- 1CAS Key Laboratory of Agro-Ecological Processes in Subtropical Region, Hunan Provincial Key Laboratory of Animal Nutritional Physiology and Metabolic Process, National Engineering Laboratory for Pollution Control and Waste Utilization in Livestock and Poultry Production, Institute of Subtropical Agriculture, Chinese Academy of Sciences, Changsha, China
- 2College of Advanced Agricultural Sciences, University of Chinese Academy of Sciences, Beijing, China
Here, we used Bama Xiang mini-pigs to explore the effects of different dietary β-hydroxy-β-methylbutyrate (HMB) levels (0, 0.13, 0.64 or 1.28%) on lipid metabolism of adipose tissue. Results showed that HMB decreased the fat percentage of pigs (linearly, P < 0.05), and the lowest value was observed in the 0.13% HMB group. Moreover, the colonic acetic acid concentration and the relative Bacteroidetes abundance were increased in response to HMB supplementation (P < 0.05). Correlation analysis identified a positive correlation between the relative Bacteroidetes abundance and acetic acid production, and a negative correlation between fat percentage and the relative Bacteroidetes abundance or acetic acid production. HMB also upregulated the phosphorylation (p) of AMPKα, Sirt1, and FoxO1, and downregulated the p-mTOR expression. Collectively, these findings indicate that reduced fat percentage in Bama Xiang mini-pigs could be induced by HMB supplementation and the mechanism might be associated with the Bacteroidetes-acetic acid-AMPKα axis.
Introduction
Obesity has increased at an alarming rate over the past years, subsequently resulting in diabetes and other metabolic diseases (Duan Y.H. et al., 2017; Duan Y. et al., 2017). There is a wealth of data indicating that an effective strategy to combat obesity is to reduce the weight of adipose tissue (Yao et al., 2016; Yin et al., 2018). Notably, there are regional differences in the sensitivity of adipose tissue depots in response to dietary manipulations and the most sensitive depot is the subcutaneous white adipose tissue (WAT) (Fickova et al., 1998; Gaiva et al., 2001; Rodríguez et al., 2002). Moreover, subcutaneous WAT accounts for ∼85% total fat mass, and a lower subcutaneous WAT is more protective compared to visceral WAT (Booth et al., 2018). Therefore, subcutaneous WAT was a focus of this study, with the goal to understand dietary nutrients to combat obesity. Evidence in the literature has demonstrated that WAT has a variety of well-documented functions, including serving as an energy storage site, regulating glucose and lipid homeostasis, and producing adipokines (Cummins et al., 2014). The two primary metabolic activities of WAT (lipogenesis and lipolysis) cooperate to maintain the relative constancy of body fat under normal conditions (Proença et al., 2014). In humans, excessive body fat contributes to obesity and diabetes (Zheng J. et al., 2021). In the meat industry, the increased WAT mass has a deleterious effect on production efficiency and meat quality (Lebret and Mourot, 1998). Therefore, maintaining an appropriate WAT mass is an important objective pursuit of animal production and human health.
Leucine functions as a direct-acting nutrient signal in WAT to affect lipid metabolism, thus favoring adiposity reduction (Yao et al., 2016; Zhang et al., 2020). Despite these positive outcomes, multiple lines of evidence have pointed out that elevated levels of circulating leucine may precede or coincide with insulin resistance and cardiovascular dysfunction, thus seriously threatening human health (Felig et al., 1969; Yang et al., 2015). In explanation of these observations, previous studies have hypothesized that the ability of adipocytes to degrade or oxidize leucine is lost (Gannon et al., 2018). Interestingly, β-hydroxy-β-methylbutyrate (HMB), a metabolite of leucine, has been reported to share similar regulatory effects on lipid metabolism with leucine (Duan et al., 2018). More importantly, HMB cannot be reversibly converted to leucine (Nissen and Abumrad, 1997). Of note, a growing number of evidence has demonstrated convincingly that dietary HMB supplementation is an effective way to regulate lipid metabolism. For example, HMB treatment (0.62%, 45 days) gave rise to fat loss in growing pigs (Duan et al., 2018). Other experiments conducted in rodent models also confirmed that animals having access to HMB showed a decline in fat mass (Wilson et al., 2012; Park et al., 2013). Thus, we shifted the focus from leucine to HMB, which may provide an alternative for safe and efficacious regulation of lipid metabolism.
Alterations in the gut-microbiota community have been reported to be associated with the occurrence and development of obesity (Marchesi et al., 2016; Wang Z. et al., 2018). The metabolic activity of the gut microbiota can be linked to host body energy homoeostasis by short-chain fatty acids (SCFAs), which are produced by gut microbiota catabolizing dietary fibers (Topping and Clifton, 2001). There is compelling evidence that nutrients can attenuate obesity by reprogramming gut microbiota (Xu et al., 2017; Yin et al., 2018; Zhang et al., 2019; Wang et al., 2020; Zhong et al., 2020). Using a rodent model, we also found that HMB could mitigate lipid metabolism disorders by reprogramming gut microbiota, as manifested by the increased ratio of Bacteroidetes to Firmicutes and elevated Bacteroidetes-produced propionic acid (Duan et al., 2019). However, the literature on the possible relationship between HMB treatment and gut microbiota is dominated by studies in rodents, whether the beneficial effects of HMB in larger mammals including pigs are mediated by gut microbiota requires further interrogation.
Bama Xiang mini-pig, a fat pig breed, is an optimal model to explore the regulation role of diets on obesity due to its anatomical and physiological similarities with humans (Chen et al., 2009; Jiang et al., 2015; Koopmans and Schuurman, 2015; Niu et al., 2017). Therefore, in this study, Bama Xiang mini-pigs were used to (1) investigate the effects of dietary HMB levels on lipid metabolism in the WAT, and (2) illustrate the potential mechanisms underlying the action of HMB on lipid metabolism. It was hypothesized that the optimal dietary HMB level could reduce fat accumulation in the WAT of Bama Xiang mini-pigs through regulating gut microbiota.
Materials and Methods
Animals and Diets
The experiments in the present study were approved by the Animal Welfare Committee of the Institute of Subtropical Agriculture, Chinese Academy of Sciences. The ethic approval number is ISA-2017-023.
Thirty-two Bama Xiang mini-pigs (8.58 ± 0.40 kg, barrow) were selected and randomly allotted to four dietary treatments (8 piglets per treatment). The HMB-Ca level of the four dietary treatments were as follows: 0 (control), 0.13, 0.64, and 1.28%. The HMB-Ca (purity ≥ 99.0%) was obtained from Jiangyin TSI Pharmaceutical Co., Ltd. All diets were isoenergetic and isonitrogenous (Zheng C. et al., 2021), and met the nutritional needs for growing-mini pigs (Gao and Liu, 2009). The pigs raised individually in cages with ad libitum access to diets and water. This experiment lasted for 60 days.
Sample Collection and Fat Percentage Measurement
At the end of the experiment, pigs were fasted overnight (12 h) and then slaughtered by electrical stunning (250 V, 0.5 A, for 5–6 s) and exsanguinating. After slaughter, samples of WAT including DSA and ASA were rapidly excised from the right side of the carcass. The samples were then either stored at −20°C for the determination of fatty acid composition or quickly frozen in liquid nitrogen and then stored at −80°C for the analysis of Western blotting. Meanwhile, carcass weight and fat mass weight were recorded, and the fat percentage was calculated by dividing the fat mass weight by carcass weight. Subsequently, the colon was quickly separated and the colonic contents were collected from a region 10 cm posterior to the ileocecal valve into sterile tubes and stored at −80°C for further analysis.
Determination of Fatty Acid Composition
The fatty acid composition of DSA and ASA was analyzed via gas-liquid chromatography of methyl esters using an Agilent 7890A GC as previously described (Yin et al., 2000; Liu et al., 2015). The following indices were calculated based on fatty acid composition: the sum of saturated fatty acids (SFA), monounsaturated fatty acids (MUFA), and polyunsaturated fatty acids (PUFA), respectively. Then, the ratio of PUFA to SFA and the ratio of n-6 to n-3 PUFA were assessed.
Adipose Tissue Histologic Analysis
At the time of sacrifice, samples of DSA and ASA (1 cm3) were collected and fixed in 4% paraformaldehyde in PBS (pH 7.3) for paraffin sections and hematoxylin and eosin staining as previously described (Zhang et al., 2021). Tissue sections were imaged at 100 × magnification using a microscope (Eclise Ci-L, Nikon, Japan). The adipocyte size (10 fields/sample) was quantified using DIXI3000 (Leica Camera, Wetzlar, Germany).
Determination of Colonic SCFAs Concentrations
About 1 g of colonic contents were used to measure the concentrations of SCFAs (acetate, propionate, butyrate, isobutyrate, valerate, and isovalerate) by using an Agilent 6890A gas chromatography (Agilent Technologies, Santa Clara, CA, United States) according to our previous study (Yin et al., 2018).
Gut Microbiota Analysis
Gut microbiota in the colon of Bama Xiang mini-pigs were determined as previously described (Duan et al., 2019; Song et al., 2019). Alpha diversity indices, including Shannon, Simpson, Chao1, and ACE, were used to evaluate the diversity and richness of gut microbiota (Lemieux-Labonte et al., 2017).
Western Blotting Analysis
The relative protein expression of phosphorylated AMPKα (p-AMPKα), FoxO1 (p-FoxO1), mTOR (p-mTOR), and Sirt1 (p-Sirt1) in the DSA and ASA were determined as previously described (Duan et al., 2013). All the primary antibodies were purchased from Cell Signaling Technology (Danvers, MA) and the secondary antibodies were obtained from Thermo Scientific Inc. (Waltham, MA, United States). The protein bands were visualized by a chemiluminescent reagent (Pierce, Rockford, United States) with a ChemiDoc XRS system (Bio-Rad, Philadelphia, PA, United States). We quantified the resultant signals using Alpha Imager 2200 software (Alpha Innotech Corporation, CA, United States) and normalized the data with the value of the inner control GAPDH.
