- 1Department of Pediatric Surgery, Erasmus University Medical Center – Sophia Children's Hospital, Rotterdam, Netherlands
- 2Department of Neuroscience, Erasmus University Medical Center, Rotterdam, Netherlands
- 3Department of Radiology and Epidemiology, Erasmus University Medical Center, Rotterdam, Netherlands
- 4Netherlands Institute for Neuroscience, Royal Netherlands Academy of Arts & Sciences, Amsterdam, Netherlands
Background: There is rapidly emerging interest in music interventions in healthcare. Music interventions are widely applicable, inexpensive, without side effects, and easy to use. It is not precisely known how they exert positive effects on health outcomes. Experimental studies in animal models might reveal more about the pathophysiological mechanisms of music interventions.
Methods: We performed a systematic review of experimental research in rodents. The electronic databases EMBASE, Medline(ovidSP), Web-Of-Science, PsycINFO, Cinahl, PubMed publisher, Cochrane, and Google scholar were searched for publications between January 1st 1960 and April 22nd 2017. Eligible were English–written, full-text publications on experimental research in rodents comparing music vs. a control situation. Outcomes were categorized in four domains: brain structure and neuro-chemistry; behavior; immunology; and physiology. Additionally, an overview was generated representing the effects of various types of music on outcomes. Bias in studies was assessed with the SYRCLE Risk of Bias tool. A meta-analysis was not feasible due to heterogeneous outcomes and lack of original outcome data.
Results: Forty-two studies were included. Music-exposed rodents showed statistically significant increases in neuro-chemistry, such as higher BDNF levels, as well as an enhanced propensity for neurogenesis and neuroplasticity. Furthermore, music exposure was linked with statistically significantly improved spatial and auditory learning, reduced anxiety-related behavior, and increased immune responses. Various statistically significant changes occurred in physiological parameters such as blood pressure and (para)sympathetic nerve activity following music interventions. The majority of studies investigated classical music interventions, but other types of music exerted positive effects on outcomes as well. The SYRCLE risk of bias assessment revealed unclear risk of bias in all studies.
Conclusions: Music interventions seem to improve brain structure and neuro-chemistry; behavior; immunology; and physiology in rodents. Further research is necessary to explore and optimize the effect of music interventions, and to evaluate its effects in humans.
Introduction
There is growing interest in music interventions and music therapy in healthcare. Music interventions have a wide applicability, and the low cost, lack of side effects and ease of use make it an interesting intervention. Music interventions involve application of music in order to improve a clinical outcome, and can be administered recorded or live. They have been widely investigated in humans and can be linked to reduced depression levels in older people (Chan et al., 2011), to reduced disruptive behaviors and anxiety, and improved cognitive functioning in patients with dementia (Chang et al., 2015). A large number of studies have shown that music interventions alleviate anxiety and pain around medical procedures (Hole et al., 2015; Vetter et al., 2015) and surgical procedures (Kuhlmann et al., 2018). Music may have a beneficial effect on anxiety, systolic blood pressure, heart-rate, respiratory rate, quality of sleep, and pain in patients with coronary heart disease (Bradt et al., 2013), and might reduce blood-pressure in chronic hypertension (Kuhlmann et al., 2016). Lastly, music interventions appear to enhance immune function and to affect neuro-endocrine responses, such as a decrease in cortisol (Fancourt et al., 2014).
Music interventions are thought to not only exert their effects in humans by improving relaxation or providing distraction for a specific situation, but also to achieve specific physiological changes in the human body. The exact mechanism of action remains unknown. Music listening can influence a person's emotions and moods (Bennett and Lengacher, 2009; Mavridis, 2015) by activating specific pleasure areas in the limbic system, such as the nucleus accumbens, amygdala, and hippocampus (Blood and Zatorre, 2001; Menon and Levitin, 2005; Berridge and Kringelbach, 2015; Mavridis, 2015). These activations in turn may release neuropeptides, such as dopamine, and endogenous opioids (Blood and Zatorre, 2001; Mavridis, 2015). It cannot be excluded that such effects also occur in animals. Some studies in rodents indeed have shown that music exposure enhanced the expression of neuropeptides in the limbic system, which are known to be involved in pleasure and reward control (Sutoo and Akiyama, 2004; Feduccia and Duvauchelle, 2008; Tasset et al., 2012).
Moreover, several experimental studies in healthy rodents and in rodent disease models found similar effects as reported in humans, such as enhanced spatial ability (Xing et al., 2016c), improved neuroplasticity (Kirste et al., 2015), anxiety reduction (Escribano et al., 2014), blood pressure lowering (Sutoo and Akiyama, 2004), and increasing immune function (Uchiyama et al., 2012; Gao et al., 2016).
The outcomes of systematic experimental studies in animal models could be of value in understanding the working mechanisms of music interventions and extending clinical applicability of therapies. To answer the question whether music interventions exert effects on brain structure, neurochemistry, behavior, immunology, and physiology in rodents, we performed a systematic review of randomized experimental studies investigating music interventions in rodents compared to control situations.
Methods
Study Design
We performed a systematic review of the literature, and reported this following the PRISMA statement for transparent reporting of systematic reviews (Moher et al., 2009).
Search Strategy and Data Sources
On April 22nd, 2017, a systematic literature search was performed in the electronical databases EMBASE, Medline(ovidSP), Web-Of-Science, PsycINFO, Cinahl, PubMed publisher, Cochrane, and Google scholar for publications that would be relevant to answer the research question (see Supplementary Material I Search Strategy). Titles and abstracts of citations were screened for relevance, and full texts of relevant citations were screened for relevance by two investigators (AK and AR) independently. In case of disagreement a third researcher (JJ) was consulted and consensus was negotiated.
Participants, Interventions, Comparators
Studies meeting the following criteria were considered for inclusion: (1) experimental study performed in rats or mice; (2) investigating the effect of music interventions on neuronal processes, behavioral effects, endocrine and/or inflammatory responses, or physiological conditions; (3) comparing the effect of a music intervention with a comparator situation without music, referred to as “control;” (4) available full-text article; (5) written in English; (6) published after 1/1/1960. There were neither limitations to the type of music administered, the music had to contain melody, harmony, and rhythm (in case the intervention solely consisted of an auditory enrichment, such as white noise, the study was excluded); nor to the type of control condition. If study populations overlapped between studies, only the most extensively described study was included.
Data Extraction and Data Analysis
The following study characteristics were collected in an Excel spreadsheet (Google Sheets, 2015): authors, year of publication, animal model characteristics (species, sex, age, number of animals, disease induced characteristics), music intervention (type, timing, duration, loudness), specific description of the music and genre, control condition (type, timing, duration, loudness), and performed tests. Study quality was assessed by two researchers (AK and AR) using the Systematic Review Center for Laboratory animal Experimentation (SYRCLE) Risk of Bias tool, which is the adapted version for animal studies of the Cochrane Risk of Bias tool (Hooijmans et al., 2014). Outcome measures were extracted by two persons separately and categorized into four areas: 1. brain structure and neuro-chemistry; 2. behavior; 3. immunology; and 4. physiology. Additionally, an overview was generated representing the effects of various types of music on outcomes. A meta-analysis was not performed due to the heterogeneity in outcomes and the lack of reporting original outcome data in reviewed studies.
