- 1Department of Bacteriology, Graduate School of Medicine, Gunma University, Maebashi, Gunma, Japan
- 2Department of Infectious Diseases and Host Defense, Graduate School of Medicine, Gunma University, Maebashi, Gunma, Japan
- 3Department of Frontier Bioscience and Research Center for Micro-Nano Technology, Hosei University, Tokyo, Japan
- 4Laboratory of Bacterial Drug Resistance, Graduate School of Medicine, Gunma University, Maebashi, Gunma, Japan
Uropathogenic Escherichia coli (UPEC) is a major causative agent of urinary tract infections. The bacteria internalize into the uroepithelial cells, where aggregate and form microcolonies. UPEC fimbriae and flagella are important for the formation of microcolonies in uroepithelial cells. PapB/FocB family proteins are small DNA-binding transcriptional regulators consisting of approximately 100 amino acids that have been reported to regulate the expression of various fimbriae, including P, F1C, and type 1 fimbriae, and adhesins. In this study, we show that TosR, a member of the PapB/FocB family is the activator of flagellar expression. The tosR mutant had similar expression levels of type 1, P and F1C fimbriae as the parent strain, but flagellar production was markedly lower than in the parent strain. Flagellin is a major component of flagella. The gene encoding flagellin, fliC, is transcriptionally activated by the sigma factor FliA. The fliA expression is induced by the flagellar master regulator FlhDC. The flhD and flhC genes form an operon. The promoter activity of fliC, fliA and flhD in the tosR mutant was significantly lower than in the parent strain. The purified recombinant TosR does not bind to fliC and fliA but to the upstream region of the flhD gene. TosR is known to bind to an AT-rich DNA sequence consisting of 29 nucleotides. The characteristic AT-rich sequence exists 550–578 bases upstream of the flhD gene. The DNA fragment lacking this sequence did not bind TosR. Furthermore, loss of the tosR gene reduced motility and the aggregation ability of UPEC in urothelial cells. These results indicate that TosR is a transcriptional activator that increases expression of the flhDC operon genes, contributing to flagellar expression and optimal virulence.
Introduction
Urinary tract infection (UTI) is one of the most common infectious diseases. Uropathogenic Escherichia coli (UPEC) is the most major causative agent of UTIs in the community, with more than 80% of cases caused by this organism (Zhang and Foxman, 2003; Flores-Mireles et al., 2015). Antimicrobial agents are commonly used to treat infections, however drug-resistant UPEC such as quinolone-resistant and extended-spectrum β-lactamase (ESBL)-producing strains have increased in recent years (Mathers et al., 2015; Walker et al., 2016). For these reasons, the development of treatments for UPEC infections is desirable.
UPEC invades into the urinary tract cells, where they aggregate and form biofilm-like microbial colonies termed intracellular bacterial community (IBC; Anderson et al., 2003). This ability allows UPEC to escape various antimicrobial agents and the immune system (Mulvey et al., 1998, 2001). Hence, it is believed that UPEC infections are more likely to become refractory and recurrent.
Fimbriae and flagella are major protein structures contributing to the virulence of UPEC. Fimbriae, such as type 1, P and F1C fimbriae, are required for bacterial adhesion to and invasion of host urothelial cells (Virkola et al., 1988; Martinez et al., 2000; Lillington et al., 2014). Flagella are required for bacterial migration to infection sites as the bacteria colonize the bladder and kidneys, and flagellum-mediated motility contributes to bacterial fitness and aggregation in infected urinary tract cells (Lane et al., 2005; Wright et al., 2005; Hirakawa et al., 2019). In a study using a UTI mouse model, UPEC flagella were shown to be highly expressed within infected mice (Lane et al., 2007). Another studies showed that the flagellum-deficient strain had lower ability to invade bladder and kidney epithelial cells and to form microcolonies compared to the flagellum-producing strain (Pichon et al., 2009; Hirakawa et al., 2019). In addition to fimbriae and flagella, hemolysin is another important UPEC virulence factor. This protein, a type of pore-forming toxin, contributes to the development of cystitis, pyelonephritis, and sepsis by destroying immune system cells such as macrophages in addition to urinary tract epithelial cells (Dhakal and Mulvey, 2012).
We are interested in characterizing proteins involved in the pathogenicity of UPEC including bacterial aggregation leading to IBC formation, thereby contributing to the elucidation of the pathogenesis of this organism and the development of novel therapeutic strategies. PapB and FocB are DNA-binding transcriptional regulators of small size consisting of approximately 100 amino acids. They have been reported to regulate the expression of P and F1C fimbriae (Forsman et al., 1989; Lindberg et al., 2008). We studied the tosR gene found on the pathogenicity island (PAI) of the UPEC CFT073 strain. This strain was isolated from the blood and urine of a patient with acute pyelonephritis. The tosR gene encodes the PapB/FocB family transcriptional regulator and is part of an operon that includes the tosC, tosB, and tosD genes downstream (Welch et al., 2002). The tosC, tosB, and tosD genes were originally presumed to encode a group of proteins of the type 1 secretion system that contribute to hemolysin secretion. The tosA gene, encoding a repeat-in-toxin (RTX) nonfimbrial adhesin, is present downstream of the tosRCBD operon, and TosC, TosB and TosD were shown to contribute to the secretion of TosA (Lloyd et al., 2009). TosR has been shown to contribute to the formation of Auf fimbriae and biofilm (Vigil et al., 2012; Luterbach et al., 2018). However, the function of TosR remains understudied.
This study demonstrated that the TosR protein/regulator contributes to UPEC’s ability to aggregate in bladder epithelial cells. The gene deletion mutant had lower motility and flagellar expression and exhibited lower levels of aggregation within the bladder epithelial cells. However, P and F1C fimbriae and hemolysin activity were at the same levels as in the parent strain. The purified TosR protein bound to upstream region of flhD which encodes the master regulator for flagellar expression. Thus, TosR is an activator for UPEC virulence-associated flagellar expression.
Materials and methods
Bacterial strains and culture conditions
The bacterial strains and plasmids used in this study are listed in Table 1, 2, respectively. All bacteria were grown in Luria-Bertani (LB) medium. The cell growth was monitored by absorbance at 600 nm. For marker selection and maintenance of plasmids, antibiotics were added to growth media at the following concentrations; 150 μg/ml ampicillin, 30 μg/ml chloramphenicol, and 50 μg/ml kanamycin.
Cloning and mutant constructions
An in-frame deletion mutant of tosR (CFT073∆tosR) was constructed by sequence overlap extension PCR according to a strategy described previously (Link et al., 1997), with primer pairs, delta1 / delta2 and delta3 /delta4 primers as described in Table 3. The upstream flanking DNA included 450 bp and the first eight amino acid codons. The downstream flanking DNA included the last one amino acid codon, the stop codon, and 450 bp of DNA. This deletion construct was ligated into BamHI and SalI-digested temperature sensitive vector pKO3 (Link et al., 1997) and introduced into the chromosome of CFT073 strain. Then, sucrose-resistant/chloramphenicol-sensitive colonies were selected at 30°C. To construct CFT073∆tosRCBD and CFT073∆tosCBD, the locus of tosR to tosD and tosC to tosD was deleted using primers, tosR-delta1/tosRD-delta2/tosRD-delta3/tosD-delta4 and tosC-delta1/tosCD-delta2/tosCD-delta3/tosD-delta4, respectively. To construct isopropyl β-D-thiogalactopyranoside (IPTG)-inducible tosR expression plasmids, pTrc99AtosR and pTrc99KtosR, the tosR gene was amplified with the primer pairs shown in Table 3. The product was digested with NcoI and SalI and ligated into similarly digested pTrc99A and pTrc99K plasmid (Hirakawa et al., 2003a,b), respectively. The pTH18krtosR plasmid was constructed by amplifying tosR and the 300-bp upstream region including the promoter and ligating the product into pTH18kr (Hashimoto-Gotoh et al., 2000). Since this plasmid is compatible with pTurboGFP-B, these plasmids were introduced together into the tosR mutant to characterize bacterial colonies within bladder epithelial cells using fluorescent images. To construct pNNflhD-P-Long and pNNflhD-P-Short, lacZ reporter plasmids, 769-bp and 300-bp upstream region of the flhD gene were amplified using primer pairs flhD-PFLong/flhD-PREcoRV and flhD-PFShort/flhD-PRHindIII and cloned into the pNN387 plasmid with a promoterless lacZ (Elledge and Davis, 1989), respectively. We also constructed an IPTG-inducible C-terminal octahistidine-tagged TosR expression plasmid, pET42ctosR. The tosR gene was amplified using pETtosR-F and pETtosR-R primers, and ligated into the pET42c vector digested with NdeI and HindIII. All constructs were confirmed by DNA sequencing.
Biofilm assays using 96-well plates
Levels of biofilm formation on 96-well plates were quantified as described previously with slight modifications (Hirakawa et al., 2019). Bacteria were cultured at 30°C for 24 h in LB medium without shaking. Each culture was diluted into LB medium containing 0.5% glucose at a 1:100 ratio, and 2.4 × 104 cells/well were seeded into the VIOLAMO 96-well flat bottom polystyrene plate (As ONE Corp., Osaka Japan, Catalogue number: 1–1,601-02). The plate was then incubated at 30°C for 24 h. Bacterial cells attached to the plate were stained with crystal violet and absorbance at 595 nm (A595) was measured. Bacterial aggregation ability was quantified as the A595 normalized to an OD600 of 1.
Infection of bladder epithelial cells
The ability of UPEC to invade bladder epithelial cells (HTB-9) was assessed by gentamicin protection assay as previously described (Hirakawa et al., 2019). We inoculated ~5.0 × 106 bacteria into ~5.0 × 105 host cells. The numbers of invaded bacterial cells were determined as ratios of CFU (as percentages) to total cell (invaded and non-invaded bacterial cells) CFU. We also imaged the bacteria in HTB-9 cells using confocal microscopy, as previously described (Hirakawa et al., 2019). A UPEC strain carrying a green fluorescence protein (GFP) expression plasmid, pTurboGFP-B (Evrogen, Moscow, Russia), was inoculated into cultured HTB-9 cells. The HTB-9 cells were stained with rhodamine-phalloidin (Life Technologies, Carlsbad, CA, USA). Fluorescent images were acquired on an Olympus FV10i-DOC microscope and processed using FV10-ASW software (Olympus Corp., Tokyo, Japan).