Statistical Analysis
Data were analyzed using one-way analysis of variance (ANOVA) using the SAS version 8.2 (SAS Institute Inc., Cary, NC, United States) software followed by Duncan’s multiple comparison test. Orthogonal polynomial contrasts were performed to determine linear and quadratic effects of increasing dietary HMB on the measured indicators using SPSS 22.0 software (SPPS Inc., Chicago, IL, United States). Results are presented as means ± standard errors. Differences between significant means were considered statistically different at P < 0.05. Probability values between 0.05 and 0.10 were considered trends.
Results
Fat Percentage and Adipocyte Size
As shown in Figure 1, linear effects of increased HMB levels on fat percentage was observed, with the highest value observed in the 1.28% HMB group and the lowest value observed in the 0.13% HMB group (P < 0.05).
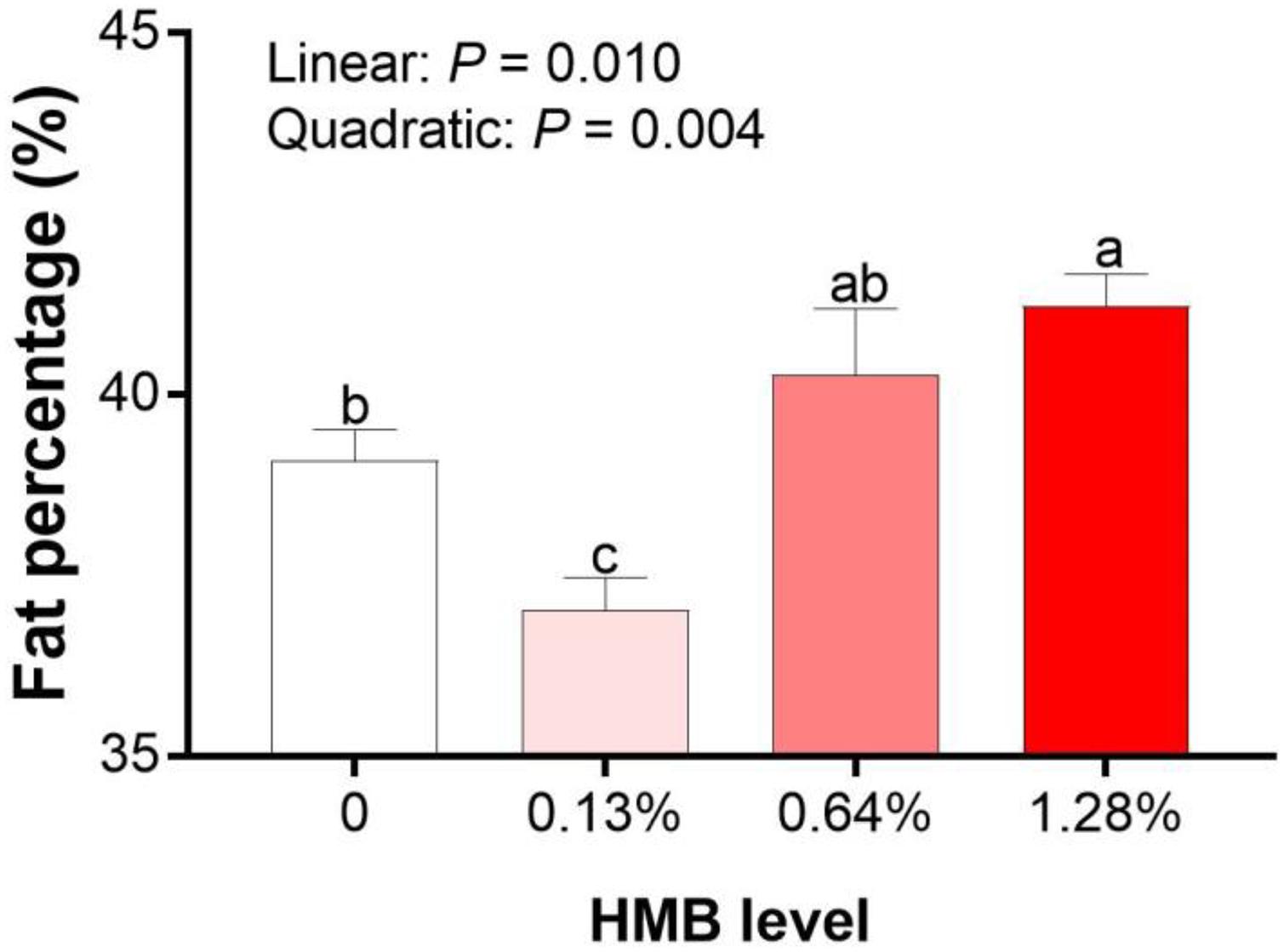
Figure 1. Effects of dietary HMB supplementation on fat percentage of Bama Xiang mini-pigs. Data are presented as mean ± SEM (n = 8). a,b,c Values with different letters are significantly different among dietary HMB treatments (P < 0.05).
As presented in Figure 2, HMB linearly increased the adipocyte mean area of DSA, and the highest/lowest value was obtained in the 0.64% HMB group/0.13% HMB group, respectively (P < 0.05). The adipocyte mean area of ASA in the 0.64% HMB group was higher than that in the other three groups (P < 0.05), and there was no significant difference among the three groups.
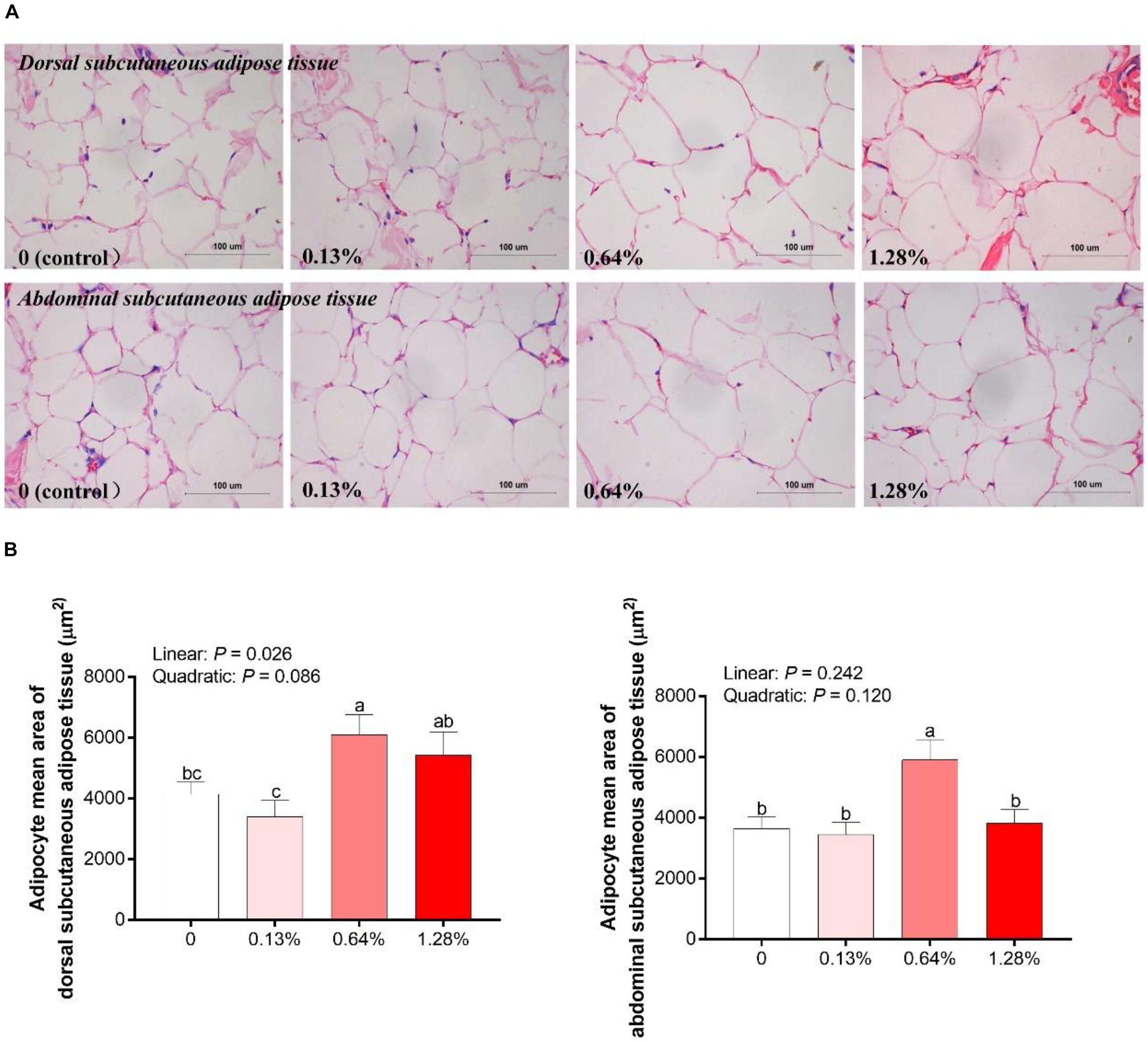
Figure 2. Analysis of hematoxylin and eosin slices of adipocytes in Bama Xiang mini-pigs offered four diets (A) and the adipocyte mean area of adipocytes (B) in the adipose tissue of Bama Xiang mini-pigs. Data are presented as mean ± SEM (n = 8). a,b,c Values with different letters are significantly different among dietary HMB treatments (P < 0.05).
Fatty Acid Composition
As depicted in Table 1, dietary HMB supplementation significantly influenced the fatty acid composition in the DSA. HMB supplementation linearly increased the concentration of C18:3 n3, with the highest value observed in the 0.13% HMB group (P < 0.05). As dietary HMB levels increased, the concentration of C18:2 n6c showed a downward trend (quadratic, P = 0.053). The concentration of C20:4 n6 was decreased in the HMB groups compared with the control (linear and quadratic, P < 0.05). The n6:n3 PUFA ratio was the highest in the 1.28% HMB group and the lowest in the 0.13% HMB group, with intermediate values observed in the other two groups (quadratic, P < 0.05). HMB quadratically decreased the sum of PUFA (P < 0.05) and the ratio of PUFA:SFA (P = 0.085). Dietary treatments did not significantly affect the sum of SFA and MUFA (P > 0.05).
