- 1INRAE, Oniris, BIOEPAR, Nantes, France
- 2Department of Veterinary Microbiology and Immunology, Western College of Veterinary Medicine, University of Saskatchewan, Saskatoon, SK, Canada
- 3INRAE, Avignon Université, UMR SQPOV, Avignon, France
- 4SECALIM, INRAE, Oniris, Nantes, France
- 5Laboratory of Animal Health Economics, Aristotle University of Thessaloniki, Thessaloniki, Greece
- 6Univ Rouen Normandie, Univ Caen Normandie, CNRS, M2C, UMR 6143, Rouen, France
- 7Chaire Agroalimentaire du Cnam, Conservatoire des Arts et Métiers, EPN7, Ploufragan, France
- 8Retired, Brittany, France
- 9Clinique des Tourbières, Frasne, France
- 10Risk Assessment Department, ANSES, Maisons-Alfort, France
- 11DGA Maîtrise NRBC, Vert-le-Petit, France
- 12Hygiene and Quality of Poultry and Pig Products Unit, ANSES, French Agency for Food, Environmental and Occupational Health Safety, Ploufragan, France
- 13Institut Pasteur, Université Paris Cité, CNR Bactéries anaérobies et Botulisme, Paris, France
- 14Department of Clinical Sciences, Faculty of Veterinary Medicine, Université de Montréal, Saint-Hyacinthe, QC, Canada
- 15Department of Veterinary and Animal Sciences, University of Copenhagen, Frederiksberg C, Denmark
Clostridium botulinum is the main causative agent of botulism, a neurological disease encountered in humans as well as animals. Nine types of botulinum neurotoxins (BoNTs) have been described so far. Amongst these “toxinotypes,” the A, the B and E are the most frequently encountered in humans while the C, D, C/D and D/C are mostly affecting domestic and wild birds as well as cattle. In France for instance, many cases and outbreaks are reported in these animal species every year. However, underestimation is very likely at least for avifauna species where the detection of dead animals can be challenging. Knowledge about BoNTs C, D, C/D, and D/C and the diseases they cause in animals and humans is still scarce and unclear. Specifically, the potential role of animal botulism outbreaks in cattle and poultry as a source of human illness needs to be further assessed. In this narrative review, we present the current knowledge about toxinotypes C, D, C/D, and D/C in cattle and poultry with, amongst various other aspects, their epidemiological cycles. We also discuss the zoonotic potential of these toxinotypes and some possible ways of risk mitigation. An adapted and effective management of botulism outbreaks in livestock also requires a better understanding of these less common and known toxinotypes.
1. Introduction
Botulism is a human and animal neurological disease caused by the action of bacterial neurotoxins produced by Gram-positive bacteria of the genus Clostridium (Hodowanec and Bleck, 2015). Typically, a flaccid paralysis, that can lead in worst cases to respiratory paralysis and heart failure causing death, is observed (Torrens, 1998; Hodowanec and Bleck, 2015; Le Maréchal et al., 2016b). Nine types of botulinum neurotoxins (BoNTs) or toxinotypes have been described (Peck et al., 2017; Doxey et al., 2018). While human botulism is mostly associated with types A, B and E and less frequently with types F, different types are associated with animal botulism, mainly in birds (wild and domestic) and cattle (Le Maréchal et al., 2016b). In birds, the toxinotypes involved are the mosaic C/D (majority), mosaic D/C, D and C (rarely) and exceptionally type E. On a world-wide basis, avian botulism is the most significant disease of waterbirds. Outbreaks in wild birds have been reported worldwide, except in Antarctica, with losses that have sometimes exceeded 50,000 dead birds (Rocke, 2006). In cattle, the toxinotypes involved are the mosaic D/C type (majority), C, mosaic C/D and rarely D. In France, the incidence over the last 10 years is on average about 10 to 30 outbreaks per year depending on the animal species considered (Le Maréchal et al., 2016b; Le Bouquin et al., 2022). However, this is probably an underestimation: in the avifauna (wild and non-captive) in particular, detection and reporting of dead birds is much less systematic than it could be for dead mammals. The literature about BoNT/C, D, C/D, and D/C mosaics (see Section 1.2) is definitely less abundant than for other toxinotypes. More specifically, the potential role of the outbreaks in cattle and poultry as a source of human contamination and ultimately of human botulism needs to be addressed. In this narrative review, we update the general knowledge about C, D, and mosaics C/D and D/C based on the situation in France, but we present this knowledge enhanced with a special focus on their zoonotic potential and related health consequences from a One Health perspective.
1.1. Clostridium botulinum – The bacteria
Clostridium botulinum consists of a group of Gram-positive, rod shaped, spore forming anaerobic bacteria (1.6–22 μm long and 0.5–2 μm wide) of the genus Clostridium whose common feature is the ability to synthesize a protein toxin called BoNT (Cato et al., 1986; Le Maréchal et al., 2016b; Poulain and Popoff, 2019), responsible for botulism, a severe neurological condition that causes flaccid paralysis. The genus Clostridium consists of about 200 species, of which about fifteen can synthesize toxins that cause disease in humans or animals (Poulain and Popoff, 2019). C. botulinum presents a great genetic diversity and is currently classified into three groups according to their biochemical, noteworthy proteolytic, characteristics (Poulain and Popoff, 2019). Thus, group I is proteolytic while groups II and III are non-proteolytic. Other species of the genus Clostridium can also produce BoNTs and constitute groups IV (so called C. argentinense), V (C. baratii) and VI (C. butyricum) of BoNT producing Clostridia (Smith et al., 2018). Group IV is proteolytic while groups V and VI are non-proteolytic.
The bacteria, in their vegetative form, are peritrichous and motile bacteria (Hodowanec and Bleck, 2015; Le Maréchal et al., 2016b). The metabolism of C. botulinum is of the chemo-organotrophic type, the end products of metabolism being acetic, butyric and propionic acids. Clostridia have a strictly anaerobic respiratory type. Some strains can tolerate low oxygen in their growing environment. Because of their metabolic characteristics, Clostridium species including C. botulinum are involved in degradation of organic matter. The physiological role of BoNTs is still not known but, according to DasGupta (2006), their presence is definitely not essential for the survival and the growth of C. botulinum strains (DasGupta, 2006).
1.2. Toxins
BoNTs are a heterogeneous family of proteins produced by C. botulinum as well as some strains of Clostridium butyricum and Clostridium baratii (Poulain and Popoff, 2019). BoNTs share a similar structure (DasGupta, 2006) and are synthetized as a single-chain polypeptide (approximately 150 kDa) that is cleaved by a protease into dichain proteins linked by a disulfide bond (Montecucco, 1986). The dichain protein is composed of a 50 kDa light chain (LC) with zinc-dependent protease activity and a 100 kDa heavy chain (HC) with an N-terminal translocation domain (Hn) and a C-terminal cell binding domain (Hc). BoNTs are divided into nine toxinotypes (A to H and X) based on neutralization of toxicity by specific antisera, using the mouse biological test and specific neutralizing antisera (Solomon and Lilly, 2001; Peck et al., 2017; Zhang et al., 2017b). BoNT genes have been sequenced from many strains and sequence comparisons have enabled the identification of sequence variations in each toxinotype. Thereby, BoNTs are divided into subtypes (Peck et al., 2017). There are eight subtypes identified for BoNT/A (A1 – A8), BoNT/B (B1 – B8) and BoNT/F (F1 – F8); twelve for BoNT/E (E1 – E12). No subtype has been identified for the other toxinotypes. However, there are two mosaic hybrid forms of BoNT/C and BoNT/D named BoNT/C/D and BoNT/D/C. BoNT/C/D is composed of the light chain of BoNT/C and the heavy chain of BoNT/D. BoNT/D/C is composed of the light chain of BoNT/D and the heavy chain of BoNT/C (Figure 1). BoNT/H is a BoNT/A and/F hybrid and might also been considered as BoNT/A/F (Barash and Arnon, 2014; Maslanka et al., 2016).
The consequence of sequence diversity in each toxinotype and subtype is not yet clear. We can speculate it might affect the properties of each BoNT including stability, receptor recognition, enzymatic activity, efficiency of entry into cells, and recognition by antibodies (identification and immunotherapy).
BoNTs are produced as protein complexes of different sizes (Schantz and Spero, 1967; Popoff, 2018). In the complex form, BoNTs are associated by non-covalent bounds with non-toxic proteins synthetized by the producing Clostridium. Two classes of complex have been described based on their composition. The Ha-BoNT complexes contain some hemagglutinins (Ha). The Ha-BoNT complexes are associated to BoNT/A (subtypes A1, A5 to 8), BoNT/B, C, D, C/D, D/C, and G. Then, the Orf-BoNT complexes contain the OrfX proteins (OrfX1 to 3) and P47 protein. The Orf-BoNT complexes are associated with BoNT/A (subtypes A2 to 4), E, F and X. All complexes contain the non-toxic non-hemagglutinin protein (NTNH).
All BoNT types have the same mode of action (Popoff, 2018). This includes four steps: (i) cell binding, (ii) internalization, (iii) membrane translocation of the light chain into the cytosol after reduction of disulfide bond, (iv) enzymatic cleavage of their target soluble N-ethylmaleimide-sensitive fusion protein attachment protein receptor (SNARE).
Each BoNT enters into demyelinated terminal nerve endings by binding to presynaptic membrane receptors constituted of a membrane glycoprotein and a ganglioside (Zhang et al., 2017a). The BoNT, bound to its receptor, is internalized by receptor-mediated endocytosis inside the synaptic vesicles (Binz and Rummel, 2009). Under the effect of acid pH in the vesicles, BoNT conformation changes with the reduction of the disulfide bond and BoNT translocates its light chain into the cytosol.
The LC, a Zn2+-dependent endopeptidase, cleaves the SNARE proteins with extremely high specificity. The BoNT/A and E cleave the 25 kD-Synaptosomal-Associated Protein (SNAP-25). The BoNT/B, D, F, G, H and X cleave the Vesicle Associated Membrane Protein or synaptobrevin (VAMP). The BoNT/C is able to cleave the SNAP-25 and the syntaxine (Gardner and Barbieri, 2018). Table 1 reports the sites of cleavage.
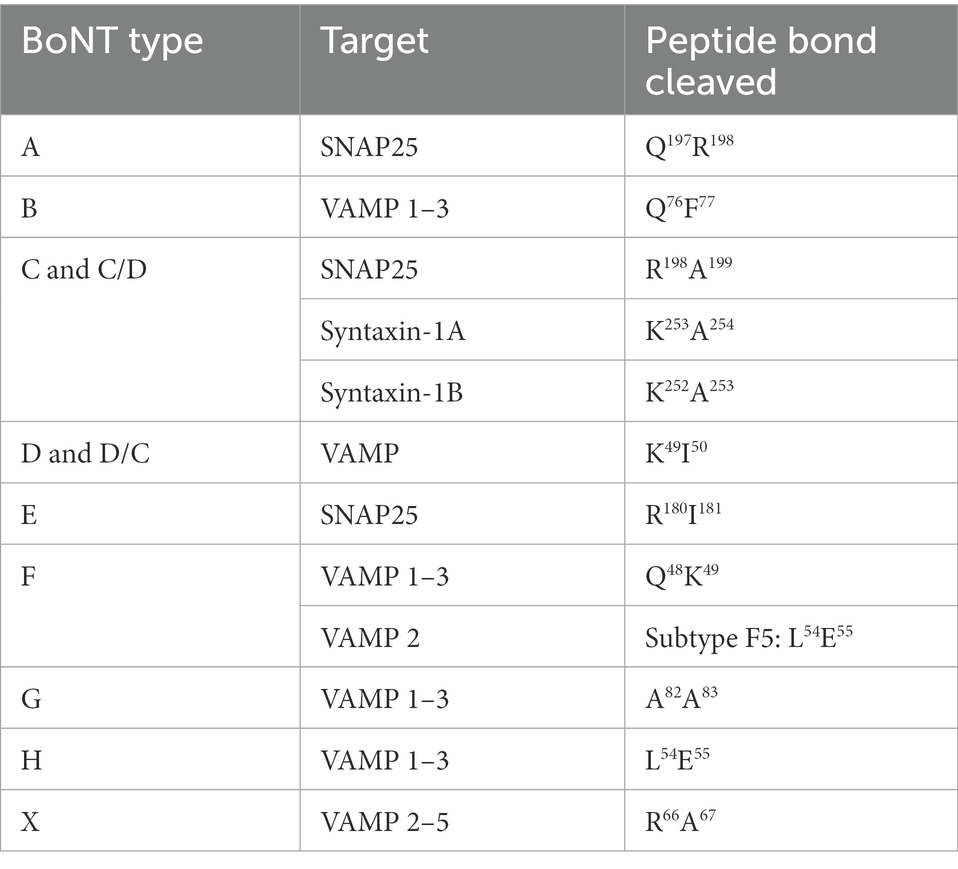
Table 1. BoNTs targets at nervous endings and their different sites of cleavage according to toxin types.
The cleavage of the SNARE inhibits the release of neurotransmitter acetylcholine. Then, the inhibition of the neurotransmission leads to a block of the neuronal activation of muscles resulting in flaccid paralysis. However, depending on the cleaved SNARE (VAMP, SNAP25 or syntaxin), the inhibition of neurotransmission varies in intensity and duration. Indeed, the BoNT duration of action is different depending on the type of toxin, ranging from weeks to several months. Table 2 reports the duration of action for the different types.
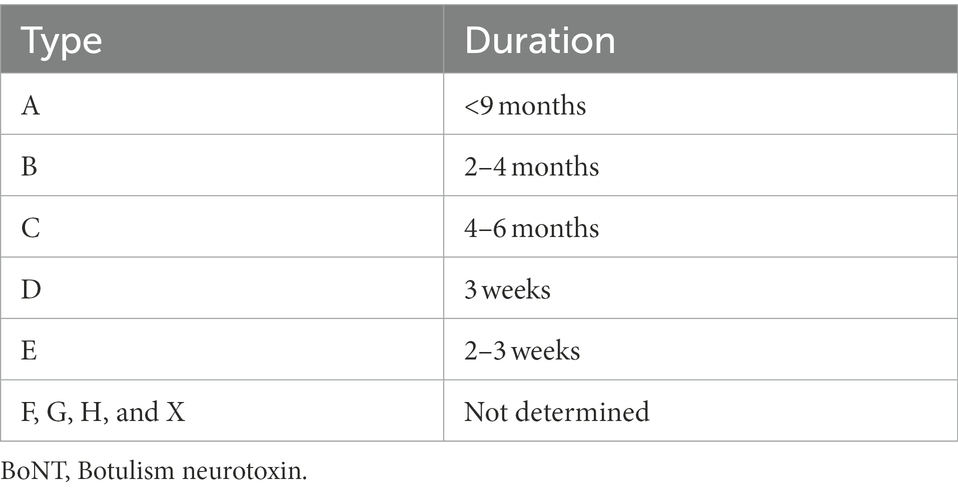
Table 2. Duration of the effect of different types of BoNT in mice (Pellett et al., 2015, 2018a,b; Gardner and Barbieri, 2018).