Hemagglutination assays
To estimate the activity of type 1 and P fimbriae, we tested the hemagglutination titers of guinea pig and human O-type erythrocytes, respectively, as previously described (Hirakawa et al., 2019).
Motility assays
Bacteria were statically grown overnight at 37°C. The bacterial cultures (2 μl) were spotted onto LB medium containing 0.3% agar and incubated for 9 h at 30°C.
Flagellum staining
Bacteria were cultured for 24 h at 30°C in Heart Infusion medium containing 1.5% agar. Flagella were stained with Victoria blue/tannic acid solution as previously described (Hirakawa et al., 2019).
RNA extraction and quantitative real-time PCR analyses
Bacteria were grown to the late-logarithmic growth phase (OD600 ~ 0.7) with shaking in LB medium. Total RNA extraction, cDNA synthesis and real-time PCR were performed as previously described (Hirakawa et al., 2021). Constitutively expressed rrsA and rpoD genes were used as an internal control. Primers are listed in Table 3.
Western blotting
To detect VSVG-tagged FliC from UPEC, bacteria were grown to early stationary phase and separated by centrifugation. The cell pellets were resuspended in 50 mM phosphate buffer containing 8 M urea and then lysed by sonication. Cell lysate (4.0 μg) proteins were separated on duplicate 10% acrylamide Tris-glycine SDS-PAGE gels. One gel was stained with Coomassie brilliant blue (CBB), and the other was electroblotted onto a polyvinylidene fluoride (PVDF) membrane (Bio-Rad Laboratories, Hercules, CA). VSVG-tagged FliC was detected with the Sigma anti-VSVG antibody (Merck KGaA, Darmstadt, Germany, Catalogue number: V4888) and the Sigma anti-rabbit horseradish peroxidase-conjugated immunoglobulin G (IgG) secondary antibody (Merck KGaA, Darmstadt, Germany, Catalogue number: A6154) using a SuperSignal West Pico kit (Thermo Fisher Scientific, Waltham, MA). VSVG-tagged FliC protein bands were visualized using a LAS-4010 luminescent image analyzer (GE Healthcare Japan, Tokyo).
Promoter assay
To measure promoter activities of flhD, fliA and fliC, UPEC strains carrying pNNflhD-P-Long, pNNflhD-P-Short, pNNfliA-P, or pNNfliC-P were grown at 37°C in LB medium. β-Galactosidase activities from lacZ expression in cell lysates were determined using a Tropix Galacto-Light Plus kit (Thermo Fisher Scientific, Waltham, MA, United States) as previously described (Hirakawa et al., 2018). The β-galactosidase activity was determined as the signal value normalized to an OD600 of 1.
Purification of TosR
C-terminally octahistidine-tagged TosR (TosR-His8) was purified from Escherichia coli Rosetta (DE3; Novagen, Philadelphia, PA). Bacteria containing the pET42ctosR plasmid were cultured at 37°C to logarithmic phase in LB medium, 0.5 mM IPTG was then added, and culture growth was continued at 16°C for 18 h. The cells were lysed using the BactYeastLysis reagent (ATTO, Tokyo, Japan) as previously described (Kurabayashi et al., 2016). An equal volume of purification buffer (50 mM Tris [pH 7.5], 200 mM NaCl, and 5% glycerol) was added to the lysate, and the mixture was centrifuged. The resulting supernatant was mixed with nickel-nitrilotriacetic acid (Ni-NTA) agarose (Qiagen, Valencia, CA) for 1 h. The agarose was washed three times with 10 mM imidazole and twice with 50 mM imidazole, and TosR-His8 was then eluted with 500 mM imidazole. The protein concentration was determined using a Bio-Rad protein assay (Bio-Rad, Hercules, CA).
Gel-shift assay
We used a 791-bp DNA probe containing the 769-bp region upstream of flhD. The DNA probe was prepared by PCR-amplifying with flhD-PFLong and flhD-PREcoRV primers, listed in Table 4. To generate the DNA probe lacking the TosR binding motif present upstream of flhD, flhD-PFShort and flhD-PRHindIII primers were used for PCR-amplification. This probe contains only the 300-bp region upstream of flhD. We also prepared for 321-bp DNA probes containing the 300-bp regions upstream of the fliA and fliC start codons by PCR-amplifying with primer pairs fliA-PF/fliA-PR and fliC-PF/fliC-PR, respectively. The probe fragments (0.30 pmol) were mixed with purified TosR-His8. After incubation for 20 min at room temperature, samples were separated by electrophoresis on a 5% nondenaturing acrylamide Tris-borate/EDTA (89 mM Tris-borate [pH 8.3], and 2 mM EDTA) gel in Tris-borate/EDTA buffer at 4°C. DNA bands in the gel were stained by 20,000-fold-diluted Novel Green Plus (BIO-HELIX Co., LTD., Keelung City Taiwan), and visualized under UV light at 300 nm.
Statistical analysis
The p value in each assay was determined by the unpaired t test with GraphPad Prism version 6.00.
Results
The tosR gene contributes to UPEC aggregation within uroepithelial cells
To investigate the role of TosR, we constructed a CFT073∆tosR strain lacking tosR. The tosR gene is speculated to form an operon together with the downstream tosC, tosB and tosD genes. TosC, TosB, and TosD were reported to be involved in the secretion of the adhesin TosA (Figure 1; Vigil et al., 2012). To clarify whether TosR, together with TosC, TosB, and TosD, is involved in the adhesion capacity of UPEC, we also constructed CFT073∆tosCBD strain with deletion from the start codon of tosC to the stop codon of tosD and CFT073∆tosRCBD strain with deletion from the start codon of tosR to the stop codon of tosD. As part of the evaluation of the adhesion capacity of UPEC, a static biofilm assay was performed. TosR overproduction has been reported to promote biofilm formation, however the tosR mutant formed biofilms comparable to the wild-type strain (static cultures at 37°C; Luterbach et al., 2018). In our experimental conditions (Bacteria were cultured at 30°C because our CFT073 strain poorly produced biofilms when cultured at 37°C), the tosR mutant (CFT073∆tosR) had a lower biofilm forming ability than the parent strain (CFT073). When the CFT073 parent strain, CFT073∆tosR, CFT073∆tosCBD, and CFT073∆tosRCBD were cultured on 96-well polystyrene plates for 24 h, the CFT073∆tosRCBD and CFT073∆tosR strains, but not CFT073∆tosCBD, showed approximately half the biofilm forming ability of the parent strain (Figure 2A). To confirm the contribution of the tosR gene to biofilm formation, we complemented the tosR gene to the CFT073∆tosR strain by introducing the pTrc99KtosR plasmid and examined its ability to form biofilms. The pTrc99KtosR plasmid allows tosR expression in an IPTG-inducible promoter-dependent manner. Initially, we performed the complementation experiment with 0.01 mM and 0.1 mM of IPTG. Even at a concentration of 0.01 mM IPTG, the complementation of biofilm formation capacity by the addition was observed (Figure 2B). Then, we observed that the bacterial growth in the 0.1 mM culture was noticeably lower, as indicated by the OD600 values, compared to both the non-complementary strain and the 0.01 mM culture. Due to this finding, subsequent experiments involving the pTrc99KtosR plasmid were conducted with the addition of 0.01 mM IPTG.
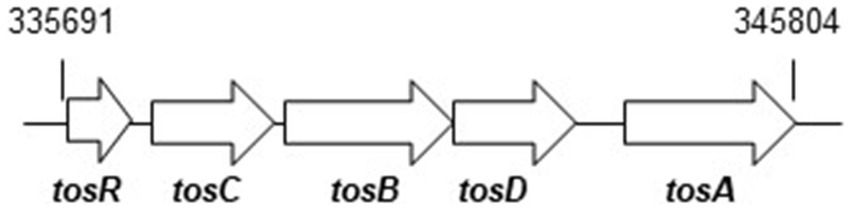
Figure 1. Locus of tosRCBDA on the UPEC CFT073 chromosome. Arrows indicate transcription/translation direction. Numbers refer to nucleotide coordinates in the UPEC CFT073 genome.
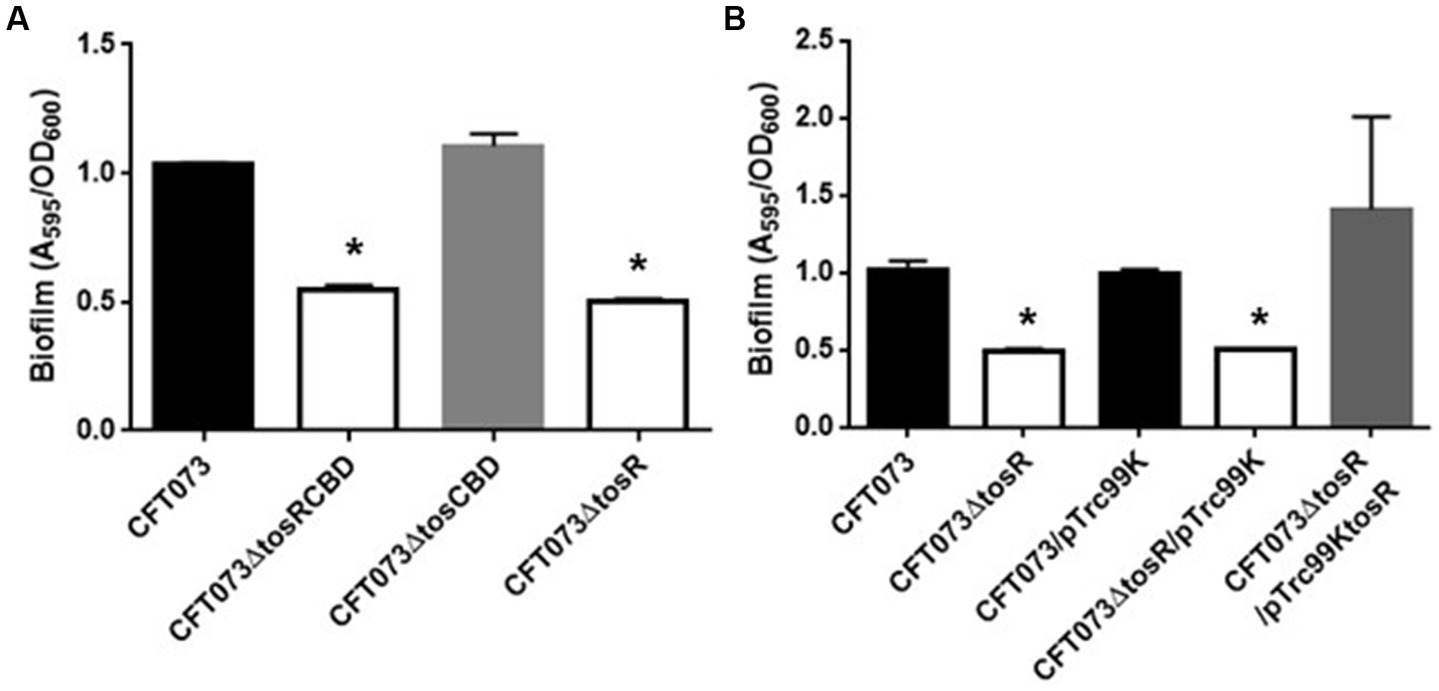
Figure 2. Biofilm formation on 96-well plates in the parent strain (CFT073) and its derivatives (A), or the parent and the tosR mutant carrying pTrc99K (empty vector) or pTrc99KtosR (tosR expression plasmid; B). Bacterial adhesion and aggregation were represented as A595 values normalized to OD600 of 1. Data plotted are the means of three biological replicates; error bars indicate standard deviations. *, P,0.01 relative to values for CFT073 (A) or CFT073/pTrc99K (B).
