- 1College of Natural Resources and Environment, South China Agricultural University, Guangzhou, China
- 2Tanzania Agricultural Research Institute, Dodoma, Tanzania
- 3School of Resources and Planning, Guangzhou Xinhua University, Guangzhou, China
- 4ISTO, UMR 7327, CNRS-Université D’Orléans, Orléans, France
- 5Department of Civil and Environmental Engineering, Shantou University, Shantou, China
Food chain contamination by soil lead (Pb), beginning with Pb uptake by leafy vegetables, is a threat to food safety and poses a potential risk to human health. This study highlights the importance of two ecologically different earthworm species (the anecic species Amynthas aspergillum and the epigeic species Eisenia fetida) as the driving force of microbial hotspots to enhance Pb accumulation in the leafy vegetable Brassica campestris at different Pb contamination levels (0, 100, 500, and 1,000 mg·kg−1). The fingerprints of phospholipid fatty acids (PLFAs) were employed to reveal the microbial mechanism of Pb accumulation involving earthworm–plant interaction, as PLFAs provide a general profile of soil microbial biomass and community structure. The results showed that Gram-positive (G+) bacteria dominated the microbial community. At 0 mg·kg−1 Pb, the presence of earthworms significantly reduced the total PLFAs. The maximum total of PLFAs was found at 100 mg·kg−1 Pb with E. fetida inoculation. A significant shift in the bacterial community was observed in the treatments with E. fetida inoculation at 500 and 1,000 mg·kg−1 Pb, where the G+/G− bacteria ratio was significantly decreased compared to no earthworm inoculation. Principal component analysis (PCA) showed that E. fetida had a greater effect on soil microbial hotspots than A. aspergillum, thus having a greater effect on the Pb uptake by B. campestris. Redundancy analysis (RDA) showed that soil microbial biomass and structure explained 43.0% (R2 = 0.53) of the total variation in Pb uptake by B. campestris, compared to 9.51% of microbial activity. G− bacteria explained 23.2% of the total variation in the Pb uptake by B. campestris, significantly higher than the other microbes. The Mantel test showed that microbial properties significantly influenced Pb uptake by B. campestris under the driving force of earthworms. E. fetida inoculation was favorable for the G− bacterial community, whereas A. aspergillum inoculation was favorable for the fungal community. Both microbial communities facilitated the entry of Pb into the vegetable food chain system. This study delivers novel evidence and meaningful insights into how earthworms prime the microbial mechanism of Pb uptake by leafy vegetables by influencing soil microbial biomass and community composition. Comprehensive metagenomics analysis can be employed in future studies to identify the microbial strains promoting Pb migration and develop effective strategies to mitigate Pb contamination in food chains.
1. Introduction
As one of the biologically non-essential metals in soil, lead (Pb) is very toxic and easily bioaccumulates in organisms even at low concentrations. Pb pollution in leafy vegetable soils poses a serious threat to food safety (Zhou et al., 2021), as the leafy vegetable food chain is a major pathway of human exposure to soil Pb contamination (Wang et al., 2021; Ibrahim and Selim, 2022). Therefore, it is important to explore the mechanisms underlying Pb accumulation in leafy vegetables to ensure food safety. Chinese cabbage (Brassica campestris) is a popular leafy vegetable because of its high nutritional value with rich essential nutrients and metabolites (Keim et al., 2020; Liu et al., 2021) and antioxidant activity (Bhavithra et al., 2021; Wang et al., 2022). Approximately 1.5% of the total agricultural land area is used for vegetable cultivation in China. In 2020, vegetable production in China accounted for 30% of the world’s total production [Food and Agriculture Organization (FAO), 2020]. Investigation of Pb accumulation in leafy vegetables in Pb-contaminated soils can provide valuable insights into the potential risks associated with consuming Pb-contaminated produce and the need for effective soil monitoring and remediation strategies.
Metal bioaccumulation in plants and along the food chain mainly depends on the complex interactions between soil organisms and plants (Wu et al., 2020). Earthworm activities in soil influence Pb bioavailability and plant uptake (Jusselme et al., 2015a). Different earthworm species differ in their ecological strategies (Bottinelli et al., 2020; Huang et al., 2021), leading to different effects on Pb entry into the food chain. Moreover, many soil microbes are well-known to influence Pb bioavailability. These microbes include Pb-solubilizing bacteria, plant growth-promoting rhizobacteria, and microbes whose bioactive metabolites help to alleviate Pb phytotoxicity (Yahaghi et al., 2018; Al-Maliki and Al-Shamary, 2022). Studies showed that soil microbial properties influence metal accumulation in rice grains and brassica leaves (Xiao et al., 2017, 2020b). The effects of earthworms on soil microbial communities have been well-documented (Liu et al., 2019; Ahmed and Al-Mutairi, 2022), and the earthworm–microbe symbiotic relationship influences Pb transformation in soil and accumulation in plants (Das and Osborne, 2018). However, it remains unclear how ecologically different earthworm species influence Pb accumulation in leafy vegetables in relation to soil microbial properties.
Potential prospects for effective Pb accumulation in plants lie in revealing the mechanisms behind the earthworm–microbe interaction since these organisms form a discrete ecological unit and serve as the primary engines for terrestrial biogeochemical processes. Earthworms have a strong influence on soil microbial abundance and community composition, mainly in three ways. First, earthworm activities such as digging and casting change the soil microenvironment. Second, the feeding and grazing activities of earthworm lead to the selection of rapidly growing soil microbes through gut-associated processes. Third, microorganisms are dispersed in the soil after surviving the transit through the earthworm digestive tract (Medina-Sauza et al., 2019; Price-Christenson et al., 2020). Bacteria, actinomycetes (ACT), and fungi may contribute differently to plant Pb accumulation due to their different activity levels, abundances, and compositions (Yu et al., 2020; Mahohi and Raiesi, 2021). Nonetheless, soil enzymes secreted by earthworms and microbes are vital indicators of soil biochemical activities and soil quality (Xu et al., 2021). Recent studies reported increased Pb uptake by plants due to changes in soil microbial activities (Liu Y. et al., 2020; Zhang et al., 2022). Previous research on Pb contamination has generally focused on its effect on soil microbial characteristics such as microbial biomass, activity, and diversity (Stefanowicz et al., 2020; Xiao et al., 2020a). However, it is still unclear how different microbial characteristics influence Pb accumulation in plants. This knowledge gap restricts us from fully understanding the mechanisms underlying the effect of microbial hotspots on the rhizospheric soil and plant root functioning.
This study was focused on the earthworm–microbe interactive effect on Pb accumulation in plants. Two ecologically different earthworm species, including the anecic species Amynthas aspergillum and the epigeic species Eisenia fetida, were investigated. E. fetida is commonly found in organic matter-rich environments such as compost heaps and is known to influence the biogeochemical cycle of heavy metals such as Pb, which has a high affinity for organic matter. A. aspergillum, a deep-burrowing earthworm species, may affect microbial activity, Pb bioavailability, and Pb uptake by plants. In this study, we tested the following two hypotheses. (1) Ecologically different earthworm species differ in lifestyle, digestive system, and feeding preference and thereby exert different effects on soil microbial biomass, structure, and activity in Pb-polluted soils. (2) Pb accumulation in plants is driven by earthworm-induced changes in soil microbial community composition and activity. To test these two hypotheses, PLFA biomarkers were identified to provide information about the abundances of bacteria, ACT, and fungi and changes in soil microbial community structure. Additionally, fluorescein diacetate (FDA) hydrolysis was measured to evaluate the overall microbial activity in the soil, and the activities of β-glucosidase (β-glu) and N-acetylglucosaminidase (NAG) were determined to investigate the transformation of carbon (C) and nitrogen (N), respectively. The findings of this study will deepen our understanding of the complex interaction between earthworm ecotypes and soil microbes and its effect on Pb accumulation by leafy vegetables (e.g., B. campestris) grown in Pb-contaminated soils. In addition, this study will shed light on the soil microbial mechanism of Pb accumulation in the vegetable food chain.
2. Materials and methods
The soil used in this study was collected from the 0–20 cm layer of a fallow vegetable field (23°54′ N, 113°27′ E) in Qingyuan City, Guangdong Province, China. The basic properties of the soil were 6.06, 16.6 g·kg−1, 1.30 g·kg−1, and 12.8 for soil pH, organic C, total N, and C:N ratio, respectively, and total Pb was 20 mg·kg−1. Both A. aspergillum and E. fetida were purchased from a biofertilizer company in Qingyuan, Guangdong Province, China. The seeds of B. campestris were provided by the Guangdong Academy of Agricultural Sciences.
The experimental design was described by Tibihenda et al. (2022). The experiment included three earthworm treatments with four replicates. The soil was spiked with PbCl2 to set up four Pb contamination levels (i.e., 0, 100, 500, and 1,000 mg·kg−1) for each earthworm treatment and aged for 10 months before the experiment started. The three earthworm treatments were: (i) SP, no earthworm inoculation; (ii) SPA, A. aspergillum was inoculated; and (iii) SPE, E. fetida was inoculated. Each pot (18.5 cm × 12 cm × 16 cm) was filled with 1 kg of soil and planted with three healthy B. campestris seedlings at the same growth stage. For the SPA and SPE treatments, 23 ± 1 g of healthy clitellate adult earthworms, or four A. aspergillum (5.73 ± 0.40 g individual−1) and approximately 58 E. fetida (0.4 ± 0.1 g individual−1), were introduced into each pot. Soil moisture in each pot was maintained at field capacity. After 30 days of cultivation, the plants were harvested, washed, and oven-dried at 60°C. The earthworms in each pot were collected, counted, weighed, and put in Petri dishes with moisture filter papers at 25°C to empty their gastrointestinal tracts for 7 days. The soil from each pot was divided into two fractions. One was stored at 4°C for later PLFA analysis, and the other was air-dried and passed through a 0.149-mm sieve for organic C and total N determination or a 2-mm sieve for the determination of enzyme activities and other soil chemical properties.
2.1. Laboratory analyses
2.1.1. Determination of enzymatic activities
The colorimetric methods used for the determination of β-glu and NAG activities were based on the detection of p-nitrophenol (PNP), with PNP-β-D-glucopyranoside and PNP-N-acetyl-β-D-glucosaminide as substrates, respectively. The soil was incubated at 37°C, and pH 5.0 citrate buffer was used. The enzyme activities were expressed as micrograms of PNP released per gram of soil per hour under standard conditions (μg PNP·g−1 soil·h−1). Briefly, 2 g of air-dried soil with 0.5 mL of distilled water was incubated at 25°C for 48 h. After 3 mL of ice water was added and mixed thoroughly, 50 μL of the soil suspension was taken, mixed with 25 μL of buffer and 50 μL of the substrate solution, and incubated on an orbital shaker at 37°C for 1 h. The absorbance of the PNP released was measured using a microplate spectrophotometer reader at 405 nm (Mora et al., 2005).
