- 1Department of Neurosurgery, Mayo Clinic, Rochester, MN, USA
- 2Department of Physiology and Biomedical Engineering, Mayo Clinic, Rochester, MN, USA
- 3Department of Radiology, Mayo Clinic, Rochester, MN, USA
- 4Department of Neurosurgery, JFK New Jersey Neuroscience Institute, Edison, NJ, USA
Tourette’s syndrome (TS) is a neurologic condition characterized by both motor and phonic tics and is typically associated with psychiatric comorbidities, including obsessive-compulsive disorder/behavior and attention-deficit hyperactivity disorder, and can be psychologically and socially debilitating. It is considered a disorder of the cortico–striato–thalamo–cortical circuitry, as suggested by pathophysiology studies and therapeutic options. Among these, deep brain stimulation (DBS) of the centromedian–parafascicular nucleus (CM-Pf) of the thalamus is emerging as a valuable treatment modality for patients affected by severe, treatment-resistant TS. Here, we review the most recent experimental evidence for the pivotal role of CM-Pf in the pathophysiology of TS, discuss potential mechanisms of action that may mediate the effects of CM-Pf DBS in TS, and summarize its clinical efficacy.
Introduction
Tourette’s syndrome (TS) is a neuropsychiatric disorder characterized by motor and phonic tics with onset during childhood; obsessive-compulsive disease and attention-deficit hyperactivity disorder (ADHD) are comorbidities present in a large subset of patients (1). Deep brain stimulation (DBS) of the centromedian–parafascicular nucleus (CM-Pf) is a therapeutic option for severe, medication-refractory TS (2). Although TS is generally considered a disorder of the basal ganglia (BG), with tics taken as evidence of failure to inhibit motor execution, its pathophysiology extends beyond a dysfunction in the BG circuits involving cortical structures in the motor, limbic, and associative networks (3). It is thought that sensorimotor and limbic circuits are affected in TS, prefrontal and limbic circuits in obsessive-compulsive disorder/behavior (OCD/OCB), and sensorimotor, orbitofrontal, and limbic circuits in ADHD (4). The CM-Pf complex, located among the caudal intralaminar nuclei of the thalamus, plays a pivotal role in these networks, providing the principal source of thalamostriatal efferents.
Despite promising preliminary results, CM-Pf DBS for TS is still not approved by the United States Food and Drug Administration and is thus an off-label treatment. A better understanding of the central role of CM-Pf in TS and its treatment can promote further investigation of this therapeutic option and, if efficacy is observed on a larger scale, improve patients’ accessibility to CM-Pf DBS. The rationale for the use of this structure as a target for DBS derives from its importance as the source of thalamostriatal efferents and its major role in functions altered in TS, including attention processing, sensorimotor gating, and motor response (5–11).
Tourette’s syndrome has long been associated with BG dysfunction. The cortico–striato–thalamo–cortical (CSTC) circuits traditionally associated with motor control include two BG pathways, one with facilitatory effects (direct pathway) and one with inhibitory effects (indirect pathway) upon movement. The dopaminergic output from the substantia nigra pars compacta to the striatum upregulates the direct pathway and downregulates the indirect pathway (12). TS has been associated with increased striatal dopaminergic release (13–17), and increased striatal dopamine has been proposed as leading to disinhibition of thalamic output to the cortex, resulting in tics (1). Positron-emission tomography (PET) findings suggest that thalamic DBS modulates dopaminergic circuitries, indicating a likely role for dopamine in therapeutic mechanisms as well (18). Abnormal striatal activity would also lead to the inefficient impulse control associated with OCD/OCB and ADHD, the common comorbidities of TS. In this conception, TS and comorbidities are considered diseases deriving from a failure in the mechanisms responsible for sensory and motor gating (19). In support of the role of BG dysfunction in TS, a recent diffusion-weighted imaging study confirmed that delayed or altered development in the CSTC networks is a possible factor leading to TS manifestations and its normalization is associated with remission of tics (20).
Considering the potential viability of CM-Pf DBS for patients with treatment-resistant TS, a review of the central role of CM-Pf in the complex pathophysiology of TS and its comorbidities is warranted.
The Role of the CM-Pf in Tourette’s Syndrome
CM-Pf Connectivity and Function
The hypothesis that the CM-Pf plays a major role in TS is supported by its anatomic and functional connections, which extend beyond motor control to limbic and associative functions. As Figure 1 shows, CM-Pf is densely connected to the BG. The main inputs to the CM-Pf derive from the globus pallidus internus (GPi), and the Pf receives input as well from the substantia nigra pars reticulata. The striatum receives most of the CM-Pf efferents. Although Eckert et al. (10) recently confirmed the extended connectivity between CM-Pf and subcortical structures in a tractography study of normal human subjects, CM-Pf connections have been mainly investigated in studies in rodents and primates, which found that CM (or its rodent equivalent, the lateral Pf nucleus) and Pf have complementary functions.
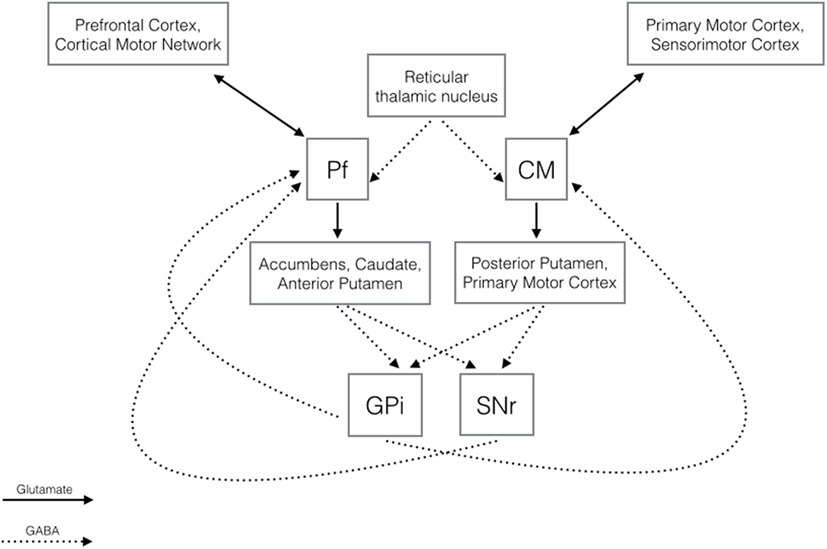
Figure 1. Diagram illustrating the main GABAergic and glutamatergic connections of the centromedian and parafascicular nuclei (21–25).
