- Faculty of Life Science and Technology, Kunming University of Science and Technology, Kunming, Yunnan, China
Various interferon (IFN)-stimulated genes (ISGs), expressed via Janus kinase–signal transducer and activator of transcription (JAK-STAT) signaling pathway-stimulated IFNs to increase antiviral effects or regulate immune response, perform different roles in virus-infected cells. In recent years, a novel ISG, SHFL, which is located in the genomic region 19p13.2 and comprises two isoforms, has been studied as a virus-inhibiting agent. Studies have shown that SHFL suppressive effects on human immunodeficiency virus-1 (HIV), Zika virus (ZIKV), dengue virus (DENV), hepatitis C virus (HCV), Japanese encephalitis virus (JEV), porcine epidemic diarrhea virus (PEDV), Human enterovirus A71 (EV-A71) and Kaposi’s sarcoma-associated herpes virus (KSHV). SHFL interacts with various viral and host molecules to inhibit viral life circle and activities, such as replication, translation, and ribosomal frameshifting, or regulates host pathways to degrade viral proteins. In this review, we summarized the functional features of SHFL to provide insights to underlying mechanisms of the antiviral effects of SHFL and explored its potential function.
1 Introduction
In the world, human beings and other animals are affected by various viruses. Organisms exposed to virus could still maintain healthy condition through the self-defense system of the host, which monitors and provides antiviral ability against pathogens and further activates immune responses of host (Skariah et al., 2022). Interferons (IFNs) were first discovered in 1957 by Isaacs and Lindenmann (1988). Then, three IFN families, including type I (α), II (β), and III (γ) IFNs, have been known to play an effective role in cellular self-defense, forming a robust first-line innate defense of host. Although IFNγ has no notable reaction to most immune cells, IFNα and IFNβ are pivotal for antiviral activities (Durbin et al., 2013). Regardless, intracellular signaling cascades can be induced by all three types of IFN via Janus kinase–signal transducer and activator of transcription (JAK-STAT) pathway, triggering the expression of IFN-stimulated genes (ISGs) that exert multiplex biological and pathological functions (Chen et al., 2017).
The shiftless antiviral inhibitor of ribosomal frameshifting (SHFL) gene was firstly predicted as an ISG in 2011 (Schoggins et al., 2011), which is also named as C19orf66, FLJ11286, IRAV, UPF0515, and RyDEN. Two isoforms were constructed by eight exons of the SHFL gene. The longer transcript (876 nt, NM_018381.4) encodes 291 amino acids (isoform-1), and the shorter one (768 nt, NM_001308277.2) encodes 255 amino acids (isoform-2) that lacks 164th–199th amino acid compared with isoform-1. The protein structure prediction of isoform-1 of SHFL revealed eight α-helices, seven β-strands, a zinc-ribbon domain (112th–135th amino acid), a nuclear localization signal (NLS, 121st–137th amino acid), a nuclear export signal (261st–269th amino acid), and a coiled-coil motif at the C-terminus (261st–285th amino acid) (Figure 1; Suzuki et al., 2016). SHFL showed high expressing level in human liver and spleen tissues, whereas a lower expression is observed in the pancreas and heart.1 Because the liver and spleen are important for human immune defense and metabolism, the high expression of SHFL in these tissues may be associated with those functions.
