- 1Department of Neurology, Ludwig Maximilians University, Munich, Germany
- 2Department of Translational Neurodegeneration, German Center for Neurodegenerative Diseases (DZNE), Munich, Germany
- 3Graduate School of Systemic Neurosciences, Ludwig-Maximilians-Universität München, Martinsried, Germany
- 4Department of Neurosurgery, Ludwig Maximilians University, Munich, Germany
Deep brain stimulation (DBS) has become the treatment of choice for advanced stages of Parkinson's disease, medically intractable essential tremor, and complicated segmental and generalized dystonia. In addition to accurate electrode placement in the target area, effective programming of DBS devices is considered the most important factor for the individual outcome after DBS. Programming of the implanted pulse generator (IPG) is the only modifiable factor once DBS leads have been implanted and it becomes even more relevant in cases in which the electrodes are located at the border of the intended target structure and when side effects become challenging. At present, adjusting stimulation parameters depends to a large extent on personal experience. Based on a comprehensive literature search, we here summarize previous studies that examined the significance of distinct stimulation strategies for ameliorating disease signs and symptoms. We assess the effect of adjusting the stimulus amplitude (A), frequency (f), and pulse width (pw) on clinical symptoms and examine more recent techniques for modulating neuronal elements by electrical stimulation, such as interleaving (Medtronic®) or directional current steering (Boston Scientific®, Abbott®). We thus provide an evidence-based strategy for achieving the best clinical effect with different disorders and avoiding adverse effects in DBS of the subthalamic nucleus (STN), the ventro-intermedius nucleus (VIM), and the globus pallidus internus (GPi).
Introduction
Since the pioneering work of Cooper et al. (1) and of Benabid et al. in the early 1990s (2), deep brain stimulation (DBS) has become the treatment of choice for advanced stages of Parkinson's disease (PD), for medically intractable essential tremor (ET), and for complicated segmental and generalized dystonia. Although overall considered an effective treatment in these diseases, a number of specific factors determine the treatment success: in addition to careful patient selection and accurate electrode placement, the effective post-operative programming of DBS devices is considered the most important factor for the individual patient outcome (3–5). Programming is the only modifiable factor once a patient has been implanted with DBS leads and it becomes even more relevant in cases in which the DBS electrodes are located at the border of the intended target structure. Current implantation techniques, using either stereotaxic frames or surgical robots, exhibit an average precision in the range of 1–2 mm from the target area (6–12). In addition, the brain itself can shift by 2–4 mm during surgery (13–15), contributing to imprecise lead placement. According to previous studies, such errors occur in up to 40% of DBS surgeries (16–20), thus underscoring the importance of post-operative programming to compensate for such variability. Inefficient stimulation may result in unnecessary follow-up visits and reduced patient satisfaction with DBS (21). Conversely, sound programming has been shown to improve patient outcomes and to avoid unnecessary lead revisions (19). In addition, improvement with re-programming highlights that proper adjustment of stimulation parameters is a major factor for successful treatment and patient satisfaction (22).
Despite established strategies for adjusting neurostimulation (23–27), DBS programming remains time- and resource-consuming. New leads with two levels of tripartite electrodes (i.e., segmented electrodes) (Abbott®, Boston Scientific®) can improve the therapeutic window (Figures 1A,B) but increase the number of possible combinations of programming parameters (28) [For a thorough review of currently implanted pulse generators (IPGs) and electrodes see: (29)]. Therefore, there is a need for sophisticated strategies on how to adjust stimulation parameters and lead configurations in a precise and effective manner once the electrodes have been implanted. We here review the current evidence for adjusting neurostimulation in different movement disorders. Regarding the biophysical and physiological effects of DBS, the reader is referred to extensive reviews on this matter (30, 31).
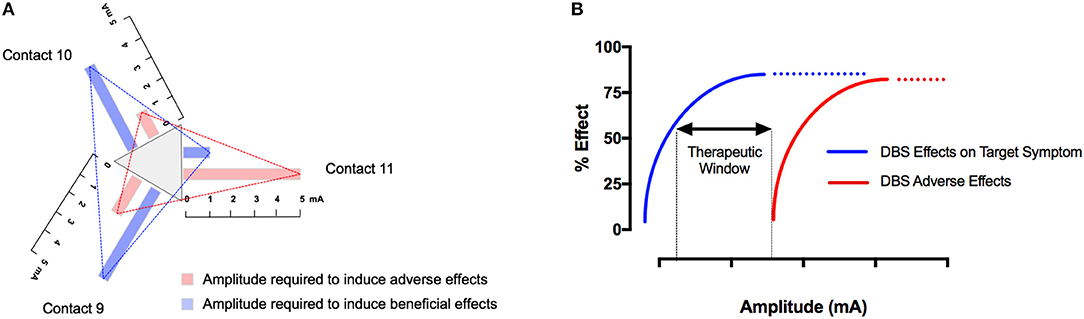
Figure 1. The therapeutic window depends on stimulation parameters and the electrode configuration. In tripartite electrodes, the therapeutic window should be determined for each segment individually by examining the beneficial and adverse effects with increasing the stimulation amplitude under defined pulse width and frequency (A). The therapeutic window in DBS is defined as the gap between the minimum stimulation current required to produce adverse effects and the current required to produce a beneficial effect. Similar to pharmacologic intervention, DSB is a tradeoff between beneficial and adverse effects. Numerous stimulation parameters, as well as the anatomical position of the respective contact, affect the therapeutic window. As a consequence, each electrode contact and each combination of pulse width and frequency thus has an individual therapeutic window (B).
Current Programming Strategies
Specific Programming Strategies for DBS of the Subthalamic Nucleus (STN)
It is thought that adjustment of stimulation parameters is best carried out by trained clinicians (3) and depends to a large extent on personal experience, whereas detailed algorithms for a disease-specific programming strategy are rare, with the exception of expert recommendations (3, 27, 32).
Assessing the Response to DBS:
In order to judge the effect of STN-DBS, rigidity is typically used in PD because it does not fluctuate, responds to stimulation adjustments within seconds (Figure 2A), and does not depend on the patient's fatigue or cooperation (33, 34). When effective stimulation is switched on, rigidity disappears within 20 s, whereas after cessation of stimulation, rigidity returns within 1 min (35) (Figure 2A). This must be taken into account when subsequent tests are performed. In the absence of rigidity, bradykinesia or (rest) tremor can be used, although the response of bradykinesia to changing the stimulation parameters is slower (33) and may be biased by fatigue and the patient's discomfort or expectations and (rest) tremor may fluctuate spontaneously. Gait speed, arm swing during gait, finger tapping, or alternating hand movements can all be measured with a stopwatch to achieve numeric data to supply evidence for a certain stimulator setting. A list of appropriate tests has been suggested (36). Also, selected items from the UPDRS-III scale are used to judge the therapeutic effect and to document effects in a systematic manner. It is noteworthy that no single clinical sign or symptom should be used alone (such as e.g., rigidity) to judge the therapeutic effect. Our clinical experience suggests that one should select from a list of possible tests two or three which characterize the symptoms of the patient best and to apply these tests in a systematic manner during the programming sessions. The contact with the lowest threshold for beneficial effects and the widest therapeutic window is then selected for chronic stimulation (23–27).
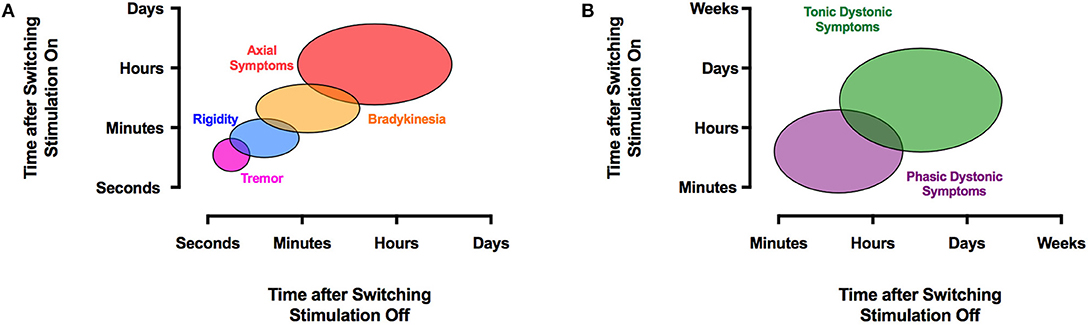
Figure 2. The effects of DBS on clinical symptoms are time-dependent. PD signs and symptoms respond to STN-DBS variably. Axial symptoms may take hours or days to improve, whereas tremor typically disappears almost instantly with STN- or VIM-DBS (A). A similar temporal disparity occurs with dystonia, where phasic dystonic symptoms respond quickly within minutes to GPi-DBS, and tonic dystonic movements may take much longer to resolve (B). The reappearance of symptoms after discontinuation of DBS exhibits a similar temporal pattern.
Electrode Configuration Adjustment
It is commonly suggested that once the leads have been implanted, each ring contact should be tested in a monopolar configuration with the electrode as negative (cathode) and the IPG as positive (anode), a process referred to as monopolar review (3, 27, 32). In some centers, this is done prior to the implantation of the IPG using externalized leads, with the option to adjust the depth of the implanted electrode during the implantation of the IPG. In these cases, stimulation is applied by an external stimulator. Initially, the pulse width and frequency are kept constant at 60 μs and 130 Hz, respectively. Each of the ring electrodes is tested separately with increasing amplitudes to determine the threshold of beneficial effects and, with further increasing the amplitude, to detect the threshold of adverse effects (3, 37). In the case of segmented electrodes, all segments of one ring are activated simultaneously (38). Most authors suggest a gradual increase of stimulation amplitude in steps of 0.1–0.5 V or 0.1–0.5 mA up to a maximum of 5 V or 5 mA, or until side effects occur (3, 25, 37).
When newer DBS leads (Boston Scientific®, Abbott®) with two levels of tripartite electrodes are used, it is suggested that after determination of the clinically most efficient ring, single contacts of this ring are screened in a similar fashion (directional or current steering) (39, 40) (Figures 1A,B). Stimulation of single segments can result in a larger therapeutic window (38). In addition, the average current threshold for obtaining a therapeutic effect was noted to be lower with the best directional stimulation (41–44). In accord, Pollo et al. reported, in their study on intraoperative segmental stimulation, a reduced threshold for clinical efficiency as well as a better clinical efficiency with segmental stimulation (39). Even with small currents of 0.3 mA, these authors were able to induce clinical effects in individual patients, which suggests that the stepwise increase of current during testing may have to be considerably lower than 0.5 mA. In the VANTAGE study, stimulation was performed with the Vercise system (Boston Scientific®) that includes a separate current source for each segment of the lead which contains 8 contacts (45). These authors stimulated the best as well as the second best segment and instructed their patients to optimize the applied current via a patient control device. The authors reported an improvement of over 60% during the ON phase on the UPDRS-III rating scale, which is above the average improvement seen with conventional ring electrodes.