The biofilm forming ability of UPEC is closely related to invasion into and aggregation within urinary tract cells (Hirakawa et al., 2019). Therefore, we infected bladder epithelial cells with the parent strain and the tosR mutant, and compared the percentage of bacteria that entered the cells by gentamicin assay. The tosR mutant had a 2.7-fold lower value than the parent strain (0.020 +/− 0.002% vs. 0.054 +/− 0.009%, respectively; Figure 3A). Since there was no significant difference in total CFU/mL between the parent and mutant strains (CFU/mL of both strains were 1.2 × 108), the reduction in invasion efficiency caused by the tosR deletion was not due to a growth defect. The complementation of the tosR mutant with pTrc99KtosR plasmid increased invasive ability (Figure 3B). We constructed a parent strain and a tosR mutant carrying a GFP-expressing plasmid. By inoculating each strain within bladder epithelial cells, we imaged UPEC cells by GFP fluorescence. In the parent strain, aggregated bacteria were observed in some places in the host cells (Figures 4A,B). On the other hand, the tosR mutant exhibited clearly fewer aggregates than the parent strain (Figures 4A,B). We have also confirmed that introduction of the tosR complementation plasmid pTH18krtosR into the tosR mutant restores the defective aggregation ability (Figures 4A,B). These results indicate that the tosR gene contributes to UPEC aggregation in urinary tract cells.
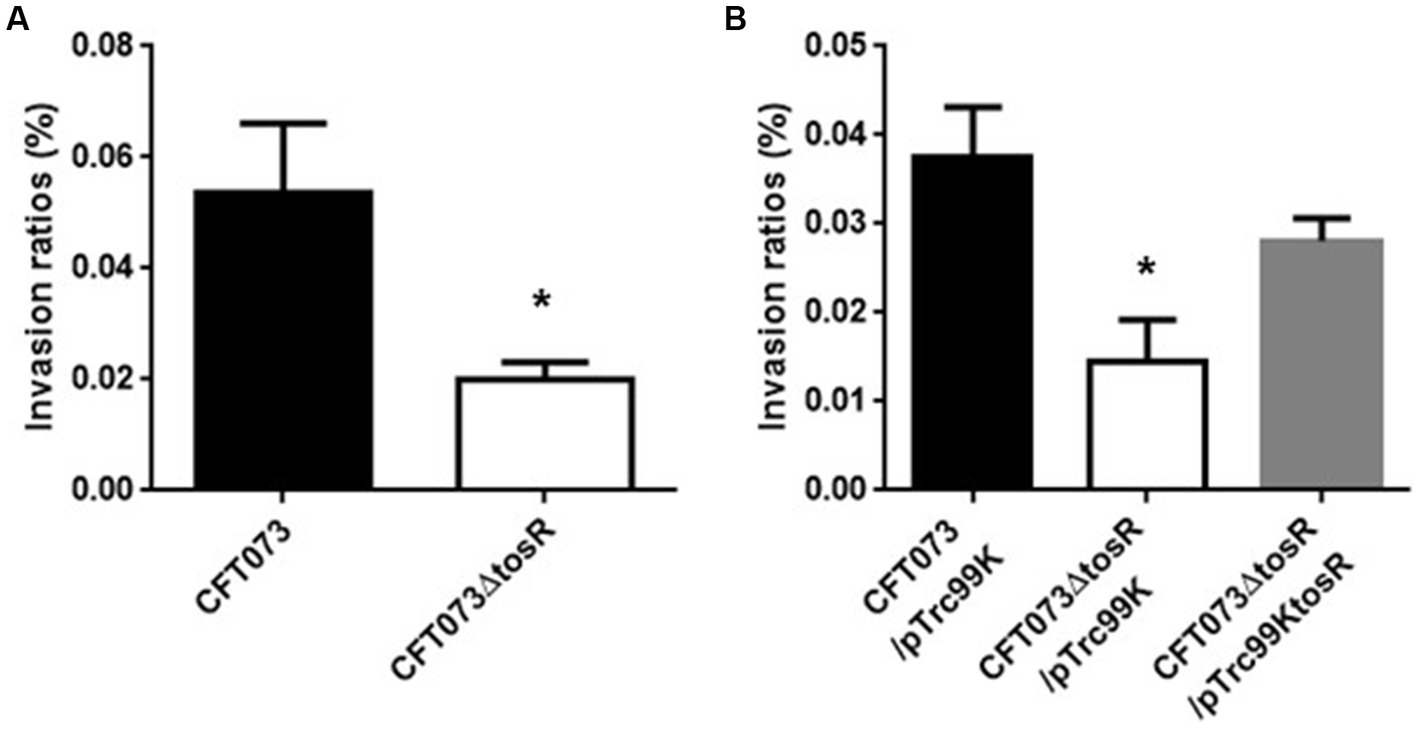
Figure 3. Internalization in bladder epithelial cells (HTB-9) of the parent strain (CFT073) and the tosR mutant (A) or the parent strain and the tosR mutant carrying pTrc99K or pTrc99KtosR (B). Values are percent CFU of internalized bacteria relative to total bacterial cell numbers. Data are means from three independent experiments; error bars indicate standard deviations. *, P,0.05 relative to values for CFT073 (A) or CFT073/pTrc99K (B).
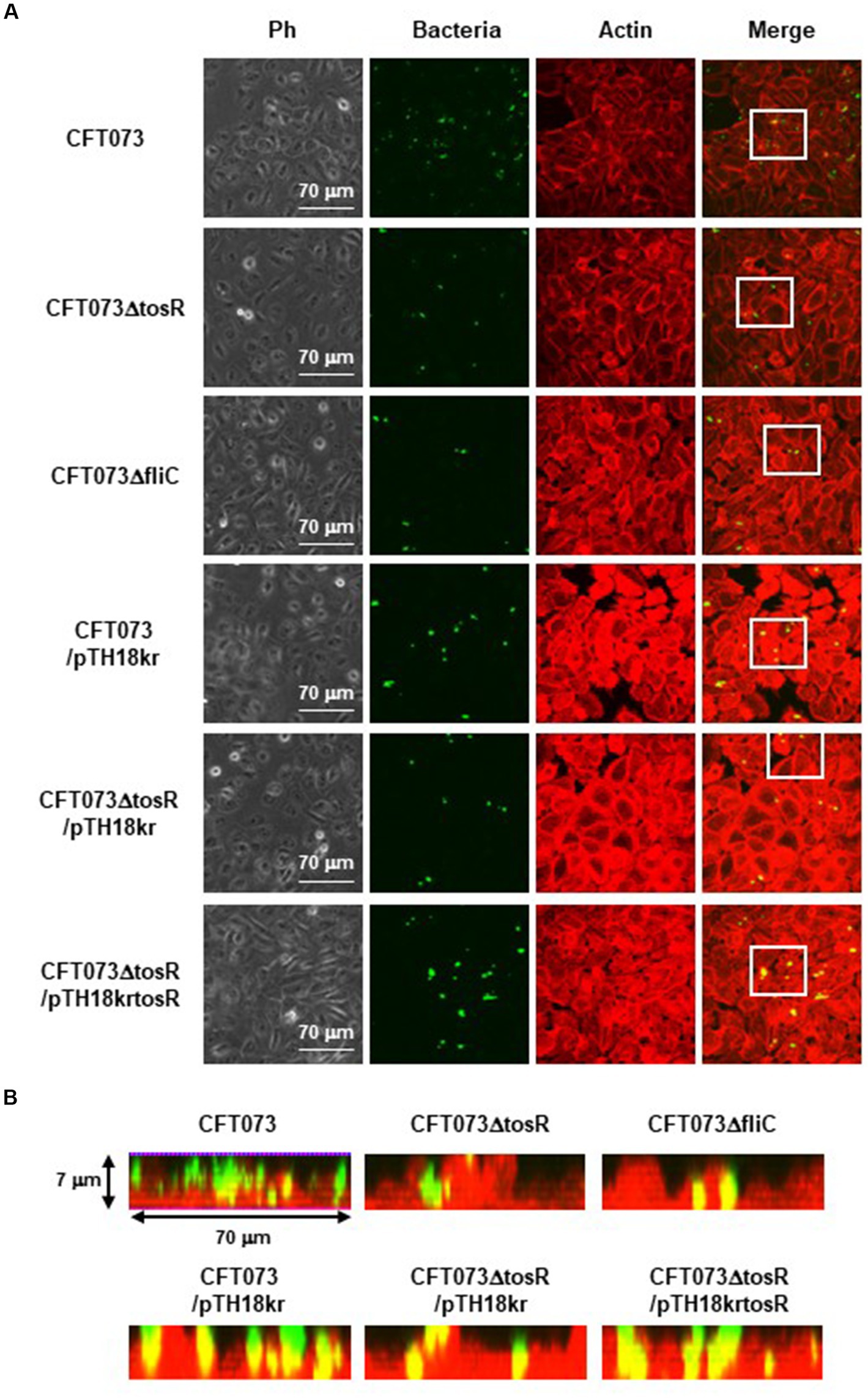
Figure 4. Aggregation within HTB-9 for the parent strain, the tosR mutant, the fliC mutant and the tosR complementation strain. Bacteria carrying a green fluorescence protein (GFP) expression plasmid, pTurboGFP-B, and HTB-9 cells (Actin) stained with rhodamine-phalloidin were imaged with green and red fluorescence, respectively, using a 60x objective. Images were taken from above, and cross-sectional images correspond to the white boxes. Ph: Phase contrast images. We performed this experiment twice, then similar results were obtained.
