- 1Key Laboratory of Wetland Ecology and Environment, Northeast Institute of Geography and Agroecology, Chinese Academy of Sciences, Changchun, China
- 2College of Resources and Environment, University of Chinese Academy of Sciences, Beijing, China
- 3State Environmental Protection Key Laboratory of Wetland Ecology and Vegetation Restoration, Institute for Peat and Mire Research, Northeast Normal University, Changchun, China
Northern peatlands are one of the largest natural sources of atmospheric methane globally. As the only biological sink of methane, different groups of methanotrophs use different carbon sources. However, the variations in microbial biomass and metabolism of different methanotrophic groups in peatlands along the altitude gradient are uncertain. We measured the concentrations and metabolic characteristics of type I (16:1ω7c and 16:1ω5c) and type II (18:1ω7c) methanotroph biomarkers using biomarkers and stable isotopes in eight Carex peatlands along an altitude gradient from 300 to 1,500 m in the Changbai Mountain, China. We found that the trends with altitude in concentrations of the type I and type II methanotroph biomarkers were different. The dominating microbial group changed from type I to type II methanotroph with increasing altitude. The concentrations of type I and type II methanotroph biomarkers were significantly affected by the total phosphorus, total nitrogen, and dissolved organic carbon, respectively. The δ13C values of type II methanotroph biomarkers changed significantly along the altitude gradient, and they were more depleted than type II methanotroph biomarkers, which indicates the difference in carbon source preference between type I and type II methanotrophs. This study highlights the difference in the concentration and carbon source utilization of type I and type II methanotrophic groups along the altitude gradient, and enhances our understanding of the metabolic process of methane mediated by methanotrophs and its impact on carbon-sink function in northern peatlands.
Introduction
It is predicted that wetlands will form the majority of methane (CH4) climate feedback by 2,100 (Dean et al., 2018). Peatlands represent large terrestrial carbon banks, which are also one of the largest natural sources of CH4 emissions into the atmosphere (Chowdhury and Dick, 2013). Even a very small change in CH4 emissions in peatlands will have a strong impact on the trajectory of future global climate change, so the metabolic process of CH4 in peatlands has attracted considerable attention (Chowdhury and Dick, 2013; Zhao et al., 2021). As the only biological sink of CH4, the methanotrophs use CH4 as the source of carbon and energy, so that the largest part of CH4 formed in peatland ecosystems is recycled and does not reach the atmosphere (Raghoebarsing et al., 2005; Kip et al., 2011). Understanding the biogeochemical processes mediated by methanotrophs in peatlands is the key in predicting future climate change.
Methanotrophs can be divided into two groups based on differences in phenotypic, chemotaxonomic classifications, and genotypic characteristics, such as the arrangement of cytoplasmic intima, dominant phospholipid fatty acids (PLFAs), and carbon assimilation pathways. The two types are: types I and II methanotrophs, which belong to the Gamma- and Alphaproteobacteria, respectively (Dedysh et al., 2007). The PLFAs are the main components of the membranes of all living cells. They also record the substrate-organic matter information (Zhao et al., 2020). The results of PLFAs combined with natural 13C content can be used to interpret the functions of microbial groups in soils (Watzinger, 2015). The carbon isotopic composition of the methanotrophs was more depleted than that of other microbial groups in peatlands. This is because the methanotrophs use CH4 with a significantly negative δ13C value as a carbon source, and significant carbon isotope fractionation will occur in the process of assimilation and absorption of CH4 (Cifuentes and Salata, 2001; Zhang et al., 2021). Due to the difference in metabolic characteristics and morphology between type I and type II methanotrophs, they might also differ in their values of δ13C. However, little is known about the δ13C characteristics of type I and type II methanotrophs. Such knowledge is very important for the understanding of the biogeochemical processes mediated by methanotrophs in peatlands.
The altitude gradient was supposed to be an ideal natural laboratory for studying climate change (Siles et al., 2017). The peatlands along the altitude gradient are important ecosystems in the Changbai Mountain regain of China, and they are extremely sensitive to climate change (Wang et al., 2021; Zhao et al., 2021). Therefore, we selected eight sedge-dominated peatlands along an altitude gradient in the Changbai Mountain to reveal the influence of the environmental changes along an altitude gradient on the concentrations and metabolic characteristics of methanotrophs in peatlands. We asked the following research questions: (1) how does the concentration of type I and type II methanotroph biomarkers change along the altitude gradient? (2) If the trends in δ13C values of type I and type II methanotrophs differ along the altitude gradient?
Materials and Methods
Study Area
We collected samples on the north slope of the Changbai Mountain (41°41′49″– 42°25′18″ N, 127°42′55″–128°16′48″ E). Changbai Mountain is located in the northeast of China and is adjacent to the border between China and North Korea. Changbai Mountain is the highest mountain on the eastern edge of Eurasia, and it is vulnerable to climate change (Wang et al., 2021; Zhao et al., 2021). The peatlands are widely distributed in the Changbai Mountains with an altitude of about 300 to 1,500 m. The total area of peatlands in this region is about 23,100 ha, of which 70% are sedge peatlands dominated by Carex species (Bao et al., 2010; Wang et al., 2017). This area belongs to a temperate continental monsoon climate, which is characterized by long, cold winters and short, cool summers. The average annual temperature (MAT) from 327 to 1,485 m in our study ranges from –0.175 to 3.3°C, and the annual precipitation (MAP) is between 605 and 766 mm.
Soil Sampling
In July 2020, we collected soil samples of the top 5 cm in 8 sedge peatlands along an altitude gradient (327, 430, 540, 683, 1,005, 1,161, 1,270, and 1,485 m; Figure 1; and Table 1). These sites were all dominated by Carex schmidtii, which is a tussock-forming sedge species (Zhao et al., 2021). All the sites were flooded during the growing season. The sampling sites were located in the hollows with a 2–5 cm water depth (Wang et al., 2017). Three replicate samples were collected at each study site. The location of each sample was at least 10 m apart from each other. The peat samples were stored at –20°C.