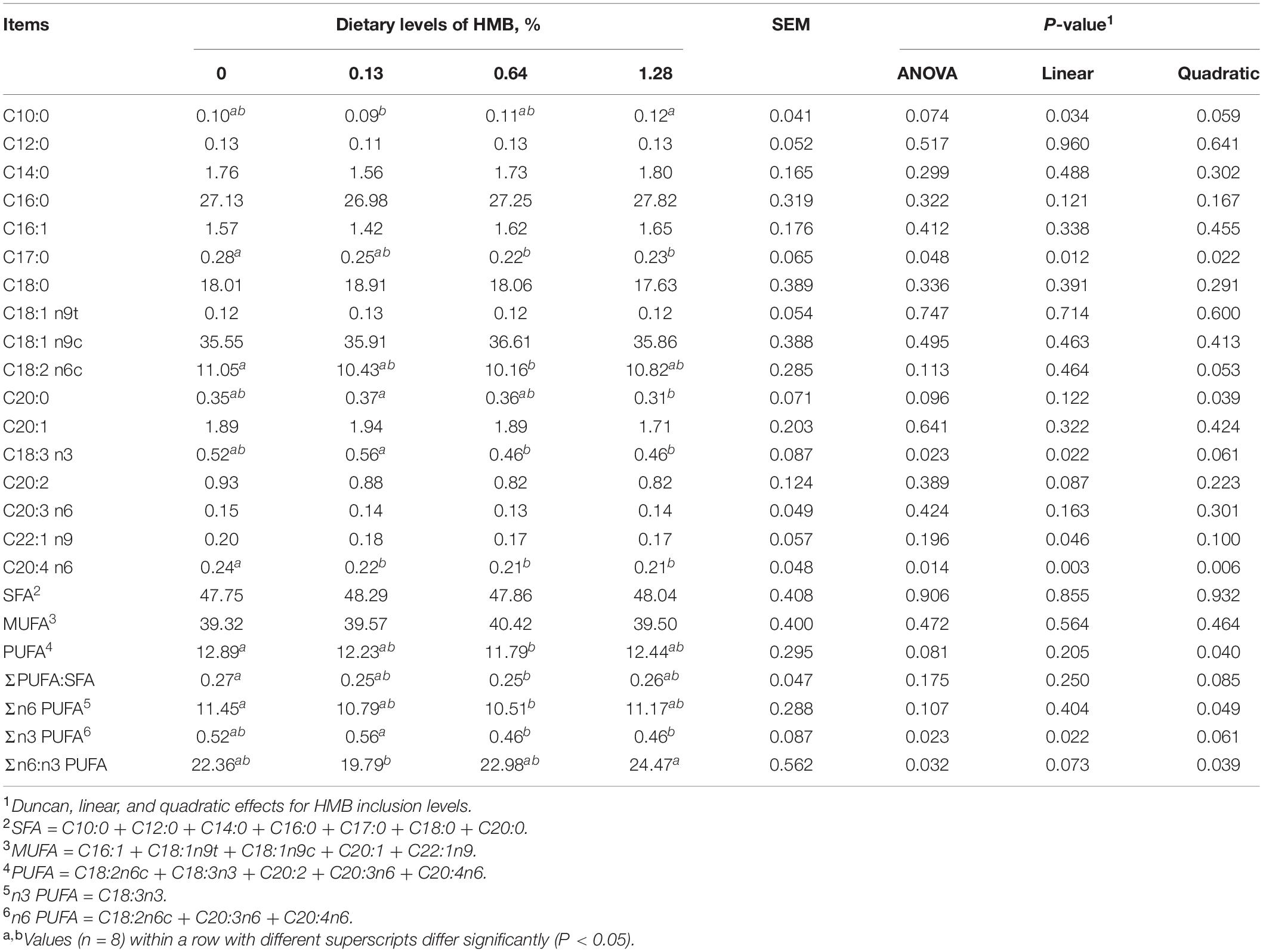
Table 1. Fatty acid composition of dorsal subcutaneous adipose tissue in Bama Xiang mini-pigs fed the diets with various levels of HMB (% of total fatty acids).
The results concerning the fatty acid composition of ASA were presented in Table 2. No significant difference was observed in the contents of C18:2 n6c, C20:3 n6, and C20:4 n6, the sum of n6 PUFA, SFA, MUFA, and PUFA, the ratio of PUFA to SFA, and the ratio of n6 to n3 PUFA (P > 0.05). HMB linearly increased the concentration of C18:3 n3, with the highest value observed in the 0.13% HMB group and the lowest value in 0.64% HMB group (P < 0.05).
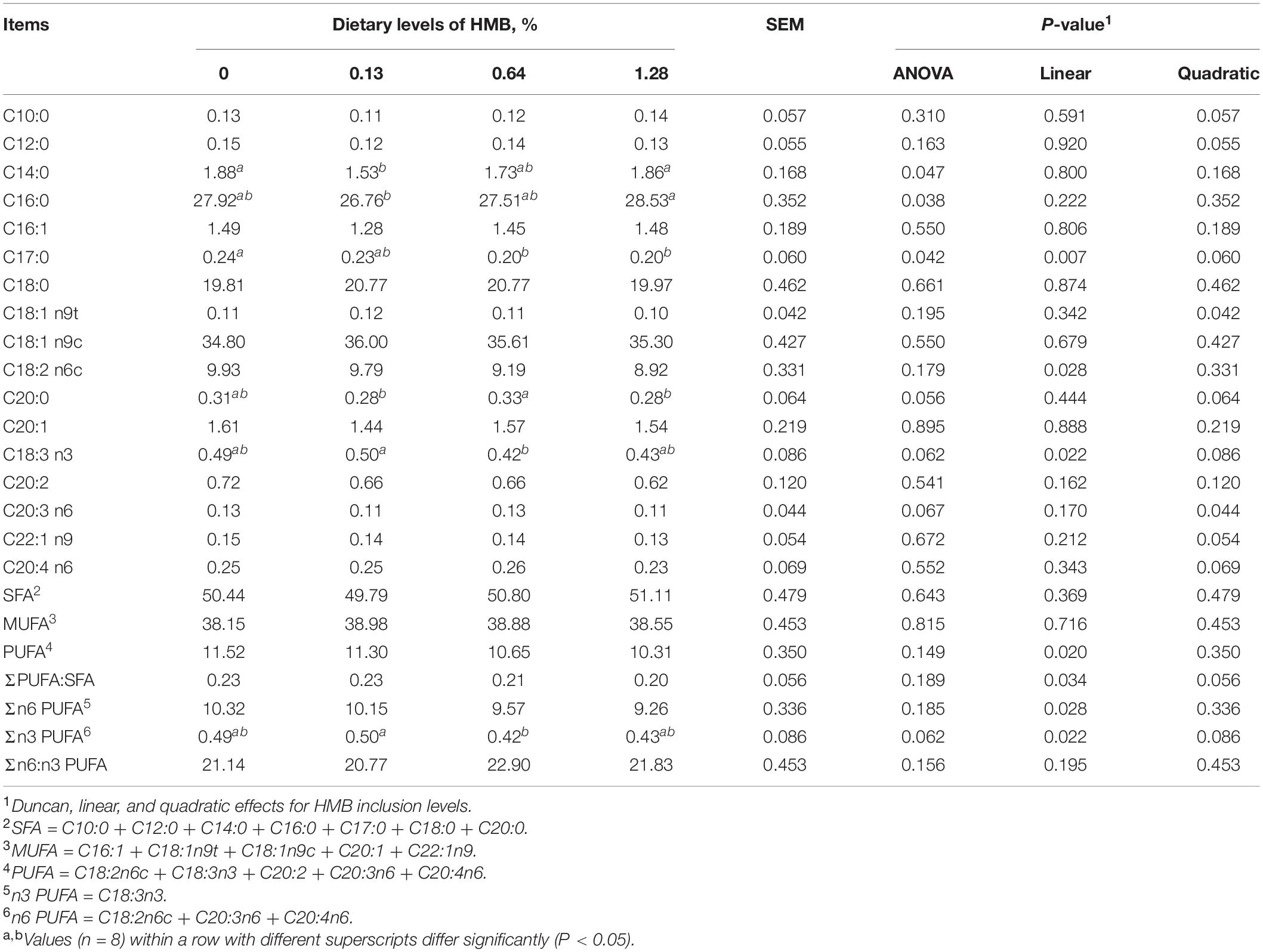
Table 2. Fatty acid composition of abdominal subcutaneous adipose tissue in Bama Xiang mini-pigs fed the diets with various levels of HMB (% of total fatty acids).
Colonic SCFA Concentrations
As shown in Figure 3, there were no linear or quadratic effects of increased HMB levels on colonic isobutyrate, valerate, and isovalerate concentrations (P > 0.05). The highest and the lowest concentrations of acetate, propionate and butyrate appeared in the 0.13% HMB group and 0.64% HMB group, respectively (P < 0.05). In addition, correlation analyses between the fat percentage and colonic acetate, propionate, butyrate, isobutyrate, valerate, and isovalerate concentrations were conducted by Pearson correlation analysis (Figure 4). The fat percentage negatively correlated with the colonic acetic acid and butyrate production (P < 0.05).