The tosR mutant exhibits lower motility followed by a lower flagellar expression, than the wild-type parent
UPEC fimbriae are important for aggregation in uroepithelial cells. Since the tosR gene is presumed to encode a PapB/FocB family transcriptional regulator, we hypothesized that the reduced aggregation ability due to TosR defect may be due to reduced expression of fimbriae. Type 1 fimbriae agglutinate guinea pig erythrocytes in the absence of mannose, i.e., their agglutination activity is mannose-sensitive, and P fimbriae can agglutinate human erythrocytes regardless of the presence of mannose (Hagberg et al., 1981; Korhonen et al., 1985). We measured the activity of type 1 and P fimbriae in the presence and absence of mannose in the parent strain and the tosR mutant, using the agglutination titers of guinea pig and human erythrocytes as indicators. However, there was no difference in the titer values exhibited by the parent strain and the mutant strain under either condition (Table 4). We also compared the transcript levels of fimbriae genes between the parent and tosR mutant strains by quantitative PCR analysis. Transcript levels of fimA and papA/papA2, genes encoding proteins for the main component of type 1 and P fimbriae, were not significantly different between the parent strain and the tosR mutant (Figure 5). This result was consistent with the results of the agglutination test using guinea pig and human erythrocytes. Transcript levels for the focA gene, which encodes F1C fimbria, were not significantly different between the parent and tosR mutant (Figure 5).
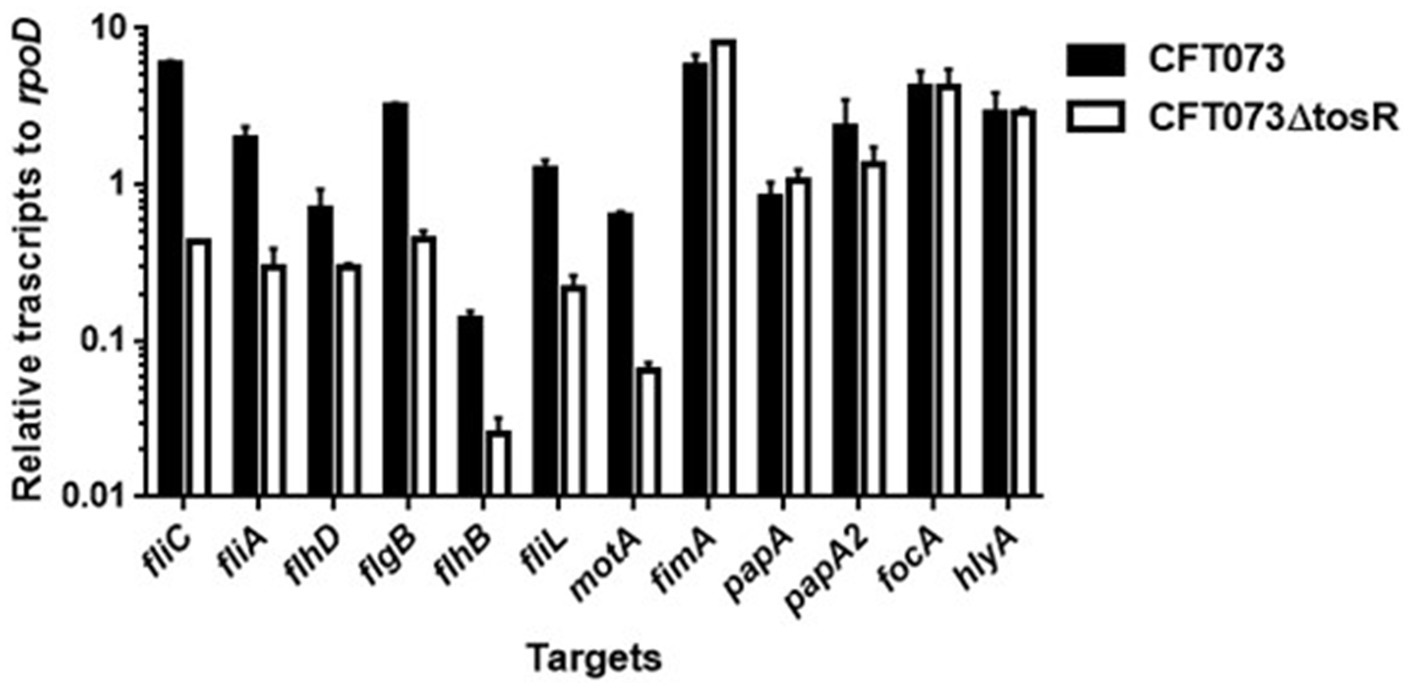
Figure 5. Transcript levels of flagellum-related and fimbrial genes and hlyA in the parent strain (CFT073) and the tosR mutant. Transcript levels were determined relative to that of rpoD. Data are means for two biological replicates; error bars indicate the ranges.
Flagella and flagellum-mediated motility of UPEC contribute to the aggregation of bacteria within urothelial cells (Hirakawa et al., 2019). Therefore, we tested whether the reduced aggregation ability caused by tosR deletion is due to reduced motility. Cultures of the parent strain and the tosR mutant were spotted on soft agar, and motility was compared by spreading of the bacteria, indicating that the tosR mutant was less motile than the parent strain (Figure 6A). As a control, the fliC mutant (CFT073∆fliC) showed no motility. Flagella are essential for motility. The results of flagellar staining showed that the tosR mutant had fewer flagella than the parent strain (Figure 6B). We also observed no flagellum in the fliC mutant. Furthermore, when the pTrc99KtosR plasmid was introduced into the tosR mutant, motility and flagellar production were increased (Figures 6A,B).
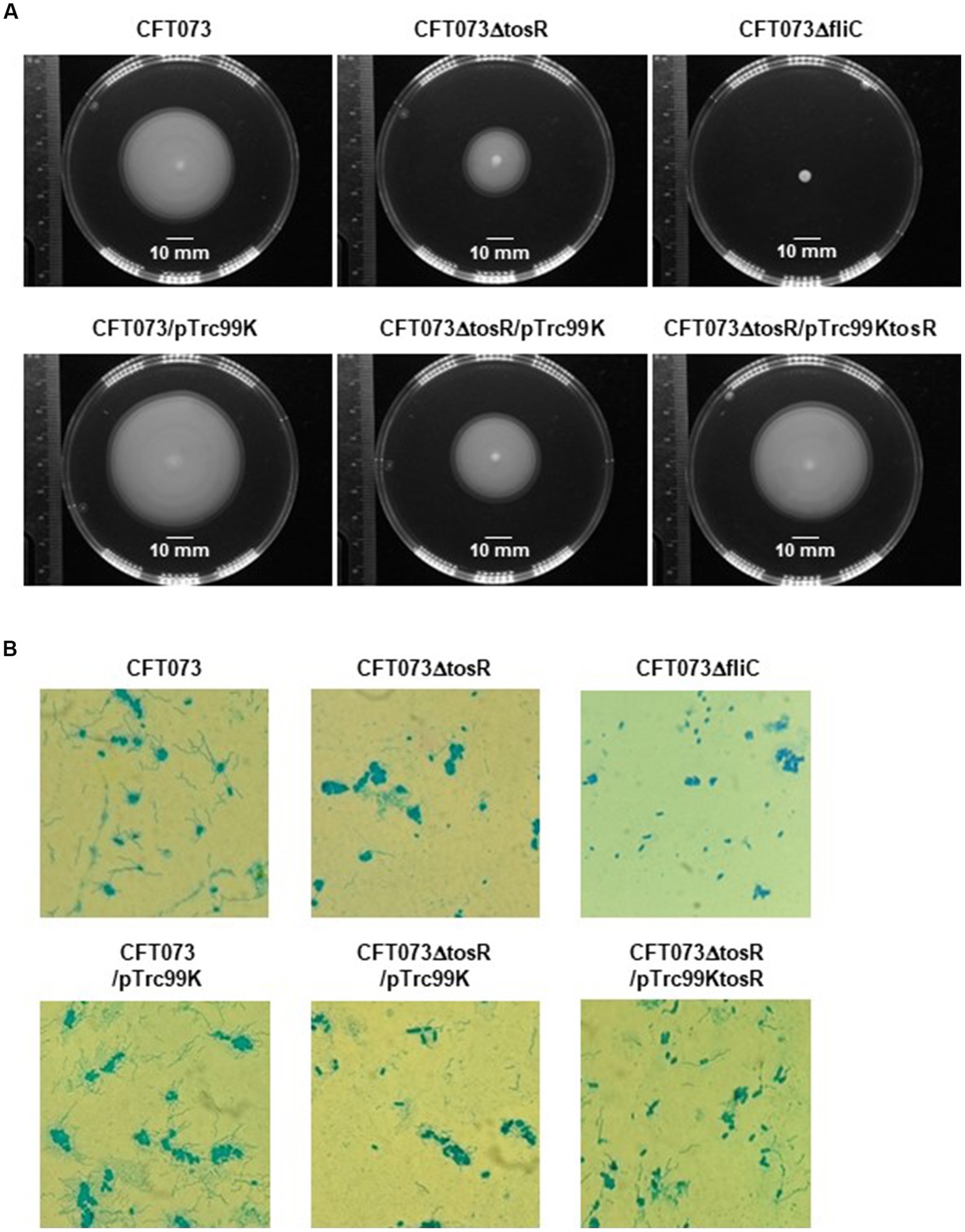
Figure 6. Motilities and flagellar production for the parent strain, the tosR mutant, the fliC mutant and the tosR complementation strain. (A) Bacterial migration on LB medium containing 0.3% agar. (B) Flagella and bacterial cells were stained with Victoria blue/tannic acid were pictured using a 100x objective. We performed this experiment twice, then similar results were obtained.