Results
Study Selection and Characteristics
The literature search resulted in 2,784 citations after removal of duplicates. Following eligibility assessment, 42 full-text articles were eligible for inclusion (see Figure 1). Detailed study characteristics are presented in Table 1. Figure 2 represents an overview of domains in rodents that seem affected by music. Thirty studies (71.4%) were in rats; twelve in mice. All studies investigated recorded music interventions played by loudspeaker. Control conditions were described as no music (17 studies, 40%); ambient noise (14 studies, 33%); white noise (5 studies, 13%); undisturbed situation (5 studies, 12%); and no stress (1 study, 2%). Twenty-eight studies (67%) involved several interventions/comparators (see Table 1).
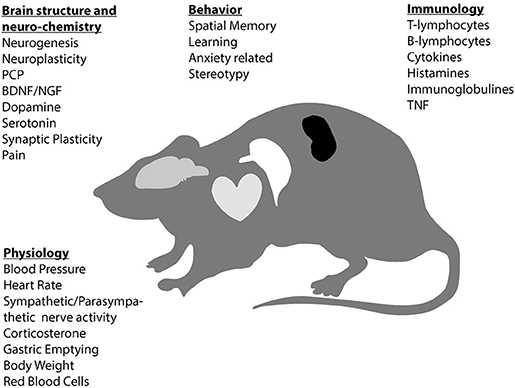
Figure 2. Music affects different domains in rodents. PCP, precursor cell proliferation; BDNF, brain derived neurotrophic factor; NGF, nerve growth factor; TNF, tumor necrosis factor.
Risk of Bias
All studies were assessed as unclear risk of bias according to the SYRCLE risk of bias tool (see Supplementary Material II SYRCLE Risk of Bias tool). Most studies did describe animal and housing characteristics, and reported some attrition bias. Information on sequence generation, allocation concealment, blinding of caregivers/investigators and random outcome assessment was barely reported.
Findings: Music and Brain Structure and Neuro-Chemistry
Twenty-three studies investigated the effects of music on the neuro-anatomy of the brain (see Table 2) (Morton et al., 2001; Nunez et al., 2002; Kim et al., 2004, 2006, 2013; Sutoo and Akiyama, 2004; Chikahisa et al., 2006; Angelucci et al., 2007a,b; Xu et al., 2007, 2009; Feduccia and Duvauchelle, 2008; Meng et al., 2009; Li et al., 2010; Marzban, 2012; Tasset et al., 2012; Kirste et al., 2015; Sheikhi and Saboory, 2015; Gao et al., 2016; Jiang et al., 2016; Lee et al., 2016; Xing et al., 2016a,c), such as neurogenesis and neuroplasticity as measured by precursor cell proliferation by bromodeoxyuridine (BrdU) labeled cells, levels of brain derived neurotrophic factor (BDNF) expression, and nerve growth factor (NGF); levels of dopamine and serotonine; seizures; expression of amyloid-β; and effects on neuronal pain pathways.
All four studies that investigated effects of music on levels of BrdU-cells found increased levels compared to a control condition (Kim et al., 2006, 2013; Kirste et al., 2015; Lee et al., 2016). Prenatal music increased the number of cells in the motor cortex and somatosensory cortex (Kim et al., 2013) as well as in the hippocampal CA1, CA2, and CA3 regions, but not in the dental gyrus (Kim et al., 2006). Moreover, the brain cells of rat fetuses exposed to music were morphologically more complex than those of rat fetuses not exposed to music (Sheikhi and Saboory, 2015). Music statistically significantly increased levels of BDNF compared to comparator situations in seven out of eight studies (Angelucci et al., 2007a,b; Li et al., 2010; Marzban, 2012; Lee et al., 2016; Xing et al., 2016a,c)—specifically in cells of the dorsal CA3 region of the hippocampus (HC), the dentate gyrus (Xing et al., 2016a), the prefrontal cortex, amygdala, and hypothalamus (Angelucci et al., 2007a,b; Li et al., 2010); whereas the NGF level was not altered in cells of the CA1 region (Xing et al., 2016a). One study found a decrease of BDNF in the cortex and no change in the HC and the cerebellum compared to comparator conditions (Chikahisa et al., 2006). One study found that music decreased nerve growth factor in the hypothalamus (Angelucci et al., 2007b), while it had no impact on the HC, frontal cortex or striatum (Angelucci et al., 2007a). In the same two studies, BDNF levels were elevated in both the HC and the hypothalamus.
The three studies investigating effects of music on dopamine levels in the brain (Sutoo and Akiyama, 2004; Feduccia and Duvauchelle, 2008; Tasset et al., 2012) found either an increase of dopamine in the nucleus accumbens (Feduccia and Duvauchelle, 2008); in the prefrontal cortex, mesencephalon and the striatum (Tasset et al., 2012); or no differences in dopamine in the motor cortex, somatosensory cortex, or nucleus accumbens (Sutoo and Akiyama, 2004). Music prevented the decrease of dopamine after administration of a D2-receptor antagonist in rats (Tasset et al., 2012). In another study, music up-regulated the expression of dopamine-related genes in mice (Meng et al., 2009). Effects of music on serotonin levels were investigated in two studies (Kim et al., 2004; Feduccia and Duvauchelle, 2008): prenatal music decreased serotonin synthesis in the dorsal and median raphe nuclei in the offsprings (Kim et al., 2004); but it increased serotonin in the nucleus accumbens after administration of methamphetamine (Feduccia and Duvauchelle, 2008).
When methamphetamine was injected in mice, exposure to either rave or classical music increased the numbers of seizures and deaths, suggesting increased methamphetamine toxicity (Morton et al., 2001). Rats exposed to music showed a significant increase in the expression of the NMDA receptor NR2B protein in their auditory cortex (Xu et al., 2009). Similarly, the expression of another glutamate receptor subunit which can be involved in synaptic plasticity, GluR2, was also significantly increased in the auditory cortex following music exposure, suggesting induced plasticity in the auditory system (Xu et al., 2007).
In a mouse model of Alzheimer's disease, addition of music to electro-acupuncture treatment statistically significantly improved the glucose metabolism level in the mice's brains, while the expression of amyloid-β, which is normally accumulated in Alzheimer's disease, was decreased (Jiang et al., 2016). Lastly, the one study examining effects of music on cancer bone pain found less pain intensity as well as decreased expression of p38α and p38β in the dorsal ganglia, which are involved in processing chronic neuropathic, inflammatory, and cancer pains (Gao et al., 2016).
