- 1Guizhou Institute of Mountain Resources, Guiyang, Guizhou, China
- 2Guizhou Agricultural Ecology and Resource Protection Station, Agriculture and Rural Affairs Department of Guizhou Province, Guiyang, Guizhou, China
Soft rot caused by Botryosphaeria dothidea is a serious fungal disease in kiwifruit observed during the storage period, and it seriously restricts the healthy and stable development of the kiwifruit industry. In the present study, the bacterial strain LMY3-5 with high antifungal activity was isolated from healthy kiwifruit tissues. Based on gyrA and 16S rRNA sequences, a phylogenetic tree was constructed, and LMY3-5 was identified as Bacillus velezensis. The 16% cell-free supernatant (CFS) of LMY3-5 suppressed B. dothidea spore germination and mycelial growth by 97.32%. The 16% CFS of LMY3-5 could effectively inhibit the expansion of kiwifruit soft rot in vitro, and the inhibition rate was 73.59%. The scanning electron microscopy (SEM) observations of B. dothidea mycelia treated with the 16% CFS for 12 h showed that the mycelia were curved, wrinkled, and sunken. Moreover, transmission electron microscopy (TEM) observations revealed blurred boundaries of mycelial cell walls as well as plasmolysis and vacuolization. Propidium iodide (PI) staining showed that the CFS treatment damaged the cell membrane of B. dothidea and affected its permeability, which led to leakage of the nucleic acids and proteins. Simultaneously, a substantial increase in the activity of key enzymes (i.e., β-1,3-glucanase and chitinase) was observed, which indicated damage to the cell wall function of B. dothidea. GC/LC–MS analysis revealed the presence of 27 antimicrobial compounds. Thus, the LMY3-5 strain showed great potential as a biocontrol agent for soft rot disease in kiwifruit.
1 Introduction
Kiwifruit is a commonly consumed fruit worldwide that originated in China (Huang, 2016), and it is rich in nutrients and contains a variety of essential amino acids, dietary fibers, minerals, and vitamins (e.g., vitamin C, vitamin E, and vitamin K) (Aneta and Paulina, 2018; Lian et al., 2019; Wang et al., 2022; Wojdylo et al., 2017; Zhu et al., 2019). Kiwifruit is widely cultivated in many countries, such as New Zealand, China, Chile, and Italy (Nazir et al., 2024), and the growth of the kiwifruit industry has led to annual increases in its planting area. However, various diseases have become increasingly prominent with expansion in the production scale. They include kiwifruit canker (Kim et al., 2019), soft rot (Guo et al., 2024), crown gall (He et al., 2022), bloom blight (Balestra et al., 2009), and leaf spot disease (Chen et al., 2022).
Kiwifruit soft rot is a major disease that affects production in China (Li et al., 2017), Korea (Kwon et al., 2011), Italy (Luongo et al., 2011), and Chile (Diaz et al., 2014). The key pathogens responsible for soft rot in kiwifruit are the fungi Diaporthe eres and Botryosphaeria dothidea (Diaz et al., 2017; Zhou et al., 2015). The disease mainly affects fruit during storage and manifests as changes in the color of the fruit, which turns from yellowish green to dark green, and the texture that becomes soft, rotten, and concave. Spraying chemical antifungal agents such as carbendazim and tebuconazole before harvest is a practical and inexpensive method of preventing kiwifruit decay (Kim et al., 2013; Shin et al., 2021). However, long-term application of chemical antifungal agents may induce drug fungicide resistance in pathogens; in addition, excessive pesticide residues can cause environmental pollution and public health problems (Bakirci et al., 2014; Yin et al., 2023). Increasing awareness of food safety and environmental protection demand environmentally friendly, efficient, and safe disease prevention and control measures.
Biological control agents have emerged as an efficient and environmentally safe method of preventing pathogen infections in fruits after harvest (Jongman et al., 2022; Shi et al., 2024; Torres-Palazzolo et al., 2024). The most successful and widely used biocontrol bacteria are Bacillus spp., which can synthesize antibacterial molecules, including bacteriocins, cell wall-disintegrating enzymes, lipopeptide antibiotics, and peptides (Hamdache et al., 2011; Awad et al., 2012). Lipopeptides can destroy the membranes and walls of fungal cells, thus causing intracellular content leakage (Cawoy et al., 2015; Zou et al., 2018). In addition, Bacillus may synthesize growth-promoting substances, such as auxins, gibberellins, indole-3-acetic acid (IAA), and cytokinins (Meng et al., 2016; Kanjanamaneesathian et al., 2013). The antifungal activity of Bacillus may be exerted via the release of microbial agents, which can be used in the preparation of antifungal films and microbial fertilizers. Moreover, these bacteria can also effectively control diseases in vegetables and fruits after harvest.
In this study, we isolated a strain of Bacillus (LMY3-5) from “Guichang” kiwifruit that showed strong antifungal activity against B. dothidea. The purpose of this study was to (1) identify the species of Bacillus strains; (2) analyze the in vitro and in vivo antifungal properties and antifungal mechanisms of the LMY3-5 cell-free supernatant (CFS); and (3) detect the antifungal metabolites of strain LMY3-5.
2 Materials and methods
2.1 Microorganisms
In 2023, strain LMY3-5 was isolated from kiwifruit in the molecular laboratory of the Guizhou Provincial Institute of Mountain Resources, and it has since been preserved in the Chinese Microbial Strain Storage Management Center (CGMCC 29700). Strain LMY3-5 was grown on 100 mL Luria-Bertani (LB) medium at 30°C and 200 rpm for 12 h to prepare the inoculum.
Five plant pathogenic fungi, B. dothidea, Diaporthe eres, Monilinia fructicola, Alternaria alternata, and Colletotrichum gloeosporioides, were isolated and identified at the molecular laboratory of Guizhou Provincial Institute of Mountain Resources. The pathogens were grown on potato dextrose agar (PDA) medium at 25°C for 5–7 days.
2.2 In vitro antagonism assay
The antagonistic activity of strain LMY3-5 against five pathogens was tested by using a dual culture technique (Yan et al., 2020). Mycelial discs (5 mm diameter) of B. dothidea, D. eres, M. fructicola, and A. alternata were placed in the middle of fresh PDA plates (90 mm). Strain LMY3-5 was inoculated at a distance of 2 cm from the mycelial disc of the fungal pathogen. Control plates were inoculated only with the mycelial discs of fungal pathogens. All experiments were carried out three times, and each treatment included three replicates of the number of plates. All media plates were kept in an incubator for 5 days at 28°C. Subsequently, the colony size (diameter) was measured to calculate the inhibition rate.
Inhibition rate (%) = [(dcontrol − dtreatment) / dcontrol] × 100.
2.3 Identification of strain LMY3-5
Strains LMY3-5 were streaked on the LB plate and cultured overnight in an incubator at 37°C. The plate was observed to determine the shape, color, size, and other characteristics of the colonies. The colonies were subjected to Gram staining and observed and imaged under a Leica DM4 B microscope.
Microbial DNA was isolated from each antifungal strain utilizing a Biomiga Bacteria DNA extraction kit (Sangon Biotech Co., Ltd.) and employed as the PCR template. 16S rRNA (Weisburg et al., 1991) and gyrA (Chun and Bae, 2000) sequences of the bacterial strains were amplified using PCR primers 27F/1492R and gyrA-42f/gyrA-1066r, respectively, and the obtained amplicons were outsourced for sequencing to Sangon Bioengineering Co., Ltd. (Shanghai, China). The sequencing results were analyzed by drawing comparisons against the GenBank database, and highly homologous sequences were downloaded as reference sequences. Bayesian inference (BI) and maximum likelihood (ML) techniques were employed using CIPRES ScienceGateway V.3.3 (Miller et al., 2010) to prepare a phylogenetic tree. The obtained tree file was viewed and resized in FigTree v.1.4.0 and exported as a PDF file.
2.4 Impact of bacterial cell-free supernatant on the growth of pathogenic fungi
The cell-free supernatant (CFS) was prepared using protocols given in a previous study (Liu et al., 2023). Strain LMY3-5 was cultured in LB medium at 30°C and 200 rpm for 1 day and used as the seed culture. Then, 5 mL of seed culture was further cultured in LB medium (100 mL) at 30°C and 200 rpm for 3 days. The supernatant was subjected to centrifugation (12,000 rpm for 15 min) and filtration (0.22 μm sterile filter) to obtain the CFS. The obtained CFS was mixed with molten PDA medium (45 ± 5°C) to a final concentration of 1, 2, 4, 8, and 16% (vol/vol) and poured into sterile petri dishes. PDA plates without the CFS were used as controls. The fungal plug was placed at the center of the PDA plate and incubated at 25°C for 3 days. All experiments were carried out three times, and each treatment included three replicates of the number of plates. After measuring the colony size, the inhibition rate was determined as discussed in section 2.1.
2.5 Influence of CFS on spore germination of B. dothidea
Conidia formed on the B. dothidea plate were scraped and diluted with sterile water to a concentration of 1 × 106 mL−1. The obtained CFS was mixed with the spore suspension to a final concentration of 0 (as control), 1, 2, 4, 8, and 16% (volume percentage of CFS in deionized Water) and shaking culture at 28°C, 120 rpm for 3 h, 6 h, 12 h. Spore germination in both groups was examined using an optical microscope. Germination was defined as an increase in the spore germ tube length beyond the spore radius. Each experiment was performed three times. After measuring the spore germination rates of both groups, the germination inhibition rate was calculated as follows:
Spore germination inhibition rate (%) = (germination rate of control / germination rate after treatment) / (germination rate of control) × 100.
2.6 Impact of CFS on kiwifruit soft rot
Fresh and healthy kiwifruits of the same size were selected, and their surfaces were disinfected. Three wounds were made on the surface of each kiwifruit using sterile toothpicks, and then the fruits were dipped into a solution containing 1, 2, 4, 8, and 16% of LMY3-5 CFS for 60 min. In the control group, the kiwifruits were dipped into in sterile water. Then, mycelia discs (5 mm diameter) of B. dothidea were placed on the kiwifruit wounds. Each treatment consisted of 20 kiwifruits, which were placed in plastic boxes maintained at 28°C and 90% relative humidity. After 7 days, the lesion diameters were estimated. Disease inhibition rate (%) was determined by the following equation: (A1 − A2) / A1 × 100, where A1 and A2 correspond to the lesion diameter of the control and treatment groups, respectively.
2.7 Morphological characteristics of B. dothidea mycelia
Fungal mycelial morphology was observed using SEM (SU-8010, Hitachi, Japan). Mycelia treated with CFS [16% (v/v)] for 12 h and fixed using 2.5% (v/v) glutaraldehyde at 4°C for 24 h. After discarding the fixative, the samples were rinsed for 15 min (3 times) with 0.1 M phosphate buffer (pH 7.2). Then, the samples were exposed to an ethanol gradient of 30, 50, 70, 80, 90, and 100% (20 min each). Subsequently, the dehydrated mycelia were critical-point-dried with CO2 and then coated with gold. After freeze-drying under vacuum, the samples were observed via SEM and images were acquired.
2.8 Structural characterization of B. dothidea cells
The cellular microstructure of B. dothidea was observed via TEM (EM1200EX, JEOL, Tokyo, Japan). Sample preparation steps were the same as those described in section 2.6. After sequentially drying in an ethanol gradient of 30, 50, 70, 80, 90, and 100%, the samples were treated for 20 min with pure acetone. The samples were then embedded with Epon812 fixative, cut into ultrathin sections, sequentially stained with uranium acetate and lead citrate, and observed and imaged via TEM.