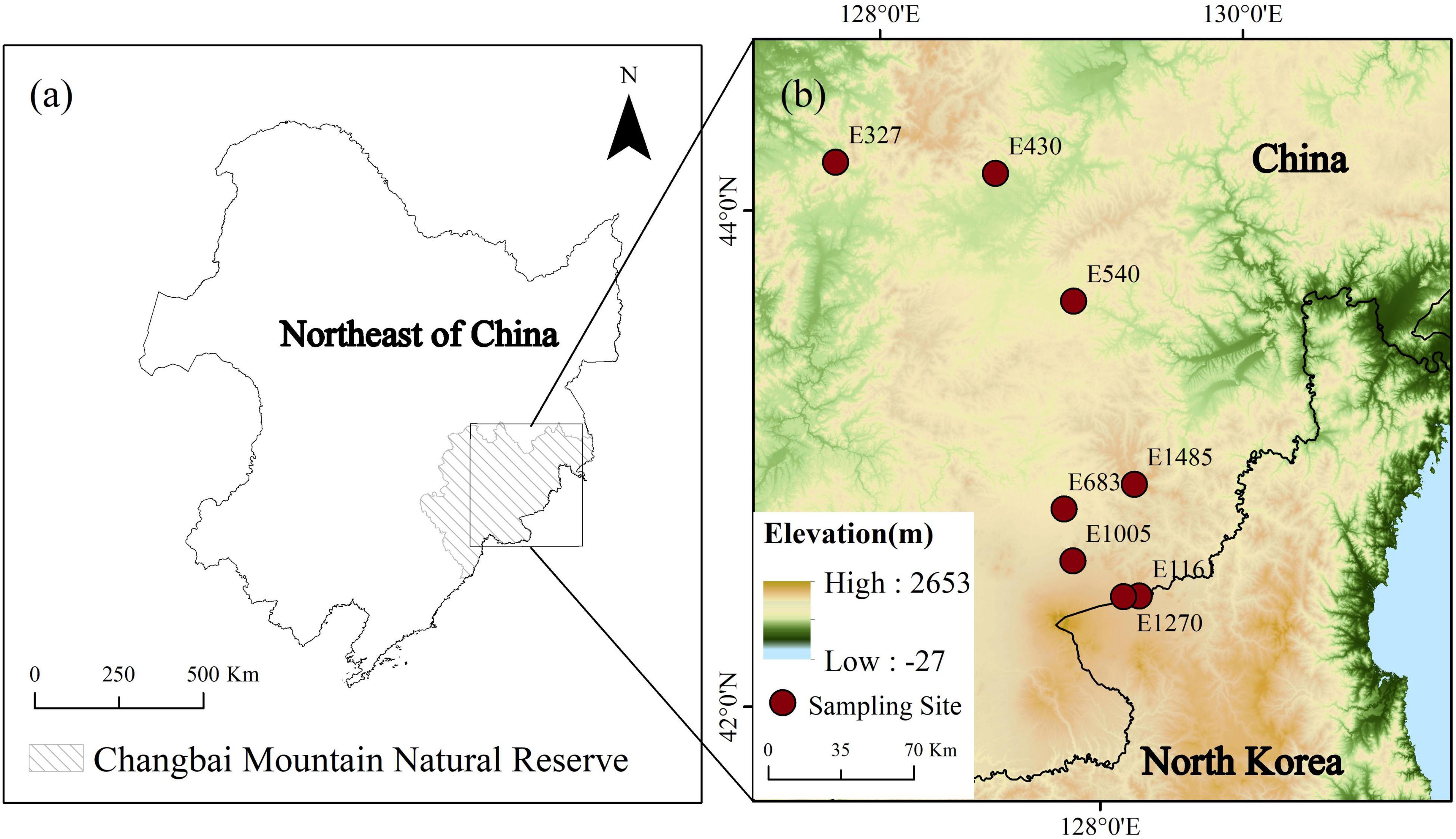
Figure 1. Locations of the sampling sites along the altitude gradient in peatlands of the Changbai Mountain, China.
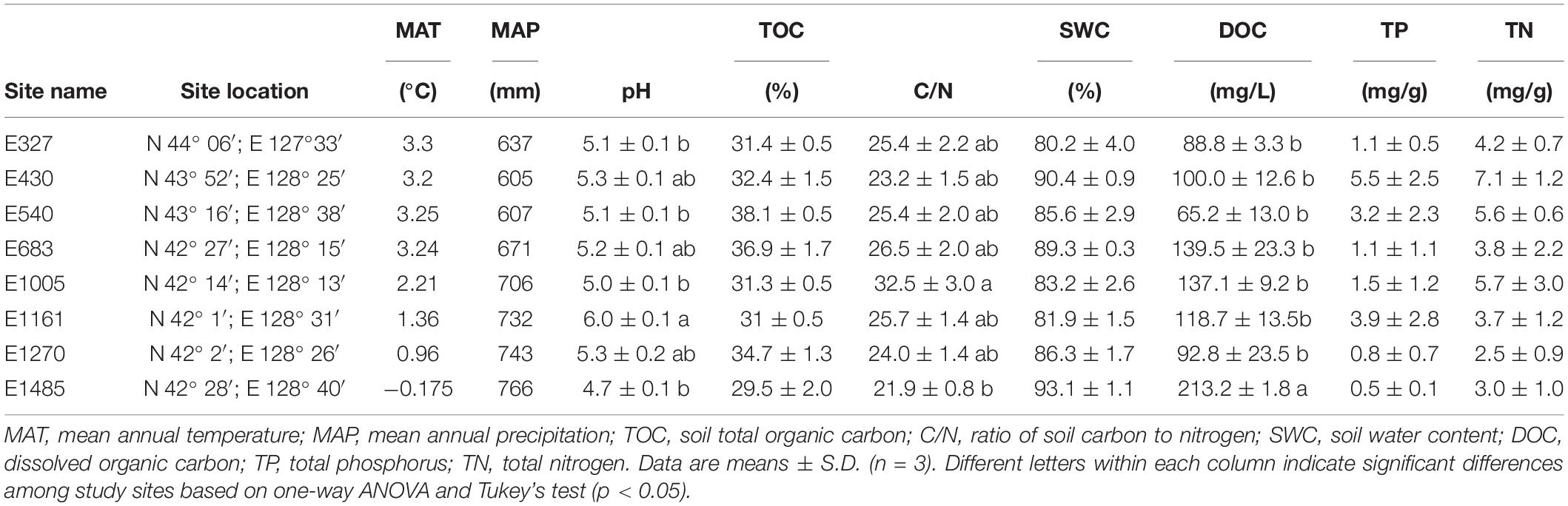
Table 1. Site information and general climate and soil characteristics in the sedge-dominated peatlands of the Changbai Mountains, China.
Soil Physical and Chemical Properties
The soil water content (SWC) was determined by the gravimetric method with the samples freeze-dried (Li et al., 2018). The dissolved organic carbon (DOC) was measured by the method of K2SO4 extraction with distilled water and 0.5 M K2SO4 at a 1:5 w/v ratio for 1 h at 20°C (Jones and Willett, 2006). Total phosphorus (TP) and total nitrogen (TN) were measured by the Kjeldahl method and the Molybdenum blue method using an automated analyzer (Smartchem 140, AMS-Alliance, French), respectively. Soil pH was determined by a potentiometric test. The total organic carbon (TOC) and carbon to nitrogen ratio (C/N) were detected on an elemental analyzer (Vario Macro cube, Hanau, Germany).
The Phospholipid Fatty Acids and the Stable Carbon Isotope Analysis
The analysis of type I and type II methanotrophs was based on PLFA technology. The extraction and separation methods of PLFAs are referred to as the Bligh Dyer method (Bligh and Dyer, 1959; Yao et al., 2012). PLFA composition was identified and analyzed on an Agilent 7890b gas chromatograph (GC) equipped with a 19091b-102 column (25 m × 0.20 mm × 0.33 μm film thickness), and cy19:0 fatty acid methyl ester was used as the external standard. The inlet temperature was 250°C and the detector temperature was 320°C. The carrier gas was helium and the column flow rate was 1.2 ml/min. The heating procedure was an initial temperature of 190°C for 1 min; followed by 10°C/min to 285°C, and finally 60°C/min to 310°C, held for 2 min. The detected compounds were automatically compared with the MIDI library to determine the carbon chain structure of fatty acids (Zhang et al., 2019). The PLFAs of 16:1ω7c and 16:1ω5c were used as the biomarkers of type I methanotroph, and the PLFA of 18:1ω7c was used as the biomarker of type II methanotroph (Bowman et al., 1991, 1993).