Stimulation Parameter Selection
In order to achieve the best clinical effect, certain stimulation parameters have been determined empirically for STN-DBS. Previous studies investigating the specific contribution of frequency, pulse width, and amplitude found that the amplitude had the greatest effect on ameliorating PD motor signs relative to energy-equivalent changes in frequency and pulse width (23, 24). In one study that examined PD patients with STN-DBS, the amplitude required to improve wrist rigidity ranged from 0.7 to 1.7 mA, and the amplitude required to generate adverse effects was in the range of 1.3–3.4 mA (23). In an intraoperative examination of clinical STN-DBS effects in 17 PD patients, Sauleau et al. found that the threshold for the vanishing of wrist rigidity was 0.94 V (at 130 Hz and 100 μs) (46). Stimulation frequencies of 50 Hz and 130 Hz improved tremor, rigidity, and bradykinesia, with rigidity improving already above a threshold of 33 Hz. In these studies, there was no significant improvement above 185 Hz for either target symptom, although some reports suggest that tremor tends to respond to a higher frequency (47). Using frequencies below 50 Hz in STN-DBS did not improve motor signs, even when the total electrical energy delivered (TEED) was similar (23). In fact, very low frequencies of 5–10 Hz have been found to worsen motor symptoms, in particular, bradykinesia, compared with no stimulation (24, 48, 49). Moro et al. demonstrated that pulse widths between 60 and 210 μs were beneficial for improving tremor and rigidity, while reduction of bradykinesia relative to baseline was only significant at 60 μs. High-pulse-width stimulation (>210 μs) was generally not well-tolerated. No difference in tremor has been observed with different pulse widths (23, 24). In addition to rigidity, tremor, and akinesia, STN-DB has a beneficial effect on off-dystonia (50, 51), whereas improvement in on-dyskinesia is predominantly a consequence of a reduced L-Dopa equivalent dose (LED) (52). Recently, IPGs became available which allow for even shorter pulse widths 60 μs. The CUSTOM-DBS study by Steigerwald et al. investigated 15 PD patients with STN-DBS and found that for STN stimulation, a shorter pulse width of 30 μs resulted in a larger therapeutic window with a non-inferior therapeutic efficacy (as measured by the UPDRS III score) when compared to the standard pulse width of 60 μs (53). Also, another group showed that stimulation using 30 μs pulse-width results in better walking and speech performance at a similar total electrical energy delivered (TEED) (54). Therefore, the previous recommendation for a fixed pulse width of 60 μs in STN DBS is clearly challenged, although future research needs to confirm these encouraging findings.
Typical Side Effects in STN-DBS
Most DBS side effects can be understood as a result of current spreading into brain regions adjacent to the target area. The STN is a relatively small, ovoid structure with a close anatomical relationship with other deep brain nuclei and tracts, including the internal capsule (lateral, anterior), the substantia nigra (ventral), the red nucleus (medial), the fibers of the third cranial nerve (medioventral), the thalamic fasciculus, also termed field H1 of Forel and composed of the ansa lenticularis and the lenticular fasciculus (mediodorsal), the sensory thalamic nuclei (dorsal), the zona incerta (ZI) and cerebello-rubro-thalamic fibers (medial dorsal, posterior), and the hypothalamus and medial forebrain bundle (anterior) (55, 56) (Figure 3). In addition to these anatomical relationships, the STN is subdivided into different territories (motor, oculomotor, associative, and limbic), each with different connections and specific functions (57). Previous studies that have analyzed the anatomical location of the most effective contacts used for chronic stimulation showed varying results: the majority of reports suggest that the most effective contacts to ameliorate PD symptoms segregate to the dorso-lateral, sensorimotor aspect of the STN (58–64), whereas current spread to the limbic and associative sub-segments may cause unwanted affective and cognitive side effects (65–68). Conversely, other studies recommended targeting other areas or even adjacent regions such as the zona incerta (ZI) or the Forel fields H1/H2 (69–76) and one study found no significant association between the position of the active contacts and the clinical effect (77). This heterogeneity may be a consequence of methodological differences among the studies, as different imaging techniques were applied to define the position of the electrodes including ventriculography, CT and MRI (78). In addition, classical studies applied numerical coordinates referenced to the stereotactic space to define the contact position, making the results difficult to interpret without knowing the patient's individual anatomy and because a volume of tissue is represented by a single point. The following adverse effects in STN-DBS can be derived from the function of the adjacent anatomical structures:
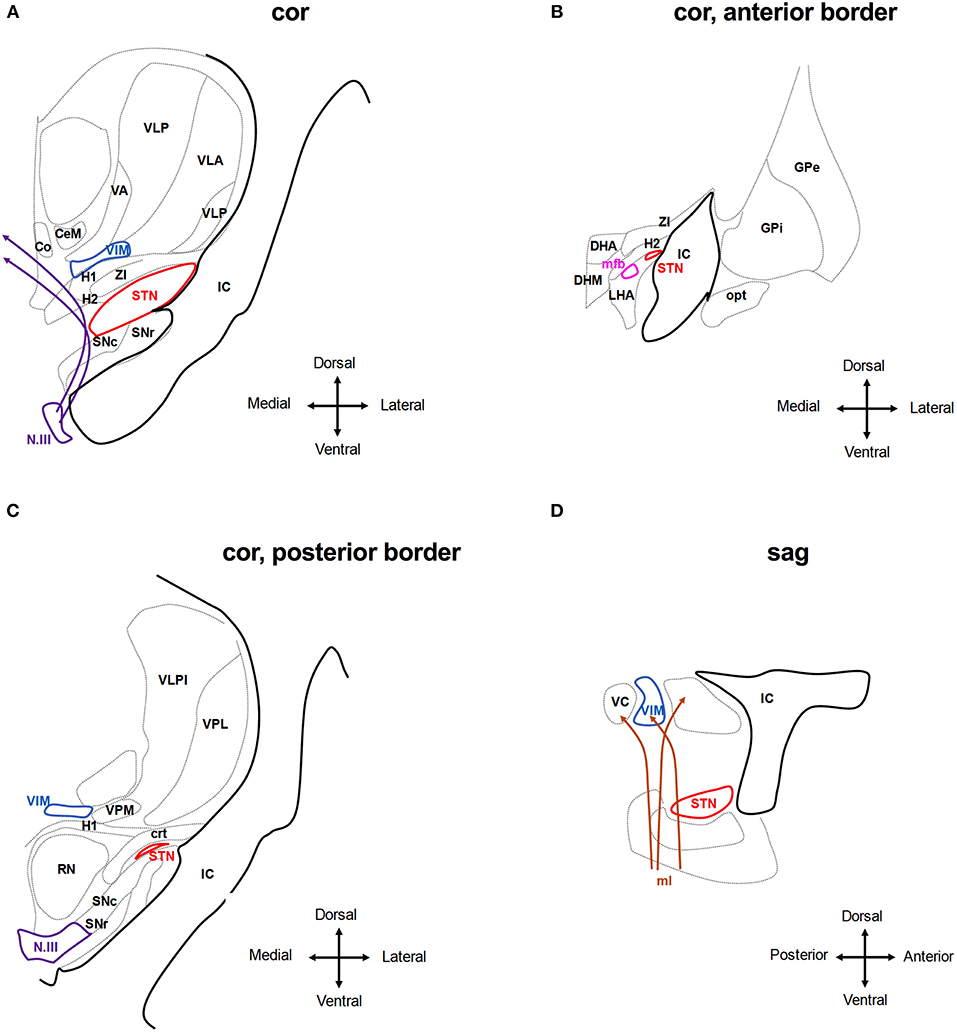
Figure 3. Anatomical relationship of the subthalamic nucleus (STN) and the ventral intermedius nucleus (VIM) to adjacent structures. The schematic shows coronar (A–C) and sagittal (D) planes through the basal ganglia at the level of the STN and VIM. Co, Commissural nucleus; CeM, central medial thalamic nucleus; VA, ventroanterior thalamic nucleus; VC, ventrocaudal nucleus; VLP, ventrolateral posterior thalamic nucleus; VPM, ventroposterior medial thalamic nucleus; IC, internal capsule; SNr, Substantia nigra pars reticulate; and SNc compacta; H1, H2, H1 and H2 Fields of Forel; ZI, zona incerta; N.III, nucleus of the third cranial nerve; DHA, dorsal hypothalamic area; DHM, dorsomedial hypothalamic nucleus; LHA, lateral hypothalamic area; mfb, medial forebrain bundle; opt, optic tracts; RN, red nucleus; crt, cerebello-rubro-thalamic tract. Stimulating the tissue medial and dorsal to the STN activates the H1 and H2 fields of Forel and the ZI and may reach to the medio-dorsal thalamic nuclei incl. the Co, CeM, and VIM. Deflection of the field to more ventral areas will activate the fibers of the N.III and the SN (A). Anterior of the STN, stimulation may activate hypothalamic nuclei and mfb as well as the IC (B,D). At the posterior border of the STN, stimulation may activate the RN and ml, in particular, if the tissue medial of the STN is activated. Stimulation of tissue dorsal of the STN may activate the crt. (C,D). Adjusted from Mai et al. (55).
Spastic muscle contractions: The most frequent adverse effects include (spastic) contractions involving the facial muscles (“facial pulling”), which often affect bilateral upper facial and contralateral lower facial muscles (79, 80) and are a consequence of current spread into the internal capsule (IC) lateral and anterior to the STN (Figures 3A,D). By modeling the electric field caused by STN-DBS, it was found that even small deviations in the electrodeposition within the STN can result in activation of large diameter myelinated IC axons over a volume that spreads outside the borders of the STN (81).
Uni- or bilateral gaze deviation: Typical oculomotor side effects are reduced gaze ipsilateral to stimulation, sometimes progressing to contralateral gaze deviation. This resembles conjugate eye deviation during frontal epileptic seizures and is therefore assumed to be caused by activating fibers stemming from the frontal eye field (FEF) which run in the internal capsule in three bundles: a dorsal trans-thalamic trajectory, an intermediate bundle crossing the subthalamic region, and a ventral bundle in the medial portion of the cerebral peduncle, which projects, among other structures, to the subthalamic nucleus (82). Analyzing 22 electrode locations which intraoperatively could elicit conjugate eye deviations, these positions were found to lie within the lateral anterosuperior border of the STN (Figures 3A,D). This resulted in the recommendation to place the lead or deflect the field to a more medial, posterior, and inferior position (83). In a single case, this phenomenon was elicited with the STN contacts which provided the best clinical efficiency and could be compensated by bilateral STN stimulation (84). These eye movements consisted of several saccades and were accompanied by turning the head. Thus, contra-versive and conjugate eye deviation cannot be generally taken as evidence for electrode misplacement. Conversely, activating the fibers of the third nerve (N.III) that run inferomedial to the STN and within the red nucleus (RN) below the STN may result in unilateral gaze deviation and diplopia (Figures 3A,C). Tamma and co-authors claim that stimulation of oculomotor fibers causes adduction or reduced abduction or elevation of the superior eyelid in the ipsilateral eye (85). Also, in another report, unilateral eye deviations were frequently seen during intraoperative test stimulation when the electrode was medial, posterior, and ventral to the final target (46). However, this far medial position makes unwanted stimulation of these fibers an extremely rare instant. In experimental stimulation of the third nerve in macaques, only small adduction of the eye was seen but prompt miosis, as expected from physiology (86). Eyelid opening apraxia has also been observed (51), although this symptom may be present as part of PD itself, and is occasionally relieved by stimulation but also can be induced by stimulation above the clinically efficient threshold (87). Mydriasis is rather frequently seen during intraoperative test stimulation and post-operative adjustment along with ipsilateral perspiration. These are quickly adapting symptoms and are not considered as evidence for a misplaced electrode. The central sympathetic tract runs medial to the red nucleus anteriorly to the aqueduct and is therefore not involved, but sympathetic fibers within the zona incerta (ZI) (88) or within the STN (Figures 3A,B) are assumed to be stimulated when mydriasis occurs.
Autonomic side effects: Nausea and excessive sweating are likely a consequence of medial and anterior current spread, presumably corresponding to tissue activation in the hypothalamus and red nucleus (85, 89) (Figures 3B,C). Approximately half of all STN-DBS cases experience dizziness, a sense of heavy- or lightheadedness, or malaise (51).
Paresthesia: Contralateral paresthesias may be due to stimulation of the medial lemniscus which conveys somatosensory information from the joints and skin and lies ventroposterior to the STN (Figure 3D). With the usual frontal entry of the lead the lowermost contacts may thus encroach on this structure (89). Mostly, paresthesias are transient but when they persist, a more dorsal contact may be chosen, if clinically effective.
