- 1State Key Laboratory of Animal Nutrition, College of Animal Science and Technology, China Agricultural University, Beijing, China
- 2Institute of Subtropical Agriculture, Chinese Academy of Sciences, Changsha, China
- 3Hubei Horwath Biotechnology Co., Ltd., Xianning, China
The current study was conducted to investigate the effect of dietary tributyrin (TB) administration on the intestinal and growth performances in Arbor Acres (AA) broilers under an isocaloric feeding regime. A total of 540 day-old healthy AA broilers were randomly assigned to five treatments with 12 replicates (pens) per treatment and nine birds per pen for 42 days. The dietary treatments were basal diet (control) and basal diet with TB at doses of 0.23 g/kg (TB1), 0.46 g/kg (TB2), 0.92 g/kg (TB3), and 1.84 g/kg (TB4). Particularly, to achieve the isocaloric and cost-saving experimental diets, soybean oil was replaced by the TB product (Eucalorie®) with equivalent metabolic energy contents, and the formulas were rebalanced with zeolite to get the sum of all the feed ingredients to 100%. On days 21 and 42, after weighing, the birds (one bird per replicate) whose body weight was close to the replicate average were euthanized to investigate the effect of dietary TB on intestinal morphology, intestinal bacterial population, and short-chain fatty acid contents. The results revealed that dietary TB administration increased the average daily gain, gain/feed ratio, and European broiler index (P < 0.05) and improved the intestinal morphology (P < 0.05) as indicated by higher villus height and the ratios of villus height/crypt depth in broilers. The incremental levels of TB increased the ileal Lactobacillus content (P = 0.05) and cecal Bacillus content (P = 0.02), respectively. Moreover, dietary TB administration also increased the contents of most of the selected short-chain fatty acids in ileal and cecal digesta (P < 0.05). Collectively, dietary TB administration quadratically improved the growth performance, intestinal morphology, beneficial bacterial population, and short-chain fatty acid levels under the isocaloric feeding regime, indicating better profit return potential in practical poultry operation.
Introduction
Unlike beef and pork, poultry meat is widely consumed due to its favorable and nutritive value and because it is free from cultural or religious restrictions (Henchion et al., 2014). Thus, poultry production is facing a promising yet challenging future accompanying the increase of global demand for human-edible protein source due to the ever-increasing human population (Wu et al., 2014; Yadav and Jha, 2019). Such demand attracted widespread concerns regarding the health and welfare for optimal growth performance and economic profit return in poultry production.
Intestinal health is of particular importance for the health and welfare of broilers. In animals, the intestinal tract is not only the place for nutrient digestion and absorption but also the largest immune organ for the protection of the body from adverse challenges, such as infectious pathogenic bacteria, virus, and parasites (Sunkara et al., 2011; Kiela and Ghishan, 2016; Citi, 2018); therefore, there is a growing need worldwide to explore strategies able to maintain the integrity of intestinal morphology and modulate the intestinal function. In this regard, butyrate would be a promising choice, as it has been well demonstrated to play fundamental roles in modulating the intestinal structure and microbial composition (Yang et al., 2018; Wang et al., 2019a; Sotira et al., 2020). In addition, aside from its primary function as a preferred energy source over glucose and glutamine for colonic epithelial cells, butyrate is a strong mitosis promoter and a differentiation agent for intestinal epithelial cells (Guilloteau et al., 2010), thus enhancing the intestinal barrier by facilitating tight junction assembly (Peng et al., 2009; Taba et al., 2020). Furthermore, butyrate derivate products could effectively induce the immune response by upregulating the gene expression of intestinal host defense peptides and controlling the proliferation of bacterial pathogens in chickens (Sunkara et al., 2011, 2012). With the above features, butyrate derivate products proved to be a valid aid for gut health maintenance.
Butyrate sodium and tributyrin (TB) are the most commonly available butyrate derivate products; however, their bio-beneficial effects on human and animals are different. Previous clinical and pharmacologic research demonstrated that TB showed advantages over sodium butyrate (Edelman et al., 2003). For example, TB could be easily absorbed to the systemic circulation and increase the butyrate concentration in the portal vein. With its longer metabolic half-life, a sufficient amount of TB could be delivered to the target tissues or organs for the initiation of beneficial effects (Chen and Breitman, 1994; Conley et al., 1998; Miyoshi et al., 2011). Thus, TB has been defined as “a stable and rapidly absorbed prodrug of butyric acid” (Gaschott et al., 2001). Interestingly, the intestinal bacterial composition could be modified in response to different butyrate sources. For example, dietary TB treatment significantly enriched the Bacteroidetes while reducing the Proteobacteria at the phylum level in broilers (Moquet, 2018). Particularly, TB efficiently induces fetal hemoglobin and human cathelicidin expression under physiologically available concentration compared with sodium butyrate, demonstrating that its beneficial bio-efficiency is superior to that of sodium butyrate (Witt et al., 2000; Jiang et al., 2013). Besides, except for acting as an energy source for animals, dietary butyrate treatment improved the apparent metabolizable energy corrected for nitrogen in broilers (Kaczmarek et al., 2016), showing an energy-sparing effect. This is of great economic interest in practical application, because the contents of energy ingredients in the formula could be reduced for cost-saving purposes. The objective of the present study was, therefore, to determine the changes in growth performance, intestinal morphology, and selected bacterial and short-chain fatty acid (SCFA) profiles in response to dietary TB treatment by partially following the isocaloric and cost-saving feed additives-supplementing strategy in broilers.
Materials and Methods
Animals, Experimental Design, and Diets
The protocol for the animal experiment was approved by the Animal Care and Use Committee of the China Agricultural University (Ethical experimental statement no. AW13501202-2-1).
