- State Key Laboratory of Respiratory Diseases, National Clinical Research Center for Respiratory Disease, Guangzhou Institute of Respiratory Health, The First Affiliated Hospital of Guangzhou Medical University, Guangzhou, China
The nosocomial pathogen Acinetobacter baumannii is a frequent cause of healthcare-acquired infections, particularly in critically ill patients, and is of serious concern due to its potential for acquired multidrug resistance. Whole-genome sequencing (WGS) is increasingly used to obtain a high-resolution view of relationships between isolates, which helps in controlling healthcare-acquired infections. Here, we conducted a retrospective study to identify epidemic situations and assess the percentage of transmission in intensive care units (ICUs). Multidrug-resistant A. baumannii (MDR-AB) were continuously isolated from the lower respiratory tract of different patients (at the first isolation in our ICU). We performed WGS, pulsed-field gel electrophoresis (PFGE), and multilocus-sequence typing (MLST) analyses to elucidate bacterial relatedness and to compare the performance of conventional methods with WGS for typing MDR-AB. From June 2017 to August 2018, A. baumannii complex strains were detected in 124 of 796 patients during their ICU stays, 103 of which were MDR-AB. Then we subjected 70 available MDR-AB strains to typing with WGS, PFGE, and MLST. Among the 70 A. baumannii isolates, 38 (54.29%) were isolated at admission, and 32(45.71%) were acquisition isolates. MLST identified 12 unique sequence types, a novel ST (ST2367) was founded. PFGE revealed 16 different pulsotypes. Finally, 38 genotypes and 23 transmissions were identified by WGS. Transmission was the main mode of MDR-AB acquisition in our ICU. Our results demonstrated that WGS was a discriminatory technique for epidemiological healthcare-infection studies. The technique should greatly benefit the identification of epidemic situations and controlling transmission events in the near future.
Introduction
Acinetobacter baumannii is a Gram-negative pathogen that causes serious nosocomial infections, especially in patients with advanced age, mechanical ventilation, respiratory failure, or a prolonged hospital stay in intensive care units (ICUs) (Vazquez-Lopez et al., 2020). In recent decades, A. baumannii has emerged as an important nosocomial pathogen that exhibits high levels of resistance to antibiotics and has become endemic in some geographical regions. Multidrug-resistant A. baumannii (MDR-AB) increases hospital stays and costs and limits therapeutic options. Critically ill patients infected with MDR-AB strains have substantially higher mortality rates (Falagas et al., 2006).
Accurate strain classification is a key process necessary for managing antimicrobial-resistant strains in health care-related infections (Kalenic and Budimir, 2009; Nishi and Hidaka, 2016). Pulsed-field gel electrophoresis (PFGE) is still used for typing analysis of A. baumannii, although it has drawbacks in terms of the time requirement and difficulty in data interpretation (Thong et al., 2002; Singh et al., 2006). Multilocus-sequence typing (MLST), the partial sequence-based typing method, is usually considered as the gold standard for global epidemiological investigations. It focuses on the clustering of isolates worldwide and is not sensitive to short duration and small-scale outbreaks (Tomaschek et al., 2016). It is considered a complementary approach of PFGE since it connects isolates with global epidemiology (Tomaschek et al., 2016). In a decade, whole-genome sequencing (WGS) has become a promising method in microbiological laboratories for strain identification, molecular epidemiology and outbreak analysis (Makke et al., 2020). It has the highest discriminatory power and is likely to be widely used with cost reduction (Halachev et al., 2014; Salipante et al., 2015; Hwang et al., 2021).
In this study, we conducted a retrospective study to identify an epidemic situation and MDR-AB-transmission events. We used reference-based single-nucleotide polymorphism (SNP) approaches to map either processed reads or assembled contigs to a reference genome, followed by SNPs calling. Then, SNPs differences among the MDR-AB isolates were processed. We aimed to describe the prevalence and molecular epidemiology of MDR-AB in the ICU through WGS-based typing and compare the performance of conventional methods with WGS for typing MDR-AB.
Materials and Methods
Setting
The First Affiliated Hospital of Guangzhou Medical University is a 1500-bed teaching hospital and a tertiary referral center for major respiratory disease in southern China. This study was conducted in the 27-bed adult ICU, which cares for patients with respiratory disease and those who have undergone thoracic surgical procedures. The ICU included 4 senior intensivists, 14 attendings, 6 residents, medical students, and nurses during the study period. Attendings and residents staffed the ICU 24 h per day and every day of the week. Three teams provided ICU medical coverage during the day: each including a senior intensivist, four attendings and two residents taking care of seven bed patients. The nurse-to-patient ratio was maintained at 1–1.5:1 during daytime and 1:3 at night.
Infection control measures mainly implemented in ICU have been previously described (Ye et al., 2015), mainly included: performing hygiene according to the WHO recommended and re-emphasis on the appropriate use of gloves, especially not allowed wear gloves to contract clean area items; implementing a cohort strategy, colonized/infected patients were admitted to a special room and treated by a dedicated team of healthcare workers; practice contact precautions for colonized/infected and newly admitted patients. Artificial airway management followed Critical Care Medicine Branch of Chinese Medical Association Guideline for diagnosis, prevention, and treatment of ventilator-associated pneumonia (Chinese Society of Critical Care Medicine, 2013).
Ethical approval was not required because the study was conducted as part of normal surveillance and management of healthcare-associated infections.
Bacterial Identification and Antimicrobial-Susceptibility Testing
Seventy-five MDR-AB complex isolates were collected from the lower respiratory tract of hospitalized ICU patients between June 2017 and August 2018 and were designated AB-1 to AB-75, based on admission date. The lower respiratory tract specimens were obtained by tracheal aspiration.