We examined the expression levels of flagellin FliC, a major constituent protein of flagella (Minamino and Imada, 2015), by Western blotting. We previously used a commercially available anti-flagellin antibody, but since no flagellin was detected even in the parent strain, we introduced the pTH18krfliC-VSVG plasmid into UPEC as an alternative method and expressed VSVG-tagged FliC as a recombinant protein (Hirakawa et al., 2021). Then, FliC was successfully detected using anti-VSVG antibody. The pTH18krfliC-VSVG plasmid contains the promoter and gene region of fliC inserted in the opposite direction of the lac promoter, which is upstream of the cloning site. In addition, the VSVG sequence is inserted just before the stop codon of fliC. Therefore, it is expected that strains carrying pTH18krfliC-VSVG will express C-terminally VSVG-tagged FliC, depending on the promoter of fliC itself. In this study, we used the same method with the pTH18krfliC-VSVG plasmid that we did previously. We compared the FliC-VSVG levels between CFT073 and CFT073∆tosR strains carrying the pTH18krfliC-VSVG plasmid. Western blotting results showed that the amount of band signal corresponding to FliC-VSVG expression from CFT073∆tosR was clearly lower than the CFT073 strain (Figure 7). In addition, when the tosR expression plasmid pTrc99AtosR was introduced into the CFT073∆tosR strain, the FliC-VSVG level was elevated. We note that the effect of tosR deletion on reduced FliC-VSVG levels appears more pronounced than the effect of reduced flagellar production and motility. This may be due to the low sensitivity of the VSVG antibody or to differences in culture conditions (For Western blotting, the bacteria were cultured in liquid, whereas for motility assays and flagellar staining, the bacteria were cultured on solid medium.).
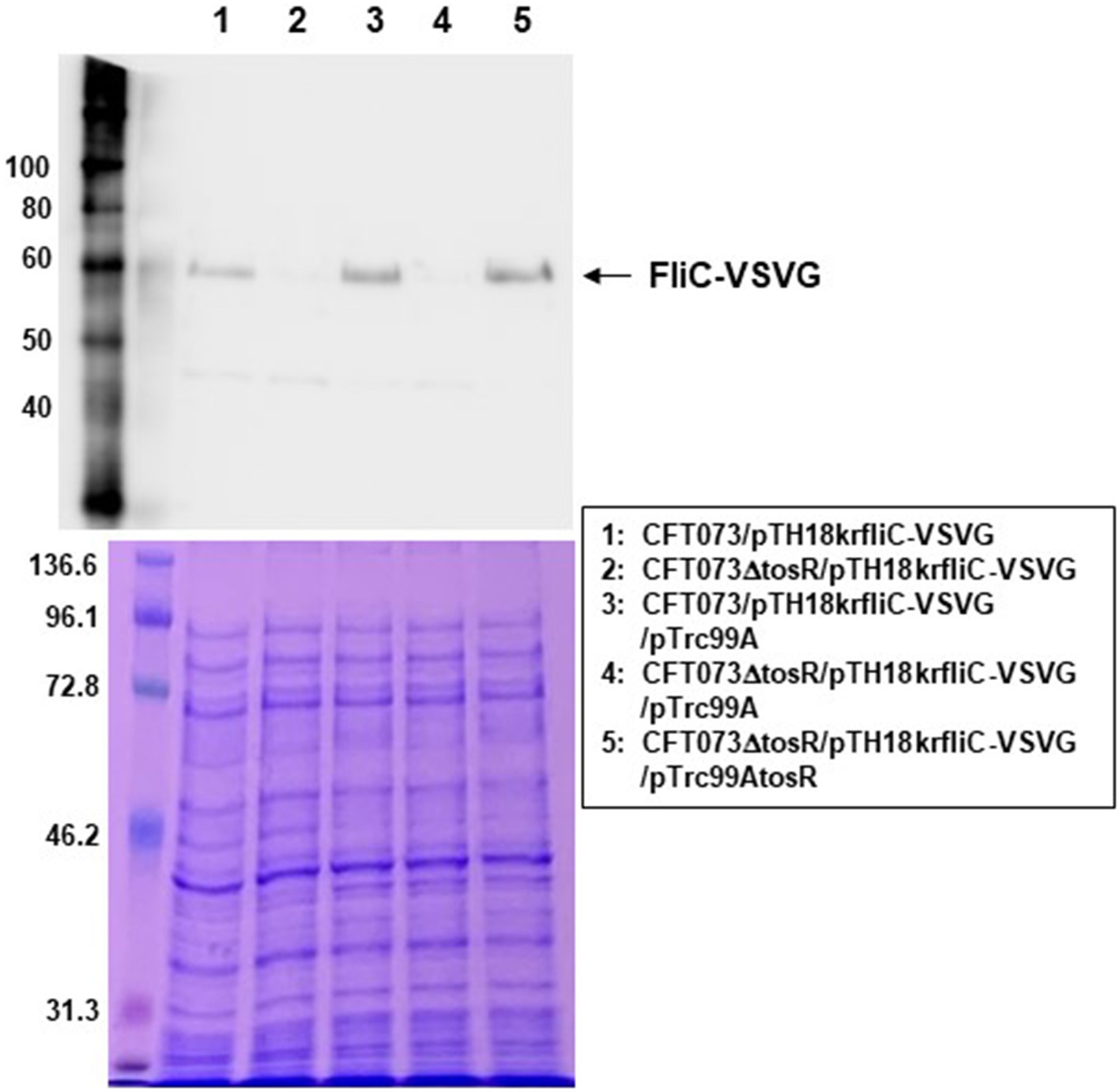
Figure 7. FliC expression in the parent strain (CFT073) and the tosR mutant. SDS-PAGE of cell lysates from the parent strain (CFT073) and the tosR mutant containing a VSVG-tagged FliC expression plasmid (pTH18krfliC-VSVG) and pTrc99A (empty vector) or pTrc99AtosR (tosR expression plasmid). Locations of molecular mass standards (in kilodaltons) are shown on the left. (Upper) VSVG-tagged FliC was visualized by probing with a VSVG antibody. (Lower) Proteins were stained with CBB. We performed this experiment twice, then similar results were obtained.
We measured the transcript levels of fliC and its regulatory genes. Consistent with the Western blotting results, the transcript level of fliC was lower in the tosR mutant than in the parental strain (Figure 6). Transcription of fliC is activated by the sigma factor FliA, which is activated by FlhD, a master regulator complex of flagellar expression and flhD is transcribed together with flhC as an operon (Soutourina and Bertin, 2003). We found that, like fliC, the transcript levels of flhD and fliA were approximately 2-and 8-fold lower in the tosR mutant than in the parental strain, respectively. In addition to the fliC, fliA, and flhD genes, the transcript level of the hlyA gene encoding hemolysin was also measured. However, no significant difference in the transcript level was observed between the parent and tosR mutant (Figure 6). Altogether, these results indicate that the reduction in aggregation ability due to tosR deletion is due to reduced motility associated with reduced flagellar production.
TosR is an activator of flhD gene expression
We investigated in more detail the role of TosR in regulating flagellar expression and its regulatory mechanisms. FlhDC also activates the transcription of genes that encode the flagellar components FlgB, FlhB, and FliL while the transcription of motA, encoding the motor protein for flagellar rotation, is induced by fliA (Macnab, 1992; Fitzgerald et al., 2014). The transcript levels of these genes in the tosR mutant were lower than those in the parental strain (Figure 6). These results indicate that reduced expression of flhDC and fliA due to tosR deletion leads to decreased transcript levels of flgB, flhB, fliL, and motA.
We also compared the promoter activities of flhD, fliA, and fliC in the parent and mutant strains. We determined the activity of each promoter by measuring the β-galactosidase activity corresponding to LacZ expression from the reporter plasmids pNNflhD-P-Long, pNNflhD-P-Short, pNNfliA-P, and pNNfliC-P. To evaluate flhD promoter activity, the pNNflhD-P-Long and pNNflhD-P-Short plasmids were used. TosR has been reported to bind to an AT-rich DNA sequence consisting of “WTWWTWTWWTWAAWKWTATKAWTDTDTD” (Luterbach et al., 2018; Figure 8A). We found a sequence similar to the TosR binding motif upstream of the flhD start codon at positions 550–578. The pNNflhD-P-Long plasmid is a reporter construct consisting of a 769-bp DNA fragment containing the entire intergenic region between flhD and its upstream gene, uspC. The pNNflhD-P-Short plasmid includes a region only 300 bases upstream of flhD lacking the AT-rich region, with a promoterless lacZ reporter gene. The promoter activity measurements revealed that these promoter activities from pNNflhD-P-Long, pNNfliA-P, and pNNfliC-P, but not pNNflhD-P-Short, were significantly lower in the tosR mutant than in the parent strain (Figure 8B). We also introduced the IPTG-inducible tosR expression plasmid pTrc99KtosR with each reporter plasmid, and measured LacZ activities. A concentration-dependent increase in LacZ activities was observed with IPTG (Figure 8C). We note that a growth defect was observed in the IPTG 0.1 mM culture similar to the biofilm assay, however a high degree of increased promoter activity was observed. These results suggest that the expression of tosR promotes the promoter activity of flhD, fliA, and fliC.