2.9 Propidium iodide (PI) staining determination
Three fresh mycelia discs of B. dothidea were inoculated in 100 mL potato dextrose broth (PDB) and cultured on a rotary shaker (150 × g at 25°C) for 3 days. Then, the mycelia were harvested using two layers of sterile coarse cotton cloth and washed three times with sterile water. The wet mycelia were then transferred to fresh PDB at final CFS concentrations of 0% (as control), 4, 8, and 16% (vol/vol) and cultured for 12 h. The control group only contained PDB-cultured hyphae. The mycelia were collected and stained with 1 mg/mL PI at 25°C in dark for 30 min, and then excess dye solution was washed off with PBS. Images were captured using confocal fluorescence microscopy (NE 910-FL, Ningbo Yongxin Optics Co., Ltd., China).
2.10 Detection of intracellular content leakage
The mycelia samples of B. dothidea were prepared according to the above-described method. The same amount of mycelium (1 g) was weighed and resuspended in PDB containing 4, 8, and 16% (volume ratio) of CFS. The control group only contained PDB-cultured hyphae. The supernatant was collected by centrifugation at 8000 × g for 5 min after culturing for 0.5 h, 1 h, 3 h, 6 h, and 12 h. Subsequently, 200 μL supernatant was placed on a multifunctional microplate (SuperMax 3,100, Shanghai Flash Biotechnology Co., Ltd., China) to record the absorbance at 280 nm and 260 nm. Each treatment was performed three times to calculate the relative leakage of proteins and nucleic acids (Liu et al., 2020; Li et al., 2021).
2.11 Activity levels of chitinase and β-1,3-glucanase
The mycelia samples of B. dothidea were prepared according to the above-described method. The same amount of mycelia (1 g) was resuspended in PDB medium containing 4, 8, and 16% (volume ratio) CFS for 3 h, 6 h, 12 h, 24 h, 48 h, and 72 h. Each treatment was conducted in triplicate. Chitinase activity assay kit (BC0820, Beijing Solarbio Science and Technology Co., Ltd., China) and β-1,3-glucanase activity assay kit (BC0360, Beijing Solarbio Science and Technology Co., Ltd., China) were used according to the manufacturer’s instructions, and the activities of chitinase and β-1,3-glucanase in B. dothidea cells were measured using spectrophotometry.
2.12 Analysis of filtrate components via GC–MS and LC–MS
The fermentation broth of LMY3-5 cultured for 7 days was filtered (0.22 μm membrane). Components of the CFS filtrate were assessed by GC-LC–MS. GC–MS (Agilent Technologies, CA, USA) was used to identify the CFS components using a DB-5MS capillary column (membrane width, 0.25 μm; 30 m × 0.25 mm) (Li et al., 2021). LC–MS analysis was performed as elaborated by Nam et al. (2015) (API2000 TM, AB Sciex, Redwood City, CA, USA) and eluted by employing an acetonitrile/water mobile phase consisting of 0.05% TFA. The MS instrument included an electrospray ionization (ESI) source and triple quadrupole spectrometer.
2.13 Statistical analyses
All data were statistically analyzed using Excel 2010 and SPSS version 25 (SPSS Inc., Chicago, IL, USA). One-way ANOVA followed by Duncan’s multiple range test was performed to determine significant differences at p < 0.05. Charts were plotted with Origin 2021.
3 Results
3.1 Antifungal activity of LMY3-5 against phytopathogenic fungi
Strain LMY3-5 showed strong inhibitory activity. In the confrontation culture experiment, the inhibition rates of D. eres, B. dothidea, A. alternata, M. fructicola, and C. gloeosporioides against mycelial growth were 72.11, 60.68%, 60.05, 68.97 and 52.18%, respectively (Figure 1).
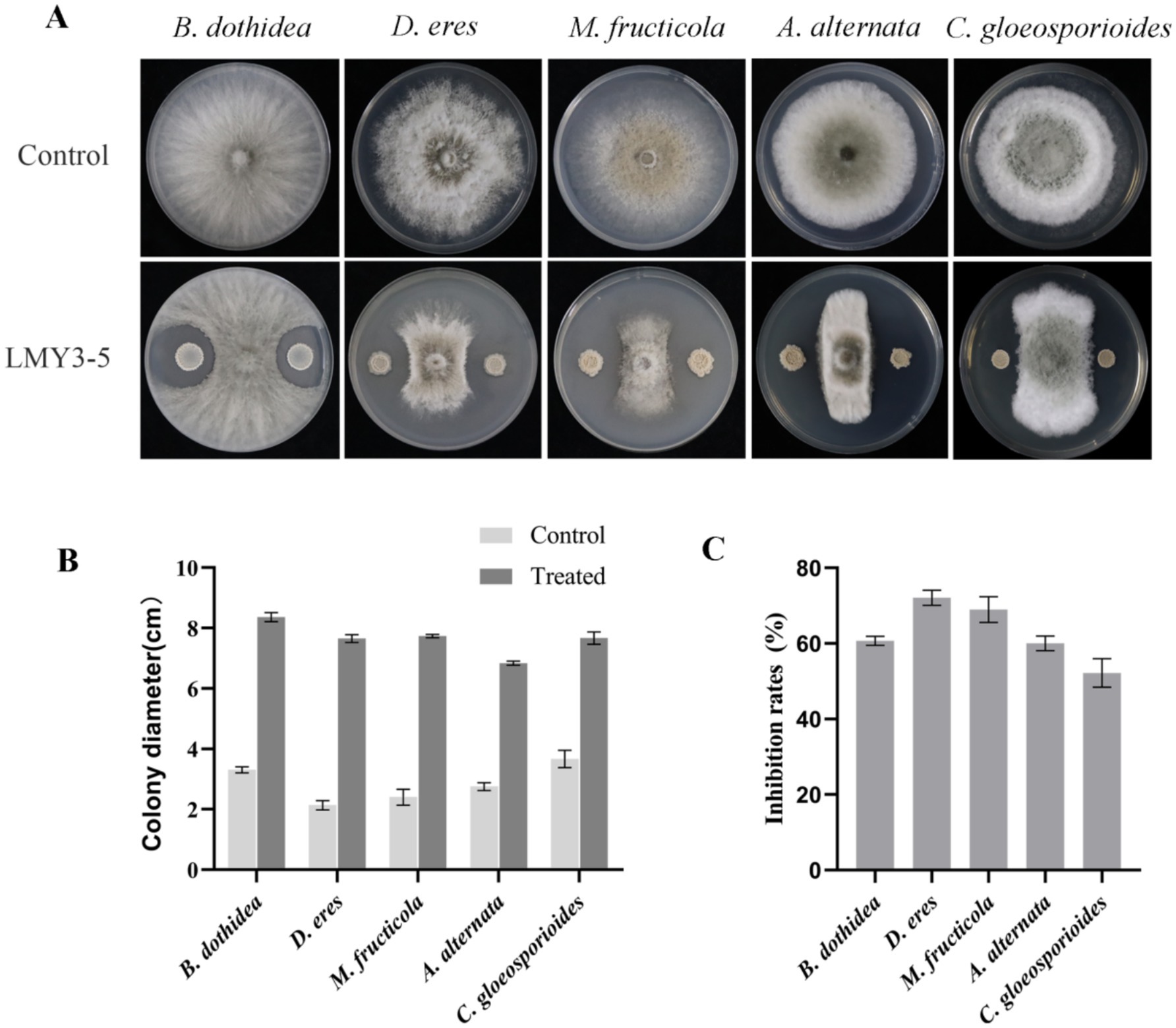
Figure 1. Antifungal activity of strain LMY3-5 against phytopathogenic fungi. (A) Mycelial growth of phytopathogenic fungi under the LMY3-5 treatment. Colony diameter(cm) (B) and Inhibition rate (C) of strain LMY3-5 against pathogenic fungi. Data are presented as the means ± SD [n (number of plates) = 3].
3.2 Identification of strains LMY3-5
After culturing for 3 days on solid LB, strain LMY3-5 produced milky white colonies with rough and wrinkled surfaces and irregular and opaque edges (Figure 2A). When stained with Gram stain, LMY3-5 retained the purple color, indicating its Gram-positive nature (Figure 2B). To further determine the taxonomic status of LMY3-5, we identified it at the molecular level. The gyrA and 16 S rRNA sequences of LMY3-5 strain were stored in the NCBI database (accession numbers PP239377 and PP231028, respectively). BLAST alignment analysis was performed on the 16 S rRNA and gyrA genes sequenced, and the corresponding sequences of 19 Bacillus strains with high homology were selected as reference sequences for multi-gene phylogenetic analysis. The phylogenetic tree is shown in Figure 3. Strains LMY3-5 and B. velezensis were clustered in one branch, and the support rate was 100/1. Based on morphological and molecular systematic results, strain LMY3-5 was identified as B. velezensis.
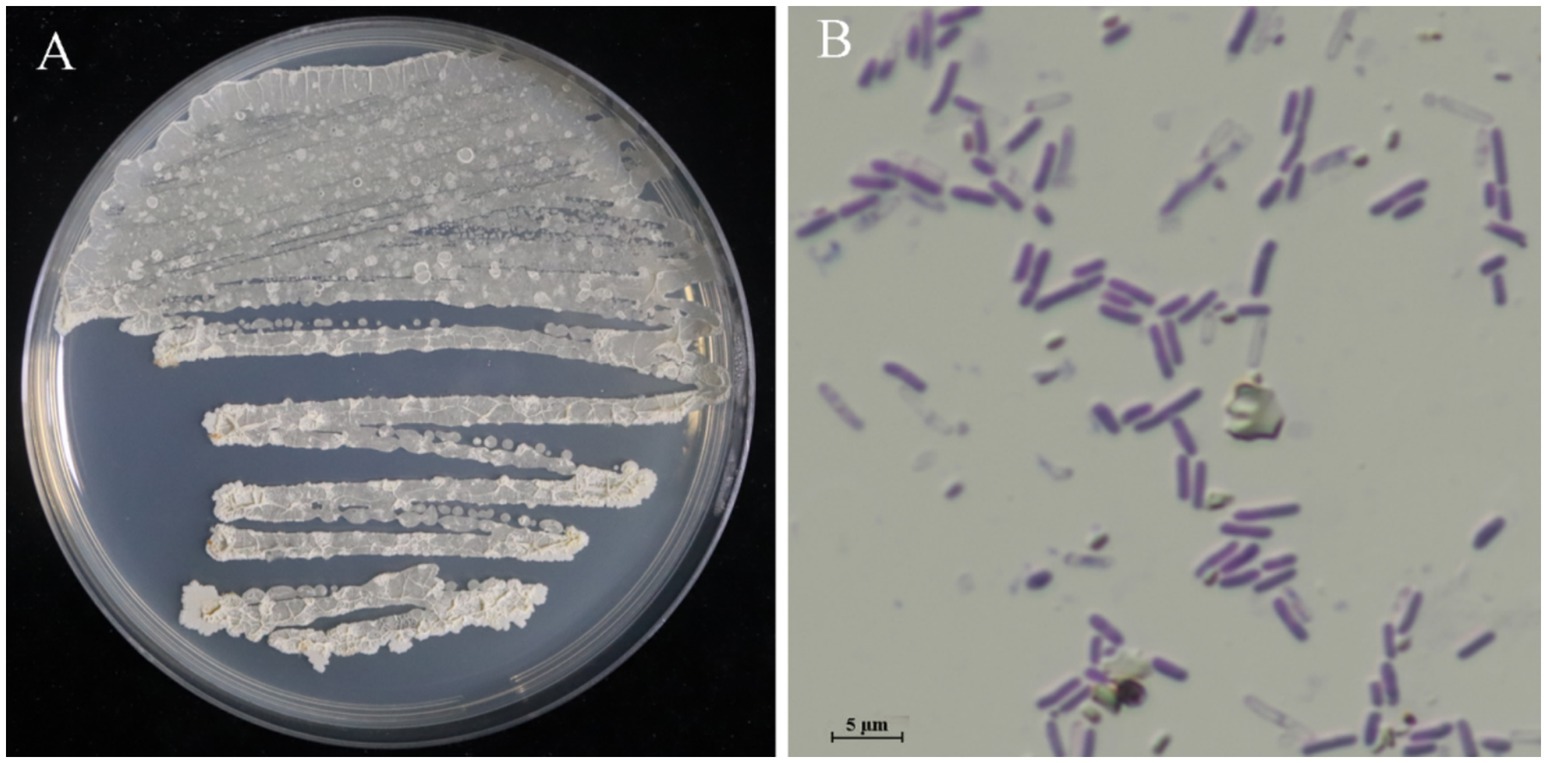
Figure 2. Colony morphology of strain LMY3-5. (A) Colony morphology in LB; (B) Gram staining results.