Strains were cryopreserved until needed for WGS. Initial identification was conducted using the VITEK2 Compact 30 System (bioMérieux). Antimicrobial susceptibility was determined via microdilution in accordance with guidelines of the Clinical and Laboratory Standards Institute (M100-S29). MDR was defined as resistance to at least one representative agent from three or more of the five antimicrobial-agent classes: cephalosporins (such as ceftazidime or cefepime), carbapenems (such as imipenem), β-lactamase inhibitors (such as cefoperazone/sulbactam), fluoroquinolones (such as ciprofloxacin), and aminoglycosides (such as amikacin) (Magiorakos et al., 2012).
PFGE Analysis
Isolates were inoculated in liquid Luria-Bertani medium and cultured overnight at 37°C. The bacterial supernatant was collected through centrifugation and resuspended in 1× Tris-ethylenediaminetetraacetic acid (EDTA) buffer (TE buffer) before an equal volume of 2% clean cut agarose was added. After immediate mixing, the liquid was placed in a mold and allowed to solidify. The block was then placed into 300 μL cell lysate (in 100 mmol/L Tris and 100 mmol/L EDTA), mixed with 5 μL proteinase K (20 mg/mL), and incubated overnight at 50°C. Next, the block was washed three times with 1 × TE, for 1 h/wash step, before being incubated overnight at 25°C with 50 U Apa I enzyme. Subsequently, PFGE was performed using a CHEF-DR III PFGE apparatus, under the following conditions: running buffer, 0.5 × TBE; 1% Pulsed-Field Certified Agarose; 14°C; 6 V/cm; angle of 120°; 0.5–20 s, overall time: 20 h. After the current ended, ethidium bromide staining was performed and images were obtained. Fingerprints were analyzed by BioNumerics software, using the unweighted pair group method and the arithmetic averages method, a 2% tolerance in strip position difference, and a 0.8% optimization value. Strains with ≥87% similarity were classified as the same subtype (representing the same clone), and those with <87% similarity were classified as different genotypes (representing different clones) (Seifert et al., 2005).
WGS and Assembly
Genomic DNA was extracted from 3 mL overnight cultures of 75 A. baumannii complex isolates, using the SPARK DNA Sample Prep Kit-96 (Enzymatics, United States). A library was generated for each isolate using the NEB Next Ultra DNA Library Prep Kit (Illumina, San Diego, CA, United States). DNA library concentrations were quantified using a Qubit® 2.0 Fluorometer and a QubitTM dsDNA HS Assay Kit, and 150-base pair (bp) paired-end reads were generated with a HiSeq®2500 instrument. Sequencing was performed at the Shanghai Biotechnology Corporation. We implemented a filtering pipeline that trimmed reads from the 3′ end with <20 sequencing quality, discarded adaptor sequences and reads <45 bp in length, removed reads containing Ns or where <50% of bases had >20 sequencing quality. Assembly statistics are displayed in Supplementary Table S1.
MLST Analysis
Conventional MLSTs were inferred in silico from WGS data. Seven MLST loci were selected using a sequence-extraction tool and then identified using a public A. baumannii MLST database.1
Single-Nucleotide Polymorphism Detection and Whole-Genome Phylogeny
Paired reads were mapped to A. baumannii strain XH386 (reference genome; GenBank accession number NZ_CP010779.1) using the Burrows–Wheeler Aligner (version 0.7.12). The original file was converted to a BAM file in SamTools. SNP and InDel results were processed simultaneously using the HaplotypeCaller tool, based on a real-time de novo algorithm with BAM files (reserved mutation sites with sequencing quality >30) and obtained VCF files. The SNPhylo program was used to remove low-quality SNPs; processed SNPs were then concatenated into a sequence and compared in the MUSCLE program. During this process, we discarded the following single-nucleotide variations (SNVs): (1) those in genes annotated as phages, transposases, or integrases, (2) those in genomic regions annotated as phages using Phage Finder, (3) those within 20 bp of the start or end of a contig, and (4) those with sequence quality <100. Tree construction was performed in PHYLIP. Trees were visualized in Evolview version 3.0.2 This analysis was performed at the Shanghai Biotechnology Corporation.
Previously, A. baumannii was estimated to have a ∼5 SNPs/year mutation rate over the whole genome (Hawkey et al., 2018). Isolates were assigned as the same genotype if their SNV numbers were ≤10.
Nucleotide Sequence Accession Numbers
Generated sequence reads were submitted to the NCBI Sequence Read Archive under the accession number PRJNA639298.
Results
Bacterial Isolates and Patients
This study spanned the period from June 2017 to August 2018, when 868 admissions (involving 796 patients) to the ICU were recorded. After deleting repetitive samples from the same patients with same isolates, we identified 124 samples as A. baumannii complex or A. baumannii using the VITEK2 Compact 30 System, of which 103 showed MDR.
Among those 103 samples, the first 75 MDR-AB complex and A. baumannii isolates obtained were retrieved from storage; WGS successfully analyzed 71 isolates. Seventy isolates were determined to be A. baumannii, and one isolate was identified as Acinetobacter calcoaceticus.
The 70 MDR-AB strains analyzed using WGS were from 70 different patients. The clinical characteristics of these 70 patients are summarized in Table 1.
Presentation With MDR-AB Upon ICU Admission and MDR-AB Acquisition in the ICU
All patients were screened for lower-respiratory tract infections within 24 h of admission and assessed at least once/week. Acquisition with MDR-AB was defined by a change in the colonization status from culture-negative to positive or a change of strains based on epidemiological data. During the study period, 55 patients (53.4%, 55/103) carried MDR-AB at the time of admission, and 48 acquisitions were identified. Among the 70 patients whose isolates were analyzed with WGS, 54.28% (n = 38) carried MDR-AB at the time of admission. Thirty-two acquisitions were identified: 13 patients who were negative during the first screening showed MDR-AB in a later screening; 19 patients showed changes of MDR-AB strains.