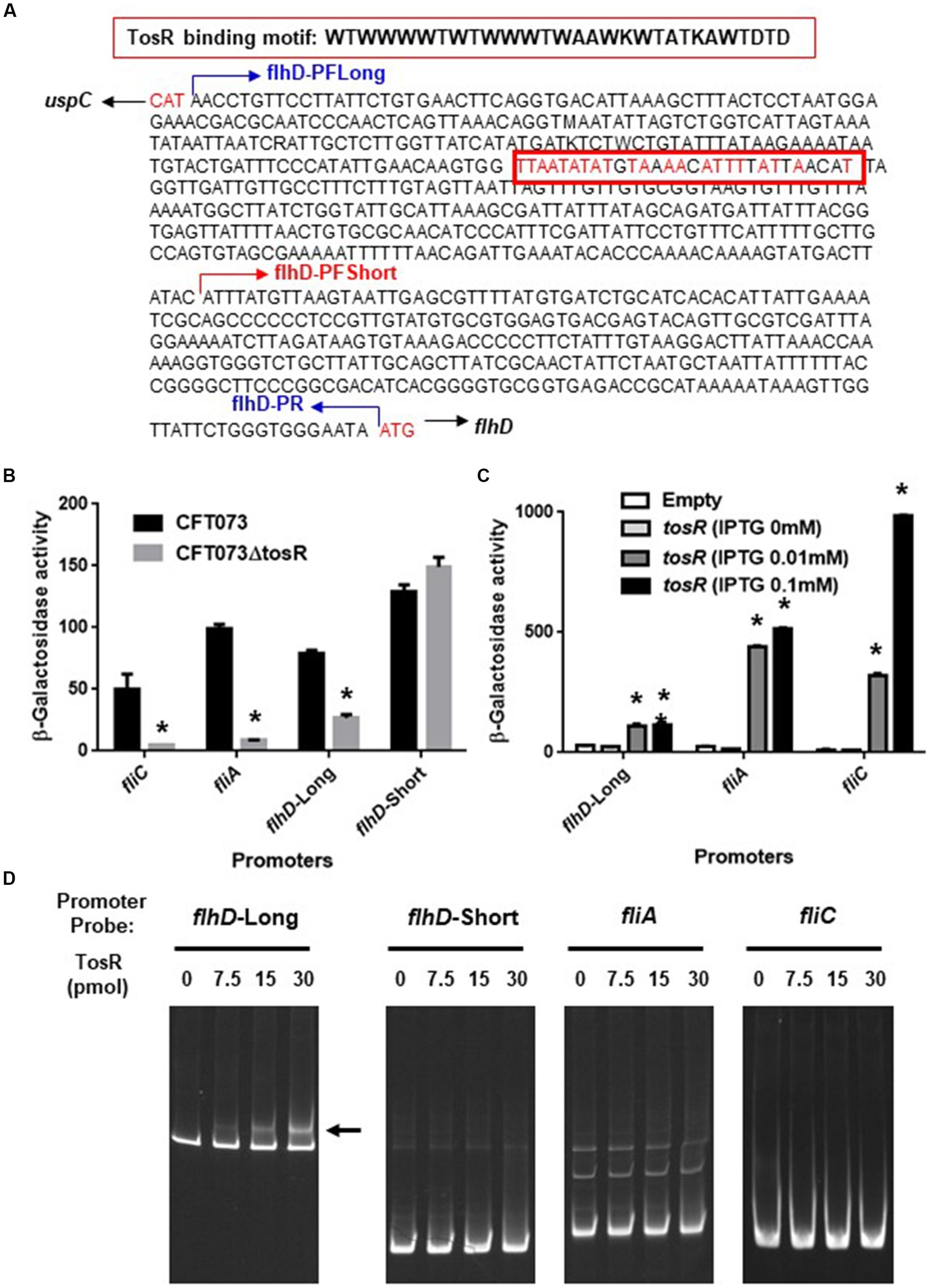
Figure 8. Promoter activities of flhD, fliA, and fliC and the TosR-binding to flhD upstream region. (A) DNA sequence upstream of flhD. flhD-PFLong, flhD-PFShort and flhD-PR are names of the primers and their locations. The red box is a DNA sequence to which TosR is presumed to bind. Nucleotides in red indicate nucleotides match within the TosR binding motif. (B) β-Galactosidase activities corresponding to flhD, fliA, and fliC promoter activities in the parent strain and the tosR mutant containing pNNflhD-Long, pNNflhD-Short, pNNfliA-P, or pNNfliC-P, the lacZ reporter plasmid. (C) β-Galactosidase activities corresponding to flhD, fliA, and fliC promoter activities in the tosR mutant carrying pTrc99K (empty) or pTrc99KtosR (IPTG-inducible tosR expression plasmid). Data are means from three independent experiments; error bars indicate standard deviations. *, P,0.01 relative to CFT073 or empty vector control. (D) Gel shift assay showing binding of TosR to the region upstream of flhD. The TosR protein (0, 7.5, 15 or 30 pmol) was added to reaction mixtures containing 0.3 pmol of DNA probe. We performed this experiment twice, then similar results were obtained. Promoters and DNA probes designated flhD-Long and flhD-Short contain the region from 769-bp (including the AT-rich site) and 300-bp (lacking the AT-rich site) upstream from the flhD start codon, respectively.
We examined the DNA binding ability of TosR by gel shift assay. In the presence of 7.5 pmol of TosR-His8 protein, a band shift was observed in the DNA probe containing the AT-rich region upstream of flhD (Figure 8D). We used a DNA probe containing only a region 300 bases upstream of flhD that lacked the AT-rich region. We also examined the binding ability of TosR to DNA probes containing the upstream 300 bases of fliA and fliC. As a result, no band shift in these DNA probes was observed even when 30 pomol of TosR-His8 was present (Figure 8D). These results indicate that TosR is an activator that binds to the AT-rich region upstream of flhD and stimulates the promoter activity of flhD.
Discussion
Flagella of UPEC contribute to bacterial aggregation leading to IBC formation in host epithelial cells (Hirakawa et al., 2019, 2021). On the other hand, flagellin binds to Toll-like receptor-5 and activate the host immune system (Miao et al., 2007). Therefore, the expression level of UPEC flagella must be tightly regulated within the infected host. Expression of the fliC gene, which encodes the major flagellar component flagellin, is activated by the sigma factor FliA (Soutourina and Bertin, 2003). Expression of fliA is activated by the master regulator of flagellar expression, FlhD-FlhC. Expression of fliC is also controlled by a number of regulatory proteins including CRP, LrhA, H-NS, QseB, RcsB, OmpR, Fur, TosEF and CytR (Bertin et al., 1994; Shin and Park, 1995; Soutourina et al., 1999; Lehnen et al., 2002; Francez-Charlot et al., 2003; Clarke and Sperandio, 2005; Engstrom et al., 2014; Kurabayashi et al., 2016; Hirakawa et al., 2020). In addition, the database RegulonDB revealed a set of potential regulators for FlhDC expression (Tierrafria et al., 2022; Figure 9). This study showed that TosR is also involved in the regulation of flagellar expression.
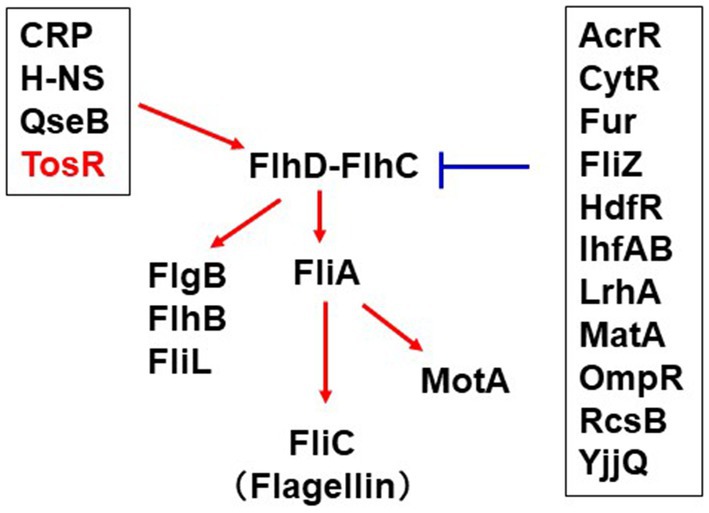
Figure 9. Overview of the regulatory mechanism of flhD expression and contribution of this study. The red arrows positively regulate flhDC transcription while the blue bars indicate repression of flhDC transcription. The information on the regulator groups framed in black was taken from RegulonDB (Tierrafria et al., 2022). CytR recently characterized in our study was added (Hirakawa et al., 2020). TosR was clarified in this study.
TosR has been characterized as a PapB/FocB family transcriptional regulator that controls the expression of the nonfimbrial adhesin TosA (Engstrom and Mobley, 2016). TosR has also been shown to bind to AT-rich DNA sequences (Luterbach et al., 2018). Previously, a transcriptome analysis identified a set of genes whose transcript levels increase or decrease upon TosR overexpression (Luterbach et al., 2018). Interestingly, no flagellar gene was identified in that study. We noticed the presence of an AT-rich sequence upstream of the flhDC operon that resembles the TosR binding motif. Our gel shift assay revealed that TosR bound to a DNA probe containing the AT-rich sequence upstream of its flhD, while it did not bind to the DNA probe lacking the AT-rich sequence. Furthermore, in promoter assays, the LacZ reporter construct of the flhD promoter containing the AT-rich sequence showed reduced LacZ activity upon tosR deletion and increased LacZ activity upon tosR overexpression. In contrast, no effect of tosR deletion was observed for the LacZ reporter construct lacking the AT-rich sequence. These results suggested that TosR positively regulates flhDC expression.
Deletion of tosR causes reduced flagellar production. This is supported by the finding that tosR deletion reduces motility. However, a study conducted by Engstrom et al., showed that the tosR mutant exhibited motility comparable to that of the parental strain (Engstrom et al., 2014). Bacterial motility can be affected by even small differences in medium, temperature, and agar concentration. In Engstrom’s assay, the bacteria were stabbed on agar medium without yeast extract and evaluated after 17 h, whereas in our assay, the bacterial cultures were spotted on agar medium containing yeast extract and evaluated after 9 h. Our assay seems to inoculate more bacteria than Engstrom’s assay. If we incubate for 17 h as in the Engstrom’s assay, both the parent and mutant strains will migrate to the edge of the plate, making it impossible to compare motility between the two strains. We believe that the difference from the Engstrom’s assay is due to the difference in medium and inoculation method.
The tosR gene, together with the tosA gene, is encoded on a pathogenicity island on the chromosome of CFT073, and this pathogenicity island containing the tosR and tosA genes is also found in many UPECs besides CFT073 (Engstrom et al., 2014). Therefore, TosR may play a significant role in the regulation of flagellar expression in UPEC.