Speech impairment: The impairment of speech frequently occurs during the initial programming and long-term follow-up of STN DBS (37, 90) but can be ameliorated through proper programming (91). Dysarthria occurs in about 25% of STN-DBS cases and may be caused by current spread into the internal capsule (strained or spastic speech) or otherwise into the pallidal and cerebello-thalamic fiber tracts (crt) medial and dorsal of the STN (92–94) (Figures 3A,C,D). It is therefore important to distinguish the different causes of DBS-induced dysarthria to be able to adjust stimulation contacts and parameters. In addition, stimulation of the STN itself may account for speech impairment. In particular, medial left-sided stimulation in right-handed patients had a negative effect on prosody, articulation, and overall intelligibility (95–97). Accordingly, higher left STN voltage is associated with deterioration of speech (98). Similarly, other reports demonstrated a strong correlation between high voltages in the left STN and speech impairment (99–101).
One report suggested high stimulation frequency to increase the risk of speech impairment (102). Another report suggested high-frequency stimulation to have a negative effect on speech-related velopharyngeal control (103).
Dyskinesia: STN-DBS may induce dyskinesia, such as choreiform, ballistic, or dystonic movements reminiscent of levodopa-induced dyskinesia (52). Dyskinesias occurring during the initial post-operative programming period are thought to indicate a good outcome and the contact inducing dyskinesia is usually the most effective in ameliorating motor symptoms (52, 104–106). Rare dystonic effects in STN-DBS included dystonia of head and neck muscles with stridor and dysphagia (107, 108).
Gait impairment and postural instability: Overall, L-Dopa responsive axial symptoms are also more likely to improve with STN-DBS and indeed, various studies reported gait improvement with STN-DBS (109–115), in particular in terms of gait velocity and amplitude of arm and leg swing. On the other hand, long-term follow-up studies (116, 117) have consistently shown that axial symptoms including gait may worsen over time in contrast to the sustained improvement of cardinal motor signs, suggesting a differential effect of DBS on the distal and axial neural control circuits (118–120). Indeed, increasing the stimulation amplitude can worsen gait and increase freezing episodes similar to no stimulation as discussed further in detail in section Specific Programming Strategies to Counteract Side Effects in STN-DBS. However, the cause of gait impairment in DBS is most likely multifactorial (121) and, apart from stimulation-induced worsening through the current spread, disease progression, medication reduction, and cognitive decline may contribute. Postural instability is the least likely to respond to DBS and STN-DBS appears to be more detrimental to postural stability as compared to GPi-DBS (122, 123). Although there is no evidence to support a certain programming strategy to avoid worsening of postural stability, a recent study suggested that limiting current spread to the non-motor territories of the STN would liberate cognitive resources that could be used to maintaining a steady posture (124, 125) and to improve postural stability (126). Because certain studies suggested that trunk ataxia to be a consequence of activating the red nucleus, directing the current to more lateral areas might be also helpful.
Acute neuropsychiatric side effects: STN-DBS may cause acute neuropsychiatric alterations in addition to preexisting psychiatric comorbidities that can decompensate during or after surgery (106). Neuropsychiatric signs can be observed in individual subjects during initial programming and may include apathy (112, 127), mirthful laughter (66) as well as acute mania (68, 128) and acute depression (129–131).
Depression: In a case described by Bejjani et al., depression occurred while all contacts were screened in the post-operative setting. When contact the most ventral (Figures 3B,C) was activated, depression set in after 5 s. of stimulation with 2.4 V. This contact was not efficient in relieving PD symptoms and was shown to be located within the substantia nigra. Stimulation of more dorsal contacts provided relief from PD motor signs without causing depression. In addition, apathy and depression may be due to a “hypodopaminergic” state as a consequence of a quick or radical reduction in dopaminergic medication (132). Recognizing depression is highly relevant since these symptoms have an even bigger impact on the live quality of DBS patients than motor function (133, 134).
Mania: Manic episodes due to STN stimulation are assumed to be a consequence of stimulating the medial and ventral aspects of the STN (135, 136). Therefore, the use of more dorsal contacts is recommended in these cases. In addition, stimulating tributary fibers from the STN to the median forebrain bundle may contribute to these symptoms (65).
Impulse Control Disorders (ICD): The relationship between DBS and ICD is complex and in part controversial (137). In general, bilateral STN-DBS was found to either ameliorate or worsen decision-making or to have no effect (138–140). STN-DBS is associated with the risk of binge eating (141, 142) and punding behavior (143). Moreover, STN-DBS may induce hypersexuality, hypomania (144, 145), or compulsive gambling (146). These effects are most likely associated with using the most ventral contacts (147–150) and are assumed to be caused by stimulating the ventromedial, limbic area of the STN (66, 149, 151) as well as the SNr (128) and the medial forebrain bundle (65) (Figures 3A,C). One therapeutic option may, therefore, be to avoid current spread into STN-related limbic circuits by deflecting the electrical field to more dorsal and lateral parts. However, ICD may also resolve or improve after surgery (152, 153) and STN-DBS might in fact be considered to treat ICD in PD (152, 153). Long-term follow-up of patients with STN-DBS showed pre-surgery ICD was abolished in most patients once L-DOPA or dopamine agonist doses were reduced (141) as was the dopamine dysregulation syndrome (154). In these studies, the de-novo onset of ICD was rare and transient with the exception of compulsive eating (141). Similar to motor symptoms, the individual patient outcomes in regard to ICD depend on several factors, including target selection, electrode location, programming settings, appropriate medical management, age, and perhaps genotype (155) and is thus difficult to predict.
Cognitive side effects: The effects of STN-DBS on cognition remain controversial. A reduced verbal fluency is well-described (156), but has been observed with and without stimulation and thus has been attributed to penetrating the caudate nucleus during surgery (157, 158). On the other hand, Morishit et al. and Isler et al. found no significant difference in cognitive decline between caudate-penetrated and caudate-spared groups. In addition, executive dysfunction and altered short term memory have been observed (159, 160). These effects are also considered to be a consequence of stimulating the ventral and medial aspect of the STN (160, 161). However, well-controlled studies did not find detrimental effects of STN-DBS on global cognitive function (162, 163). The etiology is therefore likely multifactorial and due to the surgical lesion of the frontal lobe and caudate nucleus and diseases progression (164).
Specific Programming Strategies to Counteract Side Effects in STN-DBS
Some adverse effects may be transient in nature and will disappear despite continuing stimulation (165). For instance, dyskinesia is a typical side effect of STN-DBS in PD but increasing the amplitude in minute steps and waiting for the dyskinetic symptoms to disappear after each incremental step might ultimately allow for an increase in amplitude required for symptom control despite transient dyskinesia (105). Moreover, it may be sufficient in some instances to adjust stimulation parameters in order to achieve a more symmetrical or asymmetrical DBS effect. For example, if gait disturbances are prominent in STN-DBS, reducing the stimulation amplitude on the side contralateral to the best motor response resulted in increased stride length, reduction of gait variability, and a reduction in freezing episodes (166). On the other hand, asymmetric stimulation may be helpful in ameliorating the emotional side effects of STN-DBS, that are thought to be lateralized (167). The latter study demonstrated emotional auditory stimuli to induce activity in the ventral non-oscillatory region of the right STN but not in the left ventral STN or in the dorsal regions of either the right or left STN. These results suggest that DBS of the right ventral STN may be associated with beneficial or adverse emotional effects observed STN-DBS. The authors suggest that the stimulation parameters in the right STN should be modified to counteract psychiatric side effects. This hypothesis is tempting but needs further confirmation from clinical studies. When permanent side effects occur, either the stimulating contact or the stimulation parameters may be changed or, as the last option, the electrode may be repositioned. The first step is to check the electrode position in case this is not done routinely after surgery or if post-surgical images are not available. The second step is to reduce the current of the activated contact(s) and/or choose another contact for stimulation. For example, choosing a more dorsal contact is recommended when persistent paresthesias occur as well as in psychiatric symptoms (see above). Alternative electrode configurations can be achieved by combining single contacts to a compound cathode (double or triple monopolar) or by setting another lead contact as an anode (bipolar). The latter allows the volume of tissue activated (VTA) to be restricted at the expense of higher energy consumption (3), although one should be aware that the extent of the computed VTA varies substantially with the material properties of the surrounding brain tissue (168–171). Alternatively, interleaving stimulation (Medtronic®) may be applied. Interleaving stimulation (ILS) consists of rapid and alternate activation of two electrode contacts with two distinct amplitudes and pulse widths but with the same frequency up to a maximum of 125 Hz and a delay of 4 ms between two stimuli. In general, ILS may be applied either to limit stimulation-induced adverse effects or else, to stimulate different brain regions with individualized settings in order to alleviate specific symptoms (47). For example, ILS was successfully applied for freezing of gait (additional stimulation of substantia nigra) (101) as well as tremor (additional stimulation of zona incerta) (172). However, with the exception of case reports and small case series (172–177), there are no larger prospective trials that have investigated the clinical effect of ILS. In accord with previous reports, a recent study from Kern et al. demonstrated improvement with ILS for adverse effect management predominately for the treatment of dyskinesia and improvement of PD motor symptoms with ILS (178), whereas ILS was less effective in ET and dystonia. Of note, a contact was added into the rostral zona incerta (ZI) (Figure 3A) in the majority of dyskinetic patients, thus suggesting a particular role of the ZI and the surrounding pallido-thalamic fibers for improving dyskinesia and a potential ILS target in STN-DBS. These alternative targets are under active investigation for treating dyskinesias (174, 179, 180), although sound evidence for using these structures is still lacking. A drawback of ILS is that battery drainage is likely increased with ILS as 2 independent programming settings are applied (181).
Short Pulse Width Stimulation (SPWS)
Decreasing the standard pulse width, which is currently only possible with Boston Scientific® or Abbot® devices, represents an alternative strategy to counteract unwanted side effects in STN-DBS (53, 182). For example, Reich et al. investigated pulse widths below 60 μs at a frequency of 130 Hz and found that compared to (standard) 60 μs stimulation, the therapeutic window increased by a mean of 182% with a PW of 30 μs, and decreased by 46% with a PW of 120 μs (183). Although the stimulation amplitude required for rigidity control increased with reducing pulse widths from a mean of 1.6 mA at 60 μs to 2.9 mA at 30 μs, the TEED required for the clinical effect of rigidity control decreased. This is thought to be mediated by more selective action of stimulation on the fiber tracts that are responsible for symptom relief while the neighboring thick and myelinated corticospinal and corticobulbar fibers are thought to be less affected by short pulse width stimulation (184–186).
Low-Frequency Stimulation (LFS)
If gait and balance issues such as freezing of gait (FOG) or other axial symptoms predominate, LFS (60–80 Hz) may be a good treatment strategy for PD patients with STN-DBS. FOG is a gait disorder featured by recurrent transient gait retardation and interruption that occurs in PD, PD-plus syndromes and vascular parkinsonism. Most FOG episodes are related to the OFF state in PD, but severe cases begin to suffer from ON state FOG (ON-FOG). FOG increases the risk of falls for PD patients and has a large impact on the motor function and daily life of the patients. HFS-DBS of the STN can alleviate FOG in some patients, particularly if FOG is related to medication OFF state (187–189). On the other hand, HFS-DBS may induce FOG in PD (190, 191). Pharmacological treatment options for FOG include L-DOPA (192), methylphenidate and amantadine (193, 194). Alternatively, the stimulator may be switched to LFS. LFS (60–80 Hz), compared to HFS (130 Hz), has been shown to have beneficial effects on improving FOG and other axial symptoms, such as speech and swallowing function, in PD patients with bilateral STN-DBS in some studies (190, 191, 195–198) or selected patients (199), but not in others (200–203). Some found short-term but not long- term beneficial effect (204), while others found both short-term and long-term benefits after 6 weeks, 8 months and even 10 months study periods (190, 195, 197). It is not well-understood, which factors account for the different responses of FOG and other axial symptoms to LFS. Possible factors include the presence or absence of pre-existing FOG, the frequency used (60 vs. 80 Hz), the maintenance of the TEED [TEED = (voltage2 × pulse width × frequency)/impedance)] with frequency adjustment and the location of the active contacts (ventral vs. dorsal). In most studies, adjusting for TEED appeared to be less relevant than the frequency (205). This in line with the finding that neuronal responses relative to frequency are highly non-linear as demonstrated by Huang et al. (206). In summary, it is currently unclear, which patients benefit most from LFS vs. HFS, but likely applies to patients that have pre-existing FOG at HFS-DBS on exam (190, 195–197). In some studies, switching from a high to low frequency (<100 Hz) stimulation also ameliorated speech intelligibility (207) and acoustic parameters such as hypophonia (196). On the other hand, tremor control has been observed to be worse with lower frequencies (190, 197, 204).