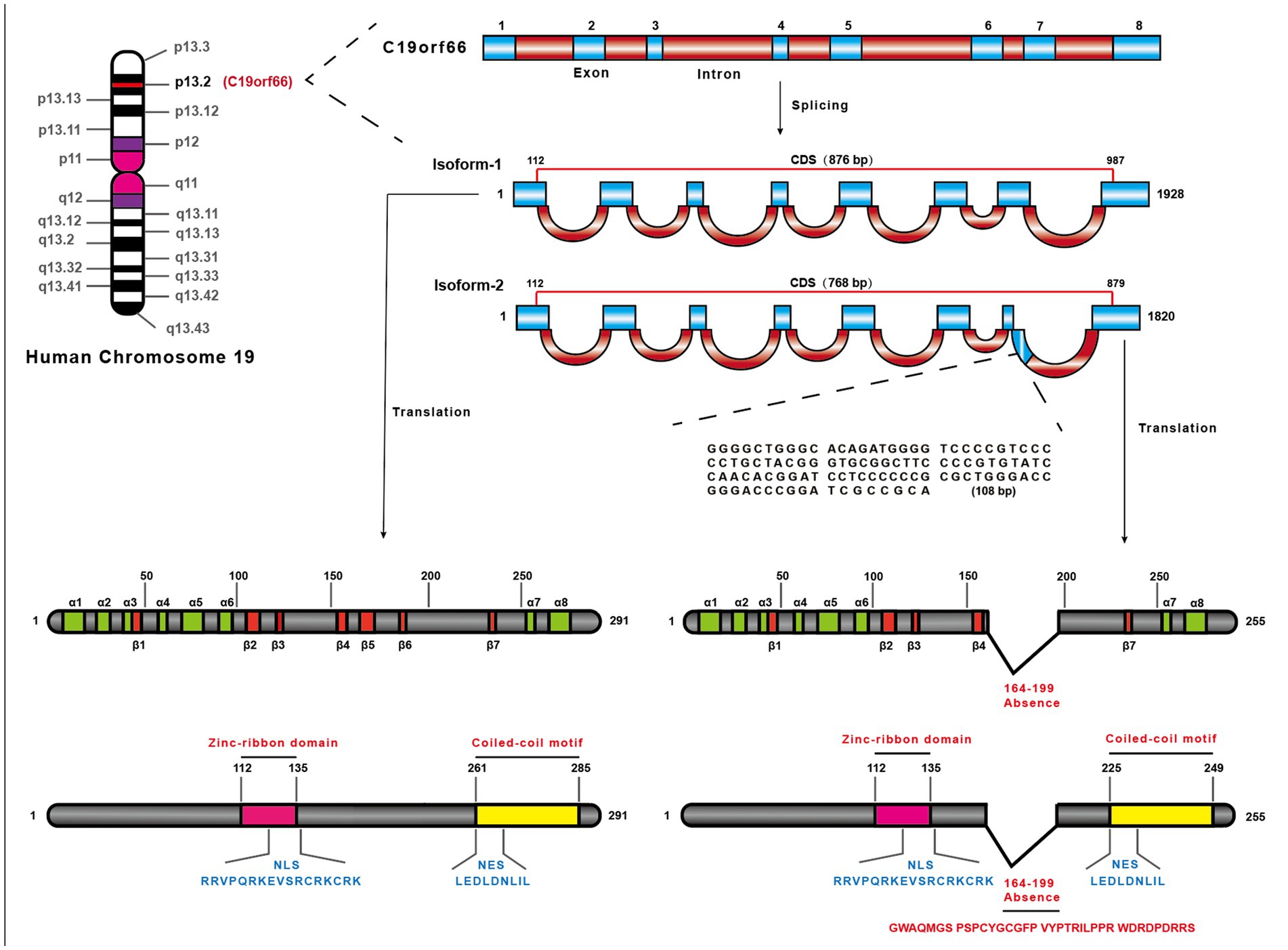
Figure 1. The location of SHFL on the human chromosome and structural characteristics. SHFL is situated on Human chromosome 19 the region p13.2, containing eight exons, and produces two isoforms caused by the alternative splicing. And the shorter one (768 bp) (NM_001308277.2), isoform-2, is different from isoform-1 (876 bp) (NM_018381.4) in the absence of 164–199 nucleotides on the seventh exon. Isoform-1 as the longer one, encoding 291 amino acids, also possesses some distinctions compared with isoform-2 which only encodes 255 amino acids. The prediction of the protein structure of isoform-1 of SHFL exposits that the isoform-1 consists of eight-α-helices and seven β-strands, and possesses the nuclear export signal (261–269 amino acids), nuclear localization signal (121–137 amino acids), a zinc-ribbon domain (112–135 amino acids), and a coiled-coil motif in C-terminus (261–285 amino acids). And the lack region of isoform-2 constitutes part of the fifth β-strand and the entire sixth β-strand.
SHFL was then detected with higher expressing level in cells infected by many viruses, such as immunodeficiency virus (HIV)-1, Zika virus (ZIKV), dengue virus (DENV), HCV, Japanese encephalitis virus (JEV), porcine epidemic diarrhea virus (PEDV), and Kaposi’s sarcoma-associated herpesvirus (KSHV), and was reported as a broad-spectrum virus-inhibiting ISG (Rodriguez and Muller, 2022). Because a protein structure could determine its function, the zinc-finger and coiled-coil domains of SHFL might be dominant to its diverse antiviral functions. In this review, we summarized the functional properties of SHFL and its underlying antiviral mechanisms against viruses with distinctively-characterized genomes to provide a theoretical basis for systematically understanding this gene.