Antibiotic-Susceptibility Testing
Drug-sensitivity test results of the 70 MDR-AB strains are shown in Supplementary Table S2. Almost all isolates were resistant to quinolones. We found that 100% of the MDR-AB strains (n = 70) were resistant to ciprofloxacin, 80% (n = 56) were resistant to levofloxacin, and 14 isolates showed intermediate resistance to levofloxacin. Resistance rates to third-generation cephalosporins (cefotaxime and ceftazidime) were 91.43 and 97.14%, respectively. In addition, 98.57% (n = 69) were resistant to imipenem or piperacillin–tazobactam, 97.14% (n = 68) were resistant to meropenem, and all tested isolates were sensitive to colistin (six strains were untested).
MLST Analysis
Ten different STs were identified using the Oxford scheme, whereas only two STs were identified using the Pasteur MLST scheme. Considering that the Oxford scheme shows a higher concordance with WGS phylogenies and better discriminates between strains with short evolutionary distances, we adopted the Oxford scheme for further analysis. We identified 12 unique STs among the 70 A. baumannii isolates, namely ST208 (n = 31), ST136 (n = 14), ST195 (n = 9), ST457 (n = 4), ST1633 (n = 3), ST1849 (n = 2), ST369 (n = 2), ST229 (n = 1), ST191 (n = 1), ST1486 (n = 1), ST1806 (n = 1), and a novel ST, ST2367(n = 1) (Figure 1). Except for the ST229 clone, belonging to complex 110 international clone VII (CC110 IC7), the remaining isolates were assigned to clonal complex 92 (also known as international clone II), widely epidemic in over 30 countries (Karah et al., 2012; Tomaschek et al., 2016). Our study identified three major STs, with the predominant population being ST208, accounting for 44.29% (31/70). ST136 was the second-most prevalent clone (20.00%, 14/70), followed by ST195 (12.86%, 9/70).
PFGE Analysis
During the period when these strains were collected, we also typed isolates using PFGE. Strains with ≥87% similarity were considered the same subtype, representing the same clone (Seifert et al., 2005). Accordingly, we identified 16 different pulsotypes (Figure 1). Notably, 27 strains were classified as clone 8, making it the most abundant epidemic clone, and it continued to spread through the ICU during the study period.
Whole-Genome SNP Phylogenetic Analysis
A phylogenetic tree based on SNPs was constructed to clarify relationships among the sequenced strains. The median of the minimum SNV number across different isolates during the research period was 426 (interquartile range: 275–948; range: 0–52,781). Five distinct primary clades were identified (clades A–E; Figure 2).
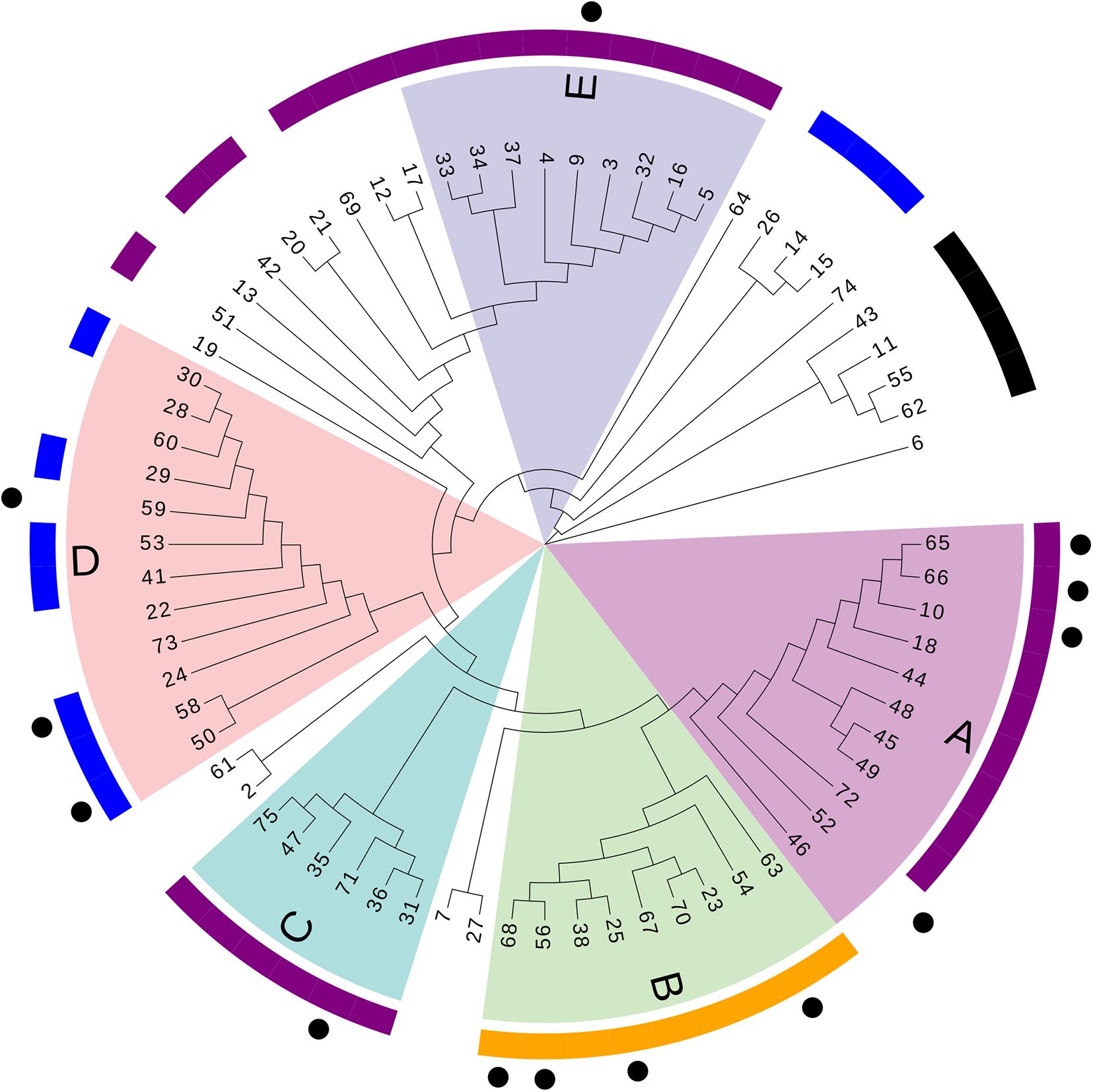
Figure 2. Phylogenetic tree based on SNPs. Numbers refer to the ID number of isolates. Different letters (A–E) and colors refer to branches of the five major clades. Outer purple ring, ST208; outer yellow ring, ST195; outer blue ring, ST136; outer black ring, ST457. Dots represent acquisitions of MDR-AB isolates of clone 8 with the same PFGE type.