The BoNTs are the most potent toxins known. They are effective on human and animals, mainly mammalians and birds. Rossetto and Montecucco (2019) summarized the lethal dose values in mice by intraperitoneal route. Table 3 reports these lethal doses in mice. In mice, the toxicity is very similar by intravenous, intraperitoneal and intramuscular injection. By oral route, the lethal dose is at least a thousand times greater.
1.3. Detection methods
Clinical signs of botulism are evocative but not specific and laboratory analysis is required to firmly confirm the suspicion (Anniballi et al., 2013b). Two main strategies are currently applied to confirm botulism. One is based on the detection of BoNT in samples while the other one is based on the detection of BoNT-producing clostridia in samples. Such analyses, during epidemiological investigations, after an outbreak in animals for example, may also contribute to identify the source of contamination or the dissemination routes. This section presents methods mostly implemented in the detection of BoNT and BoNT-producing clostridia.
Each method has advantages and disadvantages. A combination of several methods is a good way to consolidate a diagnostic. However, this approach is costlier and may delay final results. This may be prohibitive under field conditions, especially for animal botulism.
The mouse bioassay (MBA) is still considered the gold standard in BoNT detection. Since BoNT is responsible for the clinical signs, BoNT detection is still considered the best way to confirm the diagnosis. MBA is indeed one of the most sensitive test available (10 pg/ml). MBA allows for the detection of BoNT activity and not only its presence. Nevertheless, MBA has major drawbacks, such as ethical issues and a few-day delay to get results. Moreover, there is no international standardization regarding sample preparation, interpretation of clinical signs, the number of mice to be tested, their genetic background, as well as age and weight (Lindström et al., 2010). Alternative methods, such as ELISA tests (Hansbauer et al., 2016; Masters and Palmer, 2021) and Endopep-Mass Spectrometry (MS) (Björnstad et al., 2014; Drigo et al., 2020; Frye et al., 2020), have been developed and these have a lower limit of detection than MBA. However, the modalities of the validation of ELISA tests targeting either BoNT or antibodies against BoNT in animal blood are not always provided in published studies or does not always follow an official standardized protocol (Worbs et al., 2015). Moreover, controls are not systematically present, and this raises questions about conclusions that can be drawn from such tests (Lindström et al., 2006; Worbs et al., 2015). Attention should also be paid about the type of antibodies used in the ELISA tests (Lindström et al., 2006; Worbs et al., 2015). While ELISA tests will detect the presence of BoNT, Endopep-MS, like MBA,detects BoNT enzymatic activity, using synthetic peptides and further identification of cleavage product by either immunologic tests or mass spectrometry (Barr et al., 2005).
BoNT-producing clostridia are mostly detected using PCR assay after an enrichment step by culture (Anniballi et al., 2013a; Le Maréchal et al., 2017). Several methods have been published in the literature (Lindström et al., 2010). However, there is no universally agreed upon protocol for the detection of BoNT-producing clostridia in animals (Lindström et al., 2010). While a standard for a PCR detection of BoNT-producing clostridia (type A, B, E, and F) in food does exist (ISO/TC 34/SC 9 Microbiology, 2013), no reference text is available for the detection in animal samples or, more broadly, for PCR detection methods for clostridia producing BoNT/C, D, C/D, and D/C (Lindström et al., 2010). The enrichment step, preceding DNA extraction and detection, which impact the number of bacterial cells available would also deserve standardization. Moreover, as underlined by Lindström et al. (2010) and to our knowledge at the time of publication, there is no selective medium available to isolate C. botulinum group III (Lindström et al., 2010).
Recently, reverse-transcription real-time PCR assays targeting BoNT/C and D genes have been developed. It consists in the detection of BoNT complex–associated toxin gene RNA/DNA as an indirect method for the detection of BoNT presence in a sample (Masters and Palmer, 2021).
France relies mainly on two methods, MBA and PCR, implemented in two reference laboratories (the National Reference Center that is involved in human and animal botulism diagnosis and the National Reference Laboratory that is involved in animal botulism) (Vanhomwegen et al., 2013; Le Maréchal et al., 2017). In humans, botulism is usually confirmed by BoNT detection in serum, gastric samples, feces, vomitus or suspected food and/or by the detection of BoNT producing clostridia in feces or suspected food (Maupas et al., 1976). Attention should be paid to collect specimen(s) immediately for biological diagnosis confirmation as soon as botulism is clinically suspected. In particular, serum should be collected when BoNT is circulating, i.e., at the earliest at the onset of clinical signs and before the administration of antitoxin treatment (Curtiaud et al., 2022). In animals, the sampling material will vary depending on species. When analyzing serum, BoNT detection are often negative in bovines (Bano, 2019), turkeys (Le Maréchal et al., 2016a) as well as in horses (Johnson et al., 2015), even in animals with clinical signs. Analysis of other samples such as fecal material and organ tissue, using PCR assay or by use of a combination of methods to detect both BoNT and BoNT-producing clostridia markedly increases the reliability of the diagnosis. In bovine, the liver, ruminal or intestinal content, manure, suspected feed or environmental samples can be tested. The liver is recommended for avian species (Le Maréchal et al., 2016a). In fish, the presence of BoNTs in blood or the digestive tract, and the detection of BoNT-producing clostridia in the digestive tract can be used to confirm botulism. The use of Danio rerio (zebra fish) instead of mice for BoNT detection in fishes has been suggested because of its higher sensitivity to BoNT/E (Chatla et al., 2014, 2016).
2. Clostridium botulinum and its environment
2.1. Distribution and prevalence
Clostridium botulinum is a spore-forming anaerobic bacterium widely distributed in nature (Long and Tauscher, 2006; Espelund and Klaveness, 2014). C. botulinum can be found in raw water storage areas and waste water (Anza et al., 2014), trout farms (Cann and Taylor, 1982; Eklund et al., 1984), fish and environmental samples from coastal and wetland areas. Forage botulism or feed-related botulism seems to be an important pathway for farm animals. The species is present in all continents except Antarctica to our knowledge, in soil, dust, water and marine and freshwater sediments. The spores can persist in soils and sediments for decades (Long and Tauscher, 2006). C. botulinum is occasionally present in the intestinal content of healthy animals (fish, birds and mammals) (Espelund and Klaveness, 2014). The worldwide distribution and prevalence of C. botulinum differs according to regions for their toxin type. However, the ecological factors that determine the distribution remain unknown. For group I, C. botulinum type A occurs at a higher frequency in prevalence studies performed in the western United States while C. botulinum proteolytic type B dominates in the eastern United States (Shapiro et al., 1998). Regarding group II, non-proteolytic C. botulinum predominates in western Europe. C. botulinum type C and D are commonly found in Europe and in Australia (Woudstra et al., 2012; Masters and Palmer, 2021). C. botulinum E is predominately identified in aquatic habitats in northern Europe, in the United States and Canada, noteworthy in the Great Lakes region, and in the North Pacific area (Bott et al., 1966, 1968; Laycock and Loring, 1972; Horowitz, 2010; Austin and Leclair, 2011; Leclair et al., 2013, 2017). In Asia, types A to F are widely found in China (Yamakawa et al., 1988; Gao et al., 1990; Fu and Wang, 2008) but the presence of only types B, C and E was described in Japan (Yamakawa et al., 1988; Yamakawa and Nakamura, 1992; Umeda et al., 2013). All types except E are found in Indonesia (Suhadi et al., 1981). Types A, B and D are present in Australia (Eales and Turner, 1952; Murrell and Stewart, 1983; Koepke et al., 2008). In Africa, types A to D are detected in Zambia and Kenya (Nightingale and Ayim, 1980; Yamakawa et al., 1990; Karasawa et al., 2000).
2.2. Ecology
Certain plants (in particular algae) and invertebrates (in particular naturally resistant mollusks and insect larvae) can store types C, D, C/D, or D/C and type E toxins in their tissues or cells, and they have been identified as mechanical vectors (Hubálek and Halouzka, 1991; Rocke et al., 1999; Anza et al., 2016). Moreover, fish and birds can harbor the bacteria in their digestive tract as observed in the USA (Bott et al., 1968), and, depending on their sensitivity, may develop the disease. The decomposition of plant species and contaminated animal carcasses (such as fish, birds and mammals) is an optimal condition for bacterial development and toxin production. These conditions facilitate the transmission of the bacterium to species that are sensitive to the toxin, such as livestock (poultry, cattle) and humans consuming contaminated products.
Types C, D and mosaics C/D or D/C are, in nature, closely associated with wetlands rich in sediments (e.g., marshes, ponds and lakes) which are favorable to the bacterial development (Wobeser et al., 1987). The contamination of susceptible birds and the initiation of a “carcass-maggot” amplifying cycle can lead to the development of outbreaks of botulism in the avifauna (Wobeser, 1997; Soos and Wobeser, 2006). Type E, mainly identified in the northernmost regions of the northern hemisphere, is closely associated with aquatic, marine or freshwater ecosystems (Dolman, 1957; Haagsma, 1991; Horowitz, 2010; Espelund and Klaveness, 2014; Palmer et al., 2019). Carriage by fish is most often intestinal (Bott et al., 1966, 1968; Yule et al., 2006); the contamination can lead to mortality in certain species more sensitive to toxin E. The disease can also spread to aquatic or coastal birds (fish-eating birds in particular), sometimes causing the death of thousands of individuals (EPA, 2013).
Clostridium botulinum eradication is inconceivable because of its close association with natural environments in which it survives or evolves. It appears that the lethal outbreaks of botulism occasionally affecting the avifauna exposed to types C, D, C/D, or D/C and E result from multiple factors (weather, organic pollution generated by human activities, etc.) that contribute to the disruption of ecosystems and make them more favorable to outbreak occurrence (Sandler et al., 1993; Rocke et al., 1999; Lafrancois et al., 2011). Knowing these factors can make possible the identification and the implementation of control management measures (in particular the collection and destruction of animal carcasses in areas at risk) (Inger et al., 2016; Reynolds et al., 2021). Some studies demonstrated an interaction of environmental conditions (temperature, pH, sodium chloride and organic matter concentrations) affecting spore germination, outgrowth and toxin formation and proposed applied mathematical models (Barras and Kadlec, 2000; Chea et al., 2000; Zhao et al., 2003).
The prevalence of C, D, C/D, or D/C and E toxin types in agricultural fields seems quite low, considering the rare data regarding the digestive carriage in livestock and the presence of spores in farm environments (grassland, cultivated land) far from wetlands for types C, D, C/D, or D/C, or far from contaminated coasts, lakes and rivers for type E (Smith and Milligan, 1979; Gessler and Böhnel, 2006). Although relatively infrequent (even for type E), the emergence of cases of botulism in herds or flocks (often infected via contaminated feed or water) are worrisome.
3. Zoonotic aspects
3.1. Human botulism forms
Human botulism is a serious and potentially lethal disease. BoNT is the most potent bacterial toxin and definitely one of the most potent known poison (Arnon et al., 2001; Kumar et al., 2016). Botulism occurs worldwide but the number of reported cases varies between regions and countries. This variation may be due not only to real differences in incidence, but also to underreporting.
Several types of botulism are described in humans, depending on the mode of contamination and exposure to the toxin. Foodborne botulism, infant botulism, adult botulism, wound botulism, inhalation botulism and iatrogenic botulism are thus reported (Anniballi et al., 2013a).
Foodborne botulism (poisoning) is the main cause of human botulism in Europe (Popoff, 2018). It results from digestive intoxication due to the presence of the preformed toxin in the food. It is present on all continents and is of variable incidence. Overall, foods associated to foodborne botulism are very diverse (Lund and Peck, 2013). In Europe, it mainly involves homemade preserved products. Food of concern are mainly cured meats and canned vegetables, in Europe (Meurens et al., 2021; Le Bouquin et al., 2022). In a few rare cases, commercial foods or restaurant meals have been involved. After ingestion, the toxin is absorbed by the duodenum and jejunum and then passes into the bloodstream. BoNT resists gastric acidity and digestive enzymes because it forms a complex with a group of neurotoxin associated proteins or NAPs (Chellappan et al., 2014). BoNT/A, B and E are associated with foodborne botulism.
Infant botulism (toxi-infection) occurs when spores of C. botulinum are ingested from the environment or with honey (Popoff, 2018). Then, the bacteria germinate and multiply in the gastrointestinal tract and release the toxins (A, B, or F) produced in situ. This form of botulism has been reported in children from 6 days to 12 months of age, but mostly in infants from 2 to 8 months of age. Small doses of spores (10–100) are sufficient to induce intestinal colonization and toxin production (Popoff, 2018). The intestinal microbiota, which has normally an inhibitory effect on the growth of C. botulinum, does not play its inhibitory role in infants below the age of one (Popoff, 2018; Douillard et al., 2022).
Adult botulism (toxi-infection) is a rare and poorly understood form of botulism similar to infant botulism but occurring in adults (Fenicia et al., 2007; Freund et al., 2017; Harris et al., 2020). It usually involves type A toxins, but type B and F toxins have sometimes been implicated. In these patients, spores, bacteria, and toxins are found in the stool and spores may also be found in leftover food, but no preformed toxins are found. Dysbiosis is suggested but the exact causes of this alteration of the intestinal microbiota remain unknown. The intestinal microbiota, normally well established and fully functional after infancy, prevents bacterial colonization of the digestive tract. Possible imbalance of the microbiota (immune depression, prolonged antibiotic use or intestinal surgery) could be involved.
Wound botulism (inoculation) is a consequence of the contamination of wounds by C. botulinum spores (Popoff, 2018). At the vicinity of wounds, the bacteria can grow and produce neurotoxin (mostly type A or B). Since the 1980s, this rare form of botulism has been almost exclusively linked to the use of injectable drugs (Neeki et al., 2021). Previously, cases of wound botulism occurred in open fractures, deep traumatic wounds, or puncture wounds contaminated with foreign elements. The lesions have to be deep enough to enable anaerobic conditions required for spore germination and toxin production.