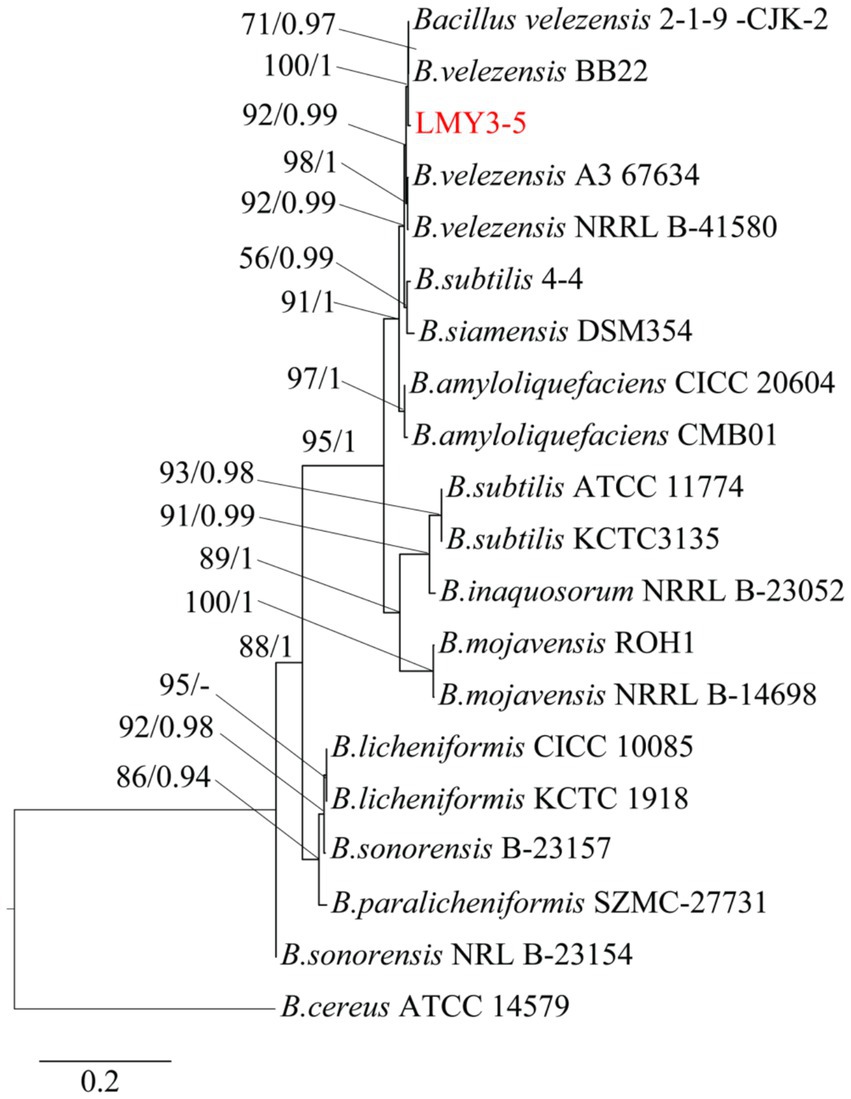
Figure 3. Maximum likelihood tree based on the gyrA and 16 S rRNA sequences. Based on 1,000 bootstrap repetitions, the BYPP support rate was >0.90 and ML value was >70%, as shown at the node.
3.3 B. dothidea mycelial growth suppression by CFS
The CFS of LMY3-5 substantially suppressed the expansion of and B. dothidea mycelia (Figure 4). The antifungal activity increased rapidly as the concentration of CFS increased. When the amount of CFS reached 16%, the inhibition rate of B. dothidea reached 83.51% (Figure 4C).
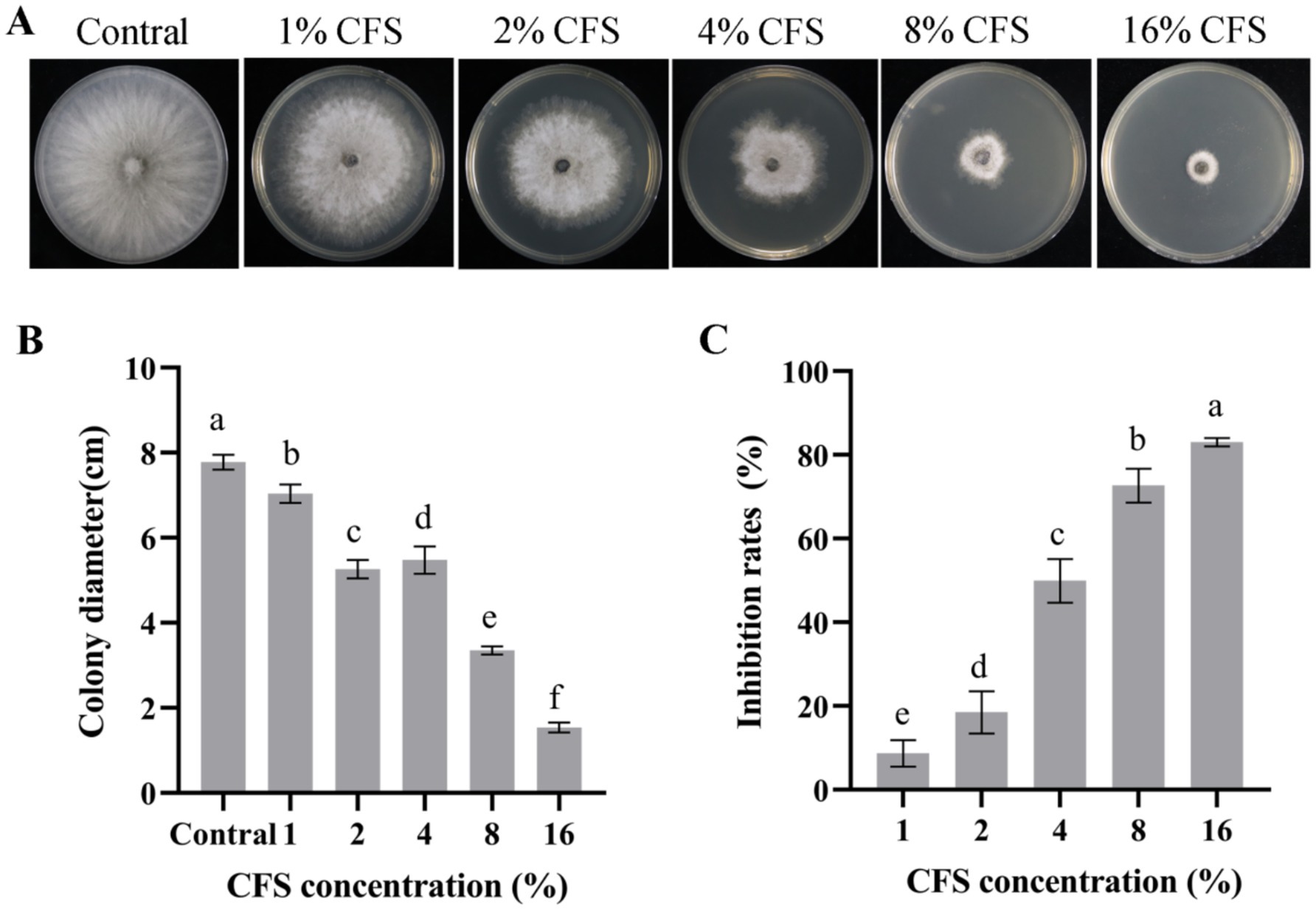
Figure 4. Antifungal effect of strain LMY3-5 on B. dothidea determined using different concentrations of CFS in vitro. (A) Mycelial growth of B. dothidea on PDA under CFS treatment. Colony diameter(cm) (B) and mycelial inhibition rate (C) of B. dothidea under CFS treatment. Data are presented as the means ± SD [n (number of plates) = 3]. Bars with the same lowercase letters indicate no significant treatment results (p < 0.05).
3.4 Impact of LMY3-5 on the germination of B. dothidea spores
Different concentrations of CFS of the antagonistic strain LMY3-5 were used to detect the suppression of germination of B. dothidea spores. Addition of the supernatant at 16.00% (v/v) led to the highest inhibition by CFS (Figure 5A) relative to that of the control. The rate of spore germination inhibition was 97.32% (Figure 5A). In the 8.00% (v/v) and 4.00% (v/v) CFS treatment groups, the spore germination inhibition rates were 72.76 and 53.65%, respectively. Overall, the CFS of LMY3-5 successfully suppressed the germination of B. dothidea spores. Stronger suppression was observed as the CFS concentration in PDB increased.