2 The inhibiting function of SHFL to RNA viruses
Many viruses belong to RNA virus family, whose genomes are positive or negative RNA (Villa et al., 2020). However, SHFL seems to indulge in the viruses of the Flaviviridae family. SHFL plays important antiviral role in DENV, ZIKV, HCV, and JEV infection. Additionally, HIV, PEDV and EV-A71 also can be inhibited by SHFL (Rodriguez and Muller, 2022).
2.1 SHFL binds with complex of NS3 and NS4A to inhibit DENV
DENV is an enveloped, positive-sense, single-stranded RNA virus, covering four serotypes (DENV 1–4). DENV has promptly disseminated across many countries and regions worldwide, arousing dengue fever and dengue hemorrhagic fever in humans (Guzman and Harris, 2015). The RNA encodes three structural proteins including capsid (C), envelope (E) and membrane (M) and seven non-structural proteins, including NS1, NS2A, NS2B, NS3, NS4A, NS4B and NS5 (Kok et al., 2023).
SHFL could be up-regulated by DENV infection, and it interacts with complex of NS3 and NS4A to inhibit DENV replication. Moreover, DENV RNA also could be band by SHFL during translation (Suzuki et al., 2016; Balinsky et al., 2017). Additionally, some host molecules can also be associated with SHFL, such as poly(A) binding protein cytoplasmic 1 (PABPC1), which can stimulate the DENV replication, along with the P body and MOV10, which are both related to the stress response.
These studies showed the main process and molecules that can be affected by SHFL and the negative effect on DENV. Interestingly, the mutation of NLS domain in the SHFL gene lead to a decreasing binding level with DENV (Suzuki et al., 2016). The arginine (R) and lysine (K) are most abundant amino acid in NLS domain (Boulikas, 1994). Thus, mutation of R and K might change the SHFL anti-DENV activity by destroyed structure. Since the NLS domain is important for SHFL functioning, we speculated that the zinc-finger domain probably also plays a crucial role in restricting DENV due to the 15 amino acids shared between the two domains.
2.2 Against ZIKV through down-regulating NS3 of ZIKV
ZIKV, a member of the Flaviviridae family, is a positive-sense, single-stranded RNA virus. Similar to DENV, its genomic RNA encodes three structural proteins and seven nonstructural proteins (Sirohi and Kuhn, 2017). Notably, the proteins NS2, NS3, and NS5 consists of RNA-dependent RNA polymerase (RdRp) and are necessary for ZIKV replication (White et al., 2016; Wu et al., 2020).
SHFL can restrain the expression of ZIKV RNA and the viral particles, and further prevent ZIKV replication. When interacting with NS2B and NS3 proteins of ZIKV, SHFL downregulates NS3 through the lysosome-dependent pathway. Although SHFL can also suppress the ZIKV envelope protein, the mechanism is still unknown (Wu et al., 2020). Interestingly, knocking out of SHFL in mice increased the ZIKV RNA expressing level, demonstrating that the mouse ortholog of SHFL (Shfl) restricts ZIKV in vivo (Hanners et al., 2021).
SHFL still inhibited viral growth via its interaction ability, but it also affected NS3 protein via the lysosome-dependent pathway. This indicates that SHFL exert anti- ZIKV activity together with the lysosome-dependent signal pathway. In the Hanners’ study (Hanners et al., 2021), the results of Shfl against ZIKV in mouse model suggest a possibility of the similarity in the pivotal structure of SHFL and its ortholog. Thus, SHFL might also play antiviral roles in other species due to its conservative structure.
2.3 Damage of HCV membrane web by SHFL
HCV is a positive-sense, single-stranded RNA flavivirus, and its genomic compositions are similar to ZIKV and DENV. The NS proteins of HCV regulate viral replication and other activities (Darius and François, 2013). Remarkably, HCV NS5A can induce phosphatidylinositol-4-phosphate (PI[4]P) production, which can promote the formation of the membranous webs and provide a platform for HCV replication (Bishé et al., 2012; Blanchard and Roingeard, 2018).
SHFL co-locates in the cellular compartment with HCV replicase complex, and it could inhibit HCV replication by decreasing the NS5A level, which is a key factor in HCV replication. Additionally, SHFL inhibits HCV by reconstructing membranous web. Mutations in zinc-finger domain of SHFL reduce the anti-HCV activity, and it means that the zinc-finger domain is a pivotal structure for SHFL in anti-HCV role in the infected cells (Kinast et al., 2020). This indicated that zinc-finger domain might play important role in SHFL location. Recently, Shfl was also reported to own the anti-HCV ability in mouse model (Zhang et al., 2023). These studies suggest SHFL could effectively inhibit viruses in flavivirus family.