The δ13C values of PLFAs components were determined using a Finnigan Delta Plus XP instrument equipped with a DB-5MS column (60 m × 0.25 mm × 0.25 μm film thickness), using CO2 as a reference gas. The temperatures of the inlet and the detector were 200 and 350°C, respectively. The oxidation furnace temperature was 950°C and the reduction temperature was 650°C. The carrier gas was helium, and the column flow rate was 1.2 ml/min. The heating program had four stages: (1) keeping 100°C for 1 min, (2) 20°C/min to 190°C, (3) 1.5°C/min to 235°C, and (4) 20°C/min to 295°C and holding for 15 min. The Pee Dee Belemnite (PDB) standard was used to report all results of the 13C/12C ratio in δ notation, expressed in difference per mil (‰): δ13C (‰) = [(Rcompounds/Rstd-1)] × 1,000, where Rcompounds was the ratio of 13C/12C between the samples, and Rstd was the ratio of 13C/12C in the PDB standard (Börjesson et al., 2016). During methylation, an additional C atom was added to the fatty acid molecule, and the δ13C values have been calibrated by formula (Williams et al., 2006).
Data Analysis
The differences in soil environmental variables, the concentrations, and the δ13C values of type I and type II methanotroph biomarkers were performed by one-way ANOVA and a subsequent Tukey’s test. Pearson’s correlation analysis was used to study the relationships between the environmental variables and the concentrations of type I and type II methanotroph biomarkers with the altitude. General linear regression was used to examine the trends in concentrations of type I and type II methanotroph biomarkers along with the altitude. All variables were examined for the normality and homogeneity of variance before analysis. The analysis was carried out in SPSS 21.0. Redundancy analysis (RDA) was used to determine the relationship between type I (16:1ω7c and 16:1ω5c) and type II (18:1ω7c) methanotroph biomarkers and environmental factors (MAT, MAP, TOC, DOC, pH, TN, TP, C/N, and altitude) by using CANOCO 5.
Results
Climate and Soil Environmental Factors Along the Altitude Gradient
In the study area, the MAT and MAP varied from 3.3 to –0.175°C and from 605 to 766 mm, respectively (Table 1). The pH ranged from 4.7 to 6.0. The TOC ranged from 29.5 to 38.1%. The TP and TN were highest at E430 (5.5 and 7.1 mg/g), and lowest at sites of E1485 and E1270, respectively. The DOC varied significantly from 88.8 mg/L at E327 to 213.2 mg/L at E1485. The C/N varied significantly from 21.9 at E1485 to 32.5 at E1005. The SWC ranged from 80.2 to 93.1%. There was no significant difference between TOC and SWC across the study sites (Table 2). Pearson’s correlation analysis showed that the altitude was negatively related to MAT, TN, and TP, and it was positively related to DOC (Table 3). There were no significant correlations between the altitude and MAP, TOC, SWC, pH, and C/N (Table 3).
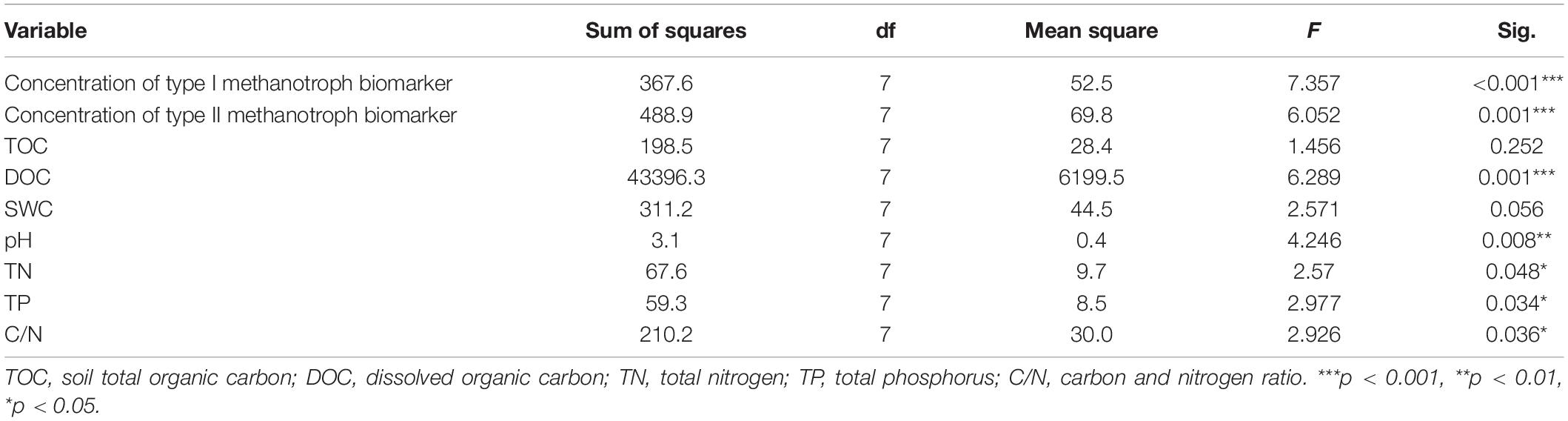
Table 2. A summary of analysis of variance (one-way ANOVA) on the effect of the altitude for soil environmental variables, and the concentrations of type I and type II methanotroph biomarkers.
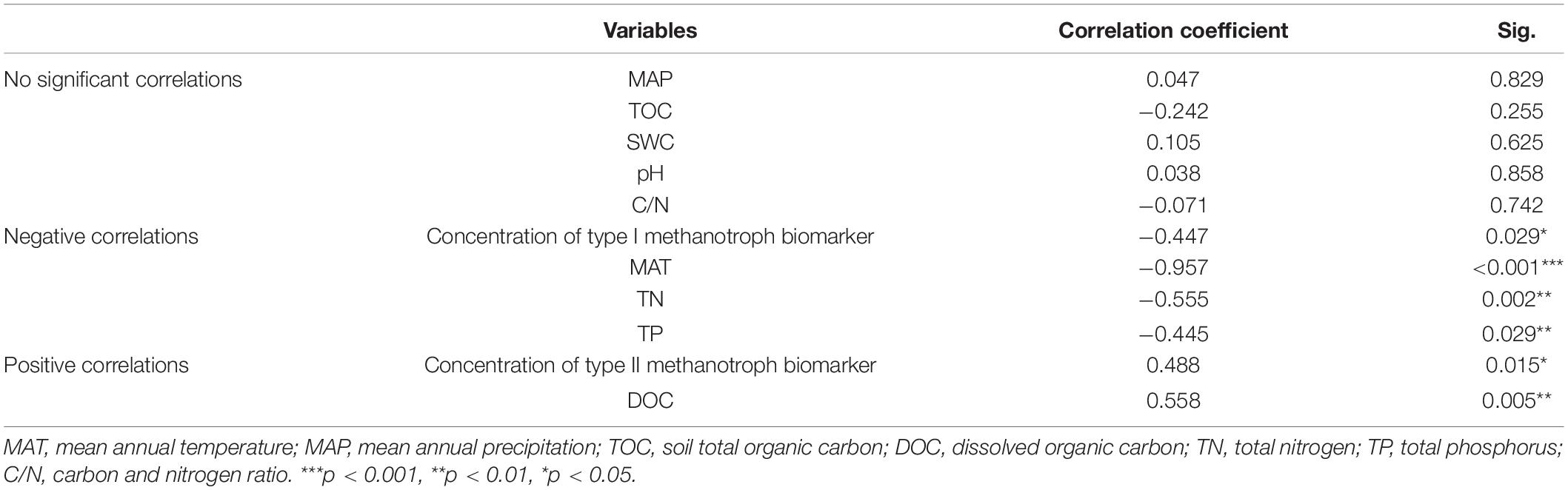
Table 3. Pearson correlation coefficients between the concentrations of type I and type II methanotroph biomarker, environmental variables and the altitude.