The overall enzymatic activity in soil was assessed by FDA hydrolysis. Air-dried soil (2 g) was incubated with FDA solution in phosphate buffer (pH 7.0) at 25°C for 48 h. The yellow fluorescein released from FDA hydrolysis was quantified using a microplate spectrophotometer reader at 490 nm (Schnvrer, 1982). FDA hydrolysis activity was expressed as μg Fluo kg−1 soil h−1.
2.1.2. Determination of soil microbial biomass and community composition
PLFA fingerprints were employed as biomarkers to estimate soil microbial biomass and community composition. The PLFA technique involved the extraction of lipids from the soil and the separation of phospholipids from the lipids. The obtained phospholipids were then methylated to form fatty acid methyl esters, which were quantified by gas chromatography. To evaluate the extraction efficiency, an internal standard fatty acid (19:0) was used, and another fatty acid (10:0) was used as an instrumental standard for the gas chromatography analysis, which was added prior to detection and quantification (Au-Quideau et al., 2016). In this study, 14:0, 15:0, 16:0, 18:0, and i18:0 were used as the signature fatty acids for general bacteria (CB), 18:2ω6c and 18:1ω9c for fungi (F), and 10Me 16:0, 10Me 17:0, and 10Me 18:0 for ACT (Frostegård and Bååth, 1996). G+ bacteria were characterized by i14:0, i15:0, a15:0, i16:0, i17:0, and a17:0, whereas G− bacteria were characterized by 16:1ω7c, 18:1ω7c, cy17:0, and cy19:0. The total bacterial biomass (total bacteria) was calculated as the sum of G+ bacteria, G− bacteria, and CB bacteria. The ratio of fungal biomass to total bacterial biomass was used to estimate the ratio of fungi to bacteria in the soil (Moore-Kucera and Dick, 2008). The total content of PLFAs (total PLFAs) was determined as soil living microbial biomass and expressed as nmol·g−1 soil (Frostegård et al., 1996).
2.2. Statistical analysis
Data were processed using the SPSS statistical software (16.0). One-way ANOVA, Duncan’s multiple range test, and t-test were used to determine the significant differences in variables such as soil enzyme activities and PLFAs between treatments. Data were presented as mean ± standard deviation, and the significance level was set at a p-value of <0.05. Principal component analysis (PCA) was performed using the Ade4 package in R statistical software (4.2.3) to evaluate the relationships between soil microbial attributes in the earthworm–plant system. The vegan package in R was used for redundancy analysis (RDA) to explore the variation in contributions of microbial attributes to Pb bioavailability and accumulation in B. campestris. The raw data were standardized to eliminate the effects of different data dimensions. In the RDA, soil microbial attributes were treated as explanatory variables, whereas soil available Pb (DTPA-Pb), plant biomass, and plant Pb (concentration and accumulation) were selected as response variables. The Mantel correlation test was then used to evaluate the strength of the correlations between explanatory variables and response variables and to find out other interactions among them.
3. Results
3.1. Effects of earthworms on soil microbial biomass and structure in the Pb-contaminated soil
The total soil microbial biomass, which was measured as the total content of PLFAs, ranged from 12.5 to 22.1 nmol·g−1 soil across the treatments (Table 1). The maximum biomass was observed at 100 mg·kg−1 Pb. At 0 mg·kg−1 Pb, the presence of earthworms significantly reduced the total content of PLFAs, by 30.9% and 29.3% in SPE and SPA, respectively (p < 0.05). When the soil was contaminated with Pb, the total content of PLFAs in SPE increased significantly, whereas that in SP did not change much, and that in SPA only increased significantly at 100 and 500 mg·kg−1 Pb. For the same Pb contamination level, the total content of PLFAs was higher in SPE than in SPA, with the difference being significant at 100 and 1,000 mg·kg−1 Pb.
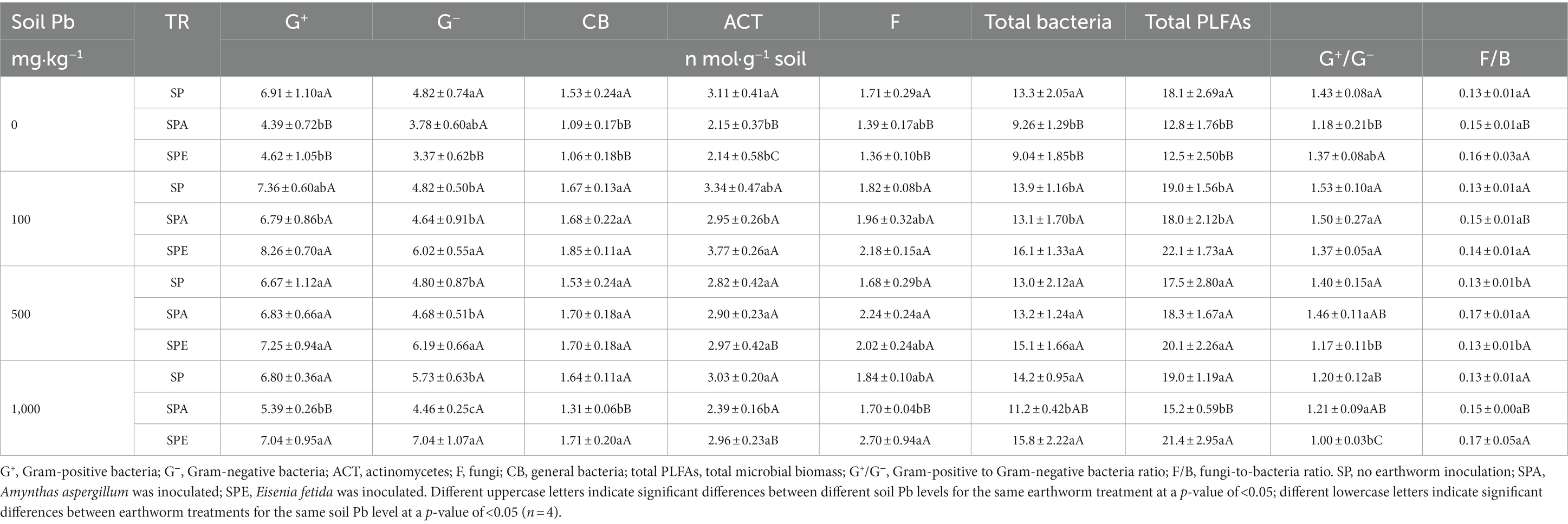
Table 1. Effects of earthworm inoculation on the biomasses of soil microbial communities and total PLFAs at different Pb contamination levels.
Generally, bacterial biomass (G+, G−, and CB) exhibited the same variation trend as the total content of PLFAs. In contrast, fungal and ACT biomasses showed different variation patterns. At 0 mg·kg−1 Pb, the presence of either earthworm species (A. aspergillum or E. fetida) significantly reduced ACT biomass, whereas only E. fetida significantly decreased fungal biomass compared to SP. In the Pb contamination treatments, ACT biomass was lower in SPA than in SPE, and the difference was statistically significant at 100 and 1,000 mg·kg−1 Pb. Both earthworm species increased fungal biomass at 100 and 500 mg·kg−1 Pb compared to SP. Noticeably, a significant difference between SPE and SPA was found at 1,000 mg·kg−1 Pb, where the fungal biomass in SPE was significantly higher (Table 1).
In this study, the proportions of specific soil microbial community biomass in total biomass revealed that the G+ bacteria had the highest relative abundance, ranging from 33% to 39%, whereas CB had the lowest relative abundance, ranging from 8% to 9% across the treatments. The relative abundances of the microbial communities followed the order of G+ > G− > ACT > F > CB (Table 1).
The G+/G− ratio ranged from 1.00 to 1.53 across the Pb levels (Table 1), with significantly higher values in SP compared to SPA or SPE except at 100 mg·kg−1 Pb. For the SPE treatment, the G+/G− ratio decreased with increasing Pb concentration in soil, with significant changes observed at 500 and 1,000 mg·kg−1 Pb. In contrast, the G+/G− ratio did not change consistently with increasing Pb concentration for the SPA treatment, with a significant difference only observed between 0 and 100 mg·kg−1 Pb (p < 0.05). The t-test showed that the G+/G− ratio was significantly higher in SPA than in SPE at 500 and 1,000 mg·kg−1 Pb (Figure 1A).
