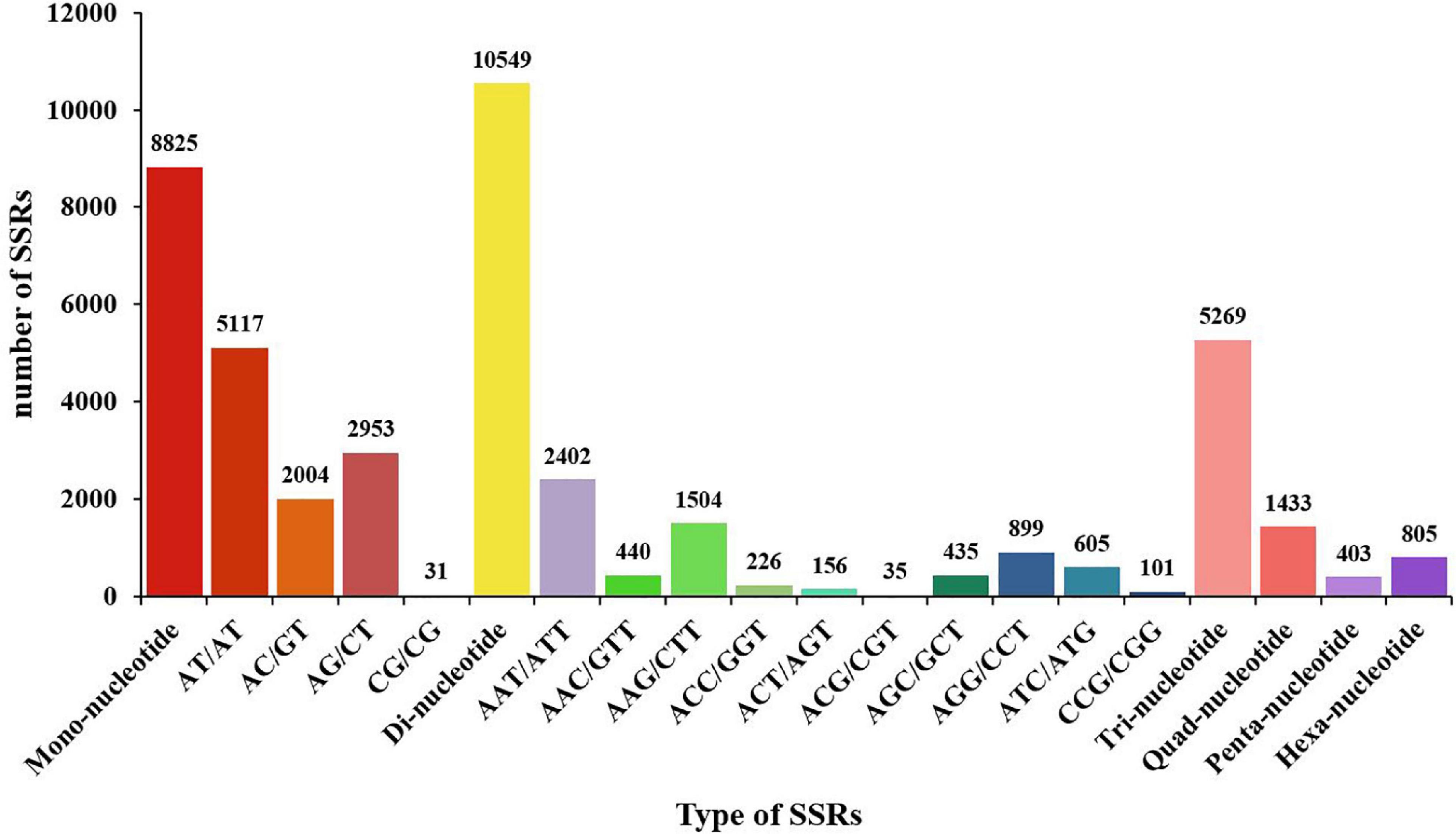
Figure 9. Distribution of SSR nucleotide classes among different nucleotide types found in the transcriptome of Marsupenaeus japonicus. The X-axis indicates the type of SSRs. The Y-axis indicates the number of SSRs.
Results of qRT-PCR
To validate the sequencing results, five upregulated genes and seven downregulated genes were chosen for the qRT-PCR analysis, including trypsin (TPS), C-type lectin 2 (CTL2), E3 ubiquitin-protein ligase RNF152-like (RNF152), HSP70, prophenoloxidase (proPO), crustin-like peptide type 4 (Crus4), copper/zinc superoxide dismutase isoform 5 (Cu/Zn-SODi5), PPAE2, C-type lysozyme (CLYZ), STAT, Dorsal, and Relish. These genes are all related to immunity. As shown in Figure 10, the expression patterns of these tested genes were consistent when the two different methods were used. The result proved that the gene expression profiles derived from RNA-seq were reliable and confirmed the expression changes of these genes in response to DIV1 infection. The transcriptome and qRT-PCR results showed that, in response to DIV1 infection, the activation of the Toll and IMD signaling pathways results in the upregulated activities of the transcription factors Dorsal and Relish. On the contrary, the expression of STAT was downregulated under DIV1 infection, which affected the role of the JAK-STAT signaling pathway in resisting viral infection (Figure 10).