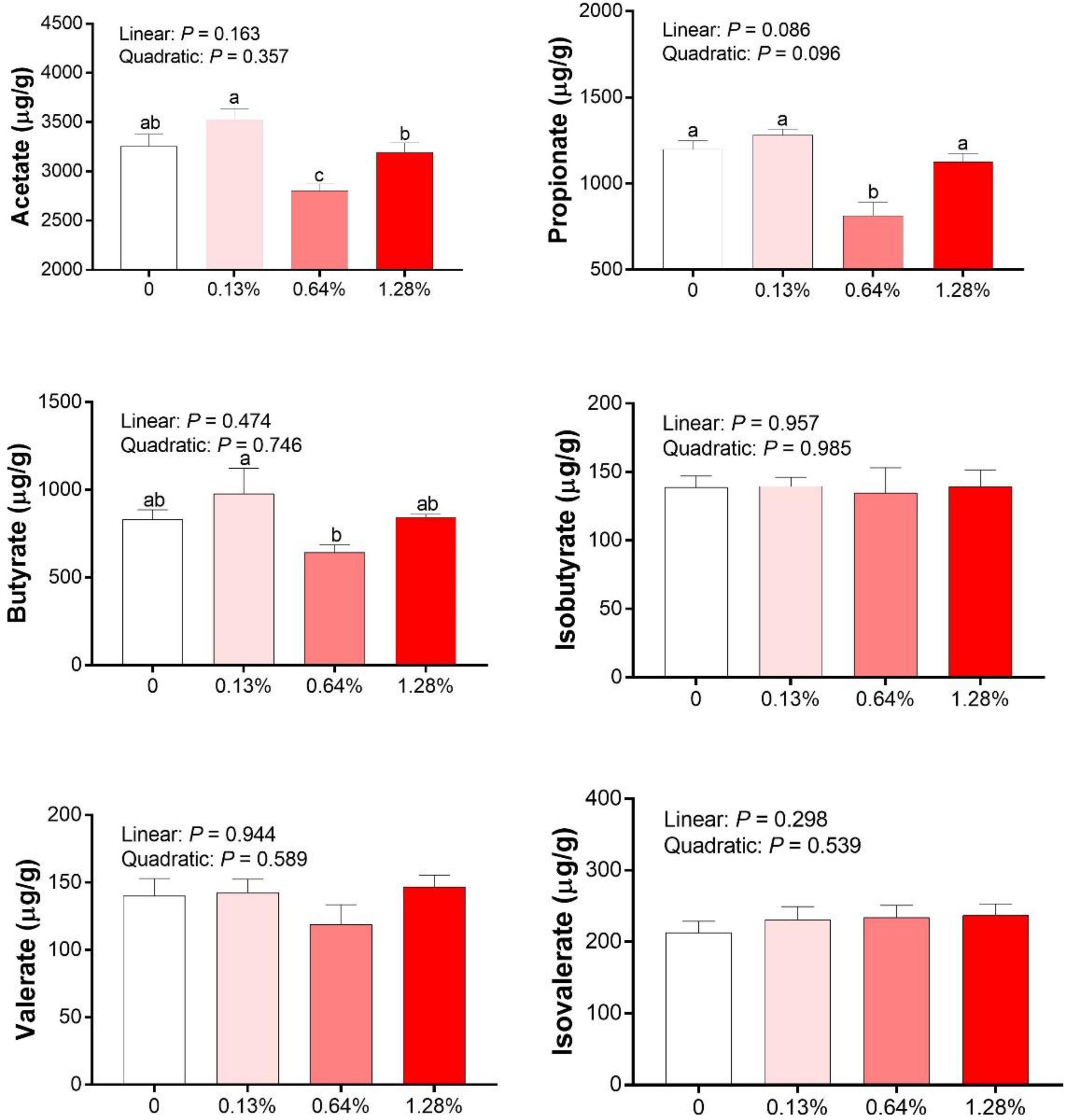
Figure 3. The SCFAs concentrations in the colonic contents of Bama Xiang mini-pigs. Data are presented as mean ± SEM (n = 8). a,b,c Values with different letters are significantly different among dietary HMB treatments (P < 0.05).
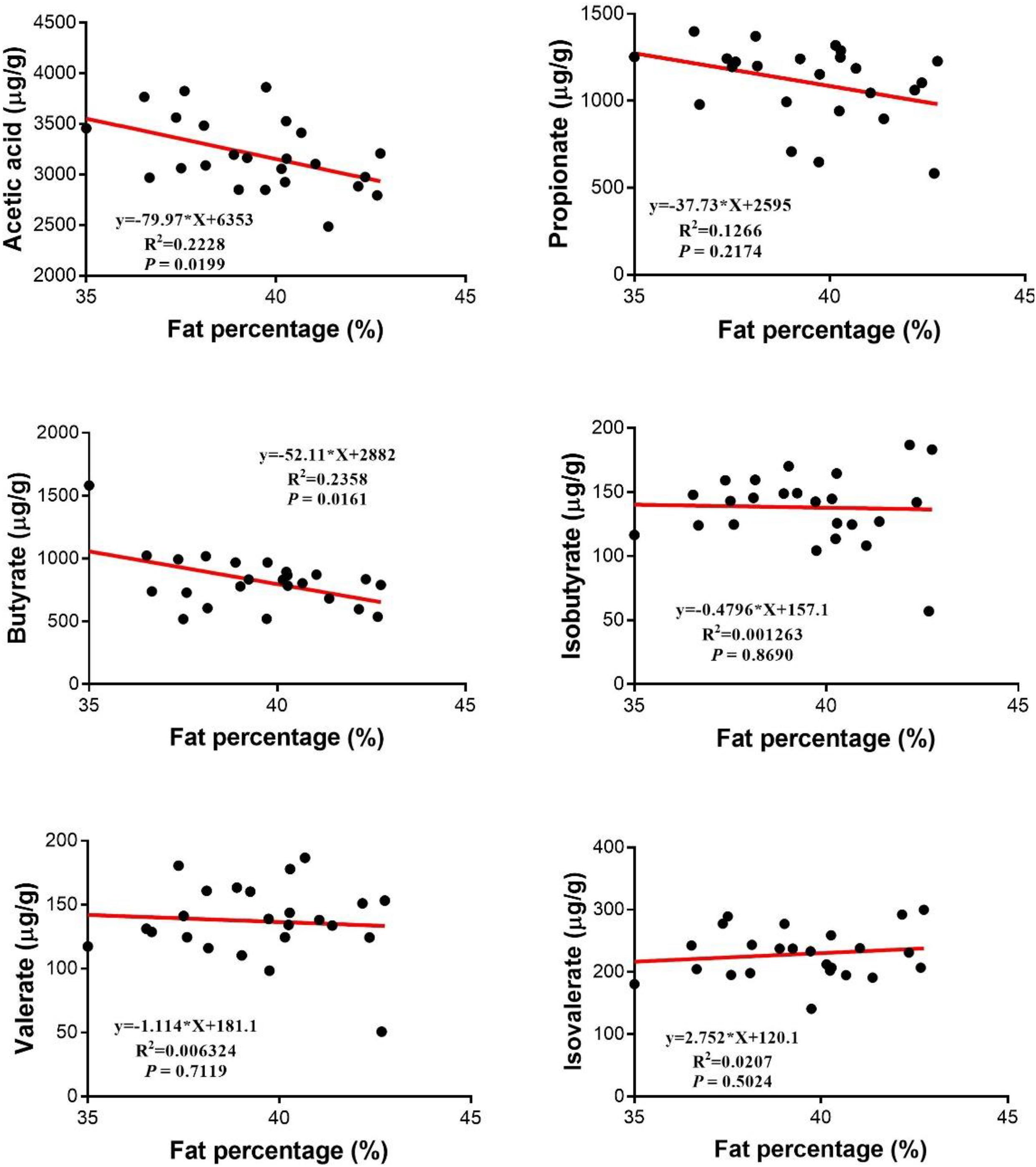
Figure 4. Relationship between SCFAs and the fat percentage. Pearson correlations were used to determine the association between the fat percentage and colonic acetate, propionate, butyrate, valerate, isobutyrate, and isobutyrate concentrations.
Gut Microbiota Composition of Bama Xiang Mini-Pigs
As shown in Figure 5A, there were no significant differences in indexes of Shannon and Simpson among the groups (P > 0.05). However, linear and quadratic effects of HMB supplementation on indexes of Chao1 and ACE (Chao1, linear, P = 0.014, quadratic, P = 0.001; ACE, linear, P = 0.012, quadratic, P = 0.021) were observed, with the highest value observed in the 0.13% HMB group and the lowest value observed in the 1.28% HMB group. To further explore the overall microbial composition, we analyzed the relative abundance of dominant taxa at the phylum level. As shown in Figure 5B, the most dominant phyla in the bacterial communities were Firmicutes, Bacteroidetes, and Proteobacteria. HMB supplementation did not significantly affect the relative abundance of Firmicutes (P > 0.05). The relative abundance of Bacteroidetes reached its greatest value in the 0.13% HMB group and was higher than that in other three groups (quadratic, P < 0.05). The relative abundance of Proteobacteria was the highest in the 0.64% HMB group and the lowest in the 0.13% HMB group (P < 0.05). Furthermore, correlation analyses between the fat percentage and the relative abundances of Bacteroidetes and Proteobacteria were conducted by Pearson correlation analysis (Figure 5C). The acetic acid concentration was positively/negatively correlated with the abundance of Bacteroidetes/Proteobacteria, respectively (P < 0.05). Moreover, the abundance of Bacteroidetes was negatively correlated with the fat percentage (P < 0.05). No correlation was observed between the abundance of Proteobacteria and the fat percentage, and between the butyrate production and the relative abundance of Bacteroidetes or Proteobacteria (P > 0.05).
