- 1Rajiv Gandhi Centre for Biotechnology, Trivandrum, India
- 2Centre for Doctoral Studies, Manipal Academy of Higher Education, Manipal, India
- 3Department of Gastroenterology, Government Medical College, Trivandrum, India
- 4Biotech Hub, Department of Zoology, Nar Bahadur Bhandari Degree College, Gangtok, India
- 5Department of Microbiology, Sikkim University, Gangtok, India
- 6STNM Hospital, Gangtok, India
Pathogenic potentials of the gastric pathogen, Helicobacter pylori, have been proposed, evaluated, and confirmed by many laboratories for nearly 4 decades since its serendipitous discovery in 1983 by Barry James Marshall and John Robin Warren. Helicobacter pylori is the first bacterium to be categorized as a definite carcinogen by the International Agency for Research on Cancer (IARC) of the World Health Organization (WHO). Half of the world’s population carries H. pylori, which may be responsible for severe gastric diseases like peptic ulcer and gastric cancer. These two gastric diseases take more than a million lives every year. However, the role of H. pylori as sole pathogen in gastric diseases is heavily debated and remained controversial. It is still not convincingly understood, why most (80–90%) H. pylori infected individuals remain asymptomatic, while some (10–20%) develop such severe gastric diseases. Moreover, several reports indicated that colonization of H. pylori has positive and negative associations with several other gastrointestinal (GI) and non-GI diseases. In this review, we have discussed the state of the art knowledge on “H. pylori factors” and several “other factors,” which have been claimed to have links with severe gastric and duodenal diseases. We conclude that H. pylori infection alone does not satisfy the “necessary and sufficient” condition for developing aggressive clinical outcomes. Rather, the cumulative effect of a number of factors like the virulence proteins of H. pylori, local geography and climate, genetic background and immunity of the host, gastric and intestinal microbiota, and dietary habit and history of medicine usage together determine whether the H. pylori infected person will remain asymptomatic or will develop one of the severe gastric diseases.
Introduction
Two gastric diseases, peptic ulcer (246,700 deaths/year) and gastric cancer (782,685 deaths/year), together are responsible for over a million of global deaths annually (Moraga et al., 2017; Bray et al., 2018). Both diseases are associated with the colonization of Helicobacter pylori in stomach (Table 1). Helicobacter pylori infection is also associated with a few relatively milder gastrointestinal (GI) diseases like non-ulcer dyspepsia (NUD) and gastritis, which often remains unnoticed (Table 1). Even several non-GI diseases like myocardial infarction, diabetes mellitus, and iron deficiency anemia are reported to be linked to H. pylori infection (Table 1; Ortiz et al., 2014). On the other hand, it has also been proposed that H. pylori infection is negatively associated with several upper-GI diseases like gastroesophageal reflux disease (GERD), Barrett’s esophagus, and esophageal cancer and also with some non-GI diseases like asthma (Table 1; Fallone et al., 2000; Wang et al., 2013; Rubenstein et al., 2014). Till date, it is not understood how H. pylori infection is positively and negatively associated with so many GI and non-GI diseases, but the H. pylori encoded virulence factors and their mechanisms of actions in the context of peptic ulcer and gastric cancer are well studied (Yamaoka, 2010; Malfertheiner et al., 2014). However, even for the gastroduodenal diseases, the H. pylori infection and the virulence factors of H. pylori fail to explain the inconsistencies in clinical outcomes that are observed within the H. pylori infected population. For example, nearly 4.4 billion people, which means more than half of the world’s population is infected with H. pylori and the majority of the H. pylori strains carry the virulence genes, but only a small fraction, usually 10–20%, develop the severe gastric diseases like peptic ulcer disease and gastric cancer (Dorer et al., 2009; Hooi et al., 2017). It is not known why 80–90% of the H. pylori infected population remains asymptomatic. Nonetheless, the observed discrepancies in H. pylori infections and the gastric diseases clearly suggest definite involvements of some “other factors” in determining the clinical status of the host. In this review, we discussed the state of the art knowledge regarding the “H. pylori virulence factors” and the “other factors” like host genetics, host immunity, geography, climate, GI microbiota, diet and medications, which together contribute to either the “development and progression” or the “prevention” of peptic ulcer and gastric cancer (Figure 1).
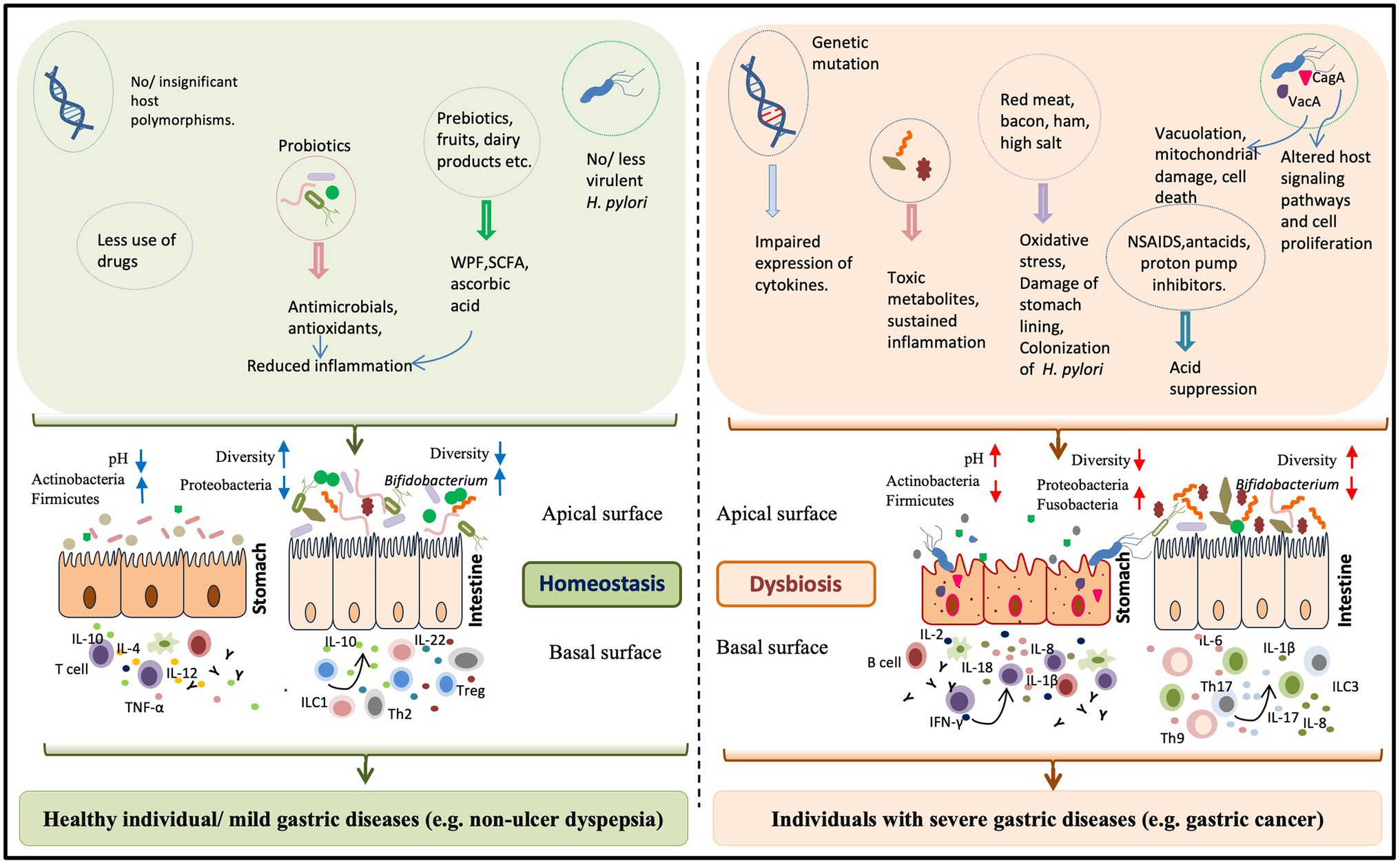
Figure 1. The impact of “H. pylori factors” and “other factors” on the gastric health and diseases. The gastric health is determined by the combination of microbial, host, and environmental factors. Normally gastric inflammation remains under control by the constant interaction between the inhabiting microbes and the host immune system. The beneficial microbes in the form of probiotics (producing antimicrobials and anti-oxidants) or in ingested ethnic fermented foods and the prebiotics play major roles in maintaining the homeostasis within the gastric milieu. For a healthy stomach, where the pH is low, the colonization is restricted to microbial phyla like Actinobacteria and Firmicutes. But, the long term colonization of H. pylori, apart from creating gastric damages due to its virulence factors like vacuolating cytotoxin A (VacA) and CagA, also leads to the loss ofgastric parietal cells and the gastric pH increases. In the altered gastric pH, several harmful bacteria (e.g., several members of the phylum Proteobacteria) become predominant. Several other factors have the potentials to disturb the gastric homeostasis. For instance, impaired expression of host immune regulators, increased consumption of red meat, and excessive use of non-steroidal anti-inflammatory drug (NSAID) and acid suppressors can enhance the pro-inflammatory response. Dysbiosis of the intestinal microbiota, particularly lower abundance of Bifidobacterium species has recently been linked to the H. pylori associated severe gastric diseases.
Helicobacter pylori Virulence
Since H. pylori is sensitive to low pH, its survival in highly acidic gastric milieu is challenging. However, the bacterium overcomes this challenge by producing a 550 kD a multimeric nickel-containing enzyme, urease, which catalyzes the hydrolysis of urea into carbonic acid and ammonia (Table 2). The released ammonia increases the pH and provides a protective local environment near H. pylori. Simultaneously, the urease can also activate monocytes and polymorphonuclear leucocytes leading to inflammatory response and epithelial damage (Mobley, 1996).
The next critical event for successful colonization of H. pylori is to establish a firm adhesion with the gastric epithelial cells, which is mediated by a group of outer membrane adhesins that interact with molecules on the host cell surface. Blood group antigen binding adhesin A (BabA) and Blood group antigen binding adhesin B (BabB) are well-characterized bacterial adhesins that interact with the host Lewis B blood group antigens (Leb; Boren et al., 1993). Helicobacter pylori strains can be classified as BabA high producers (BabA-H) with Leb binding activity, BabA low producers (BabA-L) without Leb binding activity, and BabA-negative strain (babA2-genonegative; Table 2; Fujimoto et al., 2007). Interestingly, however, infections with BabA-L strains are associated with the highest mucosal injury and have the highest risk of gastric cancer (Fujimoto et al., 2007; Chang et al., 2018). In populations where A, B and O blood groups are abundant, majority of the isolated H. pylori can bind to fucosylated blood group antigens A, B, and O (generalist). But, in the South American Amerindian population, where the O blood group is predominant, 60% of H. pylori strains binds best to the O antigen (specialist), showing that the adherence pattern is influenced by the regional abundance of A, B, and O blood groups (Aspholm-Hurtig et al., 2004). This adaptive binding is achieved by a single amino acid substitution in the Diversification Loop1(DL1) in BabA (Moonens et al., 2016). The Leb-BabA interaction is inhibited at low pH and is restored with acid neutralization. This acid responsiveness, which depends on the pH sensor sequence in BabA, differs among strains suggesting that H. pylori strains may get adapted to the distinct acid secretion pattern of each individual (Bugaytsova et al., 2017). The BabA also potentiates the activity of the H. pylori Type IV secretion system (TFSS; Ishijima et al., 2011). The H. pylori outer inflammatory protein A [OipA, also known as Helicobacter outer membrane protein (OMP) H or HopH] is an OMP that is involved in adhesion and promotes IL-8 secretion. Strains with oipA “on” status are associated with increased colonization density, neutrophil infiltration and IL-8 production. In addition, significant association has been found between the oipA “on” status and the risk of peptic ulcer and gastric cancer (Table 2; Liu et al., 2013). Other than OipA, H. pylori also possesses other OMPs like sialic acid binding adhesin (SabA, also known as Helicobacter OMP P/HopP), Helicobacter OMP Q (HopQ), Helicobacter OMP Z (HopZ), and H. pylori outer membrane (Hom) family proteins, such as HomA, HomB, HomC, and HomD. The expression of SabA correlates inversely with gastric pH and helps H. pylori adapt with the changing gastric environment. It was reported that HopQ has essential role in the translocation of CagA into host cell. Among the Hom proteins, HomB is capable of inducing IL-8 secretion (Xu et al., 2020). Adherence-associated lipoprotein A and B (AlpA/AlpB) aid H. pylori to bind with gastric epithelial cell and facilitate colonization and biofilm formation. It also induces expression of inflammatory mediators like IL-6 and IL-8. A poorly studied adhesin, LacdiNAc-specific adhesin (LabA) also mediates H. pylori adhesion to gastric epithelial cells (Baj et al., 2021). Chronic colonization of H. pylori, as evident from DNA fingerprinting studies of strains isolated from sequential biopsies, is critical in the progression of severe gastric diseases (Taylor et al., 1995). Recent study has shown that adaptability of H. pylori to the gastric environment is regulated by a small non-coding RNA HPne4160. Decreased expression of HPne4160 during chronic infection results in increased expression of OMPs and CagA (Kinoshita-Daitoku et al., 2021).