The Concentration of Methanotroph Biomarkers Along the Altitude Gradient
The concentrations of type I and type II methanotroph biomarkers changed significantly along the altitude gradient (Figure 2A). The concentration of the type I methanotroph biomarker was highest at E540 (37.7 μg/g) and lowest at E1270 (9.5 μg/g). The concentration of the type II methanotroph biomarker was highest at E1485 (31.2 μg/g) and lowest at E430 (17.3 μg/g). The concentrations of the two methanotroph biomarkers differed significantly at sites of E540, E1485, and E1270 (Figure 2A). The concentration of type I methanotroph biomarker showed a negative linear relationship with the altitude (Figure 2B), and the concentration of type II methanotroph biomarker showed a positive linear relationship with the altitude (Figure 2C). The Pearson correlation analysis showed that the altitude was negatively related to the concentration of type I methanotroph biomarker, and it was positively related to the concentration of type II methanotroph biomarker (Table 3).
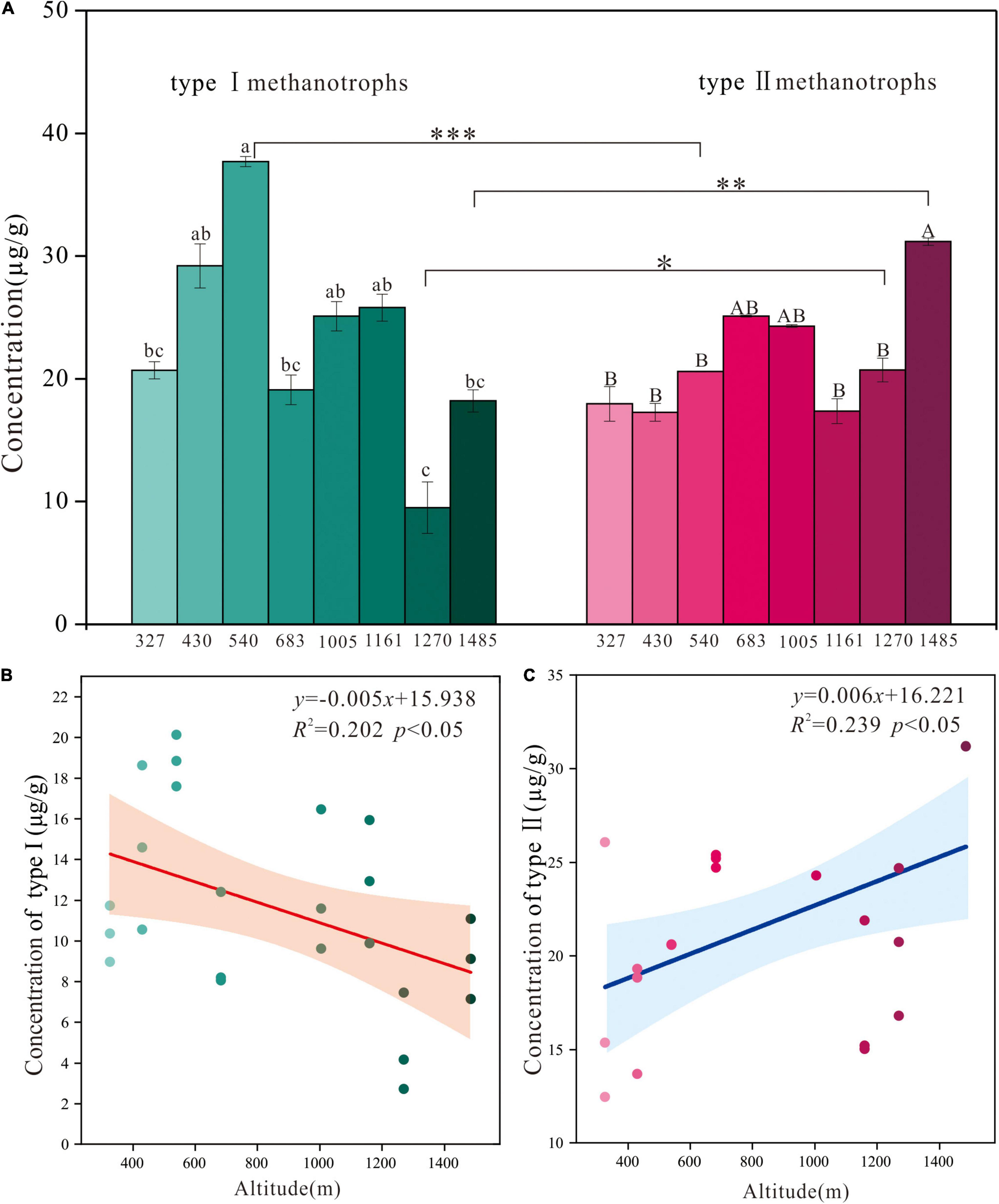
Figure 2. (A) The concentrations of type I and type II methanotroph biomarkers along the altitude gradient on the northern slope of the Changbai Mountain, China. Values were presented as means ± S.E. (n = 3). Different letters indicate significant differences among study sites based on one-way ANOVA and Tukey’s test (p < 0.05). The asterisk represents the difference between type I and type II methanotrophs at the same site (***p < 0.001, **p < 0.01, and *p < 0.05). Regression of the altitude against the concentration of type I (B) and type II (C) methanotroph biomarkers. The R2 and p values of the regression equation are shown in each panel. Significant relationships are denoted with solid lines, and shadow areas indicate the 95% confidence interval (CI) of the fit.
Redundancy analysis showed that the variation in type I (16:1ω5c and 16:1ω7c) and type II (18:1ω7c) methanotroph biomarkers explained by the first axis was 47.30, and 17.75% by the second axis (Figure 3). The environmental change along the altitude gradient was reflected along the first axis of the RDA ordination where the sites in the lower altitude were arranged from high MAT, TN, and TP on the left toward high DOC and SWC in the higher altitude on the right (Figure 3). PLFAs of 16:1ω5c and 16:1ω7c were distributed in the lower left quadrant, while 18:1ω7c was distributed in the lower right quadrant. DOC and TP explained most variation in the concentration of the type I and type II methanotroph biomarkers with the explained degree of 22.3 and 23.4%, respectively (Figure 3). The study sites of E327, E430, and E540 were distributed in the middle left quadrat, the sites of E683, E1005, E1161, and E1270 were mainly distributed in the middle right quadrat, and the site of E1485 was distributed in the lower right quadrat (Figure 3).