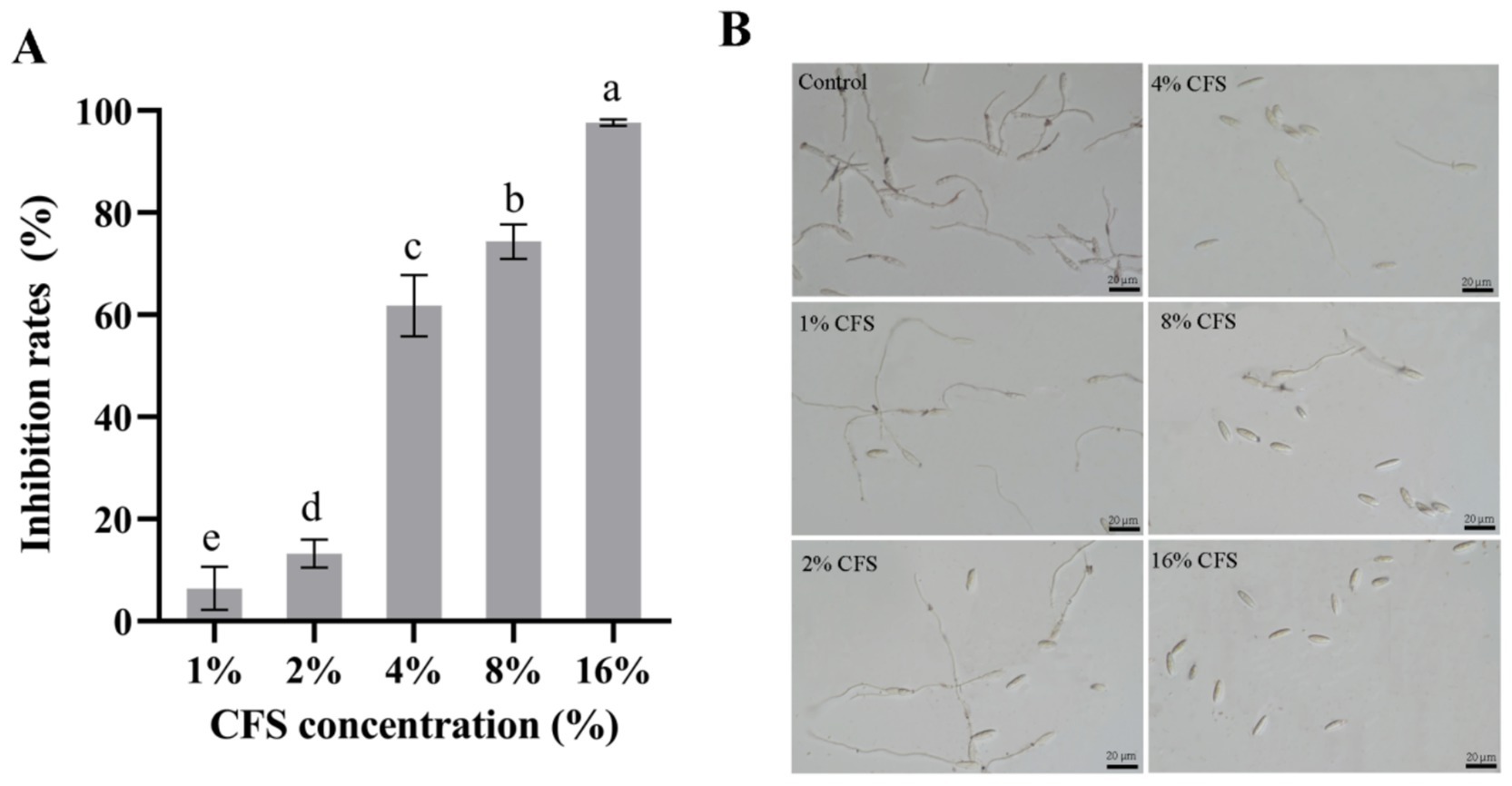
Figure 5. Inhibitory effect of LMY3-5 CFS on B. dothidea spore germination. (A) Inhibition rate of B. dothidea spore germination under CFS treatment (B) Spore germination of B. dothidea inhibited by CFS were observed under microscopy. Data are presented as the means ± SD. Bars with the same lowercase letters indicate no significant difference between treatments (p < 0.05).
3.5 Inhibition of soft rot development in kiwifruit by LMY3-5
To detect the control effect of strain LMY3-5 on kiwifruit soft rot, kiwifruits were exposed to B. dothidea. After 1 week of treatment, the kiwifruits were peeled and observed. The kiwifruit in the control group without the CFS was severely diseased, the lesion diameter was large, and the fruit was rotten. However, kiwifruits in the CFS treatment groups showed small lesion diameters. In addition, the greater the concentration of CFS, the smaller the lesion diameter and better the inhibitory effect (Figure 6). The inhibition rates of kiwifruit inoculated with B. dothidea treated with 16% CFS were 73.59% (Figure 6A). LMY3-5 could efficiently inhibit infection by B. dothidea, slow the expansion of lesions, and exert a significant control effect on soft rot in kiwifruit.
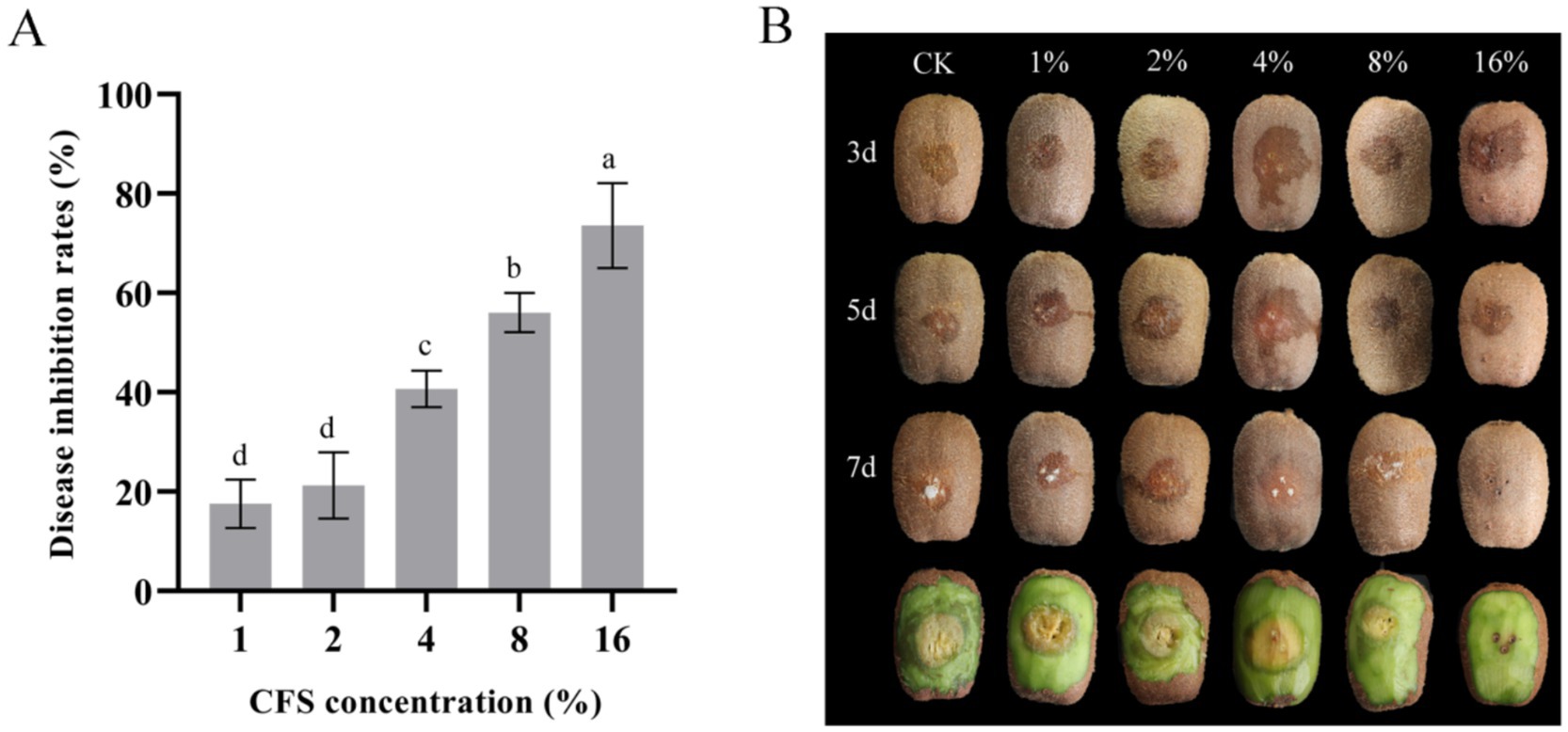
Figure 6. In vivo effect of LMY3-5 CFS on disease extension in kiwifruit caused by B. dothidea. (A) Disease inhibition rate after treatment with different concentrations of aseptic filtrates. (B) Disease extension symptom in kiwifruit after treatment with LMY3-5 CFS for 3, 5, and 7 days. Data are presented as the means ± SD [n (number of kiwifruit) = 20]. Bars with the same lowercase letters indicate no significant difference between treatments (p < 0.05).
3.6 Effects of LMY3-5 on B. dothidea mycelial morphology
Morphological variations between the mycelia of untreated and treated B. dothidea were assessed using SEM. The surface of the control mycelia was full and complete and did not show shrinkage or depressions, and the thickness was uniform (Figure 7). After treatment with the CFS, the mycelia showed abnormalities in surface morphology as well as collapse, shrinkage, and mycelial fracture.
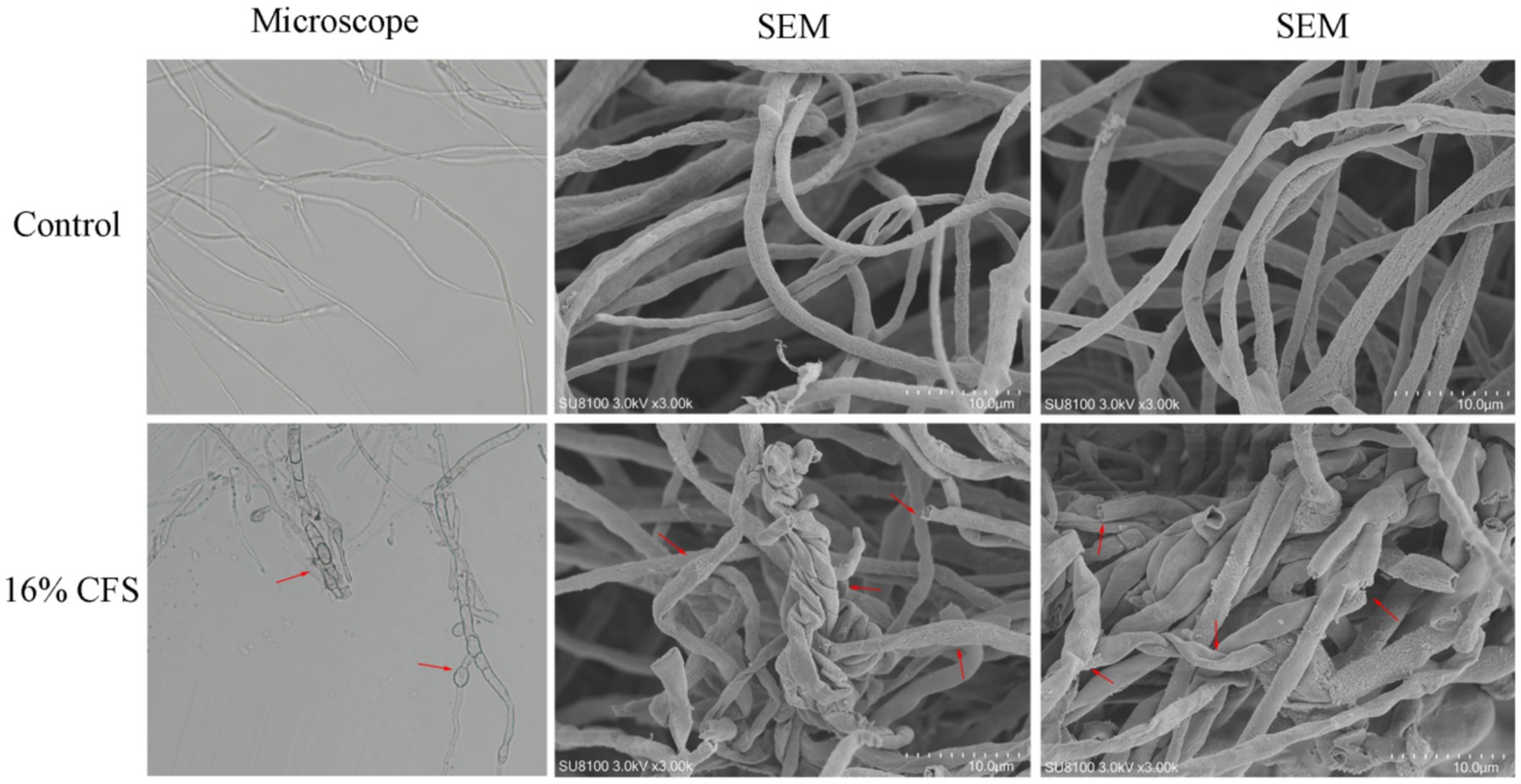
Figure 7. Effect of 16% CFS treatment on B. dothidea mycelium morphology after 12 h. Control was treated with sterile water for 12 h. The red arrow indicates collapse, shrinkage, or mycelial fracture.
