- 1Hubei International Scientific and Technological Cooperation Base of Traditional Fermented Foods, Huazhong Agricultural University, Wuhan, China
- 2College of Food Science and Technology, Huazhong Agricultural University, Wuhan, China
- 3Hubei Key Laboratory of Quality Control of Characteristic Fruits and Vegetables, Hubei Engineering University, Xiaogan, China
- 4College of Life Science and Technology, Hubei Engineering University, Xiaogan, China
Monascus spp. are traditional medicinal and edible filamentous fungi in China, and can produce various secondary metabolites, such as Monascus pigments (MPs) and citrinin (CIT). Genetic modification methods, such as gene knock-out, complementation, and overexpression, have been used extensively to investigate the function of related genes in Monascus spp.. However, the resistance selection genes that can have been used for genetic modification in Monascus spp. are limited, and the gene replacement frequency (GRF) is usually <5%. Therefore, we are committed to construct a highly efficient gene editing system without resistance selection marker gene. In this study, using M. ruber M7 as the starting strain, we successfully constructed a so-called markerlessly and highly genetic modification system including the mutants ΔmrpyrGΔmrlig4 and ΔmrpyrGΔmrlig4::mrpyrG, in which we used the endogenous gene mrpyrG from M. ruber M7 instead of the resistance marker gene as the screening marker, and simultaneously deleted mrlig4 related to non-homologous end joining in M. ruber M7. Then, the morphology, the growth rate, the production of MPs and CIT of the mutants were analyzed. And the results show that the mutant strains have normal mycelia, cleistothecia and conidia on PDA+Uridine(U) plate, the biomass of each mutant is also no different from M. ruber M7. However, the U addition also has a certain effect on the orange and red pigments yield of M. ruber M7, which needs our further study. Finally, we applied the system to delete multiple genes from M. ruber M7 separately or continuously without any resistance marker gene, and found that the average GRF of ΔmrpyrGΔmrlig4 was about 18 times of that of M. ruber M7. The markerlessly and highly genetic modification system constructed in current study not only will be used for multi-gene simultaneous modification in Monascus spp., and also lays a foundation for investigating the effects of multi-genes modification on Monascus spp..
Introduction
Monascus spp., a group of traditional medicine and edible filamentous fungi in China, can produce abundant benefit secondary metabolites (SMs) such as Monascus pigments (MPs), γ-aminobutyric acid, monacolin K, and ergosterol (Endo, 1979; Feng et al., 2012; Patakova, 2013; Wu et al., 2013). But some Monascus strains may also yield a kind of mycotoxin, citrinin (CIT) (Blanc et al., 1995; Lin et al., 2008). So, there are a lot of studies on how to improve benefit SMs amount and to decrease and even eliminate CIT content in Monascus-related products (de Carvalho et al., 2006; Hajjaj et al., 2012; Feng et al., 2014). Among them, the genetic modification method such as gene knock-out, complementation, and overexpression is considered as one of the most significant approaches to control benefit SMs and CIT production (Liu et al., 2014, 2016, 2021; Zhang et al., 2019).
However, as we know, up to now, there are limited antibiotic selection marker genes available for the genetic modification of Monascus spp., such as the genes of hygromycin (hph), neomycin (neo) (Li, 2011), pyrithiamine (pyr) (Cui and Li, 2012), and aureobasidin A (aba) (Shimizu et al., 2006). Therefore, it is very difficult to simultaneously modify multiple genes in the same Monascus strain. Moreover, the antibiotic resistance marker genes remaining in the Monascus mutants may affect their growth and metabolism, and when the mutants are used in the production of foods and food additives, there also exist potential food safety hazards (Tuteja et al., 2012; Yang et al., 2015). So, the development of the genetic modification method without antibiotic screening marker gene residues is requisite for Monascus gene modification.
The uridine (U) auxotroph has been exploited widely in the genetic transformation system for many filamentous fungi (Wang et al., 2010; Arentshorst et al., 2015; Huang et al., 2016; Nguyen et al., 2016; Zhang et al., 2020). In fungi, orotidine 5′-phosphate decarboxylase (OMP decarboxylase) encoded by pyrG gene is a key enzyme involved in the pyrimidine biosynthesis, which can catalyze the decarboxylation of OMP to form uridine monophosphate (UMP) (Caroline and Davis, 1969; Garavaglia et al., 2012). OMP decarboxylase can also transform the pyrimidine analog 5-fluoroorotic acid (5-FOA) to the toxic compound to fungi, 5′-fluoro-UMP, to kill the fungal cells with pyrG (Ying et al., 2013; Zhang et al., 2020). Therefore, U combined with 5-FOA can be used to screen a markerless knockout strain, ΔpyrG, which does not contain the foreign gene including any antibiotic selection marker gene. The strains containing pyrG can synthesize U by themselves and can grow on the media without U, so they are called as U prototrophic or U independent strains, while the strains without pyrG such as ΔpyrG cannot synthesize U by themselves and also cannot grow on the media without U, so they are known as U auxotrophic or U-dependent strains. Therefore, taking ΔpyrG as the starting strain, replacing the target gene with pyrG, and combining U with 5-FOA, the markerless modifier of the target gene can be achieved. Wang et al. (2010) obtained a pyrG gene point mutant strain of M. aurantiacus by ultraviolet mutagenesis, and successfully transferred pyrG back to the pyrG mutant strain. However, there is no report about their subsequent application research of this system.
Several studies have showed that the gene homologous recombination efficiency (GRF) of fungal gene modification is relatively low due to the existence of the non-homologous end-joining (NHEJ) pathway in fungal cells (Ishibashi et al., 2006; Shrivastav et al., 2008; Liu et al., 2018; Pannunzio et al., 2018). And usually, the GRF of Monascus genetic modification is <5% (Li and Chen, 2020). He et al. (2013, 2014) knocked out the relative genes with NHEJ pathway, including the genes of DNA-dependent protein kinase catalytic subunits of Ku70 and Ku80, and ligase 4 (Symington and Gautier, 2011), leading that GRF of Monascus was increased by 2–4 times. However, the obtained high-efficiency strains cannot continue to be used for multi-gene modification of M. ruber M7 due to the limitation of resistance screening genes.