Inhalation botulism is also very rare (Popoff, 2018). A few cases have been reported amongst laboratory workers preparing concentrated BoNTs by continuous centrifugation (Holzer, 1962) and in a few individuals following the intranasal use of contaminated cocaine (MacDonald et al., 1985). The presence of C. botulinum sinusitis or direct absorption through a nasal mucosa have been suggested as etiological hypotheses. Iatrogenic botulism (very rare) will not be presented here.
The treatment of botulism is symptomatic and involves supportive care, intubation and mechanical ventilation when needed as well as administration of botulinum antitoxin (Rasetti-Escargueil and Popoff, 2019; Rao et al., 2021). Prevention is also possible using vaccines and several approaches, including DNA-based, viral vector-based, and recombinant protein-based vaccines, have been developed and tested (Sundeen and Barbieri, 2017; Rasetti-Escargueil and Popoff, 2019).
3.2. Surveillance data
In Europe, botulism is monitored through the surveillance of zoonoses and zoonotic agents and the protection of workers. In France for instance, botulism is a notifiable disease, in humans, and to some extent in animals, regardless of the species affected (Le Bouquin et al., 2022). Reporting the disease has been mandatory in France for human disease since 1986 and between 2006 and 2022 for poultry, cattle, and wild birds.
If reporting of severe forms of human botulism is probably exhaustive, many animal botulism cases remain suspicious and are not formally reported, especially for wild birds. Surveillance of botulism in wild birds is based on event-based surveillance and unquantifiable.
A study conducted on human botulism surveillance in France over the last decade shows that the incidence of human botulism has been relatively stable over time with an average of ten outbreaks/year for a total of 100 cases (Le Bouquin et al., 2022). The incidence in Europe was around 0.02 cases/100,000 persons, similar to what has been observed in France. Similarly, animal botulism also appears to be relatively stable, although annual variations are observed (Le Bouquin et al., 2022). Each year, an average of 30 outbreaks are recorded in France on poultry farms, about 20 cases in wild birds and about 10 outbreaks in cattle, often involving a large number of animals. Few other animal species, including domestic carnivores, are affected by botulism in France. Botulism has been confirmed in 264 avian species representing 39 families (Rocke, 2006), among which Anatidae appears to be the most affected family in wild birds, at least in France (Ventujol et al., 2017). Regarding poultry, outbreaks have been reported in chicken broilers, turkeys, pheasants and to a lesser extent ducks, guinea fowls, laying hens, gooses, and quails (Souillard et al., 2014; Le Maréchal et al., 2016a, 2017; Ventujol et al., 2017).
Analysis of the toxin types occurring in France confirmed the predominance of types A and B in human botulism in both foodborne and infantile cases, and exceptionally type F (Mazuet et al., 2017; Rasetti-Escargueil et al., 2020; Le Bouquin et al., 2022). The C/D mosaic form is the predominant BoNT in birds in this country, even if BoNT/D is also observed, and only BoNT/D/C and C have been identified in recent years in cattle.
3.3. Lethal doses of BoNTs in humans
The preformed toxin (types A, B, E and F) are active in humans (Rasetti-Escargueil et al., 2020) after ingestion, injection, and inhalation; the skin offers protection against the toxin. The lethal doses in humans have been estimated by extrapolation from studies carried out in primates (Herrero et al., 1967; Franz et al., 1993). Table 4 summarizes these doses for the BoNT/A1 (Arnon et al., 2001). Regarding BoNT/B, Rasetti-Escargueil and collaborators (2020) indicate that the minimal toxic dose by ingestion in humans is about 4,000 mouse lethal doses (MLD) and a minimal dose of 30–100 ng of BoNT/B has also been reported to induce foodborne botulism in humans (Peck, 2009). The therapeutic use of BoNT in human has shown that the effect of BoNT/A and BoNT/B are comparable with a dose ratio 1:25 to 100 following the application (Bentivoglio et al., 2015). The other types of BoNTs are usually considered less toxic than type A but there are no estimated lethal doses available for humans. Compared to humans, the data available in monkeys by oral route (Rasetti-Escargueil et al., 2019) indicate that BoNT/B (160 MLD.kg−1) is the most toxic followed by the BoNT/A (650 MLD.kg−1), the BoNT/E (1,500 to 2,500 MLD.kg−1) and the BoNT/F (50,000 to 75,000 MLD.kg−1). The BoNT/C and D appear to be less potent for monkeys with death occurring at 100,000 MLD.kg−1 for BoNT/C and 600,000 MLD.kg−1 for BoNT/D. Otherwise, within each type, the toxicity of subtypes are different. For the BoNT/A, the subtypes A1 and A2 are the most potent and BoNT/A4 is the less toxic about a 1,000 fold compared to other subtypes A (Pellett et al., 2015).
3.4. BoNT/C, D, C/D, and D/C in humans
Outbreaks of botulism occur in cattle and poultry every year in many countries including France. These outbreaks are essentially caused by toxinotypes C, D, C/D, and D/C. Toxinotypes C, D, C/D, and D/C are generally not associated with human botulism. Nevertheless, the question of the zoonotic potential of these toxinotypes has emerged in humans. To the best of our knowledge, only an extremely limited number of cases of human botulism has been linked to toxinotypes C, D, C/D, and D/C (see Table 5). Most often, the few available data are rather old (before 2000), and more often published as conference proceedings than peer-reviewed publications in international journals. Moreover, no lethal dose for toxinotypes C, D, C/D, and DC was identified so far in humans. Some cases where botulism type C or D was suspected are presented in Table 5.
Maupas et al. (1976) detected BoNT/C in two out of four sera from patients suffering from botulism but excluded the hypothesis of intestinal botulism, explaining that C. botulinum type C could not produce toxins at 37°C. Thus, the hypothesis of foodborne botulism involving smoked chicken was preferred even if smoked chicken samples were never analyzed. In 1990, infant botulism type C was confirmed by the detection of a huge amount of BoNT/C in a stool sample collected from the patient suffering of botulism (Oguma et al., 1990). However, again and as for almost all cases of infant botulism, the origin could not be determined. In a recent case, the symptoms of botulism were concomitant with an outbreak of type C botulism in a family poultry farm, without biological confirmation in the patient (Martrenchar et al., 2019).
In parallel, type C botulism has been confirmed in primates (Dolman and Murakami, 1961; Smart et al., 1980; Silva et al., 2018) in facilities linked to the preparation of meals based on poorly preserved or poorly thawed poultry.
Type D botulism following the consumption of ham was suspected in Chad and presented in a communication to the French Academy of Medicine (Demarchi et al., 1958). BoNT and C. botulinum type D were detected in samples from this ham. However, the absence of further investigation in the patients and the conditions of sample collection (heterogeneity, delays, climatic conditions, transport, culture, etc.) impaired the confirmation of the zoonotic character of this outbreak.
Several hypotheses have been put forward to explain the near absence of human cases of types C, D, C/D, and D/C botulism: low host susceptibility, low human exposure or lack of surveillance. Low human susceptibility to C, D and mosaic toxins is the preferred hypothesis (Meurens et al., 2021). However, in vivo tests carried out using intramuscular route showed the “efficacy” of the toxin (particularly type C) (Eleopra et al., 1997, 2013). Thus, the low sensitivity could correspond to a low intestinal absorption of the toxins.
4. Botulism and animals
4.1. Epidemiological cycles
Clostridium botulinum is a bacterium present in both the digestive tract of animals and in the environment (soil, water, sediment, etc.) (Long and Tauscher, 2006; Le Maréchal et al., 2016b). Its ubiquitous character enables different possibilities to enter cattle herds and poultry flocks.
4.1.1. Epidemiological cycle of Clostridium botulinum in cattle farms
Clinical signs observed in cattle are due to ingestion of BoNTs and/or BoNT-producing clostridia by animals via drinking water (Doutre, 1969) or feeding (pastures (Popoff, 1989), forages (Bano et al., 2015) and on-farm manufactured feeds (Le Maréchal et al., 2019), silage (Myllykoski et al., 2009; Guizelini et al., 2019), or haylage previously contaminated with C. botulinum (Relun et al., 2017). Two major sources of contamination leading to bovine botulism outbreaks have been identified. One is the presence of animal carcasses (domestic or wild) that provide a substrate for the development of C. botulinum and production of BoNT (Le Maréchal et al., 2019). The second one is poultry manure, as poultry can be asymptomatic carriers of C. botulinum (Souillard et al., 2017, 2021). Contamination of feed or water by these two sources can occur directly with the presence of the dead animals or manure in the drinking water or in the feed. Contamination can also occur indirectly via contaminated equipment (e.g., tractor bucket), clothing [farmer, technician, veterinarian (Souillard et al., 2017, 2021)], or by airborne route (Hogg et al., 2008). Soil and feces, through intestinal carriage by animals, not only livestock but also other domestic or wild animals, can also be a source of contamination (Figure 2).
Table 6 presents the prioritization of different initial sources and contamination vehicles of C. botulinum (spore and vegetative forms and toxins) in a cattle farm, in decreasing order of importance. Contaminated poultry manure appears therefore to be the main vehicle of contamination of C. botulinum, with a direct or indirect link between the cattle farm and a poultry flock. The source of manure contamination is asymptomatic poultry carrying C. botulinum, and excreting it into the litter (Souillard et al., 2017, 2021). Contamination of water or feed by a dead animal is also a major source of contamination for outbreaks of bovine botulism (Galey et al., 2000; Le Maréchal et al., 2019). Regarding commercial feed, drinking water and cattle litter, these vehicles of contamination appear to be less important, due to good management practices that favor their control (discarding of manure and carcasses, storage conditions of feed, cleaning of equipment, no reuse of poultry litter...).
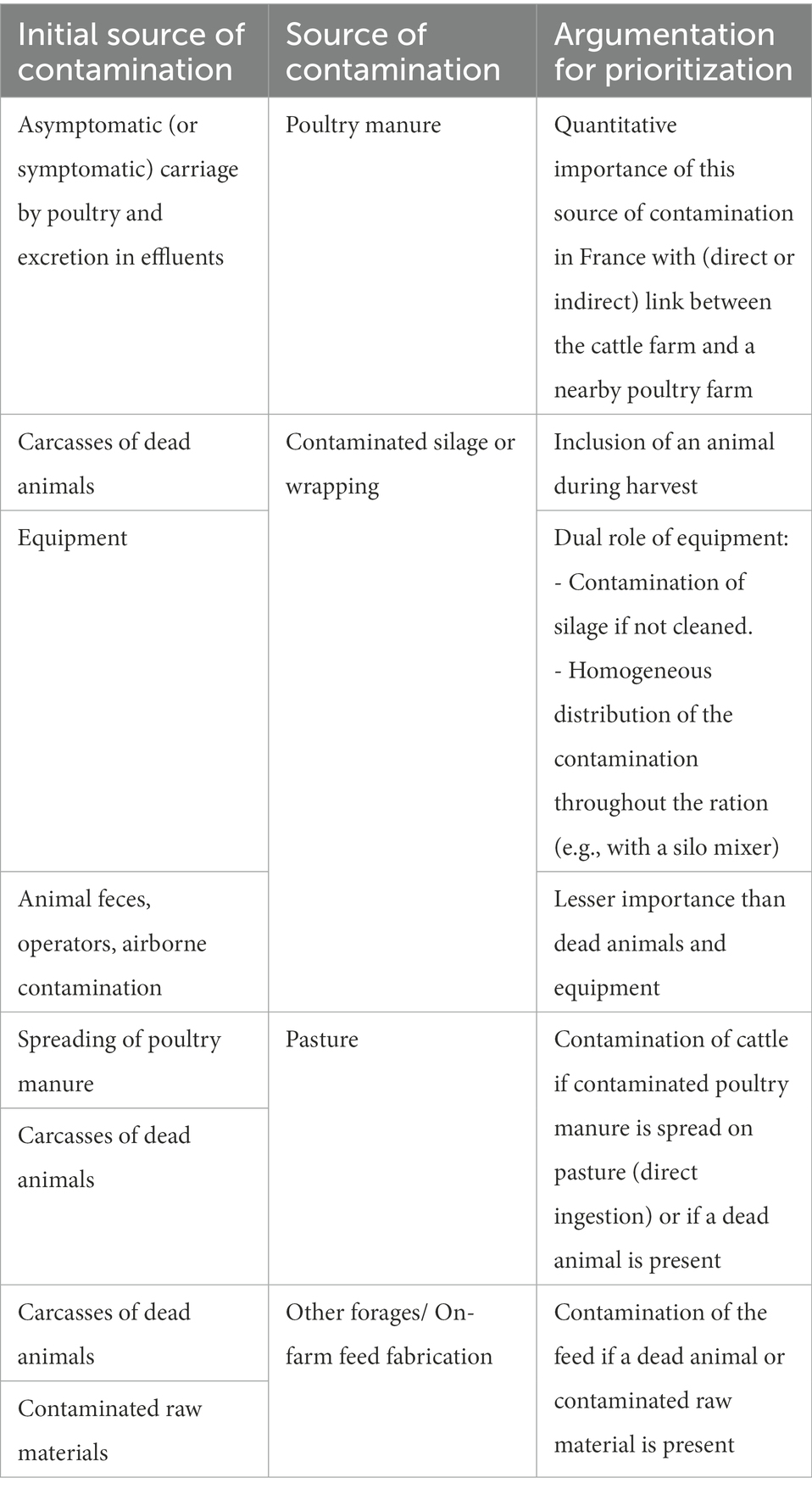
Table 6. Prioritization of importance (decreasing order) of the different initial sources and vehicles of C. botulinum contamination (spore, vegetative form and toxins) in a cattle farm (Anses, 2021).
4.1.2. Epidemiological cycle of Clostridium botulinum in poultry flocks
In poultry flocks, botulism outbreaks are mainly associated with carriage of C. botulinum by poultry (Dohms, 2008) and with any other animal species (rodents, wild birds and others) outside the farm that may enter and contaminate litter and/or feed via feces or carcasses (J.P. Vaillancourt, personal communication) (Figure 3; Table 7). Clothes and boots of personnel (e.g., farm employees, catchers, technicians) and the equipment used in the barns were also reported as possible sources of contamination (Figure 3).