A total of 540 day-old healthy Arbor Acres (AA) broiler chickens (half male and half female) were allocated equally on the basis of weight of origin into five dietary treatments in a randomized complete block design. The dietary treatments were the basal diet-fed group (control) and the groups fed with basal diet supplemented with TB, namely, groups TB1 to TB4. The dietary TB levels were 0.23 g/kg (TB1), 0.46 g/kg (TB2), 0.92 g/kg (TB3), and 1.84 g/kg (TB4). There were 12 replicates per treatment with nine birds per replicate (cage). Feed ingredients and feeding program are summarized in Table 1. Particularly, in order to achieve an isocaloric level in each formula, the soybean oil was replaced by the TB product (Eucalorie®) with equivalent metabolic energy content, and the formulas were rebalanced with zeolite to get the sum of all the feed ingredients to 100%. The TB product (Eucalorie®) contains 92% TB, with a metabolic energy value of 8 MCal/kg for broilers, and was kindly contributed by Hubei Horwath Biotechnology Co., Ltd., China. No antibiotics were used throughout the animal trial.
The temperature was maintained at 32°C for 5 days and then gradually reduced to 22°C, in keeping with normal brooding practice. A 23 h/day constant-light regime was applied through the entire animal trial. Birds were vaccinated against coccidiosis at 1 day of age following the regular vaccination program. Feed and water were freely available. The body weight was recorded individually prior to feeding in the morning on days 21 and 42, and cumulative feed consumption per cage was recorded at the same time. The dead bird numbers were recorded daily as well. The phase mortality, average daily weight gain (ADG), average daily feed intake (ADFI), feed conversion ratio (FCR), and the European broiler index (EBI) were calculated following a previous method (Kryeziu et al., 2018).
Intestinal Sections and Digesta Sampling
On days 21 and 42, after being weighed, the birds (one bird per cage) whose body weight was close to the cage average were selected and euthanized for sample collection. Middle duodenal and jejunal, as well as distal ileal, sections (approximately 2 cm in length) were collected followed by removal of digesta and connective adipose tissues, rinsed with pre-cold physiological saline (0.9% saline), and immediately fixed with 4% polyoxymethylene for later intestinal morphological examination. Approximately 2 g of contents from the distal ileum and cecum was collected and aseptically emptied in 5-ml sterile tubes for later bacterial enumeration. The remaining digesta in the distal ileum and cecum were collected in 50-ml sterile tubes, immediately frozen with liquid nitrogen, and stored at −80°C until the later analysis of volatile fatty acid (VFA) concentrations.
Intestinal Histomorphology Analysis
To evaluate the intestinal histomorphology, selected intestinal tissues were fixed with 4% polyoxymethylene for 24 h and then embedded in petrolin and sectioned into 2–3-μm sections. The sections were stained with hematoxylin first, counterstained with eosin, and finally observed under an optical microscope following previous procedures (Wang et al., 2018). The villus height (the distance from the apex of the villus to the junction of the villus and crypt) and crypt depth (the distance from the junction to the basement membrane of the epithelial cells at the bottom of the crypt) were measured on 10 intact, well-oriented villi per intestinal section, and then the ratio of villus height/crypt depth was calculated.
Intestinal Bacterial Quantitation
Conventional agar plating was used for enumerating viable bacteria. The selected intestinal bacterial quantitation, including Bacillus, Lactobacillus, and Coliforms, was performed following previous procedures with minor modifications (Proietti et al., 2009; Hu et al., 2012; Yin et al., 2014). Briefly, 0.5 g of fresh intestinal content was plated directly on appropriate selective agar media, in duplicate, by Eddy Jet Spiral Plater (Neutec Group Inc., Farmingdale, NY, United States) after a series of 10-fold dilution in physiological saline supplemented with 0.1% peptone with a detection limit of 100 CFU/g digesta. The enumeration of Bacillus was assayed on tryptone soy agar after aerobic incubation at 37°C for 24 h, that of Lactobacillus was determined on de Man, Rogosa, and Sharpe agar anaerobically incubated at 37°C for 48 h, and that of Coliform bacteria was enumerated on MacConkey agar following aerobic incubation at 37°C for 24 h. The bacterial enumeration was expressed in colony-forming units (log10 CFU/g of fresh digesta).
Intestinal SCFA Measurement
The concentrations of SCFAs, including acetic acid, propionic acid, isobutyric acid, butyric acid, isovaleric acid, valeric acid, and total VFAs, in the digesta of selected intestinal sections were determined according to previous procedures with minor modifications (Park and Park, 2014; Park and Kim, 2017). Briefly, 2 g of digesta was placed in a screw-cap tube and mixed with 4 ml of ultra-pure water and then homogenized and centrifuged at 4,000 rpm for 10 min at 4°C. Approximately 1 ml of the supernatant was aliquoted into another ampule and acidified by adding 0.2 ml of 25% HPO3 solution, mixed well and put on an ice bath for 3 h, and then centrifuged at 10,000 × g for 10 min at 4°C, and 1.0 μl of the supernatant was collected and injected into a gas chromatographic system (Model GC-15A, Shimadzu Corp., Kyoto, Japan), equipped with a column (Supelco, Inc., Bellefonte, PA, United States) filled with 10% SP-1000 and a flame ionization detector. The initial temperatures of the oven, injector, and flame ionization detector were 100, 240, and 250°C, respectively. The column was operated at 100–150°C, along with highly purified N2 (1.8 ml/min) as a carrier gas. The results were expressed as μmol/g digesta.
Statistical Analysis
The data were analyzed using the general linear model procedure of the SPSS program (version 17.0) for analysis of variance. The nature of the response to increasing concentration of dietary TB was determined by polynomial contrasts. The model included linear and quadratic contrasts for effects of dietary TB. The multiple comparisons were tested by the Tukey–Kramer method to determine differences among treatment means. A P-value < 0.05 was taken to indicate statistical significance between treatments.