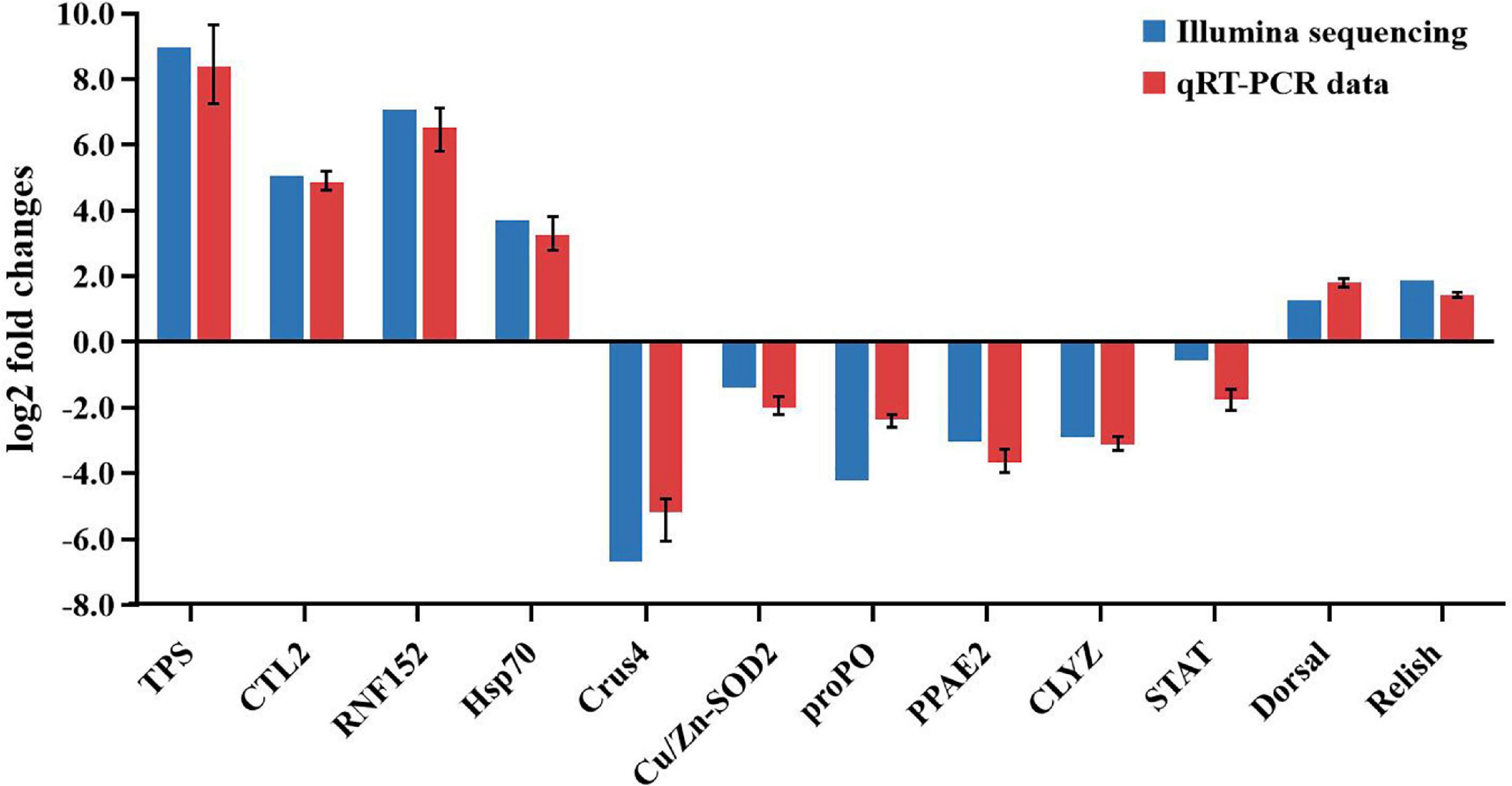
Figure 10. Comparison of the expression profiles of 12 selected genes as determined by Illumina sequencing and qRT-PCR.
Discussion
As a new type of virus, DIV1 has brought a huge challenge to the shrimp farming industry because of its lethal and virulent. Up to now, it has been found that DIV1 can be successfully infected in many species of shrimp, and transcriptome analysis has been performed on L. vannamei, P. monodon, F. merguiensis, and C. quadricarinatus infected with DIV1 (Liao X. et al., 2020; Liao X. Z. et al., 2020; Yang H. et al., 2020; He et al., 2021). These studies had found from the transcriptome level that DIV1 infection can cause a strong immune response in shrimps and found that some immune pathways and genes play an important role in the process of resisting DIV1 infection. M. japonicus is one of the most widely distributed marine shrimp species. However, there are no detailed reports and transcriptome studies on the DIV1 infection of M. japonicus. In this study, we proved that M. japonicus is the host of DIV1 by artificial injection and measured the toxicity of DIV1 to M. japonicus by the LC50 test. Enzyme activity test can reflect the impact of DIV1 infection on the immunity of M. japonicus, and the hemocyte transcriptome analysis of M. japonicus infected with DIV1 was helping to better understand the hemocyte immune mechanism of M. japonicus against DIV1 infection.
Through the method of artificial injection of DIV1, it was determined that M. japonicus could be a host of DIV1. The DIV1-infected M. japonicus showed obvious symptoms of empty stomach and intestine, yellow hepatopancreas, red body, and soft shell. In previous studies, it was also found that, after infection with DIV1, all L. vannamei, M. rosenbergii, E. carinicauda, and P. monodon had symptoms of empty stomach and intestine, atrophy of the hepatopancreas with fading color, and soft shell (Chen X. et al., 2019; Qiu et al., 2019; Liao X. et al., 2020; He et al., 2021). These symptoms can be considered as the basic symptoms of shrimp infection with DIV1. In addition, different species of shrimps infected with DIV1 seem to have some different characteristics. Some of the DIV1-infected L. vannamei and P. monodon showed a black edge of the abdominal shell (Liao X. et al., 2020; He et al., 2021), while some of the DIV1-infected M. rosenbergii were accompanied by slightly whitish muscle and mutilate antenna (Qiu et al., 2019). All of the DIV1-infected P. monodon body color turned black while all of the DIV1-infected M. japonicus body color turned red (He et al., 2021). All of the DIV1-infected M. rosenbergii had a distinct white triangle area under the carapace at the base of the frontal angle, while the hepatopancreas tissue at the base of the rostrum of all the DIV1-infected E. carinicauda had a slight cloudy white shade (Chen X. et al., 2019; Qiu et al., 2019). Not surprisingly, the LC50 results of DIV1 in M. japonicus was different from that in L. vannamei and P. monodon (Liao X. et al., 2020; He et al., 2021). This difference is caused by different species, individual size, and the quality of the aquaculture water. These comprehensive symptoms will help us to initially judge whether DIV1 infection has occurred during shrimp farming, and the LC50 test results provided reference for constructing a M. japonicus-DIV1 infection model.