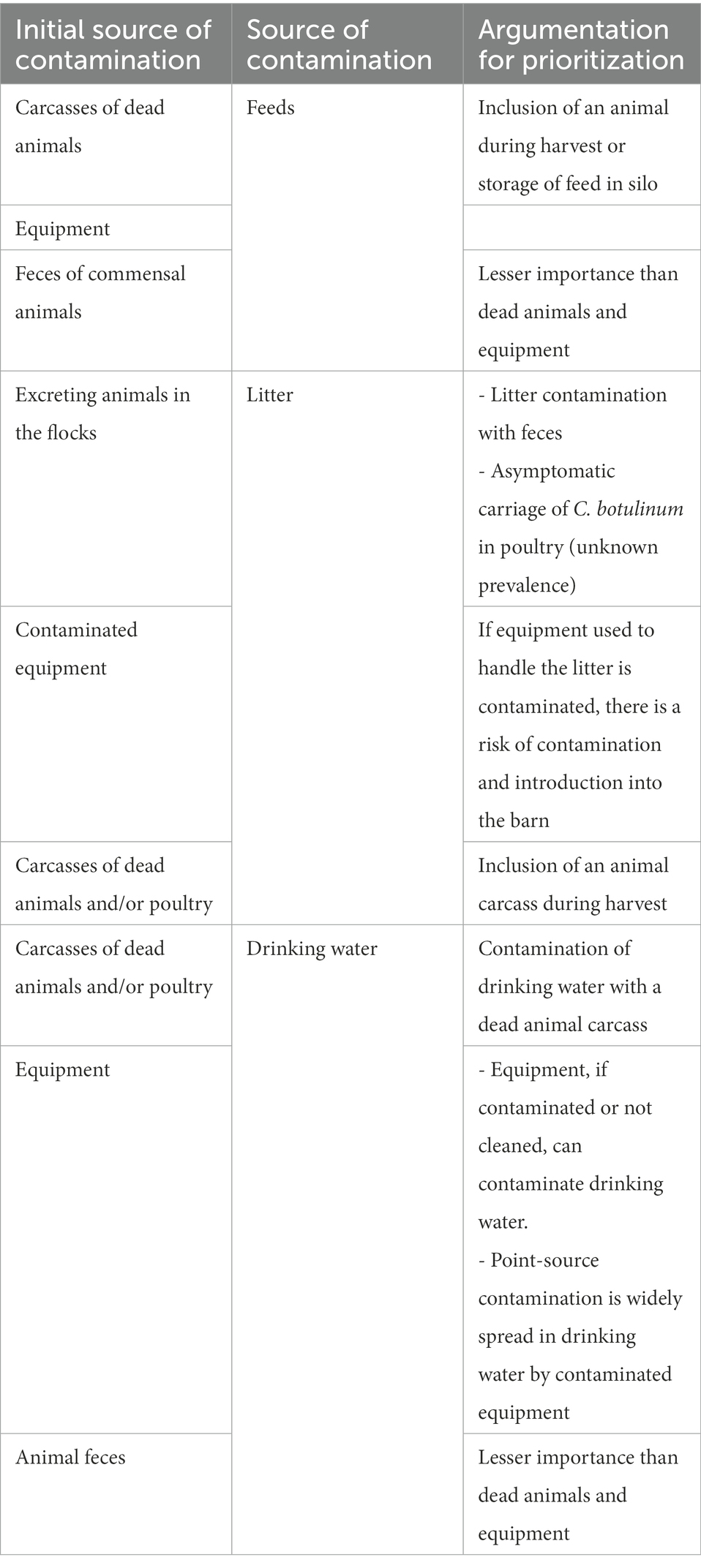
Table 7. Prioritization of importance (decreasing order) of the different initial sources and vehicles of C. botulinum contamination (spore, vegetative form and toxins) in a poultry flock.
Table 7 presents the different initial sources and vehicles of contamination of C. botulinum (spore, vegetative form and toxins) in a poultry flock and their prioritization. The feed appears to be the main vehicle of contamination of C. botulinum. Feed can be contaminated after inclusion of an infected animal at harvest or during storage in silos. In case of on-farm feed production, the harvest equipment can also be a vehicle of contamination (e.g., the same trailer for manure hauling and harvesting). For commercial feeds, good management practices are very important for controlling these fomites (Anniballi et al., 2013a). Litter (e.g., rice hulls) also appears to be a contamination vehicle for C. botulinum in poultry flocks (Bano et al., 2013; Fillo et al., 2021). Litter should be protected from wild bird droppings. Wild birds can be asymptomatic carriers of C. botulinum, although the prevalence and conditions of this carriage is unknown (Reed and Rocke, 1992; Reynolds et al., 2021).
4.2. Bovine botulism
Bovine botulism is mostly due to BoNT/C, D, C/D, and D/C (Woudstra et al., 2012; Bano, 2019) although some type A (Schocken-Iturrino et al., 1990; AFSSA, 2002; Rasetti-Escargueil et al., 2019; Frye et al., 2020) and B (Notermans et al., 1981; Divers et al., 1986; Wilson et al., 1995; Kelch et al., 2000; Yeruham et al., 2003) cases are sporadically reported in the literature. Presently, BoNT/D/C is the most common BoNT type involved in bovine botulism outbreaks in Europe.
Bovine botulism is considered to be due to the ingestion of pre-formed BoNT present in feed, water or any other sources of toxin. Animal carcasses, poultry manure as well as feed inappropriately stored provide favorable conditions for the growth of C. botulinum and the production of BoNTs. The role of C. botulinum cells themselves in the pathogenesis of bovine botulism needs to be clarified. Currently, the PCR is commonly used for the laboratory confirmation of bovine botulism through detection of the bacteria in enrichments of the liver, rumen, intestinal contents or other sample collected on symptomatic or dead animals. A biphasic distribution of mortalities may be observed. Acute clinical signs are noted in the herd 3–4 days after exposure and another set of clinical signs are recorded about 14–20 days after the first clinical observations. This clinical pattern may be linked to in situ BoNT production: a first wave of deaths being due to the ingestion of BoNT and a second wave being linked to in situ BoNT-production. This hypothesis is supported by the detection of vegetative cells in livers collected on animals that died from botulism (Le Maréchal et al., 2019).
Bovine botulism is characterized by a flaccid paralysis, generally starting by the paralysis of the tail and hind legs, then progressing to the head (Kummel et al., 2012; Bano, 2019). The incubation period varies between a couple of hours and 2 weeks. Three forms can be encountered: a peracute form with sudden lateral recumbency and death within a few hours after exposure; an acute form with typical clinical signs (anorexia, ataxia, apathy, weakness, dysphagia, increased salivation, paralysis, recumbency with head tucked into the flank, and dropping feed from the mouth) and death within 2–3 days after the onset of the disease; and a subacute form with attenuated clinical signs, from which animals might sometimes recover (mainly observed for type C outbreaks). Usually, major economic losses are associated with bovine botulism in cattle farms (up to 100% mortality rate). The differential diagnosis must include hypocalcemia, hypophosphatemia, some types of enterotoxemia, listeriosis, paralytic rabies, organophosphate or lead poisoning (Dorchies and Leze, 2017). Besides clinical signs, the diagnosis is based on the epidemiological context, in particular the suspicion of high risk sources such as poultry manure or the detection of a dead animal in the feed. As for other species, the diagnosis should be confirmed by the detection of BoNT and/or BoNT-producing clostridia in samples collected on animals or their closed environment. The analysis of several samples collected on various animals can be required in order to detect BoNT and/or BoNT-producing clostridia to confirm the diagnosis.
There is currently no curative treatment available for bovine botulism, although antitoxins might be successfully used, but at costs usually not sustainable for most farms (Guizelini et al., 2019). Vaccination can be used using toxoids targeting BoNTs. Several vaccines have been developed worldwide (Anniballi et al., 2013b) but market authorizations have been issued by only a few countries and may be only temporary as in France with a sole authorized vaccine. This “French” vaccine is only used for emergency vaccination to protect healthy animals in farms facing a botulism outbreak or to prevent disease recurrence. To prevent bovine botulism outbreaks, it is crucial to implement biosecurity measures as far as farm management and feed production and storage are concerned. The presence of animal carcasses in the feed (silage, haylage, cereals…) or feed stored under bad conditions (uncovered, exposed to wild bird droppings for example) may be the source of an outbreak. It is crucial to pay attention to all steps from feed harvesting to animal distribution. The implementation of dedicated biosecurity measures between poultry and cattle is essential to prevent cross-contamination between the two productions. The equipment needs to be cleaned and disinfected; in mixed farms, clothes and shoes should be changed between both productions.
4.3. Avian botulism
Botulism is widespread in most avian species, both wild and domestic. On a worldwide basis, avian botulism is the most significant disease of water birds. Some species, especially scavengers like vultures, are known to be resistant. For still unknown reasons, males tend to be more affected than females, in particular in turkey farms (Smart et al., 1983; Popp et al., 2012; Souillard et al., 2014).
Avian species are sensitive under experimental conditions to all BoNT types by intravenous route, with differences being observed among avian species and depending on doses (Gross and Smith, 1971; Miyazaki and Sakaguchi, 1978). In wildlife and in farm environments, only BoNT/C, D, C/D, D/C, and E have been involved in avian botulism outbreaks, and BoNT/A to a much lesser extent (Rogers et al., 2021). The most common BoNT type at the moment, at least in Europe, is C/D (Woudstra et al., 2012; Souillard et al., 2014; Le Maréchal et al., 2016a).
Whether avian botulism is due to the ingestion of preformed BoNTs or to in situ BoNT production by C. botulinum is not completely clear and it has been suggested that both sources of BoNT may likely coexist during an outbreak (Skarin et al., 2015). In broilers, it is assumed that BoNT is produced in situ in the caeca, as a large amount of toxins is necessary to get clinical signs in this avian species. The amount of BoNT normally present in the immediate environment of a flock is thought not to be high enough to cause botulism (Dohms et al., 1982; Popoff, 1989). Experimental studies have confirmed the importance of the caeca in the expression of the disease (Miyazaki and Sakaguchi, 1978; Kurazono et al., 1987; Hyun and Sakaguchi, 1989). Spores are ingested by the birds, then germinate, multiply and produce BoNT in the caeca. Spores, vegetative cells as well as BoNTs are then excreted in the litter and re-ingested by birds via coprophagy. BoNT enters blood circulation and reaches nerve endings, which leads to paralysis (Miyazaki and Sakaguchi, 1978; Kurazono et al., 1987; Hyun and Sakaguchi, 1989). Several studies have shown that C. botulinum can be detected in many organs (liver, spleen, crop) in affected birds, but the potential role of these organs colonization in the observed clinical signs or in the pathogenesis is unknown (Dohms et al., 1982; Franciosa et al., 1996; Anniballi et al., 2012, 2013a; Woudstra et al., 2012). Risk factors behind the initiation of a botulism outbreak are not clearly understood, in particular the role of asymptomatic carriage of C. botulinum by birds. One hypothesis is the ingestion of BoNT from bird carcasses. This hypothesis implies the presence of C. botulinum in the digestive tract of birds before their death. Although demonstrated, the prevalence of this carriage is highly variable. A study including 100 broiler flocks failed to detect C. botulinum type C/D in caeca (Hardy and Kaldhusdal, 2013), confirming another previous study where litters from 100 poultry farms tested negative for C. botulinum (Roberts and Aitken, 1974). In contrast, C. botulinum type C/D was detected in 11% of healthy poultry flocks in Sweden (Blomqvist et al., 2010) and in one farm out of 23 examined in France (Souillard et al., 2013). The detection of C. botulinum type D/C has been reported several times, in particular thanks to connections with a bovine botulism outbreak (Le Maréchal et al., 2020; Souillard et al., 2021). Variability in prevalence of avian botulism may be explained by the existence of C. botulinum carriage at a very low level, likely underestimated considering the low sensitivity in tested samples of currently available methods (Popoff, 1989; Le Bouquin et al., 2017).
Clinical signs in avian species are flaccid paralysis progressing from the legs to the nictitating membranes and often associated with respiratory failure. The first sign in broilers is leg followed by wing paralysis (Bano, 2019). One of the typical clinical signs is the paralysis of the bird’s neck, which has resulted in the use of “limber neck” to describe botulism. High mortality rates are reported. In France, the mean mortality rate was 13.9% in 17 investigated poultry botulism outbreaks, ranging from 2.8 to 35.2% in one study (Souillard et al., 2014) and between 1 and 25% in another study (Dohms et al., 1982). Higher mortality rates have been reported in the literature in case reports: 30 and 50% in turkey farms (Smart et al., 1983; Popp et al., 2012) and 84% in a pheasant farm (Borland, 1976). On a worldwide basis, avian botulism is the most significant disease of waterbirds (Rocke, 2006). Outbreaks with more than 100,000 dead birds have been reported in the USA (Rocke and Bollinger, 2007). Differential diagnosis should include ionophore intoxication (in particular in turkeys), lead as well as selenium intoxication, Marek disease and avian Flu. Diagnosis is based on clinical signs, the epidemiological context and laboratory results (detection of BoNT or/and BoNT-producing clostridia in samples collected on animals, feed or close environment).
Beta-lactams are successfully used in poultry farms during botulism outbreaks (Anniballi et al., 2013b). Several practices can be implemented to mitigate the lethal incidence of an outbreak: physical separation of symptomatic from non-symptomatic birds, replacing litter at higher frequency and fresh litter addition to prevent ingestion of BoNT and BoNT-producing clostridia, and regular disposal of birds found dead in barns. Vaccination can be considered, but does not seem to be commonly use in farms. Regarding wild birds, sick individuals can be cared for by feeding and watering them until disappearance of clinical signs.
Implementation of biosecurity measures in poultry farms is crucial to prevent both the initiation and recurrence of outbreaks: rodent control, proper feed storage conditions and feed distribution, and regular dead bird disposal. Due to the high resistance of spores in the environment, special attention should be paid to cleaning and disinfection operations (with sporicides, in both barn and equipment) after an outbreak of botulism to avoid recurrence. In wild birds, removal and proper disposal of dead birds is the most effective measure to prevent or mitigate outbreaks.
4.4. Control by food processing operations and during food storage
Contamination of food of animal origin, at least of raw materials, with C. botulinum type III is documented (Dodds, 1993a,b; Le Maréchal et al., 2016b). This contamination justifies that control measures should be implemented for safe food production. The principles of control of microbial hazards in food are determined by the data and parameters describing their sensitivity to physical or chemical inactivation treatments, their ability to multiply and, where appropriate, produce toxins in defined conditions. Compared to C. botulinum groups I and II, which have long been recognized as the main causes of foodborne botulism, there is very little information defining the principles of control of C. botulinum type III strains. For thermal treatments under moist heat conditions commonly applied in food processing, the decimal D reduction times at 104°C of spores of group III strains (n = 4) have been estimated to be 0.1–0.9 min (Segner and Schmidt, 1971) and about 12 min (Portinha et al., 2022) at 90°C (n = 2). These sparse data suggest a resistance to moist heat much higher than that of spores of non-proteolytic C. botulinum group II, but also a much lower resistance than that of spores of proteolytic C. botulinum group I. The sensitivity to temperature changes expressed by the z-value (temperature increase in °C resulting by 10-fold reduction in D-values) was estimated to be 5.0–6.2°C, i.e., close to z = 6.9°C of group II strains and lower than z = 11.3°C of group I strains established in meta-analyses (Diao et al., 2014; Wachnicka et al., 2016). This practically means that pasteurization treatments will be ineffective against C. botulinum type C spores as they are for other spore-forming bacteria. It also means that heat treatments to control C. botulinum group I (i.e., “botulinum cook” for 3 min at 121°C, or any treatment with similar lethality) will also control C. botulinum group III. BoNTs of types C and D seem to be more heat resistant than those of types A, B, E. However, heat treatments higher than 90°C/2 min allow total inactivation of these toxins (Roberts and Gibson, 1979).