We found 38 genotypes, with 14 genotypes identified in more than one patient. Along the 14 genotypes identified in more than one patient, we found that five genotypes consist of 16 instances where patients carried MDR-AB at the time of admission, suggesting that a common source of infection existed outside of the ICU (Figure 3).
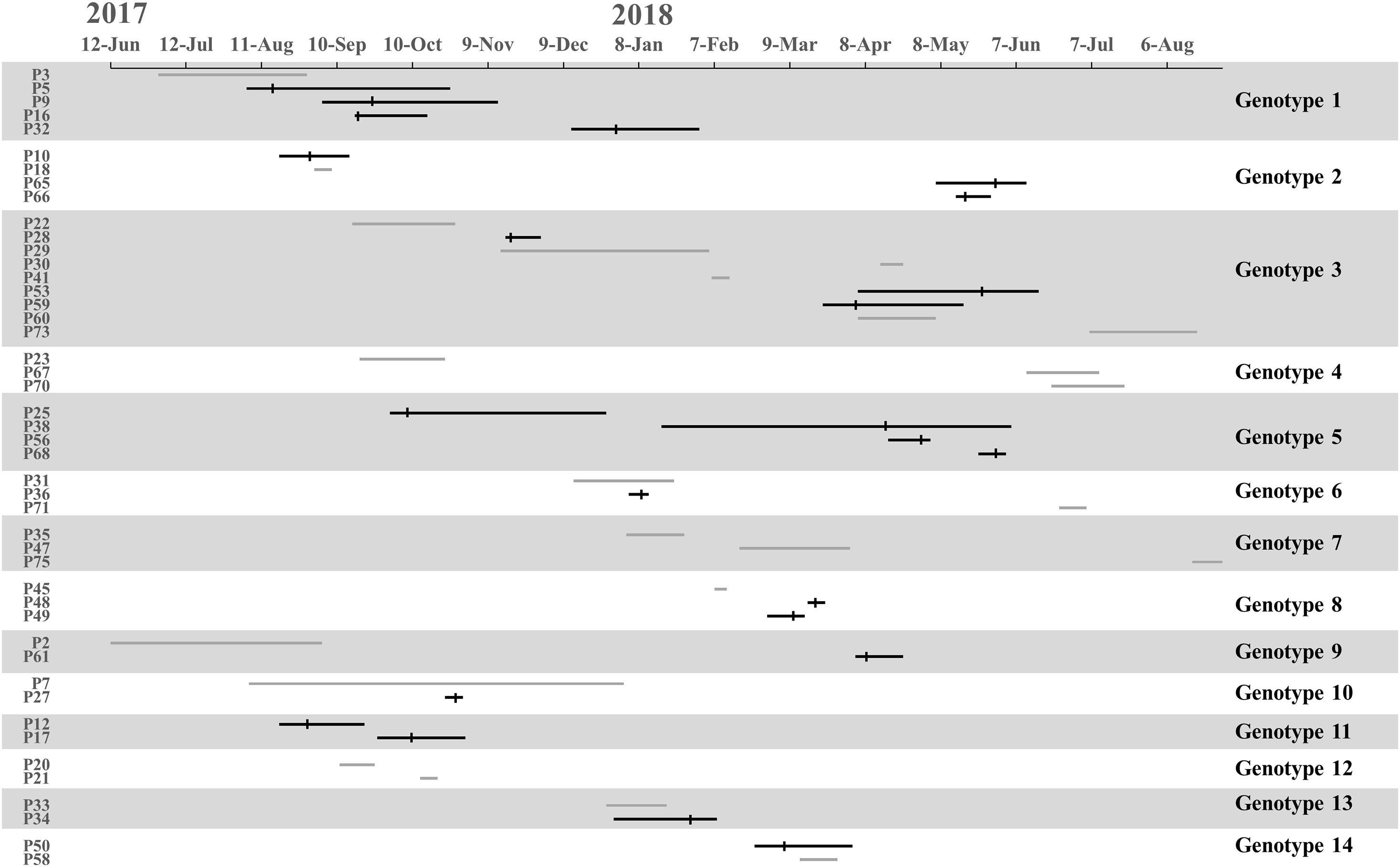
Figure 3. Timelines and overlap of major MDR-AB genotypes. The line indicates stay periods in the ICU. The gray line represents patients in whom MDR-AB isolates were detected before ICU admission. Vertical bars indicate the time at which MDR-AB-positive samples were detected.