3.7 Impact of LMY3-5 on the structure of B. dothidea
Structural variations in B. dothidea cells exposed to LMY3-5 were examined by TEM. Untreated mycelial cells were healthy, the cell membrane and cell wall were normal in shape, and the organelle contours were clear and evenly distributed in the cytoplasm (Figure 8). In treated mycelia, the cell structure was incomplete, cell walls were blurred, cellular membranes were contracted, internal structure was loose, organelles were severely dissolved, and a large cavity area appeared.
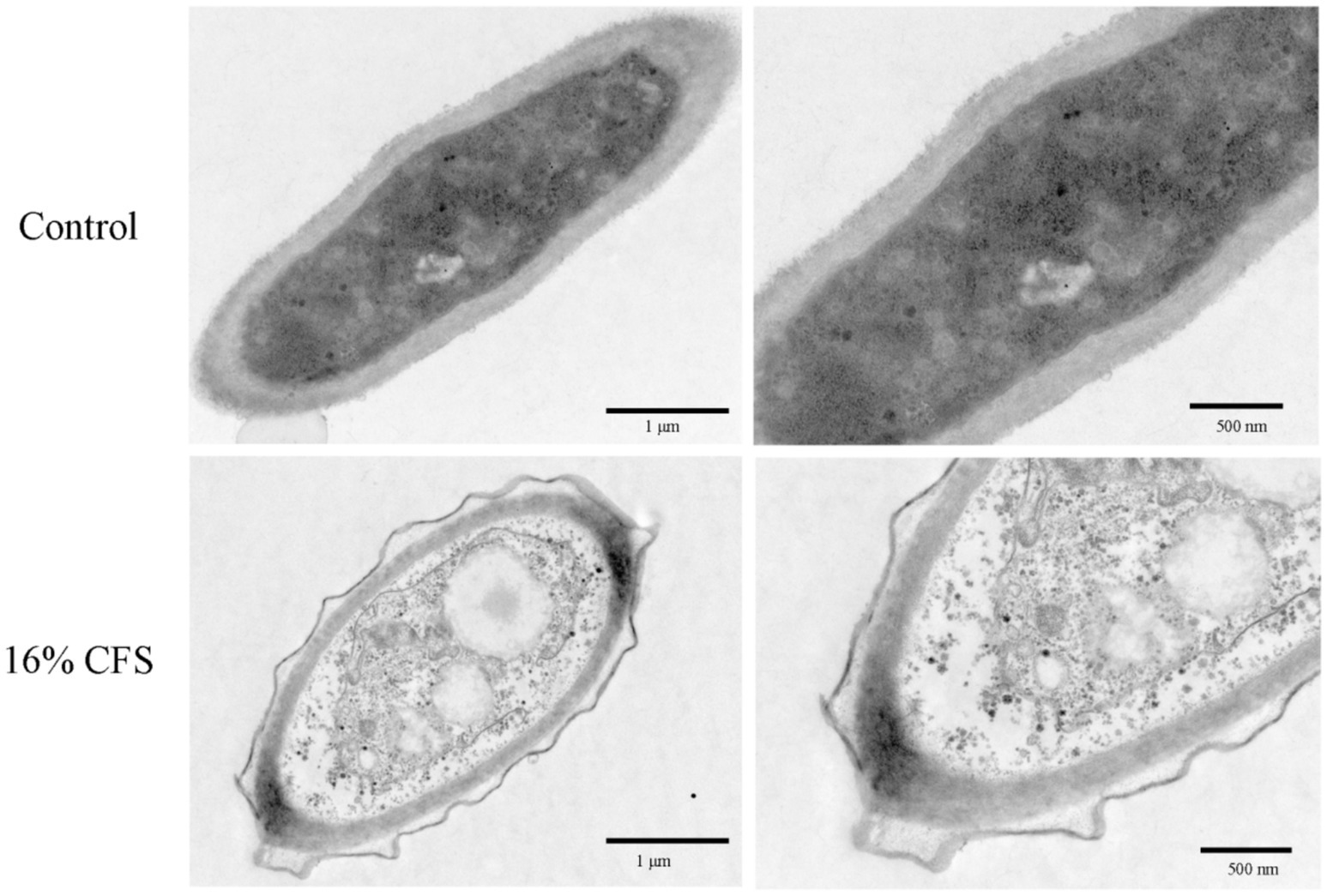
Figure 8. Transmission electron microscope scans of B. dothidea mycelia treated with 16% CFS for 12 h. Control was treated with sterile water for 12 h.
3.8 Effect of CFS on the cell membrane of B. dothidea
PI is able to stain the nucleus through the cell membrane of dead cells and middle and late apoptotic cells, thereby directly indicating the extent of damage to the mycelial cell membrane. As shown in Figure 9A, after 12 h of CFS treatment, B. dothidea hyphae showed a red color via fluorescence microscopy, and the fluorescence intensity increased with increasing CFS concentration. The results showed the noticeable destruction of the B. dothidea cell membrane by LMY3-5.
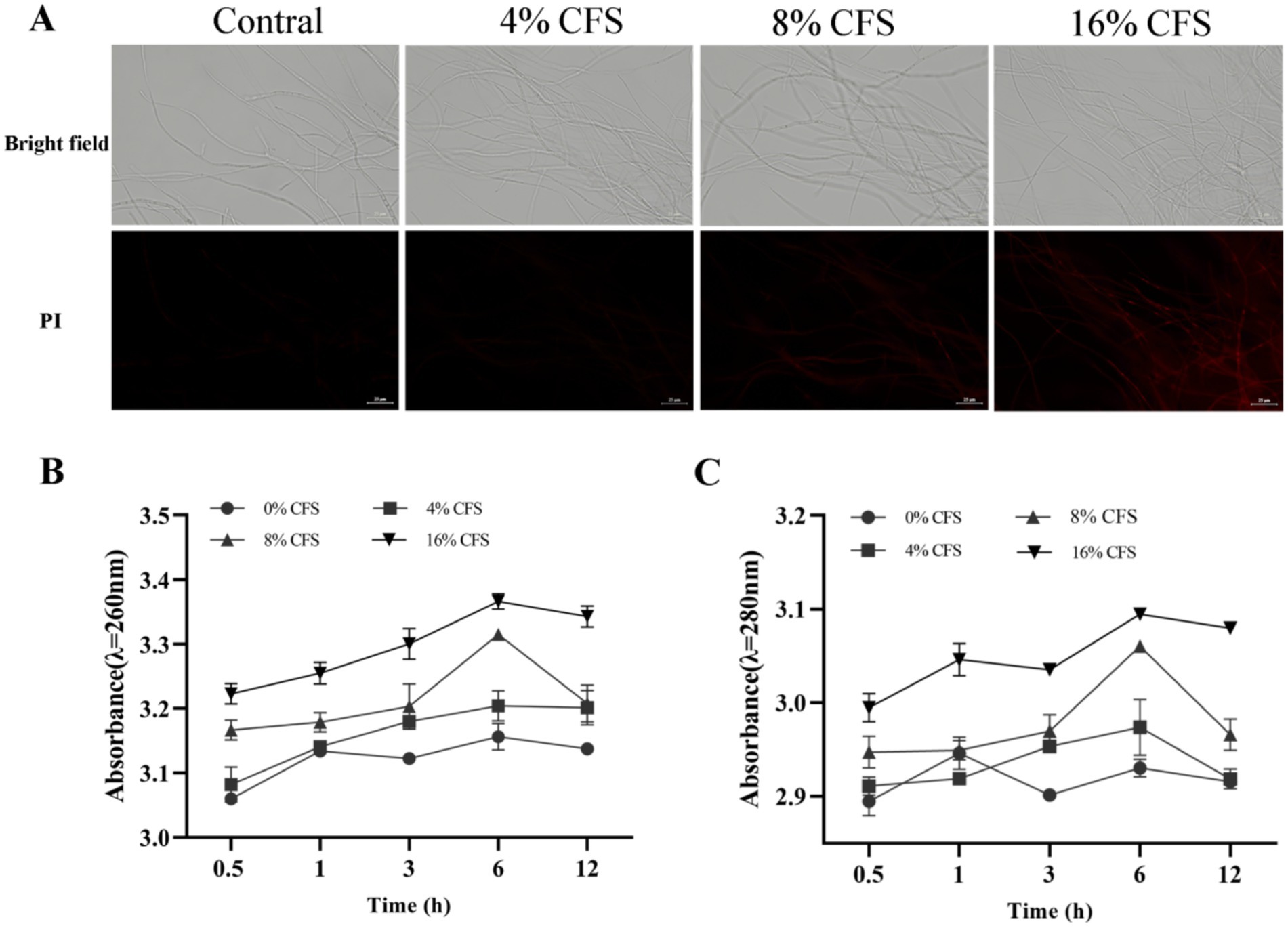
Figure 9. Effects of CFS of B. velezensis LMY3-5 on cell membrane integrity of B. dothidea. (A) Images of propidium iodide-stained cells in the dark field (PI) and bright field. (B) Concentrations of leaked nucleic acids and (C) proteins of B. dothidea under CFS treatment were measured at various time points. Data are presented as the means ± SD. Bars with the same lowercase letters indicate no significant difference between treatments (p < 0.05).
Nucleic acids and proteins are important intracellular components that can be used to evaluate changes in the permeability of the mycelial membrane. The extracellular protein and nucleic acid content of B. dothidea increased with prolonged treatment time and increased CFS concentration, whereas the CK group remained the same (Figures 9B and C). After 6 h of CFS treatment, a high quantity of nucleic acids and proteins began to leak from the B. dothidea mycelia. CFS destroyed the cell membrane and improved its permeability, resulting in a dose-dependent increase in nucleic acids, proteins, and leakage.
3.9 Impact of LMY3-5 on the activity levels of cell-wall-disintegrating enzymes in B. dothidea
Chitinase and β-1,3-glucanase are key cell wall-degrading enzymes, which are important indicators of cell wall integrity. Activity levels of these enzymes in LMY3-5-treated B. dothidea initially surged and then declined (Figure 10). Chitinase activity was highest after 12 h, while β-1,3 glucanase activity was highest after 24 h. At each measurement time point, the activity levels of these enzymes were noticeably greater in treated B. dothidea relative to that of the control. This shows that the CFS decreased the activity of key enzymes in the cell wall, thus leading to a gradual degradation of the cell wall.