The genes that encode the TFSS and the virulence protein CagA are located within the ~40 kb cag-pathogenicity island (cagPAI). The CagA N-terminal domain also contains a binding site for the α5β1 integrin and this interaction is considered to be a prerequisite for the delivery of CagA into the gastric epithelial cells (Kaplan-Türköz et al., 2012). Once inside the cell, the phosphatidyl serine (PS) interacts with the Lys-Xn-Arg-X-Arg (K-Xn-R-X-R) motif in the N-terminal domain of CagA and anchors CagA to the inner surface of cell membrane (Takahashi-Kanemitsu et al., 2020). In this motif, the Lys and Arg (R619 and R621) residues are highly conserved and exposed for both Western (e.g., 26,695 and G27, J99) and East Asian (e.g., F75) strains. Conversely, the position 636 is highly variable and the K636N mutation is associated with severe pathology (Table 2; Ulloa-Guerrero et al., 2018). Inside the cell, the CagA gets phosphorylated and forms complexes with the Src-homology 2 (SH2) domains present in the proteins like SHP2, Grb2, and CSK and alters a number of host signaling pathways that leads to abnormal cytoskeletal changes, cell proliferation, and differentiation and induces the secretion of pro-inflammatory cytokines like IL-8 through NF-κB pathway (Papadakos et al., 2013; Hatakeyama, 2014). The key phosphorylation, which leads to these cascades of downstream events, occurs at the C-terminal domain of CagA. This domain contains a conserved phosphorylayable tyrosine residue within the motifs composed of five amino acids, Glu-Pro-Ile-Tyr-Ala (EPIYA). Based on the variation in the flanking regions and the orders of spacers, the CagA-EPIYA motifs can be classified into A, B, and either C or D types. The first EPIYA motif is called as EPIYA-A, which is followed by the EPIYA-B and then either EPIYA-C or EPIYA-D. The C segment (usually 1–3 copies) is the characteristic of Western CagA (WSS), while the D segment (usually one copy) is seen in East-Asian CagA (ESS; Higashi et al., 2002). For the WSS-CagA (EPIYA-C), the degree of phosphorylation is proportional to the copy number of EPIYA-C, which influences the strength of activation of downstream pathways. However, the ESS-CagA (EPIYA-D) has stronger affinity for SHP2, which potentiates the downstream effector pathways leading to aggressive diseases (Table 2). In addition, the level of expression of CagA is found to vary among strains. Mutations in other genes in the cagPAI can also affect the virulence. For example, H. pylori strains with Y58/E59 polymorphism in the CagL carry higher risk for inducing a gastric cancer (Table 2; Yeh et al., 2019).
The vacuolating cytotoxin A (VacA) is a pore forming secreted toxin, which is composed of N-terminal (p33) and C-terminal (p55) fragments, binds to the receptors like receptor protein tyrosine phosphatase beta (RPTP-β; Yahiro et al., 2003), epidermal growth factor receptor (EGFR), and sphingomyelin and gets internalized through a clathrin independent endocytosis mechanism (Gauthier et al., 2005). The internalized VacA produces large acidic vacuoles inside the cells (Utsch and Haas, 2016). In addition, the p33 subunit of VacA enters the mitochondria and induces cytochrome C release via Bax activation and promotes cell death (Yamasaki et al., 2006). The vacA gene encodes a precursor protein with a “signal sequence” (s), a “mid-region” (m), and a “C-terminal region.” Much later, another region between the s and m regions have been identified and named as intermediate (i) region (Table 2). The vacA gene is highly polymorphic and each region (s, i, m, c, and d) has at least two alleles; for vacAs, s1 (subdivided into s1a and s1b) and s2; for vacAi, i1 (subdivided into i1a and i1b) and i2 and for vacAm, m1 (subdivided into m1a, m1b, and m1c) and m2. The vacAs1i1m1 allelic combination is strongly linked with the presence of cagA, resulting in a highly virulent strain type (vacAs1i1m1cagA+; Sugimoto et al., 2009). Infection with strains that express both cagA and vacAs1m1 simultaneously has 4.8-fold more risk of forming gastric precancerous lesions than the individuals infected with benign H. pylori strains (e.g., vacAs2m2cagA−; Table 2; Chang et al., 2018). Apart from the diversity regions “s,” “m” and “i,” vacA possess “c” and “d” regions, but their role in the pathogenesis is still poorly understood. The c1 and d1 genotypes can be used as biomarkers as they are linked with the increased risk of gastric cancer. Also, the d1/c1 strains are associated with secreted vacuolating cytotoxin type encoding s1m1i1 and d2/c2 strains are linked with non-secreted vacuolating cytotoxin type encoding s2/m2/i2 genotypes (Thi Huyen Trang et al., 2016).
Studies from Asian countries showed that the presence of duodenal ulcer promoting gene A (dupA) is associated with increased risk of duodenal ulcer with heavy neutrophil infiltration and increased IL-8 expression in the antrum (Table 2; Yamaoka et al., 1998, 1999; Lu et al., 2005). Conversely, its presence has negative correlation with the risk of gastric atrophy, intestinal metaplasia, and gastric cancer (Hussein, 2010; Shiota et al., 2010). Similarly neutrophil activating protein (NAP) of H. pylori stimulates the infiltration of neutrophil to the gastric epithelium and leads to the production of reactive oxygen species (ROS; Baj et al., 2021).
The persistence and long term colonization of H. pylori in the gastric mucosa are facilitated by the formation of biofilm especially under stress. In order to survive the harsh environment, H. pylori adopts mechanisms including morphological transformation, membrane vesicles secretion, matrix production, efflux pump activity, and intermicrobial communication, leading to the formation of biofilms (Krzyżek et al., 2020). It also promotes the exchange of genetic material between subpopulations and enhances the recombination frequency (Hathroubi et al., 2018). A recent study suggests that a laboratory strain, H. pylori strain G27, is capable of forming biofilms on both plastic (abiotic) and gastric epithelial cells (biotic). It is also found that biofilm formation is associated with the enhanced expression of multiple genes associated with flagella formation, hydrogenase activity, and acetone metabolism (Hathroubi et al., 2020). Various studies report that the biofilm formation can enhance antibiotic resistance (Fauzia et al., 2020) especially to clarithromycin (Hathroubi et al., 2018). Further, transformation from active spiral form to coccoid form under unfavorable conditions is observed in H. pylori. Persistence of non-replicable, non-culturable, less active coccoid form can cause serious damage to the gastric mucosa and is usually resistant to antibiotics (Krzyżek and Grande, 2020) and is a major challenge in H. pylori eradication, (Ierardi et al., 2020).
Although, a number of virulence factors of H. pylori were identified and their potential to contribute to the formation of gastric diseases have been proved by in vitro and in vivo methods, colonization by H. pylori strains carrying virulence genes does not ensure aggressive clinical outcomes, particularly in some geographical regions (Chattopadhyay et al., 2002; Malfertheiner et al., 2014).
Geography and Distinctiveness of Different H. Pylori Strains
The prevalence of H. pylori infection and associated gastric diseases vary widely with geography. In general, the prevalence of H. pylori infection is higher in developing countries and in resource poor settings than the developed countries. For example, the prevalence of H. pylori infection in Africa (79.1%), Asia (54.7%), and Latin America and Caribbean region (63.4%) are remarkably higher as compared to the prevalence in North America (37.1%) and Oceania (24.4%; Hooi et al., 2017). Interestingly, however, the indigenous populations of the developed countries like United States and Australia have relatively higher prevalence of H. pylori infection (Hooi et al., 2017). The geographic disparity of the prevalence of H. pylori infection could be due to the sanitation, urban vs. rural lifestyle, socioeconomic status, variability of the H. pylori strains, and antibiotic usage in the community. More intriguingly, the prevalence of gastric cancer, gastric ulcer, and duodenal ulcer do not always correlated to the prevalence of H. pylori infection. For instance, although the prevalence of H. pylori infection (80%) is very high in Africa, the incidence of gastric cancer Africa is lower and is estimated to be 4/100,000 population (Asombang et al., 2014; Smith et al., 2019). This phenomenon is known as the “African enigma” (Agha and Graham, 2005). In contrast, while the seroprevalence of H. pylori among Chinese and Japanese adults are 44 and 55%, respectively, the annual incidence of gastric cancers are really high (32–59 per 100,000 populations in China and 80–115 per 100,000 populations in Japan; Singh and Ghoshal, 2006).
The evidence of H. pylori infection has been detected in a 5,300 years old iceman mummy suggesting that H. pylori colonized human stomach since ancient time (Maixner et al., 2016). The first description of gastric ulcer was found as early as 4th century BCE as carvings on a pillar of the temple of Aesculapius at Epidaurus (Graham, 2014). Importantly, H. pylori causes long-term colonization in the human stomach, which allows the bacterium to get adapted to the particular host. Therefore, since ancient time, the bacterium has been coevolving with different human populations inhabiting different geographical regions. The present H. pylori strains isolated from distinct human populations in different geographical areas are distinct. At present, the H. pylori strains are grouped into seven distinct types: hpAfrica1, hpAfrica2, hpNEAfrica, hpEast Asia, hpAsia, hpEurope, and hpSahul (Linz et al., 2007; Lamichhane et al., 2019). The hpEurope is considered as an ancient hybrid of hpNEAfrica and hpAsia, while the hspAmerind is possibly a subpopulation of the hspEAsia (Thorell et al., 2017). However, the hspAmerind strains are now rare even within the groups having substantial Native American ancestry possibly due to the constant evolutionary pressure imposed by other competing strains (Thorell et al., 2017).
Taken together, the lineages of H. pylori strains are not uniformly dispersed in the world (van Doorn et al., 1999b). Like the variations in the H. pylori housekeeping genes, the virulence genes also vary significantly with geography. Earlier, it was noticed that the cagA1 variant is found exclusively in strains from Europe, United States, and Australia, whereas another variant, the cagA2, is found in East Asian countries (van der Ende et al., 1998). It has been found that the hpAfrica2 type strains tend to lack cagPAI, while the 87.5% of strains from China is reported as cagA positive with higher frequency of vacAi1 (85.2%) and vacAm1 (53.6%; Duncan et al., 2013; Burucoa and Axon, 2017). Moreover, the East-Asian H. pylori strains carry the “D”-type segment in the C-terminal region of the CagA protein, which facilitates stronger CagA-SHP2 interactions and enhanced downstream signaling leading to aggressive gastric diseases (Higashi et al., 2002; Figure 2). This can partially explain why the prevalence of gastric cancer is highest in East-Asia and lowest in Africa. However, even in East-Asian countries, all individuals who are infected with the virulent H. pylori strains do not develop gastric cancer. Therefore, apart from the variations in the H. pylori strains in different geographical regions, additional factors like diversities in food habits, climates, and human genetic polymorphisms may also play a significant role in determining clinical outcomes.
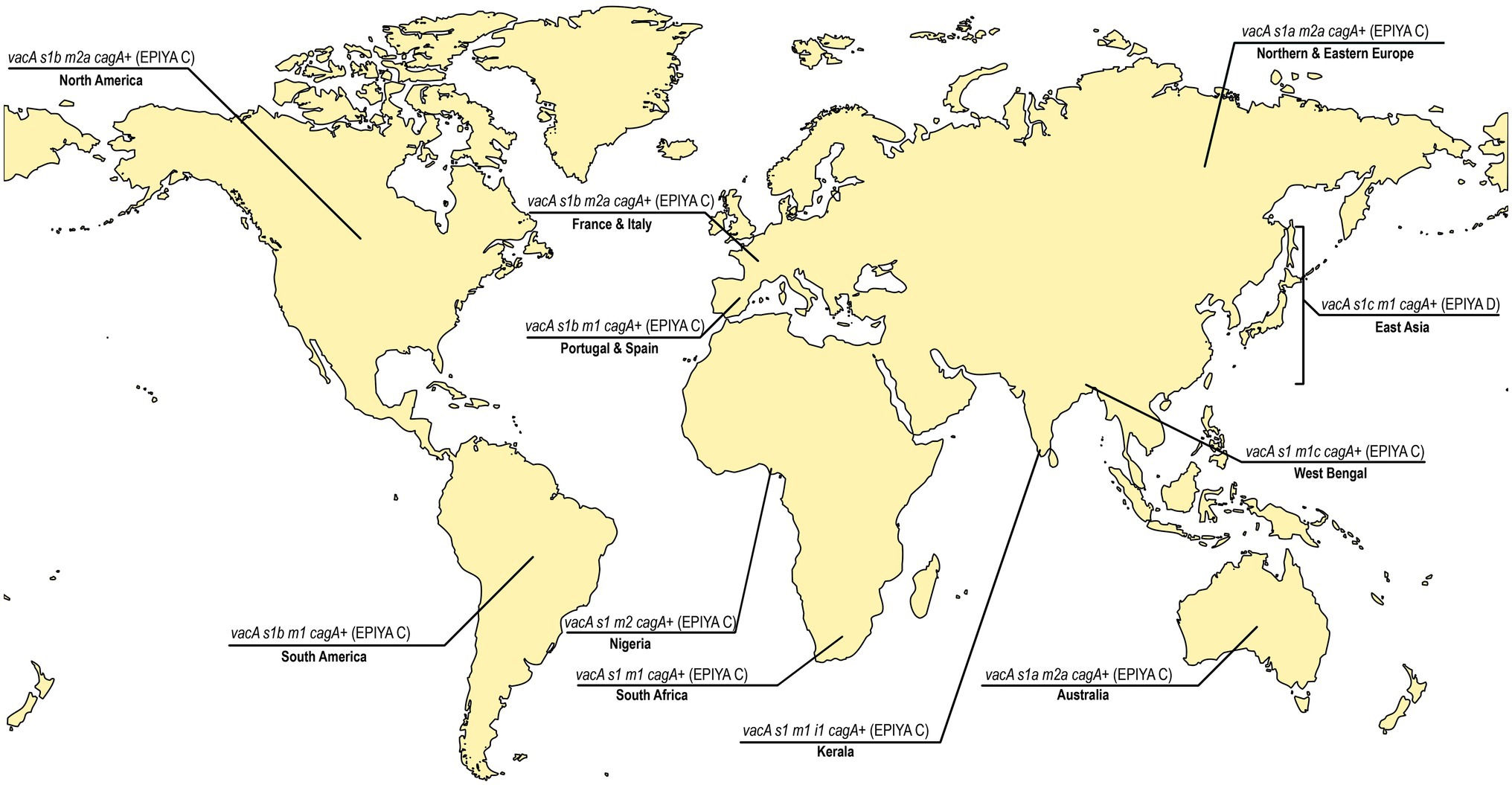
Figure 2. The predominant vacA genotypes and cagA status in different regions of the world. East Asia, North America, South America, Europe (France, Italy, Portugal Spain, Northern, and Eastern Europe), Africa (Nigeria and South Africa), India (West Bengal and Kerala), and Australia (van Doorn et al., 1999a; Mukhopadhyay et al., 2000; Chattopadhyay et al., 2002; Idowu et al., 2019; Ofori et al., 2019; Devi et al., 2021). The predominant vacA genotypes vary between and within the continents. The more virulent Glu-Pro-Ile-Tyr-Ala (EPIYA)-ABD type CagA is confined to strains circulating in East Asian countries where as the EPIYA-ABC type CagA is found in rest of the countries. The age standardized incidence of gastric cancer per 100,000 individuals in different countries has previously been reported [Eastern Asia and Eastern Europe ≥11.1; Portugal 7.3 to11.1; Spain 5 to 7.3; France 3.8 to 5; Italy 5 to 7.3; South America 7.3 to 11.1; North America 3.8 to 5; India (except West Bengal: 5 to 7.3), Australia, South Africa: 3.8 to 5;and Nigeria: <3.8; Smyth et al., 2020].