Findings: Music and Behavior
Twenty-one studies investigated the effects of music on behavioral outcomes (see Table 3) (Rauscher et al., 1998; Morton et al., 2001; Chikahisa et al., 2006, 2007; Kim et al., 2006; Angelucci et al., 2007a; Feduccia and Duvauchelle, 2008; Meng et al., 2009; Xu et al., 2009; Amagdei et al., 2010; Li et al., 2010; da Cruz et al., 2011; de Camargo et al., 2013; Escribano et al., 2014; Cruz et al., 2015; Jiang et al., 2016; Lee et al., 2016; Xing et al., 2016a,b,c; Yazdani et al., 2016)—specifically learning abilities, anxiety-related behavior and stereotypic behavior as investigated by behavioral tests explained in Supplementary Material III.
Music interventions enhanced learning abilities of rodents, specifically those involved with spatial learning (Rauscher et al., 1998; Chikahisa et al., 2006; Kim et al., 2006; Xu et al., 2009; Amagdei et al., 2010; Jiang et al., 2016; Lee et al., 2016; Xing et al., 2016a,b,c; Yazdani et al., 2016). Moreover, music statistically significantly decreased anxiety-related behavior in seven out of nine studies (Angelucci et al., 2007a; Chikahisa et al., 2007; Meng et al., 2009; Li et al., 2010; de Camargo et al., 2013; Escribano et al., 2014; Cruz et al., 2015); the remaining two studies found no differences between music and comparator groups (Amagdei et al., 2010; da Cruz et al., 2011). The anxiety-decreasing effect of music diminished after ovariectomy and was restored by progesterone (Chikahisa et al., 2007; Escribano et al., 2014). Music seemed to enhance anxiolytic effects of simvastatin (da Cruz et al., 2011; de Camargo et al., 2013). Influence of music on stereotypic behavior was investigated in two studies; music enhanced stereotypic behavior after administration of methamphetamine, but not of saline (Morton et al., 2001; Feduccia and Duvauchelle, 2008).
Findings: Music and Immunology
Seven studies investigated the effects of music on immunological outcomes (see Table 4) (McCarthy et al., 1992; Nunez et al., 2002; Lu et al., 2010; Uchiyama et al., 2012; Zhang et al., 2013; Kim et al., 2015; Gao et al., 2016), such as specific and non-specific immunity; cytokines and histamines; anaphylaxis; tumor growth; and post-transplantation immunity.
Music exposure enhanced the numbers of lymphocytes and natural killer cells as well as the levels of T-cell proliferation and phagocytosis (McCarthy et al., 1992; Nunez et al., 2002; Zhang et al., 2013). Noise stress induced by loud rock music resulted in statistically significantly decreased production of superoxide anion and IL-1, suggestive of deprived leucocyte function (McCarthy et al., 1992). Gong tone music up-regulated plasma-cells and proliferation of T-cells in rats with deprived spleen function (Zhang et al., 2013), and music exposure significantly decreased the number of eosinophils and increased cytokine levels in asthmatic rats compared to controls (Lu et al., 2010). Mice exposed to Korean Buk music showed a statistically significantly decreased production of cytokines and histamines as well as statistically significantly lower mortality from anaphylactic shock (Kim et al., 2015). Decreased tumor volume and decreased area of metastasis was found in the presence of music (Nunez et al., 2002; Gao et al., 2016). Rodents exposed to opera or classical music had statistically significantly prolonged survival after heart transplantation. Moreover, adoptive transfer of splenocytes and T-cells from music-exposed rodents into naïve recipients was associated with prolonged survival of these recipients (Uchiyama et al., 2012).
Findings: Music and Physiology
Sixteen studies investigated effects of music on physiological outcomes in rodents (see Table 5) (Bueno and Gue, 1988; McCarthy et al., 1992; Sutoo and Akiyama, 2004; Chikahisa et al., 2006; Kim et al., 2006; Angelucci et al., 2007a,b; Nakamura et al., 2007, 2009; Erken et al., 2008; Lemmer, 2008; Lu et al., 2010; Akiyama and Sutoo, 2011; Tasset et al., 2012; Sheikhi and Saboory, 2015; Gao et al., 2016), including blood pressure and heart rate; sympathetic and parasympathetic nerve activity; corticosterone levels; body weight and digestion; and red blood cell activity.
Four studies investigated effects of classical string music on blood pressure (Sutoo and Akiyama, 2004; Nakamura et al., 2007; Lemmer, 2008; Akiyama and Sutoo, 2011); of which one also investigated effects on heart rate (Lemmer, 2008). A statistically significantly decrease in blood pressure was noted in three out of four studies. High-frequency music was more effective in decreasing blood pressure than was low-frequency music, with an absent effect at the lowest frequencies (Akiyama and Sutoo, 2011). Sympathetic nerve activity and blood pressure decreased after music exposure (Nakamura et al., 2007) while parasympathetic nerve activity increased (Nakamura et al., 2009). Three out of four studies found significantly decreased corticosterone levels after music interventions (Lu et al., 2010; Tasset et al., 2012; Sheikhi and Saboory, 2015). While exposure to music was followed by a statistically significantly decrease of blood corticosterone in pregnant rats (Sheikhi and Saboory, 2015), this phenomenon was not seen in the offspring upon pre- and postnatal daily exposure to music (Chikahisa et al., 2006). Classical music exposure decreased red blood cell functioning (Erken et al., 2008). Acoustic stress by rock music increased gastric emptying, however administration of anti-corticotropic releasing factor prevented this (Bueno and Gue, 1988). Of six studies that evaluated the effect of music on body weight (Chikahisa et al., 2006; Kim et al., 2006; Angelucci et al., 2007a,b; Sheikhi and Saboory, 2015; Gao et al., 2016), one found statistically significantly weight reduction (Gao et al., 2016).
Types of Music
Overall, studies used a wide range of music interventions. Classical music was the most investigated intervention (29 studies, 70.7%; of which 14 studies used Mozart's sonata for two pianos, K.448). Table 6 represents an overview of the genres of music interventions and their effects on outcomes. Most studies investigating classical music found positive effects on outcomes regarding brain structure and neurochemistry, and on outcomes regarding behavior such as spatial memory or anxiety. Positive effects on physiological outcomes were also seen and suggested decreased sympathetic activity. Majority of these classical music studies investigated Mozart music, specifically Mozart K.448. Retrograde versions of this music piece had negative effects on spatial memory, this effect was also present when rodents heard the music for the first time. Furthermore, blood pressure decreasing effects were seen in high frequency music, while these effects were not present in low frequency music.
Other types of music showed variable effects. New age music increased neuroplasticity in one study compared to the control group, but did not affect neurogenesis or immunologic outcomes. Anxiety and learning were however improved.
Cultural music was investigated in two studies that both found positive results on immunologic functioning. Up-beat music also showed variable results. Rock music did not positively affect any outcomes, whereas electronic house music did decrease anxiety, and euphoric house music did increase dopamine and serotonin levels.