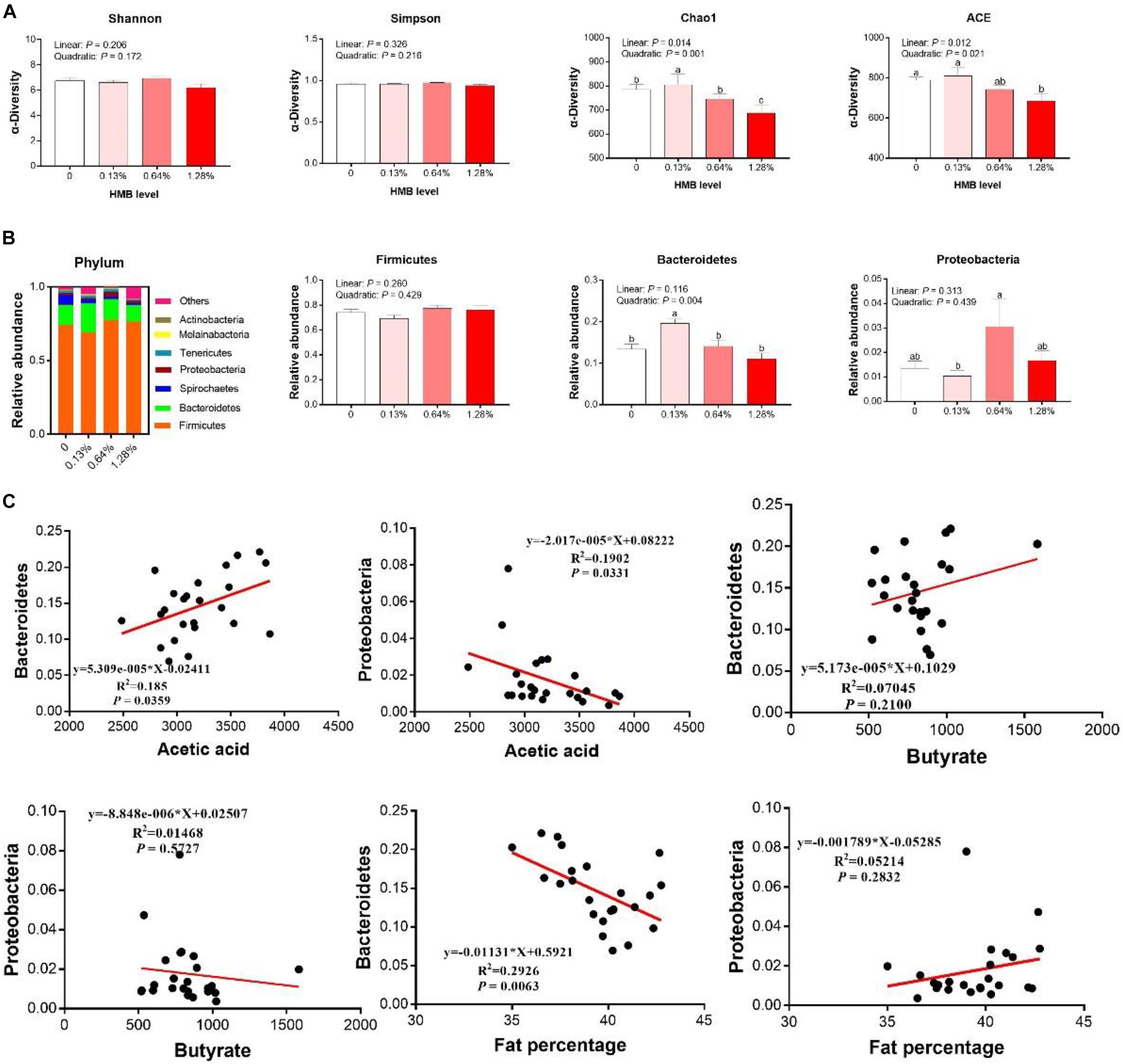
Figure 5. Dietary HMB supplementation improved gut microbiota in Bama Xiang mini-pigs. The α-diversity analysis (A), microbiota composition at the phylum level (B), the correlation analyses between the relative abundance of Bacteroidetes/Proteobacteria and acetic acid/butyrate, the correlation analyses between the relative abundance of Bacteroidetes/Proteobacteria and fat percentage (C). Data are presented as mean ± SEM (n = 8). a,b,c Values with different letters are significantly different among dietary HMB treatments (P < 0.05).
Protein Expression of p-AMPKα, p-FoxO1, p-mTOR, and p-Sirt1
The protein expression of p-AMPKα, p-FoxO1, p-mTOR, and p-Sirt1 in the control and 0.13% HMB groups were shown in Figure 6. In the DSA (Figure 6A), compared to the control group, the 0.13% HMB supplementation significantly upregulated the protein expression of p-AMPKα, p-FoxO1, and p-Sirt1 (P < 0.05), and downregulated the p-mTOR protein expression (P < 0.05). In the ASA (Figure 6B), compared to the control group, the 0.13% HMB supplementation significantly upregulated the protein expression of p-AMPKα and p-Sirt1 (P < 0.05), and downregulated the p-mTOR protein expression (P < 0.05). The protein expression of p-FoxO1 showed a trend to increase in response to HMB supplementation (P = 0.0815).
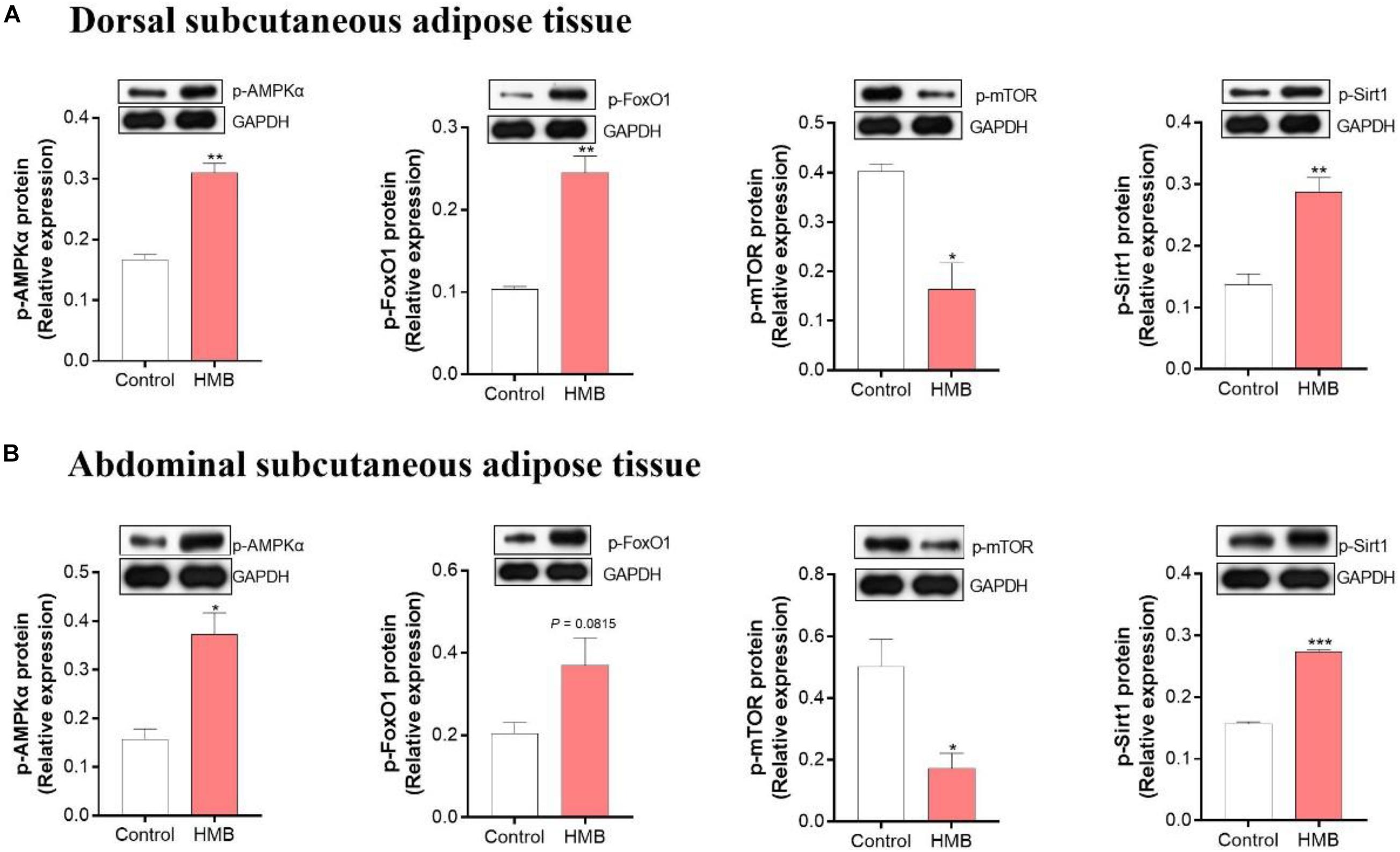
Figure 6. The expression of phosphorylation (p)-AMPKα, FoxO1, mTOR and Sirt1 in DSA (A) and ASA (B) of Bama Xiang mini-pigs fed diets with 0.13% HMB-Ca. Data are presented as mean ± SEM (n = 8). *P < 0.05, **P < 0.01, ***P < 0.001. AMPK, AMP-activated protein kinase; FoxO1, forkhead box O1; mTOR, mammalian target of rapamycin; Sirt1, silent information regulator 2 related enzyme 1.
Discussion
In the current study, we presented evidence that dietary supplementation of HMB with optimal level (0.13%) significantly inhibited fat deposition in Bama Xiang mini-pigs, as evidenced by the decreased fat percentage and adipocyte mean area of DSA. Notably, these observations did not occur at higher levels of HMB. In good agreement with our results, evidence from a rodent model has demonstrated that the maximum reduction of fat mass and mean size of WAT occurred at the HMB level of 1% (wt/vol), with higher concentrations of HMB being ineffective (Duan et al., 2019). Similar observations were also obtained in protein metabolism, with higher doses of HMB being ineffective (Wheatley et al., 2014). However, the reason why higher concentrations of HMB are unable to regulate lipid metabolism positively requires further interrogation. In addition, unlike DSA, HMB supplementation failed to reduce the adipocyte mean area of ASA. Therefore, these results suggest that the appropriate HMB dosage was 0.13% and that HMB mainly reduced the fat mass of DSA rather than of ASA.
Given the site differences in adipocyte size of subcutaneous fat depots following HMB supplementation, we speculated that there were differences in the fatty acid composition of subcutaneous fat depots in different regions. Moreover, there is compelling evidence that adipose tissue fatty acid composition is greatly associated with fat cell size (Raclot et al., 1997; Garaulet et al., 2006). It has been reported that n3 and n6 PUFAs are negatively correlated with the adipocyte size. In contrast, SFA is positively correlated with the adipocyte size (Garaulet et al., 2006). In the current study, although the SFA in DSA and ASA upon the HMB diets did not achieve the statistical significance, dietary HMB supplementation greatly elevated the n3 PUFA in selected WAT compared to the control group, and the maximum elevation of the n3 PUFA occurred at the HMB level of 0.13%. Unlike the n3 PUFA, HMB supplementation quadratically decreased n6 PUFA concentration in DSA, with the lowest value observed in the 0.64% HMB group, and did not significantly affect this parameter in ASA. These observations might underlie the mechanistic explanation for a beneficial role of HMB on the adipocyte mean area of DSA rather than ASA. Site differences in numerous metabolic activities in subcutaneous fat depots have been reported by studies in obese and non-obese humans (Lithell and Boberg, 1978; Malcom et al., 1989). Therefore, the differences in fatty acid composition between DSA and ASA indicate that HMB might differently affect metabolic activities (such as rate of deposition and mobilization) in the two subcutaneous sites.