Climate
The different climatic and weather conditions in various parts of the world may also serve as one of the determining factors for the development of gastric diseases particularly in the context of peptic ulcer associated bleeding. Helicobacter pylori infection has been shown to be positively associated with daily average sunshine time and the expression of Vitamin D receptor increases in the gastric mucosa upon H. pylori infection (Guo et al., 2014; Lu et al., 2018). However, higher average annual temperature is negatively associated with H. pylori infection and the incidence of peptic ulcer in cold climate is significantly higher than in hot climates (Yuan et al., 2015; Lu et al., 2018). This might be due to the higher gastric acid secretions in response to the cold exposures (Yang et al., 2020). Experiments on the organotypic stomach slice cultures of mice showed that the cold-stress has the potential to induce gastrin expression and enhance the gastric acid secretion (Yang et al., 2020). Furthermore, the diversity of food habits in hot and in cold climatic regions may also contribute to the observed variations in the prevalence of H. pylori infection and the prevalence of gastric diseases (discussed later under the heading, Diet).
Host Immune System, Genetics, and Associated Polymorphisms
The persistence of H. pylori in the human stomach for years is achieved by maintaining a sustained host–microbe interaction, which begins as soon as the bacteria get attached to the gastric epithelial cells. Since the genetic makeup of each individual is different, their responses to invading pathogens are also expected to vary. The initial interaction between the bacteria and innate host immune system is mediated through the pattern recognition receptors (PRRs) expressed on the gastric epithelial cells. Various toll-like receptors (TLR) are one of the key PRRs, which trigger inflammation. The TLR-2 recognizes microbial components like lipoprotein, lipoteichoic acid, and peptidoglycan. An association of TLR2-196 to -174 del polymorphism with susceptibility to gastric cancer was demonstrated in Brazilian population (Table 3; de Oliveira and Silva, 2012). The genotypes TLR2 ins/del and del/del were found more often in the individuals with gastric cancer than the healthy individuals (de Oliveira and Silva, 2012). This polymorphism is also associated with increased risk of non-cardia gastric cancer in Japan (Tahara et al., 2007b). A 22-bp deletion in TLR2 gene that alters the promoter activity decreases transcriptional activity of the gene. Similarly, the Asp299Gly (rs4986790) and Thr399Ile (rs4986791) polymorphisms of TLR4 gene were shown to be the risk factors of gastric carcinoma in Caucasian and Indian populations (Table 3; Jing et al., 2012), although these polymorphisms are rare in Japanese and Chinese populations (Tahara et al., 2007a). Rather, the Chinese individuals with TLR5 rs5744174C carriers (TC+CC), but not with the TT genotype, have the increased risk of gastric cancer (Zeng et al., 2011). Likewise, the SNPs associated with TLRs (e.g., TLR1 rs4833095 and TLR10 rs10004195) also contribute to the susceptibility to H. pylori infection and may influence the clinical outcomes (Table 3; Simawaranon et al., 2017).
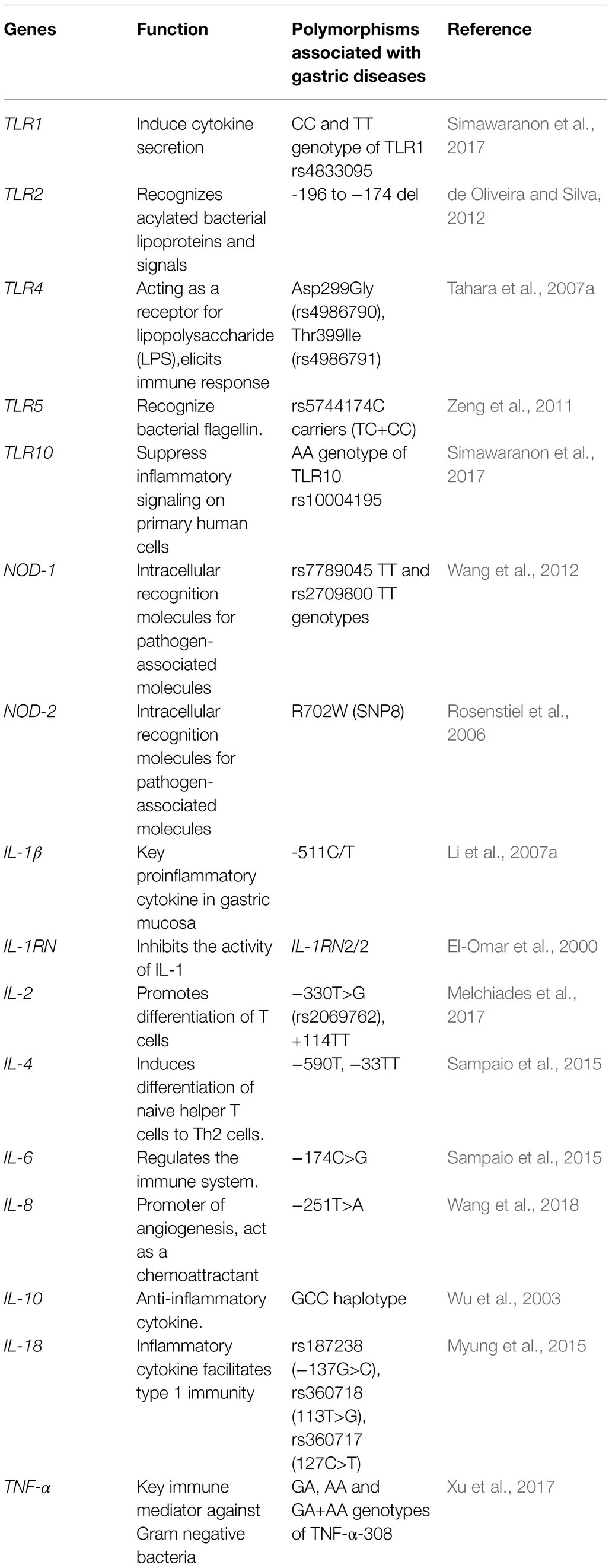
Table 3. Human genes mediating immune response and their polymorphisms associated with risk of gastric diseases.
The activation of another intracellular PRR, the nucleotide-binding oligomerization domain 1 (NOD-1), leads to the production of pro-inflammatory cytokines. Several studies suggested that the polymorphisms in nod-1 gene, the rs7789045 TT and rs2709800 TT genotypes, are linked to increased risk of gastric cancer (Table 3; Wang et al., 2012). The NOD-1 deficient mice are more prone to get infected with cagA positive strains, and the NOD-1 mutants produce lower amount of macrophage inflammatory protein-2 (MIP-2 or CXCL-2) than the wild type. The individuals carrying the R702W(SNP8) mutation in NOD-2/CARD15 gene failed to recognize the cagPAI-positive H. pylori (Table 3; Rosenstiel et al., 2006).
The mutations in the promoter and the transcribed regions of pro-inflammatory and anti-inflammatory cytokine genes lead to impaired expression of cytokines and aberrant responses to the invading pathogens. In the context of gastric physiology, IL-1β is a potent acid inhibitor and modulates various biological functions of gastric epithelial cell types. Upon H. pylori infection, IL-1β induces the pro-inflammatory immune response along with the inhibition of gastric acid leading to hypochlorhydria, which facilitates bacterial colonization. The functionally relevant polymorphisms in IL-1β promoter region could be associated with high or low IL-1β production. The IL-1β-511T+, IL-1β-31C+, and IL-1RN 2/2 polymorphisms are shown to be associated with the increased risk of hypochlorhydria and gastric cancer (El-Omar et al., 2000). In Chinese population, the individuals carrying the IL-1β-511C/T genotypes (CT carriers) have an increased risk of developing gastric cancer (Li et al., 2007a). For the Japanese population, it was shown that the carriers of the IL-1β-511T/T genotype or IL-1RN2/2 alleles had higher mucosal IL-1β than non-carriers (Table 3; Hwang et al., 2002). The presence of a variant GG genotype of IL-2 gene at −330T>G (rs2069762), and +114TT SNPs were found to be associated with an increased risk of gastric cancer in H. pylori infected Brazilian population (Melchiades et al., 2017). Similarly, a higher prevalence of IL-4 −590T and IL-4 −33TT genotypes was identified among the intestinal-type gastric cancer patients. For the Romanian population, the IL-4R −3223CÆT polymorphism has been shown to be associated with gastric cancer (Burada et al., 2012). Those, who carry the IL-4R −3223TT genotype, have 2.5-fold higher risk of developing gastric cancer (Table 3; Burada et al., 2012). The higher production of IL-6, a multifunctional cytokine, found to be associated with the −174C>G allele as compared to the CC genotype. The prevalence of IL-6 −174CG genotype is higher among the Portuguese patients with intestinal-type gastric cancer (Table 3; Sampaio et al., 2015). Likewise, theIL-18 SNPs, rs187238 (−137G>C), rs360718 (113T>G), and rs360717 (127C>T) genotypes were linked to the H. pylori- associated diseases (Myung et al., 2015). IL-8 is considered as a potential determinant in inflammation, tumor progression, metastasis, and angiogenesis (Lee et al., 2013). The presence of T-251A polymorphism in the IL-8 gene was shown to be associated with H. pylori infection and peptic ulcer (Table 3). Several studies showed that the subjects who are genetically predisposed to the enhanced production of anti-inflammatory cytokine IL-10 (particularly those who carry the GCC haplotype) are at higher risk of developing gastric cancer (Table 3; Wu et al., 2003; Sugimoto et al., 2007; Wang et al., 2018). TNF-α is a potent proinflammatory cytokine which is involved in the pathogenesis of H. pylori-associated gastric diseases (Thalmaier et al., 2002). Among the various polymorphisms in the TNF-α gene, GA, AA, and GA + AA genotypes of TNF-α-308 were identified to be significantly associated with gastric cancer when compared to homozygous GG type in Chinese population (Table 3; Xu et al., 2017).
Apart from cytokines, the dysregulation in the cellular and molecular pathways like survivin, COX-2, CDH1, and p53 also add on to higher risk of gastric cancer (Valenzuela et al., 2010). The G>A (−899) polymorphism in the cox-2 gene is related to the hyper-inflammatory responses. Similar to cox-2, mutations in the tumor suppressor gene, epithelial cadherin (E-cadherin) is linked to the hereditary diffuse gastric cancer (Liu and Chu, 2014). Mutations in the gene encoding E-cadherin leads to increased cell proliferation, migration, and reduced apoptosis Studies revealed that the promoter hyper-methylation of the E-cadherin gene has a significant role in gastric cancer (Miyazaki et al., 2007; Liu and Chu, 2014). The p53 is a tumor suppressor protein encoded by the TP53 gene, which is one of the most mutated genes related to cancers. The homozygous Pro/Pro genotype of the p53 codon 72 polymorphism increases the risk for gastric cancer (Yi and Lee, 2006). The results of a case control study in Chilean population showed that the polymorphisms in the proteins of the RAS/RAF/MEK/ERK pathways, such as rs3729931 (RAF1), rs45604736 (HRAS), rs2283792, and rs9610417 (MAPK1), are associated with gastric cancer (Gonzalez-Hormazabal et al., 2019).
Gastrointestinal Microbiota
In the GI tract, stomach has the lowest and colon has the highest number of microbes. Most of these microbes are not easy to grow in laboratory due to the lack of appropriate conditions. However, our initial knowledge on the microbial population in stomach was derived from the culture based methods, which showed that apart from H. pylori, human stomach is the niche of many other bacteria that belong to the Firmicutes, Proteobacteria, Actinobacteria, and Fusobacteria phyla (Savage, 1977; Adamsson et al., 1999). Then a culture-independent, cloning and sequence based early metagenomic study showed that the gastric microbiota are mainly dominated by the members of five bacterial phyla: Proteobacteria, Firmicutes, Bacteroidetes, Actinobacteria, and Fusobacteria (Bik et al., 2006). This was followed by a number of studies based on newer Phylochip hybridization array analysis and 454-pyrosequencing, which showed thathuman stomach, when it is not colonized with H. pylori, harbors a diverse array of microbes that belong mainly to phyla like Streptococcus, Proteobacteria, Bacteroidetes, Actinomyces, Prevotella, and Gemella (Andersson et al., 2008; Maldonado-Contreras et al., 2011). But, the human stomach that carries the burden of H. pylori infection, also suffer from dysbiosis, which is manifested by the decrease of microbial diversity, lower relative abundance of Actinobacteria and Firmicutes, and higher relative abundance of Proteobacteria other than H. pylori (Phukan et al., 2006; Handa et al., 2010; Das et al., 2017). This seems reasonable since the long-term colonization of H. pylori initiates the inflammatory cascades leading to atrophic gastritis, loss of the acid secreting parietal cells, and eventually an elevation of the gastric pH, which may preferentially allow certain microbes to proliferate faster in the altered gastric milieu and establish colonization (Figure 1). Some of these invading species, particularly the pathogenic ones that belong to the Proteobacteria phylum, may also facilitate the development of gastric diseases including cancer through various mechanisms like enhancement of inflammation, stimulation of cell proliferation, modification of stem cell dynamics, and production of toxic metabolites (Lofgren et al., 2011; Abreu and Peek, 2014). Recent studies using modern sophisticated next-generation sequencing techniques like Illumina have helped to understand the association between the composition of gut microbiota and the pathogenesis of various disease conditions. For example, a recent study revealed that patient groups having either gastric carcinoma or chronic gastritis have distinct microbiota compositions (Ferreira et al., 2018). In contrast to the patients with chronic gastritis, the patients with gastric cancer have lower abundance of Helicobacter and Neisseria, while the abundance of Achromobacter, Citrobacter, Phyllobacterium, Clostridium, Rhodococcus, and Lactobacillus was higher; Ferreira et al., 2018). Another study involving the populations of Singapore and Malaysia showed that several bacterial taxa, including Veillonella, Lactococcus, and Fusobacteriaceae were enriched in the stomach of patients with gastric cancer (Castaño-Rodríguez et al., 2017). The microbial compositions and the bacterial interactions possibly differ in different stages of gastric carcinogenesis (Coker et al., 2018). The Streptococcus, Prevotella, and Neisseria were found highly abundant in chronic gastritis patients along with H. pylori. However, for the patients with gastric carcinoma, the abundance of Helicobacter were less, while several taxa like Citrobacter, Clostridium, Lactobacillus, Achromobacter, and Rhodococcus were found to be more abundant (Ferreira et al., 2018). Most of them are commensals but also have the potentials to become opportunistic pathogens (Ferreira et al., 2018). A study from Taiwan also showed evidence of Clostridium and Fusobacterium colonization in the stomach of patients with gastric cancer (Hsieh et al., 2018).