With its output to the motor cortex and to sensorimotor putamen and afferents from the motor, premotor, and somatosensory cortex, the CM nucleus appears to be involved mainly in motor control. With efferents to the limbic (nucleus accumbens) and associative (caudate, anterior putamen) striatum, anterior cingulate, and premotor and prefrontal cortices, hypothalamus, and amygdala, and input from the prefrontal cortex, supplementary motor area, and frontal eye fields, the Pf plays a role in the limbic and associative network functions. The CM-Pf additionally projects to the subthalamic nucleus, the globus pallidus, the substantia nigra, and the pedunculopontine nucleus and receives afferents from the cerebellum, pedunculopontine nucleus, superior colliculus, brainstem reticular formation, and reticular thalamic nuclei (3, 6, 21–24).
The role of CM-Pf in many functions abnormal in patients with TS, the effects elicited on striatal and cortical activity by manipulations of CM-Pf function, the altered CM-Pf neural activity found in patients with TS, and the intimate connections of the CM-Pf complex with the striatum, a structure pivotal to TS pathophysiology, favor the hypothesis that the CM-Pf plays a central role in TS and its treatment.
The complexity of CM-Pf circuitry reflects the complexity of its functions. As mentioned above, patients with TS often present with a deficiency in sensorimotor gating, as evidenced by the reduction in prepulse inhibition (PPI) of the startle reflex. In rats with decreased PPI, DBS of the medial and lateral Pf normalizes this deficiency (11). Attention deficits are also common in TS (1), and consistent with input from the brainstem reticular formation and reticular thalamic nuclei, the CM-Pf plays a fundamental role in attention arousal (25, 26). The importance of the CM-Pf complex in reward and attention behavior is supported by electrophysiology studies in non-human primates (21, 27, 28), which have confirmed its role in processing sensory stimuli, especially stimuli that are unexpected and unprecedented and thus of high attentional value.
CM-Pf DBS and Tourette’s
The effects of CM-Pf DBS have been investigated by functional MRI (fMRI) in a large animal model (swine) of CM-Pf DBS (3). The results showed that areas of activation as measured by blood-oxygen-level dependent (BOLD) response varied based on CM versus Pf targeting. BOLD-signal changes in the sensorimotor network (primary motor, premotor, somatosensory cortex) and prefrontal cortex were predominant during CM stimulation, while Pf stimulation induced BOLD-signal changes in limbic regions (including the hippocampus, parahippocampal gyrus, and cingulate cortex). These findings are of relevance given the correlation between tic complexity and altered functional connectivity in the sensorimotor (29) and associative CSTC circuits and between OCD severity and altered functional connectivity in the limbic and associative CSTC circuits (30, 31). Although clinical investigations of the potential differential effects of CM and Pf DBS are needed before conclusions can be drawn, this study suggests that selective modulation of motor, associative, or limbic circuits by DBS of the CM or the Pf may enable more individualized treatment to predominantly target tics versus psychiatric comorbidities in the future. Many of the cortical regions modulated by CM-Pf DBS are structurally and functionally altered in TS (3). The effects of DBS on the activity of supplementary motor, premotor, and prefrontal cortex on the one hand and of the sensory network on the other are of special relevance due to the suggested role of these regions in tic generation and inhibition (32–35), and in PPI and sensorimotor gating, respectively (36).
Morphologic and Functional Findings in Tourette’s Syndrome
Widespread anatomic and functional neuroimaging abnormalities in the motor, limbic, and associative networks are a feature of TS (37). Sowell and colleagues (38) and Draper and colleagues (39) observed a cortical thinning in the premotor, motor, somatosensory, insular, and anterior cingulate cortices in TS, the severity of which correlated with severity of tics and premonitory urges. This morphological alteration was more prominent in the ventral portions of the sensory and motor homunculi, and the cortical thinning in this area positively correlated with tic severity in the oro-facial district (38). Similarly, cerebellar gray matter volume reductions correlated with tic severity in a recent MRI study performed in patients with TS and healthy controls (40). H2O PET found increased brain activity in the prefrontal, primary motor, premotor, supplementary motor, insula, cingulate, cerebellum, thalamus, and striatum correlating with tics (41, 42). Also, fMRI confirmed the increase in activity before tic onset in the limbic (anterior cingulate cortex, insula) and sensorimotor (supplementary motor, premotor, sensory associative cortex, cerebellum) networks, and at tic onset mainly in the sensorimotor network (primary motor, supplementary motor, premotor, somatosensory, sensory association cortex, cerebellum) (43). These findings are in favor of an altered function in the sensorimotor, limbic, and associative networks, with a central role of CM-Pf in modulating the brain areas involved in TS.
Motor network dysfunction has been extensively investigated in TS. PET and transcranial magnetic stimulation studies of patients with TS have found increased resting-state activation, action-related activation, and hyperexcitability in the motor network (44–47). A compromised GABAergic function in the motor system appears to contribute to hyperexcitability in the supplementary motor cortex, as suggested by an altered correlation between GABA levels and beta oscillations in this region (29). The importance of motor network dysfunction in TS is also underscored by the finding that decreased connectivity between the supplementary motor cortex and the striatum correlates with Yale Global Tic Severity Scale (YGTSS) scores (48).
Electrophysiology studies of the CM-Pf target in patients undergoing DBS surgery for TS have found bursting activity in CM-Pf prior to tic production and reduction in the alpha activity and increase in the gamma activity correlated with tic reduction during DBS (49–52). This suggests that CM-Pf DBS may reduce tics by normalizing the local neuronal discharge and affecting striatal output. In fact, in vivo stimulation of non-human primate CM nucleus results in GABA-mediated decreased cholinergic activity in the striatum (53), and Pf manipulations (electrical, lesional, or pharmacological) modulate striatal dopaminergic transmission (23).
CM-Pf glutamatergic output exerts a powerful influence over striatal activity, targeting medium-sized spiny neurons and cholinergic interneurons (21). According to one theory of TS pathophysiology, motor pattern generators in the cerebral cortex and brainstem that are associated with a specific movement may be involuntarily activated in TS due to altered regulation of striatal output, leading to tics (54). Given that the striatum is the major target for CM-Pf projections, alterations in this BG structure emphasize the role of CM-Pf in TS.