The results of the enzyme activity test found that the activities of the four immune enzymes were generally inhibited after DIV1 infection at 24 h. Oxidative burst is an important immune mechanism in shrimp. The shrimp hemocytes will produce a large amount of ROS during phagocytosis, which can effectively eliminate foreign harmful substances and pathogens, such as invading bacteria and viruses (Xian et al., 2017). However, excessive ROS can cause oxidative damage to the organism. SOD and CAT can effectively remove ROS. Under the action of SOD, ROS react with hydrogen ions to generate hydrogen peroxide and then react with hydrogen ions under the action of CAT to finally produce harmless water. After M. japonicus was infected with DIV1, the activities of SOD (p < 0.001) and CAT (p < 0.05) in the plasma were decreased significantly. It meant that the ability of M. japonicus to produce and remove ROS was unbalanced, and the excess ROS caused damage to the tissues and organs of the shrimp. The activities of LYZ and PO can directly reflect the strength of the immunity of the host. LYZ is widely distributed in the animal kingdom. It catalyzes the hydrolysis of bacterial cell walls and acts as a non-specific innate immunity factor against the invasion of bacterial pathogens (Jollès and Jollès, 1984). Some recent studies found that LYZ also shows antiviral activity against WSSV and IHHNV in shrimp (Mai and Wang, 2010; Cai et al., 2019). The melanization pathway activated by the proPO system is a principal innate immune response in shrimp (Amparyup et al., 2013; Charoensapsri et al., 2014). Upon pathogen invasion, proPO is activated by the proteolytic cleavage into the active PO, which leads to the initiation of melanin formation (Tassanakajon et al., 2017). In our study, DIV1 infection caused a significant decrease in LYZ (p < 0.001) and PO activity (p < 0.01), which meant that DIV1 inhibit the immune function of the host to a certain extent.
Through the RNA sequencing platform, a total of 52,287 unigenes were de novo assembled, and 20,342 SSR markers associated with these unigenes were obtained. Through a comparative transcriptomic analysis, 6,900 DEGs (including 3,882 upregulated genes and 3,018 downregulated genes) were screened in the DIV1-infected group and the negative control group, with a cut-off q-value <0.05 and |log2 (fold change)| > 1. These included a variety of immune enzyme-related genes, such as PPAE2, proPO, Cu/Zn-SODi5, and CLYZ. It was worth noting that the expression of all these four immune enzyme-related DEGs decreased significantly after DIV1 infection, which had the same trend as the qRT-PCR analysis and the enzyme activity test. These three different methods all proved that DIV1 infection suppressed the immune ability of M. japonicus. In order to validate the sequencing results, in addition to the four genes mentioned above, another five DEGs were selected to perform qRT-PCR, including TPS, CTL2, RNF152, HSP70, and Crus4. The results showed that the gene expression profiles derived from RNA-seq were reliable. HSP70 had been found to take part in the innate immune response of L. vannamei against WSSV and IHHNV (Valentim-Neto et al., 2014; Janewanthanakul et al., 2019). In one of our previous studies, HSP70 in P. monodon was significantly upregulated under DIV1 challenges (He et al., 2021). Similar to the previous study, HSP70 in M. japonicus was also significantly upregulated after DIV1 infection, which meant that HSP70 plays an important role in anti-viral infection. Crustin is an antimicrobial peptide (AMP) that plays a key role in the innate immunity of crustaceans (Li M. et al., 2019). In present study, DIV1 infection caused a significant downregulation of Crus4 in M. japonicus. This result was the same with that of our previous study in the sense that DIV1 infection can inhibit the expression level of Crus4 and Crus1 in P. monodon (He et al., 2021). These results suggest that DIV1 can achieve immune escape by suppressing the expression of Crustin.
To further understand the response of the hemocytes under DIV1 infection, all the DEGs were mapped to the terms in the GO and KEGG databases. The results showed that some GO terms related to virus invasion, replication, and host antiviral infection were promoted under DIV1 infection, such as carbohydrate binding, chitin binding, chitin metabolic process, and DNA replication initiation. Lectins are a kind of carbohydrate-binding proteins, which play roles in various biological processes, including immune response, cell adhesion, and glycoprotein metabolism (Wang et al., 2020). In the DIV1-infected L. vannamei, F. merguiensis, and C. quadricarinatus, the expression of C-type lectin was significantly upregulated (Yang H. et al., 2020; Liao X. et al., 2020). This phenomenon was also found in our study; some C-type lectins such as CTL1 and CTL2 were significantly upregulated after DIV1 infection. Several studies had shown that shrimp C-type lectin can bind to several structural proteins of WSSV, thereby inhibiting WSSV gene expression or reducing host mortality caused by the WSSV infection (Zhao et al., 2009; Xu et al., 2013; Kwankaew et al., 2017; Phanthipha et al., 2018). However, some shrimp C-type lectins had apparently opposite functions during viral infection. It had been found that WSSV and yellow head virus (YHV) can hijack the shrimp C-type lectin for successful adhesion and entry into the host cell (Junkunlo et al., 2012; Wang et al., 2014; He et al., 2015). These different research results reflect the functional diversity and complexity of shrimp C-type lectins. The roles of these C-type lectins in the process of DIV1 infection in M. japonicus needs further study. Previous studies showed that chitin-binding proteins can facilitate WSSV gene expression and genome replication (Li et al., 2015; Yang et al., 2018; Yang F. et al., 2020; Chen et al., 2021). Here the GO terms related to chitin and DNA replication were upregulated after DIV1 infection, including chitin binding, chitin metabolic process, and DNA replication initiation. This implied that the protein containing the chitin-binding domain was involved in the invasion and replication process of DIV1.