Alternative Electrode Targets for Axial Symptoms and Gait Disorders
If there is a beneficial effect of LFS on gait, it may be caused at least in part, by affecting neurons that project to the pedunculo-pontine nucleus (PPN) as unilateral or bilateral LFS of this structure directly and in combination with stimulation of additional target structures has been shown to improve FOG (99, 187, 208–213). The PPN has reciprocal cholinergic connections with the STN, its degeneration may be crucial in the pathophysiology of gait and balance deterioration in PD (214, 215) and stimulation of the PPN may improve axial symptoms in PD (216, 217). The PPN may be stimulated by leads in this region alone or in conjunction with the STN, the SNr, or the GPi (99, 211, 212). Interestingly, the optimal contact positions for LFS were more ventrally located in the STN than optimal contacts for 130 Hz-stimulation (198). More recently, there has been interest in the stimulation of the SNr, which is located ventrally and medially to the STN (218). One study found that among PD patients treated with STN-DBS at 130 Hz via the most distal contact of the quadripolar electrode resulted in an improvement of gait and posture (100). Subsequently, another group of researchers used interleaving to stimulate both the STN and the SNr (101) and found that FOG was significantly improved with combined STN/SNr stimulation, although other axial symptoms on UPDRS did not significantly differ. At the same time, stimulating the SN also comprises the risk of worsening akinesia and of inducing depressive symptoms. In summary, the combined stimulation of PPN plus STN, PPN plus GPi, or STN plus SNr, may be useful for the treatment of FOG in PD patients. The optimal combination of nuclei to be stimulated and the stimulation parameters need to be determined by future clinical trials. In addition to its effect on gait and balance, LFS may reduce stimulation-induced dyskinesia (219, 220). This may be particularly relevant for dorsal-projecting contacts in or close to the ZI above the STN, that have been reported to have an anti-dyskinetic effect with different stimulator settings (178, 221, 222).
Optimal Initiation Time for Programming and Adjusting Pharmacotherapy in STN-DBS
General Considerations on Post-Operative Care
The time point to initiate DBS after STN implantation surgery varies between institutions. Early programming (within the first days after surgery) satisfies the patient's wish for a timely treatment but may be hindered by a improvement in PD symptoms due to the lesion caused by the electrode (stun effect) which may last up to 2 weeks (223, 224) or even longer: the mean medication “ON” time improved 3 months after STN electrode implantation even in the absence of electrical stimulation (115), thus demonstrating an improvement with surgery alone. At which time point DBS is initiated after surgery thus depends on the procedures established in each institution. In any way, the initial programming should be performed after an overnight washout of dopaminergic drugs so that the effect of DBS can be assessed without the interference of medications (37). Adjusting anti-parkinsonian drugs typically occur after initial programming of STN-DBS. There is no specific evidence on how and when to adjust medication after STN-DBS is programmed. The insertional effect and the effect of the electrical stimulation synergize to ameliorate PD symptoms, thus requiring a reduction of the pre-operative LED to avoid dyskinesia. In addition, there may be significant placebo or nocebo effects subsequent to electrode implantation. Stopping dopaminergic medication altogether is not recommended, as this may induce a hypodopaminergic state including apathy and depression. Importantly, these symptoms may develop even weeks after the cessation of dopaminergic drugs (225–227). In particular, in patients that suffer from impulse control disorder, cutting dopamine agonists is advisable (152). Otherwise, L-Dopa should be reduced first (228, 229). Finally, reducing L-Dopa might unmask preexisting Restless Legs Syndrome that would have to be considered for treatment.
Constant Voltage vs. Constant Current Stimulation
In addition to the micro-lesion effect, the fluctuation of impedances may bias the determination of the therapeutic window in the early post-operative period (230) which might become more relevant hen using constant-voltage stimulation (CVS) where the current delivered is inversely proportional to the electrode impedance. Conversely, current-constant stimulation (CCS) may offer more stable stimulation, in particular when programming soon after surgery (231, 232). Apart from possibly affecting the outcome in an individual patient, using CCS instead of CVS might allow for an improved generalization of outcome between subjects such that knowledge gained from one set of subjects can be generalized to others. Because the total current delivered current depends on both voltage and impedance, and since voltage is held constant with CVS, potential variations in current over time will be mainly a consequence of impedance fluctuations. Data from examining non-human primates using a small version of the human DBS lead supported this hypothesis (233) as the electrode impedance progressively increased over 7 days post-implantation, resulting in a reduction of current delivered. Benabid et al. reported impedance changes in patients with VIM stimulation for ET. These authors observed an increase in impedance of 33% (on average) over 3 months following the implantation of DBS leads. Thereafter the impedance stabilized (234). Sillay et al. measured impedances in 63 DBS patients with PD, essential tremor, and dystonia at various time intervals following DBS surgery (235). All measurements were performed at >25 days post-operatively, and in the absence of changes in the stimulation parameters between time points. On average, the authors found no significant intra-patient or intra-electrode impedance changes. However, over half had a small increase in impedance over time, and 40% had a small decrease in impedance, with the largest change observed being 23% in a single subject. Hemm et al. described similar results in patients with dystonia (236) observing that impedance values changed only slightly over time within a single patient but that there were differences between patients and between active and non-active DBS contacts. However, Cheung et al. analyzed a large database of impedance measurements from 94 subjects, ranging from 6 months to 5 years after implantation. They found that a significant amount of impedance variability could be expected in chronically implanted DBS electrodes, with a range spanning from 18 to over 600 Ω (237). Studies that compared CCS and CVS did not show any significant differences in non-motor outcomes, including cognition, mood, and quality of life in a double-blind crossover trial (238). A retrospective analysis of 19 patients with PD and dystonic syndromes switched from CVS to CCS reported no change in measured clinical outcomes and therapy satisfaction at 6 months (115, 239), whereas a more recent study found better outcomes with CCS (240). Taken together, the relevance of changes in the electrode impedance and, as a result, the total electric charge transferred, is uncertain and the specific consequences of using CCS vs. VCS stimulation are not yet clear (231, 241, 242) and currently, there is no clear evidence to support an early or late post-operative initiation of DBS.
VIM-DBS in Essential Tremor
Specific Programming Strategies in VIM-DBS
Compared to STN-DBS, the evidence for adjusting neurostimulation parameters in VIM-DBS is limited. In case of ET, kinetic tremor, the principal target of stimulation adjustments, the limb can be assessed with the finger-to-nose or finger-to-finger maneuver or by asking the patient to draw a spiral, drink water from a cup or pour water from a glass into another one. In addition, postural tremor can be assessed with the arms outstretched or elbows bent (wing-beating position). In general, the programming strategies outlined above can be applied for VIM-DBS. Using a pulse width of 60 μs and a frequency of 130 Hz, the current intensity is usually increased progressively until tremor stops or until side effects are encountered. If the tremor is not optimally controlled at 3.5 volts, pulse width and then the frequency of the stimulation may be increased (243). Studies evaluating the effect of different stimulation parameters in ET showed that tremor responds best to increase the amplitude and is further improved by 25% with longer pulse widths (90–120 μs). The frequency-response curve shows an inverse linear relationship between tremor magnitude and frequency between 45 and 100 Hz and a plateau above 130 Hz, although an additional but variable effect between 130 and 200 Hz has been documented (2) (244–247). Similar to what has been demonstrated for STN-DBS, reducing the pulse width has been shown to widen the therapeutic window in ET (248) where the minimum pulse width for suppression of tremor was shown to be significantly different to that for induction of ataxia, with values of 27 and 52 μs, respectively (249). Comparing directional stimulation with segmented electrodes to conventional ring stimulation, Rebelo et al. found an increased therapeutic window and reduced current with stimulation in the best direction compared to the best omnidirectional stimulation alternative (44) (Figure 1). Likewise, alternative targets directly adjacent to the VIM have been described for ET. For instance, the caudal ZI has been examined as a target for patients with tremor suggesting that ZI stimulation may even exceed tremor control through stimulation of the VIM (250–253). These findings are consistent with results from diffusion tensor imaging data suggesting that the best tremor control is obtained with stimulation of the cerebello-thalamic afferents, which are embedded in the ZI (249).
Typical Side Effects in VIM-DBS
The VIM nucleus of the thalamus is located close to the STN in the vicinity of the internal capsule (lateral), the centromedian and parafascicular nucleus of the thalamus and the commissural nucleus (medial), the zona incerta (ZI) and H1/H2 field of Forel (ventral), the ventroanterior (VA), the ventrolateral anterior (VLA) and posterior (VLP) nuclei of the thalamus (dorsal), and the ventromedial thalamic nucleus (VM) (anterior, posterior) (55) (Figure 3). Common side effects include the following:
Paresthesia is the most common short term side effect because the electrical field reaches into the thalamic sensory nuclei dorsal to the VIM (Figure 3A). It can be transient, lasting from a few seconds to minutes, or permanent, and only resolving with reducing stimulation (2, 234, 254).
Speech impairment: Dysarthria is a significant complaint in more than half of ET patients with bilateral VIM-DBS (255), although dysarthria is common in ET even in the absence of DBS. This is relevant because clinicians often choose suboptimal stimulation parameters to avoid stimulation-induced side effects, more frequently seen in patients with bilateral VIM-DBS (255, 256). Speech impairment appears to occur more frequently with higher stimulation amplitudes and with more ventral stimulation contacts. As with STN-DBS, dysarthria may be caused by interference with the cerebello-thalamic or with motor fibers of the internal capsule (Figures 3C,D) located laterally to the VIM causing spastic dysarthria (257) and appropriate contact adjustment may be beneficial.
Gait ataxia: Another common complaint in patients with VIM-DBS is balance issues with an unsteady gait. As with speech disturbances, current spread into dentato-thalamic afferents lateral and ventral the VIM (Figure 3C) may be the cause of such gait and limb ataxia (258–260), although gait and limb ataxia can be a sign of ET itself, commonly referred to as ET-Plus (261). Switching off DBS even for several days can help to distinguish between the two, but rebound tremor needs to be considered.
Loss of Stimulation Benefit: In ET, the energy required for tremor suppression and the number of active contacts typically increase as the disease progresses and this effect is more common in ET as compared to other tremor types (262–265). Indeed, some studies showed the initial improvement in activities of daily living evident at 1 year after the DBS implantation to be lost in the long run except the ability to eat (266). The loss of long-term benefit in ET has been attributed to DBS tolerance, natural disease progression, and other factors including brain atrophy (234, 266–271). Possible strategies to avoid the adaptation of neuronal networks in ET include switching the stimulation off at night (255), inverting the electrode configuration in patients using bipolar settings or on-demand stimulation.