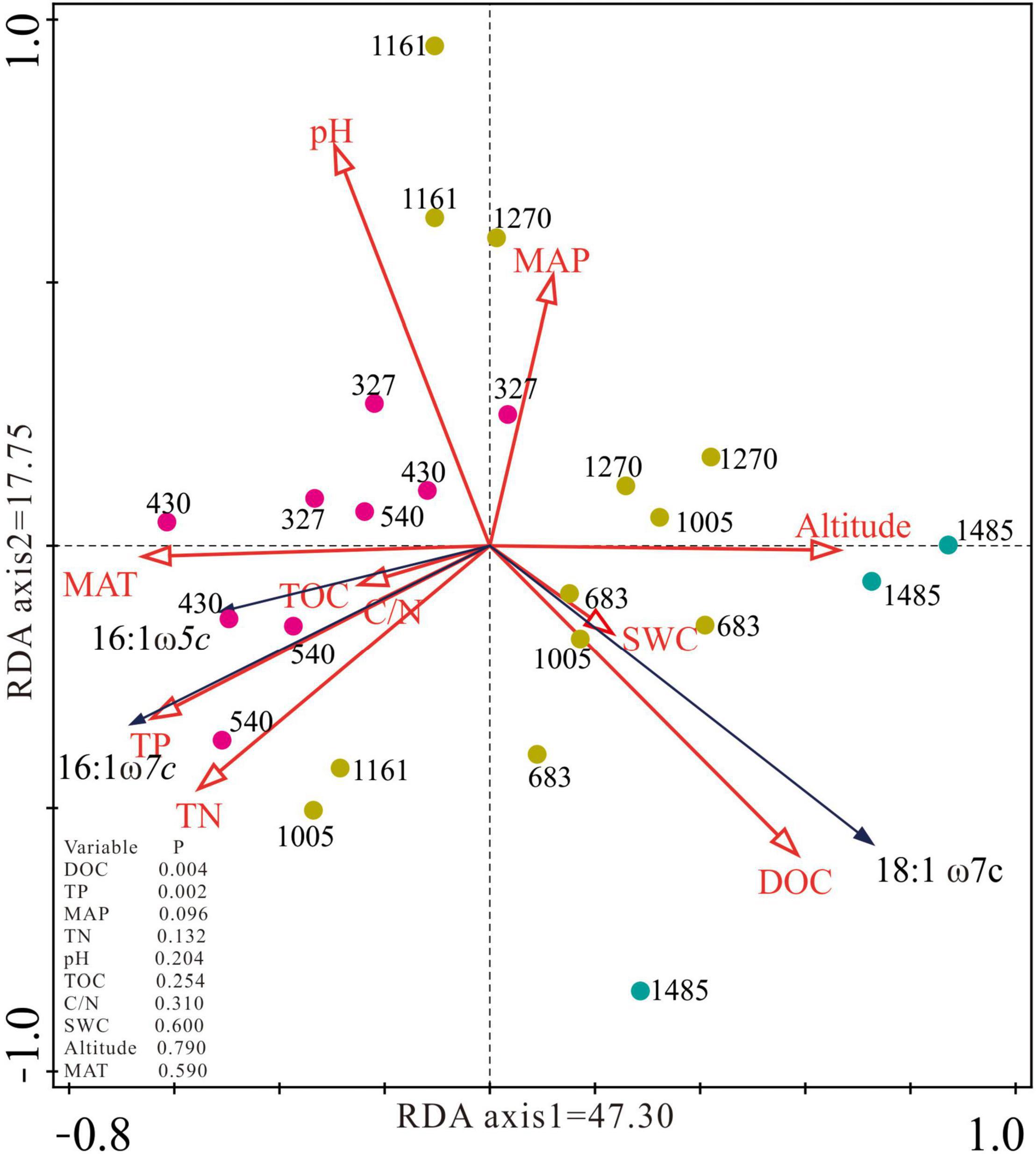
Figure 3. Redundancy analysis (RDA) ordination plot of the concentration of 16:1ω7c, 16:1ω5c, and 18:1ω7c constrained by the altitude, climate, and soil environmental factors. MAT, mean annual temperature; MAP, mean annual precipitation; TOC, soil total organic carbon; DOC, dissolved organic carbon; TN, total nitrogen; TP, total phosphorus; C/N, carbon and nitrogen ratio.
The δ13C Values of Methanotroph Biomarkers Along the Altitude Gradient
The δ13C values differed significantly between type I and type II methanotroph biomarkers (Figure 4). The δ13C values of type I methanotroph biomarkers ranged widely across each site, except for the study sites of E540 and E1005. The δ13C values of type I methanotroph biomarkers also changed significantly along the altitude gradient (Figure 4). At the same time, the δ13C values of type II methanotroph biomarkers had a small range (Figure 4). The δ13C values of type I methanotroph biomarkers were more depleted than type II methanotroph biomarkers. Furthermore, the δ13C value of type II methanotroph biomarkers at E430 was more depleted compared with the other seven study sites, but there was no significant difference between these seven sites (p > 0.05; Figure 4). Except for E430, the δ13C values of type I methanotroph biomarkers were most depleted (–53.72‰) at E1161 among all the sites, and the δ13C values of type II methanotroph biomarkers were most depleted (−34.62‰) at E1161 (Figure 4).