TosR is known to repress the expression of P fimbria while promoting the expression of Auf fimbria and Curli, amyloid-like fibers (Luterbach et al., 2018). Furthermore, TosR regulates tosA expression in a TosR level-dependent manner (Engstrom and Mobley, 2016). P and Auf fimbriae, Curli and TosA are involved in adhesion to urinary epithelial cells. The tosR mutant used in this study is actually less virulent to uroepithelial cells than the parent strain, although it is presumed to have increased P fimbria and TosA levels while expressing less Auf fimbria and Curli, at least compared to the parent strain. Colonization of UPEC within urinary tract epithelial cells is linked to UPEC’s ability to form biofilm (Hirakawa et al., 2019). UPEC colonization and biofilm formation in uroepithelial cells are positively correlated with the flagellar expression (Hirakawa et al., 2019, 2021). Taken together, we speculate that the reduced expression of Auf fmbria and Curli in the tosR defective strain, as well as the effect of reduced UPEC colonization and biofilm formation capacity due to reduced flagellar expression, mask the effect of increased P fimbria and TosA levels.
The region upstream of flhD where TosR acts is more than 500 bases away from the flhD start codon, and it is still unclear how TosR promotes flhD transcription by binding to a region far from the flhD promoter. Nucleoid-associated proteins, such as H-NS, are known to bind to DNA and modulate the local nucleoid structure, thereby regulating the expression of genes far away (Dorman, 2004). TosR is thought to regulate gene expression in a similar manner to nucleoid-associated proteins (Engstrom and Mobley, 2016). Therefore, it is possible that TosR binding to flhD promotes transcription through modulation of the local nucleoid structure.
Previous studies showed that tosR expression is repressed by H-NS in vitro (under 37°C, LB medium conditions), and its repression is alleviated in urinary tract infection mice (Vigil et al., 2011, 2012; Engstrom et al., 2014). However, in this study, the effect of tosR deletion on flagellar expression was observed at 37°C in LB medium, implying that tosR is expressed in vitro to some extents. The regulatory mechanism of tosR expression needs to be elucidated to understand the mechanism of virulence induction in UPEC, including flagellar expression.
In addition to TosR, a number of PapB/FocB family transcriptional regulators, including SfaB, DaaA, FaeB, FanA, FanB, ClpB, PefB, and AfaA, have been characterized in E. coli and Salmonella (Xia and Uhlin, 1999; Holden et al., 2001). While they have been described to regulate the expression of various fimbriae and other adhesin-related genes (Spurbeck et al., 2011), they have not been shown to regulate the flagellum. We provide evidence that TosR is a positive regulator of flagellar expression. We believe that our findings suggest additional possible functions for the PapB/FocB family of transcriptional regulators and provide further insight into the mechanisms of flagellar expression and pathogenesis in UPEC.
Data availability statement
The original contributions presented in the study are included in the article/Supplementary material, further inquiries can be directed to the corresponding author.
Author contributions
HH, HTA, IK, and HTO designed the research and wrote the manuscript. HH, MS, KN, MT, HM, AT, and KS performed the experiments. HH, IK, and HTO analyzed the data.
Funding
This research was funded by the Japan Society for the Promotion of Science (JSPS) “Grant-in-Aid for Scientific Research (B)” (Grant No. 22H02864 to HH and KS), Japan Agency Research and development [AMED] (Grant No. 22fk0108604h0902 and 22wm0225008h0203 to HTO), and Health Labour Sciences Research Grant (Grant No. 21KA1004 to HTO).
Conflict of interest
The authors declare that the research was conducted in the absence of any commercial or financial relationships that could be construed as a potential conflict of interest.
Publisher’s note
All claims expressed in this article are solely those of the authors and do not necessarily represent those of their affiliated organizations, or those of the publisher, the editors and the reviewers. Any product that may be evaluated in this article, or claim that may be made by its manufacturer, is not guaranteed or endorsed by the publisher.
References
Anderson, G. G., Palermo, J. J., Schilling, J. D., Roth, R., Heuser, J., and Hultgren, S. J. (2003). Intracellular bacterial biofilm-like pods in urinary tract infections. Science 301, 105–107. doi: 10.1126/science.1084550
Bertin, P., Terao, E., Lee, E. H., Lejeune, P., Colson, C., Danchin, A., et al. (1994). The H-NS protein is involved in the biogenesis of flagella in Escherichia coli. J. Bacteriol. 176, 5537–5540. doi: 10.1128/jb.176.17.5537-5540.1994
Clarke, M. B., and Sperandio, V. (2005). Transcriptional regulation of flh DC by QseBC and sigma (FliA) in enterohaemorrhagic Escherichia coli. Mol. Microbiol. 57, 1734–1749. doi: 10.1111/j.1365-2958.2005.04792.x
Dhakal, B. K., and Mulvey, M. A. (2012). The UPEC pore-forming toxin alpha-hemolysin triggers proteolysis of host proteins to disrupt cell adhesion, inflammatory, and survival pathways. Cell Host Microbe 11, 58–69. doi: 10.1016/j.chom.2011.12.003
Dorman, C. J. (2004). H-NS: a universal regulator for a dynamic genome. Nat. Rev. Microbiol. 2, 391–400. doi: 10.1038/nrmicro883
Elledge, S. J., and Davis, R. W. (1989). Position and density effects on repression by stationary and mobile DNA-binding proteins. Genes Dev. 3, 185–197. doi: 10.1101/gad.3.2.185
Engstrom, M. D., Alteri, C. J., and Mobley, H. L. (2014). A conserved PapB family member, TosR, regulates expression of the uropathogenic Escherichia coli RTX nonfimbrial adhesin TosA while conserved LuxR family members TosE and TosF suppress motility. Infect. Immun. 82, 3644–3656. doi: 10.1128/IAI.01608-14
Engstrom, M. D., and Mobley, H. L. (2016). Regulation of Expression of Uropathogenic Escherichia coli Nonfimbrial Adhesin TosA by PapB Homolog TosR in Conjunction with H-NS and Lrp. Infect. Immun. 84, 811–821. doi: 10.1128/IAI.01302-15
Fitzgerald, D. M., Bonocora, R. P., and Wade, J. T. (2014). Comprehensive mapping of the Escherichia coli flagellar regulatory network. PLoS Genet. 10:e1004649. doi: 10.1371/journal.pgen.1004649
Flores-Mireles, A. L., Walker, J. N., Caparon, M., and Hultgren, S. J. (2015). Urinary tract infections: epidemiology, mechanisms of infection and treatment options. Nat. Rev. Microbiol. 13, 269–284. doi: 10.1038/nrmicro3432
Forsman, K., Goransson, M., and Uhlin, B. E. (1989). Autoregulation and multiple DNA interactions by a transcriptional regulatory protein in E. coli pili biogenesis. EMBO J. 8, 1271–1277. doi: 10.1002/j.1460-2075.1989.tb03501.x
Francez-Charlot, A., Laugel, B., Van Gemert, A., Dubarry, N., Wiorowski, F., Castanie-Cornet, M. P., et al. (2003). RcsCDB His-Asp phosphorelay system negatively regulates the flhDC operon in Escherichia coli. Mol. Microbiol. 49, 823–832. doi: 10.1046/j.1365-2958.2003.03601.x
Hagberg, L., Jodal, U., Korhonen, T. K., Lidin-Janson, G., Lindberg, U., and Svanborg Eden, C. (1981). Adhesion, hemagglutination, and virulence of Escherichia coli causing urinary tract infections. Infect. Immun. 31, 564–570. doi: 10.1128/iai.31.2.564-570.1981
Hashimoto-Gotoh, T., Yamaguchi, M., Yasojima, K., Tsujimura, A., Wakabayashi, Y., and Watanabe, Y. (2000). A set of temperature sensitive-replication/−segregation and temperature resistant plasmid vectors with different copy numbers and in an isogenic background (chloramphenicol, kanamycin, lacZ, repA, par, polA). Gene 241, 185–191. doi: 10.1016/S0378-1119(99)00434-5
Hirakawa, H., Kurabayashi, K., Tanimoto, K., and Tomita, H. (2018). Oxygen Limitation Enhances the Antimicrobial Activity of Fosfomycin in Pseudomonas aeruginosa Following Overexpression of glpT Which Encodes Glycerol-3-Phosphate/Fosfomycin Symporter. Front. Microbiol. 9:1950. doi: 10.3389/fmicb.2018.01950
Hirakawa, H., Nishino, K., Hirata, T., and Yamaguchi, A. (2003a). Comprehensive studies of drug resistance mediated by overexpression of response regulators of two-component signal transduction systems in Escherichia coli. J. Bacteriol. 185, 1851–1856. doi: 10.1128/JB.185.6.1851-1856.2003
Hirakawa, H., Nishino, K., Yamada, J., Hirata, T., and Yamaguchi, A. (2003b). Beta-lactam resistance modulated by the overexpression of response regulators of two-component signal transduction systems in Escherichia coli. J. Antimicrob. Chemother. 52, 576–582. doi: 10.1093/jac/dkg406
Hirakawa, H., Suzue, K., Kurabayashi, K., and Tomita, H. (2019). The Tol-Pal System of Uropathogenic Escherichia coli Is Responsible for Optimal Internalization Into and Aggregation Within Bladder Epithelial Cells, Colonization of the Urinary Tract of Mice, and Bacterial Motility. Front. Microbiol. 10:1827. doi: 10.3389/fmicb.2019.01827
Hirakawa, H., Suzue, K., Takita, A., Kamitani, W., and Tomita, H. (2021). Roles of OmpX, an Outer Membrane Protein, on Virulence and Flagellar Expression in Uropathogenic Escherichia coli. Infect. Immun. 89, e00721–e00720. doi: 10.1128/IAI.00721-20