Like gastric microbiota, the significance of intestinal microbiota in the context of H. pylori infection and its related gastric diseases have also been tested and verified recently. In murine model, it has been shown that H. pylori colonization in stomach is capable of altering the intestinal microbiota (Kienesberger et al., 2016). In human, for the Chinese and Indian populations, H. pylori infection in stomach leads to an increase in the microbial diversity in the intestine (Figure 1; Gao et al., 2018; Devi et al., 2021). This increased diversity in the intestinal microbiota for the H. pylori infected individuals could be due to the increased pH of the stomach or could be due to the altered gut immunity. In the Chinese population, a decrease in the abundance of Bacteroidetes and an increase in the abundance of Firmicutes and Proteobacteria were observed in the intestine of the H. pylori infected individuals (Gao et al., 2018). In Indian population, for the H. pylori infected individuals, the relative abundance of genus Prevotella and genus Dialister were found to be higher, while the relative abundance of the genus Bifidobacterium and genus Bacteroidetes were found to be lower in the intestine (Devi et al., 2021). Most remarkably, the H. pylori infected patients who had developed either gastric ulcer or gastric cancer, had a significantly low relative abundance of several species of Bifidobacterium (e.g., B. Longum and B. adolescentis) in the intestine (Table 4; Figure 1; Devi et al., 2021). It is worth mentioning that the bacteria belong to genus Bifidobacterium are mostly intestinal commensal bacterium and some of them carry anti-H. pylori, anti-cancer, and anti-ulcer activities. Also, several of the Bifidobacterium strains are known probiotics and provide benefit to the host by reducing inflammation.
A number of commensal microbes in the gut provide essential health benefits to its host in different ways (Table 4). Many of them have protective effect against H. pylori and therefore their abundance is crucial. Some of the commensals have also been used as probiotics, which utilize mechanisms like production of antimicrobials and antioxidants that can inhibit urease, compete with H. pylori to bind the surface of gastric epithelial cells, block their specific membrane receptors, and stabilize the mucosal barrier of the stomach by stimulating mucus production by surface epithelial cells (Khoder et al., 2016). Recent meta-analysis studies suggest that the probiotic supplementation along with antibiotic treatment for H. pylori eradication reduces the adverse side effects and improves the rate of eradication. Certain species of Lactobacilli are capable of preventing antibiotic-associated diarrhea along with the inhibition of potentially pathogenic species. Lactobacillus acidophilus has anti-H. pylori activity (Bhatia et al., 1989). Moreover, L. acidophilus was effective in suppressing the Smad7 and NF-kB pathways, and ameliorates the H. pylori induced inflammation (Yang et al., 2012). Likewise, probiotics such as L. bulgaricus and L. salivarius have the ability to reduce inflammation by the downregulation of IL-8 secretion (Qureshi et al., 2019). In addition, another Lactobacillus species, L. casei, inhibits the urease activity and reduces the colonization of H. pylori in stomach Table 4 (Sgouras et al., 2004). Furthermore, L. reuteri and L. johnsonii La1 inhibits the adhesion of H. pylori to the epithelium by competing for the specific binding site and secreting antimicrobial substances, respectively. Also, the reuterin produced by L. reuteri ATCC 55730 exhibits an inhibitory effect on the vacA gene expression of H. pylori (Qureshi et al., 2019).
Apart from this, a significant crosstalk exists between the gut microbiota and the host immune system. The short-chain fatty acids (SCFAs), produced as a result of microbial fermentation in gut, can activate extracellular signal-regulated kinase 1/2 and p38 mitogen-activated protein kinase signaling pathways in the epithelial cells resulting in production of cytokines and chemokines (Kim et al., 2013). The reduction in the SCFAs producing bacteria results in the T cell imbalance and dysregulations in the mucosal Th17 cells (Luu and Visekruna, 2019). The composition of GI microbiota is dynamic and the impacts of several other factors on the H. pylori associated gastric diseases could actually be through the alteration of GI microbiota.
Diet
Diet is increasingly being recognized as a critical factor in determining the susceptibility of an individual toward alteration of GI microbiota, H. pylori infection and gastric diseases. For example, a case–control study in Ardebil city, Iran for 128 adults (42 gastric cancer and 86 healthy) showed that both H. pylori infection and diet had a significant relationship with gastric cancer (Table 5; Nemati et al., 2012). Consumption of salted food, red meat, processed or smoked meat, and fishes increase the risk of developing atrophic gastritis with intestinal metaplasia and gastric cancer. The H. pylori-infected stomach has a lower level of vitamin C (Zhang et al., 1998), reduced synthesis of mucin (Byrd et al., 2000), and an increased level of ROS (Handa et al., 2010). These effects could get aggravated with the higher consumption of red meat since it contains heme bound iron, which helps formation of hydroxyl radicals (OH•; Macho-González et al., 2020). The preservatives such as nitrites presents in the preserved meat increase the exogenous exposure to nitrosamines and N-nitroso compounds (NOC), which increase the risk of gastric cancer (Jakszyn and González, 2006). Therefore, high consumptions of red meat and processed meat like bacon, ham, and sausages create oxidative stresses by forming ROS, which may lead to chronic diseases like gastric cancer (Zhu et al., 2013). A study from the state of Mizoram in Northeast India showed that apart from H. pylori infection, consumption of smoked, dried, and salted meat and fish as well as fermented pork fat increase the risk of gastric cancer (Phukan et al., 2006). Excessive consumption of salt can also damage the lining of the stomach, enhance NOC formation, and can facilitate the H. pylori colonization (Fox et al., 1999). The High temperature requirement A (HtrA) protein of H. pylori enables the bacteria to survive under stresses, like extreme salt concentration, pH, and temperature (Hansen and Hilgenfeld, 2013). This is more clarified with a follow-up study on salt consumption and gastric cancer development involving 2,476 subjects for 14 years showing significant salt-gastric cancer associations (Table 5; Shikata et al., 2006). The high salt diet also enhanced the gastric damages by the CagA expressing H. pylori strains leading to carcinogenesis in Mongolian gerbils (Gaddy et al., 2013). Significant association was found between the gastritis and the consumption of beverages like coffee and tea although their association with gastric cancer remained inconclusive (Sanikini et al., 2015; Mahmoud et al., 2016). A study conducted in Korea demonstrated that the individuals who carry the (TCA+TCG) haplotype in zip11 gene and consume spicy foods have 2.6-fold higher chance for developing chronic gastritis than the individuals with CAA haplotype in zip11 gene (Ha and Bae, 2018).
On the other hand, several components in diet may have protective roles. The intake of fruits and vegetables reduce the risk of gastric cancer as well as other gastrointestinal disease (Wang et al., 2014). The ascorbic acid present in fruits helps to scavenge the reactive radicals generated in human gastric mucosa and thereby prevents the DNA damage (Drake et al., 1996). Many oligosaccharides like inulin-type fructans polysaccharides including dietary fibers benefit the GI tract with their prebiotic activities (Mitmesser and Combs, 2017). The Wheat Peptides and Fucoidan (WPF) and SCFA help to mitigate the progression of chronic superficial gastritis (Kan et al., 2020). The fermented foods like dairy products serve as a natural source for beneficial microbes and some of them also have anti-H. pylori effects (Table 5; Nair et al., 2016). Consumption of fermented foods like yogurt, buttermilk, kefir, and kimchi helps to restore the count of beneficial microbes (e.g., Bifidobacterium) in gut (Kok and Hutkins, 2018; Rezac et al., 2018; Tamang et al., 2020).
The data from several systematic reviews and meta-analysis of cohort studies show that the polyphenols present in fruits and vegetables have protective effect against type 2 diabetes and cardiovascular diseases (Del Bo et al., 2019). Further, the diet rich in polyphenols possess anti-inflammatory and antimicrobial properties and reduce oxidative stress by modulating immune pathways. Green tea is rich in polyphenol, catechin which is a potent antioxidant. Apart from this, fruits and vegetables rich in polyphenols can modulate gut microbiota by promoting the growth of beneficial gut microbes and inhibits pathogenic species (Cory et al., 2018).
The eating patterns of warm vs. cold countries are significantly different and that may also contribute to the variation in the susceptibility to gastric diseases. Bacteria grow more rapidly in the warmer climates and causes food spoilage. However, people living in warm climates generally uses more spices during cooking and many of these spices have antibacterial properties (Gutierrez and Simon, 2016). For example, turmeric, clove, oregano, thyme, cinnamon, and cumin are known to have antibacterial (against Bacillus subtilis, Pseudomonas fluorescens, Staphylococcus aureus, and Vibrio parahaemolyticus) and antifungal (against Aspergillus flavus) properties (Liu et al., 2017). The active ingredient of turmeric, curcumin, has anti-H. pylori activity in addition to its anti-inflammatory, neuroprotective, and anti-cancer properties and is proposed to be used as therapeutic agent for H. pylori induced gastric diseases (De et al., 2009; Sarkar et al., 2016).
Medication
Several epidemiological studies emphasized the association of drugs with the gastric diseases. The extensive use of anti-reflux drugs, antacids, antiplatelets, selective serotonin reuptake inhibitors (SSRIs), and Non-steroidal anti-inflammatory drugs (NSAIDs) have the potential to predispose an individual to gastric diseases. Aspirin, a commonly used NSAID, enhances the plasma pro-inflammatory cytokines such as TNF-α leading to leukocyte infiltration in gastric mucosa and induces gastric ulceration (Table 6; Musumba et al., 2009). The proton pump inhibitors, such as omeprazole, pantoprazole, and histamine H2 receptors antagonists like cimetidine and ranitidine are used as effective acid suppressors in the treatment of acid related disorders (Table 6). However, the reduction of gastric acid by the PPIs may facilitate the colonization of pathogenic bacteria in the gut (Macke et al., 2020). The prolonged use of these drugs increases the possibility of altering the gastric microbiota and leading to gastric diseases including gastric cancer (Rodriguez et al., 2006; Mărginean et al., 2018). A study on Flemish cohort showed that 10% of the inter-individual variations in gut microbiota compositions may occur due to prolonged use of antibiotics, laxatives, benzodiazepines, and antidepressants (Falony et al., 2016; Doestzada et al., 2018). On the other hand, metformin, which is used for the treatment of type II diabetes, could also preferentially promote the growth of the bacteria (e.g., Roseburia and Subdoligranulum) producing the SCFAs like butyrate (Table 6; Forslund et al., 2015; Doestzada et al., 2018). Like the medications perturb the GI microbiota, the microbiota can also affect the activity of a drug. For instance, some prodrugs such as sulfasalazine, which is used for treatment of ulcerative colitis, require bioactivation by the gut microbes (Kim, 2015).
Antibiotics like gramicidin, neomycin, bacitracin, and tyrothricin, which are used for sore throat has been found to develop antimicrobial resistance in different human pathogens (Essack et al., 2019). Emergence of the antibiotic resistant strains of H. pylori allows the bacterium to persist in the stomach for prolonged duration and eradication of the H. pylori infection becomes challenging. It is one of the 12 antibiotic-resistant “priority pathogens” and included under the “high priority” category by WHO (Tacconelli et al., 2018). Easy availability and indiscriminate use of antibiotics may result in enhanced antibiotic resistance in the present H. pylori strains. The global levels of H. pylori resistance (17.2% for clarithromycin, 26.7% for metronidazole, 11.2% for amoxicillin, 16.2% for levofloxacin, and 5.9% for tetracycline) are alarming (De Francesco et al., 2010). Thus, emergence of antibiotic resistance in H. pylori due to indiscriminate use of antibiotics and failure to eradicate the infection is a significant factor that contributes to the clinical outcomes.
Discussion
Helicobacter pylori is a definite carcinogen with proven capabilities to trigger gastric adenocarcinoma and gastric MALT-lymphoma. It also has the potential to induce other severe and often terminal illnesses like duodenal and gastric ulcers. However, only a minor fraction of the people infected with the virulent H. pylori strains develops severe gastric diseases, while most of the infections remain benign indicating involvement of multiple factors. The most studied factor in this context is the variations in the virulence factors of H. pylori. For instance, the binding affinities of H. pylori may differ among strains because of the variations in the BabA sequences affecting the adhesion to the gastric epithelium. Similarly, certain polymorphisms in the vacA and the cagA genes may result in highly virulent combinations of the expressed proteins. Infections with the strains carrying such allelic combinations (e.g., vacAs1m1cagA+) have a higher risk of developing aggressive diseases than the infections with strains carrying the less virulent allelic combinations (e.g., vacAs2m2cagA-). Moreover, H. pylori infection, apart from causing gastric epithelial damage, also alters the gastric niche leading to loss of active H. pylori infection and it was suggested that H. pylori causes gastric cancer in the “hit and run” mechanism (Hatakeyama, 2014). However, even though its contribution to cause gastric illness is well-established, in many cases, H. pylori infection alone is insufficient to induce severe gastric diseases. Here, we showed that the contributions from other etiological factors in determining the clinical outcomes cannot be denied (Figure 1).