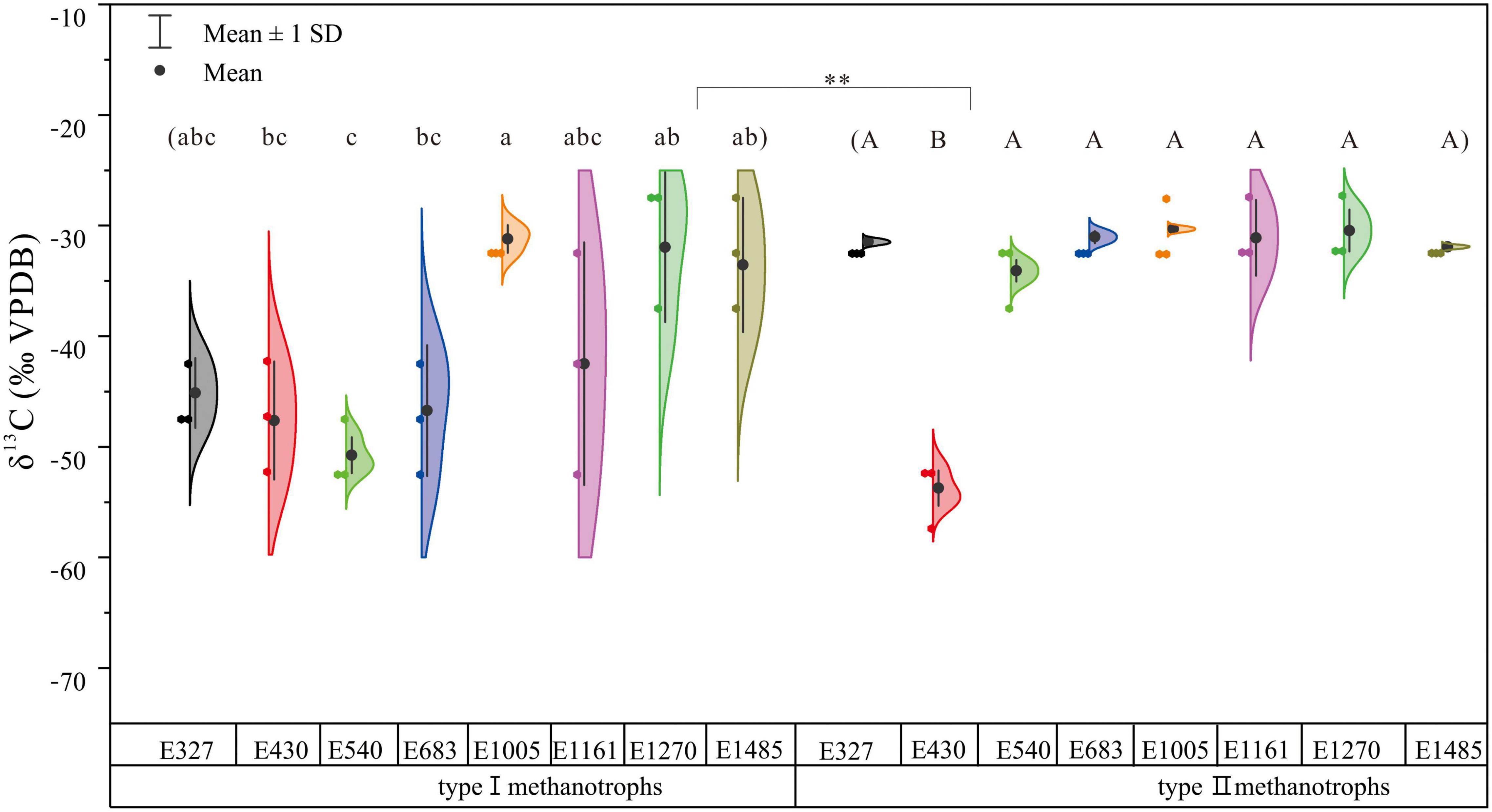
Figure 4. The δ13C values of type I and type II methanotroph biomarkers based on phospholipid fatty acids along the altitude gradient on the northern slope of the Changbai Mountain, China. Different letters indicate significant differences among study sites based on one-way ANOVA and Tukey’s test (p < 0.05). The asterisk represents the difference between type I and type II methanotrophs (**p < 0.01). The δ13C values of type I methanotroph biomarkers were the average δ13C values of 16:1ω7c and 16:1ω5c.
Discussion
Factors Affecting the Concentration of Methanotroph Biomarkers
In recent years, ecologists have tried to understand patterns in soil microbes and the associated processes along environmental gradients. For example, former researchers had studied the changes in CH4 oxidation rate under different conditions, mainly including water table, temperature, vegetation, wildfire, and season (Kim et al., 2011; Danilova et al., 2015). However, little is known about the patterns and functions of the methanotrophs along the altitude gradient and their response to environmental changes in peatlands.
In this study, we found that the concentration of type I methanotroph biomarker was negatively correlated with the altitude, while the concentration of type II methanotroph biomarker was positively correlated with the altitude (Figure 2 and Table 3), which may be caused by the change of peatland environments along with the altitude. The RDA analysis showed that the concentration of type I methanotroph biomarkers (16:1ω7c and 16:1ω5c) was mainly related to TP and TN. In this study, the altitude was negatively related to TN and TP (Table 3), so the TP and TN generally decreased as the altitude increased. Similar to our findings, other studies in paddy fields and forests found that the increase of TN and TP promoted the growth of type I methanotrophs, while type II methanotrophs were generally inhibited (Mohanty et al., 2006; Alam and Jia, 2012). The concentration of type II methanotroph biomarkers was positively correlated with DOC (Figure 3). This is because DOC is more active in the peatland carbon pool and is easily absorbed and utilized by microorganisms, especially the type II methanotroph (Zhao et al., 2021). In our study, DOC generally increased with altitude and reached its maximum at the highest altitude (E1485), where the type II methanotroph dominated at the higher altitude. Although the concentrations of type I and type II methanotroph biomarkers were both significantly correlated with the altitude, the effect of location on the concentrations of the two types of methanotroph biomarkers exceeded that of altitude (Table 2 and Figure 2).
Characteristics of Methanotroph Metabolism Along the Altitude Gradient
The δ13C values of type I and type II methanotroph biomarkers differed significantly along the altitude gradient (Figure 4). The δ13C values of type I methanotroph biomarkers were more negative and the isotope composition ranged widely (Figure 4). Type I methanotrophs primarily used CH4 with extremely negative isotope values (–65‰ ≤ δ13C ≤ –50‰) as an energy source (Whiticar et al., 1986), and type I methanotrophs combine CH4 into the membrane and use CH4 for assimilation and energy generation. The CH4 is finally converted into CO2 and released into the atmosphere (Holmes et al., 1999; Bull et al., 2000). Meanwhile, the type II methanotrophs assimilated about half of the carbon from CH4, and the rest was derived from CO2 (Hanson and Hanson, 1996; Holmes et al., 1999). Similarly, the type II methanotrophs were also found to assimilate CH4 and CO2 to form CO2 and release it into the atmosphere in the subtropical forests (Zhou et al., 2021). Interestingly, the type II methanotroph biomarker in E430 was more negative than in other sites, it may be because the abundant TN and TP in soils promoted the utilization rate of CH4, and thus increased the microbial activity and carbon isotope fractionation (Steudler et al., 1989). This phenomenon also confirmed the accuracy of 18:1ω7c as the methanotroph biomarker in the sedge peatlands.
Compared with the type II methanotrophs, the change of δ13C values of type I methanotrophs was more significant along the altitude gradient. The δ13C value of type I methanotroph biomarker was lowest in E683, this site may produce the most abundant CH4 used by methanotrophs due to the difference in vegetation (Chen et al., 2009), water table (Kim et al., 2011; Tian et al., 2015; Shao et al., 2017), and temperature (King and Adamsen, 1992; Sitaula et al., 1995). Abundant CH4 and O2 can provide a suitable environment for the growth of the methanotrophs and promote CH4 oxidation activity. Less than maximum fractionation is observed when cells are grown with reduced CH4 availability (Summons et al., 1994). The obvious change in the δ13C values of type I methanotroph biomarkers with the altitude gradient may also be due to their rapid response to the environmental changes, which has been found by other studies (Henckel et al., 2000). Meanwhile, former studies found that methanotrophs were closely related to CH4 concentration in the atmosphere (Chen et al., 2008). In our study, combining the concentrations and natural 13C content of the methanotroph biomarkers, we found that the concentrations of type I methanotrophs using CH4 as the only carbon source decreased with the increase of altitude, which further indicated that the ability of CH4 consumption at higher altitudes decreased. The peatlands at a higher altitude might retain more unstable CH4 than at a lower altitude.
Conclusion
This is the first research on the concentrations and metabolic characteristics of methanotrophs along an altitude gradient in the northern peatlands. We found that the patterns of type I and type II methanotroph biomarkers differed significantly along the altitude gradient. RDA analyses indicated that the type I methanotroph biomarkers were positively correlated with TP and TN, and the type II methanotroph biomarkers were positively correlated with DOC. Compared with type II, the change in the δ13C values of type I methanotroph biomarkers was more obvious as the altitude increased, which indicated their different preferences for substrate utilization. Our study laid the foundation for the study of CH4 metabolism in peatlands along the altitude gradient. Further research combined with CH4 flux and 16S rRNA sequencing data is required to better understand the biogeochemical process of methanotrophs in peatlands under the warming climate.