Transmission was defined as patient acquisition of a genotype cultured from a previous patient, irrespective of overlapping patient stay in the ICU. Under WGS, transmission occurred when SNVs ≤10 between isolated pathogens. We identified 23 transmissions for 11 genotypes, consisting of 16 transmissions where donors were patients who carried MDR-AB at the time of admission (for eight genotypes), and 7 transmissions where the donor was not identified (for three genotypes) (Figure 3). Genotypes 1 and 5 showed the highest number of transmissions (four cases in each genotype). These results demonstrate that the major source of transmissions in our ICU was the admission isolates, also suggested that MDR-AB spread in our ICU mainly through transmission.
According to PFGE results, 27 MDR-AB strains formed clone 8, the largest epidemic clone, which was associated with 13 acquisitions. We reexamined this prevalent clone using WGS to determine whether “outbreak” isolates and transmissions identified with this technique actually reflect the relationship between different pathogens. The 13 acquisitions of MDR-AB isolates belonging to clone 8 were divided into five WGS clades, involving seven genotypes (Figures 2, 3). Mean number of SNVs among them was 279, ranging from 0 to 602 (92–377; Figure 4), and six isolates had <10 SNVs.
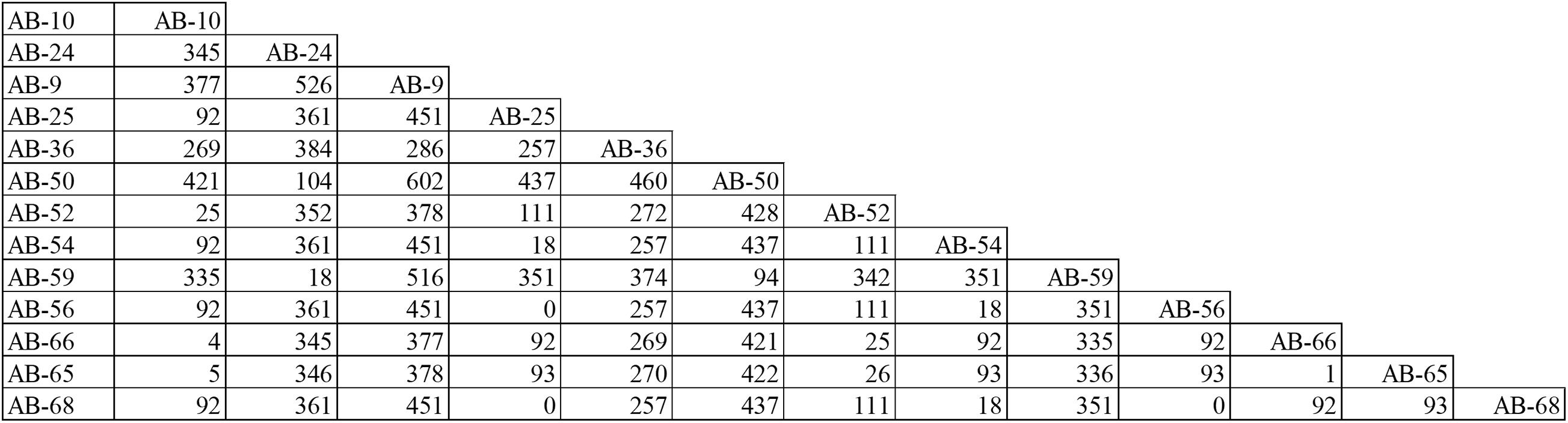
Figure 4. Single-nucleotide polymorphisms matrices for the 13 MDR-AB acquisitions belong to clone-8, as typed by PFGE.
Discussion
In this study, we used WGS to retrospectively investigate the epidemiology of clinical MDR-AB isolates in an adult ICU and to determine how frequently MDR-AB is transmitted between ICU patients.
Data from a previous epidemiological WGS study of Staphylococcus aureus suggested that, under standard infection-control measures, transmissions contributed to a small part of S. aureus acquisitions (Price et al., 2014). Price et al. (2014) reported that just 14% of patients were potentially involved in transmission at an adult ICU and high-dependency unit. Our findings demonstrated that, among the 868 ICU admissions, 23 of 103 patients with lower-respiratory tract infections acquired MDR-AB through transmission. Several factors may have contributed to the higher frequency of transmission in our ICU. First, the primary diagnosis upon ICU admission was mainly pneumonia (44/70, 62.86%). In 27 of these patients MDR-AB were cultured. Second, the samples collected also different from those of previous studies, as diagnosing lower-respiratory tract infections was the main objective of our study. Third, although the number of beds in our ICU and the study duration were similar, length of stay in our ICU was significantly longer than others reported.
Since our hospital is a center of respiratory disease in southern China, one of the most common reasons for admission to ICU is critical patients cause by respiratory diseases. Half of the patients were transferred from other hospitals where they had been treated, and 38/70 patients (56.52%) carried MDR-AB at admission. Preventing the spread of MDR-AB was a big challenge for our ICU. In our study, only two patients did not require ventilation, and most patients used antibiotics before admission into the ICU. Mechanical ventilation frequently requires tracheal intubation, which increases the risk of inhaling pathogenic bacteria or gastrointestinal tract bacteria (Coffin et al., 2008; De Waele et al., 2010). Prior use of antibiotics was demonstrated as an independent risk factor for colonization/infections with multi-resistant Gram-negative bacteria (Vasudevan et al., 2013). Along the ICU patients, bronchoscopy is a common diagnostic and therapeutic procedure which could cause nosocomial outbreak (McGrath et al., 2017). Those factors all pose risks for acquisition of MDR-AB and may contribute to transmission.