Hirakawa, H., Takita, A., Kato, M., Mizumoto, H., and Tomita, H. (2020). Roles of CytR, an anti-activator of cyclic-AMP receptor protein (CRP) on flagellar expression and virulence in uropathogenic Escherichia coli. Biochem. Biophys. Res. Commun. 521, 555–561. doi: 10.1016/j.bbrc.2019.10.165
Holden, N. J., Uhlin, B. E., and Gally, D. L. (2001). PapB paralogues and their effect on the phase variation of type 1 fimbriae in Escherichia coli. Mol. Microbiol. 42, 319–330. doi: 10.1046/j.1365-2958.2001.02656.x
Korhonen, T. K., Valtonen, M. V., Parkkinen, J., Vaisanen-Rhen, V., Finne, J., Orskov, F., et al. (1985). Serotypes, hemolysin production, and receptor recognition of Escherichia coli strains associated with neonatal sepsis and meningitis. Infect. Immun. 48, 486–491. doi: 10.1128/iai.48.2.486-491.1985
Kurabayashi, K., Agata, T., Asano, H., Tomita, H., and Hirakawa, H. (2016). Fur Represses Adhesion to, Invasion of, and Intracellular Bacterial Community Formation within Bladder Epithelial Cells and Motility in Uropathogenic Escherichia coli. Infect. Immun. 84, 3220–3231. doi: 10.1128/IAI.00369-16
Lane, M. C., Alteri, C. J., Smith, S. N., and Mobley, H. L. (2007). Expression of flagella is coincident with uropathogenic Escherichia coli ascension to the upper urinary tract. Proc. Natl. Acad. Sci. U. S. A. 104, 16669–16674. doi: 10.1073/pnas.0607898104
Lane, M. C., Lockatell, V., Monterosso, G., Lamphier, D., Weinert, J., Hebel, J. R., et al. (2005). Role of motility in the colonization of uropathogenic Escherichia coli in the urinary tract. Infect. Immun. 73, 7644–7656. doi: 10.1128/IAI.73.11.7644-7656.2005
Lehnen, D., Blumer, C., Polen, T., Wackwitz, B., Wendisch, V. F., and Unden, G. (2002). LrhA as a new transcriptional key regulator of flagella, motility and chemotaxis genes in Escherichia coli. Mol. Microbiol. 45, 521–532. doi: 10.1046/j.1365-2958.2002.03032.x
Lillington, J., Geibel, S., and Waksman, G. (2014). Biogenesis and adhesion of type 1 and P pili. Biochim. Biophys. Acta 1840, 2783–2793. doi: 10.1016/j.bbagen.2014.04.021
Lindberg, S., Xia, Y., Sonden, B., Goransson, M., Hacker, J., and Uhlin, B. E. (2008). Regulatory Interactions among adhesin gene systems of uropathogenic Escherichia coli. Infect. Immun. 76, 771–780. doi: 10.1128/IAI.01010-07
Link, A. J., Phillips, D., and Church, G. M. (1997). Methods for generating precise deletions and insertions in the genome of wild-type Escherichia coli: application to open reading frame characterization. J. Bacteriol. 179, 6228–6237. doi: 10.1128/jb.179.20.6228-6237.1997
Lloyd, A. L., Henderson, T. A., Vigil, P. D., and Mobley, H. L. (2009). Genomic islands of uropathogenic Escherichia coli contribute to virulence. J. Bacteriol. 191, 3469–3481. doi: 10.1128/JB.01717-08
Luterbach, C. L., Forsyth, V. S., Engstrom, M. D., and Mobley, H. L. T. (2018). TosR-mediated regulation of adhesins and biofilm formation in uropathogenic Escherichia coli. mSphere 3, e00222–e00218. doi: 10.1128/mSphere.00222-18
Macnab, R. M. (1992). Genetics and biogenesis of bacterial flagella. Annu. Rev. Genet. 26, 131–158. doi: 10.1146/annurev.ge.26.120192.001023
Martinez, J. J., Mulvey, M. A., Schilling, J. D., Pinkner, J. S., and Hultgren, S. J. (2000). Type 1 pilus-mediated bacterial invasion of bladder epithelial cells. EMBO J. 19, 2803–2812. doi: 10.1093/emboj/19.12.2803
Mathers, A. J., Peirano, G., and Pitout, J. D. (2015). The role of epidemic resistance plasmids and international high-risk clones in the spread of multidrug-resistant Enterobacteriaceae. Clin. Microbiol. Rev. 28, 565–591. doi: 10.1128/CMR.00116-14
Miao, E. A., Andersen-Nissen, E., Warren, S. E., and Aderem, A. (2007). TLR5 and Ipaf: dual sensors of bacterial flagellin in the innate immune system. Semin. Immunopathol. 29, 275–288. doi: 10.1007/s00281-007-0078-z
Minamino, T., and Imada, K. (2015). The bacterial flagellar motor and its structural diversity. Trends Microbiol. 23, 267–274. doi: 10.1016/j.tim.2014.12.011
Mulvey, M. A., Lopez-Boado, Y. S., Wilson, C. L., Roth, R., Parks, W. C., Heuser, J., et al. (1998). Induction and evasion of host defenses by type 1-piliated uropathogenic Escherichia coli. Science 282, 1494–1497. doi: 10.1126/science.282.5393.1494
Mulvey, M. A., Schilling, J. D., and Hultgren, S. J. (2001). Establishment of a persistent Escherichia coli reservoir during the acute phase of a bladder infection. Infect. Immun. 69, 4572–4579. doi: 10.1128/IAI.69.7.4572-4579.2001
Pichon, C., Hechard, C., Du Merle, L., Chaudray, C., Bonne, I., Guadagnini, S., et al. (2009). Uropathogenic Escherichia coli AL511 requires flagellum to enter renal collecting duct cells. Cell. Microbiol. 11, 616–628. doi: 10.1111/j.1462-5822.2008.01278.x
Shin, S., and Park, C. (1995). Modulation of flagellar expression in Escherichia coli by acetyl phosphate and the osmoregulator OmpR. J. Bacteriol. 177, 4696–4702. doi: 10.1128/jb.177.16.4696-4702.1995
Soutourina, O. A., and Bertin, P. N. (2003). Regulation cascade of flagellar expression in Gram-negative bacteria. FEMS Microbiol. Rev. 27, 505–523. doi: 10.1016/S0168-6445(03)00064-0
Soutourina, O., Kolb, A., Krin, E., Laurent-Winter, C., Rimsky, S., Danchin, A., et al. (1999). Multiple control of flagellum biosynthesis in Escherichia coli: role of H-NS protein and the cyclic AMP-catabolite activator protein complex in transcription of the flhDC master operon. J. Bacteriol. 181, 7500–7508. doi: 10.1128/JB.181.24.7500-7508.1999
Spurbeck, R. R., Stapleton, A. E., Johnson, J. R., Walk, S. T., Hooton, T. M., and Mobley, H. L. (2011). Fimbrial profiles predict virulence of uropathogenic Escherichia coli strains: contribution of ygi and yad fimbriae. Infect. Immun. 79, 4753–4763. doi: 10.1128/IAI.05621-11
Tierrafria, V. H., Rioualen, C., Salgado, H., Lara, P., Gama-Castro, S., Lally, P., et al. (2022). RegulonDB 11.0: Comprehensive high-throughput datasets on transcriptional regulation in Escherichia coli K-12. Microb. Genom. 8:mgen000833. doi: 10.1099/mgen.0.000833
Vigil, P. D., Alteri, C. J., and Mobley, H. L. (2011). Identification of in vivo-induced antigens including an RTX family exoprotein required for uropathogenic Escherichia coli virulence. Infect. Immun. 79, 2335–2344. doi: 10.1128/IAI.00110-11
Vigil, P. D., Wiles, T. J., Engstrom, M. D., Prasov, L., Mulvey, M. A., and Mobley, H. L. (2012). The repeat-in-toxin family member TosA mediates adherence of uropathogenic Escherichia coli and survival during bacteremia. Infect. Immun. 80, 493–505. doi: 10.1128/IAI.05713-11
Virkola, R., Westerlund, B., Holthofer, H., Parkkinen, J., Kekomaki, M., and Korhonen, T. K. (1988). Binding characteristics of Escherichia coli adhesins in human urinary bladder. Infect. Immun. 56, 2615–2622. doi: 10.1128/iai.56.10.2615-2622.1988
Walker, E., Lyman, A., Gupta, K., Mahoney, M. V., Snyder, G. M., and Hirsch, E. B. (2016). Clinical Management of an Increasing Threat: Outpatient Urinary Tract Infections Due to Multidrug-Resistant Uropathogens. Clin. Infect. Dis. 63, 960–965. doi: 10.1093/cid/ciw396
Welch, R. A., Burland, V., Plunkett, G. 3rd, Redford, P., Roesch, P., Rasko, D., et al. (2002). Extensive mosaic structure revealed by the complete genome sequence of uropathogenic Escherichia coli. Proc. Natl. Acad. Sci. U. S. A. 99, 17020–17024. doi: 10.1073/pnas.252529799
Wright, K. J., Seed, P. C., and Hultgren, S. J. (2005). Uropathogenic Escherichia coli flagella aid in efficient urinary tract colonization. Infect. Immun. 73, 7657–7668. doi: 10.1128/IAI.73.11.7657-7668.2005
Xia, Y., and Uhlin, B. E. (1999). Mutational analysis of the PapB transcriptional regulator in Escherichia coli. Regions important for DNA binding and oligomerization. J. Biol. Chem. 274, 19723–19730. doi: 10.1074/jbc.274.28.19723
Keywords: urinary tract infection, bacterial pathogenesis, virulence, flagella, fimbriae, Biofilm
Citation: Hirakawa H, Shimokawa M, Noguchi K, Tago M, Matsuda H, Takita A, Suzue K, Tajima H, Kawagishi I and Tomita H (2023) The PapB/FocB family protein TosR acts as a positive regulator of flagellar expression and is required for optimal virulence of uropathogenic Escherichia coli. Front. Microbiol. 14:1185804. doi: 10.3389/fmicb.2023.1185804
Edited by:
Axel Cloeckaert, Institut National de recherche pour l’agriculture, l’alimentation et l’environnement (INRAE), FranceReviewed by:
Monica Alejandra Delgado, CONICET Higher Institute of Biological Research (INSIBIO), ArgentinaClaudine Baraquet, Université de Toulon, France
Jenny-Lee Thomassin, University of Saskatchewan, Canada
Copyright © 2023 Hirakawa, Shimokawa, Noguchi, Tago, Matsuda, Takita, Suzue, Tajima, Kawagishi and Tomita. This is an open-access article distributed under the terms of the Creative Commons Attribution License (CC BY). The use, distribution or reproduction in other forums is permitted, provided the original author(s) and the copyright owner(s) are credited and that the original publication in this journal is cited, in accordance with accepted academic practice. No use, distribution or reproduction is permitted which does not comply with these terms.
*Correspondence: Hidetada Hirakawa, aGlyYWthd2FAZ3VubWEtdS5hYy5qcA==