Among the top 20 KEGG pathway enrichments influenced by DIV1 infection, half of them were found to be immune-related pathways, including the Toll and IMD signaling pathway, IL-17 signaling pathway, C-type lectin receptor signaling pathway, complement and coagulation cascades, antigen processing and presentation, necroptosis, apoptosis, NOD-like receptor signaling pathway, apoptosis—multiple species, and TNF signaling pathway. It was worth noting that almost all of these immune-related pathways were promoted after DIV1 infection, except for complement and coagulation cascades. The results reflect the fierce immune response of hemocytes during DIV1 attack. Similar to the results of the GO enrichment, the enrichment of C-type lectin receptor signaling pathway also meant that C-type lectins play a very important role in DIV1 infection. Through the analysis of the immune-related pathways, it was found that caspase-related genes were related to a variety of immune-related pathways, such as the Toll and IMD signaling pathway, IL-17 signaling pathway, C-type lectin receptor signaling pathway, necroptosis, apoptosis, NOD-like receptor signaling pathway, apoptosis—multiple species, and TNF signaling pathway. Apoptosis is characterized by a number of characteristic morphological changes in the structure of the cell (Hengartner, 2000), and caspase (cysteinyl aspartate specific proteinase) is an important protein family for the procedure of apoptosis (Fan et al., 2010). Caspase-induced apoptosis shows two sides in the process of shrimp virus infection. On the one hand, some caspase-induced shrimp cell apoptosis is a protective antiviral response—for example, the upregulated caspase 1 and caspase 3 can enhance the apoptotic activity and are involved in the innate immunity of shrimp to inhibit the WSSV replication (Chen Y. et al., 2019; Yang et al., 2019). On the other hand, the caspase-induced kakoapoptosis under viral infection can lead to massive apoptosis and finally cause the death of the infected shrimp (Flegel and Sritunyalucksana, 2011)—for example, after shrimp were infected with WSSV or YHV, the number of apoptotic cells increased with the degree of infection, and vital tissues such as the hemolymph, gills, heart, and lymphoid organs had been severely compromised (Sahtout et al., 2001; Khanobdee et al., 2002). Several recent studies found that caspase and caspase 4 of F. merguiensis, caspase 2 of L. vannamei and caspase 1 of P. monodon were all up-regulated in the hemocytes after DIV1 infection (Liao X. et al., 2020; Liao X. Z. et al., 2020; He et al., 2021). This similar situation also occurred in the present study, that is, all caspase-related genes were significantly upregulated after M. japonicus was infected with DIV1 (Table 3). It was especially worth noting that DEGs were significantly enriched in necroptosis after DIV1 infection. Necroptosis and apoptosis are two processes of cell death (Bertheloot et al., 2021). Differently from apoptosis, necroptosis is generally characterized to be the uncontrolled death of the cell, usually following a severe insult, resulting in spillage of the contents of the cell into surrounding tissues and subsequent damage thereof (D’Arcy, 2019). These results indicated that DIV1 has great toxicity and damage to host cells, and apoptosis and necroptosis may be the ultimate causes of shrimp death. The JAK-STAT, Toll, and IMD signaling pathways are considered the main pathways to regulate the innate immune response in shrimp (Li and Xiang, 2013; Li M. et al., 2019). STAT is one of the main cellular components in the JAK-STAT signaling pathway, Dorsal is the critical transcription factor in the Toll signaling pathway, and Relish plays a key role in the IMD signaling pathway (Li et al., 2009; Agaisse and Perrimon, 2010; Huang et al., 2016). WSSV infection can activate STAT in shrimp, although it is still controversial whether this activation promotes or inhibits WSSV infection (Chen et al., 2008; Sun et al., 2011; Ren et al., 2015). In the present study, DIV1 infection significantly inhibited the expression of STAT. The effect of DIV1 infection on the JAK-STAT signaling pathway of M. japonicus needs further study. A previous review concluded that WSSV infection activates the NF-κB-related signaling pathways (Toll and IMD signaling pathway), which stimulates the transcription factors including Dorsal and Relish, resulting in the expression of several sets of effectors such as AMPs (Li et al., 2018). In this study, the expression of Dorsal and Relish was significantly upregulated after DIV1 infection. It meant that the Toll and IMD signaling pathways play key roles in M. japonicus immune response to DIV1. A meta-analysis of the four reported shrimp hemocyte transcriptomes may help to further reveal the potential therapeutic targets of DIV1.
Conclusion
In conclusion, enzyme activity study and transcriptomics analysis showed that DIV1 had an inhibitory effect on the immune enzyme activity of shrimp. The immune-related signaling pathways in hemocytes were significantly activated under DIV1 challenge, and HSP70, C-type lectins, and caspase may play an important role against DIV1 infection.
Data Availability Statement
The datasets presented in this study can be found in online repositories. The names of the repository/repositories and accession number(s) can be found below: https://www.ncbi.nlm.nih.gov/, PRJNA720475.
Ethics Statement
The study protocol was approved by the Ethics Review Board of the Institutional Animal Care and Use Committee in Guangdong Ocean University. Written informed consent was obtained from the owners for the participation of their animals in this study.
Author Contributions
ZH, CS, and SZ contributed to conception and design of the study. ZH, JcZ, XC, ML, YX, JnZ, HC, and GC collected the samples and performed the experiments. ZH organized the database and wrote the first draft of the manuscript. SZ performed the writing – review and editing. CS contributed to the project administration and funding acquisition. All authors contributed to manuscript revision, read, and approved the submitted version.
Funding
This research was funded by the Key Research and Development Projects in Guangdong Province (Grant No. 2020B0202010009), the project of 2019 Annual Guangdong Provincial Special Financial Fund (Grant Nos. 231419025 and 231619003), and the Fangchenggang Science and Technology Plan Project (Grant No. AD19008017).
Conflict of Interest
GC was employed by company Haimao Seed Technology Group Co., Ltd.
The remaining authors declare that the research was conducted in the absence of any commercial or financial relationships that could be construed as a potential conflict of interest.
Publisher’s Note
All claims expressed in this article are solely those of the authors and do not necessarily represent those of their affiliated organizations, or those of the publisher, the editors and the reviewers. Any product that may be evaluated in this article, or claim that may be made by its manufacturer, is not guaranteed or endorsed by the publisher.