GPi-DBS in Generalized and Segmental Dystonia
Specific Programming Strategies in GPi-DBS
GPi-DBS has been applied worldwide as a surgical treatment alternative for medical refractory segmental or generalized dystonia. Although GPi-DBS seems to be more effective for isolated than non-isolated dystonia (272), there is no evidence that non-isolated dystonia needs a different programming approach (273–275). The role of specific stimulation parameters on dystonic symptoms is probably even less established than with VIM-DBS for ET. This is likely a consequence of the heterogeneity of symptoms. In addition, and unlike in STN- and VIM-DBS, where the effect is observed within seconds to minutes, the effect of GPi-DBS on dystonia may not occur for hours, days, or in some cases even months (Figure 2B). For instance, Krauss et al. noted that phasic dystonic movements were often relieved within minutes of stimulation onset, whereas improvement in tonic posturing took several months to fully manifest (276). When adjusting neurostimulation in dystonia, phasic dystonic movements, such as dystonic neck movements, are therefore best suited for evaluation because tonic dystonic components usually need more time to improve (277). This may be in part be due to musculoskeletal abnormalities caused by long-standing dystonic posture. Accordingly, most GPi-DBS patients fail to show a clear insertional effect (277). In accord, tonic dystonic symptoms may take a lot longer to reappear upon cessation of GPi-DBS than phasic one (278–281) (Figure 2B). In some cases, discontinuing GPi-DBS may result in a clinical rebound effect with acutely severe symptoms (282, 283). The principal programming algorithm follows the same recommendations as with PD or ET with some modifications (3). For instance, a high frequency of 185 Hz has been proposed to be effective in GPi-DBS (284). There is a debate on the selection of the contact for chronic stimulation as there is a poor correlation between benefit and stimulation in different regions of the GPi. Cheung et al. recently identified a small area located squarely in the middle of the GPi as a potential specific therapeutic target for DBS for dystonia (285), whereas recent evidence from our own group suggests that most efficient DBS electrodes displayed a close anatomic proximity to the pallidothalamic tracts (ansa and fasciculus lenticularis) between the GPi and the pyramidal tract (286). Thus, stimulation is most commonly initiated in the ventral region of the GPi above the optic tract (contacts 0 and 1) (287) with a short pulse width (60–120 μs), high frequency (130–185 Hz) and amplitude just prior to eliciting adverse effects (284, 288, 289). Due to the anatomical location of the target, delayed side effects are less likely to occur than with STN- or VIM-DBS, thus favoring a top-down approach and starting the stimulation with the highest tolerated voltage. The use of high- vs. low-frequency stimulation in dystonia has shown mixed results. Alterman et al. suggested that the use of 60 Hz stimulation can be beneficial in some patients (290), whereas another group preferred high-frequency stimulation (289). Moro et al. concluded that high-amplitude and high-frequency stimulation predict better outcome in cervical dystonia (291). Various pulse widths have been recommended in GPi-DBS. Coubes et al. recommend the use of 450 μs (292). However, another study comparing 60, 120, and 450 μs did not show any significant differences between the three groups (293).
Typical Side Effects in GPi-DBS
The GPi is surrounded by the globus pallidus externus and putamen (anterior, posterior, lateral), the internal capsule, ZI and MFB (medial), the ansa lenticularis (mediodorsal), the optical tract (ventral), the amygdala (laterodorsal), the ventral pallidum (laterodorsal) (55) (Figure 4). As with STN- and VIM-DBS, side effects in GPi stimulation can result from current spreading into neighboring regions in many cases:
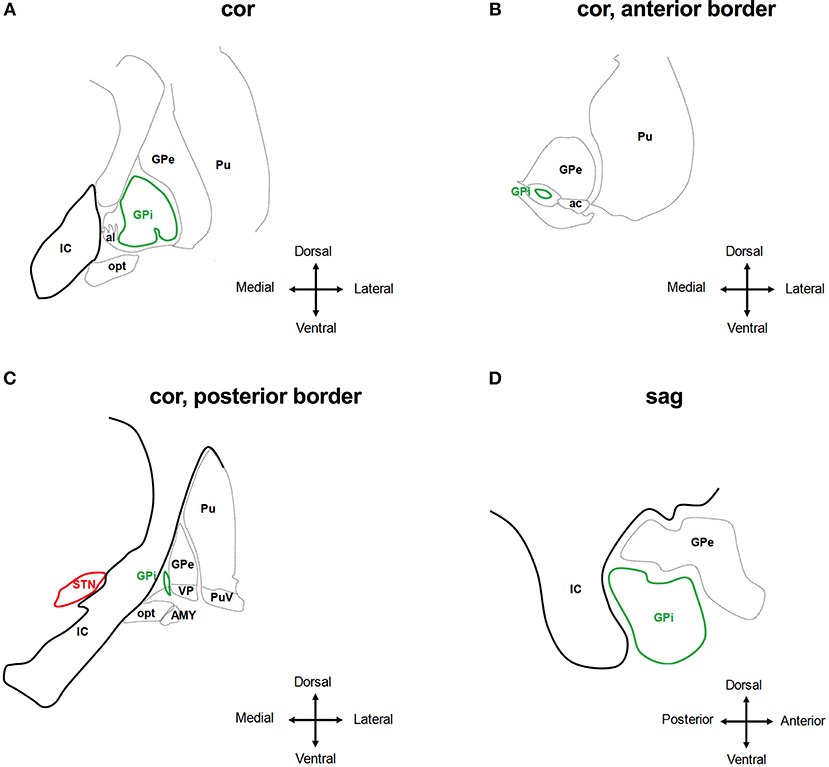
Figure 4. Anatomical relationship of the globus pallidus internus (GPi) to adjacent structures. The schematic shows coronar (A–C) and sagittal (D) planes through the basal ganglia at the level of the GPi. IC, internal capsule; GPe, globus pallidus externus; al, ansa lenticularis; Pu, putamen; opt, optic tract; AMY, amygdala; VP, ventral pallidum; PuV, ventral putamen; STN, subthalamic nucleus. Deflection of stimulation to tissue medial of the GPi will activate the IC, which is less likely the case at the anterior border of the GPi (A,B,D). The AMY and opt are activated by stimulating tissue ventral of the GPi (C). Adjusted from Mai et al. (55).
Hypo-/Bradykinesis: The occurrence of parkinsonian motor signs, such as micrographia and postural deficits, has been described as a possible adverse effect of GPi-DBS in dystonia (294–297). This may be the result of stimulating distinct regions within the GPi: whereas stimulation of the dorsal part of the GPi improves PD signs and symptoms like hypokinesia and rigidity, stimulation of the postero-ventral part suppresses levodopa-induced hyperkinesias but may lead to a deterioration of hypokinesia and gait (284, 298). As a consequence, stimulation-induced hypokinesia is more frequent with use of the ventral contacts and may be significantly reduced by switching to dorsal contacts. Because the ventral contacts are the most effective at controlling dystonic symptoms, this approach may lead to a worsening of dystonia (294, 299, 300).
Speech Impairment: In patients with primary dystonia treated with GPi-DBS, dysarthria is one of the most common stimulation-induced side effects reported in close to 30% in follow-up studies (277, 301). As with STN- or VIMN-DBS, this may be caused by current spreading into the internal capsule medial and posterior to the GPi (Figures 4A–D). In addition, stuttering may occur with GPi stimulation (257, 302), emphasizing the role of the GPi in speech fluency.
Phosphenes: These may be caused by current spread into the optic tract that is located ventral of the GPi (Figures 4A,C).
There is no specific evidence for general programming strategies to avoid speech disturbances in GPi-DBS other than the general strategies for avoiding side effects outlined above.
Conclusion
Programming the IPG is the only modifiable factor once DBS leads have been implanted and thus crucially impacts on the overall treatment success. Although our review does not provide a specific level of evidence for an overall programming strategy, we here summarized appraised strategies on how to adjust stimulation parameters and program settings in different movement disorders. Therefore, we reviewed previous studies that examined the significance of distinct stimulation strategies for ameliorating disease signs. We summarized the well-characterized significance of the stimulation amplitude, frequency and pulse width on clinical symptoms. In addition, we provided an in-depth review of potential side effects in DBS of the STN, VIM, and GPi. Based on these effects, we specifically examined more recent techniques for modulating neuronal elements, such as directional current steering, low-frequency, and short pulse-width stimulation as these strategies were shown to enlarge the therapeutic window and thus allow for a more favorable outcome in different movement disorders. In conjunction with a recommendation for managing pharmacotherapy in PD after initiation of DBS, we thus provide a concise review for STN-, VIM-, and GPi-DBS programming.
Author Contributions
TK conceived the project, conducted literature research, and wrote the paper. CP, FH, JM, and KB wrote the paper.
Funding
This work has been supported by the Lüneburg Heritage (to TK, FH, and CP).
Conflict of Interest Statement
The authors declare that the research was conducted in the absence of any commercial or financial relationships that could be construed as a potential conflict of interest.
References
1. Cooper IS, Upton AR, Amin I. Reversibility of chronic neurologic deficits. Some effects of electrical stimulation of the thalamus and internal capsule in man. Appl Neurophysiol. (1980) 43:244–58.
2. Benabid AL, Pollak P, Gervason C, Hoffmann D, Gao DM, Hommel M, et al. Long-term suppression of tremor by chronic stimulation of the ventral intermediate thalamic nucleus. Lancet. (1991) 337:403–6.
3. Volkmann J, Moro E, Pahwa R. Basic algorithms for the programming of deep brain stimulation in Parkinson's disease. Mov Disord. (2006) 21(Suppl. 14):S284–9. doi: 10.1002/mds.20961
4. Walter BL, Vitek JL. Surgical treatment for Parkinson's disease. Lancet Neurol. (2004) 3:719–28. doi: 10.1016/S1474-4422(04)00934-2
5. Machado A, Rezai AR, Kopell BH, Gross RE, Sharan AD, Benabid A-L. Deep brain stimulation for Parkinson's disease: Surgical technique and perioperative management. Mov Disord. (2006) 21:S247–58. doi: 10.1002/mds.20959
6. Mobin F, De Salles AA, Behnke EJ, Frysinger R. Correlation between MRI-based stereotactic thalamic deep brain stimulation electrode placement, macroelectrode stimulation and clinical response to tremor control. Stereotact Funct Neurosurg. (1999) 72:225–32. doi: 10.1159/000029730
7. Patel NK, Plaha P, O'Sullivan K, McCarter R, Heywood P, Gill SS. MRI directed bilateral stimulation of the subthalamic nucleus in patients with Parkinson's disease. J Neurol Neurosurg Psychiatr. (2003) 74:1631–7. doi: 10.1136/jnnp.74.12.1631
8. Burchiel KJ, McCartney S, Lee A, Raslan AM. Accuracy of deep brain stimulation electrode placement using intraoperative computed tomography without microelectrode recording. J Neurosurg. (2013) 119:301–6. doi: 10.3171/2013.4.JNS122324
9. Langsdorff von D, Paquis P, Fontaine D. In vivo measurement of the frame-based application accuracy of the Neuromate neurosurgical robot. J Neurosurg. (2015) 122:191–4. doi: 10.3171/2014.9.JNS14256
10. Lefranc M, Capel C, Pruvot AS, Fichten A, Desenclos C, Toussaint P, et al. The impact of the reference imaging modality, registration method and intraoperative flat-panel computed tomography on the accuracy of the ROSA® stereotactic robot. Stereotact Funct Neurosurg. (2014) 92:242–50. doi: 10.1159/000362936
11. D'Haese P-F, Pallavaram S, Konrad PE, Neimat J, Fitzpatrick JM, Dawant BM. Clinical accuracy of a customized stereotactic platform for deep brain stimulation after accounting for brain shift. Stereotact Funct Neurosurg. (2010) 88:81–7. doi: 10.1159/000271823
12. Bjartmarz H, Rehncrona S. Comparison of accuracy and precision between frame-based and frameless stereotactic navigation for deep brain stimulation electrode implantation. Stereotact Funct Neurosurg. (2007) 85:235–42. doi: 10.1159/000103262
13. Winkler D. The first evaluation of brain shift during functional neurosurgery by deformation field analysis. J Neurol Neurosurg Psychiatr. (2005) 76:1161–3. doi: 10.1136/jnnp.2004.047373
14. Khan MF, Mewes K, Gross RE, Škrinjar O. Assessment of brain shift related to deep brain stimulation surgery. Stereotact Funct Neurosurg. (2007) 86:44–53. doi: 10.1159/000108588
15. Hunsche S, Sauner D, Maarouf M, Poggenborg JOR, Lackner K, Sturm V, et al. Intraoperative X-ray detection and mri-based quantification of brain shift effects subsequent to implantation of the first electrode in bilateral implantation of deep brain stimulation electrodes. Stereotact Funct Neurosurg. (2009) 87:322–9. doi: 10.1159/000235804
16. Fazl A, Pourfar MH, Mogilner A. Rescue leads for suboptimal results following deep brain stimulation for Parkinson's disease. Brain Stimul. (2017) 10:462. doi: 10.1016/j.brs.2017.01.354
17. Nagy AM, Tolleson CM. Rescue procedures after suboptimal deep brain stimulation outcomes in common movement disorders. Brain Sci. (2016) 6:46. doi: 10.3390/brainsci6040046
18. Guridi J, Rodriguez-Oroz MC, Lozano AM, Moro E, Albanese A, Nuttin B, et al. Targeting the basal ganglia for deep brain stimulation in Parkinson's disease. Neurology. (2000) 55:S21–8.