The major BG morphologic changes associated with TS include reduced volume of both caudate and lenticular nuclei (55–57), and functional imaging of the striatum in TS has shown reduced activity at rest compared to controls (44, 45) and increased activity during tic production (41, 42). Reduced caudate volumes during childhood have been correlated with symptom severity in late adolescence, and larger caudate volumes were found in patients taking neuroleptics for TS compared to the caudate volumes of patients not exposed to these drugs and those of healthy subjects. These findings suggest that a reduced caudate volume may be involved in TS pathophysiology and may be partially due to increased dopaminergic signaling (56, 58).
The reduction in caudate volumes in TS could be mediated by loss, reduced development, or hypofunctioning of GABAergic and tonically active cholinergic interneurons, as suggested by (1) the reduced global striatal expression of genes involved in the steps of interneuron neurotransmission (59); (2) flumazenil-PET findings of reduced GABAergic activity in the striatum (60); and (3) post-mortem findings of reduced GABAergic interneurons in the associative and sensorimotor regions of the striatum (61, 62). In addition, selective inhibition of striatal interneuron activity was associated with dyskinesia and behavioral manifestations of TS in mice (63, 64), indicating that impairment in inhibitory striatal interneuron activity can result in tics.
These observations are at the basis of the hypothesis that the CM-Pf complex is a central node in the modulation of areas and circuitries associated with TS pathophysiology. Despite the elements in support of this theory, DBS remains an invasive treatment and its clinical distribution needs compelling clinical findings before becoming widely accepted and approved as standard of care in selected patients. A summary of available clinical reports of CM-Pf DBS is provided to address the initial translation of the described pathophysiological findings into practice (Table 1).
Clinical Outcomes of CM-Pf DBS for TS
Overall, the outcomes reported for DBS of the CM-Pf for TS have been positive for tic reduction and mixed for psychiatric comorbidities.
Tic Reduction
The first report of DBS for TS was by Vandewalle et al. (65) who targeted the junction between the CM-Pf complex, the ventrooral internus nucleus (Voi), and the substantia periventricularis (Spv), located 5 mm lateral and 4 mm posterior to the midpoint of the anterior–posterior commissure (AC–PC) line and on the AC–PC plane in a single patient. Long-term results for three patients were later reported by the same group and found that tic reduction, assessed by video recordings, was 92.6% at 10 years (subject 1), 72.2% at 1 year (subject 2), and 78% at 6 years (subject 3) (66, 67). Subsequent series have targeted coordinates at either the anterior border or at the center of the CM-Pf, as discussed below.
The largest series to date (34 patients) of intralaminar thalamus DBS for TS (target located at the CM-Pf/Vo junction, 5 mm lateral and 2 mm posterior to the midpoint of the AC–PC line and on the AC–PC plane) reported statistically significant tic reduction across the group. The average YGTSS scores of the 19 patients who reached the 2-year follow-up significantly decreased from a preoperative average of 76.9 (out of 100) to 36.7, indicating reduction in tics and in disease-related impairment (69). In 17 of these patients, the scores fell from an average of 81.1 preoperatively to 22.5 at the 5- to 6-year follow-up (84). An expansion of this series was recently published, including additional targets (85). As Table 1 shows, results from double-blinded randomized controlled trials and single-case studies or small surgical series tend to support the effectiveness of intralaminar thalamus DBS for TS (70–72, 75, 77–79, 81–83, 86).
Adverse Events
Adverse events reported in the literature are also summarized in Table 1. Reports from several groups (67, 71, 86–88) have highlighted the potential for increased incidence of complications in DBS for TS, including infections, system breakages, lead traction, and skin dehiscence, possibly associated with self-injurious behaviors, forceful head tics, obsessions, and compulsions, such as scratching associated with the implanted device or the surgical wound and scar.
Of particular note relative to the effects of DBS in patients with severe comorbidities is a patient who participated in the double-blind randomized control study conducted by Ackermans et al. At 23 years of age, she had a history of tics associated with severe self-injurious behavior, pervasive developmental disorder, depression, and compulsions. Following DBS, she developed an array of symptoms and signs (multiple limbs hypertonia, involuntary movements, opisthotonus, impaired consciousness, mutism, impairment of swallowing, nausea, anorexia) suggestive of a psychogenic movement disorder and died in a nursing home (72, 82). Blinded DBS had increased tics and reduced hypertonia. This case highlights the importance of careful evaluation to rule out possible somatoform disorders and treatment of comorbidities prior to DBS surgery. This is of particular relevance given the potential effects of Pf DBS on the limbic and associative networks. The authors did consider alteration of these circuits as a possible cause of postsurgical complications, warning against DBS in case of severe psychiatric comorbidities.
Psychiatric Comorbidities and Cognition
There is no consensus at this time on whether DBS for TS has a positive, neutral, or detrimental effect on TS-related psychiatric comorbidities and cognition. Results have varied across the literature (67, 68, 70, 71, 76, 78–84, 86, 89, 90).
Considering the complexity of TS and comorbidities, including OCD/OCB, ADHD, depression, and self-injurious behaviors, it is not surprising that in some patients some of these symptoms improve and others deteriorate (67, 82). However, it should be noted that compared to pallidal stimulation, CM-Pf DBS seems to have a more positive impact on depressed mood, emotional hypersensitivity, anxiety, and impulsivity (83, 86). Stimulation of the ventral electrode of CM-Pf leads, which can be considered Pf stimulation, has been associated with feelings of calmness, suggesting a modulation of limbic circuitry (81). This is consistent with the known connectivity of Pf and suggests the need for further investigation of the differential effects of CM and Pf stimulation found in the experimental literature (3).
In the future, large studies may help to elucidate whether the limbic circuitry modulation observed during Pf stimulation exerts positive or detrimental effects on OCD/OCB, ADHD, and depressive symptoms so as to better serve patients whose quality of life is significantly decreased by comorbidities.
Additional Targets
Other regions have been investigated as possible DBS targets for TS, including the GPi (83, 86, 91–94), the ventroanterior/ventrolateral thalamus (95), the globus pallidus externus (96), the nucleus accumbens and anterior limb of internal capsule (97, 98), and the subthalamic nucleus (99).