Footnotes
- ^ http://www.genome.jp/kegg
- ^ http://geneontology.org
- ^ ftp://ftp.ncbi.nlm.nih.gov/blast/db
- ^ http://www.uniprot.org/
- ^ https://www.ncbi.nlm.nih.gov/COG/
- ^ http://pgrc.ipk-gatersleben.de/misa/
- ^ https://www.ncbi.nlm.nih.gov/sra
References
Agaisse, H., and Perrimon, N. (2010). The roles of JAK/STAT signaling in Drosophila immune responses. Immunol. Rev. 198, 72–82. doi: 10.1111/j.0105-2896.2004.0133.x
Amparyup, P., Charoensapsri, W., and Tassanakajon, A. (2013). Prophenoloxidase system and its role in shrimp immune responses against major pathogens. Fish Shellfish Immunol. 34, 990–1001. doi: 10.1016/j.fsi.2012.08.019
Andriantahina, F., Liu, X., Huang, H., and Liu, Z. (2013). Genetic map construction and quantitative trait locus (QTL) detection of growth-related traits in Litopenaeus vannamei for selective breeding applications. PLoS One 8:e75206. doi: 10.1371/journal.pone.0075206
Arockiaraj, J., Easwvaran, S., Vanaraja, P., Singh, A., Othman, R. Y., and Bhassu, S. (2012). Molecular cloning, characterization and gene expression of an antioxidant enzyme catalase (MrCat) from Macrobrachium rosenbergii. Fish Shellfish Immunol. 32, 670–682. doi: 10.1016/j.fsi.2012.01.013
Bertheloot, D., Latz, E., and Franklin, B. S. (2021). Necroptosis, pyroptosis and apoptosis: an intricate game of cell death. Cell. Mol. Immunol. 18, 1106–1121. doi: 10.1038/s41423-020-00630-3
Bliss, C. I. (1939). The toxicity of poisons applied jointly1. Ann. Appl. Biol. 26, 585–615. doi: 10.1111/j.1744-7348.1939.tb06990.x
Bolger, A. M., Marc, L., and Bjoern, U. (2014). Trimmomatic: a flexible trimmer for Illumina sequence data. Bioinformatics 30, 2114–2120. doi: 10.1093/bioinformatics/btu170
Cai, S., Zhang, Y., Wu, F., Wu, R., Yang, S., Li, Y., et al. (2019). Identification and functional characterization of a c-type lysozyme from Fenneropenaeus penicillatus. Fish Shellfish Immunol. 88, 161–169. doi: 10.1016/j.fsi.2019.02.043
Cerenius, L., and Söderhäll, K. (2004). The prophenoloxidase-activating system in invertebrates. Immunol. Rev. 198, 116–126. doi: 10.1111/j.0105-2896.2004.00116.x
Cerenius, L., Jiravanichpaisal, P., Liu, H. P., and Soderhall, I. (2010). Crustacean Immunity. Adv. Exp. Med. Biol. 708, 239–259. doi: 10.1007/978-1-4419-8059-5_13
Charoensapsri, W., Amparyup, P., Suriyachan, C., and Tassanakajon, A. (2014). Melanization reaction products of shrimp display antimicrobial properties against their major bacterial and fungal pathogens. Dev. Comp. Immunol. 47, 150–159. doi: 10.1016/j.dci.2014.07.010
Chen, W. Y., Ho, K. C., Leu, J. H., Liu, K. F., Wang, H. C., Kou, G. H., et al. (2008). WSSV infection activates STAT in shrimp. Dev. Comp. Immunol. 32, 1142–1150. doi: 10.1016/j.dci.2008.03.003
Chen, X., Qiu, L., Wang, H., Zou, P., Dong, X., Li, F., et al. (2019). Susceptibility of Exopalaemon carinicauda to the Infection with shrimp hemocyte iridescent virus (SHIV 20141215), a strain of decapod iridescent virus 1 (DIV1). Viruses 11:387. doi: 10.3390/v11040387
Chen, Y., Han, K., Huang, X., Zhang, Z., Wan, X., and Ren, Q. (2019). Caspase-3C gene from red swamp crayfish, Procambarus clarki : characterization and expression in response to pathogenic infection. Fish Shellfish Immunol. 94, 792–799. doi: 10.1016/j.fsi.2019.10.004
Chen, Y., Kumar, R., Liu, C., and Wang, H. (2021). In Litopenaeus vannamei, the cuticular chitin-binding proteins LvDD9A and LvDD9B retard AHPND pathogenesis but facilitate WSSV infection. Dev. Comp. Immunol. 120:103999. doi: 10.1016/j.dci.2021.103999
Chen, Y., Li, C., and Huang, X. (2015). Effects of microcystin on activities of immune enzymes in the white shrimp Litopenaeus vannamei. J. Guangdong Ocean Univ. 26, 702–710. doi: 10.1007/s10646-017-1802-7
Chinchar, V. G., Hick, P., Ince, I. A., Jancovich, J. K., Marschang, R., Qin, Q., et al. (2017). ICTV virus taxonomy profile: iridoviridae. J. Gen. Virol. 98, 890–891.
Chinchar, V. G., Yu, K. H., and Jancovich, J. K. (2011). The molecular biology of frog virus 3 and other iridoviruses infecting cold-blooded vertebrates. Viruses 3, 1959–1985. doi: 10.3390/v3101959
Dall, W., Hill, B. J., Rothlisberg, P. C., and Sharples, D. J. (1990). The biology of the penaeidae. J. Crustac. Biol. 27, 489.
D’Arcy, M. S. (2019). Cell death: a review of the major forms of apoptosis, necrosis and autophagy. Cell Biol. Int. 43, 582–592. doi: 10.1002/cbin.11137
Duan, Y., Xiong, D., Wang, Y., Li, H., and Zhang, J. (2020). Toxic effects of ammonia and thermal stress on the intestinal microbiota and transcriptomic and metabolomic responses of Litopenaeus vannamei. Sci. Total Environ. 754:141867. doi: 10.1016/j.scitotenv.2020.141867
Fan, T. J., Han, L. H., Cong, R. S., and Liang, J. (2010). Caspase family proteases and apoptosis. Acta Biochim. Biophys. Sin. 37, 719–727. doi: 10.1111/j.1745-7270.2005.00108.x
FAO (2020). The State of World Fisheries and Aquaculture 2020. In: Sustainability in Action. Fisheres and Aquaculture Technical Paper. Rome: FAO.
Flegel, T. W., and Sritunyalucksana, K. (2011). Shrimp molecular responses to viral pathogens. Mar. Biotechnol. 13, 587–607. doi: 10.1007/s10126-010-9287-x
Ghani, F. M., and Bhassu, S. (2019). A new insight to biomarkers related to resistance in survived-white spot syndrome virus challenged giant tiger shrimp, Penaeus monodon. PeerJ. 7:e8107. doi: 10.7717/peerj.8107
Haas, B. J., Papanicolaou, A., Yassour, M., Grabherr, M., Blood, P. D., Bowden, J., et al. (2013). De novo transcript sequence reconstruction from RNA-seq using the trinity platform for reference generation and analysis. Nat. Protoc. 8, 1494–1512. doi: 10.1038/nprot.2013.084
He, S., Song, L., Qian, Z., Hou, F., Liu, Y., Wang, X., et al. (2015). Molecular characterization of LvAV in response to white spot syndrome virus infection in the Pacific white shrimp (Litopenaeus vannamei). Dev. Comp. Immunol. 51, 48–55. doi: 10.1016/j.dci.2015.02.020
He, Z., Chen, X., Zhao, J., Hou, D., Fu, Z., Zhong, Y., et al. (2021). Establishment of infection mode and Penaeus monodon hemocytes transcriptomics analysis under decapod iridescent virus 1 (DIV1) challenge. Aquaculture 542:736816. doi: 10.1016/j.aquaculture.2021.736816
Holmblad, T., and Söderhäll, K. (1999). Cell adhesion molecules and antioxidative enzymes in a crustacean, possible role in immunity. Aquaculture 172, 111–123. doi: 10.1016/s0044-8486(98)00446-3
Huang, C. W., Chu, P. Y., Wu, Y. F., Chan, W. R., and Wang, Y. H. (2020). Identification of functional SSR markers in freshwater ornamental shrimps neocaridina denticulata using transcriptome sequencing. Mar. Biotechnol. 22, 772–785. doi: 10.1007/s10126-020-09979-y
Huang, X., Wang, W., and Ren, Q. (2016). Dorsal transcription factor is involved in regulating expression of crustin genes during white spot syndrome virus infection. Dev. Comp. Immunol. 63, 18–26. doi: 10.1016/j.dci.2016.05.006
İnce, Ý, Özcan, O., Ilter-Akulke, A., Scully, E., and Özgen, A. (2018). Invertebrate iridoviruses: a glance over the last decade. Viruses 10:161. doi: 10.3390/v10040161
The International Committee on Taxonomy of Viruses (ICTV) (2019). One New Genus with One New Species in the Subfamily Betairidovirinae. Available online at: https://talk.ictvonline.org/files/ictv_official_taxonomy_updates_since_the_8th_report/m/animal-dna-viruses-and-retroviruses/8051 (accessed December 28, 2019).