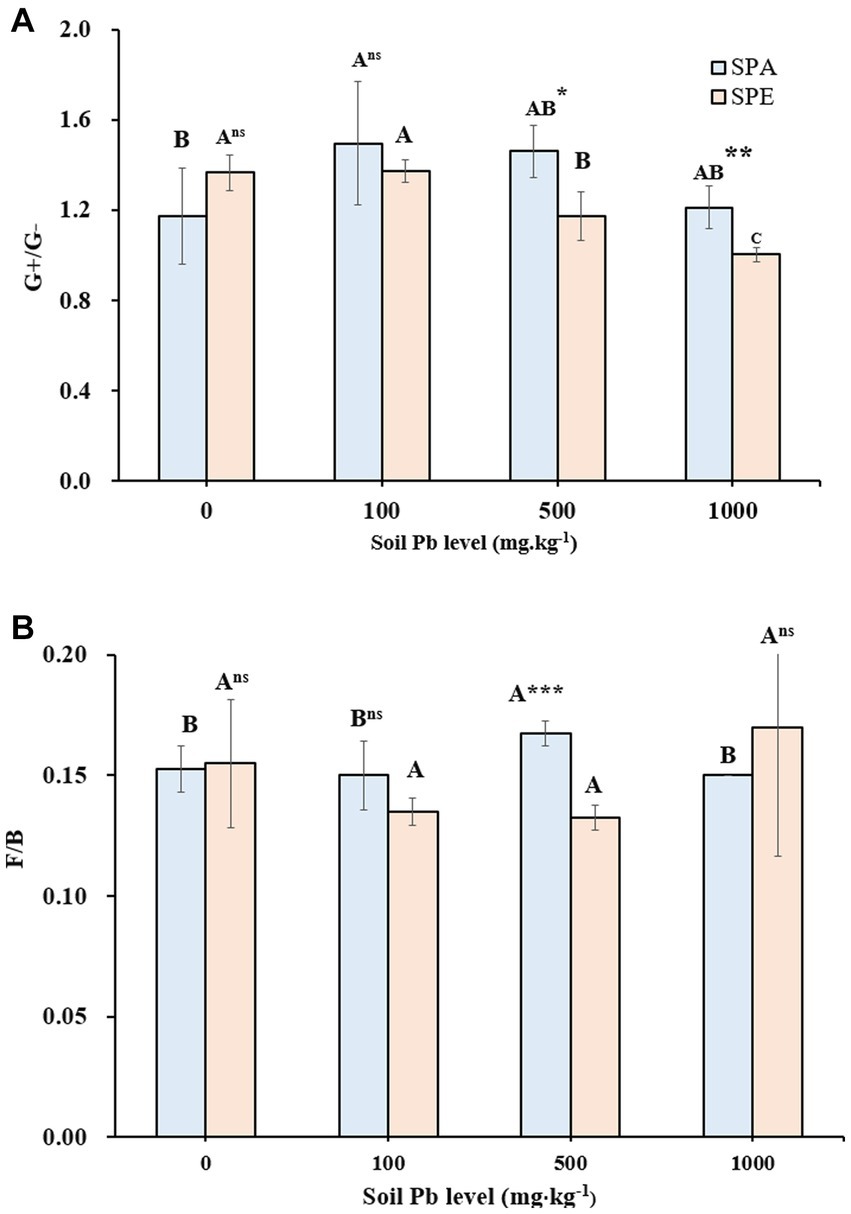
Figure 1. Gram-positive to Gram-negative bacteria ratio (G+/G−) and fungi-to-bacteria ratio (F/B) in the treatments with earthworm inoculation at different Pb contamination levels (A) G+/G− ratio (B) F/B ratio. SPA, Amynthas aspergillum was inoculated; SPE, Eisenia fetida was inoculated. Different letters indicate significant differences between different soil Pb levels for the same earthworm treatment at a p-value of <0.05. The t-test was used to compare the two earthworm treatments for the same soil Pb level, with ***p-value < 0.001, **p-value < 0.01, *p-value < 0.05, and ns p-value > 0.05 (n = 4).
The fungal to bacterial biomass (F/B) ratio ranged from 0.13 to 0.17, with higher values in SPA and SPE than in SP (Table 1). This indicates that the presence of earthworms tended to induce changes in the microbial community, and fungi seemed to benefit more from earthworm activities compared to bacteria. The t-test showed that at 500 mg·kg−1 Pb, the F/B ratio was significantly higher in SPA than in SPE (Figure 1B).
3.2. Effect of earthworms on soil microbial activities in the Pb-contaminated soil
Soil enzyme activities related to the cycling of two biologically essential elements, C and N, were studied at different levels of soil Pb contamination with or without the presence of earthworms. FDA hydrolysis, a measure of overall soil enzymatic activities, ranged from 6.70 to 223.4 μg Fluo kg−1 soil h−1, with the highest value observed in SP at 0 mg·kg−1 Pb and the lowest value also in SP but at 1000 mg·kg−1 Pb (Table 2). Generally, as the Pb concentration increased, FDA hydrolysis tended to decrease. For the same Pb contamination level, FDA hydrolysis was generally higher in SPA than in SPE, and the difference was significant at 500 mg·kg−1 Pb (p < 0.05). In general, the activities of β-glu and NAG were not affected by Pb contamination. NAG activity was stimulated by the presence of A. aspergillum at 100 and 500 mg·kg−1 Pb and by E. fetida at 100 and 1,000 mg·kg−1 Pb, whereas β-glu activity was generally inhibited by the presence of earthworms except in SPA at 500 mg·kg−1 Pb (Table 2).
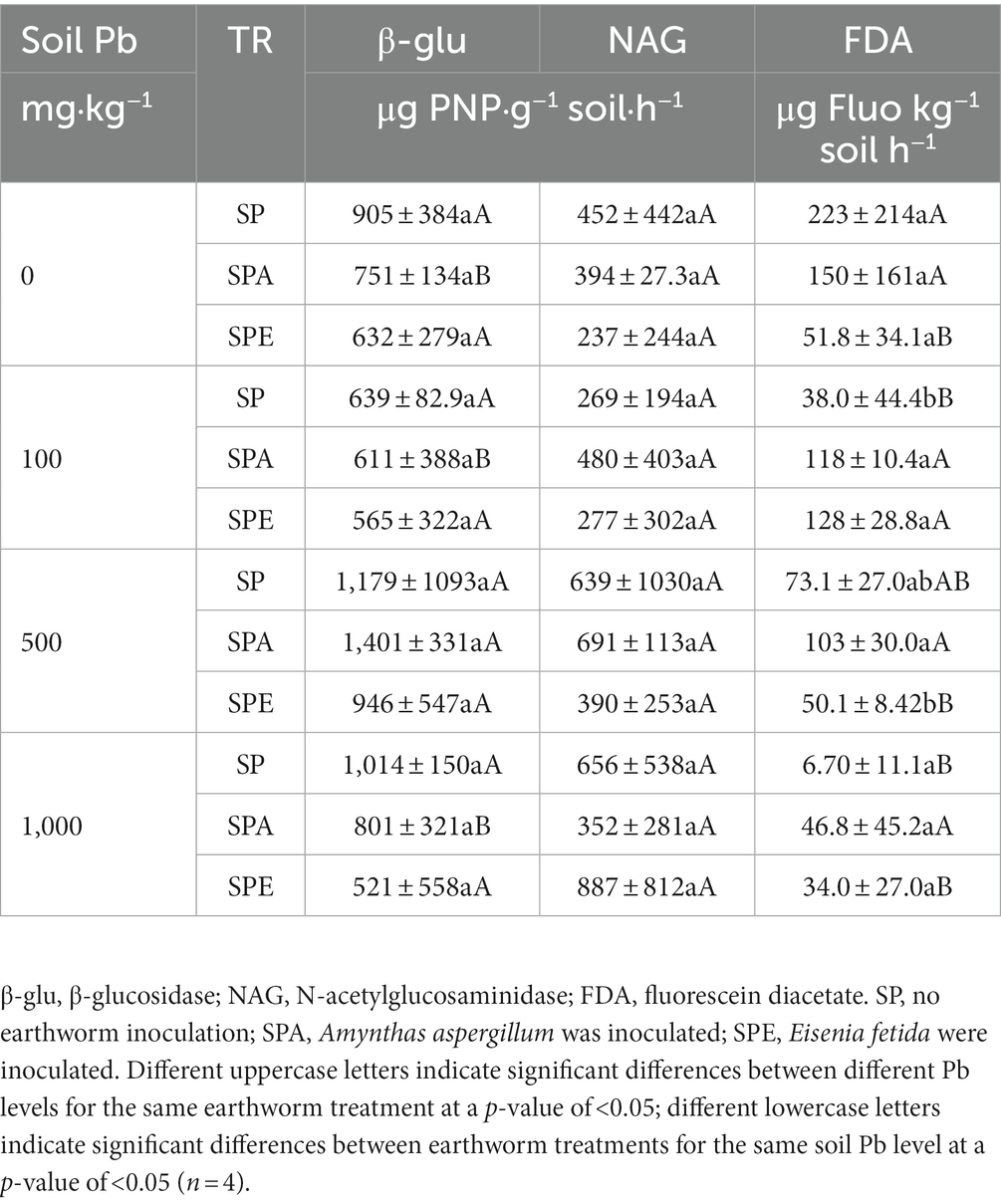
Table 2. Effects of earthworm inoculation on soil enzyme activities at different Pb contamination levels.
3.3. PCA of soil microbial attributes in the earthworm–plant system
PCA analysis revealed a clear separation of the soil microbial attributes between treatments (Figure 2). The first axis, which accounted for 51.6% of the explained inertia, indicated the effects of Pb contamination on soil microbial PLFAs (Figure 2C). Both the total content of PLFAs and the biomass of each microbial consortium were increased in the Pb-contaminated soil with the presence of E. fetida (p = 0.001). The second axis, representing 17.1% of the explained inertia, described microbial activities and changes in microbial community structure (F/B and G+/G−; Figure 2B; p = 0.012). The presence of A. aspergillum led to significant changes in microbial structure and FDA hydrolysis. Importantly, E. fetida inoculation was correlated with microbial attributes, suggesting its significant role in influencing soil microbial dynamics. The interspecies comparison of earthworms revealed the different effects of E. fetida and A. aspergillum on microbial attributes, which in turn affected Pb uptake by B. campestris in different ways.