19. Okun MS, Tagliati M, Pourfar M, Fernandez HH, Rodriguez RL, Alterman RL, et al. Management of referred deep brain stimulation failures: a retrospective analysis from 2 movement disorders centers. Arch Neurol. (2005) 62:1250–5. doi: 10.1001/archneur.62.8.noc40425
20. Rolston JD, Englot DJ, Starr PA, Larson PS. An unexpectedly high rate of revisions and removals in deep brain stimulation surgery: analysis of multiple databases. Parkinsonism Relat Disord. (2016) 33:72–7. doi: 10.1016/j.parkreldis.2016.09.014
21. Kuncel AM, Grill WM. Selection of stimulus parameters for deep brain stimulation. Clin Neurophysiol. (2004) 115:2431–41. doi: 10.1016/j.clinph.2004.05.031
22. Moro E, Poon Y-YW, Lozano AM, Saint-Cyr JA, Lang AE. Subthalamic nucleus stimulation: improvements in outcome with reprogramming. Arch Neurol. (2006) 63:1266–72. doi: 10.1001/archneur.63.9.1266
23. Rizzone M, Lanotte M, Bergamasco B, Tavella A, Torre E, Faccani G, et al. Deep brain stimulation of the subthalamic nucleus in Parkinson's disease: effects of variation in stimulation parameters. J Neurol Neurosurg Psychiatr. (2001) 71:215–9. doi: 10.1136/jnnp.71.2.215
24. Moro E, Esselink RJA, Xie J, Hommel M, Benabid AL, Pollak P. The impact on Parkinson's disease of electrical parameter settings in STN stimulation. Neurology. (2002) 59:706–13.
25. Picillo M, Lozano AM, Kou N, Puppi Munhoz R, Fasano A. Programming deep brain stimulation for Parkinson's disease: the toronto western hospital algorithms. Brain Stimul. (2016) 9:425–37. doi: 10.1016/j.brs.2016.02.004
26. Picillo M, Lozano AM, Kou N, Munhoz RP, Fasano A. Programming deep brain stimulation for tremor and dystonia: the toronto western hospital algorithms. Brain Stimul. (2016) 9:438–52. doi: 10.1016/j.brs.2016.02.003
27. Volkmann J, Herzog J, Kopper F, Deuschl G. Introduction to the programming of deep brain stimulators. Mov Disord. (2002) 17(Suppl. 3):S181–7. doi: 10.1002/mds.10162
28. Hunka K, Suchowersky O, Wood S, Derwent L, Kiss ZHT. Nursing time to program and assess deep brain stimulators in movement disorder patients. J Neurosci Nurs. (2005) 37:204–10. doi: 10.1097/01376517-200508000-00006
29. Amon A, Alesch F. Systems for deep brain stimulation: review of technical features. J Neural Transm. (2017) 124:1083–91. doi: 10.1007/s00702-017-1751-6
30. McIntyre CC, Anderson RW. Deep brain stimulation mechanisms: the control of network activity via neurochemistry modulation. J Neurochem. (2016) 139:338–45. doi: 10.1111/jnc.13649
31. Lozano AM, Lipsman N. Probing and regulating dysfunctional circuits using deep brain stimulation. Neuron. (2013) 77:406–24. doi: 10.1016/j.neuron.2013.01.020
32. Bronstein JM, Tagliati M, Alterman RL, Lozano AM, Volkmann J, Stefani A, et al. Deep brain stimulation for Parkinson disease: an expert consensus and review of key issues. Arch Neurol. (2011) 68:165. doi: 10.1001/archneurol.2010.260
33. Temperli P, Ghika J, Villemure J-G, Burkhard PR, Bogousslavsky J, Vingerhoets FJG. How do parkinsonian signs return after discontinuation of subthalamic DBS? Neurology. (2003) 60:78–81.
34. Hristova A, Lyons K, Troster AI, Pahwa R, Wilkinson SB, Koller WC. Effect and time course of deep brain stimulation of the globus pallidus and subthalamus on motor features of Parkinson's disease. Clin Neuropharmacol. (2000) 23:208–11. doi: 10.1097/00002826-200007000-00007
35. Levin J, Krafczyk S, Valkovic P, Eggert T, Claassen J, Bötzel K. Objective measurement of muscle rigidity in Parkinsonian patients treated with subthalamic stimulation. Mov Disord. (2009) 24:57–63. doi: 10.1002/mds.22291
36. Defer GL, Widner H, Marié RM, Rémy P, Levivier M. Core assessment program for surgical interventional therapies in Parkinson's disease (CAPSIT-PD). Mov Disord. (1999) 572–84.
37. Deuschl G, Herzog J, Kleiner-Fisman G, Kubu C, Lozano AM, Lyons KE, et al. Deep brain stimulation: postoperative issues. Mov Disord. (2006) 21(Suppl. 14):219–37. doi: 10.1002/mds.20957
38. Dembek TA, Reker P, Visser Vandewalle V, Wirths J, Treuer H, Klehr M, et al. Directional DBS increases side-effect thresholds-A prospective, double-blind trial. Mov Disord. (2017) 32:1380–8. doi: 10.1002/mds.27093
39. Pollo C, Kaelin-Lang A, Oertel MF, Stieglitz L, Taub E, Fuhr P, et al. Directional deep brain stimulation: an intraoperative double-blind pilot study. Brain. (2014) 137:2015–26. doi: 10.1093/brain/awu102
40. Reker P, Dembek TA, Becker J, Visser Vandewalle V, Timmermann L. Directional deep brain stimulation: a case of avoiding dysarthria with bipolar directional current steering. Parkinsonism Relat Disord. (2016) 31:156–8. doi: 10.1016/j.parkreldis.2016.08.007
41. Contarino MF, Bour LJ, Verhagen R, Lourens MAJ, de Bie RMA, van den Munckhof P, et al. Directional steering: a novel approach to deep brain stimulation. Neurology. (2014) 83:1163–9. doi: 10.1212/WNL.0000000000000823
42. Volkmann J, Chabardes S, Steinke GK, Carcieri S. 375 DIRECT DBS: a prospective, multicenter clinical trial with blinding for a directional deep brain stimulation lead. Neurosurgery. (2016) 63(Suppl. 1):211–2. doi: 10.1227/01.neu.0000489863.00935.ea
43. Schüpbach WMM, Chabardes S, Matthies C, Pollo C, Steigerwald F, Timmermann L, et al. Directional leads for deep brain stimulation: opportunities and challenges. Mov Disord. (2017) 32:1371–5. doi: 10.1002/mds.27096
44. Rebelo P, Green AL, Aziz TZ, Kent A, Schafer D, Venkatesan L, et al. Thalamic directional deep brain stimulation for tremor: spend less, get more. Brain Stimul. (2018) 11:600–6. doi: 10.1016/j.brs.2017.12.015
45. Timmermann L, Jain R, Chen L, Maarouf M, Barbe MT, Allert N, et al. Multiple-source current steering in subthalamic nucleus deep brain stimulation for Parkinson's disease (the VANTAGE study): a non-randomised, prospective, multicentre, open-label study. Lancet Neurol. (2015) 14:693–701. doi: 10.1016/S1474-4422(15)00087-3
46. Sauleau P, Raoul S, Lallement F, Rivier I, Drapier S, Lajat Y, et al. Motor and non motor effects during intraoperative subthalamic stimulation for Parkinson's disease. J Neurol. (2005) 252:457–64. doi: 10.1007/s00415-005-0675-5
47. Miocinovic S, Khemani P, Whiddon R, Zeilman P, Martinez-Ramirez D, et al. Outcomes, management, and potential mechanisms of interleaving deep brain stimulation settings. Parkinsonism Relat Disord. (2014) 20:1434–7. doi: 10.1016/j.parkreldis.2014.10.011
48. Timmermann L, Wojtecki L, Gross J, Lehrke R, Voges J, Maarouf M, et al. Ten-Hertz stimulation of subthalamic nucleus deteriorates motor symptoms in Parkinson's disease. Mov Disord. (2004) 19:1328–33. doi: 10.1002/mds.20198
49. Eusebio A, Chen CC, Lu CS, Lee ST, Tsai CH, Limousin P, et al. Effects of low-frequency stimulation of the subthalamic nucleus on movement in Parkinson's disease. Exper Neurol. (2008) 209:125–30. doi: 10.1016/j.expneurol.2007.09.007
50. Limousin P, Pollak P, Benazzouz A, Hoffmann D, Broussolle E, Perret JE, et al. Bilateral subthalamic nucleus stimulation for severe Parkinson's disease. Mov Disord. (1995) 10:672–4. doi: 10.1002/mds.870100523
51. Limousin P, Krack P, Pollak P, Benazzouz A, Ardouin C, Hoffmann D, et al. Electrical stimulation of the subthalamic nucleus in advanced Parkinson's disease. N Engl J Med. (1998) 339:1105–11. doi: 10.1056/NEJM199810153391603
52. Krack P, Pollak P, Limousin P, Benazzouz A, Deuschl G, Benabid AL. From off-period dystonia to peak-dose chorea. The clinical spectrum of varying subthalamic nucleus activity. Brain. (1999) 122(Pt 6):1133–46.
53. Steigerwald F, Timmermann L, Kuhn A, Schnitzler A, Reich MM, Kirsch AD, et al. Pulse duration settings in subthalamic stimulation for Parkinson's disease. Mov Disord. (2017) 33:165–9. doi: 10.1002/mds.27238
54. Dayal V, Grover T, Limousin P, Akram H, Cappon D, Candelario J, et al. The effect of short pulse width settings on the therapeutic window in subthalamic nucleus deep brain stimulation for Parkinson's disease. J Parkinsons Dis. (2018) 8:273–9. doi: 10.3233/JPD-171272
56. Güngör A, Baydin SS, Holanda VM, Middlebrooks EH, Isler C, Tugcu B, et al. Microsurgical anatomy of the subthalamic nucleus: correlating fiber dissection results with 3-T magnetic resonance imaging using neuronavigation. J Neurosurg. (2018) 8:1–17. doi: 10.3171/2017.10.JNS171513
57. Benarroch EE. Subthalamic nucleus and its connections: anatomic substrate for the network effects of deep brain stimulation. Neurology. (2008) 70:1991–5. doi: 10.1212/01.wnl.0000313022.39329.65
58. Herzog J, Fietzek U, Hamel W, Morsnowski A, Steigerwald F, Schrader B, et al. Most effective stimulation site in subthalamic deep brain stimulation for Parkinson's disease. Mov Disord. (2004) 19:1050–4. doi: 10.1002/mds.20056
59. Lanotte MM, Rizzone M, Bergamasco B, Faccani G, Melcarne A, Lopiano L. Deep brain stimulation of the subthalamic nucleus: anatomical, neurophysiological, and outcome correlations with the effects of stimulation. J Neurol Neurosurg Psychiatr. (2002) 72:53–8. doi: 10.1136/jnnp.72.1.53
60. Saint-Cyr JA, Hoque T, Pereira LCM, Dostrovsky JO, Hutchison WD, Mikulis DJ, et al. Localization of clinically effective stimulating electrodes in the human subthalamic nucleus on magnetic resonance imaging. J Neurosurg. (2002) 97:1152–66. doi: 10.3171/jns.2002.97.5.1152
61. Zheng Z, Zhang Y-Q, Li J-Y, Zhang X-H, Zhuang P, Li Y-J. Subthalamic deep brain stimulation for Parkinson's disease: correlation of active contacts and electrophysiologically mapped subthalamic nucleus. Chin Med J. (2009) 122:2419–22.