Janewanthanakul, S., Supungul, P., Tang, S., and Tassanakajon, A. (2019). Heat shock protein 70 from Litopenaeus vannamei (LvHSP70) is involved in the innate immune response against white spot syndrome virus (WSSV) infection. Dev. Comp. Immunol. 102:103476. doi: 10.1016/j.dci.2019.103476
Jiao, L., Dai, T., Zhong, S., Jin, M., Sun, P., and Zhou, Q. (2021). Vibrio parahaemolyticus infection influenced trace element homeostasis, impaired antioxidant function, and induced inflammation response in Litopenaeus vannamei. Biol. Trace Elem. Res. 199, 329–337. doi: 10.1007/s12011-020-02120-z
Jin, Q., and Zhu, F. (2019). Differentially expressed genes of the shrimp Marsupenaeus japonicus in response to infection by white spot syndrome virus or Vibrio alginolyticus. Fish Shellfish Immunol. 92, 348–355. doi: 10.1016/j.fsi.2019.06.034
Jiravanichpaisal, P., Lee, B. L., and Söderhäll, K. (2006). Cell-mediated immunity in arthropods: hematopoiesis, coagulation, melanization and opsonization. Immunobiology 211, 213–236. doi: 10.1016/j.imbio.2005.10.015
Jollès, P., and Jollès, J. (1984). What’s new in lysozyme research? Always a model system, today as yesterday. Mol. Cell. Biochem. 63:165.
Junkunlo, K., Prachumwat, A., Tangprasittipap, A., Senapin, S., Borwornpinyo, S., Flegel, T. W., et al. (2012). A novel lectin domain-containing protein (LvCTLD) associated with response of the whiteleg shrimp Penaeus (Litopenaeus) vannamei to yellow head virus (YHV). Dev. Comp. Immunol. 37, 334–341. doi: 10.1016/j.dci.2011.12.010
Kaizu, A., Fagutao, F. F., Kondo, H., Aoki, T., and Hirono, I. (2011). Functional analysis of C-type lysozyme in penaeid shrimp. J. Biol. Chem. 286, 44344–44349. doi: 10.1074/jbc.m111.292672
Khanobdee, K., Soowannayan, C., Flegel, T. W., Ubol, S., and Withyachumnarnkul, B. (2002). Evidence for apoptosis correlated with mortality in the giant black tiger shrimp Penaeus monodon infected with yellow head virus. Dis. Aquat. Organ. 48, 79–90. doi: 10.3354/dao048079
Kwankaew, P., Praparatana, R., Runsaeng, P., and Utarabhand, P. (2017). An alternative function of C-type lectin comprising low-density lipoprotein receptor domain from Fenneropenaeus merguiensis to act as a binding receptor for viral protein and vitellogenin. Fish Shellfish Immunol. 74, 295–308. doi: 10.1016/j.fsi.2017.12.044
Li, C., Wang, S., and He, J. (2019). The two NF-κB pathways regulating bacterial and WSSV infection of shrimp. Front. Immunol. 10:1785. doi: 10.3389/fimmu.2019.01785
Li, C., Weng, S., and He, J. (2018). WSSV-host interaction: host response and immune evasion. Fish Shellfish Immunol. 84, 558–571. doi: 10.1016/j.fsi.2018.10.043
Li, F., and Xiang, J. (2013). Recent advances in researches on the innate immunity of shrimp in China. Dev. Comp. Immunol. 39, 11–26. doi: 10.1016/j.dci.2012.03.016
Li, F., Hui, Y., Wang, D., Priya, T., Li, S., Wang, B., et al. (2009). Identification of a novel relish homolog in Chinese shrimp Fenneropenaeus chinensis and its function in regulating the transcription of antimicrobial peptides. Dev. Comp. Immunol. 33, 1093–1101. doi: 10.1016/j.dci.2009.06.001
Li, M., Ma, C., Zhu, P., Yang, Y., and Li, C. (2019). A new crustin is involved in the innate immune response of shrimp Litopenaeus vannamei. Fish Shellfish Immunol. 94, 394–406.
Li, S., Zhang, X., Sun, Z., Li, F., and Xiang, J. (2013). Transcriptome analysis on chinese shrimp Fenneropenaeus chinensis during WSSV acute infection. PLoS One 8:e58627. doi: 10.1371/journal.pone.0058627
Li, Z., Fang, L., Han, Y., Xu, L., and Feng, Y. (2015). VP24 Is a chitin-binding protein involved in white spot syndrome virus infection. J. Virol. 90, 842–850. doi: 10.1128/jvi.02357-15
Liao, X. Z., Hu, S. K., Wang, B., Qin, H. P., Zhao, J. C., He, Z. H., et al. (2019). Dietary supplementation with polypeptides improved growth performance, antibacterial immune and intestinal microbiota structure of Litopenaeus vannamei. Fish Shellfish Immunol. 92, 480–488. doi: 10.1016/j.fsi.2019.06.033
Liao, X. Z., Wang, C. G., Wang, B., Qin, H. P., Hu, S. K., Zhao, J. C., et al. (2020). Research into the hemocyte immune response of Fenneropenaeus merguiensis under decapod iridescent virus 1 (DIV1) challenge using transcriptome analysis. Fish Shellfish Immunol. 104, 8–17. doi: 10.1016/j.fsi.2020.05.053
Liao, X., Wang, C., Wang, B., Qin, H., and Zhang, S. (2020). Comparative transcriptome analysis of Litopenaeus vannamei reveals that triosephosphate isomerase-like genes play an important role during decapod iridescent virus 1 infection. Front. Immunol. 11:1904. doi: 10.3389/fimmu.2020.01904
Livak, K. J., and Schmittgen, T. D. (2001). Analysis of relative gene expression data using real-time quantitative PCR and the 2 ΔΔ C T method. Methods 25, 402–408. doi: 10.1006/meth.2001.1262
Love, M. I., Huber, W., and Anders, S. (2014). Moderated estimation of fold change and dispersion for RNA-seq data with DESeq2. Genome Biol. 15:550.