The polymorphisms in host genes encoding the immune effector proteins play important roles in the inter-individual variations in clinical outcomes since they directly add on to the susceptibility of an individual to H. pylori infection and related diseases. It is also evident that diet has a major role in either protecting an individual from gastric diseases or predisposing the individual to gastric pathologies. The enhanced oxidative stresses due to the consumptions of red meats may lead to chronic gastritis. Moreover, the NOC, which is formed by the consumption of salted food, damages the lining of stomach and facilitates the H. pylori induced gastric damages. This, on the other hand, can be mitigated by the protective effect of the WPF from wheat and the SCFA produced by the probiotics and even by the commensal microorganisms that are commonly present in the ethnic fermented foods (Nair et al., 2016). The intake of prebiotics may also be beneficial in preventing the development of severe gastric diseases. Conversely, the prolonged acid suppressions caused by the drugs like ranitidine, cimetidine, and omeprazole may increase the risk of gastric adenocarcinoma (Rodriguez et al., 2006). The extensive use of modern medicines may also lead to dysbiosis in the GI microbiota, which is a major factor in determining the susceptibility of several diseases including peptic ulcer and gastric cancer (Blaser, 2014).
Helicobacter pylori colonization in stomach also leads to dysbiosis of gastric and intestinal microbiota. Increased abundance of certain bacteria (e.g., bacteria belonging to the phyla Proteobacteria and Fusobacteria) in the stomach upon dysbiosis may have the potential to contribute in the process of ulceration and carcinogenesis. In direct contrast, several bacterial genera in the stomach (e.g., Lactobacillus) and in the intestine (e.g., Bifidobacterium) have the ability to protect the stomach from H. pylori infection and gastric diseases. Although poorly studied, but appears reasonable, it could be the ability of H. pylori to alter the GI microbiota that made the bacterium linked to a wide number of non-GI diseases (Table 1). For example, the recent studies showed the involvement of dysbiosis in the context of asthma and cardiac illness (Tang et al., 2017; Frati et al., 2019).
We conclude that even though H. pylori is the primary cause of the severe gastric diseases, the clinical outcomes are greatly influenced by “other factors” like host genetic polymorphisms, GI microbiota, diet, medication, geography, and climate. Their discrete as well as synergic effects predispose the H. pylori infected individuals to certain gastric diseases and also determine the severity of such diseases.
Author Contributions
SC conceived the idea. SA, RR, DC, TD, and SD contributed in writing the manuscript. KD, NT, JT, SL, and SC have edited the manuscript. All authors contributed to the article and approved the submitted version.
Funding
This work was supported by a grant (MED/2017/46) from the Department of Biotechnology (DBT), Government of India and a grant (ECR/2016/171) from the Science and Engineering Research Board (SERB) of Department of Science and Technology (DST), Government of India to SC as well as by the institutional support from the Rajiv Gandhi Centre for Biotechnology, and an autonomous institute under the Department of Biotechnology, Government of India.
Conflict of Interest
The authors declare that the research was conducted in the absence of any commercial or financial relationships that could be construed as a potential conflict of interest.
Publisher’s Note
All claims expressed in this article are solely those of the authors and do not necessarily represent those of their affiliated organizations, or those of the publisher, the editors and the reviewers. Any product that may be evaluated in this article, or claim that may be made by its manufacturer, is not guaranteed or endorsed by the publisher.
References
Abreu, M. T., and Peek, R. M. Jr. (2014). Gastrointestinal malignancy and the microbiome. Gastroenterology 146, 1534.e3–1546.e3. doi: 10.1053/j.gastro.2014.01.001
Adamsson, I., Nord, C. E., Lundquist, P., Sjöstedt, S., and Edlund, C. (1999). Comparative effects of omeprazole, amoxycillin plus metronidazole versus omeprazole, clarithromycin plus metronidazole on the oral, gastric and intestinal microflora in Helicobacter pylori-infected patients. J. Antimicrob. Chemother. 44, 629–640. doi: 10.1093/jac/44.5.629
Agha, A., and Graham, D. Y. (2005). Evidence-based examination of the African enigma in relation to Helicobacter pylori infection. Scand. J. Gastroenterol. 40, 523–529. doi: 10.1080/00365520510012280
Andersson, A. F., Lindberg, M., Jakobsson, H., Bäckhed, F., Nyrén, P., and Engstrand, L. (2008). Comparative analysis of human gut microbiota by barcoded pyrosequencing. PLoS One 3:e2836. doi: 10.1371/journal.pone.0002836
Asombang, A. W., Rahman, R., and Ibdah, J. A. (2014). Gastric cancer in Africa: current management and outcomes. World J Gastroenterol: WJG 20:3875. doi: 10.3748/wjg.v20.i14.3875
Aspholm-Hurtig, M., Dailide, G., Lahmann, M., Kalia, A., Ilver, D., Roche, N., et al. (2004). Functional adaptation of BabA, the H. pylori ABO blood group antigen binding adhesin. Science 305, 519–522. doi: 10.1126/science.1098801
Baj, J., Forma, A., Sitarz, M., Portincasa, P., Garruti, G., Krasowska, D., et al. (2021). Helicobacter pylori virulence factors—mechanisms of bacterial pathogenicity in the gastric microenvironment. Cell 10:27. doi: 10.3390/cells10010027
Bhatia, S. J., Kochar, N., Abraham, P., Nair, N. G., and Mehta, A. P. (1989). Lactobacillus acidophilus inhibits growth of campylobacter pylori in vitro. J. Clin. Microbiol. 27, 2328–2330. doi: 10.1128/jcm.27.10.2328-2330.1989
Bik, E. M., Eckburg, P. B., Gill, S. R., Nelson, K. E., Purdom, E. A., Francois, F., et al. (2006). Molecular analysis of the bacterial microbiota in the human stomach. Proc. Natl. Acad. Sci. 103, 732–737. doi: 10.1073/pnas.0506655103
Blaser, M. J. (2014). Missing Microbes: How the Overuse of Antibiotics Is Fueling Our Modern Plagues. United States: Macmillan publishers.
Boren, T., Falk, P., Roth, K. A., Larson, G., and Normark, S. (1993). Attachment of Helicobacter pylori to human gastric epithelium mediated by blood group antigens. Science 262, 1892–1895. doi: 10.1126/science.8018146
Bray, F., Ferlay, J., Soerjomataram, I., Siegel, R. L., Torre, L. A., and Jemal, A. (2018). Global cancer statistics 2018: GLOBOCAN estimates of incidence and mortality worldwide for 36 cancers in 185 countries. CA Cancer J. Clin. 68, 394–424. doi: 10.3322/caac.21492
Bugaytsova, J. A., Björnham, O., Chernov, Y. A., Gideonsson, P., Henriksson, S., Mendez, M., et al. (2017). Helicobacter pylori adapts to chronic infection and gastric disease via pH-responsive BabA-mediated adherence. Cell Host Microbe 21, 376–389. doi: 10.1016/j.chom.2017.02.013
Burada, F., Angelescu, C., Mitrut, P., Ciurea, T., Cruce, M., Saftoiu, A., et al. (2012). Interleukin-4 receptor− 3223C→ T polymorphism is associated with increased gastric adenocarcinoma risk. Can. J. Gastroenterol. 26, 532–536. doi: 10.1155/2012/804173
Burucoa, C., and Axon, A. (2017). Epidemiology of Helicobacter pylori infection. Helicobacter 22:e12403. doi: 10.1111/hel.12403
Byrd, J. C., Yunker, C. K., Xu, Q. S., Sternberg, L. R., and Bresalier, R. S. (2000). Inhibition of gastric mucin synthesis by Helicobacter pylori. Gastroenterology 118, 1072–1079. doi: 10.1016/S0016-5085(00)70360-X
Castaño-Rodríguez, N., Goh, K.-L., Fock, K. M., Mitchell, H. M., and Kaakoush, N. O. (2017). Dysbiosis of the microbiome in gastric carcinogenesis. Sci. Rep. 7:15957. doi: 10.1038/s41598-017-16289-2
Chang, W.-L., Yeh, Y.-C., and Sheu, B.-S. (2018). The impacts of H. pylori virulence factors on the development of gastroduodenal diseases. J. Biomed. Sci. 25:68. doi: 10.1186/s12929-018-0466-9
Chattopadhyay, S., Datta, S., Chowdhury, A., Chowdhury, S., Mukhopadhyay, A. K., Rajendran, K., et al. (2002). Virulence genes in Helicobacter pylori strains from West Bengal residents with overt H. pylori-associated disease and healthy volunteers. J. Clin. Microbiol. 40, 2622–2625. doi: 10.1128/JCM.40.7.2622-2625.2002
Chen, T. P., Hung, H. F., Chen, M. K., Lai, H. H., Hsu, W. F., Huang, K. C., et al. (2015). Helicobacter pylori infection is positively associated with metabolic syndrome in Taiwanese adults: a cross-sectional study. Helicobacter 20, 184–191. doi: 10.1111/hel.12190
Ciacci, C., Squillante, A., Rendina, D., Limauro, S., Bencivenga, C., Labanca, F., et al. (2000). Helicobacter pylori infection and peptic disease in coeliac disease. Eur. J. Gastroenterol. Hepatol. 12, 1283–1287. doi: 10.1097/00042737-200012120-00004
Coker, O. O., Dai, Z., Nie, Y., Zhao, G., Cao, L., Nakatsu, G., et al. (2018). Mucosal microbiome dysbiosis in gastric carcinogenesis. Gut 67, 1024–1032. doi: 10.1136/gutjnl-2017-314281
Cook, K. W., Crooks, J., Hussain, K., O’brien, K., Braitch, M., Kareem, H., et al. (2015). Helicobacter pylori infection reduces disease severity in an experimental model of multiple sclerosis. Front. Microbiol. 6:52. doi: 10.3389/fmicb.2015.00052
Correa, P., and Piazuelo, M. B. (2011). Helicobacter pylori infection and gastric adenocarcinoma. US Gastroenterol. Hepatol. Rev. 7, 59–64.
Cory, H., Passarelli, S., Szeto, J., Tamez, M., and Mattei, J. (2018). The role of polyphenols in human health and food systems: a mini-review. Front. Nutr. 5:87. doi: 10.3389/fnut.2018.00087
Das, A., Pereira, V., Saxena, S., Ghosh, T. S., Anbumani, D., Bag, S., et al. (2017). Gastric microbiome of Indian patients with Helicobacter pylori infection, and their interaction networks. Sci. Rep. 7:15438. doi: 10.1038/s41598-017-15510-6
De, R., Kundu, P., Swarnakar, S., Ramamurthy, T., Chowdhury, A., Nair, G. B., et al. (2009). Antimicrobial activity of curcumin against Helicobacter pylori isolates from India and during infections in mice. Antimicrob. Agents Chemother. 53, 1592–1597. doi: 10.1128/AAC.01242-08
De Francesco, V., Giorgio, F., Hassan, C., Manes, G., Vannella, L., Panella, C., et al. (2010). Worldwide H. pylori antibiotic resistance: a systematic review. J. Gastrointestin. Liver Dis. 19, 409–414.
de Oliveira, J. G., and Silva, A. E. (2012). Polymorphisms of the TLR2 and TLR4 genes are associated with risk of gastric cancer in a Brazilian population. World J Gastroenterol: WJG 18:1235. doi: 10.3748/wjg.v18.i11.1235
Del Bo, C., Bernardi, S., Marino, M., Porrini, M., Tucci, M., Guglielmetti, S., et al. (2019). Systematic review on polyphenol intake and health outcomes: is there sufficient evidence to define a health-promoting polyphenol-rich dietary pattern? Nutrients 11:1355. doi: 10.3390/nu11061355
Devi, T. B., Devadas, K., George, M., Gandhimathi, A., Chouhan, D., Retnakumar, R., et al. (2021). Low Bifidobacterium abundance in the lower gut microbiota is associated With Helicobacter pylori-related gastric ulcer and gastric Cancer. Front. Microbiol. 12:631140. doi: 10.3389/fmicb.2021.631140
Diamanti, A., Maino, C., Niveloni, S., Pedreira, S., Vazquez, H., Smecuol, E., et al. (1999). Characterization of gastric mucosal lesions in patients with celiac disease: a prospective controlled study. Am. J. Gastroenterol. 94, 1313–1319. doi: 10.1111/j.1572-0241.1999.01082.x
Doestzada, M., Vila, A. V., Zhernakova, A., Koonen, D. P. Y., Weersma, R. K., Touw, D. J., et al. (2018). Pharmacomicrobiomics: a novel route towards personalized medicine? Protein Cell 9, 432–445. doi: 10.1007/s13238-018-0547-2
Dorer, M. S., Talarico, S., and Salama, N. R. (2009). Helicobacter pylori’s unconventional role in health and disease. PLoS Pathog. 5:e1000544. doi: 10.1371/journal.ppat.1000544
Drake, I. M., Davies, M. J., Mapstone, N. P., Dixon, M. F., Schorah, C. J., White, K. L., et al. (1996). Ascorbic acid may protect against human gastric cancer by scavenging mucosal oxygen radicals. Carcinogenesis 17, 559–562.