Studies that used non-specified music interventions found positive results as well, such as increased spatial memory, increased learning and increased physical performance. Studies that used music as acoustic stressor did not find positive results on outcomes.
Seven of 42 studies compared several types of music interventions (Rauscher et al., 1998; Morton et al., 2001; Xu et al., 2007; Erken et al., 2008; Lemmer, 2008; Nakamura et al., 2009; Uchiyama et al., 2012) and allowed direct comparison of music on the outcomes due to the equality of the study conditions. A statistically significant increase in functional brain activity and plasticity was found after exposure to Nightwish music, this effect was not present after exposure to Nostalgy music (Xu et al., 2007), however absence of specific description of these two music pieces inhibited a formal comparison between the types of music. Electronic music temporarily decreased anxiety after supplementation of methamphetamine whereas classical Bach music did not (Morton et al., 2001), and classical Mozart music statistically significantly increased spatial memory compared to minimalistic classical music by Philip Glass (Rauscher et al., 1998). There was no difference in neurologic outcomes after exposure to loud classical music by Bach, or to loud modern electronic music by The Prodigy (Morton et al., 2001). Both classical and opera music significantly improved immune function and graft survival, whereas New Age music did not had any significant effect on these parameters (Uchiyama et al., 2012). Ligeti rock music, but not Mozart music, resulted in a long-lasting blood pressure decreasing effect, Mozart music on the other hand was significantly effective in reducing heart rate (Erken et al., 2008). Both classical and rock music affected the erythrocyte response to stress with higher degree of significance in the classical music group (Erken et al., 2008). Exposure to Schumann's Traumerei resulted in decreased sympathetic activity, but exposure to an Etude by Chopin did not (Nakamura et al., 2009).
Discussion
Summary of Findings
The results of this systematic review indicate that music exposure can exert positive effects on rodents' neurological, behavioral, immunological, and physiological outcomes. These results are broadly consistent with studies in humans that found that music exposure can positively affect brain structure and chemistry (Johansson, 2011; Yeh et al., 2015), behavioral read-outs (Chan et al., 2011; Thoma et al., 2013; Baird and Samson, 2015; Hole et al., 2015; Vetter et al., 2015), immunological responses (Conrad et al., 2007; Fancourt et al., 2014), and physiological parameters (Bekiroglu et al., 2013; Hole et al., 2015).
Music exposure increased rodents' spatial memory and learning in all studies that examined it. Music seems to specifically affect spatial memory, the one study examining non-spatial memory did not find any differences between the music and control situations (de Camargo et al., 2013). Exposure to music decreased anxiety in all included studies. Both spatial memory and anxiety might be affected by the level of BDNF. Low levels of BDNF have been associated with anxiety and aggressive behavior in mice (Akbarian et al., 2002; Li et al., 2010) and with anxiety and depression in humans (Martinowich et al., 2007; Brunoni et al., 2008). This protein is involved in synaptic plasticity, learning, and memory areas of the brain, such as the hypothalamus and hippocampus, and regulates neuronal structure and function (Mizuno et al., 2000; Chikahisa et al., 2006; Angelucci et al., 2007b; Marzban, 2012). In most studies examining, BDNF levels were elevated following exposure to music, and this might explain the reduced anxiety. The improved behavioral performance on spatial memory tasks and anxiety tests after music interventions is likely to be, at least in part, the effect of increased levels of BDNF. This finding suggests that music exposure has the potential to improve neuroplasticity and neurogenesis in the brain. This could be of value in the treatment of psychological disorders or acquired brain injuries and should be further explored (Kim et al., 2006; Kirste et al., 2015; Xing et al., 2016a).
Furthermore, music exposure possibly counteracts the adverse effects of stress and thereby enhances the immune function. Music interventions were associated with increased functions of cellular and humoral immunity, increased phagocytosis and increased production of lymphocytes and immunoglobulins (Zhang et al., 2013). In rodent cancer models, music exposure was associated with lower tumor volume and smaller area of metastasis (Nunez et al., 2002; Gao et al., 2016). Regarding allergic reactions such as anaphylaxis, however, the immune system seemed tempered in the presence of music—with lower production of cytokines and histamines and thereby less mortality (Kim et al., 2015). Remarkably, this effect of music also manifests itself in survival after transplantation. Enhanced production of anti-inflammatory cytokines and regulatory T-cells restrained the immune-system in the presence of music and thereby significantly lengthened the survival times of transplants (Uchiyama et al., 2012). Comparable effects of music on immunological and neurochemical functions have also been reported in humans (Bartlett et al., 1993; Stefano et al., 2004; Fancourt et al., 2014). This promising result should be further investigated.
Physiological effects induced by music are commonly explained by attenuation of autonomic function by stress reduction. Stress affects the hypothalamic-pituitary-adrenal axis and the sympathetic nervous system in humans and animals alike. Stress reduction causes the sympathetic activity to shift to more parasympathetic activity, resulting in lower heart rate and blood pressure. The blood pressure-reducing effect of music has extensively been described in humans (Bekiroglu et al., 2013; Kuhlmann et al., 2016), and it may hold for rodents as well. Corticosterone, the rodent's equivalent of human cortisol, is involved in regulating stress-responses and is an important biomarker for stress. Music interventions were associated with reduced corticosterone levels in several animal models (Chikahisa et al., 2006; Tasset et al., 2012). Comparable effects of music on cortisol have been reported in humans (Leardi et al., 2007; Koelsch et al., 2011). In addition, the blood pressure reduction might be induced by autonomic regulation via sympathetic suppression by histaminergic receptors (Nakamura et al., 2007), or on calcium level regulation via the calmodulin system (Sutoo and Akiyama, 2004; Xu et al., 2007). An increase of calcium ions enhances dopamine synthesis, and increased dopamine levels in turn may inhibit sympathetic activity via specific D2 receptors and thus reduce blood pressure (Sutoo and Akiyama, 1997, 2004; Tasset et al., 2012). Increased calcium influx in the brain might be due to excitatory impulses, also represented by enhanced synaptic transmission (Xu et al., 2007). Enhanced synaptic transmission can result in improved learning and memory functions, and boosts the formation of neural networks during brain development (Ozawa et al., 1998; Dumas, 2005; Xu et al., 2007).