Results
Growth Performance
The FCR in TB3 and TB4 groups were lower than that in the other three groups (P < 0.05), although no significant differences of ADG and ADFI were observed among treatments (P > 0.05) in the first feeding period (0–21 days). The ADG and ADFI in the second (22–42 days) and overall (0–42 days) feeding periods as well as the FCR in the second feeding period were quadratically improved in response to dietary TB administration (P < 0.05. Particularly, the ADG and ADFI were highest in the TB4 group (P < 0.05), while the FCR was lowest mainly in the TB3 and TB4 groups (P < 0.05) in these feeding periods. The results are shown in Table 2.
Histomorphology of Small Intestinal Sections
Dietary TB treatment ameliorated the duodenal and jejunal villus length, crypt depth, and villus length/crypt depth ratio at both 21 and 42 days of ages (P < 0.05). The ileal villus length at 21 days of age and the ileal crypt depth at 42 days of age in the TB treatment groups were improved when compared with those in the control group (P < 0.05). Particularly, the jejunal villus length; duodenal and jejunal crypt depth; duodenal, jejunal, and ileal villus length/crypt depth ratios at 21 days of age; and the duodenal villus length and villus length/crypt depth ratio at 42 days of age were quadratically changed in association with the dietary TB administration (P < 0.05), with the most effective being observed in the TB3 and TB4 groups. The results are shown in Table 3.
Bacterial Populations in Ileal and Cecal Digesta
Dietary TB treatment significantly (P < 0.05) affected the selected bacterial (Bacillus, Lactobacillus, and Coliforms) counts in ileal and cecal digesta (Table 4). Specifically, the ileal Bacillus and Lactobacillus counts and cecal Coliforms counts at both 21 and 42 days of age, the cecal Lactobacillus counts at 21 days of age, and the cecal Bacillus counts at 42 days of age were quadratically changed in response to the dietary TB administration (P < 0.05), with the most affected being mainly in the TB3 and TB4 groups.
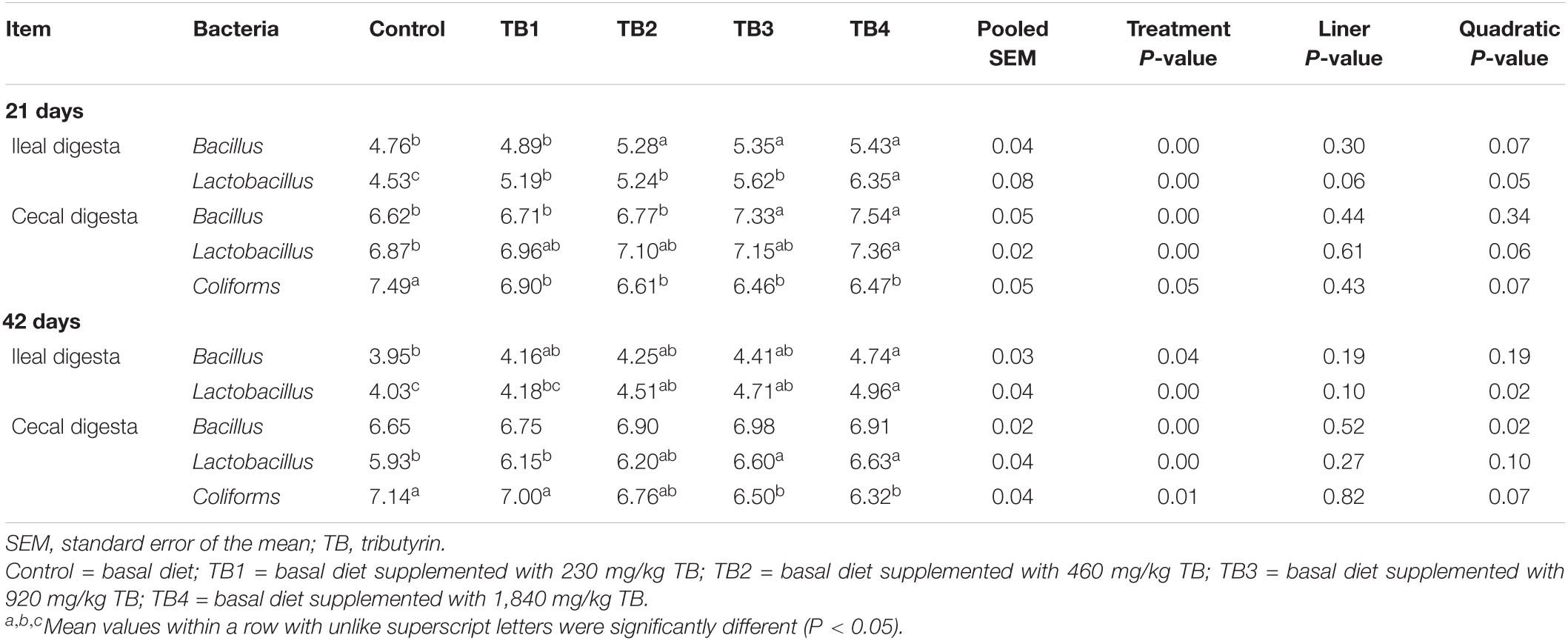
Table 4. Effect of dietary TB on ileal and cecal bacterial populations (log10 CFU/g fresh digesta) in broilers.
SCFA Concentrations in Ileal and Cecal Digesta
Dietary TB treatment significantly affected the ileal acetic acid content at 21 days of age (P < 0.05). The ileal propionic acid content at both 21 and 42 days of age and the cecal contents of all the selected SCFAs at both 21 and 42 days of age were improved in response to dietary TB treatment when compared with those in the control group (P < 0.05). Notably, the ileal acetic acid content at 21 days of age, ileal propionic acid content, and cecal propionic acid, butyric acid, and isovaleric acid contents at both 21 and 42 days of age, as well as the cecal acetic acid, isobutyric acid, valeric acid, and total cecal VFAs at 42 days of age were changed quadratically in response to the dietary TB administration (P < 0.05), with the most effective being in the TB4 group. The results are shown in Table 5.