Among the 38 admission isolates, 16 MDR-AB isolates were highly related (SNV <10) and belonged to four genotypes, comprising seven isolates of patients transferred from another hospital and nine isolates from a different department in our hospital. These findings suggest that MDR-AB had disseminated in our hospital and spread widely throughout the local Guangzhou area. The data also imply that MDR-AB adapted for persistence and transmission in a hospital environment.
Acinetobacter baumannii can survive in the environment for a long time and is potentially transmissible (Marchaim et al., 2007). Contaminated environments and equipment can act as reservoirs for MDR-AB (Chapartegui-Gonzalez et al., 2018). In our study, strains belonging to the same genotype isolated from different patients (such as genotype 1 and 5) were sustained for a long period, and the patients did not share the same time in the ICU. This indicated that some transmission events might have occurred indirectly via the contaminated environment or healthcare workers. These findings are consistent with previous research conducted in our ICU (Ye et al., 2015). Except for genotype 1 and 5, the remain genotypes involved in more than one patient did not persist for a longer time. In our study period, a bundle of infection-prevention measures was implemented, including hand hygiene, contact precautions, and cohorting with dedicated healthcare staff. All of the components of the infection prevention bundle played a role in controlling MDR-AB dissemination. Cohorting with dedicated healthcare staff was firstly implemented in 2015 in our ICU to control MDR-AB outbreak (Ye et al., 2015) and is still implemented up to now. This measure was recommended by the Centers for Disease Control (WHO, 2017) and Prevention and the European Centre for Disease Prevention and Control (Magiorakos et al., 2017) and was shown to be efficient (Abboud et al., 2016; Perez-Blanco et al., 2018). We suggested that cohort strategy maybe contribute to reduce the MDR-AB dissemination in our ICU.
Classifying pathogens to elucidate epidemiology of pathogenic bacteria and hospital outbreaks relies on typing techniques with higher discriminatory power. Using conventional epidemiological data, our study identified 32 potential acquisitions that involved 29 clones from more than two isolates identified through PFGE. When WGS data were included, we reduced the number of transmissions to 23. In comparison with PFGE ability to type MDR-AB, WGS separated isolates belonging to clone 8 into five different clades. Then, we examined the typing ability of MLST. Although each clade typed by WGS corresponded to one of the three predominant STs, ST208 was interspersed into three different clades. PFGE and MLST both show poor agreement with WGS. Our data indicated that WGS offers the advantage of resolving differences between closely related isolates (Zarrilli et al., 2013). Therefore, WGS was more suitable for typing and identifying transmission between patients, especially when the same pandemic ST isolates are identified regionally.
The PubMLST database assigned all strains to 12 STs that could be grouped into CC92 clonal complexes (also known as international clone II), except for ST229. The majority of isolates belonged to ST-208, which had spread throughout other provinces in China (Jiang et al., 2016; Ning et al., 2017). Between 2012 and 2015, ST457 was reported as a prevalent pathogen with enhanced virulence in five hospitals in southern China (Zhou et al., 2018). Our study identified just four strains belonging to ST457, three of which were isolated at the time of admission, and they were not closely related (>25 SNPs). These findings suggest our ICU was not associated with the new emerging clones.
This study had two major limitations. First, we did not test any environmental samples or healthcare workers’ hands, sources that may have contributed to the spread of MDR-AB. Second, considering the sensitivity of bacterial culture, some changes in colonization status from culture-negative to culture-positive may have been false-positives. Hence, rate of acquisition may have been overestimated.
Conclusion
In conclusion, transmission mainly contributed to MDR-AB acquisition in our ICU; thus, prevention and control of MDR-AB hospital infections must be strengthened. Our study provides a high-resolution genome-wide perspective on MDR-AB epidemiology in a healthcare setting, while contributing to the development of appropriate intervention and prevention strategies.
Data Availability Statement
The datasets presented in this study can be found in online repositories. The names of the repository/repositories and accession number(s) can be found in the article/Supplementary Material.
Author Contributions
PM and XD participated in data analysis and drafted the manuscript. LY and YW carried out the molecular genetic studies. YJ participated in the clinical sample isolation and antibiotic testing. RZ and CY managed the data collection. YX, XL, and YL participated in the study design. All authors read and approved the final manuscript.
Funding
This work was supported by grants from the National Natural Science Foundation of China (Grant Number 81770077) and the Natural Science Foundation of Guangdong Province, China (Grant Number 2017A030313712).
Conflict of Interest
The authors declare that the research was conducted in the absence of any commercial or financial relationships that could be construed as a potential conflict of interest.
Publisher’s Note
All claims expressed in this article are solely those of the authors and do not necessarily represent those of their affiliated organizations, or those of the publisher, the editors and the reviewers. Any product that may be evaluated in this article, or claim that may be made by its manufacturer, is not guaranteed or endorsed by the publisher.
Acknowledgments
We would like to thank Editage (www.editage.cn) for English language editing and Shanghai Biotechnology Corporation for whole-genome sequencing.
Supplementary Material
The Supplementary Material for this article can be found online at: https://www.frontiersin.org/articles/10.3389/fmicb.2021.715568/full#supplementary-material
Supplementary Table S1 | Whole-genome sequencing and assembly statistics of 70 isolates.
Supplementary Table S2 | General patient information and the results of drug-sensitivity testing.