Mai, W. J., and Wang, W. N. (2010). Protection of blue shrimp (Litopenaeus stylirostris) against the White Spot Syndrome Virus (WSSV) when injected with shrimp lysozyme. Fish Shellfish Immunol. 28, 727–733. doi: 10.1016/j.fsi.2010.01.002
Matthews, R. (1979). Virus taxonomy: classification and nomenclature of viruses: ninth report of the international committee on taxonomy of viruses. Intervirology 12, 129–296.
Nian, Y. Y., Chen, B. K., Wang, J., Zhong, W. T., and Yan, D. C. (2019). Transcriptome analysis of Procambarus clarkii infected with infectious hypodermal and haematopoietic necrosis virus. Fish Shellfish Immunol. 98, 766–772. doi: 10.1016/j.fsi.2019.11.027
Pertea, G., Huang, X., Liang, F., Antonescu, V., Sultana, R., Karamycheva, S., et al. (2003). TIGR Gene Indices clustering tools (TGICL): a software system for fast clustering of large EST datasets. Bioinformatics 19, 651–652.
Phanthipha, R., Pattamaporn, K., and Prapaporn, U. (2018). FmLC6: an ultimate dual-CRD C-type lectin from Fenneropenaeus merguiensis mediated its roles in shrimp defense immunity towards bacteria and virus. Fish Shellfish Immunol. 80, 200–213. doi: 10.1016/j.fsi.2018.05.043
Qin, Z., Babu, V. S., Wan, Q., Zhou, M., Liang, R., Muhammad, A., et al. (2018). Transcriptome analysis of Pacific white shrimp (Litopenaeus vannamei) challenged by Vibrio parahaemolyticus reveals unique immune-related genes. Fish Shellfish Immunol. 77, 164–174. doi: 10.1016/j.fsi.2018.03.030
Qiu, L., Chen, M. M., Wan, X. Y., Li, C., Zhang, Q. L., Wang, R. Y., et al. (2017). Characterization of a new member of Iridoviridae, Shrimp hemocyte iridescent virus (SHIV), found in white leg shrimp (Litopenaeus vannamei). Sci. Rep. 7:11834.
Qiu, L., Chen, M. M., Wan, X. Y., Zhang, Q. L., and Huang, J. (2018). Detection and quantification of shrimp hemocyte iridescent virus by TaqMan probe based real-time PCR. J. Invertebr. Pathol. 154, 95–101. doi: 10.1016/j.jip.2018.04.005
Qiu, L., Chen, X., Zhao, R. H., Li, C., and Huang, J. (2019). First description of a natural infection with shrimp hemocyte iridescent virus in farmed giant freshwater prawn, Macrobrachium rosenbergii. Viruses 17:354. doi: 10.3390/v11040354
Ren, Q., Huang, Y., He, Y., Wang, W., and Zhang, X. (2015). A white spot syndrome virus microRNA promotes the virus infection by targeting the host STAT. Sci. Rep. 5:18384.
Ren, Y., Li, J., Guo, L., Liu, J. N., Wan, H., Meng, Q., et al. (2020). Full-length transcriptome and long non-coding RNA profiling of whiteleg shrimp Penaeus vannamei hemocytes in response to Spiroplasma eriocheiris infection. Fish Shellfish Immunol. 106, 876–886. doi: 10.1016/j.fsi.2020.06.057
Sahtout, A., Hassan, M., and Shariff, M. (2001). DNA fragmentation, an indicator of apoptosis, in cultured black tiger shrimp Penaeus monodon infected with white spot syndrome virus (WSSV). Dis. Aquat. Organ. 44, 155–159. doi: 10.3354/dao044155
Seppey, M., Manni, M., and Zdobnov, E. M. (2019). BUSCO: assessing genome assembly and annotation completeness. Methods Mol. Biol. 1962, 227–245. doi: 10.1007/978-1-4939-9173-0_14
Sookruksawong, S., Sun, F., Liu, Z., and Tassanakajon, A. (2013). RNA-Seq analysis reveals genes associated with resistance to Taura syndrome virus (TSV) in the Pacific white shrimp Litopenaeus vannamei. Dev. Comp. Immunol. 41, 523–533. doi: 10.1016/j.dci.2013.07.020
Sun, C., Shao, H. L., Zhang, X. W., Zhao, X. F., and Wang, J. X. (2011). Molecular cloning and expression analysis of signal transducer and activator of transcription (STAT) from the Chinese white shrimp Fenneropenaeus chinensis. Mol. Biol. Rep. 38, 5313–5319. doi: 10.1007/s11033-011-0681-x
Tassanakajon, A., Rimphanichyakit, V., Visetnan, S., Amparyup, P., and Tang, S. (2017). Shrimp humoral responses against pathogens: antimicrobial peptides and melanization. Dev. Comp. Immunol. 80, 81–93. doi: 10.1016/j.dci.2017.05.009
Thitamadee, S., Prachumwat, A., Srisala, J., Jaroenlak, P., Salachan, P. V., Sritunyalucksana, K., et al. (2016). Review of current disease threats for cultivated penaeid shrimp in Asia. Aquaculture 452, 69–87. doi: 10.1016/j.aquaculture.2015.10.028
Valentim-Neto, P. A., Moser, J. R., Fraga, A., and Marques, M. (2014). Hsp70 expression in shrimp Litopenaeus vannamei in response to IHHNV and WSSV infection. Virusdisease 25, 437–440. doi: 10.1007/s13337-014-0236-6
Wang, F., Li, S., Xiang, J., and Li, F. (2019). Transcriptome analysis reveals the activation of neuroendocrine-immune system in shrimp hemocytes at the early stage of WSSV infection. BMC Genomics 20:247. doi: 10.1186/s12864-019-5614-4
Wang, W., Wang, M., Xiao, G., Yulong, H. U., Song, W., Kong, J., et al. (2018). Using SSR marker to trace Chinese shrimp Fenneropenaeus chinensis released in natural sea—a feasible strategy for assessment of release effect in natural resources recovery program. Prog. Fish. Sci. 39, 21–26.