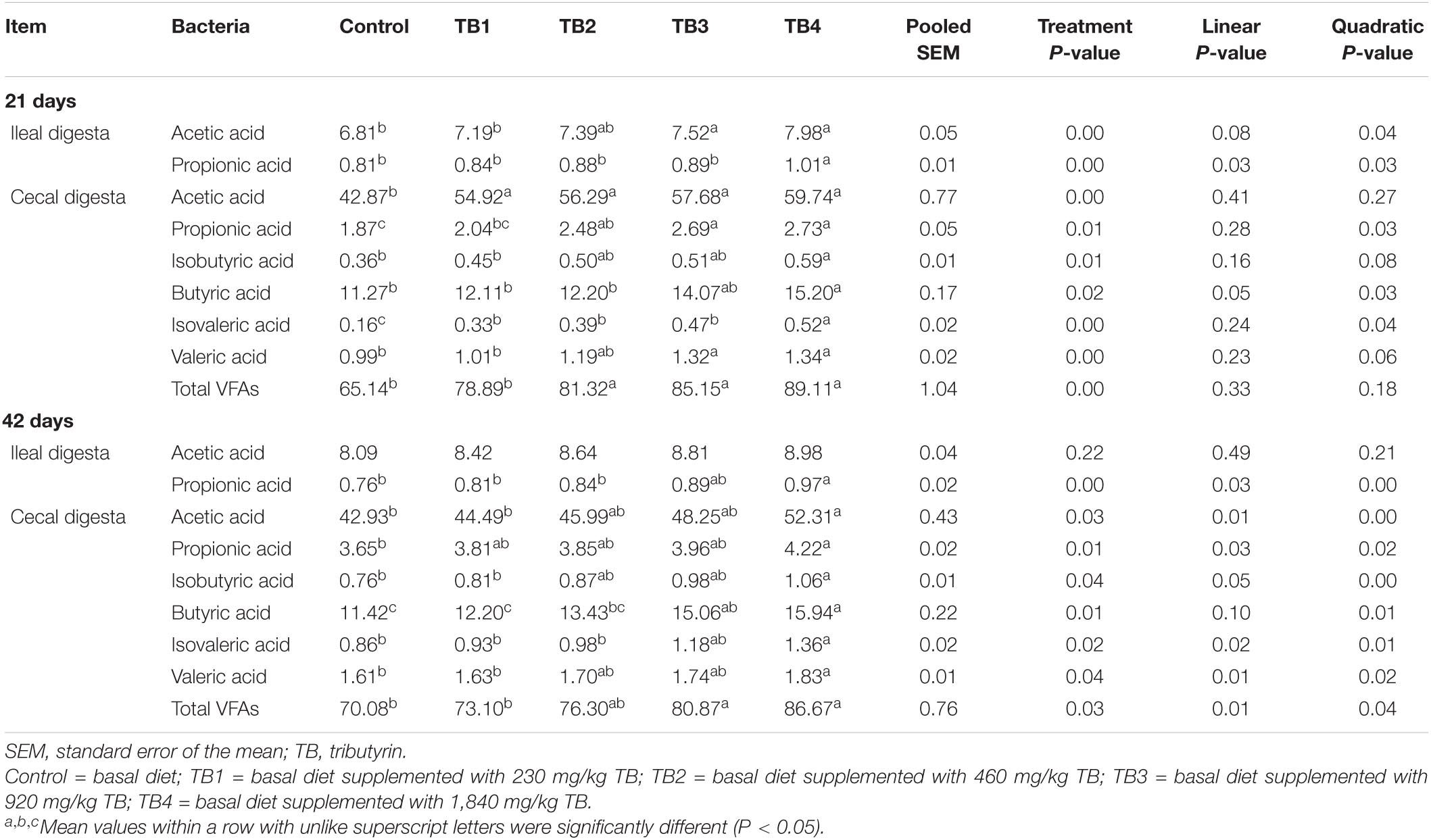
Table 5. Effect of dietary TB on SCFA concentration (μmol/g fresh digesta) in ileal and cecal digesta in broilers.
Discussion
Glyceryl butyrate has been demonstrated to beneficially improve the growth performance and carcass yield in broiler chickens (Leeson et al., 2005; Mansoub et al., 2011; Yin et al., 2016). Such effect is superior to that of probiotics and prebiotics as well as their combination (Taherpour et al., 2009), which have brought great success in the industrial poultry operation. However, with the inevitable yet sustained commercial competition, research scientists and technical experts are keen to explore novel strategies to squeeze more beneficial returns in poultry operation. One promising strategy is to supplement optimal doses of butyrate to the feed formula without increasing the feed cost burden. This is particularly challenging as solid scientific proofs are needed. It is of great interest that dietary butyrate intervention improved the apparent metabolizable energy (Kaczmarek et al., 2016), ileal energy digestible coefficient (Liu et al., 2017), and apparent ileal digestibility of methionine (Moquet et al., 2018) in broilers. In addition, the present study also showed promising results as the ADG and EBI were improved following optimal dietary TB administration at 1,840 mg/kg under the cost-saving formulating regime. Furthermore, several aggressive yet pioneered research showed that better weight gain and feed/gain ratio could be achieved when birds were fed diet with reduced crude protein and/or metabolic energy contents and supplemented with butyrate products (M’Sadeq et al., 2016; Bortoluzzi et al., 2017; Petrilla et al., 2018; Mátis et al., 2019). Taken together, these results provided scientific proofs regarding the application of butyrate for cost-saving and better profit return formulas in poultry operation.