62. Zonenshayn M, Sterio D, Kelly PJ, Rezai AR, Beric A. Location of the active contact within the subthalamic nucleus (STN) in the treatment of idiopathic Parkinson's disease. Surg Neurol. (2004) 62:216–25; discussion: 225–6. doi: 10.1016/j.surneu.2003.09.039
63. Garcia-Garcia D, Guridi J, Toledo JB, Alegre M, Obeso JA, Rodriguez-Oroz MC. Stimulation sites in the subthalamic nucleus and clinical improvement in Parkinson's disease: a new approach for active contact localization. J Neurosurg. (2016) 125:1068–79. doi: 10.3171/2015.9.JNS15868
64. Wodarg F, Herzog J, Reese R, Falk D, Pinsker MO, Steigerwald F, et al. Stimulation site within the MRI-defined STN predicts postoperative motor outcome. Mov Disord. (2012) 27:874–79. doi: 10.1002/mds.25006
65. Coenen VA, Honey CR, Hurwitz T, Rahman AA, McMaster J, Bürgel U, et al. Medial forebrain bundle stimulation as a pathophysiological mechanism for hypomania in subthalamic nucleus deep brain stimulation for Parkinson's disease. Neurosurgery. (2009) 64:1106–14; discussion: 1114–5. doi: 10.1227/01.NEU.0000345631.54446.06
66. Krack P, Kumar R, Ardouin C, Dowsey PL, McVicker JM, Benabid AL, et al. Mirthful laughter induced by subthalamic nucleus stimulation. Mov Disord. (2001) 16:867–75. doi: 10.1002/mds.1174
67. Voon V, Krack P, Lang AE, Lozano AM, Dujardin K, Schüpbach M, et al. A multicentre study on suicide outcomes following subthalamic stimulation for Parkinson's disease. Brain. (2008) 131:2720–8. doi: 10.1093/brain/awn214
68. Herzog J, Reiff J, Krack P, Witt K, Schrader B, Müller D, et al. Manic episode with psychotic symptoms induced by subthalamic nucleus stimulation in a patient with Parkinson's disease. Mov Disord. (2003) 18:1382–4. doi: 10.1002/mds.10530
69. Eisenstein SA, Koller JM, Black KD, Campbell MC, Lugar HM, Ushe M, et al. Functional anatomy of subthalamic nucleus stimulation in Parkinson disease. Ann Neurol. (2014) 76:279–95. doi: 10.1002/ana.24204
70. Voges J, Volkmann J, Allert N, Lehrke R, Koulousakis A, Freund H-J, et al. Bilateral high-frequency stimulation in the subthalamic nucleus for the treatment of Parkinson disease: correlation of therapeutic effect with anatomical electrode position. J Neurosurg. (2002) 96:269–79. doi: 10.3171/jns.2002.96.2.0269
71. Godinho F, Thobois S, Magnin M, Guenot M, Polo G, Benatru I, et al. Subthalamic nucleus stimulation in Parkinson's disease : anatomical and electrophysiological localization of active contacts. J Neurol. (2006) 253:1347–55. doi: 10.1007/s00415-006-0222-z
72. Hamel W, Fietzek U, Morsnowski A, Schrader B, Herzog J, Weinert D, et al. Deep brain stimulation of the subthalamic nucleus in Parkinson's disease: evaluation of active electrode contacts. J Neurol Neurosurg Psychiatr. (2003) 74:1036–46. doi: 10.1136/jnnp.74.8.1036
73. Johnsen EL, Sunde N, Mogensen PH, Ostergaard K. MRI verified STN stimulation site–gait improvement and clinical outcome. Eur J Neurol. (2010) 17:746–53. doi: 10.1111/j.1468-1331.2010.02962.x
74. Plaha P, Ben-Shlomo Y, Patel NK, Gill SS. Stimulation of the caudal zona incerta is superior to stimulation of the subthalamic nucleus in improving contralateral parkinsonism. Brain. (2006) 129:1732–47. doi: 10.1093/brain/awl127
75. Vergani F, Landi A, Antonini A, Parolin M, Cilia R, Grimaldi M, et al. Anatomical identification of active contacts in subthalamic deep brain stimulation. Surg Neurol. (2007) 67:140–6; discussion: 146–7. doi: 10.1016/j.surneu.2006.06.054
76. Yokoyama T, Sugiyama K, Nishizawa S, Yokota N, Ohta S, Akamine S, et al. The optimal stimulation site for chronic stimulation of the subthalamic nucleus in Parkinson's disease. Stereotact Funct Neurosurg. (2001) 77:61–7. doi: 10.1159/000064598
77. Weise LM, Seifried C, Eibach S, Gasser T, Roeper J, Seifert V, et al. Correlation of active contact positions with the electrophysiological and anatomical subdivisions of the subthalamic nucleus in deep brain stimulation. Stereotact Funct Neurosurg. (2013) 91:298–305. doi: 10.1159/000345259
78. Caire F, Ranoux D, Guehl D, Burbaud P, Cuny E. A systematic review of studies on anatomical position of electrode contacts used for chronic subthalamic stimulation in Parkinson's disease. Acta Neurochir. (2013) 155:1647–54; discussion: 1654. doi: 10.1007/s00701-013-1782-1
79. Tommasi G, Krack P, Fraix V, Le Bas J-F, Chabardes S, Benabid AL, et al. Pyramidal tract side effects induced by deep brain stimulation of the subthalamic nucleus. J Neurol Neurosurg Psychiatr. (2008) 79:813–9. doi: 10.1136/jnnp.2007.117507
80. Gorgulho AA, Shields DC, Malkasian D, Behnke E, Desalles AAF. Stereotactic coordinates associated with facial musculature contraction during high-frequency stimulation of the subthalamic nucleus. J Neurosurg. (2009) 110:1317–21. doi: 10.3171/JNS.2008.10.JNS08835
81. McIntyre CC, Mori S, Sherman DL, Thakor NV, Vitek JL. Electric field and stimulating influence generated by deep brain stimulation of the subthalamic nucleus. Clin Neurophysiol. (2004) 115:589–95. doi: 10.1016/j.clinph.2003.10.033
82. Leichnetz GR. The prefrontal cortico-oculomotor trajectories in the monkey. J Neurol Sci. (1981) 49:387–96.
83. Shields DC, Gorgulho A, Behnke E, Malkasian D, Desalles AAF. Contralateral conjugate eye deviation during deep brain stimulation of the subthalamic nucleus. J Neurosurg. (2007) 107:37–42. doi: 10.3171/JNS-07/07/0037
84. Sauleau P, Pollak P, Krack P, Courjon J-H, Vighetto A, Benabid A-L, et al. Subthalamic stimulation improves orienting gaze movements in Parkinson's disease. Clin Neurophysiol. (2008) 119:1857–63. doi: 10.1016/j.clinph.2008.04.013
85. Tamma F, Caputo E, Chiesa V, Egidi M, Locatelli M, Rampini P, et al. Anatomo-clinical correlation of intraoperative stimulation-induced side-effects during HF-DBS of the subthalamic nucleus. Neurol Sci. (2002) 23(Suppl. 2):S109–10. doi: 10.1007/s100720200093
86. Törnqvist G. Effect of oculomotor nerve stimulation on outflow facility and pupil diameter in a monkey (Cercopithecus ethiops). Invest Ophthalmol. (1970) 9:220–5.
87. Tommasi G, Krack P, Fraix V, Pollak P. Effects of varying subthalamic nucleus stimulation on apraxia of lid opening in Parkinson's disease. J Neurol. (2012) 259:1944–50. doi: 10.1007/s00415-012-6447-0
88. Pollak P, Krack P, Fraix V, Mendes A, Moro E, Chabardes S, et al. Intraoperative micro- and macrostimulation of the subthalamic nucleus in Parkinson's disease. Mov Disord. (2002) 17(Suppl. 3):S155–61. doi: 10.1002/mds.10158
89. Krack P, Fraix V, Mendes A, Benabid A-L, Pollak P. Postoperative management of subthalamic nucleus stimulation for Parkinson's disease. Mov Disord. (2002) 17(Suppl. 3):S188–97. doi: 10.1002/mds.10163
90. Piboolnurak P, Lang AE, Lozano AM, Miyasaki JM, Saint-Cyr JA, Poon Y-YW, et al. Levodopa response in long-term bilateral subthalamic stimulation for Parkinson's disease. Mov Disord. (2007) 22:990–7. doi: 10.1002/mds.21482
91. Farris S, Giroux M. Retrospective review of factors leading to dissatisfaction with subthalamic nucleus deep brain stimulation during long-term management. Surg Neurol Int. (2013) 4:69. doi: 10.4103/2152-7806.112612
92. Tsuboi T, Watanabe H, Tanaka Y, Ohdake R, Yoneyama N, Hara K, et al. Distinct phenotypes of speech and voice disorders in Parkinson's disease after subthalamic nucleus deep brain stimulation. J Neurol Neurosurg Psychiatr. (2015) 86:856–64. doi: 10.1136/jnnp-2014-308043
93. Åström M, Tripoliti E, Hariz MI, Zrinzo LU, Martinez-Torres I, Limousin P, et al. Patient-specific model-based investigation of speech intelligibility and movement during deep brain stimulation. Stereotact Funct Neurosurg. (2010) 88:224–33. doi: 10.1159/000314357
94. Tripoliti E, Zrinzo L, Martinez-Torres I, Tisch S, Frost E, Borrell E, et al. Effects of contact location and voltage amplitude on speech and movement in bilateral subthalamic nucleus deep brain stimulation. Mov Disord. (2008) 23:2377–83. doi: 10.1002/mds.22296
95. Skodda S. Effect of deep brain stimulation on speech performance in Parkinson's disease. Parkinsons Dis. (2012) 2012:850596–10. doi: 10.1155/2012/850596
96. Wang E, Verhagen Metman L, Bakay R, Arzbaecher J, Bernard B. The effect of unilateral electrostimulation of the subthalamic nucleus on respiratory/phonatory subsystems of speech production in Parkinson's disease–a preliminary report. Clin Linguist Phon. (2003) 17:283–9. doi: 10.1080/0269920031000080064
97. Santens P, De Letter M, Van Borsel J, De Reuck J, Caemaert J. Lateralized effects of subthalamic nucleus stimulation on different aspects of speech in Parkinson's disease. Brain Lang. (2003) 87:253–8. doi: 10.1016/S0093-934X(03)00142-1
98. Tripoliti E, Zrinzo L, Martinez-Torres I, Frost E, Pinto S, Foltynie T, et al. Effects of subthalamic stimulation on speech of consecutive patients with Parkinson disease. Neurology. (2011) 76:80–6. doi: 10.1212/WNL.0b013e318203e7d0
99. Stefani A, Lozano AM, Peppe A, Stanzione P, Galati S, Tropepi D, et al. Bilateral deep brain stimulation of the pedunculopontine and subthalamic nuclei in severe Parkinson's disease. Brain. (2007) 130:1596–607. doi: 10.1093/brain/awl346
100. Chastan N, Westby GWM, Yelnik J, Bardinet E, Do MC, Agid Y, et al. Effects of nigral stimulation on locomotion and postural stability in patients with Parkinson's disease. Brain. (2009) 132:172–84. doi: 10.1093/brain/awn294
101. Weiss D, Walach M, Meisner C, Fritz M, Scholten M, Breit S, et al. Nigral stimulation for resistant axial motor impairment in Parkinson's disease? A randomized controlled trial. Brain. (2013) 136:2098–108. doi: 10.1093/brain/awt122
102. Törnqvist AL, Schalén L, Rehncrona S. Effects of different electrical parameter settings on the intelligibility of speech in patients with Parkinson's disease treated with subthalamic deep brain stimulation. Mov Disord. (2005) 20:416–23. doi: 10.1002/mds.20348
103. Hammer MJ, Barlow SM, Lyons KE, Pahwa R. Subthalamic nucleus deep brain stimulation changes velopharyngeal control in Parkinson's disease. J Commun Disord. (2011) 44:37–48. doi: 10.1016/j.jcomdis.2010.07.001
104. Benabid AL, Benazzouz A, Limousin P, Koudsie A, Krack P, Piallat B, et al. Dyskinesias and the subthalamic nucleus. Ann Neurol. (2000) 47:S189–92.