2.4 Restricting the Gag/Gag-Pol ratio of HIV
HIV is a member of the Lentivirus genus of the Retroviridae family, and possesses two uniform copies of single-stranded RNA. HIV (including HIV-1 and HIV-2) contains three representative structural genes: gag, pol, and env. The formation of the Gag and Pol proteins requires the cleavage of a large 160 kDa precursor molecule by a protease (Fanales-Belasio et al., 2010). HIV-1 Gag carries a−1PRF signal near its open reading frame (ORF) terminal and impels the occurrence of −1PRF, resulting in the formation of Gag–Pol complex. Precise regulation of the ratio of Gag to Gag–Pol is necessary for HIV assembly, genome packaging, and maturation (Wang et al., 2019).
Except for directly restricting the ratio of Gag to Gag–Pol, SHFL can interact with the ribosomal proteins, such as uL5, eS31, and eRF3, and the −1PRF signal-carrying RNA. This shows that SHFL, which also has a limiting effect on HIV-1 replication, can inhibit the −1PRF of HIV-1. The region for antiviral activity including the 164th–199th amino acid of SHFL, is required for HIV-1 inhibition and the binding activity of SHFL (Wang et al., 2019; Napthine et al., 2021). These results suggest that the zinc-finger domain of SHFL might produce a key effect in HIV infection by binding −1PRF and regulating the ratio of Gag/ Gag–Pol.
2.5 Inhibiting −1PRF transcription of JEV
JEV, a positive-sense, single-stranded RNA virus, is a zoonotic mosquito-borne flavivirus that can cause a series of infectious diseases of the central nervous system (Yun and Lee, 2013). The whole genome of JEV is 11 kb, including three structural proteins C, M, and E and nonstructural proteins (from NS1 to NS5). The JEV polyprotein can be cleaved by viral and host proteases (Sharma et al., 2021). The NS3 protein has a peculiar structure and multiple enzyme activities, which is also regarded as the constituent of the JEV RNA replicase and participates in the replication of JEV (Yu et al., 2021). Similar to Gag protein of HIV, production of NS1 protein depends on −1PRF (Chen et al., 1997).
SHFL can inhibit NS3 protein via the lysosome-dependent pathway and−1PRF by downregulating the expression of the NS1 protein and further decrease the JEV titer. However, the mRNA expressing level of NS3 or NS1 is not affected. Moreover, the zinc-finger domain of SHFL is still important in JEV inhibition (Yu et al., 2021). Together with Wang’s study (Wang et al., 2019), it seems that inhibit −1PRF progress is one of the main anti-viral mechanisms of SHFL.
2.6 Inducing degradation of PEDV N protein
PEDV is a single-stranded, positive-sense RNA virus, belonging to the genus Alphacoronavirus, family Coronaviridae, and the cause of porcine epidemic diarrhea (Sun et al., 2012). PEDV genome encodes four structural proteins (spike protein [S], envelope protein [E], membrane protein [M], and nucleocapsid protein [N]), 16 nonstructural proteins (nsp1–nsp16), and one accessory protein (ORF3) (Kocherhans et al., 2001). The N proteins play crucial roles in PEDV replication and transcription (Lin et al., 2022).
PEDV infection induces the early growth response gene 1 (EGR1) expression in the host cells, which can up-regulate SHFL expression at both protein and mRNA levels by directly binding to the SHFL promoter. The highly expressed SHFL can directly interact with the N protein and stimulate its degradation depending on the ubiquitin-proteasome and autolysosome pathways, and further inhibit PEDV replication. The anti- PEDV activity of SHFL are possibly related to the E3 ubiquitin ligase membrane-associated RING-CH8 (Wang et al., 2021).
2.7 Downregulating 3D protein of EV-A71 by SHFL
Human enterovirus A71 (EV-A71) belongs to Picornavirus family, which is a non-enveloped and single-strand RNA virus. The RNA encodes a polyprotein with three regions (P1, P2 and P3), which contains four structural proteins (VP1–VP4) and seven nonstructural proteins (2A, 2B, 2C, 3A, 3B, 3C, and 3D). The 3D protein of EV-A71 is an RNA-dependent polymerase determined the viral genome synthesis (Yuan et al., 2018). EV-A71 infection could lead to the hand–foot and mouth disease (HFMD), encephalitis, and aseptic meningitis worldwide (Huang and Shih, 2014; Kinobe et al., 2022).