The gastrointestinal tract presents a heavy burden for the digestive and absorptive functions as well as disease resistance ability in animals (Mitchell and Moretó, 2006; Timbermont et al., 2010; Wasti et al., 2020). The improvement of intestinal villi height and villus length/crypt depth ratio is directly linked to better disease prevention, nutrient absorption, and growth performance in animals (Sen et al., 2012). In the present study, the intestinal villi height and villus length/crypt depth ratios were effectively improved in the TB3 and TB4 groups at both 21 and 42 days, demonstrating better morphological and functional improvement of the small intestine under the current TB administration regime. This is of significant importance for broiler production as it ensures that birds achieve a heavier marketing weight in a given time period. Interestingly, higher intestinal villi length and villus length/crypt depth ratios were correlated with higher intestinal weight and longer intestinal length in response to butyrate product administration in broilers (Gong et al., 2011; Lan et al., 2020). Particularly, a heavier gastrointestinal tract was observed in the TB-fed broilers when compared with that in the butyric acid- and sodium butyrate-fed ones (Moquet, 2018), indicating that TB is superior to butyric acid and sodium butyrate in stimulating the growth and development of the gastrointestinal tract in broilers. Besides, TB also effectively protected the intestine from lethal infection-caused hemorrhage and necrosis (Gu et al., 2017; Onrust et al., 2020) and severe oxidative stress-induced damages (Wang et al., 2019b) in broiler and pigs. Collectively, these scientific evidences well supported the improvement of growth performance and well-being in the TB-fed birds.
Gut microbiota is a strong determinant of host physiology and health status; particularly, it plays a critical role in maintaining the normal physiological function of the intestine (Sekirov et al., 2010; Le Chatelier et al., 2013). Previous research has revealed that dietary butyrate treatment beneficially reduced some specific pathogenic bacterial contents, such as Clostridium perfringens and Salmonella enteritidis, in broilers (Shojadoost et al., 2012; Onrust et al., 2020). Furthermore, a previous study with pyrosequencing techniques revealed that dietary glyceryl butyrate effectively reduced the abundance of pathogenic Mollicutes and Holdemania in the cecum of broilers (Yang et al., 2018). Because many Mollicutes members cause diseases (such as colitis) in humans and animals (Brown et al., 2010), while Holdemania has also been reported to be associated with unhealthy ceca in animals (Bailey, 2013), the reduction in the abundance of Mollicutes and Holdemania with glyceryl butyrate treatment demonstrated its beneficial effects on chicken intestinal health. Interestingly, the current results were in agreement with these findings as dietary TB administration improved the beneficial Bacillus and Lactobacillus contents while reducing the pathogenic Coliforms contents in the ileum and cecum and thus secured better health and well-being in broilers.
In the present study, the cecal concentrations of SCFAs (mainly acetic acid, propionic acid, and butyric acid) increased markedly regardless of the age in days, with higher doses of TB treatment being the most effective. Similar results were observed with higher doses of sodium butyrate treatment in broilers (Wu et al., 2018). However, differences also existed regarding the changes of these SCFA contents in response to different levels of dietary TB and/or sodium butyrate treatment. Particularly, the cecal acetic acid, propionic acid, and butyric acid contents were increased steadily with the increase of dietary TB levels. This is probably because the TB could be stably and rapidly absorbed to the blood circulation (Gaschott et al., 2001) and delivered to the whole gastrointestinal tissues through the arterial blood stream (Edelman et al., 2003; Miyoshi et al., 2011). With its longer metabolic half-life, TB could effectively stimulate the synthesis of host defense peptides in gastrointestinal cells and stimulate the proliferation of beneficial bacteria as well as prohibit that of pathogenic ones (Cuperus et al., 2013; Robinson et al., 2015; 2018). In addition, the increase of intestinal SCFA concentrations is associated with lower intestinal pH value (Meimandipour et al., 2011), which is also beneficial for the growth of intestinal probiotic bacteria in broilers. Besides, butyrate and/or propionate presence in the ceca and colon resulted in significantly heavier jejuna and longer small intestinal and total tract retention times (Moquet et al., 2018); therefore, the higher intestinal SCFA concentrations were positively correlated with the improvement of intestinal histomorphology and growth performance in the current study.
In summary, the present study has shown that dietary TB administration significantly improved the growth performance, intestinal morphology, beneficial bacterial population, and SCFA levels in a dose-dependent quadratic manner under an isocaloric and cost-saving feeding program. These findings provide a solid scientific reference for better economic profit return potential in practical poultry operation.
Data Availability Statement
The original contributions presented in the study are included in the article/supplementary material, further inquiries can be directed to the corresponding author/s.
Ethics Statement
The animal study was reviewed and approved by China Agricultural University Laboratory Animal Welfare and Animal Experimental Ethical Committee.
Author Contributions
QH designed the study. QH and BL conducted the animal trial and sample and data collection. QH and FY analyzed the data and drafted the manuscript. YG and YY reviewed the manuscript. All authors approved the final version of the manuscript.
Funding
This research was supported by the Hunan High-Level Talent Gathering Project-Innovative Talents (No. 2018RS3111).
Conflict of Interest
QH, FY, and BL were employed by company: Hubei Horwath Biotechnology Co., Ltd.
The remaining authors declare that the research was conducted in the absence of any commercial or financial relationships that could be construed as a potential conflict of interest.
Publisher’s Note
All claims expressed in this article are solely those of the authors and do not necessarily represent those of their affiliated organizations, or those of the publisher, the editors and the reviewers. Any product that may be evaluated in this article, or claim that may be made by its manufacturer, is not guaranteed or endorsed by the publisher.
References
Bailey, R. (2013). Gut health in poultry-the world within. Bogota: Avicol Escuela Técnica Internacional.