Footnotes
References
Abboud, C. S., de Souza, E. E., Zandonadi, E. C., Borges, L. S., Miglioli, L., Monaco, F. C., et al. (2016). Carbapenem-resistant Enterobacteriaceae on a cardiac surgery intensive care unit: successful measures for infection control. J. Hosp. Infect. 94, 60–64. doi: 10.1016/j.jhin.2016.06.010
Chapartegui-Gonzalez, I., Lazaro-Diez, M., Bravo, Z., Navas, J., Icardo, J. M., and Ramos-Vivas, J. (2018). Acinetobacter baumannii maintains its virulence after long-time starvation. PLoS One 13:e0201961. doi: 10.1371/journal.pone.0201961
Chinese Society of Critical Care Medicine (2013). Guideline for the prevention, diagnosis and treatment of ventilator-associated pneumonia (2013). Zhonghua Nei Ke Za Zhi 52, 524–543. doi: 10.3760/cma.j.issn.0578-1426.2013.06.024
Coffin, S., Klompas, M., Classen, D., Arias, K., Podgorny, K., Anderson, D., et al. (2008). Strategies to prevent ventilator-associated pneumonia in acute care hospitals. Infect. Control Hosp. Epidemiol. 29(Suppl. 1), S31–S40. doi: 10.1086/591062
De Waele, J., Ravyts, M., Depuydt, P., Blot, S., Decruyenaere, J., and Vogelaers, D. (2010). De-escalation after empirical meropenem treatment in the intensive care unit: fiction or reality? J. Crit. Care 25, 641–646. doi: 10.1016/j.jcrc.2009.11.007
Falagas, M. E., Bliziotis, I. A., and Siempos, I. I. (2006). Attributable mortality of Acinetobacter baumannii infections in critically ill patients: a systematic review of matched cohort and case-control studies. Crit. Care 10:R48. doi: 10.1186/cc4869
Halachev, M. R., Chan, J. Z. M., Constantinidou, C. I., Cumley, N., Bradley, C., Smith-Banks, M., et al. (2014). Genomic epidemiology of a protracted hospital outbreak caused by multidrug-resistant Acinetobacter baumanniiin Birmingham, England. Genome Med. 6:70. doi: 10.1186/s13073-014-0070-x
Hawkey, J., Ascher, D., Judd, L., Wick, R., Kostoulias, X., Cleland, H., et al. (2018). Evolution of carbapenem resistance in Acinetobacter baumannii during a prolonged infection. Microb. Genom. 4:e000165. doi: 10.1099/mgen.0.000165
Hwang, S. M., Cho, H. W., Kim, T. Y., Park, J. S., Jung, J., Song, K. H., et al. (2021). Whole-genome sequencing for investigating a health care-associated outbreak of carbapenem-resistant Acinetobacter baumannii. Diagnostics 11:201. doi: 10.3390/diagnostics11020201
Jiang, M., Liu, L., Ma, Y., Zhang, Z., Li, N., Zhang, F., et al. (2016). Acinetobacter baumannii molecular epidemiology of multi-drug resistant isolated in Shandong, China. Front. Microbiol. 7:1687. doi: 10.3389/fmicb.2016.01687
Kalenic, S., and Budimir, A. (2009). The role of microbiology laboratory in healthcare-associated infection prevention. Int. J. Infect. Control V5:i2. doi: 10.3396/ijic.V5i2.014.09
Karah, N., Sundsfjord, A., Towner, K., Samuelsen, Ø, antimicrobial, c.i, and chemotherapy, a (2012). Insights into the global molecular epidemiology of carbapenem non-susceptible clones of Acinetobacter baumannii. Drug Resist. Updat. 15, 237–247. doi: 10.1016/j.drup.2012.06.001
Magiorakos, A. P., Burns, K., Rodríguez Baño, J., Borg, M., Daikos, G., Dumpis, U., et al. (2017). Infection prevention and control measures and tools for the prevention of entry of carbapenem-resistant Enterobacteriaceae into healthcare settings: guidance from the European Centre for Disease Prevention and Control. Antimicrob. Resist. Infect. Control 6:113. doi: 10.1186/s13756-017-0259-z
Magiorakos, A. P., Srinivasan, A., Carey, R. B., Carmeli, Y., Falagas, M. E., Giske, C. G., et al. (2012). Multidrug-resistant, extensively drug-resistant and pandrug-resistant bacteria: an international expert proposal for interim standard definitions for acquired resistance. Clin. Microbiol. Infect. 18, 268–281. doi: 10.1111/j.1469-0691.2011.03570.x
Makke, G., Bitar, I., Salloum, T., Panossian, B., Alousi, S., Arabaghian, H., et al. (2020). Whole-genome-sequence-based characterization of extensively drug-resistant Acinetobacter baumannii hospital outbreak. mSphere 5:e00934-19. doi: 10.1128/mSphere.00934-19
Marchaim, D., Navon-Venezia, S., Schwartz, D., Tarabeia, J., Fefer, I., Schwaber, M. J., et al. (2007). Surveillance cultures and duration of carriage of multidrug-resistant Acinetobacter baumannii. J. Clin. Microbiol. 45, 1551–1555. doi: 10.1128/JCM.02424-06
McGrath, B. A., Ruane, S., McKenna, J., and Thomas, S. (2017). Contamination of single-use bronchoscopes in critically ill patients. Anaesthesia 72, 36–41. doi: 10.1111/anae.13622
Ning, N., Liu, X., Bao, C., Chen, S., Cui, E., Zhang, J., et al. (2017). Molecular epidemiology of bla -producing carbapenem-resistant Acinetobacter baumannii in a single institution over a 65-month period in north China. Infect. Drug Resist. 17:14. doi: 10.1186/s12879-016-2110-1
Nishi, I., and Hidaka, Y. (2016). [The role of the microbiology laboratory in healthcare-associated infection control]. Rinsho Byori 64, 338–344.