In current research, we have developed a marker recycling and highly genetic modification system, including the mutants ΔmrpyrGΔmrlig4::mrpyrG (ΔpyrG+lig4::pyrG) and ΔmrpyrGΔmrlig4 (ΔpyrG+lig4) for M. ruber M7, in which we used the endogenous gene mrpyrG (the homologous gene of OMP decarboxylase gene) in M. ruber M7 instead of the resistance marker gene as the screening marker, and simultaneously deleted mrlig4 related to the NHEJ. Then, the morphologies, growth rates, the production of MPs and CIT of the mutants were determined. Finally, we applied the system to delete multiple genes including mrpigG, mrpigH, and mrpigI relative to MPs biosynthesis in M. ruber M7 (Chen et al., 2017) separately or continuously without any resistance marker gene, and found that the average GRF of ΔpyrG+lig4 was about 18 times of that of M. ruber M7, which shows that the system is suitable for multiple genes modification of Monascus spp..
Materials and methods
Fungal strains, culture media and growth conditions
M. ruber M7 [CCAM 070120, Culture Collection of State Key Laboratory of Agricultural Microbiology, which is part of China Center for Type Culture Collection (CCTCC), Wuhan, China] (Chen and Hu, 2005), the model microorganism in our lab, was used as a DNA donor and for transformation (Shao et al., 2009). All the strains used in this study are described in Table 1. All the strains are maintained on PDA slants with/without 10 mmol/ml uridine and 0.75 mg/ml 5-FOA at 28°C (Thai et al., 2021).
Cloning and analysis of the pyrG gene
Amino acid sequences encoded by mrpyrG were predicted using SoftBerry's FGENESH program (http://www.softberry.com), and the mrpyrG functional regions were analyzed using the Pfam 33.1 program (http://pfam.xfam.org/). Homology of the deduced amino acid sequence was analyzed using the BlastP program on the NCBI website (http://blast.ncbi.nlm.nih.gov/Blast.cgi).
Deletion of the mrpyrG and mrlig4 genes
To construct a markerlessly and highly efficient genetic modification system, the genes of mrpyrG and mrlig4 were deleted according to the homologous recombination strategy as described previously (Liu et al., 2014). Genomic DNA of M. ruber M7 was extracted according to previous description (Shao et al., 2009) for amplification of the entire mrpyrG and mrlig4 gene sequences and their 5′- and 3′-flanking regions. Using these amplified DNA sequences, the mrpyrG deletion cassette was constructed by double-joint PCR (Yu et al., 2004), and the mrlig4 cassette was constructed using the Seamless Cloning and assembly kit (Li et al., 2021). Then, these two cassettes were digested separately by Kpn I/Xba I, Hind III/Kpn I, and then ligated with pCAMBIA3300 vector digested with the same restricted enzymes to form recombinant vectors respectively, which were transformed into Agrobacterium tumefaciens EHA105 cells that were used to introduce the constructed cassettes region into the hosts (Shao et al., 2009). The construction procedure is showed in Figure 1 (Li et al., 2021). The relative primer pairs are shown in Table 2.
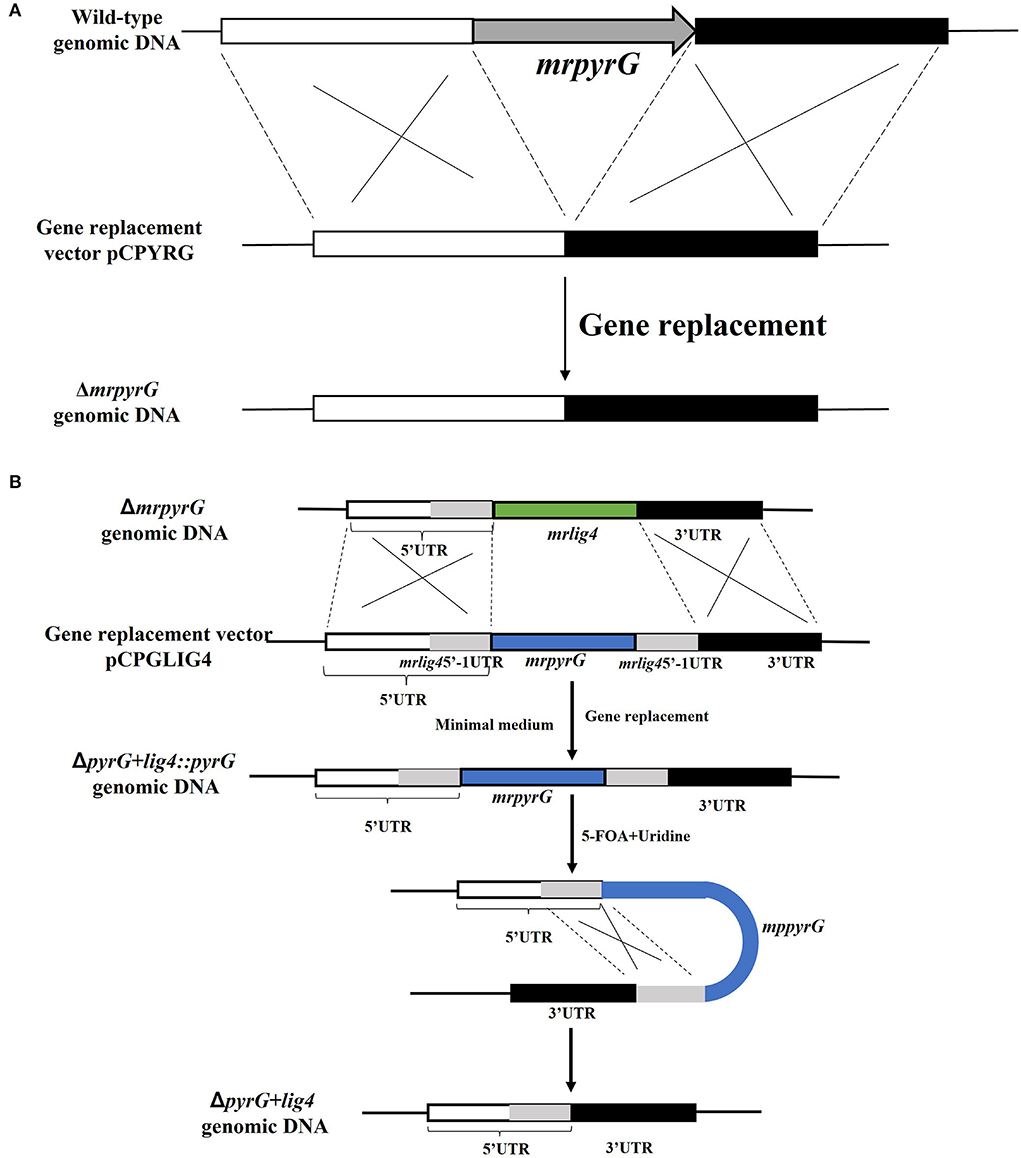
Figure 1. Deletion of mrpyrG and mrlig4 in M. ruber M7. (A) Strategy to construct mrpyrG markerless deletion strain. (B) Strategy to construct mrlig4 markerless deletion strain.