Wang, X. W., Vasta, G. R., and Wang, J. X. (2020). The functional relevance of shrimp C-type lectins in host-pathogen interactions. Dev. Comp. Immunol. 109, 103708. doi: 10.1016/j.dci.2020.103708
Wang, X. W., Xu, Y. H., Xu, J. D., Zhao, X. F., and Wang, J. X. (2014). Collaboration between a soluble C-type lectin and calreticulin facilitates white spot syndrome virus infection in shrimp. J. Immunol. 193, 2106–2117. doi: 10.4049/jimmunol.1400552
Wei, W., Yang, S., Wang, C., Shi, L., and Chan, S. (2017). Gill transcriptomes reveal involvement of cytoskeleton remodeling and immune defense in ammonia stress response in the banana shrimp Fenneropenaeus merguiensis. Fish Shellfish Immunol. 71, 319–328. doi: 10.1016/j.fsi.2017.10.028
Wu, W., Chen, L., Li, Y., and Zhu, C. (2014). Effects of tributyltin on the activities of immunologic enzyme in blood serum of the Macrobrachium rosenbergill. J. Guangdong Ocean Univ. 34, 17–21.
Xian, J. A., Zhang, X. X., Wang, A. L., Li, J. T., and Ye, J. M. (2017). Oxidative burst activity in haemocytes of the freshwater prawn Macrobrachium rosenbergii. Fish Shellfish Immunol. 73, 272–278. doi: 10.1016/j.fsi.2017.12.028
Xu, L., Wang, T., Fa Ng, L., and Feng, Y. (2016). Isolation and preliminary characterization of a new pathogenic iridovirus from redclaw crayfish Cherax quadricarinatus. Dis. Aquat. Organ. 120, 17–26. doi: 10.3354/dao03007
Xu, Y. H., Bi, W. J., Wang, X. W., Zhao, Y. R., and Wang, J. X. (2013). Two novel C-type lectins with a low-density lipoprotein receptor class A domain have antiviral function in the shrimp Marsupenaeus japonicus. Dev. Comp. Immunol. 42, 323–332. doi: 10.1016/j.dci.2013.10.003
Yang, F., Li, S., Li, F., and Xiang, J. (2018). A cuticle protein from the Pacific white shrimp Litopenaeus vannamei involved in WSSV infection. Dev. Comp. Immunol. 81, 303–311. doi: 10.1016/j.dci.2017.12.018
Yang, F., Li, S., Xiang, J., Zhao, X., and Li, F. (2020). Transcriptome analysis reveals the regulation of the shrimp STAT on host chitin-binding domain containing proteins and energy metabolism process during WSSV infection. Fish Shellfish Immunol. 100, 345–357. doi: 10.1016/j.fsi.2020.03.026
Yang, G., Wang, J., Luo, T., and Zhang, X. (2019). White spot syndrome virus infection activates Caspase 1-mediated cell death in crustacean. Virology 528, 37–47. doi: 10.1016/j.virol.2018.12.004
Yang, H., Wei, X., Wang, R., Zeng, L., Yang, Y., Huang, G., et al. (2020). Transcriptomics of Cherax quadricarinatus hepatopancreas during infection with decapod iridescent virus 1 (DIV1) - sciencedirect. Fish Shellfish Immunol. 98, 832–842. doi: 10.1016/j.fsi.2019.11.041
Zeng, D., Chen, X., Xie, D., Zhao, Y., Yang, C., Li, Y., et al. (2014). Transcriptome analysis of pacific white shrimp (Litopenaeus vannamei) hepatopancreas in response to taura syndrome virus (TSV) experimental infection. Fish. Sci. Technol. Guangxi. 8, 4–10.
Zhao, Z. Y., Yin, Z. X., Xu, X. P., Weng, S. P., Rao, X. Y., Dai, Z. X., et al. (2009). A novel C-type lectin from the shrimp Litopenaeus vannamei possesses anti-white spot syndrome virus activity. J. Virol. 83, 347–356. doi: 10.1128/jvi.00707-08
Zhu, C., Shi, D., Liao, S., He, P., and Jia, R. (2020). Effects of Synechococcus sp. PCC 7942 harboring vp19, vp28, and vp (19 + 28) on the survival and immune response of Litopenaeus vannamei infected WSSV. Fish Shellfish Immunol. 99, 1–8. doi: 10.1016/j.fsi.2020.01.028
Zhu, X. F., Guo, H., Li, G. L., and Zhu, C. H. (2021). Effects of dietary hydrolyzable tannins on growth performance, antioxidant capacity, intestinal microora and resistance against Vibrio parahaemolyticus of juvenile Pacic white shrimp, Litopenaeus vannamei (Boone, 1931). Aquac. Rep. 19:100601. doi: 10.1016/j.aqrep.2021.100601
Keywords: decapod iridescent virus 1, Marsupenaeus japonicus, enzyme activity, transcriptomic analysis, immunity response
Citation: He Z, Zhao J, Chen X, Liao M, Xue Y, Zhou J, Chen H, Chen G, Zhang S and Sun C (2021) The Molecular Mechanism of Hemocyte Immune Response in Marsupenaeus japonicus Infected With Decapod Iridescent Virus 1. Front. Microbiol. 12:710845. doi: 10.3389/fmicb.2021.710845
Received: 17 May 2021; Accepted: 28 July 2021;
Published: 26 August 2021.
Edited by:
Rongtuan Lin, McGill University, CanadaReviewed by:
Alphis G. Ponniah, Retired, Chennai, IndiaFei Zhu, Zhejiang Agriculture and Forestry University, China
Copyright © 2021 He, Zhao, Chen, Liao, Xue, Zhou, Chen, Chen, Zhang and Sun. This is an open-access article distributed under the terms of the Creative Commons Attribution License (CC BY). The use, distribution or reproduction in other forums is permitted, provided the original author(s) and the copyright owner(s) are credited and that the original publication in this journal is cited, in accordance with accepted academic practice. No use, distribution or reproduction is permitted which does not comply with these terms.
*Correspondence: Chengbo Sun, c3VuY2JAZ2RvdS5lZHUuY24=; Shuang Zhang, enNodWFuZ0BnZG91LmVkdS5jbg==