Perez-Blanco, V., Redondo-Bravo, L., Ruiz-Carrascoso, G., Pano-Pardo, J. R., Gomez-Gil, R., Robustillo-Rodela, A., et al. (2018). Epidemiology and control measures of an OXA-48-producing Enterobacteriaceae hospital-wide oligoclonal outbreak. Epidemiol. Infect. 146, 656–662. doi: 10.1017/S0950268818000249
Price, J. R., Golubchik, T., Cole, K., Wilson, D. J., Crook, D. W., Thwaites, G. E., et al. (2014). Whole-genome sequencing shows that patient-to-patient transmission rarely accounts for acquisition of Staphylococcus aureus in an intensive care unit. Clin. Infect. Dis. 58, 609–618. doi: 10.1093/cid/cit807
Salipante, S. J., SenGupta, D. J., Cummings, L. A., Land, T. A., Hoogestraat, D. R., and Cookson, B. T. (2015). Application of whole-genome sequencing for bacterial strain typing in molecular epidemiology. J. Clin. Microbiol. 53, 1072–1079. doi: 10.1128/JCM.03385-14
Seifert, H., Dolzani, L., Bressan, R., van der Reijden, T., van Strijen, B., Stefanik, D., et al. (2005). Standardization and interlaboratory reproducibility assessment of pulsed-field gel electrophoresis-generated fingerprints of Acinetobacter baumannii. J. Clin. Microbiol. 43, 4328–4335. doi: 10.1128/JCM.43.9.4328-4335.2005
Singh, A., Goering, R. V., Simjee, S., Foley, S. L., and Zervos, M. J. (2006). Application of molecular techniques to the study of hospital infection. Clin. Microbiol. Rev. 19, 512–530. doi: 10.1128/CMR.00025-05
Thong, K. L., Goh, Y. L., Radu, S., Noorzaleha, S., Yasin, R., Koh, Y. T., et al. (2002). Genetic diversity of clinical and environmental strains of Salmonella enterica serotype Weltevreden isolated in Malaysia. J. Clin. Microbiol. 40, 2498–2503. doi: 10.1128/jcm.40.7.2498-2503.2002
Tomaschek, F., Higgins, P. G., Stefanik, D., Wisplinghoff, H., and Seifert, H. (2016). Head-to-Head comparison of two multi-locus sequence typing (MLST) schemes for characterization of Acinetobacter baumannii outbreak and sporadic isolates. PLoS One 11:e0153014. doi: 10.1371/journal.pone.0153014
Vasudevan, A., Mukhopadhyay, A., Goh, E. Y., Li, J., and Tambyah, P. A. (2013). Risk factors for infection/colonization caused by resistant Gram negative bacilli in critically ill patients (an observational study of 1633 critically ill patients). Prev. Med. 57, S70–S73. doi: 10.1016/j.ypmed.2012.12.003
Vazquez-Lopez, R., Solano-Galvez, S. G., Juarez Vignon-Whaley, J. J., Abello Vaamonde, J. A., Padro Alonzo, L. A., Rivera Resendiz, A., et al. (2020). Acinetobacter baumannii resistance: a real challenge for clinicians. Antibiotics 9:205. doi: 10.3390/antibiotics9040205
WHO (Eds) (2017). “WHO Guidelines Approved by the Guidelines Review Committee,” in Guidelines for the Prevention and Control of Carbapenem-Resistant Enterobacteriaceae, Acinetobacter baumannii and Pseudomonas aeruginosa in Health Care Facilities, (Geneva: World Health Organization Copyright © World Health Organization 2017).
Ye, D., Shan, J., Huang, Y., Li, J., Li, C., Liu, X., et al. (2015). A gloves-associated outbreak of imipenem-resistant Acinetobacter baumannii in an intensive care unit in Guangdong, China. BMC Infect. Dis. 15:179. doi: 10.1186/s12879-015-0917-9
Zarrilli, R., Pournaras, S., Giannouli, M., and Tsakris, A. (2013). Global evolution of multidrug-resistant Acinetobacter baumannii clonal lineages. Int. J. Antimicrob. Agents 41, 11–19. doi: 10.1016/j.ijantimicag.2012.09.008
Keywords: Acinetobacter baumannii, epidemiology, whole-genome sequencing, intensive care unit, multi-drug resistance
Citation: Mao P, Deng X, Yan L, Wang Y, Jiang Y, Zhang R, Yang C, Xu Y, Liu X and Li Y (2021) Whole-Genome Sequencing Elucidates the Epidemiology of Multidrug-Resistant Acinetobacter baumannii in an Intensive Care Unit. Front. Microbiol. 12:715568. doi: 10.3389/fmicb.2021.715568
Received: 27 May 2021; Accepted: 26 August 2021;
Published: 13 September 2021.
Edited by:
Michael Kemp, University of Southern Denmark, DenmarkReviewed by:
Dinesh Sriramulu, Independent Researcher, Chennai, IndiaUga Dumpis, Pauls Stradiņš Clinical University Hospital, Latvia
Copyright © 2021 Mao, Deng, Yan, Wang, Jiang, Zhang, Yang, Xu, Liu and Li. This is an open-access article distributed under the terms of the Creative Commons Attribution License (CC BY). The use, distribution or reproduction in other forums is permitted, provided the original author(s) and the copyright owner(s) are credited and that the original publication in this journal is cited, in accordance with accepted academic practice. No use, distribution or reproduction is permitted which does not comply with these terms.
*Correspondence: Yimin Li, ZHJ5aW1pbmxpQHZpcC4xNjMuY29t