The GPi is the most commonly used target after CM-Pf. Results from a recent double-blind randomized crossover trial including 13 patients showed significant tic reduction during GPi stimulation (average 15.3% improvement in YGTSS score during the stimulation-on phase compared to stimulation-off and to baseline phases) (94). Additionally, in a double-blind randomized controlled trial comparing CM-Pf and GPi DBS, stimulation of either target was found to be effective for tic suppression in three patients, with better results obtained with GPi than with CM-Pf DBS (78.3 versus 44.7% reduction in YGTSS scores) (83, 86). Open-label studies including more than ten patients report percent tic score reductions ranging between 44.8 and 52.3, with 59.6% of patients experiencing a YGTSS reduction of 50% or higher (91–93). Further studies comparing CM-Pf and GPi stimulation are warranted to establish a definitive target of choice in the treatment of TS.
Future Perspectives
As summarized in Table 1, the reports available in the literature display a large range of efficacy outcomes for CM-Pf DBS and of patient population sizes. This is partially related to the small patient group necessitating and being evaluated for DBS treatment and warrants additional investigation to support CM-Pf DBS as a standard therapeutic option in selected patients affected by TS. If multi-center double-blind randomized controlled trials may help achieve larger subject numbers, these are often difficult to implement. Based on current literature characteristics and results, DBS treatment for TS may advance mainly through multiple single-institution, double-blind, randomized controlled studies widely distributed nationally and internationally, which will provide the necessary evidence in support or against the efficacy of this treatment. DBS treatment and patient care will be then performed according to institutional capabilities and therefore not only more feasible but also more closely representative of the following clinical practice outside of randomized controlled studies.
Conclusion
CM-Pf DBS is a therapeutic option in carefully selected patients affected by severe, treatment refractory TS. CSTC circuitry dysfunction is strongly implicated in the pathophysiology of TS, and the centrality of the CM-Pf to this mechanism makes it a promising target. Larger-scale clinical studies are warranted to confirm the initial promising findings of DBS-related tic suppression. Additionally, the CM appears to play a role in motor functions and the Pf in limbic and associative functions that are associated with TS, and continued investigation of the differential effects of targeting CM for motor symptoms and Pf for psychiatric comorbidities will help determine if more individualized DBS therapy for TS is possible and viable in the future. Large studies investigating the differential long-term outcomes of CM and Pf stimulation for tic control, comorbidities, and cognitive functioning and comparing the available DBS targets for TS, might help DBS for TS transition from an experimental to a more readily available therapeutic option, thus improving the lives of patients with severe, intractable TS and comorbidities.
Author Contributions
PT: conception and design of the work; interpretation of literature; drafting of the work; final approval of the version to be published; and agreement to be accountable for all aspects of the work. H-KM, AB, and KL: design of the work; critical revision; final approval of the version to be published; and agreement to be accountable for all aspects of the work.
Conflict of Interest Statement
The authors declare that the research was conducted in the absence of any commercial or financial relationships that could be construed as a potential conflict of interest.
Funding
This work is supported in part by The Grainger Foundation.
References
1. Leckman JF. Tourette’s syndrome. Lancet (2002) 360(9345):1577–86. doi:10.1016/s0140-6736(02)11526-1
2. Schrock LE, Mink JW, Woods DW, Porta M, Servello D, Visser-Vandewalle V, et al. Tourette syndrome deep brain stimulation: a review and updated recommendations. Mov Disord (2014) 30(4):448–71. doi:10.1002/mds.26094
3. Kim JP, Min HK, Knight EJ, Duffy PS, Abulseoud OA, Marsh MP, et al. Centromedian-parafascicular deep brain stimulation induces differential functional inhibition of the motor, associative, and limbic circuits in large animals. Biol Psychiatry (2013) 74(12):917–26. doi:10.1016/j.biopsych.2013.06.024
4. Sheppard DM, Bradshaw JL, Purcell R, Pantelis C. Tourette’s and comorbid syndromes: obsessive compulsive and attention deficit hyperactivity disorder. A common etiology? Clin Psychol Rev (1999) 19(5):531–52. doi:10.1016/S0272-7358(98)00059-2
5. Kinomura S, Larsson J, Gulyas B, Roland PE. Activation by attention of the human reticular formation and thalamic intralaminar nuclei. Science (1996) 271(5248):512–5. doi:10.1126/science.271.5248.512
6. Van der Werf YD, Witter MP, Groenewegen HJ. The intralaminar and midline nuclei of the thalamus. Anatomical and functional evidence for participation in processes of arousal and awareness. Brain Res Brain Res Rev (2002) 39(2–3):107–40. doi:10.1016/S0165-0173(02)00181-9
7. Kimura M, Minamimoto T, Matsumoto N, Hori Y. Monitoring and switching of cortico-basal ganglia loop functions by the thalamo-striatal system. Neurosci Res (2004) 48(4):355–60. doi:10.1016/j.neures.2003.12.002