Excision of the mrpyrG marker by using 5-FOA
Since the U auxotrophs were resistant to 5-FOA in M. ruber M7, positive selection for ΔmrpyrG strains was carried out using 5-FOA. It was expected that the pyrG inserted at the lig4 locus would be excised out by homologous recombination with the direct repeats, in which the flanking regions of the lig4 were directly connected without leaving any ectopic/foreign DNA fragments (Figure 1B). Conidia of the ΔpyrG+lig4::pyrG strains(105 cfu/ml) were spread onto the agar medium containing 5-FOA and U after 5–8 day cultivation and the resulting 5-FOA resistant strains exhibited U auxotrophy.
Analysis of phenotypic characterization and biomass
M. ruber M7, ΔmrpyrG, ΔpyrG+lig4::pyrG, ΔpyrG+lig4, were, respectively, inoculated on PDA, PDA+U, PDA+U+5-FOA plates for 5 days at 28°C to observe the colonial and microscopic morphologies (Huang et al., 2016).
Biomass was determined according to the published paper (Lai et al., 2011) with minor modification. One milliliter freshly harvested spore (105 cfu/ml) of each strain was inoculated on PDA and PDA+U plates covered with cellophane membranes, and incubated at 28°C for 11 days, the samples were taken every 2 days from the 3rd day to the 11th day of culture. Then, these samples were vacuum freeze-dried and weighed.
Detection of citrinin and Monascus pigments
In total, 1 ml freshly harvested spore (105 cfu/ml) of aforementioned strains was inoculated on PDA and PDA+U plates covered with cellophane membranes, and incubated at 28°C for 11 days, respectively, to detect the intracellular MPs and extracellular CIT.40 mg freeze-dried media powder was extracted by 1 mL 80% (v/v) methanol, and subjected to 30 min ultrasonication treatment to detect the citrinin content by UPLC (Waters, America) with previous method (Liu et al., 2019), and injection volume was 2 μL. And 20 mg freeze-dried mycelia was extracted by 1 mL 80%(v/v) methanol, and subjected to 30 min ultrasonication treatment. Dilute the methanol extract to an appropriate amount, use 80% (v/v) methanol as the control (CK), and measure the absorbance at 380 nm, 470 nm, and 520 nm by the ultraviolet-visible spectrophotometry. The absorbance value multiplied by the dilution factor is the color value of yellow, orange, and red MPs, respectively. The final contents of Monascus pigments and citrinin were expressed as U/mg and μg/mg, respectively.
Results
Construction of genetic modification system with markerless and highly efficient system
Sequence analysis of mrpyrG in M. ruber M7
Sequence prediction of mrpyrG by SoftBerry's FGENESH program has revealed that the putative mrpyrG gene consists only of an 828 bp open reading frame (ORF) which consists of 2 exon and encodes 275 amino acids. A database search with NCBI-Blastp has demonstrated that the deduced 275-amino acid sequence encoded by mryrG shares 100% similarity with the amino orotidine-5′-phosphate decarboxylase of M. aurantiacus (GenBank: ADE43948.1), 81.39% similarity with PyrG of Penicillium chrysogenum (GenBank: XP-002 558877.1). Besides, prediction of Pfam has indicated that MrpyrG belongs to the DRE–TIM metallolyase superfamily.
Verification of the ΔmrpyrG, ΔpyrG+lig4::pyrG, ΔpyrG+lig4 strains
Through genetic transformation mediated by Agrobacterium tumefaciens, 2 putative mrpyrG mutants (ΔmrpyrG), 2 putative mutants (ΔpyrG+lig4::pyrG), and 1 ΔpyrG+lig4 mutant were obtained, respectively. The PCR verification results of these mutants are shown in Figure 2.
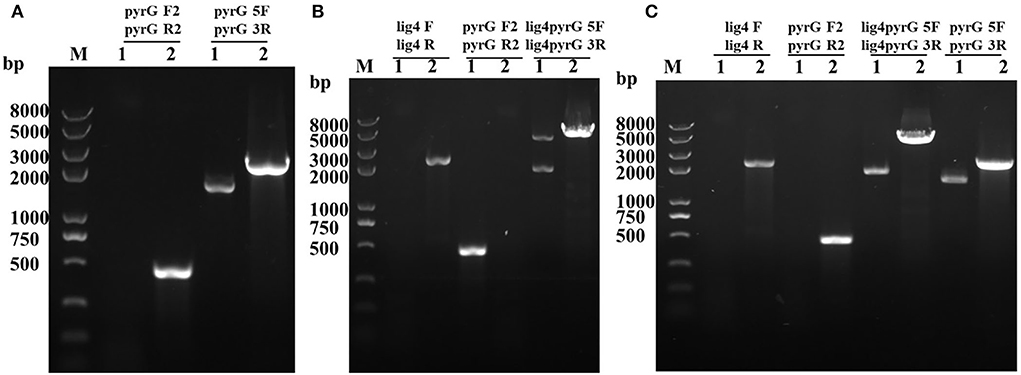
Figure 2. PCR analysis of ΔmrpyrG, ΔpyrG+lig4::pyrG, and ΔpyrG+lig4. (A) Confirmation of mrpyrG deletion. Lane 1: ΔmrpyrG; Lane 2: M7; M: Trans 2K plus II marker; (B) Confirmation of mrlig4 markerless deletion in ΔmrpyrG strain. Lane 1: ΔpyrG+lig4::pyrG; Lane 2: ΔmrpyrG; (C) Confirmation of mrpyrG homologous recombination events in ΔpyrG+lig4::pyrG strain. Lane 1: ΔpyrG+lig4; Lane 2: M7; M: Trans 2K plus II marker.