It is well-known that the occurrence and development of obesity is closely associated with alterations in composition, diversity, and function of the gut microbiota (Shen et al., 2013; Gérard, 2015; ‘Graham et al., 2015). To explore whether dietary HMB supplementation reduced the fat deposition via gut microbiota, we analyzed the composition of gut microbiota in the colon of Bama Xiang mini-pigs. Our data showed that HMB supplementation (0.13%) increased the relative abundance of Bacteroidetes, and the increased Bacteroidetes was positively associated with the reduction of fat percentage. These results are well-matched with works in a rodent model (Duan et al., 2019). Moreover, our data showed that the relative abundance of Proteobacteria was the highest in the 0.64% HMB group and the lowest in the 0.13% HMB group. It has been reported that Proteobacteria is related to intestinal inflammation and gastrointestinal diseases (Kang et al., 2019; Bakhti et al., 2020; Zeng et al., 2020). Therefore, evidence from the current study shows that HMB supplementation at the level of 0.13% leads to the elevation of the beneficial bacteria Bacteroidetes and the reduction of the harmful bacteria Proteobacteria. Based on the results from our previous study (Duan et al., 2019) and the present study, we speculated that alterations in gut microbiota composition might contribute to the reduced fat deposition of Bama Xiang mini-pigs observed in the 0.13% HMB group. However, our knowledge of how HMB targets gut microbiota to reduce fat deposition is far from complete so that it needs to be further explored.
Several recent studies have demonstrated that the bacterial metabolites SCFAs exert a role in the action of gut microbiota (Yin et al., 2018; Duan et al., 2019; Hu et al., 2019), which motivated us to analyze the concentrations of SCFAS in the colon of Bama Xiang mini-pigs. Our data showed that HMB supplementation (0.13%) tended to reverse the decrease of acetic acid, and acetic acid production was negatively correlated with the fat percentage. These results are in accordance with other studies, which have demonstrated that diets-elevated colonic production of acetic acid contributes to the reduction of body weight in high fat diets-induced obese mice (Yin et al., 2018). Upon further investigation, we found that the colonic acetic acid production and the relative Bacteroidetes abundance showed a positive correlation. A major route to form acetic acid from dietary carbohydrates is driven by the abundant Bacteroidetes (Miller, 1978). Therefore, these results partially indicated that HMB supplementation affects gut microbiota compositions (especially for Bacteroidetes), which further generated acetic acid to reduce fat deposition. Strong evidence for the role of acetic acid on obesity comes from several rodent studies (Yamashita et al., 2007, 2009; Beh et al., 2017). In contrast to the results observed in Bama Xiang mini-pigs, data from rodent studies showed that the beneficial effects of HMB on obesity was mediated by gut microbiota-propionic acid axis (Duan et al., 2019). The inconsistency of the two reports might be due to the differences in experimental models, the experimental approaches, and the varied doses used in each experiment. This discrepancy suggests that results from rodent studies are not readily translated to human models of obesity.
Interestingly, there is evidence showing that the activation of AMPKα in adipose tissue could be one possible contributing mechanism for the beneficial effects of acetic acid (Fu et al., 2018; Liu et al., 2019). The enzyme AMPKα exerts key roles in regulating energy homeostasis (Shen et al., 2013; Yamashita, 2016). Evidence from in vivo and in vitro studies suggests that AMPKα activation in adipose tissue could suppressing lipogenesis by impairing the mTOR signaling (Gaidhu et al., 2009; Wang Q. et al., 2018) and promoting the phosphorylation of FoxO1 (Greer et al., 2007; Chen et al., 2010). On the other hand, the phosphorylation of AMPKα could promote lipolysis by activating Sirt1 directly (Canto et al., 2009). In the current study, Western blot analysis revealed that HMB markedly upregulated the phosphorylation of AMPK, FoxO1, and Sirt1, and downregulated the phosphorylation of mTOR in selected WAT. These results are perfectly in line with a recent study which reported that HMB might regulate lipid metabolism via the AMPKα-mTOR and AMPKα-Sirt1 signaling pathways in the perirenal adipose tissue of Large white × Landrace pigs (Duan et al., 2018).
Conclusion
In summary, our data showed that dietary HMB supplementation at the level of 0.13% increased n3 PUFA and reduced fat mass in adipose tissue of Bama Xiang mini-pigs, and the favorable effects were more pronounced in the DSA. The beneficial effects of HMB on reducing fat deposition were likely regulated by the Bacteroidetes-acetic acid-AMPKα axis (Figure 7). These findings may provide valuable information for understanding the mechanisms of action of HMB in combating obesity. Areas that need further exploration include the research of mechanisms whereby HMB elevated the relative Bacteroidetes abundance and the acetic acid production. Although our data were encouraging, these findings should be confirmed with clinical studies with long-term follow-up.
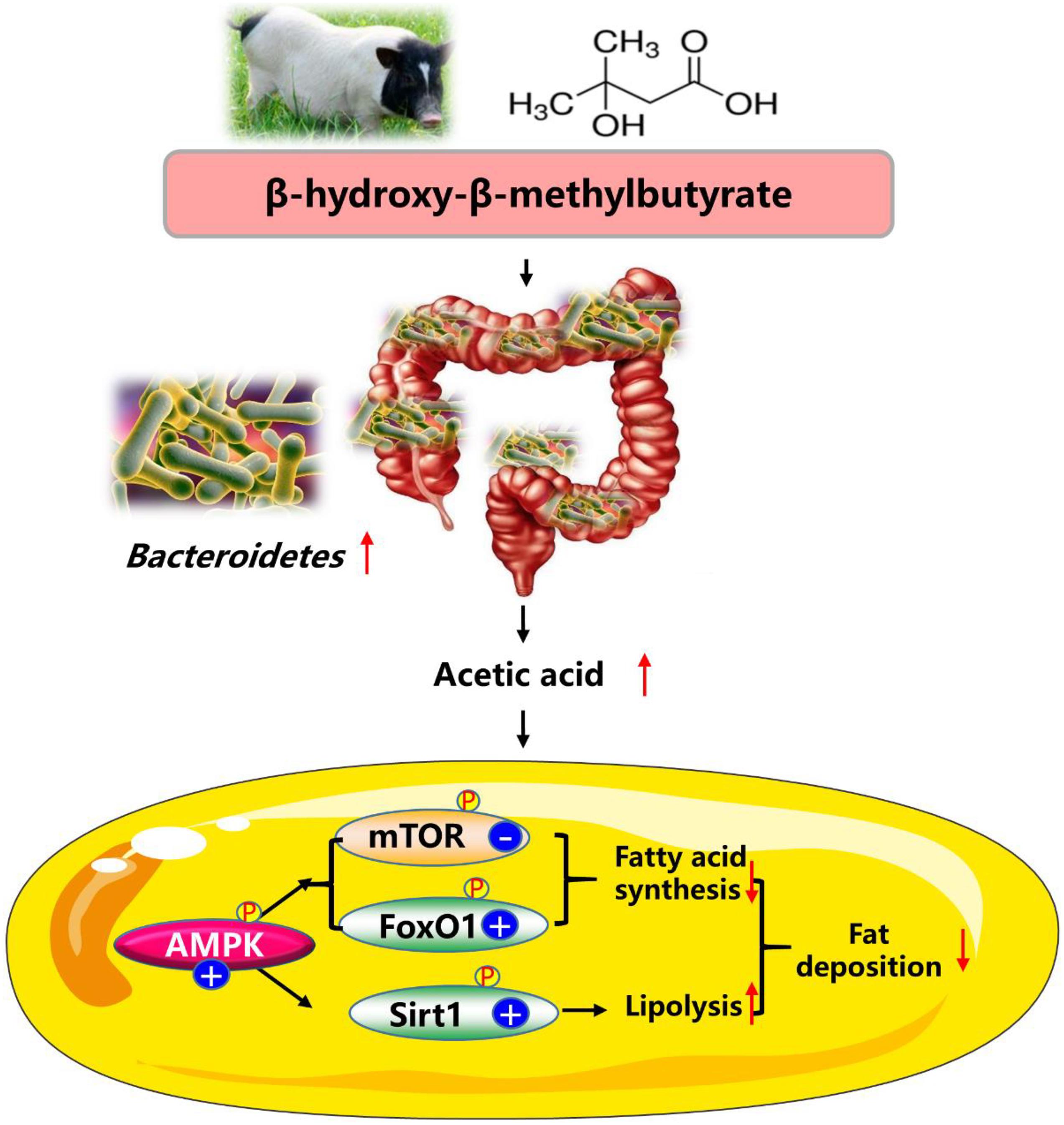
Figure 7. Proposed mechanisms of dietary HMB supplementation for modulating the lipid metabolism in adipose tissue of Bama Xiang mini-pigs. AMPK, AMP-activated protein kinase; FoxO1, forkhead box O1; mTOR, mammalian target of rapamycin; Sirt1, silent information regulator 2 related enzyme 1.
Data Availability Statement
The raw data supporting the conclusions of this article will be made available by the authors, without undue reservation.
Ethics Statement
The animal study was reviewed and approved by Animal Welfare Committee of the Institute of Subtropical Agriculture, Chinese Academy of Sciences. Written informed consent was obtained from the owners for the participation of their animals in this study.
Author Contributions
YD contributed to the conception of the study. JZ, CZ, BS, SZ, YZ, LZ, and GD performed the experiment. JZ and CZ contributed equally to data analysis and manuscript preparation. FL and QG revised the manuscript. All authors read and finalized the manuscript.
Funding
This study was jointly supported by the Natural Science Foundation of Guangxi Province (2020JJA130102 and 2018JJB130239), the National Natural Science Foundation of China (U19A2037 and 31802077), the Changsha Natural Science Funds for Distinguished Young Scholar (kq2009020), Young Elite Scientists Sponsorship Program by CAST (2019QNRC001), Special funds for the construction of innovative provinces in Hunan Project (2019NK2193 and 2019RS3022), China Agriculture Research System of MOF and MARA (CARS-35), the “Strategic Priority Research Program” of the Chinese Academy of Sciences (XDA24030204), Open Fund of Key Laboratory of Agro-ecological Processes in Subtropical Region, Chinese Academy of Sciences (ISA2020203), and Taishan industry leading talent project special funds.