For radiation treatments, a D10 value of 2.1 KGy was established for type C spores, not dramatically different from D10 = 3.3 kGy for group I spores and D10 = 1.4 kGy for group II spores (Desmonts et al., 2001). Unfortunately, no data are available regarding the resistance of group III spores to common food industry disinfectants (chlorine, peracetic acid, hydrogen peroxide, ozone, etc.) or to other physical treatments (dry heat, UV-C, pulsed light, high hydrostatic pressure combined with temperature) to determine whether it would be lower, similar or higher than the resistance of group I and II C. botulinum strains.
Little work has also been done on the growth of C. botulinum group III in foodstuffs. Growth limits (temperature, NaCl, pH) for C. botulinum have been established for some strains. No growth at 10°C, growth of some strains at 12.8°C and above, and growth of all strains at 15.6°C have been reported (Segner et al., 1971). No growth was observed at salt concentrations above 3% NaCl, at pH 4.9 and below. In haddock, growth was found to be as rapid as that obtained in a laboratory culture medium in optimal growth conditions. In the absence of other specific data on growth or toxin production in foodstuffs, the nature of the media from which outbreaks of type C botulism originated suggests good adaptation of C. botulinum group III in many matrices containing food of animal origin. The temperature, pH or water activity that control the growth of group I strains (type A and B proteolytic) will most likely also control group III strains (Roberts and Gibson, 1979). In this context, growth tests or food challenges with surrogate microorganisms may be considered. Such surrogate strains of C. botulinum group I and group II have been proposed (Boix et al., 2022; Koukou et al., 2022; Poortmans et al., 2022). Data and models established with C. sporogenes as a surrogate of C. botulinum group I can help to define the control measures to prevent growth (Boix et al., 2022; Koukou et al., 2022). Unfortunately, no surrogate microorganism for C. botulinum group III has been defined yet.
Unfortunately, again, no data are available to suggest that the sensitivity of C. botulinum group III to other environmental factors present during food storage (redox, CO2-enriched modified atmosphere, etc.) or to preservatives is less than, similar to, or greater than the susceptibility of C. botulinum group I and II strains. In particular, the effect of nitrate and nitrite on C. botulinum group III, which are preservatives commonly applied for the control of C. botulinum groups I and II in meat processing and curing of meat or fish (Sofos et al., 1979; Skovgaard, 1992), is not documented to our knowledge, likely because of a very low association to human botulism, and thus an estimated unnecessity of specific control.
5. Conclusion
Botulism is still a major concern for animal and human health. The toxinotypes C, D, C/D, and D/C are far less known than A and B, especially regarding their potential impact on human health. The analysis of human botulism surveillance data worldwide over the period 1976–2018 confirms that the overwhelming majority of botulism forms are foodborne and infant botulism. The types of BoNTs involved are types A and B, then E, occasionally F. Over this period, no human cases of type C, C/D, D or D/C have been identified.
To address the issue of a potential zoonotic aspect of types C and D, we reviewed the existing literature on the rare cases reported worldwide since the 1950s (Meyer, 1953; Prévot et al., 1953; Demarchi et al., 1958; Fleming, 1960; Rey et al., 1964; Matveev et al., 1966; Maupas et al., 1976; Oguma et al., 1990; Martrenchar et al., 2019). The original articles were found and their reading seem to indicate a causal relationship between exposure to BoNT and/or C. botulinum type C and the occurrence of human botulism cases (two confirmed outbreaks). However, the sources of contamination have not been formally confirmed and there is still uncertainty about the zoonotic origin of these cases.
For type D, only one outbreak of foodborne botulism has been identified worldwide during the study period, for which exposure to type D BoNT was only suspected (Demarchi et al., 1958). The low sensitivity of humans to C, D and mosaic toxins is the preferred hypothesis to explain the almost total absence of case related to types C, D, C/D, and D/C. However, despite this low sensitivity of humans, spreading as fertilizer of manure or of agricultural waste known to be contaminated by C. botulinum and/or BoNT on crops of vegetables eaten raw should be avoided. Additionally, a thorough decontamination using biocides and more particularly sporicides (chlorine and hydrogen peroxide products being the most effective) must be implemented in farms affected by an outbreak of animal botulism. Land spreading of contaminated manure is not acceptable without a proper treatment of effluents (Anses, 2022). However, if the option of spreading the effluents from a botulism outbreak is chosen, the spreading equipment must limit emission of dust and aerosol. Effluent spreading must be performed under calm weather conditions and respect sufficient distances (at least 400 meters for effluents of poultry origin) to pastures and routes frequented by animals, to open cattle stabling, and dwellings and areas of human activity. Spreading on field crops is recommended and spreading on grasslands must be excluded. The vaccination of cattle in mixed farms and in any situation of potential exposure to animal botulism can be encouraged as a preventive measure. In addition to these recommendations, there is the need to wear a dust mask for operators carrying out the spreading.
As mentioned in this review, botulism occurs quite frequently in wild or farm birds and in bovine and involves types C, D, C/D, and D/C. It has also been reported with types C and D in other animal species such as horse (Swink and Gilsenan, 2022) and mink (Phaneuf et al., 1972; Wilson et al., 2015). However, these types of botulism are extremely rare in humans and implementation and maintenance of biosecurity measures could as well potentially contribute to keep these types of botulism extremely rare.
Author contributions
FM coordinated and supervised the review work and contributed to writing and editing. FC, MFe, M-EF, MFo, PF, J-PG, LGr, LGu, DH, PK, SLB-L, CLM, CM, HM, KP, J-PV, and CW were all involved in the writing and editing process to a similar extent. All authors contributed to the article and approved the submitted version.
Acknowledgments
We are grateful to the ANSES expert committee panel in charge of assessing animal health and animal welfare risk, and to the working group “Botulism,” including the authors as well as Catherine Belloc, Stéphane Bertagnoli, Alain Boissy, Henri-Jean Boulouis, Eric Collin, Jean-Claude Desfontis, David Fretin, Emmanuelle Gilot-Fromont, Etienne Giraud, Nadia Haddad, Viviane Hénaux, Elsa Jourdain, Sophie Le Poder-Alcon, Monique L’Hostis, Gilles Meyer, Elodie Monchatre-Leroy, Pierre Mormède, Carine Paraud, Ariane Payne, Carole Peroz-Sapede, Claire Ponsart, Claude Saegerman, Gaëlle Simon, Michèle Tremblay, Nathalie Arnich, Isabelle Attig, Carole Catastini, Charlotte Dunoyer, Nabila Haddache, and Elissa Khamisse. We acknowledge Florent Roussillon from Press Citron company (presscitron.fr) for the Figures 2, 3 illustrations.
Conflict of interest
The authors declare that the research was conducted in the absence of any commercial or financial relationships that could be construed as a potential conflict of interest.
Publisher’s note
All claims expressed in this article are solely those of the authors and do not necessarily represent those of their affiliated organizations, or those of the publisher, the editors and the reviewers. Any product that may be evaluated in this article, or claim that may be made by its manufacturer, is not guaranteed or endorsed by the publisher.
References
AFSSA (2002). Rapport sur le botulisme d’origine aviaire et bovine. Report from the AFSSA expert committee. Available at: http://www.avicampus.fr/PDF/botulismeAFSSA.pdf
Anniballi, F., Auricchio, B., Delibato, E., Antonacci, M., De Medici, D., and Fenicia, L. (2012). Multiplex real-time PCR SYBR green for detection and typing of group III Clostridium botulinum. Vet. Microbiol. 154, 332–338. doi: 10.1016/j.vetmic.2011.07.018
Anniballi, F., Auricchio, B., Woudstra, C., Fach, P., Fiore, A., Skarin, H., et al. (2013a). Multiplex real-time PCR for detecting and typing Clostridium botulinum group III organisms and their mosaic variants. Biosecur. Bioterror. 11, S207–S214. doi: 10.1089/bsp.2012.0084
Anniballi, F., Fiore, A., Löfström, C., Skarin, H., Auricchio, B., Woudstra, C., et al. (2013b). Management of animal botulism outbreaks: from clinical suspicion to practical countermeasures to prevent or minimize outbreaks. Biosecur. Bioterror. 11, S191–S199. doi: 10.1089/bsp.2012.0089
Anses (2021). Evaluation des mesures de maîtrise en filière bovine lors de la détection de cas de botulisme. France: Anses.
Anses (2022). Décontamination et gestion des sous-produits animaux lors des cas de botulisme bovin et aviaire. France: Anses.
Anza, I., Vidal, D., Feliu, J., Crespo, E., and Mateo, R. (2016). Differences in the vulnerability of waterbird species to botulism outbreaks in Mediterranean wetlands: an assessment of ecological and physiological factors. Appl. Environ. Microbiol. 82, 3092–3099. doi: 10.1128/AEM.00119-16
Anza, I., Vidal, D., Laguna, C., Díaz-Sánchez, S., Sánchez, S., Chicote, A., et al. (2014). Eutrophication and bacterial pathogens as risk factors for avian botulism outbreaks in wetlands receiving effluents from urban wastewater treatment plants. Appl. Environ. Microbiol. 80, 4251–4259. doi: 10.1128/AEM.00949-14
Arnon, S. S., Schechter, R., Inglesby, T. V., Henderson, D. A., Bartlett, J. G., Ascher, M. S., et al. (2001). Botulinum toxin as a biological weapon: medical and public health management. JAMA 285, 1059–1070. doi: 10.1001/jama.285.8.1059
Austin, J. W., and Leclair, D. (2011). Botulism in the north: a disease without borders. Clin. Infect. Dis. 52, 593–594. doi: 10.1093/cid/ciq256
Bano, L., Drigo, I., Tonon, E., Agnoletti, F., Giovanardi, D., and Morandini, E. (2013). Rice hulls as a possible source of Clostridium botulinum type C spores for poultry. Vet. Rec. 173, 427–428. doi: 10.1136/vr.f6521
Bano, L., Drigo, I., Tonon, E., Berto, G., Tavella, A., Woudstra, C., et al. (2015). Evidence for a natural humoral response in dairy cattle affected by persistent botulism sustained by non-chimeric type C strains. Anaerobe 36, 25–29. doi: 10.1016/j.anaerobe.2015.09.007
Barash, J. R., and Arnon, S. S. (2014). A novel strain of Clostridium botulinum that produces type B and type H botulinum toxins. J. Infect. Dis. 209, 183–191. doi: 10.1093/infdis/jit449
Barr, J. R., Moura, H., Boyer, A. E., Woolfitt, A. R., Kalb, S. R., Pavlopoulos, A., et al. (2005). Botulinum neurotoxin detection and differentiation by mass spectrometry. Emerg. Infect. Dis. 11, 1578–1583. doi: 10.3201/eid1110.041279
Barras, S. C., and Kadlec, J. A. (2000). Abiotic predictors of avian botulism outbreaks in Utah. Wildlife Soc. B. 1973-2006, 724–729.
Bentivoglio, A. R., Del Grande, A., Petracca, M., Ialongo, T., and Ricciardi, L. (2015). Clinical differences between botulinum neurotoxin type A and B. Toxicon 107, 77–84. doi: 10.1016/j.toxicon.2015.08.001
Binz, T., and Rummel, A. (2009). Cell entry strategy of clostridial neurotoxins. J. Neurochem. 109, 1584–1595. doi: 10.1111/j.1471-4159.2009.06093.x
Björnstad, K., Tevell Åberg, A., Kalb, S. R., Wang, D., Barr, J. R., Bondesson, U., et al. (2014). Validation of the Endopep-MS method for qualitative detection of active botulinum neurotoxins in human and chicken serum. Anal. Bioanal. Chem. 406, 7149–7161. doi: 10.1007/s00216-014-8170-4
Blomqvist, G., Skarin, H., Lindberg, A., and Båverud, V. (2010). “Surveillance for Clostridium botulinum type C or C/D in Swedish broilers,” in 16th World Veterinary Poultry Association congress (Marrakech, Morocco).
Boix, E., Coroller, L., Couvert, O., Planchon, S., van Vliet, A. H. M., Brunt, J., et al. (2022). Synergistic interaction between pH and NaCl in the limits of germination and outgrowth of Clostridium sporogenes and group I Clostridium botulinum vegetative cells and spores after heat treatment. Food Microbiol. 106:104055. doi: 10.1016/j.fm.2022.104055
Borland, E. D. (1976). Outbreak of botulism in reared East Anglian pheasants. Vet. Rec. 99, 220–221. doi: 10.1136/vr.99.11.220
Bott, T. L., Deffner, J. S., McCoy, E., and Foster, E. M. (1966). Clostridium botulinum type E in fish from the Great Lakes. J. Bacteriol. 91, 919–924. doi: 10.1128/jb.91.3.919-924.1966
Bott, T. L., Johnson, J., Foster, E. M., and Sugiyama, H. (1968). Possible origin of the high incidence of Clostridium botulinum type E in an inland bay (Green Bay of Lake Michigan). J. Bacteriol. 95, 1542–1547. doi: 10.1128/jb.95.5.1542-1547.1968
Cann, D. C., and Taylor, L. Y. (1982). An outbreak of botulism in rainbow trout, Salmo gairdneri Richardson, farmed in Britain. J. Fish Dis. 5, 393–399. doi: 10.1111/j.1365-2761.1982.tb00495.x
Cato, E. P., George, W. L., and Finegold, S. M. (1986). “Genus Clostridium,” in Bergey’s Manual of Systematic Bacteriology. eds. P. H. A. Sneath, N. S. Mair, M. E. Sharpe, and J. G. Holt (Baltimore: Williams and Wilkins), 1141–1200.