Bortoluzzi, C., Pedroso, A. A., Mallo, J. J., Puyalto, M., Kim, W. K., and Applegate, T. J. (2017). Sodium butyrate improved performance while modulating the cecal microbiota and regulating the expression of intestinal immune-related genes of broiler chickens. Poult. Sci. 96, 3981–3993. doi: 10.3382/ps/pex218
Brown, D. R., May, M., Bradbury, J. M., and Johansson, K. E. (2010). Class I. Mollicutes in Bergey’s manual of systematic bacteriology, Vol. 4. Berlin: Springer, 568–724.
Chen, Z. X., and Breitman, T. R. (1994). Tributyrin: a prodrug of butyric acid for potential clinical application in differentiation therapy. Cancer Res. 54, 3494–3499.
Citi, S. (2018). Intestinal barriers protect against disease. Science 359, 1097–1098. doi: 10.1126/science.aat0835
Conley, B. A., Egorin, M. J., Tait, N., Rosen, D. M., Sausville, E. A., Dover, G., et al. (1998). Phase I study of the orally administered butyrate prodrug, tributyrin, in patients with solid tumors. Clin. Cancer Res. 4, 629–634.
Cuperus, T., Coorens, M., van Dijk, A., and Haagsman, H. P. (2013). Avian host defense peptides. Dev. Comp. Immunol. 41, 352–369. doi: 10.1016/j.dci.2013.04.019
Edelman, M. J., Bauer, K., Khanwani, S., Tait, N., Trepel, J., Karp, J., et al. (2003). Clinical and pharmacologic study of tributyrin: an oral butyrate prodrug. Cancer Chemother. Pharmacol. 51, 439–444. doi: 10.1007/s00280-003-0580-5
Gaschott, T., Steinhilber, D., Milovic, V., and Stein, J. (2001). Tributyrin, a stable and rapidly absorbed prodrug of butyric acid, enhances antiproliferative effects of dihydroxycholecalciferol in human colon cancer cells. J. Nutr. 131, 1839–1843. doi: 10.1093/jn/131.6.1839
Gong, J., Yin, F., Yang, X., Yang, C., Yu, H., Yin, Y., et al. (2011). “Potential of butyrate glycerides as an alternative to dietary antibiotic: a mechanistic study with broilers from nutrition perspectives,” in Conference poster, (Washington, D.C: USDA).
Gu, Y., Song, Y., Yin, H., Lin, S., Zhang, X., Che, L., et al. (2017). Dietary supplementation with tributyrin prevented weaned pigs from growth retardation and lethal infection via modulation of inflammatory cytokines production, ileal expression, and intestinal acetate fermentation. J. Anim. Sci. 95, 226–238. doi: 10.2527/jas.2016.0911
Guilloteau, P., Martin, L., Eeckhaut, V., Ducatelle, R., Zabielski, R., and Imerseel, F. V. (2010). From the gut to the peripheral tissues: The multiple effects of butyrate. Nutr. Res. Rev. 23, 366–384. doi: 10.1017/S0954422410000247
Henchion, M., McCarthy, M., Resconi, V. C., and Troy, D. (2014). Meat consumption: trends and quality matters. Meat. Sci. 98, 561–568. doi: 10.1016/j.meatsci.2014.06.007
Hu, C. H., Gu, L. Y., Luan, Z. S., Song, J., and Zhu, K. (2012). Effects of montmorillonite-zinc oxide hybrid on performance diarrhea, intestinal permeability and morphology of weanling pigs. Anim. Feed Sci. Technol. 177, 108–115. doi: 10.1016/j.anifeedsci.2012.07.028
Jiang, W., Sunkara, L. T., Zeng, X., Deng, Z., Myers, S. M., and Zhang, G. (2013). Differential regulation of human cathelicidin LL-37 by free fatty acids and their analogs. Peptides 50, 129–138. doi: 10.1016/j.peptides.2013.10.008
Kaczmarek, S. A., Barri, A., Hejdysz, M., and Rutkowski, A. (2016). Effect of different doses of coated butyric acid on growth performance and energy utilization in broilers. Poult. Sci. 95, 851–859. doi: 10.3382/ps/pev382
Kiela, P. R., and Ghishan, F. K. (2016). Physiology of intestinal absorption and secretion. Best. Pract. Res. Clin. Gastroenterol. 30, 145–159. doi: 10.1016/j.bpg.2016.02.007
Kryeziu, A. J., Mestani, N., Berisha, Sh, and Kamberi, M. A. (2018). The European performance indicators of broiler chickens as influenced by stocking density and sex. Agronomy Res. 16, 483–491. doi: 10.15159/ar.18.040
Lan, R., Li, S., Chang, Q., An, L., and Zhao, Z. (2020). Sodium butyrate enhances growth performance and intestinal development in broilers. Czech J. Anim. Sci. 65, 1–12. doi: 10.17221/190/2019-CJAS
Le Chatelier, E., Nielsen, T., Qin, J., Prifti, E., Hildebrand, F., Falony, G., et al. (2013). Richness of human gut microbiome correlates with metabolic markers. Nature 500, 541–546. doi: 10.1038/nature12506
Leeson, S., Namkung, H., Antongiovanni, M., and Lee, E. H. (2005). Effect of butyric acid on the performance and carcass yield of broiler chickens. Poul. Sci. 84, 1418–1422. doi: 10.1093/ps/84.9.1418
Liu, J. D., Bayir, H. O., Cosby, D. E., Cox, N. A., Williams, S. M., and Fowler, J. (2017). Evaluation of encapsulated sodium butyrate on growth performance, energy digestibility, gut development, and Salmonella colonization in broilers. Poult. Sci. 96, 3638–3644. doi: 10.3382/ps/pex174
M’Sadeq, S. A., Swick, R. A., Choct, M., and Wu, S. B. (2016). The role of coated sodium butyrate on performance of broilers fed high protein and reduced energy diets. J. Appl. Anim. Nutr. 4:E2. doi: 10.1017/jan.2015.13
Mansoub, N. H., Rahimpour, K., Majedi asl, L., Mohammad Nezhady, M. A., Zabihi, S. L., and Mohammadi Kalhor, M. (2011). Effect of different level of butyric acid glycerides on performance and serum composition of broiler chickens. World J. Zool. 6, 179–182.