8. Cukiert A, Burattini JA, Cukiert CM, Argentoni-Baldochi M, Baise-Zung C, Forster CR, et al. Centro-median stimulation yields additional seizure frequency and attention improvement in patients previously submitted to callosotomy. Seizure (2009) 18(8):588–92. doi:10.1016/j.seizure.2009.06.002
9. Metzger CD, Eckert U, Steiner J, Sartorius A, Buchmann JE, Stadler J, et al. High field fMRI reveals thalamocortical integration of segregated cognitive and emotional processing in mediodorsal and intralaminar thalamic nuclei. Front Neuroanat (2010) 4:138. doi:10.3389/fnana.2010.00138
10. Eckert U, Metzger CD, Buchmann JE, Kaufmann J, Osoba A, Li M, et al. Preferential networks of the mediodorsal nucleus and centromedian-parafascicular complex of the thalamus – a DTI tractography study. Hum Brain Mapp (2012) 33(11):2627–37. doi:10.1002/hbm.21389
11. Angelov SD, Dietrich C, Krauss JK, Schwabe K. Effect of deep brain stimulation in rats selectively bred for reduced prepulse inhibition. Brain Stimul (2014) 7(4):595–602. doi:10.1016/j.brs.2014.03.013
12. Israelashvili M, Loewenstern Y, Bar-Gad I. Abnormal neuronal activity in Tourette syndrome and its modulation using deep brain stimulation. J Neurophysiol (2015) 114(1):6–20. doi:10.1152/jn.00277.2015
13. Singer HS, Szymanski S, Giuliano J, Yokoi F, Dogan AS, Brasic JR, et al. Elevated intrasynaptic dopamine release in Tourette’s syndrome measured by PET. Am J Psychiatry (2002) 159(8):1329–36. doi:10.1176/appi.ajp.159.8.1329
14. Serra-Mestres J, Ring HA, Costa DC, Gacinovic S, Walker Z, Lees AJ, et al. Dopamine transporter binding in Gilles de la Tourette syndrome: a [123I]FP-CIT/SPECT study. Acta Psychiatr Scand (2004) 109(2):140–6. doi:10.1111/j.0001-690X.2004.00214.x
15. Cheon KA, Ryu YH, Namkoong K, Kim CH, Kim JJ, Lee JD. Dopamine transporter density of the basal ganglia assessed with [123I]IPT SPECT in drug-naive children with Tourette’s disorder. Psychiatry Res (2004) 130(1):85–95. doi:10.1016/j.pscychresns.2003.06.001
16. Albin RL, Koeppe RA, Bohnen NI, Nichols TE, Meyer P, Wernette K, et al. Increased ventral striatal monoaminergic innervation in Tourette syndrome. Neurology (2003) 61(3):310–5. doi:10.1212/01.WNL.0000076181.39162.FC
17. Wong DF, Brasic JR, Singer HS, Schretlen DJ, Kuwabara H, Zhou Y, et al. Mechanisms of dopaminergic and serotonergic neurotransmission in Tourette syndrome: clues from an in vivo neurochemistry study with PET. Neuropsychopharmacology (2008) 33(6):1239–51. doi:10.1038/sj.npp.1301528
18. Kuhn J, Janouschek H, Raptis M, Rex S, Lenartz D, Neuner I, et al. In vivo evidence of deep brain stimulation-induced dopaminergic modulation in Tourette’s syndrome. Biol Psychiatry (2012) 71(5):e11–3. doi:10.1016/j.biopsych.2011.09.035
19. Leckman JF, Bloch MH, Scahill L, King RA. Tourette syndrome: the self under siege. J Child Neurol (2006) 21(8):642–9. doi:10.1177/08830738060210081001
20. Debes N, Jeppesen S, Raghava JM, Groth C, Rostrup E, Skov L. Longitudinal magnetic resonance imaging (MRI) analysis of the developmental changes of Tourette syndrome reveal reduced diffusion in the cortico-striato-thalamo-cortical pathways. J Child Neurol (2015) 30(10):1315–26. doi:10.1177/0883073814560629
21. Minamimoto T, Kimura M. Participation of the thalamic CM-Pf complex in attentional orienting. J Neurophysiol (2002) 87(6):3090–101. doi:10.1152/jn.00564.2001
22. Haber SN, Calzavara R. The cortico-basal ganglia integrative network: the role of the thalamus. Brain Res Bull (2009) 78(2–3):69–74. doi:10.1016/j.brainresbull.2008.09.013
23. Smith Y, Raju D, Nanda B, Pare JF, Galvan A, Wichmann T. The thalamostriatal systems: anatomical and functional organization in normal and parkinsonian states. Brain Res Bull (2009) 78(2–3):60–8. doi:10.1016/j.brainresbull.2008.08.015
24. Sadikot AF, Rymar VV. The primate centromedian-parafascicular complex: anatomical organization with a note on neuromodulation. Brain Res Bull (2009) 78(2–3):122–30. doi:10.1016/j.brainresbull.2008.09.016
25. Ferrarelli F, Tononi G. The thalamic reticular nucleus and schizophrenia. Schizophr Bull (2011) 37(2):306–15. doi:10.1093/schbul/sbq142
26. Haber S, McFarland NR. The place of the thalamus in frontal cortical-basal ganglia circuits. Neuroscientist (2001) 7(4):315–24. doi:10.1177/107385840100700408
27. Matsumoto N, Minamimoto T, Graybiel AM, Kimura M. Neurons in the thalamic CM-Pf complex supply striatal neurons with information about behaviorally significant sensory events. J Neurophysiol (2001) 85(2):960–76.
28. Minamimoto T, Hori Y, Kimura M. Complementary process to response bias in the centromedian nucleus of the thalamus. Science (2005) 308(5729):1798–801. doi:10.1126/science.1109154
29. Tinaz S, Belluscio BA, Malone P, van der Veen JW, Hallett M, Horovitz SG. Role of the sensorimotor cortex in Tourette syndrome using multimodal imaging. Hum Brain Mapp (2014) 35(12):5834–46. doi:10.1002/hbm.22588
30. Worbe Y, Malherbe C, Hartmann A, Pelegrini-Issac M, Messe A, Vidailhet M, et al. Functional immaturity of cortico-basal ganglia networks in Gilles de la Tourette syndrome. Brain (2012) 135(Pt 6):1937–46. doi:10.1093/brain/aws056
31. Worbe Y, Marrakchi-Kacem L, Lecomte S, Valabregue R, Poupon F, Guevara P, et al. Altered structural connectivity of cortico-striato-pallido-thalamic networks in Gilles de la Tourette syndrome. Brain (2014) 138(Pt 2):472–82. doi:10.1093/brain/awu311
32. Draper A, Stephenson MC, Jackson GM, Pepes S, Morgan PS, Morris PG, et al. Increased GABA contributes to enhanced control over motor excitability in Tourette syndrome. Curr Biol (2014) 24(19):2343–7. doi:10.1016/j.cub.2014.08.038