The results from Figure 2A reveal that no DNA band was amplified when the genome of the putative ΔmrpyrG strain was used as template with the primer pair pyrG-F2/pyrG-R2 (Table 2). Meanwhile, amplicons of M. ruber M7 (1.73 kb) and ΔmrpyrG (2.3 kb) different in sizes were observed when primers pyrG5F/pyrG3R (Table 2) were used. The results from Figure 2B show that no DNA band was amplified when the genome of the putative ΔpyrG+lig4::pyrG strain was used as template with the primer pair lig4F/lig4R (Table 2), while a 2.2 kb product appeared using the genome of the ΔmrpyrG strain. Meanwhile, amplicons of ΔpyrG+lig4::pyrG (3.8 kb) and ΔmrpyrG (4.37 kb) different in sizes were observed when primers lig4pyrG5F/lig4pyrG5R (Table 2) were used. The 2.1 kb band in Lane 1 of Figure 2B generated by the primer lig4pyrG5F/lig4pyrG5R may be the homogenous sequence of 5′UTR and 5′-1UTR of the mrlig4 knockout cassette. The results from Figure 2C displays that no DNA band was amplified when the genome of the putative ΔpyrG+lig4 strain was used as DNA template with the primer pair lig4F/lig4R and pyrGF2/pyrGR2, while a 2.2 kb product and a 0.5 kb product appeared, respectively, using the genome of the ΔpyrG+lig4::pyrG strain. Meanwhile, amplicons of M. ruber M7 (4.37kb) and ΔpyrG+lig4 (2.1 kb) different in sizes were observed when primers lig4pyrG5F/lig4pyrG3R (Table 2) were used. Besides, amplicons of M. ruber M7 (1.73 kb) and ΔpyrG+lig4 (2.3 kb) different in sizes were observed when primers pyrG5F/pyrG3R were used. These PCR results demonstrate that all the mutants are successfully constructed.
Characteristics of M. ruber M7, ΔmrpyrG, ΔpyrG+lig4::pyrG and ΔpyrG+lig4
Morphologies and biomasses of M. ruber M7, ΔmrpyrG, ΔpyrG+lig4::pyrG and ΔpyrG+lig4
M. ruber M7, ΔmrpyrG, ΔpyrG+lig4::pyrG and ΔpyrG+lig4 strains were cultured for 5d at 28°C to observe colonial morphologies on PDA, PDA+U, and PDA+U+ 5-FOA plates. At the same time, these strains were cultured for 7 d at 28°C to observe microscopic morphologies on PDA and PDA+U plates.
It can be seen from Figure 3I that M. ruber M7 with mrpyrG can synthesize U and transform 5-FOA into the toxic compound 5-fluorouracil, so it can grow on PDA plate but not on PDA+5-FOA plate. U auxotrophic strains (ΔmrpyrG and ΔpyrG+lig4) cannot synthesize U and cannot transform 5-FOA into 5-fluorouracil, and cannot grow on PDA plate, but can grow on PDA+U and PDA+U+5-FOA plates. The colonial morphologies of U prototrophic strain (ΔpyrG+lig4::pyrG) on PDA, PDA+U, and PDA+U+5-FOA plates is consistent with M. ruber M7. These results once again show that the construction of each mutant is right. Meanwhile, the microscopic results in Figure 3II shows that mrpyrG-deficient strains (ΔpyrG and ΔpyrG+lig4) have normal mycelia, cleistothecia, and conidia on PDA+U plate, which are no different from M. ruber M7. At the same time, the U prototrophic strain (ΔpyrG+lig4::pyrG) also normal mycelia, cleistothecia and conidia on PDA and PDA+U plates, which is also no difference from M. ruber M7. Moreover, the biomass of each mutant on PDA and/or PDA+U plate is not obviously different from that of M. ruber M7 (Figure 3III).
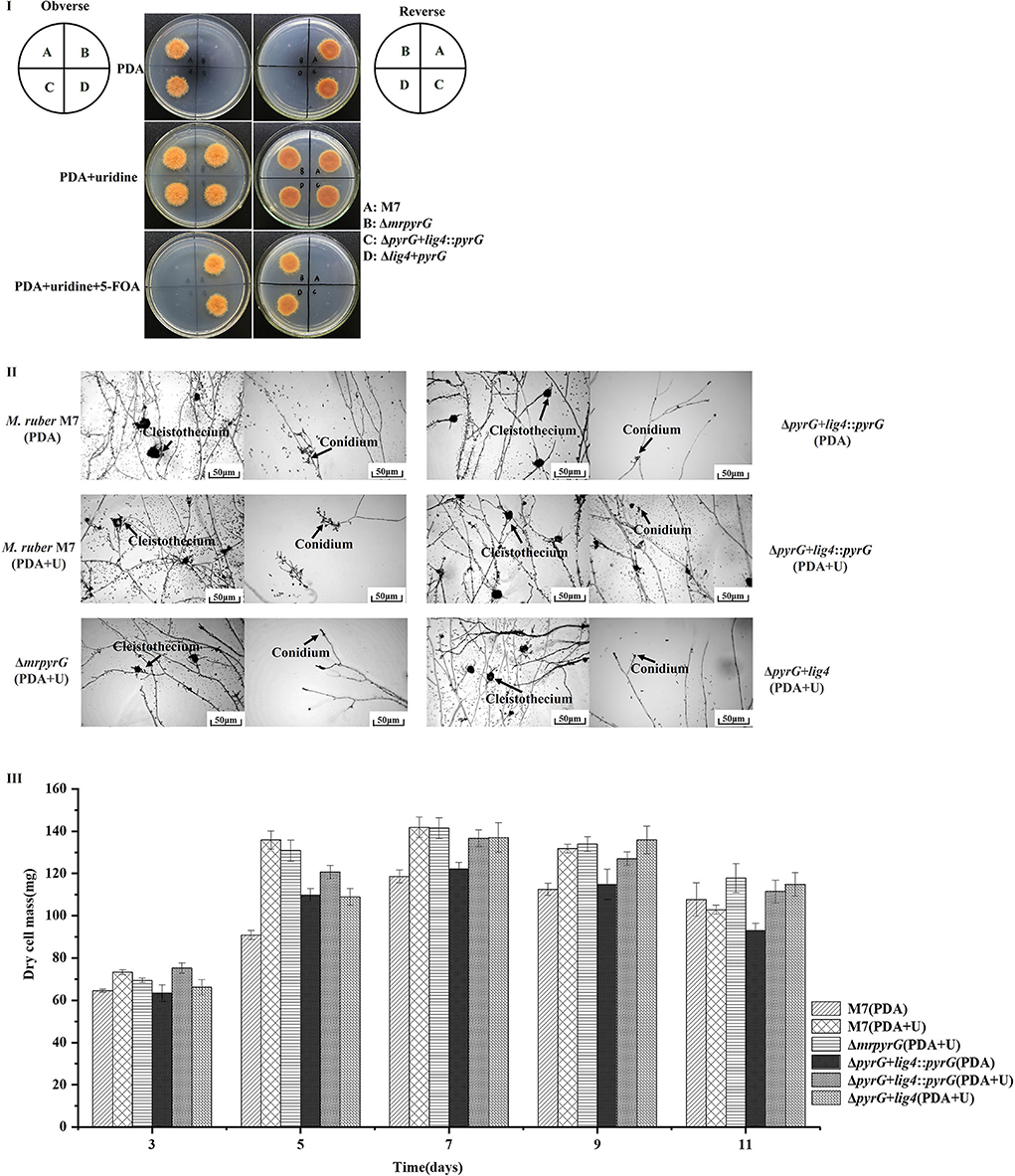
Figure 3. Morphologies and biomasses of M. ruber M7 and derivative markerless deletion strains. (I) Colonial morphologies on PDA, PDA+U and PDA+U+ 5-FOA plates. (II) Cleistothecia and conidia formation on PDA and PDA+U plates. (III) Biomass (dry cell weight).