Chatla, K., Gaunt, P. S., Petrie-Hanson, L., Ford, L., and Hanson, L. A. (2016). Zebrafish sensitivity to botulinum neurotoxins. Toxins 8:E132. doi: 10.3390/toxins8050132
Chatla, K., Gaunt, P., Petrie-Hanson, L., Hohn, C., Ford, L., and Hanson, L. (2014). Zebrafish (Danio rerio) bioassay for visceral toxicosis of catfish and botulinum neurotoxin serotype E. J. Vet. Diagn. Investig. 26, 240–245. doi: 10.1177/1040638713519642
Chea, F. P., Chen, Y., Montville, T. J., and Schaffner, D. W. (2000). Modeling the germination kinetics of Clostridium botulinum 56A spores as affected by temperature, pH, and sodium chloride. J. Food Prot. 63, 1071–1079. doi: 10.4315/0362-028x-63.8.1071
Chellappan, G., Kumar, R., Cai, S., and Singh, B. R. (2014). Role of neurotoxin associated proteins in the low pH induced structural changes in the botulinum neurotoxin complex. Protein J. 33, 557–564. doi: 10.1007/s10930-014-9588-4
Curtiaud, A., Merdji, H., Mazuet, C., Boyer, P., and Demiselle, J. (2022). A young couple with rapidly progressive muscle and respiratory paralysis. Am. J. Med. 135, e425–e426. doi: 10.1016/j.amjmed.2022.08.018
DasGupta, B. R. (2006). Botulinum neurotoxins: perspective on their existence and as polyproteins harboring viral proteases. J. Gen. Appl. Microbiol. 52, 1–8. doi: 10.2323/jgam.52.1
Demarchi, J., Mourgues, C., Orio, J., and Prevot, A. R. (1958). Existence of type D botulism in man. Bull. Acad. Natl Med. 142, 580–582.
Desmonts, M. H., Strasser, A., Hasselmann, C., Marchioni, E., and Hausser, F. (2001). “Les traitements ionisants des aliments,” in Traitement ionisants et hautes pressions des aliments. eds. M. Federighi and J. L. Tholozan (Paris: Economica), 1–20.
Diao, M. M., André, S., and Membré, J.-M. (2014). Meta-analysis of D-values of proteolytic Clostridium botulinum and its surrogate strain Clostridium sporogenes PA 3679. Int. J. Food Microbiol. 174, 23–30. doi: 10.1016/j.ijfoodmicro.2013.12.029
Divers, T. J., Bartholomew, R. C., Messick, J. B., Whitlock, R. H., and Sweeney, R. W. (1986). Clostridium botulinum type B toxicosis in a herd of cattle and a group of mules. J. Am. Vet. Med. Assoc. 188, 382–386.
Dodds, K. L. (1993a). “Clostridium botulinum in foods,” in Clostridium botulinum Ecology and Control in Foods. eds. A. H. W. Hauschild and K. L. Dodds (New York, USA: Marcel Dekker), 53–68.
Dodds, K. L. (1993b). “Clostridium botulinum in the environment,” in Clostridium botulinum Ecology and Control in Foods. eds. A. H. W. Hauschild and K. L. Dodds (New York, USA: Dekker), 21–51.
Dohms, J. E. (2008). “Botulism,” in Diseases of Poultry. ed. Y. Saif (Ames, IA: Blackwell Pub. Professional).
Dohms, J. E., Allen, P. H., and Rosenberger, J. K. (1982). Cases of type C botulism in broiler chickens. Avian Dis. 26, 204–210. doi: 10.2307/1590044
Dolman, C. E. (1957). Type E (fish-borne) botulism: a review. Jpn. J. Med. Sci. Biol. 10, 383–395. doi: 10.7883/yoken1952.10.383
Dolman, C. E., and Murakami, L. (1961). Clostridium botulinum type F with recent observations on other types. J. Infect. Dis. 109, 107–128. doi: 10.1093/infdis/109.2.107
Dorchies, B., and Leze, V. (2017). Botulisme. Lettre d’information de l’organisation vétérinaire à vocation technique. Available at: https://www.gtv-bretagne.org/upload/document/lettre-d-information-ovvt-n-2-336.pdf (Accessed August 8, 2022).
Douillard, F. P., Derman, Y., Woudstra, C., Selby, K., Mäklin, T., Dorner, M. B., et al. (2022). Genomic and phenotypic characterization of Clostridium botulinum isolates from an infant botulism case suggests adaptation signatures to the gut. MBio 13:e0238421. doi: 10.1128/mbio.02384-21
Doutre, M. P. (1969). Fréquence au Sénégal du botulisme animal d’origine hydrique. Rev. Elev. Med. Vet. Pay. 22, 29–31. doi: 10.19182/remvt.7659
Doxey, A. C., Mansfield, M. J., and Montecucco, C. (2018). Discovery of novel bacterial toxins by genomics and computational biology. Toxicon 147, 2–12. doi: 10.1016/j.toxicon.2018.02.002
Drigo, I., Tonon, E., Pascoletti, S., Anniballi, F., Kalb, S. R., and Bano, L. (2020). Detection of active BoNT/C and D by EndoPep-MS using MALDI Biotyper instrument and comparison with the mouse test bioassay. Toxins 13:10. doi: 10.3390/toxins13010010
Eales, C. E., and Turner, A. W. (1952). Description of Clostridium botulinum type D recovered from soil in South Australia. Aust. J. Exp. Biol. Med. Sci. 30, 295–300. doi: 10.1038/icb.1952.27
Eklund, M. W., Poysky, F. T., Peterson, M. E., Peck, L. W., and Brunson, W. D. (1984). Type E botulism in salmonids and conditions contributing to outbreaks. Aquaculture 41, 293–309. doi: 10.1016/0044-8486(84)90198-4
Eleopra, R., Montecucco, C., Devigili, G., Lettieri, C., Rinaldo, S., Verriello, L., et al. (2013). Botulinum neurotoxin serotype D is poorly effective in humans: an in vivo electrophysiological study. Clin. Neurophysiol. 124, 999–1004. doi: 10.1016/j.clinph.2012.11.004
Eleopra, R., Tugnoli, V., Rossetto, O., Montecucco, C., and De Grandis, D. (1997). Botulinum neurotoxin serotype C: a novel effective botulinum toxin therapy in human. Neurosci. Lett. 224, 91–94. doi: 10.1016/S0304-3940(97)13448-6
EPA (2013). Type E botulism outbreaks: a manual for beach managers and the public | U.S. Geological Survey. Available at: https://www.usgs.gov/publications/type-e-botulism-outbreaks-manual-beach-managers-and-public (Accessed August 10, 2022).
Espelund, M., and Klaveness, D. (2014). Botulism outbreaks in natural environments – an update. Front. Microbiol. 5:287. doi: 10.3389/fmicb.2014.00287
Fenicia, L., Anniballi, F., and Aureli, P. (2007). Intestinal toxemia botulism in Italy, 1984–2005. Eur. J. Clin. Microbiol. Infect. Dis. 26, 385–394. doi: 10.1007/s10096-007-0301-9
Fillo, S., Giordani, F., Tonon, E., Drigo, I., Anselmo, A., Fortunato, A., et al. (2021). Extensive genome exploration of Clostridium botulinum group III field strains. Microorganisms 9:2347. doi: 10.3390/microorganisms9112347
Fleming, R. H. (1960). Report on four cases of botulism in Gatooma, S. Rhodesia. Cent. Afr. J. Med. 6, 91–94.
Franciosa, G., Fenicia, L., Caldiani, C., and Aureli, P. (1996). PCR for detection of Clostridium botulinum type C in avian and environmental samples. J. Clin. Microbiol. 34, 882–885. doi: 10.1128/jcm.34.4.882-885.1996
Franz, D. R., Pitt, L. M., Clayton, M. A., Hanes, M. A., and Rose, K. J. (1993). “Efficacy of prophylactic and therapeutic Administration of Antitoxin for inhalation botulism,” in Botulinum and Tetanus Neurotoxins: Neurotransmission and Biomedical Aspects. ed. B. R. DasGupta (Boston, MA: Springer US), 473–476.
Freund, B., Hayes, L., Rivera-Lara, L., Sumner, C., Chaudhry, V., Chatham-Stephens, K., et al. (2017). Adult intestinal colonization botulism mimicking brain death. Muscle Nerve 56, E27–E28. doi: 10.1002/mus.25689
Frye, E. A., Egan, C., Perry, M. J., Crouch, E. E., Burbank, K. E., and Kelly, K. M. (2020). Outbreak of botulism type a in dairy cows detected by MALDI-TOF mass spectrometry. J. Vet. Diagn. Investig. 32, 722–726. doi: 10.1177/1040638720943127
Fu, S.-W., and Wang, C.-H. (2008). An overview of type E botulism in China. Biomed. Environ. Sci. 21, 353–356. doi: 10.1016/S0895-3988(08)60054-9
Galey, F. D., Terra, R., Walker, R., Adaska, J., Etchebarne, M. A., Puschner, B., et al. (2000). Type C botulism in dairy cattle from feed contaminated with a dead cat. J. Vet. Diagn. Investig. 12, 204–209. doi: 10.1177/104063870001200302
Gao, Q. Y., Huang, Y. F., Wu, J. G., Liu, H. D., and Xia, H. Q. (1990). A review of botulism in China. Biomed. Environ. Sci. 3, 326–336.
Gardner, A. P., and Barbieri, J. T. (2018). Light chain diversity among the botulinum neurotoxins. Toxins 10:E268. doi: 10.3390/toxins10070268
Gessler, F., and Böhnel, H. (2006). Persistence and mobility of a Clostridium botulinum spore population introduced to soil with spiked compost. FEMS Microbiol. Ecol. 58, 384–393. doi: 10.1111/j.1574-6941.2006.00183.x
Gross, W. B., and Smith, L. D. (1971). Experimental botulism in gallinaceous birds. Avian Dis. 15, 716–722. doi: 10.2307/1588859
Guizelini, C. C., Lemos, R. A. A., de Paula, J. L. P., Pupin, R. C., Gomes, D. C., Barros, C. S. L., et al. (2019). Type C botulism outbreak in feedlot cattle fed contaminated corn silage. Anaerobe 55, 103–106. doi: 10.1016/j.anaerobe.2018.11.003
Haagsma, J. (1991). Pathogenic anaerobic bacteria and the environment. Rev. Sci. Tech. 10, 749–764. doi: 10.20506/rst.10.3.569
Hansbauer, E.-M., Skiba, M., Endermann, T., Weisemann, J., Stern, D., Dorner, M. B., et al. (2016). Detection, differentiation, and identification of botulinum neurotoxin serotypes C, CD, D, and DC by highly specific immunoassays and mass spectrometry. Analyst 141, 5281–5297. doi: 10.1039/c6an00693k
Hardy, S. P., and Kaldhusdal, M. (2013). Type C and C/D toxigenic Clostridium botulinum is not normally present in the intestine of healthy broilers. Vet. Microbiol. 165, 466–468. doi: 10.1016/j.vetmic.2013.03.022
Harris, R. A., Anniballi, F., and Austin, J. W. (2020). Adult intestinal toxemia botulism. Toxins 12:81. doi: 10.3390/toxins12020081
Herrero, B. A., Ecklung, A. E., Streett, C. S., Ford, D. F., and King, J. K. (1967). Experimental botulism in monkeys–a clinical pathological study. Exp. Mol. Pathol. 6, 84–95. doi: 10.1016/0014-4800(67)90007-x
Hodowanec, A., and Bleck, T. (2015). “Clostridium botulinum (Botulism),” in Mandell, Douglas, and Bennett’s Principles and Practice of Infectious Diseases Philadelphia (USA: Elsevier), 2763–2767.
Hogg, R., Livesey, C., and Payne, J. (2008). Diagnosis and implications of botulism. In Pract. 30, 392–397. doi: 10.1136/inpract.30.7.392
Horowitz, B. Z. (2010). Type E botulism. Clin. Toxicol. 48, 880–895. doi: 10.3109/15563650.2010.526943
Hubálek, Z., and Halouzka, J. (1991). Persistence of Clostridium botulinum type C toxin in blow fly (Calliphoridae) larvae as a possible cause of avian botulism in spring. J. Wildl. Dis. 27, 81–85. doi: 10.7589/0090-3558-27.1.81
Hyun, S. H., and Sakaguchi, G. (1989). Implication of coprophagy in pathogenesis of chicken botulism. Nihon Juigaku Zasshi 51, 582–586. doi: 10.1292/jvms1939.51.582
Inger, R., Cox, D. T. C., Per, E., Norton, B. A., and Gaston, K. J. (2016). Ecological role of vertebrate scavengers in urban ecosystems in the UK. Ecol. Evol. 6, 7015–7023. doi: 10.1002/ece3.2414
ISO/TC 34/SC 9 Microbiology (2013). ISO/TS 17919:2013 microbiology of the food chain — polymerase chain reaction (PCR) for the detection of food-borne pathogens — detection of botulinum type a, B, E and F neurotoxin-producing clostridia. ISO. Available at: https://www.iso.org/standard/61010.html (Accessed December 2, 2022).
Johnson, A. L., McAdams-Gallagher, S. C., and Aceto, H. (2015). Outcome of adult horses with botulism treated at a veterinary hospital: 92 cases (1989-2013). J. Vet. Intern. Med. 29, 311–319. doi: 10.1111/jvim.12502
Karasawa, T., Wang, X., Maegawa, T., Nakamura, S., Hangombe, B. M., and Isogai, E. (2000). Demonstration of botulinum toxins of types B and D in soil samples from Zambia. Ann. Trop. Med. Parasitol. 94, 409–411. doi: 10.1080/00034983.2000.11813558
Kelch, W. J., Kerr, L. A., Pringle, J. K., Rohrbach, B. W., and Whitlock, R. H. (2000). Fatal Clostridium botulinum toxicosis in eleven Holstein cattle fed round bale barley haylage. J. Vet. Diagn. Investig. 12, 453–455. doi: 10.1177/104063870001200511
Koepke, R., Sobel, J., and Arnon, S. S. (2008). Global occurrence of infant botulism, 1976–2006. Pediatrics 122, e73–e82. doi: 10.1542/peds.2007-1827
Koukou, I., Stergioti, T., la Cour, R., Gkogka, E., and Dalgaard, P. (2022). Clostridium sporogenes as surrogate for proteolytic C. botulinum – Development and validation of extensive growth and growth-boundary model. Food Microbiol. 107:104060. doi: 10.1016/j.fm.2022.104060
Kumar, R., Dhaliwal, H. P., Kukreja, R. V., and Singh, B. R. (2016). The botulinum toxin as a therapeutic agent: molecular structure and mechanism of action in motor and sensory systems. Semin. Neurol. 36, 010–019. doi: 10.1055/s-0035-1571215
Kummel, J., Krametter-Froetscher, R., Six, G., Brunthaler, R., Baumgartner, W., and Altenbrunner-Martinek, B. (2012). Descriptive study of botulism in an Austrian dairy herd: a case report. Vet. Med. 57, 143–149.