Working Mechanisms of Music
The specific mechanisms by which music exerts its effects are unknown. It seems that at least the auditory pathway must be intact, as effects of music were not seen after lesions of the eardrum (Uchiyama et al., 2012), cochlea, auditory cortex, and suprachiasmatic nucleus (Nakamura et al., 2007). As for the type of music, most of the studies in this review used classical music, with a preference for music composed by Mozart. This may be described to what is known as the Mozart-effect (Rauscher et al., 1998), which implies an enhanced effect on spatial memory by listening to music composed by Mozart. As the findings of replication studies are inconsistent (Newman et al., 1995; Rideout and Laubach, 1996; Steele et al., 1999) a firm conclusion on the Mozart-effect cannot be drawn. Most of the 12 studies investigating other types of music, including folk music such as Korean Buk music (Kim et al., 2015) or Gong tone music (Zhang et al., 2013) found statistically significant results as well, suggesting there is more to music than just the classical component. Different physiological effects were observed when playing different musical pieces, even when the music was in the same genre (Lemmer, 2008) or from roughly the same classical style (Nakamura et al., 2009). More complex classical music seemed of more value to spatial memory than minimalistic classical music did (Rauscher et al., 1998). One study compared tonal classical music of Mozart with the avant-garde classical music of Ligeti, the latter characterized by micro tonality and dissonant harmonies that could be subjectively described as unsettling (Lemmer, 2008). Both pieces yielded opposite effects, suggesting that musical factors like tone, harmony, or melody are all important in exerting effects. Effects of specific intervals, rhythm, and melodies can also be seen in another study in which rats' spatial performance was negatively affected with reversed versions of the music, while original versions positively affected performance compared to controls (Xing et al., 2016c). In this study rhythm appeared to be a crucial element (Xing et al., 2016c). In other studies, rhythm also appeared to be important to achieve positive effects. Cultural music involving Gong tone or Buk instruments, both characterized by strong rhythmic patterns, induced positive effects on immunology (Zhang et al., 2013; Kim et al., 2015). These specific components of music triggering pathophysiological mechanisms warrant further investigation.
While low-frequency music altered or even abolished effects of music in rodents, higher frequency notes resulted in better responses (Akiyama and Sutoo, 2011; Uchiyama et al., 2012). Hearing abilities of rodents differ from those of humans, varying from 500 Hz to 64 kHz in rats and 2 kHz to 80 kHz in mice to 20 Hz to 20 kHz in humans (Heffner and Heffner, 2007; Rosen and Howell, 2013), which could explain improvement of results with higher frequency notes. No significant differences on neurologic outcomes were found between exposure to classical or rave music after methamphetamine injection (Morton et al., 2001), however, music was played loudly and this might have been so stressful that it suppressed any effects. In addition, impaired immune function was seen after exposure to loud rock music (McCarthy et al., 1992), again suggesting that music volume might affect any outcomes.
Limitations
The outcome of this systematic review faces several limitations. The sample sizes of the included studies were generally small. Additionally, we found a substantial unclear risk of bias (see Supplementary Material II) with the SYRCLE risk of bias tool (Hooijmans et al., 2014). Music interventions were heterogeneous and sometimes sparsely described. Furthermore, studies were performed in different populations and also with various types of control situations. Not every study considered the day-night cycle of rodents. When interpreting the results of this review, one should be aware of these limitations.
Conclusion
This systematic review finds music interventions to improve outcomes of brain structure and neuro-chemistry, behavior, immunology, and physiology in rodents. These results support application of music as intervention in many healthcare areas. Future studies in both rodents and humans could look more into matters of musical complexity, rhythm, and pitch as well as the frequency with which music interventions are offered.
Author Contributions
AK, AdR, and JJ conceived the study idea. AK and AdR coordinated the systematic review. AK and AdR screened abstracts and full texts. AK and AdR wrote the first draft of the manuscript and judged risk of bias in the studies. AK, AdR, MH, CDZ, and JJ interpreted the data. AK, AdR, MH, CDZ, and JJ critically revised the manuscript. AK, AdR, MH, CDZ, and JJ had full access to all of the data in the study and can take responsibility for the integrity of the data and the accuracy of the data analysis.
Conflict of Interest Statement
CDZ was supported by ZonMw, NWO-ALW, ERC-Adv and ERC-PoC outside of the submitted work. MH was supported by ZonMw, EIBIR, ESR, and CUP outside of the submitted work.
The remaining authors declare that the research was conducted in the absence of any commercial or financial relationships that could be construed as a potential conflict of interest.
Acknowledgments
We kindly thank Wichor Bramer, biomedical information specialist, Erasmus Medical Center, Rotterdam, the Netherlands; for his expertise with the literature search. We thank Victor Fu, Ph.D. student, Erasmus Medical Center, Rotterdam, the Netherlands; for his help with screening papers of the literature search update. Ko Hagoort, MA, Erasmus Medical Center, Rotterdam, the Netherlands; is thanked for critically reviewing the manuscript.
Supplementary Material
The Supplementary Material for this article can be found online at: https://www.frontiersin.org/articles/10.3389/fnbeh.2018.00301/full#supplementary-material
Abbreviations
SYRCLE, Systematic Review Centre for Laboratory animal Experimentation; BrdU, bromodeoxyuridine; BDNF, brain derived neurotrophic factor; HC, hippocampus; NMDA, N-methyl-D-aspartate; NR2B, subunit NMDA receptor; GluR2, glutamate receptor.
References