Data Availability Statement
The original contributions presented in the study are included in the article/supplementary material, further inquiries can be directed to the corresponding author.
Author Contributions
MZ, MW, and GW designed the study. MZ, MW, YZ, and MJ performed the field investigation and collected the data. MZ and GW conducted the statistical analysis and wrote the manuscript. All authors reviewed and contributed to the manuscript writing.
Funding
This study was supported by the National Natural Science Foundation of China (41877075, 41871081, U19A2042, and 42077070), the Professional Association of the Alliance of International Science Organizations (ANSO-PA-2020-14), and the Youth Innovation Promotion Association CAS (2019234).
Conflict of Interest
The authors declare that the research was conducted in the absence of any commercial or financial relationships that could be construed as a potential conflict of interest.
Publisher’s Note
All claims expressed in this article are solely those of the authors and do not necessarily represent those of their affiliated organizations, or those of the publisher, the editors and the reviewers. Any product that may be evaluated in this article, or claim that may be made by its manufacturer, is not guaranteed or endorsed by the publisher.
References
Alam, M. S., and Jia, Z. J. (2012). Inhibition of methane oxidation by nitrogenous fertilizers in a paddy soil. Front. Microbiol. 3:00246. doi: 10.3389/fmicb.2012.00246
Bao, K. S., Yu, X. F., Jia, L., and Wang, G. P. (2010). Recent carbon accumulation in Changbai Mountain peatlands, northeast China. Mount. Res. Dev. 30, 33–41. doi: 10.1659/MRD-JOURNAL-D-09-00054.1
Bligh, E. G., and Dyer, W. J. (1959). A rapid method of total lipid extraction and purification. Can. J. Biochem. Physiol. 37, 911–917.
Börjesson, G., Menichetti, L., Thornton, B., Campbell, C. D., and Kätterer, T. (2016). Seasonal dynamics of the soil microbial community: assimilation of old and young carbon sources in a long-term field experiment as revealed by natural 13C abundance. Eur. J. Soil Sci. 67, 79–89. doi: 10.1111/ejss.12309
Bowman, J. P., Skerratt, J. H., Nichols, P. D., and Sly, L. L. (1991). Phospholipid Fatty-acid and lipopolysaccharide fatty-acid signature lipids in methane-utilizing bacteria. FEMS Microbiol. Ecol. 85, 15–22. doi: 10.1016/0378-1097(91)90627-M
Bowman, J. P., Sly, L. I., Nichols, P. D., and Hayward, A. C. (1993). Revised taxonomy of the methanotrophs – description of methylobacter gen-nov, emendation of methylococcus, validation of methylosinus and methylocystis species, and a proposal that the family methylococcaceae includes only the group-I methanotrophs. Int. J. Syst. Bacteriol. 43, 735–753. doi: 10.1099/00207713-44-2-375
Bull, I. D., Parekh, N. R., Hall, G. H., Ineson, P., and Evershed, R. P. (2000). Detection and classification of atmospheric methanotrophs in soil. Nature 405, 175–178. doi: 10.1038/35012061
Chen, H., Wu, N., Yao, S. P., Gao, Y. H., Zhu, D., Wang, Y. F., et al. (2009). High methane emissions from a littoral zone on the Qinghai-Tibetan Plateau. Atmos. Environ. 43, 4995–5000. doi: 10.1016/j.atmosenv.2009.07.001
Chen, Y., Dumont, M. G., McNamara, N. P., Chamberlain, P. M., Bodrossy, L., Stralis-Pavese, N., et al. (2008). Diversity of the active methanotrophic community in acidic peatlands as assessed by mRNA and SIP-PLFA analyses. Environ. Microbiol. 10, 446–459. doi: 10.1111/j.1462-2920.2007.01466.x
Chowdhury, T. R., and Dick, R. P. (2013). Ecology of aerobic methanotrophs in controlling methane fluxes from wetlands. Appl. Soil Ecol. 65, 8–22. doi: 10.1016/j.apsoil.2012.12.014
Cifuentes, L. A., and Salata, G. G. (2001). Significance of carbon isotope discrimination between bulk carbon and extracted phospholipid fatty acids in selected terrestrial and marine environments. Organ. Geochem. 32, 613–621. doi: 10.1016/S0146-6380(00)00198-4
Danilova, O. V., Belova, S. E., Kulichevskaya, I. S., and Dedysh, S. N. (2015). Decline of activity and shifts in the methanotrophs community structure of an ombrotrophic peat bog after wildfire. Microbiology 84, 624–629. doi: 10.1134/S0026261715050045
Dean, J. F., Middelburg, J. J., Rickmann, T., Aerts, R., Blauw, L. G., Egger, M., et al. (2018). Methane feedbacks to the global climate system in a warmer world. Rev. Geophys. 56, 207–250. doi: 10.1002/2017RG000559
Dedysh, S. N., Belova, S. E., Bodelier, P. L. E., Smirnova, K. V., Khmelenina, V. N., Chidthaisong, A., et al. (2007). Methylocystis heyeri sp. nov., a novel type II methanotrophic bacterium possessing ‘signature’ fatty acids of type I methanotrophs. Int. J. Syst. Evol. Microbiol. 57, 472–479. doi: 10.1099/ijs.0.64623-0
Hanson, R. S., and Hanson, T. E. (1996). Methanotrophs bacteria. Microbiol. Rev. 60, 439–471. doi: 10.1128/MMBR.60.2.439-471.1996
Henckel, T., Roslev, P., and Conrad, R. (2000). Effects of O2 and CH4 on presence and activity of the indigenous methanotrophic community in rice field soil. Environ. Microbiol. 2, 666–679. doi: 10.1046/j.1462-2920.2000.00149.x
Holmes, A. J., Roslev, P., McDonald, I. R., Iversen, N., Henriksen, K., and Murrell, J. C. (1999). Characterization of methanotrophic bacterial populations in soils showing atmospheric methane uptake. Appl. Environ. Microbiol. 65, 3312–3318. doi: 10.1128/aem.65.8.3312-3318.1999
Jones, D. L., and Willett, V. B. (2006). Experimental evaluation of methods to quantify dissolved organic nitrogen (DON) and dissolved organic carbon (DOC) in soil. Soil Biol. Biochem. 38, 991–999. doi: 10.1016/j.soilbio.2005.08.012
Kim, Y., Tero, T., Hell, J., Anuliina, P., Kaisa, L., Eeva-Stina, T., et al. (2011). CH4 production and oxidation processes in a boreal fen ecosystem after long-term water table drawdown. Glob. Change Biol. 17, 1311–1320. doi: 10.1111/j.1365-2486.2010.02290.x
King, G. M., and Adamsen, A. P. S. (1992). Effects of temperature on methane consumption in a forest soil and in pure cultures of the methanotroph Methylomonas rubra. Appl. Environ. Microbiol. 58, 2758–2763. doi: 10.1128/AEM.58.9.2758-2763.1992