MPs and CIT production analysis of ΔmrpyrG, ΔpyrG+lig4::pyrG, ΔpyrG+lig4 and M. ruber M7
Previous studies (Chen et al., 2017) have demonstrated that M. ruber M7 can produce MPs and CIT, but no MK, so the yields of MPs and CIT produced by M. ruber M7 and its mutants, were analyzed to uncover the effect of mrpyrG on them (Figure 4).
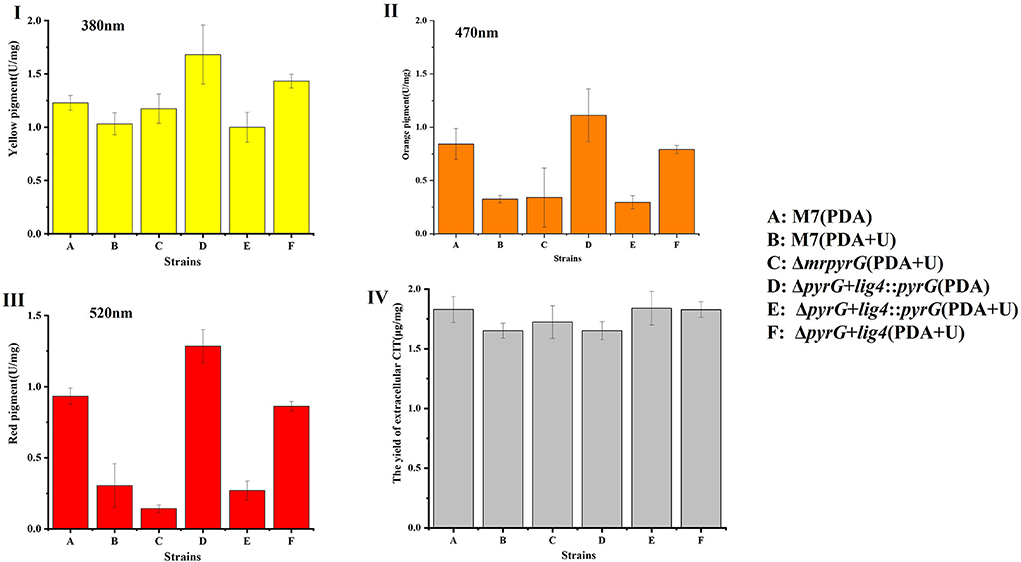
Figure 4. Production of intracellular MPs and extracellular CIT by M. ruber M7 and derivative markerless deletion strains on the PDA supplied with/without uridine. (I) The yield of yellow pigments; (II) The yield of orange pigments; (III) The yield of red pigments; and (IV) The yield of CIT.
Compared with M. ruber M7, the yellow pigment production of all the mutant strains changed to some extent, but it showed an irregular trend (Figure 4I). And for the U auxotrophic strains ΔmrpyrG and ΔpyrG+lig4, the addition of U affected the production of orange and red pigments to a certain extent (Figures 4II,III). In addition, it can be found that the U addition also has a certain effect on the orange and red pigments yield of M. ruber M7.
With regard to CIT, the results (Figure 4IV) show that CIT produced by ΔmrpyrG, ΔpyrG+lig4::pyrG and ΔpyrG+lig4 was not apparently different from those of M. ruber M7 on different media, which indicates that mrpyrG and mrlig4 have no effect on the CIT production.
Application of gene markerless and highly efficient modification system
Taking M. ruber M7 and ΔpyrG+lig4 as the starting strains to knock out mrpigG and mrpigI in the MPs gene cluster of M. ruber M7 (Chen et al., 2017), respectively, and calculate the number of transformants and the number of disruptants (knockouts) via PCR verification with related primers shown in Table 2 from both starting strains. And the GRFs, referred as the number of disruptants divided by the number of transformants, are shown in Table 3. Furthermore, taking ΔpyrG+lig4+pigG as a starting strain, mrpigH and mrpigI genes were continuously deleted, and successfully got the multi-gene mutants ΔpyrG+lig4+pigG +pigH+pigI, and ΔpyrG+lig4+pigG+pigH+ pigI::pyrG. As shown in Table 3, when mrpigG was knocked out using the ΔpyrG+lig4 strain as the starting strain, the GRF reached to 46.7% (21/45), while using M. ruber M7 as the starting strain, the GRF of pigG was only 2.6% (3/115). Meanwhile, when mrpigI was knocked out, the GRF was 44.4% (4/9) in the ΔpyrG+lig4 strain and 2.4% (2/85) in M. ruber M7. In general, the average GRFs for mrpigG and mrpigI in ΔpyrG+lig4 was about 18 times of that of M. ruber M7.