Akbarian, S., Rios, M., Liu, R. J., Gold, S. J., Fong, H. F., Zeiler, S., et al. (2002). Brain-derived neurotrophic factor is essential for opiate-induced plasticity of noradrenergic neurons. J. Neurosci. 22, 4153–4162. doi: 10.1523/JNEUROSCI.22-10-04153.2002
Akiyama, K., and Sutoo, D. (2011). Effect of different frequencies of music on blood pressure regulation in spontaneously hypertensive rats. Neurosci. Lett. 487, 58–60. doi: 10.1016/j.neulet.2010.09.073
Amagdei, A., Baltes, F. R., Avram, J., and Miu, A. C. (2010). Perinatal exposure to music protects spatial memory against callosal lesions. Int. J. Dev. Neurosci. 28, 105–109. doi: 10.1016/j.ijdevneu.2009.08.017
Angelucci, F., Fiore, M., Ricci, E., Padua, L., Sabino, A., and Tonali, P. A. (2007a). Investigating the neurobiology of music: brain-derived neurotrophic factor modulation in the hippocampus of young adult mice. Behav. Pharmacol. 18, 491–496. doi: 10.1097/FBP.0b013e3282d28f50
Angelucci, F., Ricci, E., Padua, L., Sabino, A., and Tonali, P. A. (2007b). Music exposure differentially alters the levels of brain-derived neurotrophic factor and nerve growth factor in the mouse hypothalamus. Neurosci. Lett. 429, 152–155. doi: 10.1016/j.neulet.2007.10.005
Baird, A., and Samson, S. (2015). Music and dementia. Prog. Brain Res. 217, 207–235. doi: 10.1016/bs.pbr.2014.11.028
Bartlett, D., Kaufman, D., and Smeltekop, R. (1993). The effects of music listening and perceived sensory experiences on the immune-system as measured by interleukin-1 and cortisol. J. Music Ther. 30, 194–209. doi: 10.1093/jmt/30.4.194
Bekiroglu, T., Ovayolu, N., Ergun, Y., and Ekerbicer, H. C. (2013). Effect of Turkish classical music on blood pressure: a randomized controlled trial in hypertensive elderly patients. Complement. Ther. Med. 21, 147–154. doi: 10.1016/j.ctim.2013.03.005
Bennett, M. P., and Lengacher, C. (2009). Humor and laughter may influence health, I. V. Humor and immune function. Evid. Based Compl. Alt. 6, 159–164. doi: 10.1093/ecam/nem149
Berridge, K. C., and Kringelbach, M. L. (2015). Pleasure systems in the brain. Neuron 86, 646–664. doi: 10.1016/j.neuron.2015.02.018
Blood, A. J., and Zatorre, R. J. (2001). Intensely pleasurable responses to music correlate with activity in brain regions implicated in reward and emotion. Proc. Natl. Acad. Sci. U.S.A. 98, 11818–11823. doi: 10.1073/pnas.191355898
Bradt, J., Dileo, C., and Potvin, N. (2013). Music for stress and anxiety reduction in coronary heart disease patients. Cochrane Database Syst. Rev. 12:CD006577. doi: 10.1002/14651858.CD006577.pub3
Brunoni, A. R., Lopes, M., and Fregni, F. (2008). A systematic review and meta-analysis of clinical studies on major depression and BDNF levels: implications for the role of neuroplasticity in depression. Int. J. Neuropsychopharmacol. 11, 1169–1180. doi: 10.1017/S1461145708009309
Bueno, L., and Gue, M. (1988). Evidence for the involvement of corticotropin-releasing factor in the gastrointestinal disturbances induced by acoustic and cold stress in mice. Brain Res. 441, 1–4. doi: 10.1016/0006-8993(88)91376-5
Chan, M. F., Wong, Z. Y., and Thayala, N. V. (2011). The effectiveness of music listening in reducing depressive symptoms in adults: a systematic review. Complement. Ther. Med. 19, 332–348. doi: 10.1016/j.ctim.2011.08.003
Chang, Y. S., Chu, H., Yang, C. Y., Tsai, J. C., Chung, M. H., Liao, Y. M., et al. (2015). The efficacy of music therapy for people with dementia: a meta-analysis of randomised controlled trials. J. Clin. Nurs. 24, 3425–3440. doi: 10.1111/jocn.12976
Chikahisa, S., Sano, A., Kitaoka, K., Miyamoto, K. I., and Sei, H. (2007). Anxiolytic effect of music depends on ovarian steroid in female mice. Behav. Brain Res. 179, 50–59. doi: 10.1016/j.bbr.2007.01.010
Chikahisa, S., Sei, H., Morishima, M., Sano, A., Kitaoka, K., Nakaya, Y., et al. (2006). Exposure to music in the perinatal period enhances learning performance and alters BDNF/TrkB signaling in mice as adults. Behav. Brain Res. 169, 312–319. doi: 10.1016/j.bbr.2006.01.021
Conrad, C., Niess, H., Jauch, K. W., Bruns, C. J., Hartl, W. H., and Welker, L. (2007). Overture for growth hormone: requiem for interleukin-6? Crit. Care Med. 35, 2709–2713. doi: 10.1097/00003246-200712000-00005
Cruz, J. N., Lima, D. D., Dal Magro, D. D., and Cruz, J. G. P. (2015). Anxiolytic effect of mozart music over short and long photoperiods as part of environmental enrichment in captive rattus norvegicus (Rodentia: Muridae). Scand. J. Lab. Anim. Sci. 41, 1–7.
da Cruz, J. N., de Lima, D. D., Dal Magro, D. D., and da Cruz, J. G. P. (2011). The power of classic music to reduce anxiety in rats treated with simvastatin. Basic Clin Neurosci. 2, 5–11.
de Camargo, A. M., de Lima, D. D., Dal Magro, D. D., Seubert, J. K., da Cruz, J. N., and da Cruz, J. G. P. (2013). Adjuvant effects of classical music on simvastatin induced reduction of anxiety but not object recognition memory in rats. Psychol. Neurosci. 6, 403–410. doi: 10.3922/j.psns.2013.3.19
Dumas, T. C. (2005). Developmental regulation of cognitive abilities: modified composition of a molecular switch turns on associative learning. Prog. Neurobiol. 76, 189–211. doi: 10.1016/j.pneurobio.2005.08.002
Erken, G., Bor-Kucukatay, M., Erken, H. A., Kursunluoglu, R., and Genc, O. (2008). Influence of classical and rock music on red blood cell rheological properties in rats. Med. Sci. Monit. 14, BR28–BR33.
Escribano, B., Quero, I., Feijoo, M., Tasset, I., Montilla, P., and Tunez, I. (2014). Role of noise and music as anxiety modulators: relationship with ovarian hormones in the rat. Appl. Anim. Behav. Sci. 152, 73–82. doi: 10.1016/j.applanim.2013.12.006
Fancourt, D., Ockelford, A., and Belai, A. (2014). The psychoneuroimmunological effects of music: a systematic review and a new model. Brain Behav. Immun. 36, 15–26. doi: 10.1016/j.bbi.2013.10.014
Feduccia, A. A., and Duvauchelle, C. L. (2008). Auditory stimuli enhance MDMA-conditioned reward and MDMA-induced nucleus accumbens dopamine, serotonin and locomotor responses. Brain Res. Bull. 77, 189–196. doi: 10.1016/j.brainresbull.2008.07.007
Gao, J., Chen, S., Lin, S., and Han, H. (2016). Effect of music therapy on pain behaviors in rats with bone cancer pain. J. BUON. 21, 466–472.
Heffner, H. E., and Heffner, R. S. (2007). Hearing ranges of laboratory animals. J. Am. Assoc. Lab. Anim. Sci. 46, 20–22.