Duncan, S. S., Bertoli, M. T., Kersulyte, D., Valk, P. L., Tamma, S., Segal, I., et al. (2013). Genome sequences of three hpAfrica2 strains of Helicobacter pylori. Genome Announc. 1, e00729–e00713. doi: 10.1128/genomeA.00729-13
El-Omar, E. M., Carrington, M., Chow, W.-H., Mccoll, K. E., Bream, J. H., Young, H. A., et al. (2000). Interleukin-1 polymorphisms associated with increased risk of gastric cancer. Nature 404, 398–402. doi: 10.1038/35006081
Essack, S., Bell, J., Burgoyne, D. S., Duerden, M., and Shephard, A. (2019). Topical (local) antibiotics for respiratory infections with sore throat: An antibiotic stewardship perspective. J. Clin. Pharm. Ther. 44, 829–837. doi: 10.1111/jcpt.13012
Fallone, C. A., Barkun, A. N., Friedman, G., Mayrand, S., Loo, V., Beech, R., et al. (2000). Is Helicobacter pylori eradication associated with gastroesophageal reflux disease? Am. J. Gastroenterol. 95, 914–920. doi: 10.1111/j.1572-0241.2000.01929.x
Falony, G., Joossens, M., Vieira-Silva, S., Wang, J., Darzi, Y., Faust, K., et al. (2016). Population-level analysis of gut microbiome variation. Science 352, 560–564. doi: 10.1126/science.aad3503
Fauzia, K. A., Miftahussurur, M., Syam, A. F., Waskito, L. A., Doohan, D., Rezkitha, Y. A. A., et al. (2020). Biofilm formation and antibiotic resistance phenotype of Helicobacter pylori clinical isolates. Toxins 12:473. doi: 10.3390/toxins12080473
Ferreira, R. M., Pereira-Marques, J., Pinto-Ribeiro, I., Costa, J. L., Carneiro, F., Machado, J. C., et al. (2018). Gastric microbial community profiling reveals a dysbiotic cancer-associated microbiota. Gut 67, 226–236. doi: 10.1136/gutjnl-2017-314205
Forslund, K., Hildebrand, F., Nielsen, T., Falony, G., Le Chatelier, E., Sunagawa, S., et al. (2015). Disentangling type 2 diabetes and metformin treatment signatures in the human gut microbiota. Nature 528, 262–266. doi: 10.1038/nature15766
Fox, J. G., Dangler, C. A., Taylor, N. S., King, A., Koh, T. J., and Wang, T. C. (1999). High-salt diet induces gastric epithelial hyperplasia and parietal cell loss, and enhances Helicobacter pylori colonization in C57BL/6 mice. Cancer Res. 59, 4823–4828.
Franchini, M., and Veneri, D. (2004). Helicobacter pylori infection and immune thrombocytopenic purpura: an update. Helicobacter 9, 342–346. doi: 10.1111/j.1083-4389.2004.00238.x
Frati, F., Salvatori, C., Incorvaia, C., Bellucci, A., Di Cara, G., Marcucci, F., et al. (2019). The role of the microbiome in asthma: the gut–lung axis. Int. J. Mol. Sci. 20:123. doi: 10.3390/ijms20010123
Fujimoto, S., Ojo, O. O., Arnqvist, A., Wu, J. Y., Odenbreit, S., Haas, R., et al. (2007). Helicobacter pylori BabA expression, gastric mucosal injury, and clinical outcome. Clin. Gastroenterol. Hepatol. 5, 49–58. doi: 10.1016/j.cgh.2006.09.015
Gaddy, J. A., Radin, J. N., Loh, J. T., Zhang, F., Washington, M. K., Peek, R. M., et al. (2013). High dietary salt intake exacerbates Helicobacter pylori-induced gastric carcinogenesis. Infect. Immun. 81, 2258–2267. doi: 10.1128/IAI.01271-12
Gao, J.-J., Zhang, Y., Gerhard, M., Mejias-Luque, R., Zhang, L., Vieth, M., et al. (2018). Association between gut microbiota and Helicobacter pylori-related gastric lesions in a high-risk population of gastric cancer. Front. Cell. Infect. Microbiol. 8:202. doi: 10.3389/fcimb.2018.00202
Gauthier, N. C., Monzo, P., Kaddai, V., Doye, A., Ricci, V., and Boquet, P. (2005). Helicobacter pylori VacA cytotoxin: a probe for a clathrin-independent and Cdc42-dependent pinocytic pathway routed to late endosomes. Mol. Biol. Cell 16, 4852–4866. doi: 10.1091/mbc.e05-05-0398
Gonzalez-Hormazabal, P., Musleh, M., Bustamante, M., Stambuk, J., Pisano, R., Valladares, H., et al. (2019). Polymorphisms in RAS/RAF/MEK/ERK pathway are associated with gastric cancer. Genes 10:20. doi: 10.3390/genes10010020
Graham, D. Y. (2014). History of Helicobacter pylori, duodenal ulcer, gastric ulcer and gastric cancer. World J Gastroenterol: WJG 20:5191. doi: 10.3748/wjg.v20.i18.5191
Guo, L., Chen, W., Zhu, H., Chen, Y., Wan, X., Yang, N., et al. (2014). H elicobacter pylori induces increased expression of the vitamin D receptor in immune responses. Helicobacter 19, 37–47. doi: 10.1111/hel.12102
Gutierrez, R., and Simon, S. A. (2016). Why do people living in hot climates like their food spicy? Temperature 3, 48–49. doi: 10.1080/23328940.2015.1119616
Ha, E., and Bae, J.-H. (2018). Zinc transporter SLC39A11 polymorphisms are associated with chronic gastritis in the Korean population: the possible effect on spicy food intake. Nutr. Res. 57, 78–85. doi: 10.1016/j.nutres.2018.04.014
Handa, O., Naito, Y., and Yoshikawa, T. (2010). Helicobacter pylori: a ROS-inducing bacterial species in the stomach. Inflamm. Res. 59, 997–1003. doi: 10.1007/s00011-010-0245-x
Hansen, G., and Hilgenfeld, R. (2013). Architecture and regulation of HtrA-family proteins involved in protein quality control and stress response. Cell. Mol. Life Sci. 70, 761–775. doi: 10.1007/s00018-012-1076-4
Hatakeyama, M. (2014). Helicobacter pylori CagA and gastric cancer: a paradigm for hit-and-run carcinogenesis. Cell Host Microbe 15, 306–316. doi: 10.1016/j.chom.2014.02.008
Hathroubi, S., Hu, S., and Ottemann, K. M. (2020). Genetic requirements and transcriptomics of Helicobacter pylori biofilm formation on abiotic and biotic surfaces. NPJ Biofilms Microbiomes 6:56. doi: 10.1038/s41522-020-00167-3
Hathroubi, S., Servetas, S. L., Windham, I., Merrell, D. S., and Ottemann, K. M. (2018). Helicobacter pylori biofilm formation and its potential role in pathogenesis. Microbiol. Mol. Biol. Rev. 82, e00001–e00018. doi: 10.1128/MMBR.00001-18
Higashi, H., Tsutsumi, R., Fujita, A., Yamazaki, S., Asaka, M., Azuma, T., et al. (2002). Biological activity of the Helicobacter pylori virulence factor CagA is determined by variation in the tyrosine phosphorylation sites. Proc. Natl. Acad. Sci. 99, 14428–14433. doi: 10.1073/pnas.222375399
Hooi, J. K., Lai, W. Y., Ng, W. K., Suen, M. M., Underwood, F. E., Tanyingoh, D., et al. (2017). Global prevalence of Helicobacter pylori infection: systematic review and meta-analysis. Gastroenterology 153, 420–429. doi: 10.1053/j.gastro.2017.04.022
Hsieh, Y.-Y., Tung, S.-Y., Pan, H.-Y., Yen, C.-W., Xu, H.-W., Lin, Y.-J., et al. (2018). Increased abundance of Clostridium and Fusobacterium in gastric microbiota of patients with gastric cancer in Taiwan. Sci. Rep. 8:158. doi: 10.1038/s41598-017-18596-0
Hussein, N. (2010). The association of dupA and Helicobacter pylori-related gastroduodenal diseases. Eur. J. Clin. Microbiol. Infect. Dis. 29, 817–821. doi: 10.1007/s10096-010-0933-z
Hwang, I.-R., Kodama, T., Kikuchi, S., Sakai, K., Peterson, L. E., Graham, D. Y., et al. (2002). Effect of interleukin 1 polymorphisms on gastric mucosal interleukin 1β production in Helicobacter pylori infection. Gastroenterology 123, 1793–1803. doi: 10.1053/gast.2002.37043
Idowu, A., Mzukwa, A., Harrison, U., Palamides, P., Haas, R., Mbao, M., et al. (2019). Detection of Helicobacter pylori and its virulence genes (cag A, dup A, and vac A) among patients with gastroduodenal diseases in Chris Hani Baragwanath Academic Hospital, South Africa. BMC Gastroenterol. 19:73. doi: 10.1186/s12876-019-0986-0
Ierardi, E., Losurdo, G., Mileti, A., Paolillo, R., Giorgio, F., Principi, M., et al. (2020). The puzzle of coccoid forms of Helicobacter pylori: beyond basic science. Antibiotics 9:293. doi: 10.3390/antibiotics9060293
Ishijima, N., Suzuki, M., Ashida, H., Ichikawa, Y., Kanegae, Y., Saito, I., et al. (2011). BabA-mediated adherence is a potentiator of the Helicobacter pylori type IV secretion system activity. J. Biol. Chem. 286, 25256–25264. doi: 10.1074/jbc.M111.233601
Jakszyn, P., and González, C. A. (2006). Nitrosamine and related food intake and gastric and oesophageal cancer risk: a systematic review of the epidemiological evidence. World J Gastroenterol: WJG 12, 4296–4303. doi: 10.3748/wjg.v12.i27.4296
Jing, J.-J., Li, M., and Yuan, Y. (2012). Toll-like receptor 4 Asp299Gly and Thr399Ile polymorphisms in cancer: a meta-analysis. Gene 499, 237–242. doi: 10.1016/j.gene.2012.03.045
Kan, J., Cheng, J., Xu, L., Hood, M., Zhong, D., Cheng, M., et al. (2020). The combination of wheat peptides and fucoidan protects against chronic superficial gastritis and alters gut microbiota: a double-blinded, placebo-controlled study. Eur. J. Nutr. 59, 1655–1666. doi: 10.1007/s00394-019-02020-6
Kaplan-Türköz, B., Jiménez-Soto, L. F., Dian, C., Ertl, C., Remaut, H., Louche, A., et al. (2012). Structural insights into Helicobacter pylori oncoprotein CagA interaction with β1 integrin. Proc. Natl. Acad. Sci. 109, 14640–14645. doi: 10.1073/pnas.1206098109
Khoder, G., Al-Menhali, A. A., Al-Yassir, F., and Karam, S. M. (2016). Potential role of probiotics in the management of gastric ulcer. Exp. Ther. Med. 12, 3–17. doi: 10.3892/etm.2016.3293
Kienesberger, S., Cox, L. M., Livanos, A., Zhang, X.-S., Chung, J., Perez-Perez, G. I., et al. (2016). Gastric Helicobacter pylori infection affects local and distant microbial populations and host responses. Cell Rep. 14, 1395–1407. doi: 10.1016/j.celrep.2016.01.017
Kikuchi, T., Kobayashi, T., Yamashita, T., Ohashi, K., Sakamaki, H., and Akiyama, H. (2011). Eight-year follow-up of patients with immune thrombocytopenic purpura related to H. pylori infection. Platelets 22, 59–64. doi: 10.3109/09537104.2010.515272
Kim, D.-H. (2015). Gut microbiota-mediated drug-antibiotic interactions. Drug Metab. Dispos. 43, 1581–1589. doi: 10.1124/dmd.115.063867
Kim, M. H., Kang, S. G., Park, J. H., Yanagisawa, M., and Kim, C. H. (2013). Short-chain fatty acids activate GPR41 and GPR43 on intestinal epithelial cells to promote inflammatory responses in mice. Gastroenterology 145, 396.e10–406.e10. doi: 10.1053/j.gastro.2013.04.056
Kinoshita-Daitoku, R., Kiga, K., Miyakoshi, M., Otsubo, R., Ogura, Y., Sanada, T., et al. (2021). A bacterial small RNA regulates the adaptation of Helicobacter pylori to the host environment. Nat. Commun. 12:2085. doi: 10.1038/s41467-021-22317-7
Kok, C. R., and Hutkins, R. (2018). Yogurt and other fermented foods as sources of health-promoting bacteria. Nutr. Rev. 76, 4–15. doi: 10.1093/nutrit/nuy056
Krzyżek, P., and Grande, R. (2020). Transformation of Helicobacter pylori into Coccoid forms as a challenge for research determining activity of antimicrobial substances. Pathogens 9:184. doi: 10.3390/pathogens9030184
Krzyżek, P., Grande, R., Migdał, P., Paluch, E., and Gościniak, G. (2020). Biofilm formation as a complex result of virulence and adaptive responses of Helicobacter pylori. Pathogens 9:1062. doi: 10.3390/pathogens9121062
Labenz, J., Blum, A., Bayerdorffer, E., Meining, A., Stolte, M., and Borsch, G. (1997). Curing Helicobacter pylori infection in patients with duodenal ulcer may provoke reflux esophagitis. Gastroenterology 112, 1442–1447. doi: 10.1016/S0016-5085(97)70024-6
Lamichhane, B., Chua, E.-G., Wise, M. J., Laming, C., Marshall, B. J., and Tay, C.-Y. (2019). The complete genome and methylome of Helicobacter pylori hpNEAfrica strain HP14039. Gut Pathog. 11:7. doi: 10.1186/s13099-019-0284-y
Lee, K. E., Khoi, P. N., Xia, Y., Park, J. S., Joo, Y. E., Kim, K. K., et al. (2013). Helicobacter pylori and interleukin-8 in gastric cancer. World J Gastroenterol: WJG 19:8192. doi: 10.3748/wjg.v19.i45.8192
Lee, S. P., Lee, S. Y., Kim, J. H., Sung, I. K., Park, H. S., Shim, C. S., et al. (2015). Correlation Between H elicobacter pylori infection, I g E hypersensitivity, and allergic disease in Korean adults. Helicobacter 20, 49–55. doi: 10.1111/hel.12173