Discussion
In 2008, Maruyama and Kitamoto first described that the multiple gene disruptions with marker recycling were done in the highly efficient gene-targeting background in filamentous fungi (Maruyama and Kitamoto, 2008). They generated a ligD(lig4)-disruptant for highly efficient gene-disruption frequency in A. oryzae., then two proteinase genes(tppA and pepE) were disrupted continuously at very high frequency (~90%) in ΔligD strain with pyrG as the screening marker. After that, pyrG has been successfully applied to Aspergillus terreus (Huang et al., 2016) and Aspergillus niger (Arentshorst et al., 2015) as a selection marker. But, there are no related reports in Monascus spp.. In recent years, genes involved in the biosynthesis of citrinin, monacolin K (MK), and pigments, and G protein signaling pathway (Sakai et al., 2009; Li, 2011; Shao et al., 2014; Chen et al., 2017, 2019) have been cloned and analyzed, which made an important step forward in understanding the secondary metabolism in Monascus spp.. However, because of the limitation of resistance selection marker genes, multiple-gene editing cannot be performed in the same strain. In this study, based on the issues that there are limited antibiotic screening marker genes available for Monascus gene modification and the low GRF (He et al., 2014), mrpyrG and mrlig4 genes from M. ruber M7 were knocked out in sequence, leading that a so-called markerlessly and highly efficient gene modification system was successfully constructed without any antibiotic screening marker gene, in which GRF is about 18 times higher than that of M. ruber M7. And single or multiple gene(s) related with MPs of M. ruber M7 was (were) deleted by this gene modification system.
Although the colonial and microscopic morphologies, biomasses (Figures 3I,III) and CIT production (Figure 4IV) of auxotrophic strains (ΔmrpyrG and ΔpyrG+lig4) and U prototrophic strain (ΔpyrG+lig4::pyrG) on PDA, PDA+U, and PDA+U+5-FOA plates were consistent with those of M. ruber M7, U addition could obviously affected the production of orange and red pigments of the U prototrophy strains and some U auxotrophy strains (ΔmrpyrG) (Figures 4I,III). Therefore, when the gene markerless modification system constructed in this study is used to investigate the gene(s) function(s) from Monascus spp., especially the function(s) of related gene(s) in the MPs gene cluster, it is necessary to re-introduce pyrG into the mutants without pyrG to avoid the extra addition of U. And why U addition has an effect on the production of the orange and red pigments needs to further be explored.
When the ΔpyrG+lig4 strain from M. ruber M7 was taken as the starting strain, its GRFs for mrpigG and mrpigI reached 46.7 and 44.4%, respectively, due to mrlig4 loss, which is much (18 times) higher than those of M. ruber M7 used as the starting strain (Table 3). However, the positive effect of the mrlig4 mutant of M. ruber M7 on the GRF did not reach the level observed in some other fungi, which GRFs of almost 100% were obtained (Ishibashi et al., 2006; Bugeja et al., 2012). There are also studies that showed inactivation of the ku70 or ku80 genes involved in the NHEJ DNA repair pathway can greatly increase GRFs of filamentous fungi (Zhang et al., 2011; He et al., 2013). Therefore, in the future the GRFs may be further improved if the relative genes, such as mrku70 or/and mrku80, with NHEJ pathway, is/are knocked out. Moreover, the characteristics of the multi-gene mutants ΔpyrG+lig4+pigG+pigH+pigI and ΔpyrG+lig4+pigG+pigH+pigI::pyrG should be investigated, too.
In this study, we construct a markerlessly and highly efficient gene modification system successfully to knock out endogenous mrpyrG and mrlig4 gene without introducing any antibiotic screening marker gene, in which GRF is about 18 times higher than that of M. ruber M7. Besides, we have successfully applied this system to multiple-gene knock out in Monascus spp.. However, in our study, we also found that the U addition can make an effect on the yield of MPs, and the mechanism is not clear, which requires our further study.
Data availability statement
The original contributions presented in the study are included in the article/supplementary materials, further inquiries can be directed to the corresponding author/s.
Author contributions
NX and LL conceived, designed, and did research. NX wrote the manuscript, too. FC revised the manuscript. All authors read and approved the manuscript.
Funding
This work was supported by the National Natural Science Foundation of China (Nos. 31730068 and 31330059).
Acknowledgments
Funding from the National Natural Science Foundation of China (Nos. 31730068 and 31330059) is gratefully acknowledged.
Conflict of interest
The authors declare that the research was conducted in the absence of any commercial or financial relationships that could be construed as a potential conflict of interest.
Publisher's note
All claims expressed in this article are solely those of the authors and do not necessarily represent those of their affiliated organizations, or those of the publisher, the editors and the reviewers. Any product that may be evaluated in this article, or claim that may be made by its manufacturer, is not guaranteed or endorsed by the publisher.
References
Arentshorst, M., Lagendijk, E. L., and Ram, A. F. (2015). A new vector for efficient gene targeting to the pyrG locus in Aspergillus. niger. Fungal. Biol Biotechnol. 2, 2. doi: 10.1186/s40694-015-0012-4
Blanc, P. J., Laussac, J. P., Le Bars, J., Le Bars, P., Loret, M. O., Pareilleux, A., et al. (1995). Characterization of monascidin A from Monascus as citrinin. Int. J. Food. Microbiol 27, 201–213. doi: 10.1016/0168-1605(94)00167-5
Bugeja, H. E., Boyce, K. J., Weerasinghe, H., Beard, S., Jeziorowski, A., Pasricha, S., et al. (2012). Tools for high efficiency genetic manipulation of the human pathogen Penicillium marneffei Fungal. Genet. Biol. 49, 772–778. doi: 10.1016/j.fgb.2012.08.003
Caroline, D. F., and Davis, R. H. (1969). Pyrimidine synthesis in Neurospora. crassa: Regulation of enzyme activities. J. Bacteriol. 100, 1378–1384. doi: 10.1128/jb.100.3.1378-1384.1969
Chen, F., and Hu, X. (2005). Study on red fermented rice with high concentration of monacolin K and low concentration of citrinin. Int. J. Food. Microbiol. 103, 331–337. doi: 10.1016/j.ijfoodmicro.2005.03.002
Chen, W., Chen, R., Liu, Q., He, Y., He, K., Ding, X., et al. (2017). Orange, red, yellow: Biosynthesis of azaphilone pigments in Monascus fungi. Chem. Sci. 8, 4917–4925. doi: 10.1039/C7SC00475C
Chen, W., Feng, Y., Molnar, I., and Chen, F. (2019). Nature and nurture: confluence of pathway determinism with metabolic and chemical serendipity diversifies Monascus azaphilone pigments. Nat. Prod. Rep. 36, 561–572. doi: 10.1039/C8NP00060C
Cui, H., and Li, Y. (2012). Transformation of Monascus protoplasts using pyridine thiamin resistance gene as selective marker. J. Anhui. Agri. 40, 11591–11593.