Recently, EV-A71 is reported to facilitate the expression of SHFL (Tan et al., 2023). SHFL interacts with and downregulates viral 3D protein by the K48 ubiquitin–protease pathway. Then, EV-A71 propagation was inhibited. Tan et al. (2023) also found that the zinc-finger domain and 36 amino acids (164–199) were crucial for SHFL to bind with 3D protein.
Above all, SHFL showed wide-spectrum against RNA virus. It seems that some common anti-viral mechanisms are identified when SHFL inhibit RNA viruses, such as binding to RdRp protein of virus, suppressing −1PRF. In addition, SHFL might be induced not only by IFN but also by other factors, i.e., EGR1 (Woodson and Kehn-Hall, 2022).
3 The inhibition function of SHFL to DNA virus
3.1 Inhibiting expression of crucial genes in KSHV
KSHV, also known as human herpesvirus-8, is a Rhadinovirus of the gamma herpesvirus subfamily. During infection, the genome of this linearized double-stranded DNA virus circularizes to form an episome and remains present in the nucleus (Veettil et al., 2014). The life cycle of KSHV is divided into the two phases: the latency and the lytic phase. These two phases ensure KSHV could continuously survive in the host and infect to new individual (Broussard and Damania, 2020). The shutoff and exonuclease (SOX) protein of KSHV can degrade mRNAs in the host cells through targeting the special motif off most host mRNAs (Rodriguez et al., 2019).
SHFL contains a long stem-loop structure in the 3’UTR semblable with SOX resistance element, which could protect host mRNAs from destruction. In addition, SHFL can inhibit the KSHV replication and mobilize immune factors’ activities of host (Rodriguez et al., 2019, 2022). However, the anti-KSHV mechanisms of SHFL is not clear. We prefer to that SHFL inhibit KSHV through other pathways differed from that of anti-RNA virus. Although SHFL shows the activity to inhibit the viral titer of herpes simplex virus and adenovirus, but the anti-viral mechanisms are not studied (Suzuki et al., 2016).
3.2 Genetic association between the SHFL gene and hepatitis B virus (HBV)
HBV is an enveloped DNA virus belonging to the Hepadnaviridae family and causes advanced liver disease and hepatocellular carcinoma (Li et al., 2022). In our previous studies, the association between HBV infection in the Yunnan population and the genetic polymorphisms in the interleukin 28B, IFN lambda 4, myxovirus resistance (Mx)A, and MxB genes has been determined (Zhang et al., 2014; Zheng et al., 2022). Although there is no direct evidence that SHFL inhibits HBV proliferation, the genetic polymorphisms of the SHFL gene have been related to the biochemical indices of HBV patients in Yunnan. Inversely, the single nucleotide polymorphisms with different genotypes of SHFL might affect its expression by changing the association of transcriptional factors (Liu et al., 2023). This result displayed the relationship between HBV infection/ disease progression of HBV patients and the genetic characteristics of SHFL. However, whether SHFL could inhibit HBV replication need further study.
4 Future perspectives of SHFL
SHFL has garnered the attention of researchers owing to its particular structure and diverse virus-inhibiting function. In this review, we aimed to provide a comprehensive understanding of the antiviral functions of SHFL and the virus-associated specific molecules involved in inhibition processes. However, the following questions remain unanswered and need further study:
(i) Because most anti-viral studies of SHFL focus on the zinc-finger domain and 164th–199th amino acid, the function of other domains of SHFL are needed further investigate. The coiled-coil domain showed important roles in anti-viral activity of some ISGs, such as TRIM5α (Song, 2009). Thus, we speculate that the coiled-coil domain of SHFL might play important roles.
(ii) Both human SHFL and mouse Shfl gene showed wide-spectrum antiviral activities. Thus, this gene might be against zoonosis viruses.
(iii) Until now, although SHFL could inhibit both DNA viruses and RNA viruses, the species of DNA virus are rare. In addition, the anti-viral mechanisms are distinct among different viruses (Table 1), but it is still unclear in some virus infection. Thus, more studies should be further performed in various viruses.
In conclusion, we summarized the functional characteristics of SHFL. SHFL showed widely anti-viral spectrum in both DNA and RNA viruses. The studies suggest that the functional structure of SHFL is the zinc-finger domain, especial the 164th–199th amino acids. The anti-viral mechanisms of SHFL focus on inhibiting -1PRF, degrading RdRp of viruses, decreasing viral RNA expressing level. It is worth much to study the SHFL in future.