33. Thomalla G, Jonas M, Baumer T, Siebner HR, Biermann-Ruben K, Ganos C, et al. Costs of control: decreased motor cortex engagement during a Go/NoGo task in Tourette’s syndrome. Brain (2014) 137(Pt 1):122–36. doi:10.1093/brain/awt288
34. Ganos C, Kuhn S, Kahl U, Schunke O, Feldheim J, Gerloff C, et al. Action inhibition in Tourette syndrome. Mov Disord (2014) 29(12):1532–8. doi:10.1002/mds.25944
35. Jackson GM, Draper A, Dyke K, Pepes SE, Jackson SR. Inhibition, disinhibition, and the control of action in Tourette syndrome. Trends Cogn Sci (2015) 19(11):655–65. doi:10.1016/j.tics.2015.08.006
36. Buse J, Beste C, Herrmann E, Roessner V. Neural correlates of altered sensorimotor gating in boys with Tourette syndrome: a combined EMG/fMRI study. World J Biol Psychiatry (2015) 17(3):1–11. doi:10.3109/15622975.2015.1112033
37. Neuner I, Kupriyanova Y, Stocker T, Huang R, Posnansky O, Schneider F, et al. White-matter abnormalities in Tourette syndrome extend beyond motor pathways. Neuroimage (2010) 51(3):1184–93. doi:10.1016/j.neuroimage.2010.02.049
38. Sowell ER, Kan E, Yoshii J, Thompson PM, Bansal R, Xu D, et al. Thinning of sensorimotor cortices in children with Tourette syndrome. Nat Neurosci (2008) 11(6):637–9. doi:10.1038/nn.2121
39. Draper A, Jackson GM, Morgan PS, Jackson SR. Premonitory urges are associated with decreased grey matter thickness within the insula and sensorimotor cortex in young people with Tourette syndrome. J Neuropsychol (2015) 10(1):143–53. doi:10.1111/jnp.12089
40. Tobe RH, Bansal R, Xu D, Hao X, Liu J, Sanchez J, et al. Cerebellar morphology in Tourette syndrome and obsessive-compulsive disorder. Ann Neurol (2010) 67(4):479–87. doi:10.1002/ana.21918
41. Stern E, Silbersweig DA, Chee KY, Holmes A, Robertson MM, Trimble M, et al. A functional neuroanatomy of tics in Tourette syndrome. Arch Gen Psychiatry (2000) 57(8):741–8. doi:10.1001/archpsyc.57.8.741
42. Lerner A, Bagic A, Boudreau EA, Hanakawa T, Pagan F, Mari Z, et al. Neuroimaging of neuronal circuits involved in tic generation in patients with Tourette syndrome. Neurology (2007) 68(23):1979–87. doi:10.1212/01.wnl.0000264417.18604.12
43. Bohlhalter S, Goldfine A, Matteson S, Garraux G, Hanakawa T, Kansaku K, et al. Neural correlates of tic generation in Tourette syndrome: an event-related functional MRI study. Brain (2006) 129(Pt 8):2029–37. doi:10.1093/brain/awl050
44. Eidelberg D, Moeller JR, Antonini A, Kazumata K, Dhawan V, Budman C, et al. The metabolic anatomy of Tourette’s syndrome. Neurology (1997) 48(4):927–34. doi:10.1212/WNL.48.4.927
45. Pourfar M, Feigin A, Tang CC, Carbon-Correll M, Bussa M, Budman C, et al. Abnormal metabolic brain networks in Tourette syndrome. Neurology (2011) 76(11):944–52. doi:10.1212/WNL.0b013e3182104106
46. Ziemann U, Paulus W, Rothenberger A. Decreased motor inhibition in Tourette’s disorder: evidence from transcranial magnetic stimulation. Am J Psychiatry (1997) 154(9):1277–84. doi:10.1176/ajp.154.9.1277
47. Zapparoli L, Porta M, Paulesu E. The anarchic brain in action: the contribution of task-based fMRI studies to the understanding of Gilles de la Tourette syndrome. Curr Opin Neurol (2015) 28(6):604–11. doi:10.1097/WCO.0000000000000261
48. Cheng B, Braass H, Ganos C, Treszl A, Biermann-Ruben K, Hummel FC, et al. Altered intrahemispheric structural connectivity in Gilles de la Tourette syndrome. Neuroimage Clin (2014) 4:174–81. doi:10.1016/j.nicl.2013.11.011
49. Marceglia S, Servello D, Foffani G, Porta M, Sassi M, Mrakic-Sposta S, et al. Thalamic single-unit and local field potential activity in Tourette syndrome. Mov Disord (2010) 25(3):300–8. doi:10.1002/mds.22982
50. Maling N, Hashemiyoon R, Foote KD, Okun MS, Sanchez JC. Increased thalamic gamma band activity correlates with symptom relief following deep brain stimulation in humans with Tourette’s syndrome. PLoS One (2012) 7(9):e44215. doi:10.1371/journal.pone.0044215
51. Priori A, Giannicola G, Rosa M, Marceglia S, Servello D, Sassi M, et al. Deep brain electrophysiological recordings provide clues to the pathophysiology of Tourette syndrome. Neurosci Biobehav Rev (2013) 37(6):1063–8. doi:10.1016/j.neubiorev.2013.01.011
52. Bour LJ, Ackermans L, Foncke EM, Cath D, van der Linden C, Visser Vandewalle V, et al. Tic related local field potentials in the thalamus and the effect of deep brain stimulation in Tourette syndrome: report of three cases. Clin Neurophysiol (2014) 126(8):1578–88. doi:10.1016/j.clinph.2014.10.217
53. Nanda B, Galvan A, Smith Y, Wichmann T. Effects of stimulation of the centromedian nucleus of the thalamus on the activity of striatal cells in awake rhesus monkeys. Eur J Neurosci (2009) 29(3):588–98. doi:10.1111/j.1460-9568.2008.06598.x
54. Albin RL, Mink JW. Recent advances in Tourette syndrome research. Trends Neurosci (2006) 29(3):175–82. doi:10.1016/j.tins.2006.01.001
55. Peterson B, Riddle MA, Cohen DJ, Katz LD, Smith JC, Hardin MT, et al. Reduced basal ganglia volumes in Tourette’s syndrome using three-dimensional reconstruction techniques from magnetic resonance images. Neurology (1993) 43(5):941–9. doi:10.1212/WNL.43.5.941
56. Peterson BS, Thomas P, Kane MJ, Scahill L, Zhang H, Bronen R, et al. Basal Ganglia volumes in patients with Gilles de la Tourette syndrome. Arch Gen Psychiatry (2003) 60(4):415–24. doi:10.1001/archpsyc.60.4.415
57. Leckman JF, Bloch MH, Smith ME, Larabi D, Hampson M. Neurobiological substrates of Tourette’s disorder. J Child Adolesc Psychopharmacol (2010) 20(4):237–47. doi:10.1089/cap.2009.0118