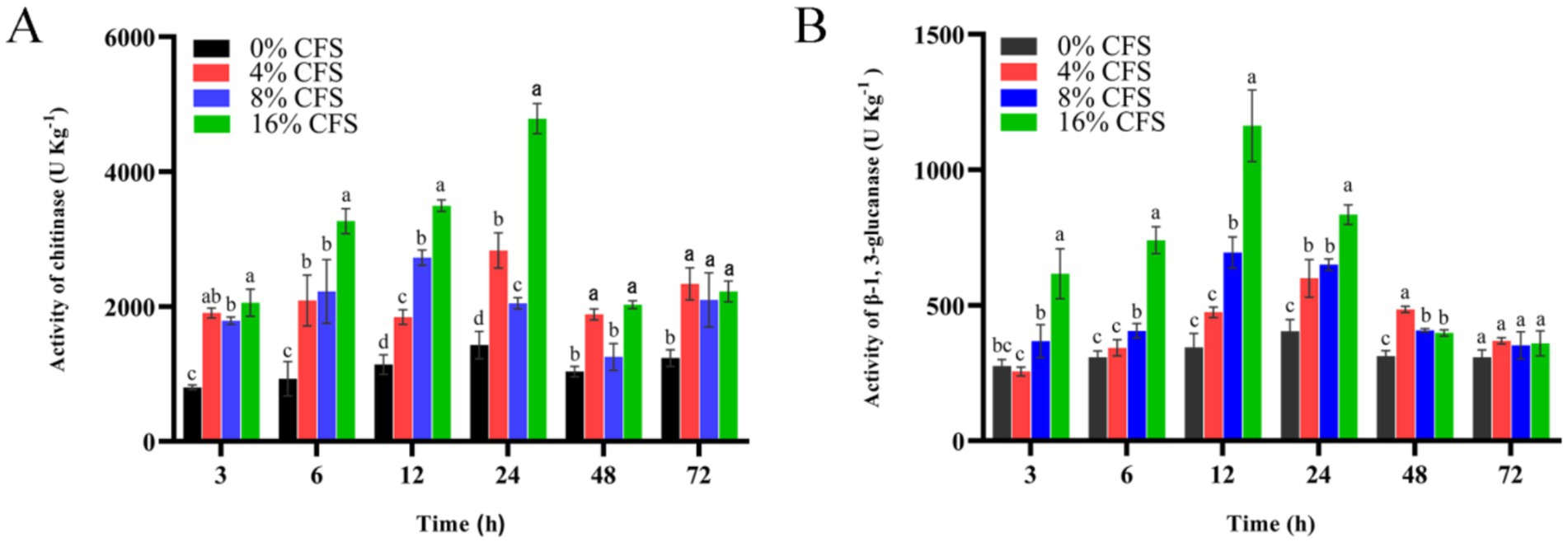
Figure 10. Impact of LMY3-5 on the chitinase (A) and glucanase (B) activities in B. dothidea. Data are displayed as the mean ± SE. Bars with the same lowercase letters indicate no significant difference between treatments (p < 0.05).
3.10 GC–LC–MS identification of CFS components
A total of 181 volatile components were identified from LMY3-5 CFS by GC–MS. A literature review revealed that 10 compounds to have antibacterial or antifungal activity (Table 1). The LC–MS results showed that a total of 2,901 compounds were detected, of which 204 components had similarity >80% and 17 metabolic components showed antibacterial or antifungal activity (Table 2).
4 Discussion
Kiwifruit soft rot severely constrains the development of the kiwi industry. Currently, the prevention and control of kiwifruit soft rot primarily rely on chemical agents. In this study, the B. velezensis strain LMY3-5 was screened from the endophytes of kiwifruit and found to exhibit a marked inhibitory effect on the pathogen B. dothidea, which causes kiwifruit soft rot. B. velezensis has been confirmed to possess antimicrobial activity and can effectively suppress diseases such as peach gummosis (Kang et al., 2024), apple canker (Yuan et al., 2022), and pear ring rot (Yang et al., 2023). However, few studies have reported on the control effect of B. velezensis on kiwifruit soft rot. In this study, we demonstrated that B. velezensis LMY3-5 can effectively inhibit the expansion of kiwifruit soft rot. This is the first time that B. velezensis with biocontrol properties has been isolated from the endophytes of kiwifruit. Thus, our findings enrich the resource library for the biological control of kiwifruit soft rot and offer new possibilities for the biological control of this disease.
B. velezensis has a broad antifungal spectrum and is non-pathogenic to plants (Liu et al., 2017). The antimicrobial action in bacillus spp. is the most critical, and it is achieved by the production of antibiotic compounds, such as lipopeptides, peptides, bacteriocins, and volatile substances (Shafi et al., 2017; Fira et al., 2018). These substances inhibit pathogenic fungal hyphae growth and spore germination by acting on the fungal cell wall, cell membrane, and nuclear membrane and inducing apoptosis (Ramarathnam et al., 2007; Gu et al., 2017; Jin et al., 2020). In this experiment, the sterile fermentation broth of B. velezensis LMY3-5 affected B. dothidea by inhibiting hyphal growth and spore germination, damaging the cell membrane and cell wall, altering the morphology of the fungal body, increasing the hyphal permeability, and causing leakage of the nucleic acid content, thus leading to an imbalance of the intracellular environment and even cell death. These results are consistent with previous studies, suggesting that the B. velezensis strain LMY3-5 can produce multiple antimicrobial substances. However, the specific mechanisms of action and metabolic products remain to be elucidated in further experimental research.
The genus Bacillus is metabolically vigorous and capable of producing a variety of antimicrobial organic compounds, which can be broadly categorized into aldehydes, ketones, alcohols, esters, and organic acids (Zheng et al., 2013). However, even within the same species, different strains of Bacillus can produce varying antimicrobial compounds (Aloo et al., 2019). This study aims to preliminarily reveal the types of antimicrobial organic compounds produced by B. velezensis LMY3-5 by analyzing the components of its sterile filtrate using GC–MS and LC–MS techniques. The study found that the sterile filtrate contained 27 antifungal compounds, including isobutyric acid, dibutyl phthalate, nonanal, decanal, vanillin, and 2,4-di-tert-butylphenol. Previous reports have confirmed the antifungal capabilities of these substances. Aldehyde substances such as nonanal, decanal, and vanillin can inhibit the growth of the mycelium of A. alternata, Penicillium, Botrytis cinerea, and Sclerotinia sclerotiorum (Yang et al., 2021; Zhang et al., 2017; Zhang et al., 2013). The ketone methyl isobutyl ketone and ester dibutyl phthalate have strong antifungal activity against Penicillium italicum and B. cinerea, respectively (Morita et al., 2019; Li et al., 2022). Isobutyric acid has a broad spectrum of antimicrobial activity and also exhibits antibacterial activity against a variety of oral microorganisms (Huang et al., 2011). 4-Guanidinobutyric acid inhibits the growth of Helicobacter pylori and shows cytotoxicity to human gastric cancer cell lines SNU638 and AGS; thus, it can be used for the treatment and prevention of gastric damage (Hwang and Jeong, 2012). The presence of these compounds not only confirms the potential of B. velezensis LMY3-5 as a biocontrol agent but also reveals further possibilities for its application in the pharmaceutical industry.
In this experiment, B. velezensis LMY3-5 showed significant biocontrol potential and inhibited the expansion rates of B. dothidea, the causative agent of kiwifruit soft rot, by 73.59%. However, the efficacy of this strain in controlling kiwifruit soft rot under field conditions still needs to be evaluated through further field trials. Moreover, this study conducted a preliminary investigation into the antifungal mechanism of this strain at the cellular level. Future research could combine transcriptomics, proteomics, metabolomics, and multi-omics analyses to delve deeper into the molecular mechanisms of action, with the aim of providing theoretical support for the development of more comprehensive and efficient biocontrol strategies.
Data availability statement
The original contributions presented in the study are included in the article/supplementary material, further inquiries can be directed to the corresponding author.
Author contributions
CR: Data curation, Methodology, Writing – original draft, Writing – review & editing. YL: Conceptualization, Formal analysis, Visualization, Writing – original draft. WS: Software, Writing – original draft. BT: Funding acquisition, Methodology, Writing – review & editing.
Funding
The author(s) declare that financial support was received for the research and/or publication of this article. This research was supported by the Guizhou Provincial Science and Technology Projects [ZK(2024)541].
Conflict of interest
The authors declare that the research was conducted in the absence of any commercial or financial relationships that could be construed as a potential conflict of interest.
Generative AI statement
The authors declare that no Gen AI was used in the creation of this manuscript.
Publisher’s note
All claims expressed in this article are solely those of the authors and do not necessarily represent those of their affiliated organizations, or those of the publisher, the editors and the reviewers. Any product that may be evaluated in this article, or claim that may be made by its manufacturer, is not guaranteed or endorsed by the publisher.