Hole, J., Hirsch, M., Ball, E., and Meads, C. (2015). Music as an aid for postoperative recovery in adults: a systematic review and meta-analysis. Lancet 386, 1659–1671. doi: 10.1016/S0140-6736(15)60169-6
Hooijmans, C. R., Rovers, M. M., de Vries, R. B., Leenaars, M., Ritskes-Hoitinga, M., and Langendam, M. W. (2014). SYRCLE's risk of bias tool for animal studies. BMC Med. Res. Methodol. 14:43. doi: 10.1186/1471-2288-14-43
Jiang, J., Liu, G., Shi, S., and Li, Z. (2016). Musical electroacupuncture may be a better choice than electroacupuncture in a mouse model of Alzheimer's disease. Neural Plast. 2016:3131586. doi: 10.1155/2016/3131586
Johansson, B. B. (2011). Current trends in stroke rehabilitation. A review with focus on brain plasticity. Acta Neurol. Scand. 123, 147–159. doi: 10.1111/j.1600-0404.2010.01417.x
Kim, C. H., Lee, S. C., Shin, J. W., Chung, K. J., Lee, S. H., Shin, M. S., et al. (2013). Exposure to music and noise during pregnancy influences neurogenesis and thickness in motor and somatosensory cortex of rat pups. Int. Neurourol. J. 17, 107–113. doi: 10.5213/inj.2013.17.3.107
Kim, E. K., Kim, H., Lee, M. H., Kim, Y. S., Yang, H. Y., Chang, H. K., et al. (2004). Influence of prenatal noise and music on the 5-hydroxytryptamine synthesis and the tryptophan hydroxylase expression in the raphe nuclei of young rats. Neurosci. Res. Commun. 35, 118–129. doi: 10.1002/nrc.20026
Kim, H., Lee, M. H., Chang, H. K., Lee, T. H., Lee, H. H., Shin, M. C., et al. (2006). Influence of prenatal noise and music on the spatial memory and neurogenesis in the hippocampus of developing rats. Brain Dev. 28, 109–114. doi: 10.1016/j.braindev.2005.05.008
Kim, H. Y., Ko, K. J., Nam, S. Y., Jeong, H. J., and Kim, H. M. (2015). The sound of a buk (Korean Traditional Drum) attenuates anaphylactic reactions by the activation of estrogen receptor-beta. Int. Arch. Allergy Immunol. 167, 242–249. doi: 10.1159/000439567
Kirste, I., Nicola, Z., Kronenberg, G., Walker, T. L., Liu, R. C., and Kempermann, G. (2015). Is silence golden? Effects of auditory stimuli and their absence on adult hippocampal neurogenesis. Brain Struct. Funct. 220, 1221–1228. doi: 10.1007/s00429-013-0679-3
Koelsch, S., Fuermetz, J., Sack, U., Bauer, K., Hohenadel, M., Wiegel, M., et al. (2011). Effects of music listening on cortisol levels and propofol consumption during spinal anesthesia. Front. Psychol. 2:58. doi: 10.3389/fpsyg.2011.00058
Kuhlmann, A. Y., Etnel, J. R., Roos-Hesselink, J. W., Jeekel, J., Bogers, A. J., and Takkenberg, J. J. (2016). Systematic review and meta-analysis of music interventions in hypertension treatment: a quest for answers. BMC Cardiovasc. Disord. 16:69. doi: 10.1186/s12872-016-0244-0
Kuhlmann, A. Y. R., de Rooij, A., Kroese, L. F., van Dijk, M., Hunink, M. G. M., and Jeekel, J. (2018). Meta-analysis evaluating music interventions for anxiety and pain in surgery. Br. J. Surg. 105, 773–783. doi: 10.1002/bjs.10853
Leardi, S., Pietroletti, R., Angeloni, G., Necozione, S., Ranalletta, G., and Del Gusto, B. (2007). Randomized clinical trial examining the effect of music therapy in stress response to day surgery. Br. J. Surg. 94, 943–947. doi: 10.1002/bjs.5914
Lee, S. M., Kim, B. K., Kim, T. W., Ji, E. S., and Choi, H. H. (2016). Music application alleviates short-term memory impairments through increasing cell proliferation in the hippocampus of valproic acid-induced autistic rat pups. J. Exerc. Rehabil. 12, 148–155. doi: 10.12965/jer.1632638.319
Lemmer, B. (2008). Effects of music composed by Mozart and Ligeti on blood pressure and heart rate circadian rhythms in normotensive and hypertensive rats. Chronobiol. Int. 25, 971–986. doi: 10.1080/07420520802539415
Li, W. J., Yu, H., Yang, J. M., Gao, J., Jiang, H., Feng, M., et al. (2010). Anxiolytic effect of music exposure on BDNFMet/Met transgenic mice. Brain Res. 1347, 71–79. doi: 10.1016/j.brainres.2010.05.080
Lu, Y., Liu, M., Shi, S., Jiang, H., Yang, L., Liu, X., et al. (2010). Effects of stress in early life on immune functions in rats with asthma and the effects of music therapy. J. Asthma 47, 526–531. doi: 10.3109/02770901003801964
Martinowich, K., Manji, H., and Lu, B. (2007). New insights into BDNF function in depression and anxiety. Nat. Neurosci. 10, 1089–1093. doi: 10.1038/nn1971
Marzban, M. (2012). The effect of Mozart's music on hippocampal content of BDNF in postnatal rats. Eur. J. Neurol. 19:475.
Mavridis, I. N. (2015). Music and the nucleus accumbens. Surg. Radiol. Anat. 37, 121–125. doi: 10.1007/s00276-014-1360-0
McCarthy, D. O., Ouimet, M. E., and Daun, J. M. (1992). The effects of noise stress on leukocyte function in rats. Res. Nurs. Health 15, 131–137. doi: 10.1002/nur.4770150207
Meng, B., Zhu, S., Li, S., Zeng, Q., and Mei, B. (2009). Global view of the mechanisms of improved learning and memory capability in mice with music-exposure by microarray. Brain Res. Bull. 80, 36–44. doi: 10.1016/j.brainresbull.2009.05.020
Menon, V., and Levitin, D. J. (2005). The rewards of music listening: response and physiological connectivity of the mesolimbic system. Neuroimage 28, 175–184. doi: 10.1016/j.neuroimage.2005.05.053
Mizuno, M., Yamada, K., Olariu, A., Nawa, H., and Nabeshima, T. (2000). Involvement of brain-derived neurotrophic factor in spatial memory formation and maintenance in a radial arm maze test in rats. J. Neurosci. 20, 7116–7121. doi: 10.1523/JNEUROSCI.20-18-07116.2000
Moher, D., Liberati, A., Tetzlaff, J., Altman, D. G., and Grp, P. (2009). Preferred reporting items for systematic reviews and meta-analyses: the PRISMA statement. BMJ 339:b2535. doi: 10.1136/bmj.b2535
Morton, A. J., Hickey, M. A., and Dean, L. C. (2001). Methamphetamine toxicity in mice is potentiated by exposure to loud music. Neuroreport 12, 3277–3281. doi: 10.1097/00001756-200110290-00026
Nakamura, T., Tanida, M., Niijima, A., Hibino, H., Shen, J., and Nagai, K. (2007). Auditory stimulation affects renal sympathetic nerve activity and blood pressure in rats. Neurosci. Lett. 416, 107–112. doi: 10.1016/j.neulet.2007.01.080
Nakamura, T., Tanida, M., Niijima, A., and Nagai, K. (2009). Effect of auditory stimulation on parasympathetic nerve activity in urethane-anesthetized rats. In vivo 23, 415–420.