Author contributions
XW: Writing – original draft. A-MZ: Conceptualization, Writing – review & editing.
Funding
The author(s) declare financial support was received for the research, authorship, and/or publication of this article. This study was supported by the National Natural Science Foundation of China (32160148), and Yunnan Ten Thousand Talents Plan Young & Elite Talents Project.
Conflict of interest
The authors declare that the research was conducted in the absence of any commercial or financial relationships that could be construed as a potential conflict of interest.
Publisher’s note
All claims expressed in this article are solely those of the authors and do not necessarily represent those of their affiliated organizations, or those of the publisher, the editors and the reviewers. Any product that may be evaluated in this article, or claim that may be made by its manufacturer, is not guaranteed or endorsed by the publisher.
Footnotes
References
Balinsky, C. A., Schmeisser, H., Wells, A. I., Ganesan, S., Jin, T., Singh, K., et al. (2017). IRAV(FLJ11286), an interferon-stimulated gene with antiviral activity against dengue virus, interacts with MOV10. J. Virol. 91:e01606-16. doi: 10.1128/JVI.01606-16
Bishé, B., Syed, G., and Siddiqui, A. (2012). Phosphoinositides in the hepatitis C virus life cycle. Viruses 4, 2340–2358. doi: 10.3390/v4102340
Blanchard, E., and Roingeard, P. (2018). The hepatitis C virus-induced membranous web in liver tissue. Cells 7:191. doi: 10.3390/cells7110191
Boulikas, T. (1994). Putative nuclear localization signals (NLS) in protein transcription factors. J. Cell. Biochem. 55, 32–58. doi: 10.1002/jcb.240550106
Broussard, G., and Damania, B. (2020). Regulation of KSHV latency and lytic reactivation. Viruses 12:1034. doi: 10.3390/v12091034
Chen, C. J., Kuo, M. D., Chien, L. J., Hsu, S. L., Wang, Y. M., and Lin, J. H. (1997). RNA-protein interactions: involvement of NS3, NS5, and 3′ noncoding regions of Japanese encephalitis virus genomic RNA. J. Virol. 71, 3466–3473. doi: 10.1128/JVI.71.5.3466-3473.1997
Chen, K., Liu, J., and Cao, X. (2017). Regulation of type I interferon signaling in immunity and inflammation: a comprehensive review. J. Autoimmun. 83, 1–11. doi: 10.1016/j.jaut.2017.03.008
Darius, M., and François, P. (2013). “Hepatitis C virus proteins: from structure to function” in Hepatitis C virus: from molecular virology to antiviral therapy. Current topics in microbiology and immunology. ed. R. Bartenschlager (Berlin: Springer), 113–142.
Durbin, R. K., Kotenko, S. V., and Durbin, J. E. (2013). Interferon induction and function at the mucosal surface. Immunol. Rev. 255, 25–39. doi: 10.1111/imr.12101
Fanales-Belasio, E., Raimondo, M., Suligoi, B., and Buttò, S. (2010). HIV virology and pathogenetic mechanisms of infection: a brief overview. Annali Dell’stituto Superiore Di Sanità 46, 5–14. doi: 10.4415/ANN_10_01_02
Guzman, M. G., and Harris, E. (2015). Dengue. Lancet 385, 453–465. doi: 10.1016/S0140-6736(14)60572-9
Hanners, N. W., Mar, K. B., Boys, I. N., Eitson, J. L., De, P. C., Blake Richardson, R., et al. (2021). Shiftless inhibits flavivirus replication in vitro and is neuroprotective in a mouse model of Zika virus pathogenesis. Proc. Natl. Acad. Sci. 118:e2111266118. doi: 10.1073/pnas.2111266118
Huang, P.-J., and Shih, S. (2014). Update on enterovirus 71 infection. Curr. Opin. Virol. 5, 98–104. doi: 10.1016/j.coviro.2014.03.007