References
Almenar, E., Del Valle, V., Catala, R., and Gavara, R. (2007). Active package for wild strawberry fruit (Fragaria vesca L.). J. Agr. Food Chem. 55, 2240–2245. doi: 10.1021/jf062809m
Aloo, B. N., Makumba, B. A., and Mbega, E. R. (2019). The potential of Bacilli rhizobacteria for sustainable crop production and environmental sustainability. Microbiol. Res. 219, 26–39. doi: 10.1016/j.micres.2018.10.011
Aneta, W., and Paulina, N. (2018). Anticholinergic effects of Actinidia arguta fruits and their polyphenol content determined by liquid chromatography-photodiode array detector-quadrupole/time of flight-mass spectrometry (LC-MS-PDA-Q/TOF). Food Chem. 271, 216–223. doi: 10.1016/j.foodchem.2018.07.084
Awad, H., EL-Shahed, K., Aziz, R., Sarmidi, M., and El-Enshasy, H. (2012). Antibiotics as microbial secondary metabolites, production and application. J. Teknol. 59, 101–111. doi: 10.11113/jt.v59.1593
Bakirci, G. T., Acay, D. B. Y., Bakirci, F., and Otles, S. (2014). Pesticide residues in fruits and vegetables from the Aegean region, Turkey. Food Chem. 160, 379–392. doi: 10.1016/j.foodchem.2014.02.051
Balestra, G. M., Mazzaglia, A., and Rossetti, A. (2009). Outbreak of bacterial blossom blight caused by Pseudomonas viridiflava on Actinidia chinensis kiwifruit plants in Italy. Plant Dis. 92:1707. doi: 10.1094/PDIS-92-12-1707A
Bellahcen, T. O., Cherki, M., Cayuela Sanchez, J. A., Cherif, A., and El Amrani, A. (2019). Chemical composition and antibacterial activity of the essential oil of Spirulina platensis from Morocco. J. Essent Oil Bear Pl. 22, 1265–1276. doi: 10.1080/0972060x.2019.1669492
Bi, Y., Liu, G., Yu, Q., Liang, Q., Xu, Z., Cui, M., et al. (2021). Anti-Vibrio dibutyl phthalate from marine-derived Streptomyces sp. S073. Res. Vet. Sci. 140, 198–202. doi: 10.1016/j.rvsc.2021.09.001
Blagodatskikh, I. V., Vyshivannaya, O. V., Alexandrova, A. V., Bezrodnykh, E. A., Zelenikhin, P. V., Kulikov, S. N., et al. (2018). Antibacterial activity and cytotoxicity of betainated oligochitosane derivatives. Microbiology 87, 725–731. doi: 10.1134/s0026261718050041
Cawoy, H., Debois, D., Franzil, L., De Pauw, E., Thonart, P., and Ongena, M. (2015). Lipopeptides as main ingredients for inhibition of fungal phytopathogens by Bacillus subtilis /amyloliquefaciens. Microb. Biotechnol. 8, 281–295. doi: 10.1111/1751-7915.12238
Chen, T., Wu, X., Dai, Y., Yin, X., Zhao, Z., Zhang, Z., et al. (2022). Sensitivity testing of natural antifungal agents on fusarium fujikuroi to investigate the potential for sustainable control of kiwifruit leaf spot disease. J. Fungi 8:239. doi: 10.3390/jof8030239
Choi, J. N., Kim, J., Ponnusamy, K., Lim, C., Kim, J. G., Muthaiya, M. J., et al. (2013). Metabolic changes of Phomopsis longicolla fermentation and its effect on antimicrobial activity against Xanthomonas oryzae. J. Microbiol. Biotechnol. 23, 177–183. doi: 10.4014/jmb.1210.10020
Chun, J., and Bae, K. S. (2000). Phylogenetic analysis of Bacillus subtilis and related taxa based on partial gyrA gene sequence. Antonie Van Leeuwenhoek 78, 123–127. doi: 10.1023/A:1026555830014
Deniz, K., and Ferda, S. (2019). Antibiotic-resistant staphylococcus aureus does not develop resistance to vanillic acid and 2-Hydroxycinnamic acid after continuous exposure in vitro. ACS Omega 4, 15393–15400. doi: 10.1021/acsomega.9b01336
Diaz, G. A., Latorre, B. A., Jara, S., Ferrada, E., Naranjo, P., Rodriguez, J., et al. (2014). First report of Diaporthe novem causing postharvest rot of kiwifruit during controlled atmosphere storage in Chile. Plant Dis. 98, 1274–1275. doi: 10.1094/pdis-02-14-0183-pdn
Diaz, G. A., Latorre, B. A., Lolas, M., Ferrada, E., Naranjo, P., and Zoffoli, J. P. (2017). Identification and characterization of Diaporthe ambigua, D.Australafricana, D.Novem, and D.Rudis causing a postharvest fruit rot in kiwifruit. Plant Dis. 101, 1402–1410. doi: 10.1094/pdis-10-16-1535-re
dos Santos, E. A. R., Tadielo, L. E., Schmiedt, J. A., Possebon, F., Pereira, M. O., Pereira, J. G., et al. (2023). Effect of ginger essential oil and 6-gingerol on a multispecies biofilm of Listeria monocytogenes, Salmonella typhimurium, and Pseudomonas aeruginosa. Braz. J. Microbiol. 54, 3041–3049. doi: 10.1007/s42770-023-01075-2
Fan, Y., Feng, H., Liu, L., Zhang, Y., Xin, X., and Gao, D. (2020). Chemical components and antibacterial activity of the essential oil of Six Pyrrosia species. Chem. Biodivers. 17:526. doi: 10.1002/cbdv.202000526
Ferreira, F. d. S., de Araujo Neto, J. B., Oliveira-Tintino, C. D. d. M., de Araujo, A. C. J., Ribeiro-Filho, J., Freitas, P. R., et al. (2023). Chemical composition and antibacterial effects of Etlingera elatior (Jack) R. M. Smith against Staphylococcus aureus efflux pumps. Chem. Biol. Interact. 386:110751. doi: 10.1016/j.cbi.2023.110751
Fira, D., Dimkic, I., Beric, T., Lozo, J., and Stankovic, S. (2018). Biological control of plant pathogens by Bacillus species. J. Biotechnol. 285, 44–55. doi: 10.1016/j.jbiotec.2018.07.044
Fujita, K., Chavasiri, W., and Kubo, I. (2015). Anti-Salmonella activity of volatile compounds of Vietnam coriander. Phytother. Res. 29, 1081–1087. doi: 10.1002/ptr.5351
Gu, Q., Yang, Y., Yuan, Q., Shi, G., Wu, L., Lou, Z., et al. (2017). Bacillomycin D produced by Bacillus amyloliquefaciens is involved in the antagonistic interaction with the plant-pathogenic fungus fusarium graminearum. Appl. Environ. Microbiol. 83:e01075-17. doi: 10.1128/AEM.01075-17
Guo, Z., Ni, Y., Gao, H., Ding, G., and Zeng, Y. (2024). A dual-branch selective attention capsule network for classifying kiwifruit soft rot with hyperspectral images. Sci. Rep. 14:10664. doi: 10.1038/s41598-024-61425-4
Gupta, R., Luxami, V., and Paul, K. (2021). Insights of 8-hydroxyquinolines, a novel target in medicinal chemistry. Bioorg. Chem. 108:104633. doi: 10.1016/j.bioorg.2021.104633
Hamdache, A., Lamarti, A., Aleu, J., and Collado, I. G. (2011). Non-peptide metabolites from the genus Bacillus. J. Nat. Prod. 74, 893–899. doi: 10.1021/np100853e
Han, C. V., and Bhat, R. (2014). In vitro control of food-borne pathogenic bacteria by essential oils and solvent extracts of underutilized flower buds of Paeonia suffruticosa (Andr.). Ind. Crop. Prod. 54, 203–208. doi: 10.1016/j.indcrop.2014.01.014
He, L., Shi, J., Zhao, Z., Ran, F., Mo, F., Long, Y., et al. (2022). First report of grown gall of kiwifruit (Actinidia deliciosa) caused by Agrobacterium fabacearum in China and the establishment of loop-mediated isothermal amplification technique. Int. J. Mol. Sci. 23:207. doi: 10.3390/ijms23010207
Huang, C. B., Alimova, Y., Myers, T. M., and Ebersole, J. L. (2011). Short- and medium-chain fatty acids exhibit antimicrobial activity for oral microorganisms. Arch. Oral Biol. 56, 650–654. doi: 10.1016/j.archoralbio.2011.01.011
Hwang, I. Y., and Jeong, C. S. (2012). Inhibitory effects of 4-Guanidinobutyric acid against gastric lesions. Biomol. Ther. 20, 239–244. doi: 10.4062/biomolther.2012.20.2.239
Islam, M. T., Ahn, S. Y., Cho, S. M., and Yun, H. K. (2013). Isolation of antibacterial compounds from hairy vetch (Vicia villosa) against grapevine crown gall pathogen. Hortic. Environ. Biote 54, 338–345. doi: 10.1007/s13580-013-0028-8
Jin, P., Wang, H., Tan, Z., Xuan, Z., Dahar, G. Y., Li, Q. X., et al. (2020). Antifungal mechanism of bacillomycin D from Bacillusi velezensis HN-2 against Colletotrichum gloeosporioides Penz. Pestic. Biochem. Physiol. 163, 102–107. doi: 10.1016/j.pestbp.2019.11.004
Jongman, M., Carmichael, P., Loeto, D., and Gomba, A. (2022). Advances in use of biocontrol applications in preharvest and postharvest environments, a food safety milestone. J. Food Saf. 42:12957. doi: 10.1111/jfs.12957
Kang, T. A., Lee, G., Kim, K., Hahn, D., Shin, J. H., and Kim, W. C. (2024). Biocontrol of peach gummosis by Bacillus velezensis KTA01 and its antifungal mechanism. J. Microbiol. Biotechnol. 34, 296–305. doi: 10.4014/jmb.2310.10005
Kanjanamaneesathian, M., Wiwattanapatapee, R., Rotniam, W., Pengnoo, A., Wongpetkhiew, W., and Tanmala, V. (2013). Application of a suspension concentrate formulation of Bacillus velezensis to control root rot of hydroponically-grown vegetables. N. Z. Plant Prot. 66, 229–234. doi: 10.30843/nzpp.2013.66.5556
Kim, M. J., Chae, D. H., Cho, G., Kim, D. R., and Kwak, Y. S. (2019). Characterization of antifungal strains against kiwifruit bacterial canker pathogen. Plant Pathol. 35, 473–485. doi: 10.5423/ppj.Oa.05.2019.0154
Kim, G., Sung, J. J., Jae-Seoun, H. U. R., and Jin, K. Y. (2013). Optimal spray time, interval and number of preventive fungicides for the control of fruit rots of green and gold kiwifruit cultivars. Res. Plant Dis. 19, 1–6. doi: 10.5423/rpd.2013.19.1.001
Kwon, S. Y., Kim, G. H., Koh, Y. J., Lee, Y. S., Shon, S. H., Kim, S. C., et al. (2011). Incidence rates of postharvest fruit rots and detection rates of their pathogens on new kiwifruit cultivars bred in Korea. Korean J. Plant Res. 24, 599–603. doi: 10.7732/KJPR.2011.24.5.599
Li, C., Jing, Y., Cheng, L., Si, Z., Mou, Z., Niu, D., et al. (2023). The antifungal activity of trans-cinnamic acid and its priming effect in apple in response to Valsa Mali. Plant Pathol. 72, 1595–1603. doi: 10.1111/ppa.13786
Li, W., Long, Y., Mo, F., Shu, R., Yin, X., Wu, X., et al. (2021). Antifungal activity and biocontrol mechanism of Fusicolla violacea J-1 against soft rot in kiwifruit caused by Alternaria alternata. J. Fungi. 7:937. doi: 10.3390/jof7110937
Li, L., Pan, H., Chen, M., Zhang, S., and Zhong, C. (2017). Isolation and identification of pathogenic fungi causing postharvest fruit rot of kiwifruit (Actinidia chinensis) in China. J. Phytopathol. 165, 782–790. doi: 10.1111/jph.12618
Li, L., Pan, H., Deng, L., Qian, G., Wang, Z., Li, W., et al. (2022). The antifungal activity and mechanism of silver nanoparticles against four pathogens causing kiwifruit post-harvest rot. Front. Microbiol. 13:988633. doi: 10.3389/fmicb.2022.988633
Lian, L., Zhang, S., Yu, Z., Ge, H., Qi, S., Zhang, X., et al. (2019). The dietary freeze-dried fruit powder of Actinidia arguta ameliorates dextran sulphate sodium-induced ulcerative colitis in mice by inhibiting the activation of MAPKs. Food Funct. 10, 5768–5778. doi: 10.1039/c9fo00664h
Liu, X., Cai, J., Chen, H., Zhong, Q., Hou, Y., Chen, W., et al. (2020). Antibacterial Activity and Mechanism of Linalool against Pseudomonas aeruginosa. Microb Pathog. 141:103980. doi: 10.1016/j.micpath.2020.103980