Li, W., Minohara, M., Su, J. J., Matsuoka, T., Osoegawa, M., Ishizu, T., et al. (2007b). Helicobacter pylori infection is a potential protective factor against conventional multiple sclerosis in the Japanese population. J. Neuroimmunol. 184, 227–231. doi: 10.1016/j.jneuroim.2006.12.010
Li, C., Xia, H. H. X., Xie, W., Hu, Z., Ye, M., Li, J., et al. (2007a). Association between interleukin-1 gene polymorphisms and Helicobacter pylori infection in gastric carcinogenesis in a Chinese population. J. Gastroenterol. Hepatol. 22, 234–239. doi: 10.1111/j.1440-1746.2006.04379.x
Linz, B., Balloux, F., Moodley, Y., Manica, A., Liu, H., Roumagnac, P., et al. (2007). An African origin for the intimate association between humans and Helicobacter pylori. Nature 445, 915–918. doi: 10.1038/nature05562
Liu, X., and Chu, K.-M. (2014). E-cadherin and gastric cancer: cause, consequence, and applications. Biomed. Res. Int. 2014:637308. doi: 10.1155/2014/637308
Liu, J., He, C., Chen, M., Wang, Z., Xing, C., and Yuan, Y. (2013). Association of presence/absence and on/off patterns of Helicobacter pylori oipA gene with peptic ulcer disease and gastric cancer risks: a meta-analysis. BMC Infect. Dis. 13:555. doi: 10.1186/1471-2334-13-555
Liu, Q., Meng, X., Li, Y., Zhao, C.-N., Tang, G.-Y., and Li, H.-B. (2017). Antibacterial and antifungal activities of spices. Int. J. Mol. Sci. 18:1283. doi: 10.3390/ijms18061283
Lofgren, J. L., Whary, M. T., Ge, Z., Muthupalani, S., Taylor, N. S., Mobley, M., et al. (2011). Lack of commensal flora in Helicobacter pylori–infected INS-GAS mice reduces gastritis and delays intraepithelial neoplasia. Gastroenterology 140, 210–220. doi: 10.1053/j.gastro.2010.09.048
Lu, C., Yu, Y., Li, L., Yu, C., and Xu, P. (2018). Systematic review of the relationship of Helicobacter pylori infection with geographical latitude, average annual temperature and average daily sunshine. BMC Gastroenterol. 18:50. doi: 10.1186/s12876-018-0779-x
Lu, H., Hsu, P.-I., Graham, D. Y., and Yamaoka, Y. (2005). Duodenal ulcer promoting gene of Helicobacter pylori. Gastroenterology 128, 833–848. doi: 10.1053/j.gastro.2005.01.009
Luu, M., and Visekruna, A. (2019). Short-chain fatty acids: bacterial messengers modulating the immunometabolism of T cells. Eur. J. Immunol. 49, 842–848. doi: 10.1002/eji.201848009
Macho-González, A., Garcimartín, A., López-Oliva, M. E., Bastida, S., Benedí, J., Ros, G., et al. (2020). Can meat and meat-products induce oxidative stress? Antioxidants 9:638. doi: 10.3390/antiox9070638
Macke, L., Schulz, C., Koletzko, L., and Malfertheiner, P. (2020). Systematic review: the effects of proton pump inhibitors on the microbiome of the digestive tract—evidence from next-generation sequencing studies. Aliment. Pharmacol. Ther. 51, 505–526. doi: 10.1111/apt.15604
Mahmoud, S. S., Gasmi, F. M., Solan, Y. O., Al-Harbi, F. A., Al-Harbi, S. A., Hummadi, T. A., et al. (2016). Prevalence and predictors of gastritis among patients attending health care facilities in Jazan, KSA. Int. J. Prev. Health Sci. 2, 1–7. doi: 10.17354/ijpphs/2016/18
Maixner, F., Krause-Kyora, B., Turaev, D., Herbig, A., Hoopmann, M. R., Hallows, J. L., et al. (2016). The 5300-year-old Helicobacter pylori genome of the iceman. Science 351, 162–165. doi: 10.1126/science.aad2545
Maldonado-Contreras, A., Goldfarb, K. C., Godoy-Vitorino, F., Karaoz, U., Contreras, M., Blaser, M. J., et al. (2011). Structure of the human gastric bacterial community in relation to Helicobacter pylori status. ISME J. 5, 574–579. doi: 10.1038/ismej.2010.149
Malfertheiner, P., Link, A., and Selgrad, M. (2014). Helicobacter pylori: perspectives and time trends. Nat. Rev. Gastroenterol. Hepatol. 11:628. doi: 10.1038/nrgastro.2014.99
Mărginean, M. O., Meliț, L. E., Mocanu, S., and Săsăran, V. (2018). Ibuprofen, a potential cause of acute hemorrhagic gastritis in children-a case report. J. Crit. Care Med. 4:143. doi: 10.2478/jccm-2018-0022
Melchiades, J. L., Zabaglia, L. M., Sallas, M. L., Orcini, W. A., Chen, E., Smith, M. A., et al. (2017). Polymorphisms and haplotypes of the interleukin 2 gene are associated with an increased risk of gastric cancer. The possible involvement of Helicobacter pylori. Cytokine 96, 203–207. doi: 10.1016/j.cyto.2017.04.020
Mitmesser, S., and Combs, M. (2017). “Chapter 23 - prebiotics: inulin and other oligosaccharides,” in The Microbiota in Gastrointestinal Pathophysiology. eds. M. H. Floch, Y. Ringel, and W. A. Walker (Boston: Academic Press), 201–208.
Miyazaki, T., Murayama, Y., Shinomura, Y., Yamamoto, T., Watabe, K., Tsutsui, S., et al. (2007). E-cadherin gene promoter hypermethylation in H. pylori-induced enlarged fold gastritis. Helicobacter 12, 523–531. doi: 10.1111/j.1523-5378.2007.00519.x
Mobley, H. (1996). The role of Helicobacter pylori urease in the pathogenesis of gastritis and peptic ulceration. Aliment. Pharmacol. Ther. 10, 57–64. doi: 10.1046/j.1365-2036.1996.22164006.x
Moonens, K., Gideonsson, P., Subedi, S., Bugaytsova, J., Romaõ, E., Mendez, M., et al. (2016). Structural insights into polymorphic ABO glycan binding by Helicobacter pylori. Cell Host Microbe 19, 55–66. doi: 10.1016/j.chom.2015.12.004
Moraga, P., Abajobir, A. A., Abbafati, C., Abbas, K. M., Abd-Allah, F., Abera, S. F., et al. (2017). Global, regional, and national age-sex specific mortality for 264 causes of death, 1980-2016: a systematic analysis for the global burden of disease study 2016. Lancet 390, 1151–1210. doi: 10.1016/S0140-6736(17)32152-9
Mukhopadhyay, A. K., Kersulyte, D., Jeong, J.-Y., Datta, S., Ito, Y., Chowdhury, A., et al. (2000). Distinctiveness of genotypes of Helicobacter pylori in Calcutta, India. J. Bacteriol. 182, 3219–3227. doi: 10.1128/JB.182.11.3219-3227.2000
Musumba, C., Pritchard, D., and Pirmohamed, M. (2009). Cellular and molecular mechanisms of NSAID-induced peptic ulcers. Aliment. Pharmacol. Ther. 30, 517–531. doi: 10.1111/j.1365-2036.2009.04086.x
Myung, D.-S., Lee, W.-S., Park, Y.-L., Kim, N., Oh, H.-H., Kim, M.-Y., et al. (2015). Association between interleukin-18 gene polymorphism and Helicobacter pylori infection in the Korean population. Sci. Rep. 5:11535. doi: 10.1038/srep11535
Nair, M. R., Chouhan, D., Sen Gupta, S., and Chattopadhyay, S. (2016). Fermented foods: are they tasty medicines for Helicobacter pylori associated peptic ulcer and gastric cancer? Front. Microbiol. 7:1148. doi: 10.3389/fmicb.2016.01148
Narayanan, M., Reddy, K. M., and Marsicano, E. (2018). Peptic ulcer disease and Helicobacter pylori infection. Mo. Med. 115:219.
Nemati, A., Mahdavi, R., and Baghi, A. N. (2012). Case-control study of dietary pattern and other risk factors for gastric cancer. Health Promot. Perspect. 2:20. doi: 10.5681/hpp.2012.003
Ofori, E. G., Adinortey, C. A., Bockarie, A. S., Kyei, F., Tagoe, E. A., and Adinortey, M. B. (2019). Helicobacter pylori infection, virulence genes’ distribution and accompanying clinical outcomes: The West Africa situation. Biomed. Res. Int. 2019:7312908. doi: 10.1155/2019/7312908
Ortiz, M., Rosado-Carrión, B., and Bredy, R. (2014). Role of Helicobacter pylori infection in Hispanic patients with anemia. Bol. As. med. Puerto Rico 106, 13–18.
Papadakos, K. S., Sougleri, I. S., Mentis, A. F., Hatziloukas, E., and Sgouras, D. N. (2013). Presence of terminal EPIYA phosphorylation motifs in Helicobacter pylori CagA contributes to IL-8 secretion, irrespective of the number of repeats. PLoS One 8:e56291. doi: 10.1371/journal.pone.0056291
Phukan, R. K., Narain, K., Zomawia, E., Hazarika, N. C., and Mahanta, J. (2006). Dietary habits and stomach cancer in Mizoram, India. J. Gastroenterol. 41, 418–424. doi: 10.1007/s00535-006-1761-x
Polyzos, S. A., Zeglinas, C., Artemaki, F., Doulberis, M., Kazakos, E., Katsinelos, P., et al. (2018). Helicobacter pylori infection and esophageal adenocarcinoma: a review and a personal view. Ann. Gastroenterol. 31:8. doi: 10.20524/aog.2017.0213
Qureshi, N., Li, P., and Gu, Q. (2019). Probiotic therapy in Helicobacter pylori infection: a potential strategy against a serious pathogen? Appl. Microbiol. Biotechnol. 103, 1573–1588. doi: 10.1007/s00253-018-09580-3
Rezac, S., Kok, C. R., Heermann, M., and Hutkins, R. (2018). Fermented foods as a dietary source of live organisms. Front. Microbiol. 9:1785. doi: 10.3389/fmicb.2018.01785
Robinson, K. (2015). Helicobacter pylori-mediated protection against extra-gastric immune and inflammatory disorders: the evidence and controversies. Diseases 3, 34–55. doi: 10.3390/diseases3020034
Rodriguez, L. G., Lagergren, J., and Lindblad, M. (2006). Gastric acid suppression and risk of oesophageal and gastric adenocarcinoma: a nested case control study in the UK. Gut 55, 1538–1544. doi: 10.1136/gut.2005.086579
Rosenstiel, P., Hellmig, S., Hampe, J., Ott, S., Till, A., Fischbach, W., et al. (2006). Influence of polymorphisms in the NOD1/CARD4 and NOD2/CARD15 genes on the clinical outcome of Helicobacter pylori infection. Cell. Microbiol. 8, 1188–1198. doi: 10.1111/j.1462-5822.2006.00701.x
Rubenstein, J. H., Inadomi, J. M., Scheiman, J., Schoenfeld, P., Appelman, H., Zhang, M., et al. (2014). Association between Helicobacter pylori and Barrett's esophagus, erosive esophagitis, and gastroesophageal reflux symptoms. Clin. Gastroenterol. Hepatol. 12, 239–245. doi: 10.1016/j.cgh.2013.08.029
Sampaio, A. M., Balseiro, S. C., Silva, M. R., Alarcão, A., D’aguiar, M. J., Ferreira, T., et al. (2015). Association between IL-4 and IL-6 expression variants and gastric cancer among portuguese population. GE Port. J. Gastroenterol. 22, 143–152. doi: 10.1016/j.jpge.2015.04.001
Sanikini, H., Dik, V. K., Siersema, P. D., Bhoo-Pathy, N., Uiterwaal, C. S., Peeters, P. H., et al. (2015). Total, caffeinated and decaffeinated coffee and tea intake and gastric cancer risk: results from the EPIC cohort study. Int. J. Cancer 136, E720–E730. doi: 10.1002/ijc.29223
Sarkar, A., De, R., and Mukhopadhyay, A. K. (2016). Curcumin as a potential therapeutic candidate for Helicobacter pylori associated diseases. World J. Gastroenterol. 22, 2736–2748. doi: 10.3748/wjg.v22.i9.2736
Sato, Y., Yoneyama, O., Azumaya, M., Takeuchi, M., Sasaki, S. Y., Yokoyama, J., et al. (2015). The relationship between iron deficiency in patients with Helicobacter pylori-infected nodular gastritis and the serum prohepcidin level. Helicobacter 20, 11–18. doi: 10.1111/hel.12170
Savage, D. C. (1977). Microbial ecology of the gastrointestinal tract. Annu. Rev. Microbiol. 31, 107–133. doi: 10.1146/annurev.mi.31.100177.000543
Sgouras, D., Maragkoudakis, P., Petraki, K., Martinez-Gonzalez, B., Eriotou, E., Michopoulos, S., et al. (2004). In vitro and in vivo inhibition of Helicobacter pylori by Lactobacillus casei strain Shirota. Appl. Environ. Microbiol. 70, 518–526. doi: 10.1128/AEM.70.1.518-526.2004
Shikata, K., Kiyohara, Y., Kubo, M., Yonemoto, K., Ninomiya, T., Shirota, T., et al. (2006). A prospective study of dietary salt intake and gastric cancer incidence in a defined Japanese population: the Hisayama study. Int. J. Cancer 119, 196–201. doi: 10.1002/ijc.21822
Shiota, S., Matsunari, O., Watada, M., Hanada, K., and Yamaoka, Y. (2010). Systematic review and meta-analysis: the relationship between the Helicobacter pylori dupA gene and clinical outcomes. Gut pathog. 2:13. doi: 10.1186/1757-4749-2-13
Simawaranon, T., Wattanawongdon, W., and Tongtawee, T. (2017). Toll-like receptors are associated with Helicobacter pylori infection and gastric mucosa pathology. Jundishapur J. Microbiol. 10:e58351. doi: 10.5812/jjm.58351
Singh, K., and Ghoshal, U. C. (2006). Causal role of Helicobacter pylori infection in gastric cancer: an Asian enigma. World J Gastroenterol: WJG 12:1346. doi: 10.3748/wjg.v12.i9.1346