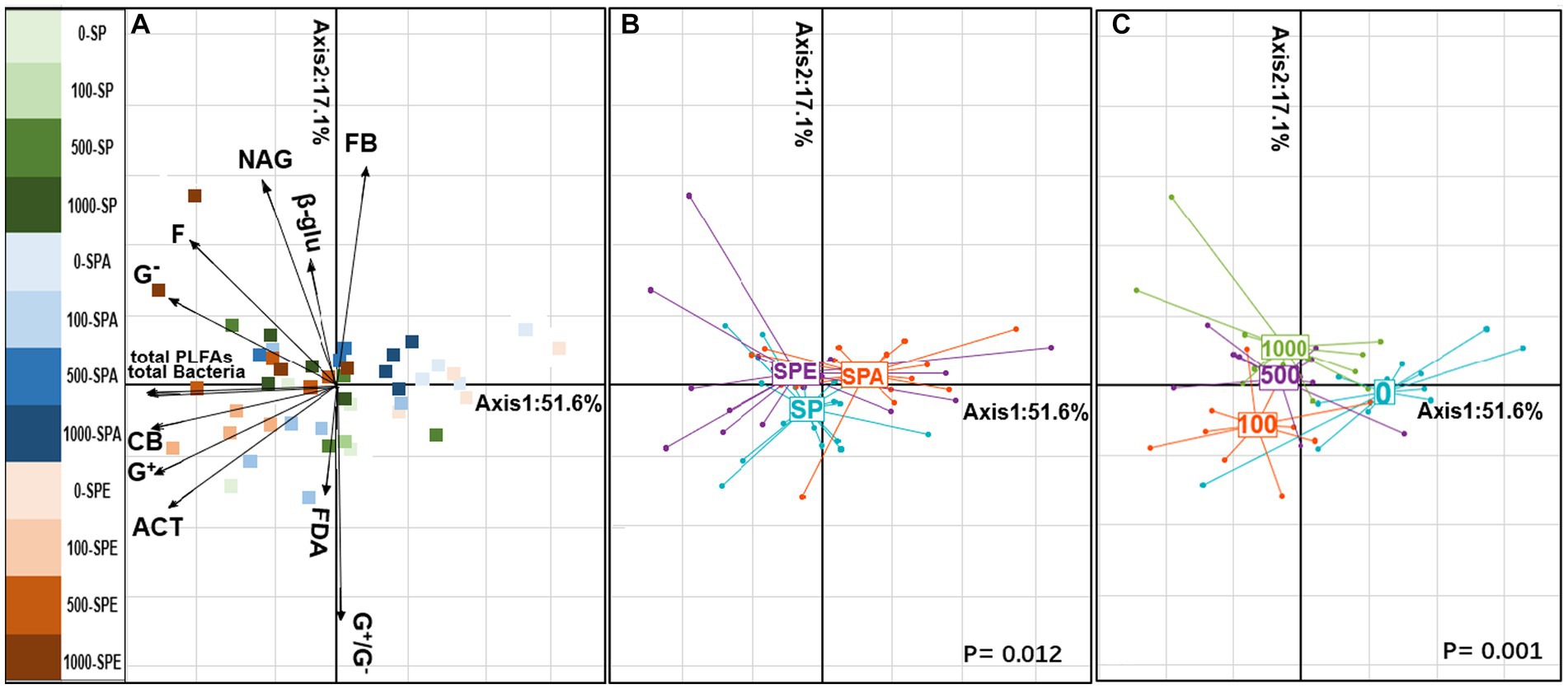
Figure 2. Principal component analysis (PCA) illustrating the distribution of soil microbial attributes in the earthworm–plant system (A) PCA projection of soil microbial properties with scattered treatment points. (B) Earthworm treatment plot. (C) Treatments of different Pb contamination levels of 0, 100, 500, and 1,000 mg kg−1 Pb. G+, Gram-positive bacteria; G−, Gram-negative bacteria; ACT, actinomycetes; F, fungi; CB, general bacteria; total bacteria, total bacterial biomass; and total PLFAs, total microbial biomass; G+/G−, Gram-positive to Gram-negative bacteria ratio; F/B, fungi-to-bacteria ratio; β-glu, β-glucosidase; NAG, N-acetylglucosaminidase; FDA, fluorescein diacetate. SP, no earthworm inoculation; SPA, Amynthas aspergillum was inoculated; SPE, Eisenia fetida was inoculated.
3.4. Effect of earthworm activity on the Pb uptake and growth of Brassica campestris
The Pb uptake by B. campestris in this study was examined by focusing on the following parameters: soil available Pb (DTPA-Pb), plant biomass, and plant Pb (concentration and accumulation). Earthworm inoculation led to a Pb increase in soil availability compared to SP. Different effects on plant biomass were observed, with E. fetida promoting and A. aspergillum inhibiting B. campestris growth. As a result of the higher plant biomass, the SPE treatment exhibited a higher Pb accumulation in B. campestris at 100, 500, and 1,000 mg·kg−1 Pb. In contrast, the SPA treatment exhibited a higher Pb concentration in B. campestris, especially at 500 and 1,000 mg·kg−1 Pb (Supplementary Table S1). Earthworm introduction had an effect on the physio-chemical properties of the Pb-contaminated soil, including pH, oxidation–reduction potential (Eh), total N, available N, organic C, dissolved organic C, and cation exchange capacity (Supplementary Table S2). The soil property changes highlighted the significant influence of earthworms on soil biogeochemical processes and Pb migration in the food chain.
3.5. Earthworm survival rate in the Pb-contaminated soil
The survival rate of A. aspergillum was consistently high, 81.3%, 87.5%, 100%, and 87.5% at 0, 100, 500, and 1,000 mg kg−1 Pb, respectively. The survival rates of E. fetida were 84.0%, 46.1%, 52.6%, and 62.3% at 0, 100, 500, and 1,000 mg kg−1 Pb, respectively. The consistently higher survival rate of A. aspergillum at all Pb levels demonstrated the greater tolerance of A. aspergillum to Pb contamination compared to E. fetida (Tibihenda et al., 2022).
3.6. Relationships between soil microbial attributes, plant biomass, and Pb in soil and plant
The RDA analysis showed that axes 1 and 2 explained 85.0% and 12.2% of the total variation in response variables, respectively (Figure 3A). The soil microbial attributes as explanatory variables significantly explained 52.5% of the variation in response variables (R2 = 0.53, Figure 3B). Specifically, soil microbial biomass and community structure explained 43.0% of the observed variation in response variables, whereas microbial activities explained 9.51%. The G− bacteria contributed 23.2% of the total variation in response variables, significantly higher than any other explanatory variable. FDA hydrolysis had a notably high contribution of 6.58%, making it the most prominent microbial activity variable. The ranking order of the top three explanatory variables based on their contributions to the total variation in response variables was as follows: G− bacteria (23.2%, p = 0.001***) > FDA hydrolysis (6.58%, p = 0.019*) > G+ bacteria (5.90%, p = 0.031*; Figure 3B). At the high Pb contamination levels (500 and 1,000 mg·kg−1), SPE showed strong linear correlations with many explanatory variables and response variables such as plant biomass, DTPA-Pb, and plant Pb accumulation, whereas SPA was correlated with F/B ratio, plant Pb concentration, and DTPA-Pb. In contrast, at low Pb contamination levels (0 and 100 mg·kg−1), all earthworm treatments (SP, SPA, and SPE) were associated with FDA hydrolysis and the G+/G− ratio. This implies that the increase in the G+/G− ratio was negatively correlated with microbial attributes and inhibited Pb uptake.
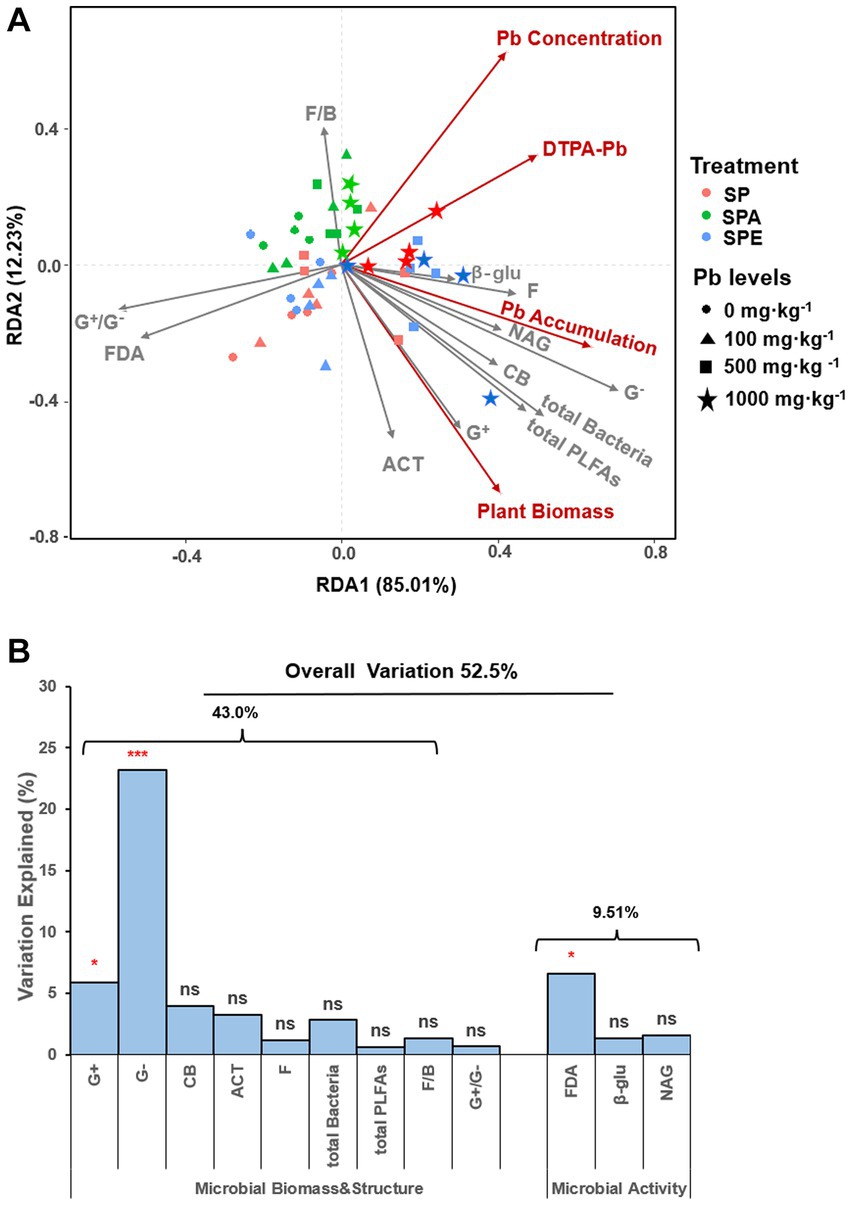
Figure 3. Redundancy analysis (RDA) of soil microbial attributes and plant Pb uptake in the different treatments (A) Diagram of RDA of soil microbial attributes as explanatory variables computed over response variables (plant biomass and Pb uptake). (B) Contribution of each microbial attribute to the variation in plant biomass and Pb uptake. G+, Gram-positive bacteria; G−, Gram-negative bacteria; ACT, actinomycetes; F, fungi; CB, general bacteria; total PLFAs, total microbial biomass; total bacteria, total bacterial biomass; G+/G−, Gram-positive to Gram-negative bacteria ratio; F/B, fungi-to-bacteria ratio; β-glu, β-glucosidase; NAG, N-acetylglucosaminidase; FDA, fluorescein diacetate, SP, no earthworm inoculation; SPA: A. aspergillum was inoculated; SPE, E. fetida was inoculated; soil Pb contamination levels include 0, 100, 500, and 1,000 mg kg−1 Pb. Permutation test was run 999 times to reveal the percentages of variation explained by the explanatory variables, the significance levels indicated by ***p-value < 0.001, **p-value < 0.01, *p-value < 0.05, no significant (ns), and p-value > 0.05.