Liu, F., Gao, R. Q., Zhang, F., Ren, Y., Li, W., and He, B. (2023). Postharvest biocontrol of green mold (Penicillium digitatum) in citrus by Bacillus velezensis strain S161 and its mode of action. Biol. Control 187:105392. doi: 10.1016/j.biocontrol.2023.105392
Liu, J., Huang, R., Song, Q., Xiong, H., Ma, J., Xia, R., et al. (2021). Combinational antibacterial activity of Nisin and 3-Phenyllactic acid and their co-production by engineered Lactococcus lactis. Front. Bioeng. Biotechnol. 9:612105. doi: 10.3389/fbioe.2021.612105
Liu, G., Kong, Y., Fan, Y., Geng, C., Peng, D., and Sun, M. (2017). Whole-genome sequencing of Bacillus velezensis LS69, a strain with a broad inhibitory spectrum against pathogenic bacteria. J. Biotechnol. 249, 20–24. doi: 10.1016/j.jbiotec.2017.03.018
Luongo, L., Santori, A., Riccioni, L., and Belisario, A. (2011). Phomopsis sp. associated with post -harvest fruit rot of kiwifruit in Italy. J. Plant Pathol. 93, 205–209. doi: 10.4454/jpp.v93i1.293
Ma, Y., He, Y. H., Deng, P., Zhang, S. Y., Ding, Y. Y., Zhang, Z. J., et al. (2023). Repurposing salicylamides to combat phytopathogenic bacteria and induce plant defense responses. Chem. Biodivers. 20:e202300998. doi: 10.1002/cbdv.202300998
Meng, Q., Jiang, H., and Hao, J. J. (2016). Effects of Bacillus velezensis strain BAC03 in promoting plant growth. Blol Control. 98, 18–26. doi: 10.1016/j.biocontrol.2016.03.010
Miller, M. A., Pfeiffer, W. T., and Schwartz, T. (2010). Creating the CIPRES science Gateway for Inference of Large Phylogenetic Trees. GCE 10, 1–8. doi: 10.1109/GCE.2010.5676129
Morita, T., Tanaka, I., Ryuda, N., Ikari, M., Ueno, D., and Someya, T. (2019). Antifungal spectrum characterization and identification of strong volatile organic compounds produced by Bacillus pumilus TM-R. Heliyon. 5:e01817. doi: 10.1016/j.heliyon.2019.e01817
Nam, J., Yun, H., Kim, J., Kim, P. I., Kim, S. W., Lee, H. B., et al. (2015). Isolation and NMR analysis of antifungal Fengycin a and B from Bacillus amyloliquefaciens subsp plantarum BC32-1. Bull. Korean Chem. Soc. 36, 1316–1321. doi: 10.1002/bkcs.10250
Nazir, M. F., Lou, J., Wang, Y., Zou, S., and Huang, H. (2024). Kiwifruit in the omics age, advances in genomics, breeding, and beyond. Plan. Theory 13:2156. doi: 10.3390/plants13152156
Peng, Q., Yang, J., Wang, Q., Suo, H., Hamdy, A. M., and Song, J. (2023). Antifungal effect of metabolites from a new strain Lactiplantibacillus Plantarum LPP703 isolated from naturally fermented yak yogurt. Food Secur. 12:10181. doi: 10.3390/foods12010181
Priya, B., Utreja, D., and Kalia, A. (2022). Schiff bases of Indole-3-Carbaldehyde, synthesis and evaluation as antimicrobial agents. Russ. J. Bioorg. Chem. 48, 1282–1290. doi: 10.1134/s1068162022060188
Rajamanikyam, M., Vadlapudi, V., Parvathaneni, S. P., Koude, D., Sripadi, P., Misra, S., et al. (2017). Isolation and characterization of phthalates from Brevibacterium mcbrellner that cause cytotoxicity and cell cycle arrest. EXCLI J. 16, 375–387. doi: 10.17179/excli2017-145
Ramarathnam, R., Bo, S., Chen, Y., Fernando, W. G. D., Xuewen, G., and de Kievit, T. (2007). Molecular and biochemical detection of fengycin- and bacillomycin D-producing Bacillus spp., antagonistic to fungal pathogens of canola and wheat. Can. J. Microbiol. 53, 901–911. doi: 10.1139/W07-049
Seenivasan, A., Manikkam, R., Kaari, M., Sahu, A. K., Said, M., and Dastager, S. G. (2022). 2,4-Di-tert-butylphenol (2,4-DTBP) purified from Streptomyces sp. KCA1 from Phyllanthus niruri, isolation, characterization, antibacterial and anticancer properties. J. King Saud Univ. Sci. 34:102088. doi: 10.1016/j.jksus.2022.102088
Shafi, J., Tian, H., and Ji, M. S. (2017). Bacillus species as versatile weapons for plant pathogens, a review. Biotechnol. Biotec. Eq. 31, 446–459. doi: 10.1080/13102818.2017.1286950
Shi, C., Xiang, L., and Jiahu, G. (2024). Exploring the frontier of fruit diseases management, advances in nano-based and biocontrol strategies and underlying action mechanism. S. Afr. J. Bot. 166, 612–623. doi: 10.1016/j.sajb.2024.01.060
Shin, Y. H., Ledesma, M., Whitman, S., Tyson, J., Zange, B., Kim, K. D., et al. (2021). Suppression of pipe rot on 'Zesy002' kiwifruit with commercial agrochemicals. Plant Pathol. J. 37, 347–355. doi: 10.5423/PPJ.OA.03.2021.0046
Soltani, B., Ghorbanpour, M., Ziegler, C. J., Ebadi-Nahari, M., and Mohammad-Rezaei, R. (2020). Nickel (II) and cobalt (II) complexes with bidentate nitrogen-sulfur donor pyrazole derivative ligands, syntheses, characterization, X-ray structure, electrochemical studies, and antibacterial activity. Polyhedron 180:114423. doi: 10.1016/j.poly.2020.114423
Torres-Palazzolo, C., Ferreyra, S., Hugalde, I. P., Kuhn, Y., Combina, M., and Ponsone, M. L. (2024). Recent advances in biocontrol and other alternative strategies for the management of postharvest decay in table grapes. Int. J. Food Microbiol. 420:110766. doi: 10.1016/j.ijfoodmicro.2024.110766
Wang, J., Zhang, J., Ma, J., Liu, L., Li, J., Shen, T., et al. (2022). The major component of cinnamon oil as a natural substitute against Fusarium solani on Astragalus membranaceus. J. Appl. Microbiol. 132, 3125–3141. doi: 10.1111/jam.15458
Wei, L., Chen, Y., Tan, W., Li, Q., Gu, G., Dong, F., et al. (2018). Synthesis, characterization, and antifungal activity of pyridine-based triple quaternized chitosan derivatives. Molecules 23:2604. doi: 10.3390/molecules23102604
Weisburg, W. G., Barns, S. M., Pelletier, D. A., and Lane, D. J. (1991). 16S ribosomal DNA amplification for phylogenetic study. J. Bacteriol. 173, 697–703. doi: 10.1128/JB.173.2.697-703.1991
Wojdylo, A., Nowicka, P., Oszmianski, J., and Golis, T. (2017). Phytochemical compounds and biological effects of Actinidia fruits. J. Funct. Foods 30, 194–202. doi: 10.1016/j.jff.2017.01.018
Wu, C. Y., Wang, C. Y., Sun, G. J., Li, Y. Q., Liang, Y., Hua, D. L., et al. (2023). Antibacterial characteristics of allyl methyl disulfide and dimethyl trisulfide of Allium tenuissimum flower essential oil against Escherichia coli O157, H7. Ind. Crop. Prod. 202:117058. doi: 10.1016/j.indcrop.2023.117058
Xie, Y., Peng, Q., Ji, Y., Xie, A., Yang, L., Mu, S., et al. (2021). Isolation and identification of antibacterial bioactive compounds from Bacillus megaterium L2. Front. Microbiol. 12:645484. doi: 10.3389/fmicb.2021.645484
Xing, S. C., Mi, J. D., Chen, J. Y., Hu, J. X., and Liao, X. D. (2020). Metabolic activity of Bacillus coagulans R11 and the health benefits of and potential pathogen inhibition by this species in the intestines of laying hens under lead exposure. Sci. Total Environ. 709:134507. doi: 10.1016/j.scitotenv.2019.134507
Yan, F., Li, C., Ye, X., Lian, Y., and Wang, X. (2020). Antifungal activity of lipopeptides from Bacillus amyloliquefaciens MG3 against Colletotrichum gloeosporioides in loquat fruits. Blol. Control. 146:104281. doi: 10.1016/j.biocontrol.2020.104281
Yang, J., Chen, Y. Z., Yu, X. W., Tao, L., Zhang, Y. D., Wang, S. R., et al. (2021). Inhibitory effects and mechanisms of vanillin on gray mold and black rot of cherry tomatoes. Pestic. Biochem. Physiol. 175:104859. doi: 10.1016/j.pestbp.2021.104859
Yang, S., Song, M., Qi, L., Liu, G., Zhou, S., Qiao, Y., et al. (2023). Effectiveness of a novel endophyte Bacillus velezensis strain B1 in the biocontrol of pear postharvest ring rot. Sci. Hortic. 9:1049. doi: 10.3390/horticulturae9091049
Yin, Y. N., Miao, J. Q., Shao, W. Y., Liu, X. L., Zhao, Y. F., and Ma, Z. H. (2023). Fungicide resistance, Progress in understanding mechanism, monitoring, and management. Phytopathology 113, 707–718. doi: 10.1094/phyto-10-22-0370-kd
Yuan, H., Shi, B., Wang, L., Huang, T., Zhou, Z., Hou, H., et al. (2022). Isolation and characterization of Bacillus velezensis strain P2-1 for biocontrol of apple postharvest decay caused by Botryosphaeria dothidea. Front. Microbiol. 12:808938. doi: 10.3389/fmicb.2021.808938
Zhang, X., Li, B., Wang, Y., Guo, Q., Lu, X., Li, S., et al. (2013). Lipopeptides, a novel protein, and volatile compounds contribute to the antifungal activity of the biocontrol agent Bacillus atrophaeus CAB-1. Appl. Microbiol. Biol. 97, 9525–9534. doi: 10.1007/s00253-013-5198-x
Zhang, J. H., Sun, H. L., Chen, S. Y., Zeng, L., and Wang, T. T. (2017). Anti-fungal activity, mechanism studies on α-Phellandrene and nonanal against Penicillium cyclopium. Bot. Stud. 58:13. doi: 10.1186/s40529-017-0168-8
Zheng, M., Shi, J., Shi, J., Wang, Q., and Li, Y. (2013). Antimicrobial effects of volatiles produced by two antagonistic Bacillus strains on the anthracnose pathogen in postharvest mangos. Blol Control. 65, 200–206. doi: 10.1016/j.biocontrol.2013.02.004
Zhou, Y., Gong, G., Cui, Y., Zhang, D., Chang, X., Hu, R., et al. (2015). Identification of Botryosphaeriaceae species causing kiwifruit rot in Sichuan Province, China. Plant Dis. 99, 699–708. doi: 10.1094/pdis-07-14-0727-re
Zhu, R., Zhang, X., Wang, Y., Zhang, L., Zhao, J., Chen, G., et al. (2019). Characterization of polysaccharide fractions from fruit of Actinidia arguta and assessment of their antioxidant and antiglycated activities. Carbohydr. Polym. 210, 73–84. doi: 10.1016/j.carbpol.2019.01.037
Keywords: kiwifruit soft rot, Botryosphaeria dothidea , Bacillus velezensis , antifungal activity, biocontrol
Citation: Ren C, Liu Y, Su W and Tian B (2025) Bacillus velezensis LMY3-5 for the biocontrol of soft rot in kiwifruit: antifungal action and underlying mechanisms. Front. Microbiol. 16:1562366. doi: 10.3389/fmicb.2025.1562366
Edited by:
Tatjana Cosic, University of Belgrade, SerbiaReviewed by:
Farhan Nabi, South China Agricultural University, ChinaMohammad Sayyar Khan, University of Agriculture, Peshawar, Pakistan
Copyright © 2025 Ren, Liu, Su and Tian. This is an open-access article distributed under the terms of the Creative Commons Attribution License (CC BY). The use, distribution or reproduction in other forums is permitted, provided the original author(s) and the copyright owner(s) are credited and that the original publication in this journal is cited, in accordance with accepted academic practice. No use, distribution or reproduction is permitted which does not comply with these terms.
*Correspondence: Bing Tian, dGlhbmJpbmc1NjVAMTYzLmNvbQ==
†These authors have contributed equally to this work and share first authorship