Newman, J., Rosenbach, J. H., Burns, K. L., Latimer, B. C., Matocha, H. R., and Vogt, E. R. (1995). An experimental test of “the mozart effect”: does listening to his music improve spatial ability? Percept. Mot. Skills 81(3 Pt 2):1379–1387. doi: 10.2466/pms.1995.81.3f.1379
Nunez, M. J., Mana, P., Linares, D., Riveiro, M. P., Balboa, J., Suarez-Quintanilla, J., et al. (2002). Music, immunity and cancer. Life Sci. 71, 1047–1057. doi: 10.1016/S0024-3205(02)01796-4
Ozawa, S., Kamiya, H., and Tsuzuki, K. (1998). Glutamate receptors in the mammalian central nervous system. Prog. Neurobiol. 54, 581–618. doi: 10.1016/S0301-0082(97)00085-3
Rauscher, F. H., Robinson, K. D., and Jens, J. J. (1998). Improved maze learning through early music exposure in rats. Neurol. Res. 20, 427–432. doi: 10.1080/01616412.1998.11740543
Rideout, B. E., and Laubach, C. M. (1996). EEG correlates of enhanced spatial performance following exposure to music. Percept. Mot. Skills 82, 427–432. doi: 10.2466/pms.1996.82.2.427
Rosen, S., and Howell, P. (2013). Signals and Systems for Speech and Hearing, 2nd Edn. Boston, MA: Brill.
Sheikhi, S., and Saboory, E. (2015). Neuroplasticity changes of rat brain by musical stimuli during fetal period. Cell J. 16, 448–455. doi: 10.22074/cellj.2015.490
Steele, K. M., dalla Bella, S., Peretz, I., Dunlop, T., Dawe, L. A., Humphrey, G. K., et al. (1999). Prelude or requiem for the 'Mozart effect'? Nature 400, 827–828. doi: 10.1038/23611
Stefano, G. B., Zhu, W., Cadet, P., Salamon, E., and Mantione, K. J. (2004). Music alters constitutively expressed opiate and cytokine processes in listeners. Med. Sci. Monit. 10, Ms18–Ms27.
Sutoo, D., and Akiyama, K. (1997). Regulation of blood pressure with calcium-dependent dopamine synthesizing system in the brain and its related phenomena. Brain Res. Brain Res. Rev. 25, 1–26. doi: 10.1016/S0165-0173(97)00018-0
Sutoo, D., and Akiyama, K. (2004). Music improves dopaminergic neurotransmission: demonstration based on the effect of music on blood pressure regulation. Brain Res. 1016, 255–262. doi: 10.1016/j.brainres.2004.05.018
Tasset, I., Quero, I., Garcia-Mayorgaz, A. D., Causse Del Rio, M., Tunez, I., and Montilla, P. (2012). Changes caused by haloperidol are blocked by music in Wistar rat. J. Physiol. Biochem. 68, 175–179. doi: 10.1007/s13105-011-0129-8
Thoma, M. V., La Marca, R., Bronnimann, R., Finkel, L., Ehlert, U., and Nater, U. M. (2013). The effect of music on the human stress response. PLoS ONE 8:e70156. doi: 10.1371/journal.pone.0070156
Uchiyama, M., Jin, X., Zhang, Q., Hirai, T., Amano, A., Bashuda, H., et al. (2012). Auditory stimulation of opera music induced prolongation of murine cardiac allograft survival and maintained generation of regulatory CD4+CD25+ cells. J. Cardiothorac. Surg. 7:26. doi: 10.1186/1749-8090-7-26
Vetter, D., Barth, J., Uyulmaz, S., Uyulmaz, S., Vonlanthen, R., Belli, G., et al. (2015). Effects of art on surgical patients: a systematic review and meta-analysis. Ann. Surg. 262, 704–713. doi: 10.1097/SLA.0000000000001480
Xing, Y., Chen, W., Wang, Y., Jing, W., Gao, S., Guo, D., et al. (2016a). Music exposure improves spatial cognition by enhancing the BDNF level of dorsal hippocampal subregions in the developing rats. Brain Res. Bull. 121, 131–137. doi: 10.1016/j.brainresbull.2016.01.009
Xing, Y., Qin, Y., Jing, W., Zhang, Y., Wang, Y., Guo, D., et al. (2016b). Exposure to Mozart music reduces cognitive impairment in pilocarpine-induced status epilepticus rats. Cogn. Neurodyn. 10, 23–30. doi: 10.1007/s11571-015-9361-1
Xing, Y., Xia, Y., Kendrick, K., Liu, X., Wang, M., Wu, D., et al. (2016c). Mozart, mozart rhythm and retrograde mozart effects: evidences from behaviours and neurobiology bases. Sci. Rep. 6:18744. doi: 10.1038/srep18744
Xu, F., Cai, R., Xu, J., Zhang, J., and Sun, X. (2007). Early music exposure modifies GluR2 protein expression in rat auditory cortex and anterior cingulate cortex. Neurosci. Lett. 420, 179–183. doi: 10.1016/j.neulet.2007.05.005
Xu, J., Yu, L., Cai, R., Zhang, J., and Sun, X. (2009). Early auditory enrichment with music enhances auditory discrimination learning and alters NR2B protein expression in rat auditory cortex. Behav. Brain Res. 196, 49–54. doi: 10.1016/j.bbr.2008.07.018
Yazdani, F., Naghshvarian, M., Salehi, A., and Marzban, M. (2016). Effects of dexamphetamine and music on reversal learning. Iran J. Psychiatr. Behav. Sci. 10:e3483. doi: 10.17795/ijpbs-3483
Yeh, S. H., Lin, L. W., Chuang, Y. K., Liu, C. L., Tsai, L. J., Tsuei, F. S., et al. (2015). Effects of music aerobic exercise on depression and brain-derived neurotrophic factor levels in community dwelling women. Biomed Res. Int. 2015:135893. doi: 10.1155/2015/135893
Keywords: music, acoustic stimulation, animal models, neurogenesis, anxiety, brain, systematic review
Citation: Kühlmann AYR, de Rooij A, Hunink MGM, De Zeeuw CI and Jeekel J (2018) Music Affects Rodents: A Systematic Review of Experimental Research. Front. Behav. Neurosci. 12:301. doi: 10.3389/fnbeh.2018.00301
Received: 21 November 2017; Accepted: 20 November 2018;
Published: 14 December 2018.
Edited by:
Nuno Sousa, Instituto de Pesquisa em Ciências da Vida e da Saúde (ICVS), PortugalReviewed by:
Maria Herrojo Ruiz, Goldsmiths, University of London, United KingdomAllan V. Kalueff, Institute of Translational Biomedicine, Saint Petersburg State University, Russia
Copyright © 2018 Kühlmann, de Rooij, Hunink, De Zeeuw and Jeekel. This is an open-access article distributed under the terms of the Creative Commons Attribution License (CC BY). The use, distribution or reproduction in other forums is permitted, provided the original author(s) and the copyright owner(s) are credited and that the original publication in this journal is cited, in accordance with accepted academic practice. No use, distribution or reproduction is permitted which does not comply with these terms.
*Correspondence: A. Y. Rosalie Kühlmann, YS5rdWhsbWFubkBlcmFzbXVzbWMubmw=; cm9zYWxpZWt1aGxtYW5uQGdtYWlsLmNvbQ==
†These authors have contributed equally to this work