Smith, S., Fowora, M., and Pellicano, R. (2019). Infections with Helicobacter pylori and challenges encountered in Africa. World J. Gastroenterol. 25:3183. doi: 10.3748/wjg.v25.i25.3183
Smyth, E. C., Nilsson, M., Grabsch, H. I., Van Grieken, N. C., and Lordick, F. (2020). Gastric cancer. Lancet 396, 635–648. doi: 10.1016/S0140-6736(20)31288-5
Sugimoto, M., Furuta, T., Shirai, N., Nakamura, A., Kajimura, M., Sugimura, H., et al. (2007). Effects of interleukin-10 gene polymorphism on the development of gastric cancer and peptic ulcer in Japanese subjects. J. Gastroenterol. Hepatol. 22, 1443–1449. doi: 10.1111/j.1440-1746.2006.04613.x
Sugimoto, M., Zali, M., and Yamaoka, Y. (2009). The association of vacA genotypes and Helicobacter pylori-related gastroduodenal diseases in the Middle East. Eur. J. Clin. Microbiol. Infect. Dis. 28, 1227–1236. doi: 10.1007/s10096-009-0772-y
Tacconelli, E., Carrara, E., Savoldi, A., Harbarth, S., Mendelson, M., Monnet, D. L., et al. (2018). Discovery, research, and development of new antibiotics: the WHO priority list of antibiotic-resistant bacteria and tuberculosis. Lancet Infect. Dis. 18, 318–327. doi: 10.1016/S1473-3099(17)30753-3
Tahara, T., Arisawa, T., Shibata, T., Hirata, I., and Nakano, H. (2007a). Absence of common polymorphisms of toll like receptor 4 (TLR4): Asp299Gly, Thr399Ile in patients with gastroduodenal diseases in Japan. J. Clin. Biochem. Nutr. 40, 62–65. doi: 10.3164/jcbn.40.62
Tahara, T., Arisawa, T., Wang, F., Shibata, T., Nakamura, M., Sakata, M., et al. (2007b). Toll-like receptor 2 −196 to 174del polymorphism influences the susceptibility of Japanese people to gastric cancer. Cancer Sci. 98, 1790–1794. doi: 10.1111/j.1349-7006.2007.00590.x
Takahashi-Kanemitsu, A., Knight, C. T., and Hatakeyama, M. (2020). Molecular anatomy and pathogenic actions of Helicobacter pylori CagA that underpin gastric carcinogenesis. Cell. Mol. Immunol. 17, 50–63. doi: 10.1038/s41423-019-0339-5
Tamang, J. P., Cotter, P. D., Endo, A., Han, N. S., Kort, R., Liu, S. Q., et al. (2020). Fermented foods in a global age: east meets west. Compr. Rev. Food Sci. Food Saf. 19, 184–217. doi: 10.1111/1541-4337.12520
Tang, W. W., Kitai, T., and Hazen, S. L. (2017). Gut microbiota in cardiovascular health and disease. Circ. Res. 120, 1183–1196. doi: 10.1161/CIRCRESAHA.117.309715
Taylor, N. S., Fox, J. G., Akopyants, N. S., Berg, D. E., Thompson, N., Shames, B., et al. (1995). Long-term colonization with single and multiple strains of Helicobacter pylori assessed by DNA fingerprinting. J. Clin. Microbiol. 33, 918–923. doi: 10.1128/jcm.33.4.918-923.1995
Thalmaier, U., Lehn, N., Pfeffer, K., Stolte, M., Vieth, M., and Schneider-Brachert, W. (2002). Role of tumor necrosis factor alpha in Helicobacter pylori gastritis in tumor necrosis factor receptor 1-deficient mice. Infect. Immun. 70, 3149–3155. doi: 10.1128/IAI.70.6.3149-3155.2002
Thi Huyen Trang, T., Thanh Binh, T., and Yamaoka, Y. (2016). Relationship between vacA types and development of gastroduodenal diseases. Toxins 8:182. doi: 10.3390/toxins8060182
Thorell, K., Yahara, K., Berthenet, E., Lawson, D. J., Mikhail, J., Kato, I., et al. (2017). Rapid evolution of distinct Helicobacter pylori subpopulations in the Americas. PLoS Genet. 13:e1006546. doi: 10.1371/journal.pgen.1006730
Ulloa-Guerrero, C. P., Delgado, M. D. P., and Jaramillo, C. A. (2018). Structural analysis of variability and interaction of the N-terminal of the oncogenic effector CagA of Helicobacter pylori with Phosphatidylserine. Int. J. Mol. Sci. 19:3273. doi: 10.3390/ijms19103273
Utsch, C., and Haas, R. (2016). VacA’s induction of VacA-containing vacuoles (VCVs) and their immunomodulatory activities on human T cells. Toxins 8:190. doi: 10.3390/toxins8060190
Valenzuela, M., Perez-Perez, G., Corvalan, A. H., Carrasco, G., Urra, H., Bravo, D., et al. (2010). Helicobacter pylori-induced loss of the inhibitor-of-apoptosis protein survivin is linked to gastritis and death of human gastric cells. J. Infect. Dis. 202, 1021–1030. doi: 10.1086/656143
van Der Ende, A., Pan, Z. J., Bart, A., Van Der Hulst, R. W., Feller, M., Xiao, S. D., et al. (1998). cagA-positive Helicobacter pylori populations in China and The Netherlands are distinct. Infect. Immun. 66, 1822–1826. doi: 10.1128/IAI.66.5.1822-1826.1998
van Doorn, L. J., Figueiredo, C., Mégraud, F., Pena, S., Midolo, P., Queiroz, D. M. D. M., et al. (1999a). Geographic distribution of vacA allelic types of Helicobacter pylori. Gastroenterology 116, 823–830.
van Doorn, L. J., Figueiredo, C., Sanna, R., Blaser, M. J., and Quint, W. G. (1999b). Distinct variants of Helicobacter pylori cagA are associated with vacA subtypes. J. Clin. Microbiol. 37, 2306–2311.
Wang, P., Zhang, L., Jiang, J.-M., Ma, D., Tao, H.-X., Yuan, S.-L., et al. (2012). Association of NOD1 and NOD2 genes polymorphisms with Helicobacter pylori related gastric cancer in a Chinese population. World J Gastroenterol: WJG 18:2112. doi: 10.3748/wjg.v18.i17.2112
Wang, Q., Chen, Y., Wang, X., Gong, G., Li, G., and Li, C. (2014). Consumption of fruit, but not vegetables, may reduce risk of gastric cancer: results from a meta-analysis of cohort studies. Eur. J. Cancer 50, 1498–1509. doi: 10.1016/j.ejca.2014.02.009
Wang, Q., Yu, C., and Sun, Y. (2013). The association between asthma and Helicobacter pylori: a meta-analysis. Helicobacter 18, 41–53. doi: 10.1111/hel.12012
Wang, X., Yang, F., Xu, G., and Zhong, S. (2018). The roles of IL-6, IL-8 and IL-10 gene polymorphisms in gastric cancer: A meta-analysis. Cytokine 111, 230–236. doi: 10.1016/j.cyto.2018.08.024
Watari, J., Chen, N., Amenta, P. S., Fukui, H., Oshima, T., Tomita, T., et al. (2014). Helicobacter pylori associated chronic gastritis, clinical syndromes, precancerous lesions, and pathogenesis of gastric cancer development. World J Gastroenterol: WJG 20:5461. doi: 10.3748/wjg.v20.i18.5461
Westerik, N., Reid, G., Sybesma, W., and Kort, R. (2018). The probiotic lactobacillus rhamnosus for alleviation of Helicobacter pylori-associated gastric pathology in East Africa. Front. Microbiol. 9:1873. doi: 10.3389/fmicb.2018.01873
Wu, M. S., Wu, C. Y., Chen, C. J., Lin, M. T., Shun, C. T., and Lin, J. T. (2003). Interleukin-10 genotypes associate with the risk of gastric carcinoma in Taiwanese Chinese. Int. J. Cancer 104, 617–623. doi: 10.1002/ijc.10987
Xu, Y., Cao, X., Jiang, J., Chen, Y., and Wang, K. (2017). TNF-α-308/−238 polymorphisms are associated with gastric cancer: a case-control family study in China. Clin. Res. Hepatol. Gastroenterol. 41, 103–109. doi: 10.1016/j.clinre.2016.05.014
Xu, C., Soyfoo, D. M., Wu, Y., and Xu, S. (2020). Virulence of Helicobacter pylori outer membrane proteins: an updated review. Eur. J. Clin. Microbiol. Infect. Dis. 39, 1821–1830. doi: 10.1007/s10096-020-03948-y
Yahiro, K., Wada, A., Nakayama, M., Kimura, T., Ogushi, K.-I., Niidome, T., et al. (2003). Protein-tyrosine phosphatase α, RPTPα, is a Helicobacter pylori VacA receptor. J. Biol. Chem. 278, 19183–19189. doi: 10.1074/jbc.M300117200
Yamaoka, Y. (2010). Mechanisms of disease: Helicobacter pylori virulence factors. Nat. Rev. Gastroenterol. Hepatol. 7:629. doi: 10.1038/nrgastro.2010.154
Yamaoka, Y., Kita, M., Kodama, T., Sawai, N., Tanahashi, T., Kashima, K., et al. (1998). Chemokines in the gastric mucosa in Helicobacter pylori infection. Gut 42, 609–617.
Yamaoka, Y., Kodama, T., Kita, M., Imanishi, J., Kashima, K., and Graham, D. (1999). Relation between clinical presentation, Helicobacter pylori density, interleukin 1β and 8 production, and cagA status. Gut 45, 804–811. doi: 10.1136/gut.45.6.804
Yamasaki, E., Wada, A., Kumatori, A., Nakagawa, I., Funao, J., Nakayama, M., et al. (2006). Helicobacter pylori vacuolating cytotoxin induces activation of the proapoptotic proteins Bax and Bak, leading to cytochrome c release and cell death, independent of vacuolation. J. Biol. Chem. 281, 11250–11259. doi: 10.1074/jbc.M509404200
Yang, C., Sugimoto, K., Murata, Y., Hirata, Y., Kamakura, Y., Koyama, Y., et al. (2020). Molecular mechanisms of Wischnewski spot development on gastric mucosa in fatal hypothermia: an experimental study in rats. Sci. Rep. 10:1877. doi: 10.1038/s41598-020-58894-8
Yang, G. H., Wu, J. S., Yang, Y. C., Huang, Y. H., Lu, F. H., and Chang, C. J. (2014). Gastric H elicobacter pylori infection associated with risk of diabetes mellitus, but not prediabetes. J. Gastroenterol. Hepatol. 29, 1794–1799. doi: 10.1111/jgh.12617
Yang, Y.-J., Chuang, C.-C., Yang, H.-B., Lu, C.-C., and Sheu, B.-S. (2012). Lactobacillus acidophilus ameliorates H. pylori-induced gastric inflammation by inactivating the Smad7 and NFκB pathways. BMC Microbiol. 12:38. doi: 10.1186/1471-2180-12-38
Yeh, Y.-C., Kuo, H.-Y., Chang, W.-L., Yang, H.-B., Lu, C.-C., Cheng, H.-C., et al. (2019). H. pylori isolates with amino acid sequence polymorphisms as presence of both HtrA-L171 & CagL-Y58/E59 increase the risk of gastric cancer. J. Biomed. Sci. 26:4. doi: 10.1186/s12929-019-0498-9
Yi, S. Y., and Lee, W. J. (2006). A p53 genetic polymorphism of gastric cancer: difference between early gastric cancer and advanced gastric cancer. World J. Gastroenterol. 12, 6536–6539. doi: 10.3748/wjg.v12.i40.6536
Yu, X. J., Yang, X., Feng, L., Wang, L. L., and Dong, Q. J. (2017). Association between Helicobacter pylori infection and angiographically demonstrated coronary artery disease: a meta-analysis. Exp. Ther. Med. 13, 787–793. doi: 10.3892/etm.2017.4028
Yuan, X. G., Xie, C., Chen, J., Xie, Y., Zhang, K. H., and Lu, N. H. (2015). Seasonal changes in gastric mucosal factors associated with peptic ulcer bleeding. Exp. Ther. Med. 9, 125–130. doi: 10.3892/etm.2014.2080
Zeng, H.-M., Pan, K.-F., Zhang, Y., Zhang, L., Ma, J.-L., Zhou, T., et al. (2011). Genetic variants of toll-like receptor 2 and 5, Helicobacter pylori infection, and risk of gastric cancer and its precursors in a chinese population. Cancer Epidemiol. Biomark. Prev. 20, 2594–2602. doi: 10.1158/1055-9965.EPI-11-0702
Zevit, N., Balicer, R. D., Cohen, H. A., Karsh, D., Niv, Y., and Shamir, R. (2012). Inverse association between Helicobacter pylori and pediatric asthma in a high-prevalence population. Helicobacter 17, 30–35. doi: 10.1111/j.1523-5378.2011.00895.x
Zhang, Z., Patchett, S., Perrett, D., Katelaris, P., Domizio, P., and Farthing, M. (1998). The relation between gastric vitamin C concentrations, mucosal histology, and CagA seropositivity in the human stomach. Gut 43, 322–326. doi: 10.1136/gut.43.3.322
Keywords: gastric cancer and peptic ulcer, Helicobacter pylori, other factors, clinical outcomes, inconsistancy
Citation: Alexander SM, Retnakumar RJ, Chouhan D, Devi TNB, Dharmaseelan S, Devadas K, Thapa N, Tamang JP, Lamtha SC and Chattopadhyay S (2021) Helicobacter pylori in Human Stomach: The Inconsistencies in Clinical Outcomes and the Probable Causes. Front. Microbiol. 12:713955. doi: 10.3389/fmicb.2021.713955
Edited by:
Alain Pierre Gobert, Vanderbilt University Medical Center, United StatesReviewed by:
Tomoko Hanawa, Kyorin University, JapanGrażyna Gościniak, Wroclaw Medical University, Poland
Copyright © 2021 Alexander, Retnakumar, Chouhan, Devi, Dharmaseelan, Devadas, Thapa, Tamang, Lamtha and Chattopadhyay. This is an open-access article distributed under the terms of the Creative Commons Attribution License (CC BY). The use, distribution or reproduction in other forums is permitted, provided the original author(s) and the copyright owner(s) are credited and that the original publication in this journal is cited, in accordance with accepted academic practice. No use, distribution or reproduction is permitted which does not comply with these terms.
*Correspondence: Santanu Chattopadhyay, c2FudGFudUByZ2NiLnJlcy5pbg==