Isaacs, A., and Lindenmann, J. (1988). Virus interference: I. The interferon. CA Cancer J. Clin. 38, 280–290. doi: 10.3322/canjclin.38.5.280
Kinast, V., Plociennikowska, A., Anggakusuma, B., Bracht, T., Todt, D., Brown, R. J. P., et al. (2020). SHFL is an interferon-induced inhibitor of HCV replication that restricts formation of the viral replication organelle. J. Hepatol. 73, 549–558. doi: 10.1016/j.jhep.2020.03.047
Kinobe, R., Ageng Wiyatno, I., Artika, M., and Safari, D. (2022). Insight into the enterovirus A71: a review. Rev. Med. Virol. 32:e2361. doi: 10.1002/rmv.2361
Kocherhans, R., Bridgen, A., Ackermann, M., and Tobler, K. (2001). Completion of the porcine epidemic diarrhoea coronavirus (PEDV) genome sequence. Virus Genes 23, 137–144. doi: 10.1023/a:1011831902219
Kok, B. H., Lim, H. T., Lim, C. P., Lai, N. S., Leow, C. Y., and Leow, C. H. (2023). Dengue virus infection – a review of pathogenesis, vaccines, diagnosis and therapy. Virus Res. 324:199018. doi: 10.1016/j.virusres.2022.199018
Li, Q., Sun, B., Zhuo, Y., Jiang, Z., Li, R., Lin, C., et al. (2022). Interferon and interferon-stimulated genes in HBV treatment. Front. Immunol. 13:1034968. doi: 10.3389/fimmu.2022.1034968
Lin, F., Zhang, H., Li, L., Yang, Y., Zou, X., Chen, J., et al. (2022). PEDV: insights and advances into types, function, structure, and receptor recognition. Viruses 14:1744. doi: 10.3390/v14081744
Liu, N., Liu, M., Yang, J., Dong, S., Yue, M., Huang, P., et al. (2023). Association of genetic polymorphisms in the SHFL gene and biochemical indices of HBV infected individuals in Yunnan. Front. Cell. Infect. Microbiol. 13:1180366. doi: 10.3389/fcimb.2023.1180366
Napthine, S., Hill, C., Nugent, H. C. M., and Brierley, I. (2021). Modulation of viral programmed ribosomal frameshifting and stop codon readthrough by the host restriction factor shiftless. Viruses 13:1230. doi: 10.3390/v13071230
Rodriguez, W., Mehrmann, T., Hatfield, D., and Muller, M. (2022). Shiftless restricts viral gene expression and influences RNA granule formation during Kaposi’s sarcoma-associated herpesvirus lytic replication. J. Virol. 96:e0146922. doi: 10.1128/jvi.01469-22
Rodriguez, W., and Muller, M. (2022). Shiftless, a critical piece of the innate immune response to viral infection. Viruses 14:1338. doi: 10.3390/v14061338
Rodriguez, W. J., Srivastav, K., and Muller, M. (2019). SHFL broadly escapes virus-induced endonuclease cleavage and restricts Kaposi’s sarcoma-associated herpesvirus. J. Virol. 93:e00373-19. doi: 10.1128/JVI.00373-19
Schoggins, J. W., Wilson, S. J., Panis, M., Murphy, M. Y., Jones, C. T., Bieniasz, P., et al. (2011). A diverse range of gene products are effectors of the type I interferon antiviral response. Nature 472, 481–485. doi: 10.1038/nature09907
Sharma, K. B., Vrati, S., and Kalia, M. (2021). Pathobiology of Japanese encephalitis virus infection. Mol. Asp. Med. 81:100994. doi: 10.1016/j.mam.2021.100994
Sirohi, D., and Kuhn, R. J. (2017). Zika virus structure, maturation, and receptors. J. Infect. Dis. 216, S935–S944. doi: 10.1093/infdis/jix515
Skariah, S., Sultan, A. A., and Mordue, D. G. (2022). IFN-induced cell-autonomous immune mechanisms in the control of intracellular protozoa. Parasitol. Res. 121, 1559–1571. doi: 10.1007/s00436-022-07514-7
Song, B. (2009). “TRIM5alpha” in HIV interactions with host cell proteins. Current topics in microbiology and immunology. eds. P. Spearman and E. O. Freed (Berlin: Springer), 47–66.