Conflict of Interest
The authors declare that the research was conducted in the absence of any commercial or financial relationships that could be construed as a potential conflict of interest.
Publisher’s Note
All claims expressed in this article are solely those of the authors and do not necessarily represent those of their affiliated organizations, or those of the publisher, the editors and the reviewers. Any product that may be evaluated in this article, or claim that may be made by its manufacturer, is not guaranteed or endorsed by the publisher.
Abbreviations
AMPK α, AMP-activated protein kinase α; ASA, abdominal subcutaneous adipose tissue; DSA, dorsal subcutaneous adipose tissue; FoxO1, forkhead box O 1; HMB, β-hydroxy - β-methylbutyrate; mTOR, mammalian target of rapamycin; MUFA, monounsaturated fatty acid; PUFA, polyunsaturated fatty acid; SCFA, short chain fatty acid; SFA, saturated fatty acid; Sirt1, silent information regulator 2 related enzyme 1.
References
Bakhti, S. Z., Latifi-Navid, S., and Safaralizadeh, R. (2020). Helicobacter pylori-related risk predictors of gastric cancer: the latest models, challenges, and future prospects. Cancer Med. 9, 4808–4822. doi: 10.1002/cam4.3068
Beh, B. K., Mohamad, N. E., Yeap, S. K., Ky, H., Boo, S. Y., Chua, J. Y. H., et al. (2017). Anti-obesity and anti-inflammatory effects of synthetic acetic acid vinegar and Nipa vinegar on high-fat-diet-induced obese mice. Sci. Rep. 7:6664.
Booth, A. D., Magnuson, A. M., Fouts, J., Wei, Y., Wang, D., Pagliassotti, M. J., et al. (2018). Subcutaneous adipose tissue accumulation protects systemic glucose tolerance and muscle metabolism. Adipocyte 7, 261–272. doi: 10.1080/21623945.2018.1525252
Canto, C., Gerhart-Hines, Z., Feige, J. N., Lagouge, M., Noriega, L., Milne, J. C., et al. (2009). AMPK regulates energy expenditure by modulating NAD(+) metabolism and SIRT1 activity. Nature 458, 1056–U1140.
Chen, B. L., Ma, Y. D., Meng, R. S., Xiong, Z. J., Wang, H. N., Zeng, J. Y., et al. (2010). Activation of AMPK inhibits cardiomyocyte hypertrophy by modulating of the FOXO1/MuRF1 signaling pathway in vitro. Acta Pharmacol. Sin. 31, 798–804. doi: 10.1038/aps.2010.73
Chen, H., Liu, Y. Q., Li, C. H., Guo, X. M., and Huang, L. J. (2009). The susceptibility of three strains of Chinese minipigs to diet-induced type 2 diabetes mellitus. Lab. Anim. (NY) 38, 355–363. doi: 10.1038/laban1109-355
Cummins, T. D., Holden, C. R., Sansbury, B. E., Gibb, A. A., Shah, J., Zafar, N., et al. (2014). Metabolic remodeling of white adipose tissue in obesity. Am. J. Physiol. Endocrinol. Metab. 307, E262–E277.
Duan, Y., Li, F., Li, L., Fan, J., Sun, X., and Yin, Y. (2013). n-6:n-3 PUFA ratio is involved in regulating lipid metabolism and inflammation in pigs. Br. J. Nutr. 111, 445–451. doi: 10.1017/s0007114513002584
Duan, Y., Li, F., Wang, W., Guo, Q., Wen, C., Li, Y., et al. (2017). Interleukin-15 in obesity and metabolic dysfunction: current understanding and future perspectives. Obes. Rev. 18, 1147–1158. doi: 10.1111/obr.12567
Duan, Y., Zhang, L., Li, F., Guo, Q., Long, C., Yin, Y., et al. (2018). beta-Hydroxy-beta-methylbutyrate modulates lipid metabolism in adipose tissues of growing pigs. Food Funct. 9, 4836–4846. doi: 10.1039/c8fo00898a
Duan, Y., Zhong, Y., Xiao, H., Zheng, C., Song, B., Wang, W., et al. (2019). Gut microbiota mediates the protective effects of dietary beta-hydroxy-beta-methylbutyrate (HMB) against obesity induced by high-fat diets. FASEB J. 33, 10019–10033. doi: 10.1096/fj.201900665rr
Duan, Y. H., Li, F. N., Tan, B., Yao, K., and Yin, Y. L. (2017). Metabolic control of myofibers: promising therapeutic target for obesity and type 2 diabetes. Obes. Rev. 18, 647–659. doi: 10.1111/obr.12530
Felig, P., Marliss, E., and Cahill, G. F. Jr. (1969). Plasma amino acid levels and insulin secretion in obesity. N. Engl. J. Med. 281, 811–816. doi: 10.1056/nejm196910092811503
Fickova, M., Hubert, P., Cremel, G., and Leray, C. (1998). Dietary (n-3) and (n-6) polyunsaturated fatty acids rapidly modify fatty acid composition and insulin effects in rat adipocytes. J. Nutr. 128, 512–519. doi: 10.1093/jn/128.3.512
Fu, C., Liu, L., and Li, F. (2018). Acetate alters the process of lipid metabolism in rabbits. Animal 12, 1895–1902. doi: 10.1017/s1751731117003275
Gaidhu, M. P., Fediuc, S., Anthony, N. M., So, M., Mirpourian, M., Perry, R. L. S., et al. (2009). Prolonged AICAR-induced AMP-kinase activation promotes energy dissipation in white adipocytes: novel mechanisms integrating HSL and ATGL. J. Lipid Res. 50, 704–715. doi: 10.1194/jlr.m800480-jlr200
Gaiva, M. H., Couto, R. C., Oyama, L. M., Couto, G. E., Silveira, V. L., Riberio, E. B., et al. (2001). Polyunsaturated fatty acid-rich diets: effect on adipose tissue metabolism in rats. Br. J. Nutr. 86, 371–377. doi: 10.1079/bjn2001392
Gannon, N. P., Schnuck, J. K., and Vaughan, R. A. (2018). BCAA metabolism and insulin sensitivity–dysregulated by metabolic status? Mol. Nutr. Food. Res. 62:e1700756.
Gao, Q., and Liu, Y. (2009). Research advances on nutrient requirements of laboratory minipigs. Chin. J. Comp. Med. 19, 74–78.
Garaulet, M., Hernandez-Morante, J. J., Lujan, J., Tebar, F. J., and Zamora, S. (2006). Relationship between fat cell size and number and fatty acid composition in adipose tissue from different fat depots in overweight/obese humans. Int. J. Obes. (Lond) 30, 899–905. doi: 10.1038/sj.ijo.0803219
Graham, C., Mullen, A., and Whelan, K. (2015). Obesity and the gastrointestinal microbiota: a review of associations and mechanisms. Nutr. Rev. 73, 376–385. doi: 10.1093/nutrit/nuv004
Greer, E. L., Dowlatshahi, D., Banko, M. R., Villen, J., Hoang, K., Blanchard, D., et al. (2007). An AMPK-FOXO pathway mediates longevity induced by a novel method of dietary restriction in C. elegans. Curr. Biol. 17, 1646–1656. doi: 10.1016/j.cub.2007.08.047
Hu, C., Li, F., Duan, Y., Yin, Y., and Kong, X. (2019). Dietary supplementation with leucine or in combination with arginine decreases body fat weight and alters gut microbiota composition in finishing pigs. Front. Microbiol. 10:1767. doi: 10.3389/fmicb.2019.01767
Jiang, G., Li, L., Fan, J., Zhang, B., Oso, A. O., Xiao, C., et al. (2015). Dietary soy isoflavones differentially regulate expression of the lipid-metabolic genes in different white adipose tissues of the female Bama mini-pigs. Biochem. Biophys. Res. Commun. 461, 159–164. doi: 10.1016/j.bbrc.2015.04.006
Kang, Z., Lu, M., Jiang, M., Zhou, D., and Huang, H. (2019). Proteobacteria acts as a pathogenic risk-factor for chronic abdominal pain and diarrhea in post-cholecystectomy syndrome patients: a gut microbiome metabolomics study. Med. Sci. Monit. 25, 7312–7320. doi: 10.12659/msm.915984
Koopmans, S. J., and Schuurman, T. (2015). Considerations on pig models for appetite, metabolic syndrome and obese type 2 diabetes: from food intake to metabolic disease. Eur. J. Pharmacol. 759, 231–239. doi: 10.1016/j.ejphar.2015.03.044
Lebret, B., and Mourot, J. (1998). Characteristics and quality of pig adipose tissues. Influence of rearing factors. Prod. Anim. 11, 131–143.
Lemieux-Labonte, V., Simard, A., Willis, C. K. R., and Lapointe, F. J. (2017). Enrichment of beneficial bacteria in the skin microbiota of bats persisting with white-nose syndrome. Microbiome 5:115.
Lithell, H., and Boberg, J. (1978). The lipoprotein lipase activity of adipose tissue from different sites in obese women and the relationships to the cell size. Int. J. Obes. 2, 47–52.