The Mantel correlation test was utilized to examine the correlations between the explanatory and response variables (Figure 4). The bacterial communities, such as the G+ bacteria, G− bacteria, and CB, were significantly positively correlated with DTPA-Pb (p < 0.01), plant biomass (p < 0.001), Pb accumulation (p < 0.01), and Pb concentration (p < 0.05). ACT biomass was positively correlated with DTPA-Pb (p < 0.001), Pb accumulation (p < 0.01), plant biomass (p < 0.05), and Pb concentration (p < 0.05). Fungal biomass was positively correlated with DTPA-Pb only (p < 0.05). Total PLFAs and total bacteria were significantly positively correlated with all four response variables. Moreover, the G+/G− ratio was significantly correlated with Pb accumulation (p < 0.05), whereas the F/B ratio did not show a significant effect on the response variables. FDA hydrolysis was significantly correlated with DTPA-Pb (p < 0.01). Remarkably, β-glu and NAG activities were significantly correlated with soil microbes. For instance, the G− bacteria, CB, and fungi were correlated with NAG activity. Total PLFAs and total bacteria were significantly correlated with the constituent microbial communities and NAG activity. Some explanatory variables did not contribute significantly to the total variation in response variables but exhibited significant correlations in the Mantel test. These results support the finding of RDA and provide convincing proof that soil microbial attributes, particularly microbial biomass and community, affect Pb uptake by plants with the driving force of earthworms.
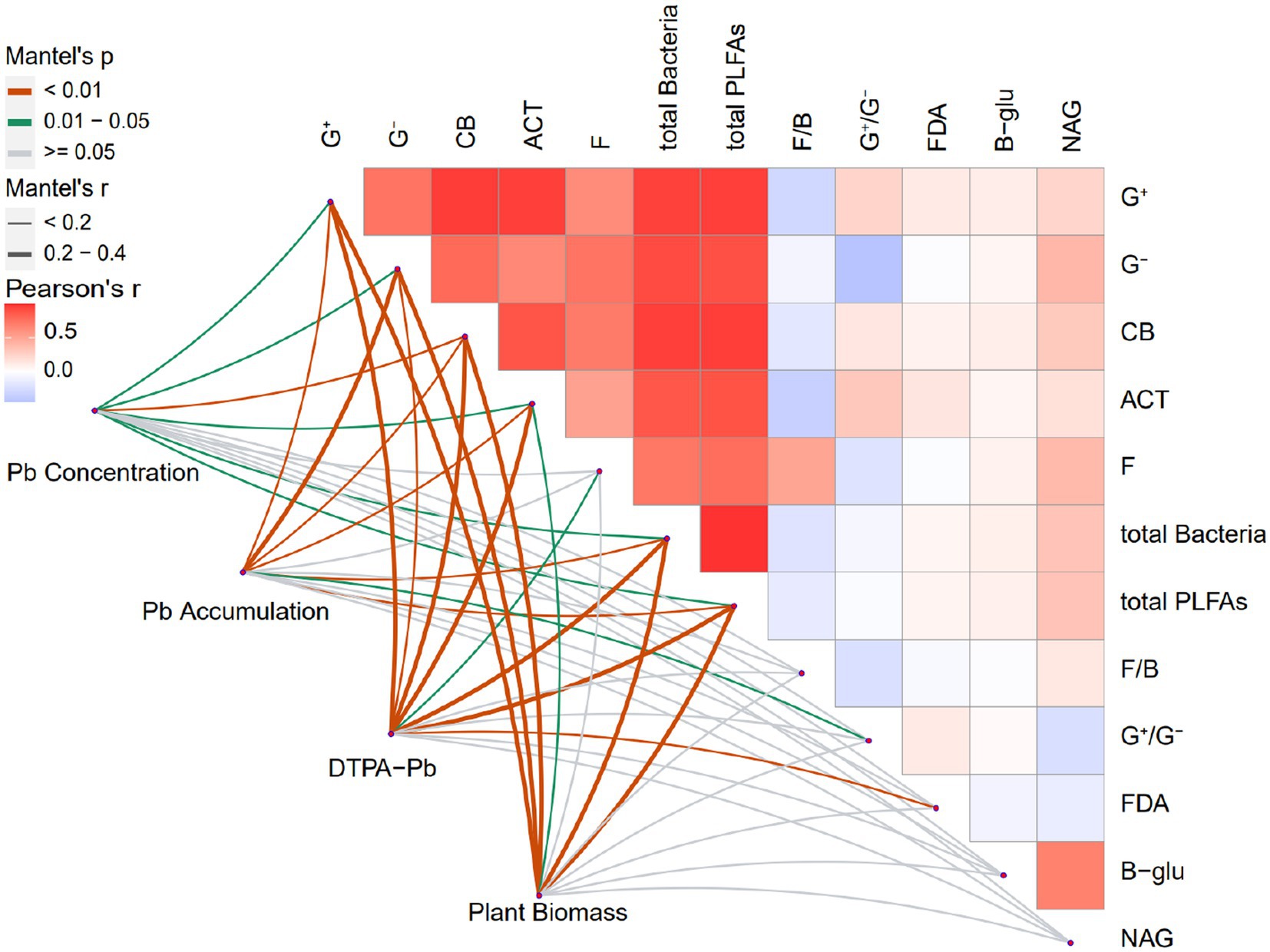
Figure 4. Mantel correlation analysis between soil microbial attributes and Pb uptake indicators in different treatments. G+, Gram-positive bacteria; G−, Gram-negative bacteria; ACT, actinomycetes; F, fungi; CB, general bacteria; total bacteria, total bacteria biomass; total PLFAs, total microbial biomass; G+/G−, Gram-positive to Gram-negative bacteria ratio; F/B, fungi to-bacteria ratio; β-glu, β-glucosidase; NAG, N-acetylglucosaminidase; FDA, fluorescein diacetate. SP, no earthworm inoculation; SPA, Amynthas aspergillum was inoculated; SPE, Eisenia fetida was inoculated. ns, not significant p-value >0.05, *p-value < 0.05, **p-value < 0.01, ***p-value < 0.001 (n = 4).
4. Discussion
4.1. Microbial property variations induced by earthworms in the Pb-contaminated soil
The earthworms had a greater influence on the microbial characteristics than on the chemical properties of the Pb-contaminated soil (Supplementary Figure S1). This finding aligns with previous research indicating that the behavioral and feeding traits of earthworms could lead to soil microbial community composition changes as well as soil enzyme activity changes (Omosigho et al., 2022).
At 0 mg·kg−1 Pb, the presence of earthworms dramatically reduced the total microbial biomass, which was due to the earthworms feeding on the microbes (Table 1; Edwards and Arancon, 2022). The microbes ingested by earthworms may be killed by the microbicidal substances in the gastrointestinal fluid or may not be able to survive the anaerobic conditions in the earthworm gut (Nechitaylo et al., 2010; Gómez-Brandón et al., 2011; Thakur et al., 2022). Although both G+ and G− bacterial biomasses were decreased by earthworm presence (Table 1; Figure 1A), the latter was less negatively influenced. This may be attributed not only to the presence of amino acids, mucosal saccharides, and soluble organic C but also to the neutral pH and anaerobic conditions in the earthworm gut, which are more favorable for the survival and growth of G− bacteria (Liu et al., 2018; Hu et al., 2020; Liu P. et al., 2020).
Soil Pb contamination deleteriously affects enzyme activities, microbial diversity, and microbial abundance (Xiao et al., 2020a; Sun et al., 2023). Pb contamination did not significantly affect the total microbial biomass in SP (Table 1; Figure 2), suggesting that the microbiome in soil may have developed effective Pb resistance and tolerance mechanisms (Mao et al., 2022), resulting in resilience in maintaining microbial biomass under Pb stress. Interestingly, the total microbial biomass was increased in SPE at 100, 500, and 1,000 mg·kg−1 Pb (Table 1). This may be because when exposed to high levels of Pb, E. fetida secretes a diverse range of substances such as defensive peptides called drilodefensins and other metabolites for Pb detoxification (Gudeta et al., 2023). These metabolites nourish the soil microbes, leading to an increase in total microbial biomass (Table 1). In addition, E. fetida prefers high-quality organic matter, which is a good energy source for soil microbes and thereby promotes a high microbial biomass (Lin et al., 2016; Sun et al., 2019; Yadav and Singh, 2023). Another reason for the increased microbial biomass that should not be ignored is the death of E. fetida due to Pb toxicity (Tibihenda et al., 2022), leading to less microbes being ingested and killed in the earthworm gut. The higher G+/G− ratios in SPA compared to SPE at 100, 500, and 1,000 mg·kg−1 Pb indicate that the presence of A. aspergillum might be more favorable for the G+ bacteria compared to E. fetida. The predominance of G+ bacteria can also be credited to their exceptional survival in Pb-contaminated environments (Biswas et al., 2021; Borozan et al., 2021). Earthworms generally promote soil fungal and ACT biomasses through their physical and biochemical activities (Medina-Sauza et al., 2019; Song et al., 2020). In contrast, Pb contamination has inhibitory effects on soil fungal and ACT biomasses (Xiao et al., 2020a,b). As a result, the combined effect of earthworm presence and Pb contamination led to complex changes in soil fungal and ACT biomasses.
Microbial activities in soil are biological fingerprints and reliable indicators of Pb toxicity (Luo et al., 2018). In contrast to the study of Yang et al. (2014), this study demonstrated a significant negative correlation between FDA hydrolysis and soil Pb contamination level in SP (Table 2; Figure 2). A high Pb level in the soil would lead to the denaturation of enzymes such as esterases, proteases, and lipases involved in FDA hydrolysis (Patle et al., 2018; Ramana et al., 2021; Li et al., 2022). The different feeding habits of different earthworm ecotypes can have different influences on microbial activity (Boughattas et al., 2019). This may be the reason why the presence of A. aspergillum led to a higher FDA hydrolysis activity than that of E. fetida. The toxic effect of Pb on soil enzyme activity is primarily due to its binding to the active sites of enzyme proteins (Dick, 1997; Utobo and Tewari, 2015). This suggests that the interface between Pb and enzyme active sites or substrates may be limited even at high soil Pb concentrations, resulting in minimal inhibition of enzyme activities responsible for soil N (NAG) and C (β-glu) transformation. Since a majority of enzymes are released by soil microbes (Wang et al., 2023), the presence and activity of the latter have an important effect on the activities of the former. In this study, soil enzyme activities, notably NAG activity, were enhanced by both earthworm species (Table 2), which was also found in a previous study (Jusselme et al., 2013), indicating the potential of earthworms to stimulate microbial activities in Pb-contaminated soils. In summary, the findings confirm our hypothesis that each earthworm ecotype has a unique effect on soil enzyme activities, microbial biomass, and community composition in Pb-contaminated soils due to its distinct lifestyle and feeding preference.