Sun, R.-Q., Cai, R.-J., Chen, Y.-Q., Liang, P.-S., Chen, D.-K., and Song, C.-X. (2012). Outbreak of porcine epidemic diarrhea in suckling piglets, China. Emerg. Infect. Dis. 18, 161–163. doi: 10.3201/eid1801.111259
Suzuki, Y., Chin, W.-X., Han, Q., Ichiyama, K., Lee, C. H., Eyo, Z. W., et al. (2016). Characterization of RyDEN (SHFL) as an interferon-stimulated cellular inhibitor against dengue virus replication. PLoS Pathog. 12:e1005357. doi: 10.1371/journal.ppat.1005357
Tan, C., Qin, X., Tan, Y., Dong, X., Chen, D., Liang, L., et al. (2023). SHFL inhibits enterovirus A71 infection by triggering degradation of viral 3Dpol protein via the ubiquitin–proteasome pathway. J. Med. Virol. 95:e29030. doi: 10.1002/jmv.29030
Veettil, M., Bandyopadhyay, C., Dutta, D., and Chandran, B. (2014). Interaction of KSHV with host cell surface receptors and cell entry. Viruses 6, 4024–4046. doi: 10.3390/v6104024
Villa, T. G., Abril, A. G., Sánchez, S., de Miguel, T., and Sánchez-Pérez, A. (2020). Animal and human RNA viruses: genetic variability and ability to overcome vaccines. Arch. Microbiol. 203, 443–464. doi: 10.1007/s00203-020-02040-5
Wang, H., Kong, N., Jiao, Y., Dong, S., Sun, D., Chen, X.-Y., et al. (2021). EGR1 suppresses porcine epidemic diarrhea virus replication by regulating IRAV to degrade viral nucleocapsid protein. J. Virol. 95:e0064521. doi: 10.1128/JVI.00645-21
Wang, X., Xuan, Y., Han, Y., Ding, X., Ye, K., Yang, F., et al. (2019). Regulation of HIV-1 gag-pol expression by shiftless, an inhibitor of programmed −1 ribosomal frameshifting. Cells 176, 625–635.e14. doi: 10.1016/j.cell.2018.12.030
White, M. K., Wollebo, H. S., David Beckham, J., Tyler, K. L., and Khalili, K. (2016). Zika virus: an emergent neuropathological agent. Ann. Neurol. 80, 479–489. doi: 10.1002/ana.24748
Woodson, C. M., and Kehn-Hall, K. (2022). Examining the role of EGR1 during viral infections. Front. Microbiol. 13:1020220. doi: 10.3389/fmicb.2022.1020220
Wu, Y., Yang, X., Yao, Z., Dong, X., Zhang, D., Hu, Y., et al. (2020). SHFL interrupts Zika virus replication by inducing lysosomal degradation of viral NS3. PLoS Negl. Trop. Dis. 14:e0008083. doi: 10.1371/journal.pntd.0008083
Yu, D., Zhao, Y., Pan, J., Yang, X., Liang, Z., Xie, S., et al. (2021). SHFL inhibits Japanese encephalitis virus replication by targeting -1 PRF and the NS3 protein. Virol. Sin. 36, 1443–1455. doi: 10.1007/s12250-021-00423-6
Yuan, J., Shen, L., Wu, J., Zou, X., Gu, J., Chen, J., et al. (2018). Enterovirus A71 proteins: structure and function. Front. Microbiol. 9:286. doi: 10.3389/fmicb.2018.00286
Yun, S.-I., and Lee, Y.-M. (2013). Japanese encephalitis: the virus and vaccines. Hum. Vaccin. Immunother. 10, 263–279. doi: 10.4161/hv.26902
Zhang, Y., Kinast, V., Sheldon, J., Frericks, N., Todt, D., Zimmer, M. M., et al. (2023). Mouse liver-expressed shiftless is an evolutionarily conserved antiviral effector restricting human and murine hepaciviruses. Microbiol. Spectr. 11:e0128423. doi: 10.1128/spectrum.01284-23
Zhang, A.-M., Ma, K., Song, Y., Wang, B., Feng, Y., Liu, L., et al. (2014). Genetic polymorphisms of the IFNλ genes are associated with biochemical features in Han Chinese with HCV infection from Yunnan Province, China. Infect. Genet. Evol. 21, 161–165. doi: 10.1016/j.meegid.2013.11.013
Keywords: interferon-stimulated genes, SHFL, antiviral effects, RNA viruses, ribosomal frameshifting
Citation: Wang X and Zhang A-M (2023) Functional features of a novel interferon-stimulated gene SHFL: a comprehensive review. Front. Microbiol. 14:1323231. doi: 10.3389/fmicb.2023.1323231
Edited by:
Peirong Jiao, South China Agricultural University, ChinaReviewed by:
Ram Kumar, Guru Angad Dev Veterinary and Animal Sciences University, IndiaYouichi Suzuki, Osaka Medical and Pharmaceutical University, Japan
Copyright © 2023 Wang and Zhang. This is an open-access article distributed under the terms of the Creative Commons Attribution License (CC BY). The use, distribution or reproduction in other forums is permitted, provided the original author(s) and the copyright owner(s) are credited and that the original publication in this journal is cited, in accordance with accepted academic practice. No use, distribution or reproduction is permitted which does not comply with these terms.
*Correspondence: A-Mei Zhang, zam1980@yeah.net