Liu, L., Fu, C., and Li, F. (2019). Acetate affects the process of lipid metabolism in rabbit liver, skeletal muscle and adipose tissue. Animals (Basel) 9:799. doi: 10.3390/ani9100799
Liu, Y., Li, F., He, L., Tan, B., Deng, J., Kong, X., et al. (2015). Dietary protein intake affects expression of genes for lipid metabolism in porcine skeletal muscle in a genotype-dependent manner. Br. J. Nutr. 113, 1069–1077. doi: 10.1017/s0007114514004310
Malcom, G. T., Bhattacharyya, A. K., Velez-Duran, M., Guzman, M. A., Oalmann, M. C., and Strong, J. P. (1989). Fatty acid composition of adipose tissue in humans: differences between subcutaneous sites. Am. J. Clin. Nutr. 50, 288–291. doi: 10.1093/ajcn/50.2.288
Marchesi, J. R., Adams, D. H., Fava, F., Hermes, G. D., Hirschfield, G. M., Hold, G., et al. (2016). The gut microbiota and host health: a new clinical frontier. Gut 65, 330–339. doi: 10.1136/gutjnl-2015-309990
Miller, T. L. (1978). The pathway of formation of acetate and succinate from pyruvate by Bacteroides succinogenes. Arch. Microbiol. 117, 145–152. doi: 10.1007/bf00402302
Nissen, S. L., and Abumrad, N. N. (1997). Nutritional role of the leucine metabolite β-hydroxy β-methylbutyrate (HMB). J. Nutr. Biochem. 8, 300–311. doi: 10.1016/s0955-2863(97)00048-x
Niu, M., Xiang, L., Liu, Y., Zhao, Y., Yuan, J., Dai, X., et al. (2017). Adiponectin induced AMP-activated protein kinase impairment mediates insulin resistance in Bama mini-pig fed high-fat and high-sucrose diet. Asian Australas. J. Anim. Sci. 30, 1190–1197. doi: 10.5713/ajas.17.0006
Park, B. S., Henning, P. C., Grant, S. C., Lee, W. J., Lee, S. R., Arjmandi, B. H., et al. (2013). HMB attenuates muscle loss during sustained energy deficit induced by calorie restriction and endurance exercise. Metabolism 62, 1718–1729. doi: 10.1016/j.metabol.2013.06.005
Proença, A. R. G., Sertié, R. A. L., Oliveira, A. C., Campaãa, A. B., Caminhotto, R. O., Chimin, P., et al. (2014). New concepts in white adipose tissue physiology. Braz. J. Med. Biol. Res. 47, 192–205.
Raclot, T., Groscolas, R., Langin, D., and Ferré, P. (1997). Site-specific regulation of gene expression by n-3 polyunsaturated fatty acids in rat white adipose tissues. J. Lipid Res. 38, 1963–1972. doi: 10.1016/s0022-2275(20)37127-3
Rodríguez, V. M., Picó, C., Portillo, M. P., Teresa Macarulla, M., and Palou, A. (2002). Dietary fat source regulates ob gene expression in white adipose tissue of rats under hyperphagic feeding. Br. J. Nutr. 87, 427–434. doi: 10.1079/bjnbjn2002570
Shen, J., Obin, M. S., and Zhao, L. (2013). The gut microbiota, obesity and insulin resistance. Mol. Aspects Med. 34, 39–58. doi: 10.1016/j.mam.2012.11.001
Song, B., Zhong, Y. Z., Zheng, C. B., Li, F. N., Duan, Y. H., and Deng, J. P. (2019). Propionate alleviates high-fat diet-induced lipid dysmetabolism by modulating gut microbiota in mice. J. Appl. Microbiol. 127, 1546–1555. doi: 10.1111/jam.14389
Topping, D. L., and Clifton, P. M. (2001). Short-chain fatty acids and human colonic function: roles of resistant starch and nonstarch polysaccharides. Physiol. Rev. 81, 1031–1064. doi: 10.1152/physrev.2001.81.3.1031
Wang, Q., Liu, S., Zhai, A., Zhang, B., and Tian, G. (2018). AMPK-mediated regulation of lipid metabolism by phosphorylation. Biol. Pharm. Bull. 41, 985–993. doi: 10.1248/bpb.b17-00724
Wang, Y., Yao, W., Li, B., Qian, S., Wei, B., Gong, S., et al. (2020). Nuciferine modulates the gut microbiota and prevents obesity in high-fat diet-fed rats. Exp. Mol. Med. 52, 1959–1975. doi: 10.1038/s12276-020-00534-2
Wang, Z., Yuan, D., Duan, Y., Li, S., and Hou, S. (2018). Key factors involved in obesity development. Eat. Weight Disord. 23, 267–274. doi: 10.1007/s40519-017-0428-3
Wheatley, S. M., El-Kadi, S. W., Suryawan, A., Boutry, C., Orellana, R. A., Nguyen, H. V., et al. (2014). Protein synthesis in skeletal muscle of neonatal pigs is enhanced by administration of beta-hydroxy-beta-methylbutyrate. Am. J. Physiol. Endocrinol. Metab. 306, E91–E99.
Wilson, J. M., Grant, S. C., Lee, S. R., Masad, I. S., Park, Y. M., Henning, P. C., et al. (2012). Beta-hydroxy-beta-methyl-butyrate blunts negative age-related changes in body composition, functionality and myofiber dimensions in rats. J. Int. Soc. Sports Nutr. 9:18. doi: 10.1186/1550-2783-9-18
Xu, P., Wang, J., Hong, F., Wang, S., Jin, X., Xue, T., et al. (2017). Melatonin prevents obesity through modulation of gut microbiota in mice. J. Pineal. Res. 62:e12399. doi: 10.1111/jpi.12399
Yamashita, H. (2016). Biological function of acetic acid-improvement in obesity and glucose tolerance by acetic acid in type 2 diabetic rats. Crit. Rev. Food. Sci. Nutr. 56 (Suppl. 1), S171–S175.
Yamashita, H., Fujisawa, K., Ito, E., Idei, S., Kawaguchi, N., Kimoto, M., et al. (2007). Improvement of obesity and glucose tolerance by acetate in type 2 diabetic Otsuka Long-Evans Tokushima Fatty (OLETF) rats. Biosci. Biotechnol. Biochem. 71, 1236–1243. doi: 10.1271/bbb.60668
Yamashita, H., Maruta, H., Jozuka, M., Kimura, R., Iwabuchi, H., Yamato, M., et al. (2009). Effects of acetate on lipid metabolism in muscles and adipose tissues of type 2 diabetic Otsuka Long-Evans Tokushima Fatty (OLETF) rats. Biosci. Biotechnol. Biochem. 73, 570–576. doi: 10.1271/bbb.80634
Yang, Y., Wu, Z., Meininger, C. J., and Wu, G. (2015). L-Leucine and NO-mediated cardiovascular function. Amino Acids 47, 435–447. doi: 10.1007/s00726-014-1904-y
Yao, K., Duan, Y., Li, F., Tan, B., Hou, Y., Wu, G., et al. (2016). Leucine in obesity: therapeutic prospects. Trends Pharmacol. Sci. 37, 714–727. doi: 10.1016/j.tips.2016.05.004
Yin, J., Li, Y., Han, H., Chen, S., Gao, J., Liu, G., et al. (2018). Melatonin reprogramming of gut microbiota improves lipid dysmetabolism in high-fat diet-fed mice. J. Pineal. Res. 65:e12524. doi: 10.1111/jpi.12524
Yin, Y. L., McEvoy, J., Schulze, H., Hennig, U., Souffrant, W.-B., and McCracken, K. (2000). Apparent digestibility (ileal and overall) of nutrients and endogenous nitrogen losses in growing pigs fed wheat (var. Soissons) or its by-products without or with xylanase supplementation. Live. Prod. Sci. 62, 119–132. doi: 10.1016/s0301-6226(99)00129-3
Zeng, H., Larson, K. J., Cheng, W. H., Bukowski, M. R., Safratowich, B. D., Liu, Z., et al. (2020). Advanced liver steatosis accompanies an increase in hepatic inflammation, colonic, secondary bile acids and Lactobacillaceae/Lachnospiraceae bacteria in C57BL/6 mice fed a high-fat diet. J. Nutr. Biochem. 78:108336. doi: 10.1016/j.jnutbio.2019.108336
Zhang, L., Li, F., Guo, Q., Duan, Y., Wang, W., Yang, Y., et al. (2021). Different proportions of branched-chain amino acids modulate lipid metabolism in a finishing pig model. J. Agric. Food. Chem. 69, 7037–7048. doi: 10.1021/acs.jafc.1c02001
Zhang, L., Li, F., Guo, Q., Duan, Y., Wang, W., Zhong, Y., et al. (2020). Leucine supplementation: a novel strategy for modulating lipid metabolism and energy homeostasis. Nutrients 12:1299. doi: 10.3390/nu12051299
Zhang, L., Shi, M., Ji, J., Hu, X., and Chen, F. (2019). Gut microbiota determines the prevention effects of Luffa cylindrica (L.) roem supplementation against obesity and associated metabolic disorders induced by high-fat diet. FASEB J. 33, 10339–10352. doi: 10.1096/fj.201900488r
Zheng, C., Song, B., Guo, Q., Zheng, J., Li, F., Duan, Y., et al. (2021). Alterations of the muscular fatty acid composition and serum metabolome in bama xiang mini-pigs exposed to dietary beta-hydroxy beta-methyl butyrate. Animals 11:1190. doi: 10.3390/ani11051190
Zheng, J., Xiao, H., Duan, Y., Song, B., Zheng, C., Guo, Q., et al. (2021). Roles of amino acid derivatives in the regulation of obesity. Food Funct. 12, 6214–6225. doi: 10.1039/d1fo00780g
Keywords: β-hydroxy-β-methylbutyrate, lipid metabolism, gut microbiota, acetic acid, AMPKα, Bama Xiang mini-pigs
Citation: Zheng J, Zheng C, Song B, Guo Q, Zhong Y, Zhang S, Zhang L, Duan G, Li F and Duan Y (2021) HMB Improves Lipid Metabolism of Bama Xiang Mini-Pigs via Modulating the Bacteroidetes-Acetic Acid-AMPKα Axis. Front. Microbiol. 12:736997. doi: 10.3389/fmicb.2021.736997
Received: 06 July 2021; Accepted: 28 July 2021;
Published: 16 August 2021.
Edited by:
Jia Yin, Hunan Normal University, ChinaReviewed by:
Shuai Zhang, China Agricultural University, ChinaTarique Hussain, Nuclear Institute for Agriculture and Biology, Pakistan
Miao Yu, Institute of Animal Science, Guangdong Academy of Agricultural Sciences, China
Copyright © 2021 Zheng, Zheng, Song, Guo, Zhong, Zhang, Zhang, Duan, Li and Duan. This is an open-access article distributed under the terms of the Creative Commons Attribution License (CC BY). The use, distribution or reproduction in other forums is permitted, provided the original author(s) and the copyright owner(s) are credited and that the original publication in this journal is cited, in accordance with accepted academic practice. No use, distribution or reproduction is permitted which does not comply with these terms.
*Correspondence: Yehui Duan, ZHVhbnllaHVpQGlzYS5hYy5jbg==
†These authors have contributed equally to this work