4.2. Influence of soil microorganisms on Pb uptake by Brassica campestris with earthworm inoculation
Earthworms influence soil microbial functioning, mitigate the toxic effect of metals on microbial population, structure, and diversity (Šrut et al., 2019; Ren et al., 2021), and promote metal transfer from soil to plant. The RDA results revealed that the soil microbial attributes significantly influenced Pb uptake by B. campestris, with a contribution of 52.5%, much higher than the 17% reported by Xiao et al. (2020b). Explaining 43% of the total variation in Pb uptake, soil microbial biomass and community structure had a greater influence on Pb transfer from soil to plant compared to microbial activity (Figure 3B). The increase in soil microbial biomass due to earthworm activities suggests a likely more diverse soil microbial community with a more diverse genetic pool for more diverse soil functions (Tardy et al., 2014; Li et al., 2018) to influence Pb uptake by plants. Pb-accumulating microbes might serve as a potential Pb reservoir for plant Pb uptake (Pham et al., 2022; Rajivgandhi et al., 2022). The G− bacteria in the drilosphere and rhizosphere might play an important role in the Pb uptake by B. campestris. They promote Pb uptake under the synergistic effects of plant root exudates and earthworms (Das and Osborne, 2018; WeiXie et al., 2022). G− bacteria release growth regulators, siderophores, exopolysaccharides, and organic acids, which change Pb bioavailability and boost plant growth by reducing Pb phytotoxic effects (Jusselme et al., 2015a; Alexandra et al., 2022). Although G+ bacteria were abundant in the Pb-contaminated soil and expected to have a major influence on Pb uptake, they only accounted for 5.90% of the total variation in Pb uptake. The possible reason could be the lack of functioning G+ bacterial strains that can influence Pb availability. Earthworm introduction altered fungal abundance, which was positively correlated with soil DTPA-Pb (Figure 4), emphasizing the noteworthy symbiotic colonization of plant roots by fungi to enhance soil Pb bioavailability (Manzoor et al., 2019). The correlations between fungal biomass and enzyme activities (Figure 4) indicate that soil fungi probably secrete enzymes in the rhizosphere and the drilosphere (Frey, 2019). As a result, enzymatic breakdown of organic and inorganic Pb-bearing substrates occurs, and DTPA-Pb increases, which explains the high Pb concentration in B. campestris (Supplementary Table S1; Figure 3A). The direct and indirect influences of ACT on Pb uptake by vegetables have rarely been reported. In this study, the earthworms had an effect on ACT biomass, and the latter was correlated with Pb uptake (Figure 4), which was consistent with previous studies (Ali et al., 2017; Liu X. et al., 2020). ACT are well-known to secrete various growth-promoting substances and secondary metabolites, such as metal chelators and organic acids, which promote plant growth and solubilize and mobilize metals, including Pb (Taj and Rajkumar, 2016; Shanthi, 2021). Therefore, ACT played a crucial role in promoting Pb uptake by B. campestris. This study provides evidence and new insights into the microbial mechanism of Pb uptake by B. campestris driven by earthworms via influencing soil microbial biomass and community structure. Comprehensive metagenomics analysis can be employed in future studies to identify the microbial strains promoting Pb uptake by plants.
The increased Pb accumulation in B. campestris was mainly a result of plant growth promotion enhanced by E. fetida (Figure 3A). Though biomass increase would lead to Pb concentration dilution (known as the growth dilution effect), the latter would stimulate Pb uptake, eventually resulting in higher Pb accumulation in B. campestris. Therefore, earthworms play an important role in promoting metal bioaccumulation and plant tolerance to heavy metal stress in contaminated soils (Mahohi and Raiesi, 2021). The decreased plant biomass in SPA led to higher Pb concentration compared to SPE. Moreover, some studies have demonstrated that plant Pb uptake is related to plant biomass, soil microbial biomass, and community structure (Jusselme et al., 2012, 2015b), which is consistent with our findings in the present study. E. fetida played a role in promoting Pb transfer in the vegetable food chain system by decreasing the G+/G− ratio (Table 1), which was associated with Pb uptake increase (Figure 3A). This finding supports the well-established idea that a change in microbial community structure is always accompanied by a change in microbial activity and community function (Price-Christenson et al., 2020). Furthermore, it was found that SPA exhibited high F/B ratios, DTPA-Pb, and plant Pb concentrations (Figures 1B, 3A). This indicates that A. aspergillum introduction tended to shift the microbial community toward being fungi-dominated, which can promote Pb transfer from the soil into the vegetable food chain by improving Pb bioavailability. These findings highlight the potential influence of earthworms on soil ecosystems and the entry of metals into the food chain.
5. Conclusion and perspective
Hitherto, studies have provided direct evidence of the cause–effect interaction between earthworms and microbes in the context of Pb accumulation in leafy vegetable systems. However, no study has been conducted to investigate how ecologically different earthworm species differ in promoting the entry of Pb into the food chain. In this study, both soil microbial biomass and community composition had a stronger effect than microbial activity on Pb transfer into the vegetable food chain. The possible soil microbial mechanism of Pb accumulation in B. campestris is driven by earthworms, which influence microbial biomass and community structure. In the Pb-polluted soil, the two ecologically different earthworm species exhibited different effects on soil enzyme activities, microbial biomass, and community composition due to their different lifestyles and feeding preferences. However, both earthworm species contributed to Pb uptake by B. campestris, which raises a concern about food safety. Understanding the interactions between soil microbes and Pb can help develop effective strategies to reduce Pb contamination in the food chain. Therefore, comprehensive metagenomics analysis can be employed in future studies to identify the microbial strains promoting Pb uptake in vegetable soil systems.
Data availability statement
The original contributions presented in the study are included in the article/Supplementary material, further inquiries can be directed to the corresponding author.
Ethics statement
The manuscript presents research on animals that do not require ethical approval for their study.
Author contributions
CT and HZ: writing-original draft, methodology, formal analysis, and data curation. KL: investigation and funding acquisition. JD: investigation and methodology. XL: data curation and investigation. MM-H: investigation and review and editing. SH and YL: review and editing. MZ and LX: methodology. CZ: conceptualization, methodology, formal analysis, data curation, writing—review and editing, funding acquisition, and project administration. All authors contributed to the article and approved the submitted version.
Funding
This study was financially supported by various funding sources, including the Natural Science Foundation of China (grant no. 41201305), the National Science and Technology Fundamental Resources Investigation Program of China (2018FY100300), the Guangdong provincial Natural Science Foundation (grant no. 2021A1515011543), the Guangdong provincial Agricultural Science and Technology Development and Resources and Environmental Protection Management Project (grant no. 2022KJ161), and the National Key Research and Development Program of China (grant nos. 2016YFD0201301 and 2016YFD0201200). This study was also supported by the China Scholarship Council in China and the scientific section of the French Embassy in Beijing.
Acknowledgments
The authors express their gratitude to Minghui Li and Yingying Cui for collecting soil samples.
Conflict of interest
The authors declare that the research was conducted in the absence of any commercial or financial relationships that could be construed as a potential conflict of interest.
Publisher’s note
All claims expressed in this article are solely those of the authors and do not necessarily represent those of their affiliated organizations, or those of the publisher, the editors and the reviewers. Any product that may be evaluated in this article, or claim that may be made by its manufacturer, is not guaranteed or endorsed by the publisher.
Supplementary material
The Supplementary material for this article can be found online at: https://www.frontiersin.org/articles/10.3389/fmicb.2023.1240707/full#supplementary-material
References
Ahmed, N., and Al-Mutairi, K. A. (2022). Earthworms effect on microbial population and soil fertility as well as their interaction with agriculture practices. Sustain. Sci. 14:7803. doi: 10.3390/su14137803
Alexandra, L., Hervé, C., Pierre, G., Jean-Yves, C., and Thierry, L. (2022). Effect of Pseudomonas putida-producing pyoverdine on copper uptake by Helianthus annuus cultivated on vineyard soils. Sci. Total Environ. 809:152113. doi: 10.1016/j.scitotenv.2021.152113
Ali, A., Guo, D., Mahar, A., Wang, Z., Muhammad, D., Li, R., et al. (2017). Role of Streptomyces pactum in phytoremediation of trace elements by Brassica juncea in mine polluted soils. Ecotoxicol. Environ. Saf. 144, 387–395. doi: 10.1016/j.ecoenv.2017.06.046
Al-Maliki, S., and Al-Shamary, A. (2022). Vital evidence for arbuscular mycorrhizal fungi, bacteria and cattail plant to remove Pb-cd heavy metals from contaminated soils. Acta Ecol. Sin. 42, 392–397. doi: 10.1016/j.chnaes.2022.05.008
Au-Quideau, S. A., McIntosh, A. C. S., Norris, C. E., Lloret, E., Swallow, M. J. B., and Hannam, K. (2016). Extraction and analysis of microbial phospholipid fatty acids in soils. JoVE 114:e54360. doi: 10.3791/54360
Biswas, R., Halder, U., Kabiraj, A., Mondal, A., and Bandopadhyay, R. (2021). Overview on the role of heavy metals tolerance on developing antibiotic resistance in both Gram-negative and Gram-positive bacteria. Archives of Microbiology 203, 2761–70. doi: 10.1007/s00203-021-02275-w
Bhavithra, P., Balakumbahan, R., Kannan, P., and Sivakumar, V. (2021). Underutilized leafy vegetables in western ghats region for nutritional security. Plant Arch. 21, 32–39. doi: 10.51470/Plantarchives.2021.v21.no1.005
Borozan, A. B., Misca, C. D., Morar, A., Morar, D., Obistioiu, A., Raba, D. N., et al. (2021). Soil pollution with heavy metals and bioremediation methods. Algae 104, 32. doi: 10.17930/AGL202115
Bottinelli, N., Hedde, M., Jouquet, P., and Capowiez, Y. (2020). An explicit definition of earthworm ecological categories – marcel bouché’s triangle revisited. Geoderma 372:114361. doi: 10.1016/j.geoderma.2020.114361
Boughattas, I., Hattab, S., Alphonse, V., Livet, A., Giusti-Miller, S., Boussetta, H., et al. (2019). Use of earthworms Eisenia andrei on the bioremediation of contaminated area in north of Tunisia and microbial soil enzymes as bioindicator of change on heavy metals speciation. J. Soil. Sediment. 19, 296–309. doi: 10.1007/s11368-018-2038-8
Das, A., and Osborne, J. W. (2018). Enhanced lead uptake by an association of plant and earthworm bioaugmented with bacteria. Pedosphere 28, 311–322. doi: 10.1016/S1002-0160(18)60021-9
Dick, R. P. (1997). “Soil enzyme activities as integrative indicators of soil health” in Biological indicators of soil health. Eds. Pankhurst C. E., Doube B. M., Gupta V. V. S. R. (CAB, Wallingford), 121–156.