Kurazono, H., Hosokawa, M., Matsuda, H., and Sakaguchi, G. (1987). Fluid accumulation in the ligated intestinal loop and histopathological changes of the intestinal mucosa caused by Clostridium botulinum C2 toxin in the pheasant and chicken. Res. Vet. Sci. 42, 349–353. doi: 10.1016/S0034-5288(18)30717-3
Lafrancois, B. M., Riley, S. C., Blehert, D. S., and Ballmann, A. E. (2011). Links between type E botulism outbreaks, lake levels, and surface water temperatures in Lake Michigan, 1963–2008. J. Great Lakes Res. 37, 86–91. doi: 10.1016/j.jglr.2010.10.003
Laycock, R. A., and Loring, D. H. (1972). Distribution of Clostridium botulinum type E in the Gulf of St. Lawrence in relation to the physical environment. Can. J. Microbiol. 18, 763–773. doi: 10.1139/m72-121
Le Bouquin, S., Le Maréchal, C., Ballan, V., Rouxel, S., Léon, D., Balaine, L., et al. (2017). Investigation d’un cas de botulisme aviaire dans un élevage de poules pondeuses plein-air. Bulletin épidémiologique, santé animale et alimentation, 13–17.
Le Bouquin, S., Lucas, C., Souillard, R., Le Maréchal, C., Petit, K., Kooh, P., et al. (2022). Human and animal botulism surveillance in France from 2008 to 2019. Front. Publ. Heal. 10:1003917. doi: 10.3389/fpubh.2022.1003917
Le Maréchal, C., Ballan, V., Rouxel, S., Bayon-Auboyer, M.-H., Baudouard, M.-A., Morvan, H., et al. (2016a). Livers provide a reliable matrix for real-time PCR confirmation of avian botulism. Anaerobe 38, 7–13. doi: 10.1016/j.anaerobe.2015.10.014
Le Maréchal, C., Hulin, O., Macé, S., Chuzeville, C., Rouxel, S., Poëzevara, T., et al. (2019). A case report of a botulism outbreak in beef cattle due to the contamination of wheat by a roaming cat carcass: from the suspicion to the management of the outbreak. Animals 9:E1025. doi: 10.3390/ani9121025
Le Maréchal, C., Rouxel, S., Ballan, V., Houard, E., Poezevara, T., Bayon-Auboyer, M.-H., et al. (2017). Development and validation of a new reliable method for the diagnosis of avian botulism. PLoS One 12:e0169640. doi: 10.1371/journal.pone.0169640
Le Maréchal, C., Souillard, R., Villaggi, Y., Kuntz, G., Le Bouquin, S., Scalabrino, A., et al. (2020). Botulisme bovin: importance de la biosécurité pour prévenir les contaminations croisées avec les ateliers de volailles. Bulletin épidémiologique, santé animale et alimentation, 1–4.
Le Maréchal, C., Woudtsra, C., and Fach, P. (2016b). “Botulism,” in Clostridial Diseases of Animals. eds. F. A. Uzal, G. Songer, J. F. Prescott, and M. R. Popoff (Ames, Iowa, USA: John Wiley & Sons, Inc), 303–330.
Leclair, D., Farber, J. M., Doidge, B., Blanchfield, B., Suppa, S., Pagotto, F., et al. (2013). Distribution of Clostridium botulinum type E strains in Nunavik, Northern Quebec, Canada. Appl. Environ. Microbiol. 79, 646–654. doi: 10.1128/AEM.05999-11
Leclair, D., Farber, J. M., Pagotto, F., Suppa, S., Doidge, B., and Austin, J. W. (2017). Tracking sources of Clostridium botulinum type E contamination in seal meat. Int. J. Circumpol. Heal. 76, 1–8. doi: 10.1080/22423982.2017.1380994
Lindström, M., Kiviniemi, K., and Korkeala, H. (2006). Hazard and control of group II (non-proteolytic) Clostridium botulinum in modern food processing. Int. J. Food Microbiol. 108, 92–104. doi: 10.1016/j.ijfoodmicro.2005.11.003
Lindström, M., Myllykoski, J., Sivelä, S., and Korkeala, H. (2010). Clostridium botulinum in cattle and dairy products. Crit. Rev. Food Sci. Nutr. 50, 281–304. doi: 10.1080/10408390802544405
Long, S. C., and Tauscher, T. (2006). Watershed issues associated with Clostridium botulinum: a literature review. J. Wat. Heal. 4, 277–288. doi: 10.2166/wh.2006.016b
Lund, B. M., and Peck, M. W. (2013). “Clostridium botulinum,” in Guide to Foodborne Pathogens. eds. R. G. Labbé and S. Garcia (Oxford: Wiley-Blackwell), 91–111.
MacDonald, K. L., Rutherford, G. W., Friedman, S. M., Dietz, J. R., Kaye, B. R., McKinley, G. F., et al. (1985). Botulism and botulism-like illness in chronic drug abusers. Ann. Intern. Med. 102, 616–618. doi: 10.7326/0003-4819-102-5-616
Martrenchar, A., Djossou, F., Stagnetto, C., Dupuy, C., Brulez, E., Attica, C., et al. (2019). Is botulism type C transmissible to human by consumption of contaminated poultry meat? Analysis of a suspect outbreak in French Guyana. Anaerobe 56, 49–50. doi: 10.1016/j.anaerobe.2019.02.008
Maslanka, S. E., Lúquez, C., Dykes, J. K., Tepp, W. H., Pier, C. L., Pellett, S., et al. (2016). A novel botulinum neurotoxin, previously reported as serotype H, has a hybrid-like structure with regions of similarity to the structures of serotypes a and F and is neutralized with serotype a antitoxin. J. Infect. Dis. 213, 379–385. doi: 10.1093/infdis/jiv327
Masters, A. M., and Palmer, D. G. (2021). Confirmation of botulism diagnosis in Australian bird samples by ELISA and RT rtPCR. J. Vet. Diagn. Investig. 33, 684–694. doi: 10.1177/10406387211014486
Matveev, K. I., Nefedjeva, N. P., Bulatova, T. I., and Sokolov, I. S. (1966). “Epidemiology of botulism in the U.S.R.R,” in Botulism. eds. M. Ingram and T. A. Roberts (London, UK: Chapman and Hall), 1–10.
Maupas, P., Lamagnere, J. P., Lamisse, F., and Laugier, J. (1976). Botulisme de Type C. Intérêt de la Recherche de la Toxémie. Méd. Maladies Infect. 6, 207–210. doi: 10.1016/S0399-077X(76)80079-0
Mazuet, C., Legeay, C., Sautereau, J., Bouchier, C., Criscuolo, A., Bouvet, P., et al. (2017). Characterization of Clostridium baratii type F strains responsible for an outbreak of botulism linked to beef meat consumption in France. PLoS. Currents 9, 1–16. doi: 10.1371/currents.outbreaks.6ed2fe754b58a5c42d0c33d586ffc606
Meurens, F., Boulouis, H.-J., Carlin, F., Federighi, M. M., Filippitzi, M.-E., Fournier, M., et al. (2021). Clostridium botulinum: mise à jour des connaissances sur les différentes formes des types C, D, mosaïque C/D et D/C et E. Available at: https://hal-anses.archives-ouvertes.fr/anses-03674557 (Accessed November 14, 2022).
Meyer, K. F. (1953). Food poisoning (concluded). N. Engl. J. Med. 249, 843–852. doi: 10.1056/NEJM195311192492105
Miyazaki, S., and Sakaguchi, G. (1978). Experimental botulism in chickens: the cecum as the site of production and absorption of botulinum toxin. Jpn. J. Med. Sci. Biol. 31, 1–15. doi: 10.7883/yoken1952.31.1
Montecucco, C. (1986). How do tetanus and botulinum toxins bind to neuronal membranes? Trends Biochem. Sci. 11, 314–317. doi: 10.1016/0968-0004(86)90282-3
Murrell, W. G., and Stewart, B. J. (1983). Botulism in New South Wales, 1980–1981. Med. J. Aust. 1, 13–17. doi: 10.5694/j.1326-5377.1983.tb136015.x
Myllykoski, J., Lindström, M., Keto-Timonen, R., Söderholm, H., Jakala, J., Kallio, H., et al. (2009). Type C bovine botulism outbreak due to carcass contaminated non-acidified silage. Epidemiol. Infect. 137, 284–293. doi: 10.1017/S0950268808000939
Neeki, M. M., Dong, F., Emond, C., Lee, C., Neeki, A. S., Hajjafar, K., et al. (2021). Early diagnosis and critical management of wound botulism in the emergency department: a single center experience and literature review. Int. J. Emerg. Med. 14:56. doi: 10.1186/s12245-021-00375-4
Nightingale, K. W., and Ayim, E. N. (1980). Outbreak of botulism in Kenya after ingestion of white ants. Br. Med. J. 281, 1682–1683. doi: 10.1136/bmj.281.6256.1682-a
Notermans, S., Dufrenne, J., and Oosterom, J. (1981). Persistence of Clostridium botulinum type B on a cattle farm after an outbreak of botulism. Appl. Environ. Microbiol. 41, 179–183. doi: 10.1128/aem.41.1.179-183.1981
Oguma, K., Yokota, K., Hayashi, S., Takeshi, K., Kumagai, M., Itoh, N., et al. (1990). Infant botulism due to Clostridium botulinum type C toxin. Lancet 336, 1449–1450. doi: 10.1016/0140-6736(90)93157-k
Palmer, J. S., Hough, R. L., West, H. M., and Avery, L. M. (2019). A review of the abundance, behaviour and detection of clostridial pathogens in agricultural soils. Eur. J. Soil Sci. 70, 911–929. doi: 10.1111/ejss.12847
Peck, M. W. (2009). Biology and genomic analysis of Clostridium botulinum. Adv. Microb. Physiol. 55:320. doi: 10.1016/S0065-2911(09)05503-9
Peck, M. W., Smith, T. J., Anniballi, F., Austin, J. W., Bano, L., Bradshaw, M., et al. (2017). Historical perspectives and guidelines for botulinum neurotoxin subtype nomenclature. Toxins 9:E38. doi: 10.3390/toxins9010038
Pellett, S., Bradshaw, M., Tepp, W. H., Pier, C. L., Whitemarsh, R. C. M., Chen, C., et al. (2018a). The light chain defines the duration of action of botulinum toxin serotype a subtypes. MBio 9, e00089–e00018. doi: 10.1128/mBio.00089-18
Pellett, S., Tepp, W. H., Lin, G., and Johnson, E. A. (2018b). Substrate cleavage and duration of action of botulinum neurotoxin type FA (“H, HA”). Toxicon 147, 38–46. doi: 10.1016/j.toxicon.2017.12.048
Pellett, S., Tepp, W. H., Whitemarsh, R. C. M., Bradshaw, M., and Johnson, E. A. (2015). In vivo onset and duration of action varies for botulinum neurotoxin A subtypes 1-5. Toxicon 107, 37–42. doi: 10.1016/j.toxicon.2015.06.021
Phaneuf, J. B., Fredette, V., and Vinet, G. (1972). Botulism in a mink ranch in Quebec. Can. Vet. J. 13, 75–77.
Poortmans, M., Vanoirbeek, K., Dorner, M. B., and Michiels, C. W. (2022). Selection and development of nontoxic nonproteolytic Clostridium botulinum surrogate strains for food challenge testing. Foods 11:1577. doi: 10.3390/foods11111577
Popoff, M. R. (1989). Revue sur l’épidémiologie du botulisme bovin en France et analyse de sa relation avec les élevages de volailles. Rev. Sci. Tech. 8, 129–145. doi: 10.20506/rst.8.1.404
Popoff, M. (2018). “Botulinum toxins, diversity, mode of action, epidemiology of botulism in France,” in Botulinum Toxin. ed. N. Serdev (London, UK: IntechOpen).
Popp, C., Hauck, R., Gad, W., and Hafez, H. M. (2012). Type C botulism in a commercial Turkey farm: a case report. Avian Dis. 56, 760–763. doi: 10.1637/10274-061212-Case.1
Portinha, I. M., Douillard, F. P., Korkeala, H., and Lindström, M. (2022). Sporulation strategies and potential role of the exosporium in survival and persistence of Clostridium botulinum. Int. J. Mol. Sci. 23:754. doi: 10.3390/ijms23020754
Poulain, B., and Popoff, M. R. (2019). Why are botulinum neurotoxin-producing bacteria so diverse and botulinum neurotoxins so toxic? Toxins 11:E34. doi: 10.3390/toxins11010034
Prévot, A. R., Silloc, R., and Quentin, M. (1953). Existence en France du botulisme bovin de type C. Bull. Acad. Vét. Fr. 106, 73–78. doi: 10.4267/2042/69056
Rao, A. K., Sobel, J., Chatham-Stephens, K., and Luquez, C. (2021). Clinical guidelines for diagnosis and treatment of botulism, 2021. MMWR Recomm. Rep. 70, 1–30. doi: 10.15585/mmwr.rr7002a1
Rasetti-Escargueil, C., Lemichez, E., and Popoff, M. R. (2019). Public health risk associated with botulism as foodborne zoonoses. Toxins 12:E17. doi: 10.3390/toxins12010017
Rasetti-Escargueil, C., Lemichez, E., and Popoff, M. R. (2020). Human botulism in France, 1875–2016. Toxins 12:E338. doi: 10.3390/toxins12050338
Rasetti-Escargueil, C., and Popoff, M. R. (2019). Antibodies and vaccines against botulinum toxins: available measures and novel approaches. Toxins 11:528. doi: 10.3390/toxins11090528
Reed, T. M., and Rocke, T. E. (1992). The role of avian carcasses in botulism epizootics. Wildl. Soc. Bull. 1973-2006, 175–182.
Relun, A., Dorso, L., Douart, A., Chartier, C., Guatteo, R., Mazuet, C., et al. (2017). A large outbreak of bovine botulism possibly linked to a massive contamination of grass silage by type D/C Clostridium botulinum spores on a farm with dairy and poultry operations. Epidemiol. Infect. 145, 3477–3485. doi: 10.1017/S0950268817002382
Rey, M., Diop Mar, I., Baylet, R., Armengaud, M., Michel, R., Bon-Nardot, R., et al. (1964). Du botulisme en pays Serer, à propos de six cas hospitalisés. Bull. Soc. Méd. Afr. noire 9, 34–44.