58. Bloch MH, Leckman JF, Zhu H, Peterson BS. Caudate volumes in childhood predict symptom severity in adults with Tourette syndrome. Neurology (2005) 65(8):1253–8. doi:10.1212/01.wnl.0000180957.98702.69
59. Lennington JB, Coppola G, Kataoka-Sasaki Y, Fernandez TV, Palejev D, Li Y, et al. Transcriptome analysis of the human striatum in Tourette syndrome. Biol Psychiatry (2016) 79(5):372–82. doi:10.1016/j.biopsych.2014.07.018
60. Lerner A, Bagic A, Simmons JM, Mari Z, Bonne O, Xu B, et al. Widespread abnormality of the gamma-aminobutyric acid-ergic system in Tourette syndrome. Brain (2012) 135(Pt 6):1926–36. doi:10.1093/brain/aws104
61. Kalanithi PS, Zheng W, Kataoka Y, DiFiglia M, Grantz H, Saper CB, et al. Altered parvalbumin-positive neuron distribution in basal ganglia of individuals with Tourette syndrome. Proc Natl Acad Sci U S A (2005) 102(37):13307–12. doi:10.1073/pnas.0502624102
62. Kataoka Y, Kalanithi PS, Grantz H, Schwartz ML, Saper C, Leckman JF, et al. Decreased number of parvalbumin and cholinergic interneurons in the striatum of individuals with Tourette syndrome. J Comp Neurol (2010) 518(3):277–91. doi:10.1002/cne.22206
63. Gittis AH, Leventhal DK, Fensterheim BA, Pettibone JR, Berke JD, Kreitzer AC. Selective inhibition of striatal fast-spiking interneurons causes dyskinesias. J Neurosci (2011) 31(44):15727–31. doi:10.1523/JNEUROSCI.3875-11.2011
64. Xu M, Kobets A, Du JC, Lennington J, Li L, Banasr M, et al. Targeted ablation of cholinergic interneurons in the dorsolateral striatum produces behavioral manifestations of Tourette syndrome. Proc Natl Acad Sci U S A (2015) 112(3):893–8. doi:10.1073/pnas.1419533112
65. Vandewalle V, van der Linden C, Groenewegen HJ, Caemaert J. Stereotactic treatment of Gilles de la Tourette syndrome by high frequency stimulation of thalamus. Lancet (1999) 353(9154):724. doi:10.1016/s0140-6736(98)05964-9
66. Visser-Vandewalle V, Temel Y, Boon P, Vreeling F, Colle H, Hoogland G, et al. Chronic bilateral thalamic stimulation: a new therapeutic approach in intractable Tourette syndrome. Report of three cases. J Neurosurg (2003) 99(6):1094–100. doi:10.3171/jns.2003.99.6.1094
67. Ackermans L, Duits A, Temel Y, Winogrodzka A, Peeters F, Beuls EA, et al. Long-term outcome of thalamic deep brain stimulation in two patients with Tourette syndrome. J Neurol Neurosurg Psychiatry (2010) 81(10):1068–72. doi:10.1136/jnnp.2009.176859
68. Servello D, Porta M, Sassi M, Brambilla A, Robertson MM. Deep brain stimulation in 18 patients with severe Gilles de la Tourette syndrome refractory to treatment: the surgery and stimulation. J Neurol Neurosurg Psychiatry (2008) 79(2):136–42. doi:10.1136/jnnp.2006.104067
69. Servello D, Sassi M, Brambilla A, Defendi S, Porta M. Long-term, post-deep brain stimulation management of a series of 36 patients affected with refractory Gilles de la Tourette syndrome. Neuromodulation (2010) 13(3):187–94. doi:10.1111/j.1525-1403.2009.00253.x
70. Lee MW, Au-Yeung MM, Hung KN, Wong CK. Deep brain stimulation in a Chinese Tourette’s syndrome patient. Hong Kong Med J (2011) 17(2):147–50.
71. Motlagh MG, Smith ME, Landeros-Weisenberger A, Kobets AJ, King RA, Miravite J, et al. Lessons learned from open-label deep brain stimulation for Tourette syndrome: eight cases over 7 years. Tremor Other Hyperkinet Mov (N Y) (2013) 3.
72. Duits A, Ackermans L, Cath D, Visser-Vandewalle V. Unfavourable outcome of deep brain stimulation in a Tourette patient with severe comorbidity. Eur Child Adolesc Psychiatry (2012) 21(9):529–31. doi:10.1007/s00787-012-0285-6
73. Idris Z, Ghani AR, Mar W, Bhaskar S, Wan Hassan WN, Tharakan J, et al. Intracerebral haematomas after deep brain stimulation surgery in a patient with Tourette syndrome and low factor XIIIA activity. J Clin Neurosci (2010) 17(10):1343–4. doi:10.1016/j.jocn.2010.01.054
74. Savica R, Stead M, Mack KJ, Lee KH, Klassen BT. Deep brain stimulation in tourette syndrome: a description of 3 patients with excellent outcome. Mayo Clin Proc (2012) 87(1):59–62. doi:10.1016/j.mayocp.2011.08.005
75. Testini P, Zhao CZ, Stead M, Duffy PS, Klassen BT, Lee KH. Centromedian-parafascicular complex deep brain stimulation for Tourette syndrome: a retrospective study. Mayo Clin Proc (2016) 91(2):218–25. doi:10.1016/j.mayocp.2015.11.016
76. Bajwa RJ, de Lotbiniere AJ, King RA, Jabbari B, Quatrano S, Kunze K, et al. Deep brain stimulation in Tourette’s syndrome. Mov Disord (2007) 22(9):1346–50. doi:10.1002/mds.21398
77. Shields DC, Cheng ML, Flaherty AW, Gale JT, Eskandar EN. Microelectrode-guided deep brain stimulation for Tourette syndrome: within-subject comparison of different stimulation sites. Stereotact Funct Neurosurg (2008) 86(2):87–91. doi:10.1159/000112429
78. Kaido T, Otsuki T, Kaneko Y, Takahashi A, Omori M, Okamoto T. Deep brain stimulation for Tourette syndrome: a prospective pilot study in Japan. Neuromodulation (2011) 14(2):123–8; discussion 9. doi:10.1111/j.1525-1403.2010.00324.x
79. Maciunas RJ, Maddux BN, Riley DE, Whitney CM, Schoenberg MR, Ogrocki PJ, et al. Prospective randomized double-blind trial of bilateral thalamic deep brain stimulation in adults with Tourette syndrome. J Neurosurg (2007) 107(5):1004–14. doi:10.3171/JNS-07/11/1004
80. Schoenberg MR, Maddux BN, Riley DE, Whitney CM, Ogrocki PK, Gould D, et al. Five-months-postoperative neuropsychological outcome from a pilot prospective randomized clinical trial of thalamic deep brain stimulation for Tourette syndrome. Neuromodulation (2014) 18(2):97–104. doi:10.1111/ner.12233