Edwards, C. A., and Arancon, N. Q. (2022). “Interactions between earthworms, microorganisms, and other invertebrates” in Biology and ecology of earthworms (New York, NY: Springer US), 275–301.
Food and Agriculture Organization (FAO). (2020). FAOSTAT. FAO. Food and agriculture Organization of the United Nations, Rome, Italy. Available at: (http://www.fao.org/faostat/en/#home).
Frey, S. D. (2019). Mycorrhizal fungi as mediators of soil organic matter dynamics. Annu. Rev. Ecol. Evol. Syst. 50, 237–259. doi: 10.1146/annurev-ecolsys-110617-062331
Frostegård, A., and Bååth, E. (1996). The use of phospholipid fatty acid analysis to estimate bacterial and fungal biomass in soil. Biol. Fertil. Soils 22, 59–65. doi: 10.1007/BF00384433
Frostegård, Å., Tunlid, A., and Bååth, E. (1996). Changes in microbial community structure during long-term incubation in two soils experimentally contaminated with metals. Soil Biol. Biochem. 28, 55–63. doi: 10.1016/0038-0717(95)00100-X
Gómez-Brandón, M., Aira, M., Lores, M., and Domínguez, J. (2011). Epigeic earthworms exert a bottleneck effect on microbial communities through gut associated processes. PloS One 6:e24786. doi: 10.1371/journal.pone.0024786
Gudeta, K., Kumar, V., Bhagat, A., Julka, J. M., Bhat, S. A., Ameen, F., et al. (2023). Ecological adaptation of earthworms for coping with plant polyphenols, heavy metals, and microplastics in the soil: a review. Heliyon 2023:e14572. doi: 10.1016/j.heliyon.2023.e14572
Hu, J., Zhao, H., Wang, Y., Yin, Z., and Kang, Y. (2020). The bacterial community structures in response to the gut passage of earthworm (Eisenia fetida) feeding on cow dung and domestic sludge: Illumina high-throughput sequencing-based data analysis. Ecotoxicol. Environ. Saf. 190:110149. doi: 10.1016/j.ecoenv.2019.110149
Huang, C., Ge, Y., Yue, S., Qiao, Y., and Liu, L. (2021). Impact of soil metals on earthworm communities from the perspectives of earthworm ecotypes and metal bioaccumulation. J. Hazard. Mater. 406:124738. doi: 10.1016/j.jhazmat.2020.124738
Ibrahim, E. A., and Selim, E.-M. M. (2022). Pollution and health risk assessment of trace metal in vegetable field soils in the eastern nile delta, Egypt. Environ. Monit. Assess. 194:540. doi: 10.1007/s10661-022-10199-1
Jusselme, M. D., Miambi, E., Lebeau, T., and Rouland-Lefevre, C. (2015a). “Role of earthworms on phytoremediation of heavy metal-polluted soils” in In heavy metal contamination of soils: Monitoring and remediation. eds. I. Sherameti and A. Varma (Cham: Springer international publishing), 279–298.
Jusselme, M. D., Miambi, E., Mora, P., Diouf, M., and Rouland-Lefèvre, C. (2013). Increased lead availability and enzyme activities in root-adhering soil of Lantana camara during phytoextraction in the presence of earthworms. Sci. Total Environ. 445-446, 101–109. doi: 10.1016/j.scitotenv.2012.12.054
Jusselme, M. D., Poly, F., Lebeau, T., Rouland-lefèvre, C., and Miambi, E. (2015b). Effects of earthworms on the fungal community and microbial activity in root-adhering soil of Lantana camara during phytoextraction of lead. Appl Soil Ecol. 96, 151–158. doi: 10.1016/j.apsoil.2015.07.011
Jusselme, M. D., Poly, F., Miambi, E., Mora, P., Blouin, M., Pando, A., et al. (2012). Effect of earthworms on plant Lantana camara Pb-uptake and on bacterial communities in root-adhering soil. Sci. Total Environ. 416, 200–207. doi: 10.1016/j.scitotenv.2011.10.070
Keim, J. P., Gandarillas, M., Benavides, D., Cabanilla, J., Pulido, R. G., Balocchi, O. A., et al. (2020). Nutrient concentrations and profile of non-structural carbohydrates vary among different Brassica forages. Anim. Prod. Sci. 60, 1503–1513. doi: 10.1071/an19472
Li, L., Xu, M., Eyakub Ali, M., Zhang, W., Duan, Y., and Li, D. (2018). Factors affecting soil microbial biomass and functional diversity with the application of organic amendments in three contrasting cropland soils during a field experiment. PloS One 13:e0203812. doi: 10.1371/journal.pone.0203812
Li, H., Yao, J., Min, N., Chen, Z., Li, M., Pang, W., et al. (2022). Comprehensive evaluation of metal (loid) s pollution risk and microbial activity characteristics in non-ferrous metal smelting contaminated site. J. Clean. Prod. 344:130999. doi: 10.1016/j.jclepro.2022.130999
Lin, Z., Zhen, Z., Wu, Z., Yang, J., Zhong, L., Hu, H., et al. (2016). The impact on the soil microbial community and enzyme activity of two earthworm species during the bioremediation of pentachlorophenol-contaminated soils. J. Hazard. Mater. 301, 35–45. doi: 10.1016/j.jhazmat.2015.08.034
Liu, T., Chen, X., Gong, X., Lubbers, I. M., Jiang, Y., Feng, W., et al. (2019). Earthworms coordinate soil biota to improve multiple ecosystem functions. Curr. Biol. 29, 3420–3429.e5. doi: 10.1016/j.cub.2019.08.045
Liu, X., Guo, D., Ren, C., Li, R., Du, J., Guan, W., et al. (2020). Performance of Streptomyces pactum–assisted phytoextraction of cd and Pb: in view of soil properties, element bioavailability, and phytoextraction indices. Environ. Sci. Pollut. Res. 27, 43514–43525. doi: 10.1007/s11356-020-09842-6
Liu, D., Lian, B., Wu, C., and Guo, P. (2018). A comparative study of gut microbiota profiles of earthworms fed in three different substrates. Symbiosis 74, 21–29. doi: 10.1007/s13199-017-0491-6
Liu, W., Liu, Y., and Kleiber, T. (2021). A review of progress in current research on Chinese flowering cabbage (Brassica campestris L. ssp. chinensis var. utilis Tsen et lee). J. Elem. 26:2076. doi: 10.5601/jelem.2020.25.4.2076
Liu, Y., Sun, X., Li, S., Li, S., Zhou, W., Ma, Q., et al. (2020). Influence of green waste compost on Pb-polluted soil remediation, soil quality improvement, and uptake by Pakchoi cabbage (Brassica campestris L. ssp). Environ. Sci. Pollut. Res. 27, 7693–7701. doi: 10.1007/s11356-019-07505-9
Liu, P., Yang, Y., and Li, M. (2020). Responses of soil and earthworm gut bacterial communities to heavy metal contamination. Environ. Pollut. 265:114921. doi: 10.1016/j.envpol.2020.114921
Luo, Z., Ma, J., Chen, F., Li, X., and Zhang, S. (2018). Effects of Pb smelting on the soil bacterial community near a secondary lead plant. Int. J. Environ. Res. Public Health 15:1030. doi: 10.3390/ijerph15051030
Mahohi, A., and Raiesi, F. (2021). The performance of mycorrhizae, rhizobacteria, and earthworms to improve Bermuda grass (Cynodon dactylon) growth and Pb uptake in a Pb-contaminated soil. Environ. Sci. Pollut. Res. Int. 28, 3019–3034. doi: 10.1007/s11356-020-10636-z
Manzoor, M., Gul, I., Kallerhoff, J., and Arshad, M. (2019). Fungi-assisted phytoextraction of lead: tolerance, plant growth–promoting activities and phytoavailability. Environ. Sci. Pollut. Res. 26, 23788–23797. doi: 10.1007/s11356-019-05656-3
Mao, Y., Tan, H., Wang, M., Jiang, T., Wei, H., Xu, W., et al. (2022). Research progress of soil microorganisms in response to heavy metals in rice. J. Agric. Food Chem. 70, 8513–8522. doi: 10.1021/acs.jafc.2c01437
Medina-Sauza, R. M., Álvarez-Jiménez, M., Delhal, A., Reverchon, F., Blouin, M., Guerrero-Analco, J. A., et al. (2019). Earthworms building up soil microbiota, a review. Front. Environ. Sci. 7:81. doi: 10.3389/fenvs.2019.00081
Moore-Kucera, J., and Dick, R. P. (2008). PLFA profiling of microbial community structure and seasonal shifts in soils of a Douglas-fir chronosequence. Microb. Ecol. 55, 500–511. doi: 10.1007/s00248-007-9295-1
Mora, P., Miambi, E., Jiménez, J. J., Decaëns, T., and Rouland, C. (2005). Functional complement of biogenic structures produced by earthworms, termites and ants in the neotropical savannas. Soil Biol. Biochem. 37, 1043–1048. doi: 10.1016/j.soilbio.2004.10.019
Nechitaylo, T. Y., Yakimov, M. M., Godinho, M., Timmis, K. N., Belogolova, E., Byzov, B. A., et al. (2010). Effect of the earthworms Lumbricus terrestris and Aporrectodea caliginosa on bacterial diversity in soil. Microb. Ecol. 59, 574–587. doi: 10.1007/s00248-009-9604-y
Omosigho, H. O., Swart, E., Sizmur, T. P., Spurgeon, D. J., Svendsen, C., and Shaw, L. J. (2022). Assessing the efficacy of antibiotic treatment to produce earthworms with a suppressed microbiome. Eur. J. Soil Biol. 108:103366. doi: 10.1016/j.ejsobi.2021.103366
Patle, P., Navnage, N., and Barange, P. (2018). Fluorescein diacetate (FDA): measure of total microbial activity and as indicator of soil quality. Int. J. Curr. Microbiol. App. Sci. 7, 2103–2107. doi: 10.20546/ijcmas.2018.706.249
Pham, V. H. T., Kim, J., Chang, S., and Chung, W. (2022). Bacterial biosorbents, an efficient heavy metals green clean-up strategy: prospects, challenges, and opportunities. Microorganisms 10:610. doi: 10.3390/microorganism10030610
Price-Christenson, G. J., Johnston, M. R., Herrick, B. M., and Yannarell, A. C. (2020). Influence of invasive earthworms (Amynthas spp.) on Wisconsin forest soil microbial communities and soil chemistry. Soil Biol. Biochem. 149:107955. doi: 10.1016/j.soilbio.2020.107955
Rajivgandhi, G., Ramachandran, G., Chackaravarthi, G., Maruthupandy, M., Quero, F., Chelliah, C. K., et al. (2022). Metal tolerance and biosorption of Pb ions by Bacillus cereus RMN 1 (MK521259) isolated from metal contaminated sites. Chemosphere 308:136270. doi: 10.1016/j.chemosphere.2022.136270
Ramana, S., Tripathi, A. K., Bharati, K., Singh, A. B., Kumar, A., Sahu, A., et al. (2021). Tolerance of cotton to elevated levels of Pb and its potential for phytoremediation. Environ. Sci. Pollut. Res. 28, 32299–32309. doi: 10.1007/s11356-021-13067-6
Ren, C., Teng, Y., Chen, X., Shen, Y., Xiao, H., and Wang, H. (2021). Impacts of earthworm introduction and cadmium on microbial communities composition and function in soil. Environ. Toxicol. Pharmacol. 83:103606. doi: 10.1016/j.etap.2021.103606
Schnvrer, J. R. (1982). Fluorescein diacetate hydrolysis as a measure of total microbial activity in soil and litter. Appl. Environ. Microbiol. 43, 1256–1261. doi: 10.1128/aem.43.6.1256-1261.1982
Shanthi, V. (2021). “Actinomycetes: Implications and prospects in sustainable agriculture,” in Biofertilizers: Study and impact. Hoboken, NJ, USA: Wiley. 335–370.