Mátis, G., Petrilla, J., Kulcsár, A., van den Bighelaar, H., Boomsma, B., Neogrády, Z., et al. (2019). Effects of dietary butyrate supplementation and crude protein level on carcass traits and meat composition of broiler chickens. Arch. Anim. Breed. 62, 527–536. doi: 10.5194/aab-62-527-2019
Meimandipour, A., Soleimanifarjam, A., Azhar, K., Hair-Bejo, M., Shuhaimi, M., Nateghi, L., et al. (2011). Age effects on short chain fatty acids concentrations and pH values in the gastrointestinal tract of broiler chickens. Arch. Geflügelk 5, S164–S168.
Mitchell, M. A., and Moretó, M. (2006). “Absorptive function of the small intestine: adaptations meeting demand,” in Avian Gut Function in Health and Disease, 1st Edn, ed. G. C. Perry (London: CAB International), 43–64. doi: 10.1079/9781845931803.0043
Miyoshi, M., Sakaki, H., Usami, M., Iizuka, N., Shuno, K., Aoyama, M., et al. (2011). Oral administration of tributyrin increases concentration of butyrate in the portal vein and prevents lipopolysaccharide-induced liver injury in rats. Clin. Nutr. 30, 252–258. doi: 10.1016/j.clnu.2010.09.012
Moquet, P. C. A. (2018). Butyrate in broiler diets: impact of butyrate presence in distinct gastrointestinal tract segments on digestive function, microbiota composition and immune responses. Ph. D. thesis. The Netherlands: Wageningen University.
Moquet, P. C. A., Salami, S. A., Onrust, L., Hendriks, W. H., and Kwakkel, R. P. (2018). Butyrate presence in distinct gastrointestinal tract segments modifies differentially digestive processes and amino acid bioavailability in young broiler chickens. Poult. Sci. 97, 167–176.1. doi: 10.3382/ps/pex279
Onrust, L., Baeyen, S., Haesebrouck, F., Ducatelle, R., and Van Immerseel, F. (2020). Effect of in feed administration of different butyrate formulations on Salmonella Enteritidis colonization and cecal microbiota in broilers. Vet. Res. 51:56. doi: 10.1186/s13567-020-00780-2
Park, B. S., and Park, S. O. (2014). Bifidogenic effects of the oral administration of fly maggot extract on organic acid, cecal microorganisms, thymus and spleen weights and blood lipids in rats. J. Life Sci. 24, 784–790. doi: 10.5352/JLS.2014.24.7.784
Park, S. O., and Kim, W. K. (2017). Effects of betaine on biological functions in meat-type ducks exposed to heat stress. Poult. Sci. 96, 1212–1218. doi: 10.3382/ps/pew416
Peng, L., Li, Z., Green, R. S., Holzman, I. R., and Lin, J. (2009). Butyrate enhances the intestinal barrier by facilitating tight junction assembly via activation of AMP-activated protein kinase in Caco-2 cell monolayers. J. Nutr. 139, 1619–1625. doi: 10.3945/jn.109.104638
Petrilla, J., Mátis, G., Kulcsár, A., Talapka, P., Bíró, E., Mackei, M., et al. (2018). Effect of dietary cereal type, crude protein and butyrate supplementation on metabolic parameters of broilers. Acta Vet. Hung. 66, 408–452. doi: 10.1556/004.2018.037
Proietti, P. C., Bosco, A. D., Hilbert, F., Franciosini, M. P., and Castellini, C. (2009). Evaluation of intestinal bacterial flora of conventional and organic broilers using culture-based methods. Ital. J. Anim. Sci. 8, 51–63. doi: 10.4081/ijas.2009.51
Robinson, K., Deng, Z., Hou, Y., and Zhang, G. (2015). Regulation of the intestinal barrier function by host defense peptides. Front. Vet. Sci. 2:57. doi: 10.3389/fvets.2015.00057
Robinson, K., Ma, X., Liu, Y., Qiao, S., Hou, Y., and Zhang, G. (2018). Dietary modulation of endogenous host defense peptide synthesis as an alternative approach to in-feed antibiotics. Anim. Nutr. 4, 160–169. doi: 10.1016/j.aninu.2018.01.003
Sekirov, I., Russell, S. L., Antunes, L. C. M., and Finlay, B. B. (2010). Gut Microbiota in health and disease. Physiol. Rev. 90, 859–904. doi: 10.1152/physrev.00045.2009
Sen, S., Ingale, S. L., Kim, Y. W., Kim, J. S., Kim, K. H., et al. (2012). Effect of supplementation of Bacillus subtilis LS 1-2 to broiler diets on growth performance, nutrient retention, caecal microbiology and small intestinal morphology. Res. Vet. Sci. 93, 264–268. doi: 10.1016/j.rvsc.2011.05.021
Shojadoost, B., Vince, A. R., and Prescott, J. F. (2012). The successful experimental induction of necrotic enteritis in chickens by Clostridium perfringens: a critical review. Vet. Res. 43:74. doi: 10.1186/1297-9716-43-74
Sotira, S., Dell’Anno, M., Caprarulo, V., Hejna, M., Pirrone, F., Callegari, M. L., et al. (2020). Effects of tributyrin supplementation on growth performance, insulin, blood metabolites and gut microbiota in weaned piglets. Animals 10:726. doi: 10.3390/ani10040726
Sunkara, L. T., Achanta, M., Schreiber, N. B., Bommineni, Y. R., Dai, G., Jiang, W., et al. (2011). Butyrate enhances disease resistance of chickens by inducing antimicrobial host defense peptide gene expression. PLoS One 6:e27225. doi: 10.1371/journal.pone.0027225
Sunkara, L. T., Jiang, W., and Zhang, G. (2012). Modulation of antimicrobial host defense peptide gene expression by free fatty acids. PLoS One 7:e49558. doi: 10.1371/journal.pone.0049558
Taba, M. W., Marques, T. M., Markgren, M., Löfvendahl, L., Brummer, R. J., and Wall, R. (2020). Acute effects of butyrate on induced hyper-permeability and tight junction protein expression in human colonic tissues. Biomolecules 10:766. doi: 10.3390/biom10050766
Taherpour, K., Moravej, H., Shivazad, M., Adibmoradi, M., and Yakhchali, B. (2009). Effects of dietary probiotic, prebiotic and butyric acid glycerides on performance and serum composition in broiler chickens. Afr. J. Biotechnol. 8, 2329–2334.