Reynolds, M. H., Johnson, K. N., Schvaneveldt, E. R., Dewey, D. L., Uyehara, K. J., and Hess, S. C. (2021). Efficacy of detection canines for avian botulism surveillance and mitigation. Conserv. Sci. Pract. 3:e397. doi: 10.1111/csp2.397
Roberts, T. A., and Aitken, I. D. (1974). “Botulism in birds and mammals in Great Britain and an assessment of the toxicity of Clostridium botulinum type C toxin in the domestic fowl,” in Spore Research 1973. eds. A. N. Barker, G. W. Gould, and J. Wolf (London: Academic Press), 1–9.
Roberts, T. A., and Gibson, A. M. (1979). The relevance of Clostridium botulinum type C in public health and food processing. Int. J. Food Sci. Tech. 14, 211–226. doi: 10.1111/j.1365-2621.1979.tb00866.x
Rocke, T. E. (2006). “The global importance of avian botulism,” in Waterbirds around the world. eds. G. C. Boere, C. A. Galbraith, and D. E. Stroud (United Kingdom: Edinburgh), 422–426.
Rocke, T. E., and Bollinger, T. K. (2007). “Avian botulism,” in Infectious diseases of wild birds. eds. N. J. Thomas, B. Hunter, and C. T. Atkinson (Oxford, UK: Blackwell Publishing Ltd), 377–416.
Rocke, T. E., Euliss, N., and Samuel, M. D. (1999). Environmental characteristics associated with occurrence of avian botulism in wetlands of a northern California refuge. J. Wildl. Manag. 63, 358–368. doi: 10.2307/3802520
Rogers, K. H., Viera, O. G., Uzal, F. A., Peronne, L., and Mete, A. (2021). Mortality of Western gulls (Larus occidentalis) associated with botulism type a in coastal Southern California, USA. J. Wildl. Dis. 57, 657–661. doi: 10.7589/JWD-D-20-00153
Rossetto, O., and Montecucco, C. (2019). Tables of toxicity of botulinum and tetanus neurotoxins. Toxins 11:E686. doi: 10.3390/toxins11120686
Sandler, R. J., Rocke, T. E., Samuel, M. D., and Yuill, T. M. (1993). Seasonal prevalence of Clostridium botulinum type C in sediments of a northern California wetland. J. Wildl. Dis. 29, 533–539. doi: 10.7589/0090-3558-29.4.533
Schantz, E. J., and Spero, L. (1967). “Molecular size of C. botulinum toxins,” in Botulism 1966 (London: Chapman and Hall), 296–301.
Schocken-Iturrino, R. P., Avila, F. A., Berchielli, S. C., and Nader Filho, A. (1990). First case of type A botulism in zebu (Bos indicus). Vet. Rec. 126, 217–218.
Segner, W. P., and Schmidt, C. F. (1971). Heat resistance of spores of marine and terrestrial strains of Clostridium botulinum type C. Appl. Microbiol. 22, 1030–1033. doi: 10.1128/am.22.6.1030-1033.1971
Segner, W. P., Schmidt, C. F., and Boltz, J. K. (1971). Minimal growth temperature, sodium chloride tolerance, pH sensitivity, and toxin production of marine and terrestrial strains of Clostridium botulinum type C. Appl. Microbiol. 22, 1025–1029. doi: 10.1128/am.22.6.1025-1029.1971
Shapiro, R. L., Hatheway, C., and Swerdlow, D. L. (1998). Botulism in the United States: a clinical and epidemiologic review. Ann. Intern. Med. 129, 221–228. doi: 10.7326/0003-4819-129-3-199808010-00011
Silva, R. O. S., Martins, R. A., Assis, R. A., Oliveira Junior, C. A., and Lobato, F. C. F. (2018). Type C botulism in domestic chickens, dogs and black-pencilled marmoset (Callithrix penicillata) in Minas Gerais, Brazil. Anaerobe 51, 47–49. doi: 10.1016/j.anaerobe.2018.03.013
Skarin, H., Lindgren, Y., and Jansson, D. S. (2015). Investigations into an outbreak of botulism caused by Clostridium botulinum type C/D in laying hens. Avian Dis. 59, 335–340. doi: 10.1637/10861-051214-Case
Skovgaard, N. (1992). Microbiological aspects and technological need: technological needs for nitrates and nitrites. Food Addit. Contam. 9, 391–397. doi: 10.1080/02652039209374089
Smart, J. L., Laing, P. W., and Winkler, C. E. (1983). Type C botulism in intensively farmed turkeys. Vet. Rec. 113, 198–200. doi: 10.1136/vr.113.9.198
Smart, J. L., Roberts, T. A., McCullagh, K. G., Lucke, V. M., and Pearson, H. (1980). An outbreak of type C botulism in captive monkeys. Vet. Rec. 107, 445–446. doi: 10.1136/vr.107.19.445
Smith, G. R., and Milligan, R. A. (1979). Clostridium botulinum in soil on the site of the former Metropolitan (Caledonian) Cattle Market, London. J. Hyg. 83, 237–241. doi: 10.1017/s0022172400026024
Smith, T., Williamson, C. H. D., Hill, K., Sahl, J., and Keim, P. (2018). Botulinum neurotoxin-producing bacteria. Isn’t it time that we called a species a species? MBio 9, e01469–e01418. doi: 10.1128/mBio.01469-18
Sofos, J. N., Busta, F. F., and Allen, C. E. (1979). Botulism control by nitrite and sorbate in cured meats: a review 1. J. Food Prot. 42, 739–770. doi: 10.4315/0362-028X-42.9.739
Solomon, H. M., and Lilly, T. (2001). BAM Chapter 17: Clostridium botulinum. FDA. Available at: https://www.fda.gov/food/laboratory-methods-food/bam-chapter-17-clostridium-botulinum (Accessed December 2, 2022).
Soos, C., and Wobeser, G. (2006). Identification of primary substrate in the initiation of avian botulism outbreaks. J. Wildl. Manag. 70, 43–53. doi: 10.2193/0022-541X(2006)70[43:IOPSIT]2.0.CO;2
Souillard, R., Grosjean, D., Le Gratiet, T., Poezevara, T., Rouxel, S., Balaine, L., et al. (2021). Asymptomatic carriage of C. botulinum type D/C in broiler flocks as the source of contamination of a massive botulism outbreak on a dairy cattle farm. Front. Microbiol. :12:679377. doi: 10.3389/fmicb.2021.679377
Souillard, R., Le Maréchal, C., Ballan, V., Rouxel, S., Léon, D., Balaine, L., et al. (2017). Investigation of a type C/D botulism outbreak in free-range laying hens in France. Avian Pathol. 46, 195–201. doi: 10.1080/03079457.2016.1240355
Souillard, R., Woudstra, C., Dia, M., Léon, D., Toux, J.-Y., Guimaraes, V., et al. (2013). “Recherche de Clostridium botulinum neurotoxinogène par PCR temps réel dans des élevages de volailles atteints de botulisme et des élevages indemnes” in Proceedings of the 10ème Journées de la Recherche Avicole et Palmipèdes à foie Gras (France: La Rochelle), 341–345.
Souillard, R., Woudstra, C., Le Maréchal, C., Dia, M., Bayon-Auboyer, M. H., Chemaly, M., et al. (2014). Investigation of Clostridium botulinum in commercial poultry farms in France between 2011 and 2013. Avian Pathol. 43, 458–464. doi: 10.1080/03079457.2014.957644
Suhadi, F., Thayib, S. S., and Sumarsono, N. (1981). Distribution of Clostridium botulinum around fishing areas of the western part of Indonesian waters. Appl. Environ. Microbiol. 41, 1468–1471. doi: 10.1128/aem.41.6.1468-1471.1981
Sundeen, G., and Barbieri, J. T. (2017). Vaccines against botulism. Toxins 9:268. doi: 10.3390/toxins9090268
Swink, J. M., and Gilsenan, W. F. (2022). Clostridial diseases (botulism and tetanus). Vet. Clin. N. Am.-Equine 38, 269–282. doi: 10.1016/j.cveq.2022.05.004
Torrens, J. K. (1998). Clostridium botulinum was named because of association with sausage poisoning. BMJ 316:151. doi: 10.1136/bmj.316.7125.151c
Umeda, K., Wada, T., Kohda, T., and Kozaki, S. (2013). Multi-locus variable number tandem repeat analysis for Clostridium botulinum type B isolates in Japan: comparison with other isolates and genotyping methods. Infect. Gen. Evol. 16, 298–304. doi: 10.1016/j.meegid.2013.02.022
Vanhomwegen, J., Berthet, N., Mazuet, C., Guigon, G., Vallaeys, T., Stamboliyska, R., et al. (2013). Application of high-density DNA resequencing microarray for detection and characterization of botulinum neurotoxin-producing clostridia. PLoS One 8:e67510. doi: 10.1371/journal.pone.0067510
Ventujol, A., Decors, A., Le Maréchal, C., Toux, J. Y., Allain, V., Mazuet, C., et al. (2017). Avian botulism in France: analysis of cases reported by two surveillance networks both in the wild and in poultry farms between 2000 and 2013. Épidémiol. Sant. Anim. 72, 85–102.
Wachnicka, E., Stringer, S., Barker, G., and Peck, M. (2016). Systematic assessment of heat resistance for non-proteolytic Clostridium botulinum spores. Appl. Environ. Microbiol. 82:6019. doi: 10.1128/AEM.01737-16
Wilson, D. J., Baldwin, T. J., Whitehouse, C. H., and Hullinger, G. (2015). Causes of mortality in farmed mink in the Intermountain West, North America. J. Vet. Diagn. Investig. 27, 470–475. doi: 10.1177/1040638715586438
Wilson, R. B., Boley, M. T., and Corwin, B. (1995). Presumptive botulism in cattle associated with plastic-packaged hay. J. Vet. Diagn. Investig. 7, 167–169. doi: 10.1177/104063879500700131
Wobeser, G. (1997). Avian botulism–another perspective. J. Wildl. Dis. 33, 181–186. doi: 10.7589/0090-3558-33.2.181
Wobeser, G., Marsden, S., and MacFarlane, R. J. (1987). Occurrence of toxigenic Clostridium botulinum type C in the soil of wetlands in Saskatchewan. J. Wildl. Dis. 23, 67–76. doi: 10.7589/0090-3558-23.1.67
Worbs, S., Fiebig, U., Zeleny, R., Schimmel, H., Rummel, A., Luginbühl, W., et al. (2015). Qualitative and quantitative detection of botulinum neurotoxins from complex matrices: results of the first international proficiency test. Toxins 7, 4935–4966. doi: 10.3390/toxins7124857
Woudstra, C., Skarin, H., Anniballi, F., Fenicia, L., Bano, L., Drigo, I., et al. (2012). Neurotoxin gene profiling of Clostridium botulinum types C and D native to different countries within Europe. Appl. Environ. Microbiol. 78, 3120–3127. doi: 10.1128/AEM.07568-11
Yamakawa, K., Kamiya, S., Nishida, S., Yoshimura, K., Yu, H., Lu, D. Y., et al. (1988). Distribution of Clostridium botulinum in Japan and in Shinkiang district of China. Microbiol. Immunol. 32, 579–587. doi: 10.1111/j.1348-0421.1988.tb01419.x
Yamakawa, K., Kamiya, S., Yoshimura, K., Nakamura, S., and Ezaki, T. (1990). Clostridium botulinum in the soil of Kenya. Ann. Trop. Med. Parasitol. 84, 201–203. doi: 10.1080/00034983.1990.11812457
Yamakawa, K., and Nakamura, S. (1992). Prevalence of Clostridium botulinum type E and coexistence of C. botulinum nonproteolytic type B in the river soil of Japan. Microbiol. Immunol. 36, 583–591. doi: 10.1111/j.1348-0421.1992.tb02058.x
Yeruham, I., Elad, D., Avidar, Y., Grinberg, K., Tiomkin, D., and Monbaz, A. (2003). Outbreak of botulism type B in a dairy cattle herd: clinical and epidemiological aspects. Vet. Rec. 153, 270–272. doi: 10.1136/vr.153.9.270
Yule, A. M., Barker, I. K., Austin, J. W., and Moccia, R. D. (2006). Toxicity of Clostridium botulinum type E neurotoxin to Great Lakes fish: implications for avian botulism. J. Wildl. Dis. 42, 479–493. doi: 10.7589/0090-3558-42.3.479
Zhang, S., Berntsson, R. P.-A., Tepp, W. H., Tao, L., Johnson, E. A., Stenmark, P., et al. (2017a). Structural basis for the unique ganglioside and cell membrane recognition mechanism of botulinum neurotoxin DC. Nat. Commun. 8:1637. doi: 10.1038/s41467-017-01534-z
Zhang, S., Masuyer, G., Zhang, J., Shen, Y., Lundin, D., Henriksson, L., et al. (2017b). Identification and characterization of a novel botulinum neurotoxin. Nat. Commun. 8:14130. doi: 10.1038/ncomms14130
Keywords: Clostridium, zoonosis, cattle, poultry, toxins, botulism
Citation: Meurens F, Carlin F, Federighi M, Filippitzi M-E, Fournier M, Fravalo P, Ganière J-P, Grisot L, Guillier L, Hilaire D, Kooh P, Le Bouquin-Leneveu S, Le Maréchal C, Mazuet C, Morvan H, Petit K, Vaillancourt J-P and Woudstra C (2023) Clostridium botulinum type C, D, C/D, and D/C: An update. Front. Microbiol. 13:1099184. doi: 10.3389/fmicb.2022.1099184
Edited by:
Fabrizio Anniballi, National Institute of Health (ISS), ItalyReviewed by:
John W. Austin, Health Canada, CanadaSaugata Majumder, Albany Medical College, United States
Copyright © 2023 Meurens, Carlin, Federighi, Filippitzi, Fournier, Fravalo, Ganière, Grisot, Guillier, Hilaire, Kooh, Le Bouquin-Leneveu, Le Maréchal, Mazuet, Morvan, Petit, Vaillancourt and Woudstra. This is an open-access article distributed under the terms of the Creative Commons Attribution License (CC BY). The use, distribution or reproduction in other forums is permitted, provided the original author(s) and the copyright owner(s) are credited and that the original publication in this journal is cited, in accordance with accepted academic practice. No use, distribution or reproduction is permitted which does not comply with these terms.
*Correspondence: François Meurens, ✉ ZnJhbmNvaXMubWV1cmVuc0BpbnJhZS5mcg==
†These authors have contributed equally to this work
‡ORCID: François Meurens https://orcid.org/0000-0002-0353-4871