de Carvalho, J. C., Pandey, A., Oishi, B. O., Brand, D., Rodriguez-Léon, J. A., Soccol, C. R., et al. (2006). Relation between growth, respirometric analysis and biopigments production from Monascus by solid-state fermentation. Biochem. Eng. J. 29, 262–269. doi: 10.1016/j.bej.2006.01.008
Endo, A (1979). Monacolin K, a new hypocholesterolemic agent produced by a Monascus species. J. Antibiot. 32, 852–854. doi: 10.7164/antibiotics.32.852
Feng, Y., Shao, Y., and Chen, F. (2012). Monascus pigments. Appl. Microbiol. Biotechnol. 96, 1421–1440. doi: 10.1007/s00253-012-4504-3
Feng, Y., Shao, Y., Zhou, Y., and Chen, F. (2014). Production and optimization of monacolin K by citrinin-free Monascus pilosus MS-1 in solid-state fermentation using non-glutinous rice and soybean flours as substrate. Eur. Food. Res. Technol. 239, 629–636. doi: 10.1007/s00217-014-2259-z
Garavaglia, M., Rossi, E., and Landini, P. (2012). The pyrimidine nucleotide biosynthetic pathway modulates production of biofilm determinants in Escherichia. coli. PLoS ONE 7, e31252. doi: 10.1371/journal.pone.0031252
Hajjaj, H., Francois, J. M., Goma, G., and Blanc, P. J. (2012). Effect of amino acids on red pigments and citrinin production in Monascus ruber. J. Food Sci. 77, M156–9. doi: 10.1111/j.1750-3841.2011.02579.x
He, Y., Liu, Q., Shao, Y., and Chen, F. (2013). Ku70 and ku80 null mutants improve the gene targeting frequency in Monascus ruber M7. Appl. Microbiol. Biotechnol. 97, 4965–76. doi: 10.1007/s00253-013-4851-8
He, Y., Shao, Y., and Chen, F. (2014). Efficient gene targeting in ligase. IV-deficient Monascus ruber M7 by perturbing the non-homologous end joining pathway. Fungal. Biol. 118, 846–854. doi: 10.1016/j.funbio.2014.07.003
Huang, X., Chen, M., Li, J., and Lu, X. (2016). Establishing an efficient gene-targeting system in an itaconic-acid producing Aspergillus terreus strain. Biotechnol. Lett. 38, 1603–1610. doi: 10.1007/s10529-016-2143-y
Ishibashi, K., Suzuki, K., Ando, Y., Takakura, C., and Inoue, H. (2006). Nonhomologous chromosomal integration of foreign DNA is completely dependent on MUS-53 (human Lig4 homolog) in Neurospora. Proc. Natl. Acad. Sci. U.S.A. 103, 14871–14876. doi: 10.1073/pnas.0604477103
Lai, Y., Wang, L., Qing, L., and Chen, F. (2011). Effects of cyclic AMP on development and secondary metabolites of. Monascus ruber M7. Lett. Appl. Microbiol. 52, 420–426. doi: 10.1111/j.1472-765X.2011.03022.x
Li, L (2011). Cloning and functional analysis of genes of G-protein signaling pathways in Monascus ruber (Dissertation). Huazhong Agricultural University, Wuhan, China.
Li, L., and Chen, F. (2020). Effects of mrpigG on development and secondary metabolism of Monascus ruber M7. J. Fungi (Basel). 6, 156. doi: 10.3390/jof6030156
Li, L., Xu, N., and Chen, F. (2021). Inactivation of mrpigH gene in Monascus ruber M7 results in increased Monascus pigments and decreased citrinin with mrpyrG selection marker. J. Fungi. 7, 1094. doi: 10.3390/jof7121094
Lin, Y. L., Wang, T. H., Lee, M. H., and Su, N. W. (2008). Biologically active components and nutraceuticals in the Monascus-fermented rice: a review. Appl. Microbiol. Biotechnol. 77, 965–973. doi: 10.1007/s00253-007-1256-6
Liu, J., Lei, M., Zhou, Y., and Chen, F. (2019). A comprehensive analysis of the small GTPases Ypt7 involved in the regulation of fungal development and secondary metabolism in Monascus ruber M7. Front. Microbiol. 10, 452. doi: 10.3389/fmicb.2019.00452
Liu, J., Wu, J., Cai, X., Zhang, S., Liang, Y., Lin, Q., et al. (2021). Regulation of secondary metabolite biosynthesis in Monascus purpureus via cofactor metabolic engineering strategies. Food. Microbiol. 95, 103689. doi: 10.1016/j.fm.2020.103689
Liu, M., Rehman, S., Tang, X., Gu, K., Fan, Q., Chen, D., et al. (2018). Methodologies for Improving HDR Efficiency. Front. Genet 9, 691. doi: 10.3389/fgene.2018.00691
Liu, Q., Cai, L., Shao, Y., Zhou, Y., Li, M., Wang, X., et al. (2016). Inactivation of the global regulator LaeA in Monascus ruber results in a species-dependent response in sporulation and secondary metabolism. Fungal. Bio. 120, 297–305. doi: 10.1016/j.funbio.2015.10.008
Liu, Q., Xie, N., He, Y., Wang, L., Shao, Y., Zhao, H., et al. (2014). MpigE, a gene involved in pigment biosynthesis in Monascus ruber M7. Appl. Microbiol. Biotechnol. 98, 285–296. doi: 10.1007/s00253-013-5289-8
Maruyama, J., and Kitamoto, K. (2008). Multiple gene disruptions by marker recycling with highly efficient gene-targeting background (ΔligD) in Aspergillus oryzae. Biotechnol. Lett. 30, 1811–1817. doi: 10.1007/s10529-008-9763-9
Nguyen, K. T., Ho, Q. N., Pham, T. H., Phan, T. N., and Tran, V. T. (2016). The construction and use of versatile binary vectors carrying. pyrG auxotrophic marker and fluorescent reporter genes for Agrobacterium-mediated transformation of. Aspergillus. oryzae. World. J. Microbiol. Biotechnol. 32, 204. doi: 10.1007/s11274-016-2168-3
Pannunzio, N. R., Watanabe, G., and Lieber, M. R. (2018). Nonhomologous DNA end-joining for repair of DNA double-strand breaks. J. Biol. Chem. 293, 10512–10523. doi: 10.1074/jbc.TM117.000374