Kip, N., Ouyang, W., van Winden, J., Raghoebarsing, A., van Niftrik, L., Pol, A., et al. (2011). Detection, isolation, and characterization of acidophilic methanotrophs from Sphagnum mosses. Appl. Environ. Microbiol. 77, 5643–5654. doi: 10.1128/AEM.05017-11
Li, Y., Zhao, S. H., Pei, H. Y., Qian, S., Zang, Q. J., Dang, X. Y., et al. (2018). Distribution of glycerol dialkyl glycerol tetraethers in surface soils along an altitudinal transect at cold and humid Mountain Changbai: implications for the reconstruction of paleoaltimetry and paleoclimate. Sci. China Earth Sci. 61, 925–939. doi: 10.1007/s11430-017-9168-9
Mohanty, S. R., Bodelier, P. L. E., Floris, V., and Conrad, R. (2006). Differential Effects of Nitrogenous Fertilizers on Methane-Consuming Microbes in Rice Field and Forest Soils. Appl. Environ. Microbiol. 72, 1346–1354. doi: 10.1128/AEM.72.2.1346-1354.2006
Raghoebarsing, A. A., Smolders, A. J., Schmid, M. C., Rijpstra, W. I. C., Wolters-Arts, M., Derksen, J., et al. (2005). Methanotrophic symbionts provide carbon for photosynthesis in peat bogs. Nature 436, 1153–1156. doi: 10.1038/nature03802
Shao, X. X., Sheng, X. C., Wu, M., Wu, H., and Ning, X. (2017). Methane production potential and emission at different water levels in the restored reed wetland of Hangzhou Bay. PLoS One 12:e01851079. doi: 10.1371/journal.pone.0185709
Siles, J. A., Cajthaml, T., Filipová, A., Minerbi, S., and Margesin, S. (2017). Altitudinal, seasonal and interannual shifts in microbial communities and chemical composition of soil organic matter in Alpine forest soils. Soil Biol. Biochem. 112, 1–13. doi: 10.1016/j.soilbio.2017.04.014
Sitaula, B. K., Bakken, L. R., and Abrahamsen, G. (1995). CH4 uptake by temperate forest soil: effect of N input and soil acidification. Soil Biol. Biochem. 27, 871–880. doi: 10.1016/0038-0717(95)00017-9
Steudler, P. A., Bowden, R. D., Melillo, J. M., and Aber, J. D. (1989). Influence of nitrogen fertilization on methane uptake in temperate forest soils. Lett. Nat. 341, 314–316. doi: 10.1038/341314a0
Summons, R. E., Jahnke, L. L., and Roksandic, Z. (1994). Carbon isotopic fractionation in lipids from methsnotrophic bacteria: relevance for interpretation of the geochemical record of biomarkers. Geochim. et Cosmochim. Acta 58, 2853–2863. doi: 10.1016/0016-7037(94)90119-8
Tian, J. Q., Shu, C., Chen, H., Qiao, Y. C., Yang, G., Xiong, W., et al. (2015). Response of archaeal communities to water regimes under simulated warming and drought conditions in Tibetan Plateau wetlands. J. Soils Sediment. 15, 179–188. doi: 10.1007/s11368-014-0978-1
Wang, M., Wang, G. D., Wang, S. Z., and Jiang, M. (2017). Structure and richness of Carex meyeriana tussocks in peatlands of northeastern China. Wetlands 38, 15–23. doi: 10.1007/s13157-017-0952-y
Wang, M., Wang, S. Z., Cao, Y. W., Jiang, M., Wang, G. D., and Dong, Y. M. (2021). The effects of hummock-hollow microtopography on soil organic carbon stocks and soil labile organic carbon fractions in a sedge peatland in Changbai Mountain, China. Catena 201:105204. doi: 10.1016/j.catena.2021.105204
Watzinger, A. (2015). Microbial phospholipid biomarkers and stable isotope methods help reveal soil functions. Soil Biol. Biochem. 86, 98–107. doi: 10.1016/j.soilbio.2015.03.019
Whiticar, M., Faber, E., and Schoell, M. (1986). Biogenic methane formation in marine and freshwater environments: CO2 reduction vs. acetate fermentation-Isotope evidence. Geochim. et Cosmochim. Acta 50, 693–709. doi: 10.1016/0016-7037(86)90346-7
Williams, M. A., Myrold, D. D., and Bottomley, P. J. (2006). Carbon flow from 13C-labeled straw and root residues into the phospholipid fatty acids of a soil microbial community under field conditions. Soil Biol. Biochem. 38, 759–768. doi: 10.1016/j.soilbio.2005.07.001
Yao, H., Thornton, B., and Paterson, E. (2012). Incorporation of 13C-labelled rice rhizodeposition carbon into soil microbial communities under different water status. Soil Biol. Biochem. 53, 72–77. doi: 10.1016/j.soilbio.2012.05.006
Zhang, Y. M., Naafs, B. D. A., Huang, X. Y., Song, Q. W., Xue, J. T., Wang, R. C., et al. (2021). Variations in wetland hydrology drive rapid changes in the microbial community, carbon metabolic activity, and greenhouse gas fluxes. Geochim. et Cosmochim. Acta 317, 269–285. doi: 10.1016/j.gca.2021.11.014
Zhang, Y. Y., Zheng, N. G., Wang, J., Yao, H. Y., Qiu, Q. F., and Chapman, S. J. (2019). High turnover rate of free phospholipids in soil confirms the classic hypothesis of PLFA methodology. Soil Biol. Biochem. 135, 323–330. doi: 10.1016/j.soilbio.2019.05.023
Zhao, M. L., Wang, M., Zhao, Y. T., Wang, G. D., Xue, Z. S., and Jiang, M. (2021). Variations in soil microbial communities in the sedge-dominated peatlands along an altitude gradient on the northern slope of Changbai Mountain, China. Ecol. Indicat. 129:107964. doi: 10.1016/j.ecolind.2021.107964
Zhao, M. L., Zhang, Y. M., Zhang, Z. Q., and Huang, X. Y. (2020). Comparison of microbial community in topsoil among different habitats in Dajiuhu, Hubei province: evidence from phospholipid fatty acids. Earth Sci. 45, 1877–1886. doi: 10.3799/dqkx.2019.272
Keywords: altitude gradient, methanotroph biomarker, metabolic characteristics, sedge peatland, competitive distribution
Citation: Zhao M, Wang M, Zhao Y, Jiang M and Wang G (2022) Variations in Concentration and Carbon Isotope Composition of Methanotroph Biomarkers in Sedge Peatlands Along the Altitude Gradient in the Changbai Mountain, China. Front. Microbiol. 13:892430. doi: 10.3389/fmicb.2022.892430
Received: 09 March 2022; Accepted: 19 April 2022;
Published: 18 May 2022.
Edited by:
Maraike Probst, University of Innsbruck, AustriaReviewed by:
Meng Wang, Northwest A&F University, ChinaXinhou Zhang, Nanjing Normal University, China
Copyright © 2022 Zhao, Wang, Zhao, Jiang and Wang. This is an open-access article distributed under the terms of the Creative Commons Attribution License (CC BY). The use, distribution or reproduction in other forums is permitted, provided the original author(s) and the copyright owner(s) are credited and that the original publication in this journal is cited, in accordance with accepted academic practice. No use, distribution or reproduction is permitted which does not comply with these terms.
*Correspondence: Guodong Wang, d2FuZ2dkQGlnYS5hYy5jbg==