Song, K., Sun, Y., Qin, Q., Sun, L., Zheng, X., Terzaghi, W., et al. (2020). The effects of earthworms on fungal diversity and community structure in farmland soil with returned straw. Front. Microbiol. 11:594265. doi: 10.3389/fmicb.2020.594265
Šrut, M., Menke, S., Höckner, M., and Sommer, S. (2019). Earthworms and cadmium – heavy metal resistant gut bacteria as indicators for heavy metal pollution in soils? Ecotoxicol. Environ. Saf. 171, 843–853. doi: 10.1016/j.ecoenv.2018.12.102
Stefanowicz, A. M., Kapusta, P., Zubek, S., Stanek, M., and Woch, M. W. (2020). Soil organic matter prevails over heavy metal pollution and vegetation as a factor shaping soil microbial communities at historical Zn–Pb mining sites. Chemosphere 240:124922. doi: 10.1016/j.chemosphere.2019.124922
Sun, X., Sun, M., Chao, Y., Shang, X., Wang, H., Pan, H., et al. (2023). Effects of lead pollution on soil microbial community diversity and biomass and on invertase activity. Soil Ecol. 5, 118–127. doi: 10.1007/s42832-022-0134-6
Sun, Y., Zhao, L., Li, X., Hao, Y., Xu, H., Weng, L., et al. (2019). Stimulation of earthworms (Eisenia fetida) on soil microbial communities to promote metolachlor degradation. Environ. Pollut. 248, 219–228. doi: 10.1016/j.envpol.2019.01.058
Taj, Z. Z., and Rajkumar, M. (2016). “Perspectives of plant growth-promoting actinomycetes in heavy metal phytoremediation” in Plant growth promoting actinobacteria: A new avenue for enhancing the productivity and soil fertility of grain legumes. Eds. Subramaniam, Gopalakrishnan, Arumugam, Sathya, and Rajendran (Singapore: Springer) 213–231.
Tardy, V., Mathieu, O., Lévêque, J., Terrat, S., Chabbi, A., Lemanceau, P., et al. (2014). Stability of soil microbial structure and activity depends on microbial diversity. Environ. Microbiol. Rep. 6, 173–183. doi: 10.1111/1758-2229.12126
Thakur, S., Lone, A., Yellaboina, S., Tambat, S., Yadav, A. N., Jain, S., et al. (2022). Metagenomic insights into the gut microbiota of Eudrilus eugeniae (Kinberg) and its potential roles in agroecosystem. Curr. Microbiol. 79:295. doi: 10.1007/s00284-022-02988-1
Tibihenda, C., Zhang, M., Zhong, H., Xiao, L., Wu, L., Dai, J., et al. (2022). Growth and Pb uptake of Brassica campestris enhanced by two ecological earthworm species in relation to soil physicochemical properties. Front. Environ. Sci. 10:889. doi: 10.3389/fenvs.2022.884889
Utobo, E., and Tewari, L. (2015). Soil enzymes as bioindicators of soil ecosystem status. Appl. Ecol. Environ. Res. 13, 147–169. doi: 10.15666/aeer/1301_147169
Wang, Z., Bao, J., Wang, T., Moryani, H. T., Kang, W., Zheng, J., et al. (2021). Hazardous heavy metals accumulation and health risk assessment of different vegetable species in contaminated soils from a typical mining city, Central China. Int. J. Environ. Res. Public Health 18:2617. doi: 10.3390/ijerph18052617
Wang, Y., Di, H., Cheng, W., Ren, G., Luo, S., Ma, J., et al. (2022). Variation in the main health-promoting compounds and antioxidant activity of different edible parts of purple flowering stalks (Brassica campestris var. purpuraria) and green flowering stalks (Brassica campestris var. campestris). Plan. Theory 11:1664. doi: 10.3390/plants11131664
Wang, Q., Jin, T., Fu, Y., Chen, B., Crotty, F., Murray, P. J., et al. (2023). Spatial change in glomalin-related soil protein and its relationships with soil enzyme activities and microbial community structures during urbanization in Nanchang, China. Geoderma 434:116476. doi: 10.1016/j.geoderma.2023.116476
WeiXie, L., Yang, R., Liu, B., Lei, N., Peng, S., Li, J., et al. (2022). Effects of Pb-, cd-resistant bacterium Pantoea sp. on growth, heavy metal uptake and bacterial communities in oligotrophic growth substrates of Lolium multiflorum lam. Environ. Sci. Pollut. Res. Int. 29, 50742–50754. doi: 10.1007/s11356-022-19180-4
Wu, Y., Chen, C., Wang, G., Xiong, B., Zhou, W., Xue, F., et al. (2020). Mechanism underlying earthworm on the remediation of cadmium-contaminated soil. Sci. Total Environ. 728:138904. doi: 10.1016/j.scitotenv.2020.138904
Xiao, L., Guan, D., Peart, M. R., Chen, Y., Li, Q., and Dai, J. (2017). The influence of bioavailable heavy metals and microbial parameters of soil on the metal accumulation in rice grain. Chemosphere 185, 868–878. doi: 10.1016/j.chemosphere.2017.07.096
Xiao, L., Yu, Z., Liu, H., Tan, T., Yao, J., Zhang, Y., et al. (2020a). Effects of cd and Pb on diversity of microbial community and enzyme activity in soil. Ecotoxicology 29, 551–558. doi: 10.1007/s10646-020-02205-4
Xiao, L., Zhang, C., Wang, G., Guan, D., Zhang, R., Chen, Y., et al. (2020b). Influencing pathways of soil microbial attributes on accumulation of heavy metals in brassica (Brassica campestris L. ssp.chinensis var.utilis Tsen et lee) leaves. Environ. Pollut. 262:114215. doi: 10.1016/j.envpol.2020.114215
Xu, Z., Yang, Z., Zhu, T., Shu, W., and Geng, L. (2021). Ecological improvement of antimony and cadmium contaminated soil by earthworm Eisenia fetida: soil enzyme and microorganism diversity. Chemosphere 273:129496. doi: 10.1016/j.chemosphere.2020.129496
Yadav, P. K., and Singh, N. S. (2023). Role of earthworms in soil health and variables influencing their population dynamic: a review. Pharma Innov J 12, 1961–1965.
Yahaghi, Z., Shirvani, M., Nourbakhsh, F., De La Pena, T. C., Pueyo, J. J., and Talebi, M. (2018). Isolation and characterization of Pb-solubilizing bacteria and their effects on Pb uptake by Brassica juncea: implications for microbe-assisted phytoremediation. J. Microbiol. Biotechnol. 28, 1156–1167. doi: 10.4014/jmb.1712.12038
Yang, J.-Y., He, Z.-L., Yang, X.-E., and Li, T.-Q. (2014). Effect of lead on soil enzyme activities in two red soils. Pedosphere 24, 817–826. doi: 10.1016/S1002-0160(14)60069-2
Yu, P., Sun, Y., Huang, Z., Zhu, F., Sun, Y., and Jiang, L. (2020). The effects of ectomycorrhizal fungi on heavy metals’ transport in Pinus massoniana and bacteria community in rhizosphere soil in mine tailing area. J. Hazard. Mater. 381:121203. doi: 10.1016/j.jhazmat.2019.121203
Zhang, Y., Huang, H., Yu, H., Zhan, J., Ye, D., Zheng, Z., et al. (2022). The changes of rhizosphere characteristics contributed to enhanced Pb accumulation in Athyrium wardii (hook.) Makino after nitrilotriacetic acid application. Environ. Sci. Pollut. Res. 29, 6184–6193. doi: 10.1007/s11356-021-15476-z
Keywords: earthworm, plant–soil system, Pb accumulation, microbial community structure, enzyme activity
Citation: Tibihenda C, Zhong H, Liu K, Dai J, Lin X, Motelica-Heino M, Hou S, Zhang M, Lu Y, Xiao L and Zhang C (2023) Ecologically different earthworm species are the driving force of microbial hotspots influencing Pb uptake by the leafy vegetable Brassica campestris. Front. Microbiol. 14:1240707. doi: 10.3389/fmicb.2023.1240707
Edited by:
Qiaoyun Huang, Huazhong Agricultural University, ChinaReviewed by:
Ronggui Tang, Zhejiang Agriculture and Forestry University, ChinaJingtao Wu, South China Botanical Garden (CAS), China
Copyright © 2023 Tibihenda, Zhong, Liu, Dai, Lin, Motelica-Heino, Hou, Zhang, Lu, Xiao and Zhang. This is an open-access article distributed under the terms of the Creative Commons Attribution License (CC BY). The use, distribution or reproduction in other forums is permitted, provided the original author(s) and the copyright owner(s) are credited and that the original publication in this journal is cited, in accordance with accepted academic practice. No use, distribution or reproduction is permitted which does not comply with these terms.
*Correspondence: Chi Zhang, zhangchi2012@scau.edu.cn
†These authors have contributed equally to this work