Patakova, P (2013). Monascus secondary metabolites: Production and biological activity. J. Ind. Microbiol. Biotechnol. 40, 169–181. doi: 10.1007/s10295-012-1216-8
Sakai, K., Kinoshita, H., and Nihira, T. (2009). Identification of mokB involved in monacolin K biosynthesis in Monascus pilosus. Biotechnol. Lett. 31, 1911–1916. doi: 10.1007/s10529-009-0093-3
Shao, Y., Ding, Y., Zhao, Y., Yang, S., Xie, B., Chen, F., et al. (2009). Characteristic analysis of transformants in T-DNA mutation library of. Monascus ruber. World. J. Microbiol. Biotechnol. 25, 989–995. doi: 10.1007/s11274-009-9977-6
Shao, Y., Lei, M., Mao, Z., Zhou, Y., and Chen, F. (2014). Insights into Monascus biology at the genetic level. Appl Microbiol. Biotechnol. 98, 3911–3922. doi: 10.1007/s00253-014-5608-8
Shimizu, T., Kinoshita, H., and Nihira, T. (2006). Development of transformation system in Monascus purpureus using an autonomous replication vector with aureobasidin A resistance gene. Biotechnol. Lett 28, 115–120. doi: 10.1007/s10529-005-4956-y
Shrivastav, M., De Haro, L. P., and Nickoloff, J. A. (2008). Regulation of DNA double-strand break repair pathway choice. Cell. Res. 18, 134–147. doi: 10.1038/cr.2007.111
Symington, L. S., and Gautier, J. (2011). Double-strand break end resection and repair pathway choice. Annu. Rev. Genet. 45, 247–271. doi: 10.1146/annurev-genet-110410-132435
Thai, H. D., Nguyen, B. T., Nguyen, V. M., Nguyen, Q. H., and Tran, V. T. (2021). Development of a new Agrobacterium-mediated transformation system based on a dual auxotrophic approach in the filamentous fungus Aspergillus oryzae. World. J. Microbiol. Biotechnol. 37, 92. doi: 10.1007/s11274-021-03060-z
Tuteja, N., Verma, S., Sahoo, R. K., Raveendar, S., and Reddy, I. N. (2012). Recent advances in development of marker-free transgenic plants: regulate and biosafety concern. J. Biosci. 37, 167–197. doi: 10.1007/s12038-012-9187-5
Wang, B. H., Xu, Y., and Li, Y. P. (2010). Use of the pyrG gene as a food-grade selection marker in Monascus. Biotechnol. Lett. 32, 1631–1635. doi: 10.1007/s10529-010-0336-3
Wu, M. D., Cheng, M. J., Yech, Y. J., Chen, Y. L., Chen, K. P., Yang, P. H., et al. (2013). Monascusazaphilones A-C, three new azaphilone analogues isolated from the fungus Monascus purpureus BCRC 38108. Nat. Prod. Res. 27, 1145–1152. doi: 10.1080/14786419.2012.715289
Yang, F., Njire, M. M., Liu, J., Wu, T., Wang, B., Liu, T., et al. (2015). Engineering more stable, selectable marker-free autoluminescent mycobacteria by one step. PLoS ONE. 10, e0119341. doi: 10.1371/journal.pone.0119341
Ying, S. H., Feng, M. G., and Keyhani, N. O. (2013). Use of uridine auxotrophy (ura3) for markerless transformation of the mycoinsecticide Beauveria bassiana. Appl Microbiol. Biotechnol. 97, 3017–3025. doi: 10.1007/s00253-012-4426-0
Yu, J. H., Hamari, Z., Han, K. H., Seo, J. A., Reyes-Dominguez, Y., Scazzocchio, C., et al. (2004). Double-joint PCR: A PCR-based molecular tool for gene manipulations in filamentous fungi. Fungal. Genet. Biol. 41, 973–981. doi: 10.1016/j.fgb.2004.08.001
Zhang, C., Liang, J., Zhang, A., Hao, S., Zhang, H., Zhu, Q., et al. (2019). Overexpression of monacolin K biosynthesis genes in the. Monascus purpureus azaphilone polyketide pathway. J. Agric. Food. Chem. 67, 2563–2569. doi: 10.1021/acs.jafc.8b05524
Zhang, J., Mao, Z., Xue, W., Li, Y., Tang, G., Wang, A., et al. (2011). Ku80 gene is related to non-homologous end-joining and genome stability in Aspergillus niger. Curr Microbiol. 62, 1342–1346. doi: 10.1007/s00284-010-9853-5
Zhang, L., Zheng, X., Cairns, T. C., Zhang, Z., Wang, D., Zheng, P., et al. (2020). Disruption or reduced expression of the orotidine-5'-decarboxylase gene pyrG increases citric acid production: A new discovery during recyclable genome editing in Aspergillus niger. Microb. Cell. Fac. 19, 76. doi: 10.1186/s12934-020-01334-z
Keywords: Monascus ruber M7, genetic modification system, resistance selection marker, mrlig4, mrpyrG
Citation: Xu N, Li L and Chen F (2022) Construction of gene modification system with highly efficient and markerless for Monascus ruber M7. Front. Microbiol. 13:952323. doi: 10.3389/fmicb.2022.952323
Received: 25 May 2022; Accepted: 27 June 2022;
Published: 01 August 2022.
Edited by:
Xucong Lv, Fuzhou University, ChinaReviewed by:
Zhilong Wang, Shanghai Jiao Tong University, ChinaZhenqiang Wu, South China University of Technology, China
Copyright © 2022 Xu, Li and Chen. This is an open-access article distributed under the terms of the Creative Commons Attribution License (CC BY). The use, distribution or reproduction in other forums is permitted, provided the original author(s) and the copyright owner(s) are credited and that the original publication in this journal is cited, in accordance with accepted academic practice. No use, distribution or reproduction is permitted which does not comply with these terms.
*Correspondence: Fusheng Chen, Y2hlbmZzQG1haWwuaHphdS5lZHUuY24=
†These authors have contributed equally to this work and share first authorship