Timbermont, L., Lanckriet, A., Dewulf, J., Nollet, N., Schwarzer, K., Haesebrouck, F., et al. (2010). Control of Clostridium perfringens-induced necrotic enteritis in broilers by target-released butyric acid, fatty acids and essential oils. Avian. Pathol. 39, 117–121. doi: 10.1080/03079451003610586
Wang, C., Cao, S., Zhang, Q., Shen, Z., Feng, J., Hong, Q., et al. (2019b). Dietary tributyrin attenuates intestinal inflammation, enhances mitochondrial function, and induces mitophagy in piglets challenged with diquat. J. Agric. Food Chem. 67, 1409–1417. doi: 10.1021/acs.jafc.8b06208
Wang, C., Shen, Z., Cao, S., Zhang, Q., Peng, Y., Hong, Q., et al. (2019a). Effects of tributyrin on growth performance, intestinal microflora and barrier function of weaned pigs. Anim. Feed Sci. Technol. 258, 14311. doi: 10.1016/j.anifeedsci.2019.114311
Wang, X., Yu, H., Shan, A., Jin, Y., Fang, H., Zhao, Y., et al. (2018). Toxic effects of Zearalenone on intestinal microflora and intestinal mucosal immunity in mice. Food Agric. Immunol. 29, 1002–1011. doi: 10.1080/09540105.2018.1503233
Wasti, S., Sah, N., and Mishra, B. (2020). Impact of heat stress on poultry health and performances, and potential mitigation strategies. Anim 10:1266. doi: 10.3390/ani10081266
Witt, O., Schmejkal, S., and Pekrun, A. (2000). Tributyrin plus all-trans-retinoic acid efficiently induces fetal hemoglobin expression in human erythroleukemia cells. Am. J. Hematol. 64, 319–321. doi: 10.1002/1096-8652(200008)64
Wu, G., Fanzo, J., Miller, D. D., Pingali, P., Post, M., Steiner, J. L., et al. (2014). Production and supply of high-quality food protein for human consumption: sustainability, challenges, and innovations. Ann. N. Y. Acad. Sci. 1321, 1–19. doi: 10.1111/nyas.12500
Wu, W., Xiao, Z., An, W., Dong, Y., and Zhang, B. (2018). Dietary sodium butyrate improves intestinal development and function by modulating the microbial community in broilers. PLoS One 13:e0197762. doi: 10.1371/journal.pone.0197762
Yadav, S., and Jha, R. (2019). Strategies to modulate the intestinal microbiota and their effects on nutrient utilization, performance, and health of poultry. J. Anim. Sci. Biotechnol. 10:2. doi: 10.1186/s40104-018-0310-9
Yang, X., Yin, F., Yang, Y., Lepp, D., Yu, H., Ruan, Z., et al. (2018). Dietary butyrate glycerides modulate intestinal microbiota composition and serum metabolites in broilers. Sci. Rep. 8:4940. doi: 10.1038/s41598-018-22565-6
Yin, F., Farzan, A., Wang, Q., Yu, H., Yin, Y., Hou, Y., et al. (2014). Reduction of Salmonella enterica serovar Typhimurium DT104 infection in experimentally challenged weaned pigs fed a lactobacillus-fermented feed. Foodborne Pathog. Dis. 11, 628–634. doi: 10.1089/fpd.2013.1676
Keywords: tributyrin, growth performance, intestinal morphology, intestinal bacterial population, intestinal short-chain fatty acids, broiler
Citation: Hu Q, Yin F, Li B, Guo Y and Yin Y (2021) Dietary Tributyrin Administration Improves Intestinal Morphology and Selected Bacterial and Short-Chain Fatty Acid Profiles in Broilers Under an Isocaloric Feeding Regime. Front. Microbiol. 12:715712. doi: 10.3389/fmicb.2021.715712
Received: 27 May 2021; Accepted: 12 July 2021;
Published: 04 August 2021.
Edited by:
Hauke Smidt, Wageningen University and Research, NetherlandsReviewed by:
Jaime Parra-Suescún, National University of Colombia, Medellin, ColombiaTarique Hussain, Nuclear Institute for Agriculture and Biology, Pakistan
Copyright © 2021 Hu, Yin, Li, Guo and Yin. This is an open-access article distributed under the terms of the Creative Commons Attribution License (CC BY). The use, distribution or reproduction in other forums is permitted, provided the original author(s) and the copyright owner(s) are credited and that the original publication in this journal is cited, in accordance with accepted academic practice. No use, distribution or reproduction is permitted which does not comply with these terms.
*Correspondence: Yulong Yin, eWlueXVsb25nQGlzYS5hYy5jbg==