81. Okun MS, Foote KD, Wu SS, Ward HE, Bowers D, Rodriguez RL, et al. A trial of scheduled deep brain stimulation for Tourette syndrome: moving away from continuous deep brain stimulation paradigms. JAMA Neurol (2013) 70(1):85–94. doi:10.1001/jamaneurol.2013.580
82. Ackermans L, Duits A, van der Linden C, Tijssen M, Schruers K, Temel Y, et al. Double-blind clinical trial of thalamic stimulation in patients with Tourette syndrome. Brain (2011) 134(Pt 3):832–44. doi:10.1093/brain/awq380
83. Welter ML, Mallet L, Houeto JL, Karachi C, Czernecki V, Cornu P, et al. Internal pallidal and thalamic stimulation in patients with Tourette syndrome. Arch Neurol (2008) 65(7):952–7. doi:10.1001/archneur.65.7.952
84. Porta M, Servello D, Zanaboni C, Anasetti F, Menghetti C, Sassi M, et al. Deep brain stimulation for treatment of refractory Tourette syndrome: long-term follow-up. Acta Neurochir (Wien) (2012) 154(11):2029–41. doi:10.1007/s00701-012-1497-8
85. Servello D, Zekaj E, Saleh C, Lange N, Porta M. Deep brain stimulation in Gilles de la Tourette syndrome: what does the future hold? A cohort of 48 patients. Neurosurgery (2016) 78(1):91–100. doi:10.1227/NEU.0000000000001004
86. Houeto JL, Karachi C, Mallet L, Pillon B, Yelnik J, Mesnage V, et al. Tourette’s syndrome and deep brain stimulation. J Neurol Neurosurg Psychiatry (2005) 76(7):992–5. doi:10.1136/jnnp.2004.043273
87. Servello D, Sassi M, Gaeta M, Ricci C, Porta M. Tourette syndrome (TS) bears a higher rate of inflammatory complications at the implanted hardware in deep brain stimulation (DBS). Acta Neurochir (Wien) (2011) 153(3):629–32. doi:10.1007/s00701-010-0851-y
88. Kim W, Pouratian N. Deep brain stimulation for Tourette syndrome. Neurosurg Clin N Am (2014) 25(1):117–35. doi:10.1016/j.nec.2013.08.009
89. Porta M, Brambilla A, Cavanna AE, Servello D, Sassi M, Rickards H, et al. Thalamic deep brain stimulation for treatment-refractory Tourette syndrome: two-year outcome. Neurology (2009) 73(17):1375–80. doi:10.1212/WNL.0b013e3181bd809b
90. Rzesnitzek L, Wachter T, Kruger R, Gharabaghi A, Plewnia C. Suppression of extrapyramidal side effects of doxepin by thalamic deep brain stimulation for Tourette syndrome. Neurology (2011) 77(18):1708–9. doi:10.1212/WNL.0b013e318236485f
91. Cannon E, Silburn P, Coyne T, O’Maley K, Crawford JD, Sachdev PS. Deep brain stimulation of anteromedial globus pallidus interna for severe Tourette’s syndrome. Am J Psychiatry (2012) 169(8):860–6. doi:10.1176/appi.ajp.2012.11101583
92. Sachdev PS, Mohan A, Cannon E, Crawford JD, Silberstein P, Cook R, et al. Deep brain stimulation of the antero-medial globus pallidus interna for Tourette syndrome. PLoS One (2014) 9(8):e104926. doi:10.1371/journal.pone.0104926
93. Zhang JG, Ge Y, Stead M, Zhang K, Yan SS, Hu W, et al. Long-term outcome of globus pallidus internus deep brain stimulation in patients with Tourette syndrome. Mayo Clin Proc (2014) 89(11):1506–14. doi:10.1016/j.mayocp.2014.05.019
94. Kefalopoulou Z, Zrinzo L, Jahanshahi M, Candelario J, Milabo C, Beigi M, et al. Bilateral globus pallidus stimulation for severe Tourette’s syndrome: a double-blind, randomised crossover trial. Lancet Neurol (2015) 14(6):595–605. doi:10.1016/S1474-4422(15)00008-3
95. Huys D, Bartsch C, Koester P, Lenartz D, Maarouf M, Daumann J, et al. Motor improvement and emotional stabilization in patients with Tourette syndrome after deep brain stimulation of the ventral anterior and ventrolateral motor part of the thalamus. Biol Psychiatry (2016) 79(5):392–401. doi:10.1016/j.biopsych.2014.05.014
96. Piedimonte F, Andreani JC, Piedimonte L, Graff P, Bacaro V, Micheli F, et al. Behavioral and motor improvement after deep brain stimulation of the globus pallidus externus in a case of Tourette’s syndrome. Neuromodulation (2013) 16(1):55–8; discussion 8. doi:10.1111/j.1525-1403.2012.00526.x
97. Kuhn J, Lenartz D, Mai JK, Huff W, Lee SH, Koulousakis A, et al. Deep brain stimulation of the nucleus accumbens and the internal capsule in therapeutically refractory Tourette-syndrome. J Neurol (2007) 254(7):963–5. doi:10.1007/s00415-006-0404-8
98. Neuner I, Podoll K, Lenartz D, Sturm V, Schneider F. Deep brain stimulation in the nucleus accumbens for intractable Tourette’s syndrome: follow-up report of 36 months. Biol Psychiatry (2009) 65(4):e5–6. doi:10.1016/j.biopsych.2008.09.030
Keywords: Tourette, tics, DBS, centromedian–parafascicular, CM-Pf, thalamus
Citation: Testini P, Min H-K, Bashir A and Lee KH (2016) Deep Brain Stimulation for Tourette’s Syndrome: The Case for Targeting the Thalamic Centromedian–Parafascicular Complex. Front. Neurol. 7:193. doi: 10.3389/fneur.2016.00193
Received: 15 March 2016; Accepted: 24 October 2016;
Published: 10 November 2016
Edited by:
Marina Bentivoglio, University of Verona, ItalyReviewed by:
Maria Fiorella Contarino, Leiden University Medical Center, NetherlandsRenato Puppi Munhoz, Pontifícia Universidade Católica do Paraná, Brazil
Copyright: © 2016 Testini, Min, Bashir and Lee. This is an open-access article distributed under the terms of the Creative Commons Attribution License (CC BY). The use, distribution or reproduction in other forums is permitted, provided the original author(s) or licensor are credited and that the original publication in this journal is cited, in accordance with accepted academic practice. No use, distribution or reproduction is permitted which does not comply with these terms.
*Correspondence: Kendall H. Lee, bGVlLmtlbmRhbGxAbWF5by5lZHU=