105. Limousin P, Pollak P, Hoffmann D, Benazzouz A, Perret JE, Benabid AL. Abnormal involuntary movements induced by subthalamic nucleus stimulation in parkinsonian patients. Mov Disord. (1996) 11:231–5. doi: 10.1002/mds.870110303
106. Houeto J-L, Welter M-L, Bejjani P-B, Tezenas du Montcel S, Bonnet A-M, Mesnage V, et al. Subthalamic stimulation in Parkinson disease: intraoperative predictive factors. Arch Neurol. (2003) 60:690–4. doi: 10.1001/archneur.60.5.690
107. Fagbami OY, Donato AA. Stridor and dysphagia associated with subthalamic nucleus stimulation in Parkinson disease. J Neurosurg. (2011) 115:1005–6. doi: 10.3171/2011.7.JNS11602
108. Yanase M, Kataoka H, Kawahara M, Hirabayashi H, Yamanaka T, Hirano M, et al. Fixed epiglottis associated with subthalamic nucleus stimulation in Parkinson's disease. J Neurol Neurosurg Psychiatr. (2008) 79:332–3. doi: 10.1136/jnnp.2007.133280
109. Follett KA, Weaver FM, Stern M, Hur K, Harris CL, Luo P, et al. Pallidal versus subthalamic deep-brain stimulation for Parkinson's disease. N Engl J Med. (2010) 362:2077–91. doi: 10.1056/NEJMoa0907083
110. Odekerken VJJ, van Laar T, Staal MJ, Mosch A, Hoffmann CFE, Nijssen PCG, et al. Subthalamic nucleus versus globus pallidus bilateral deep brain stimulation for advanced Parkinson's disease (NSTAPS study): a randomised controlled trial. Lancet Neurol. (2013) 12:37–44. doi: 10.1016/S1474-4422(12)70264-8
111. Anderson VC, Burchiel KJ, Hogarth P, Favre J, Hammerstad JP. Pallidal vs subthalamic nucleus deep brain stimulation in Parkinson disease. Arch Neurol. (2005) 62:554–60. doi: 10.1001/archneur.62.4.554
112. Witt K, Daniels C, Reiff J, Krack P, Volkmann J, Pinsker MO, et al. Neuropsychological and psychiatric changes after deep brain stimulation for Parkinson's disease: a randomised, multicentre study. Lancet Neurol. (2008) 7:605–14. doi: 10.1016/S1474-4422(08)70114-5
113. Zangaglia R, Pacchetti C, Pasotti C, Mancini F, Servello D, Sinforiani E, et al. Deep brain stimulation and cognitive functions in Parkinson's disease: a three-year controlled study. Mov Disord. (2009) 24:1621–8. doi: 10.1002/mds.22603
114. Williams A, Gill S, Varma T, Jenkinson C, Quinn N, Mitchell R, et al. Deep brain stimulation plus best medical therapy versus best medical therapy alone for advanced Parkinson's disease (PD SURG trial): a randomised, open-label trial. Lancet Neurol. (2010) 9:581–91. doi: 10.1016/S1474-4422(10)70093-4
115. Okun MS, Gallo BV, Mandybur G, Jagid J, Foote KD, Revilla FJ, et al. Subthalamic deep brain stimulation with a constant-current device in Parkinson's disease: an open-label randomised controlled trial. Lancet Neurol. (2012) 11:140–9. doi: 10.1016/S1474-4422(11)70308-8
116. Robertson LT, Horak FB, Anderson VC, Burchiel KJ, Hammerstad JP. Assessments of axial motor control during deep brain stimulation in parkinsonian patients. Neurosurgery. (2001) 48:544–51; discussion: 551–2. doi: 10.1097/00006123-200103000-00017
117. Rizzone MG, Fasano A, Daniele A, Zibetti M, Merola A, Rizzi L, et al. Long-term outcome of subthalamic nucleus DBS in Parkinson's disease: from the advanced phase towards the late stage of the disease? Parkinsonism Relat Disord. (2014) 20:376–81. doi: 10.1016/j.parkreldis.2014.01.012
118. St George RJ, Nutt JG, Burchiel KJ, Horak FB. A meta-regression of the long-term effects of deep brain stimulation on balance and gait in PD. Neurology. (2010) 75:1292–9. doi: 10.1212/WNL.0b013e3181f61329
119. Hausdorff JM, Gruendlinger L, Scollins L, O'Herron S, Tarsy D. Deep brain stimulation effects on gait variability in Parkinson's disease. Mov Disord. (2009) 24:1688–92. doi: 10.1002/mds.22554
120. Stolze H, Klebe S, Poepping M, Lorenz D, Herzog J, Hamel W, et al. Effects of bilateral subthalamic nucleus stimulation on parkinsonian gait. Neurology. (2001) 57:144–6. doi: 10.1007/s00415-005-0896-7
121. Hamani C, Richter E, Schwalb JM, Lozano AM. Bilateral subthalamic nucleus stimulation for Parkinson's disease: a systematic review of the clinical literature. Neurosurgery. (2008) 62(Suppl. 2):863–74. doi: 10.1227/01.neu.0000316288.75736.1c
122. St George RJ, Carlson-Kuhta P, Nutt JG, Hogarth P, Burchiel KJ, Horak FB. The effect of deep brain stimulation randomized by site on balance in Parkinson's disease. Mov Disord. (2014) 29:949–53. doi: 10.1002/mds.25831
123. Fasano A, Aquino CC, Krauss JK, Honey CR, Bloem BR. Axial disability and deep brain stimulation in patients with Parkinson disease. Nat Rev Neurol. (2015) 11:98–110. doi: 10.1038/nrneurol.2014.252
124. Isler C, Albi A, Schaper FLWVJ, Temel Y, Duits A. Neuropsychological outcome in subthalamic nucleus stimulation surgeries with electrodes passing through the caudate nucleus. Stereotact Funct Neurosurg. (2016) 94:413–20. doi: 10.1159/000453278
125. Morishita T, Okun MS, Jones JD, Foote KD, Bowers D. Cognitive declines after deep brain stimulation are likely to be attributable to more than caudate penetration and lead location. Brain. (2014) 137:e274. doi: 10.1093/brain/awu008
126. McIntyre CC, Richardson SJ, Frankemolle AM, Varga G, Noecker AM, Alberts JL. Improving postural stability via computational modeling approach to deep brain stimulation programming. Conf Proc IEEE Eng Med Biol Soc. (2011) 2011:675–6. doi: 10.1109/IEMBS.2011.6090151
127. Funkiewiez A, Ardouin C, Caputo E, Krack P, Fraix V, Klinger H, et al. Long term effects of bilateral subthalamic nucleus stimulation on cognitive function, mood, and behaviour in Parkinson's disease. J Neurol Neurosurg Psychiatr. (2004) 75:834–9. doi: 10.1136/jnnp.2002.009803
128. Ulla M, Thobois S, Lemaire J-J, Schmitt A, Derost P, Broussolle E, et al. Manic behaviour induced by deep-brain stimulation in Parkinson's disease: evidence of substantia nigra implication? J Neurol Neurosurg Psychiatr. (2006) 77:1363–6. doi: 10.1136/jnnp.2006.096628
129. Simmons DB, Dashtipour K. The quantitative measurement of reversible acute depression after subthalamic deep brain stimulation in a patient with Parkinson disease. Case Rep Neurol Med. (2015) 2015:165846–5. doi: 10.1155/2015/165846
130. Tommasi G, Lanotte M, Albert U, Zibetti M, Castelli L, Maina G, et al. Transient acute depressive state induced by subthalamic region stimulation. J Neurol Sci. (2008) 273:135–8. doi: 10.1016/j.jns.2008.06.012
131. Bejjani BP, Damier P, Arnulf I, Thivard L, Bonnet AM, Dormont D, et al. Transient acute depression induced by high-frequency deep-brain stimulation. N Engl J Med. (1999) 340:1476–80. doi: 10.1056/NEJM199905133401905
132. Fasano A, Appel-Cresswell S, Jog M, Zurowkski M, Duff-Canning S, Cohn M, et al. Medical management of Parkinson's disease after initiation of deep brain stimulation. Can J Neurol Sci. (2016) 43:626–34. doi: 10.1017/cjn.2016.274
133. Maier F, Lewis CJ, Horstkoetter N, Eggers C, Kalbe E, Maarouf M, et al. Patients' expectations of deep brain stimulation, and subjective perceived outcome related to clinical measures in Parkinson's disease: a mixed-method approach. J Neurol Neurosurg Psychiatr. (2013) 84:1273–81. doi: 10.1136/jnnp-2012-303670
134. Tröster AI, Pahwa R, Fields JA, Tanner CM, Lyons KE. Quality of life in Essential Tremor Questionnaire (QUEST): development and initial validation. Parkinsonism Relat Disord. (2005) 11:367–73. doi: 10.1016/j.parkreldis.2005.05.009
135. Chopra A, Tye SJ, Lee KH, Sampson S, Matsumoto J, Adams A, et al. Underlying neurobiology and clinical correlates of mania status after subthalamic nucleus deep brain stimulation in Parkinson's disease: a review of the literature. J Neuropsychiatry Clin Neurosci. (2012) 24:102–10. doi: 10.1176/appi.neuropsych.10070109
136. Greenhouse I, Gould S, Houser M, Hicks G, Gross J, Aron AR. Stimulation at dorsal and ventral electrode contacts targeted at the subthalamic nucleus has different effects on motor and emotion functions in Parkinson's disease. Neuropsychologia. (2011) 49:528–34. doi: 10.1016/j.neuropsychologia.2010.12.030
137. Broen M, Duits A, Visser Vandewalle V, Temel Y, Winogrodzka A. Impulse control and related disorders in Parkinson's disease patients treated with bilateral subthalamic nucleus stimulation: a review. Parkinsonism Relat Disord. (2011) 17:413–7. doi: 10.1016/j.parkreldis.2011.02.013
138. Ballanger B, van Eimeren T, Moro E, Lozano AM, Hamani C, Boulinguez P, et al. Stimulation of the subthalamic nucleus and impulsivity: release your horses. Ann Neurol. (2009) 66:817–24. doi: 10.1002/ana.21795
139. Boller JK, Barbe MT, Pauls KAM, Reck C, Brand M, Maier F, et al. Decision-making under risk is improved by both dopaminergic medication and subthalamic stimulation in Parkinson's disease. Exper Neurol. (2014) 254:70–7. doi: 10.1016/j.expneurol.2014.01.005
140. Brandt J, Rogerson M, Al-Joudi H, Reckess G, Shpritz B, Umeh CC, et al. Betting on DBS: Effects of subthalamic nucleus deep brain stimulation on risk taking and decision making in patients with Parkinson's disease. Neuropsychology. (2015) 29:622–31. doi: 10.1037/neu0000164
141. Amami P, Dekker I, Piacentini S, Ferré F, Romito LM, Franzini A, et al. Impulse control behaviours in patients with Parkinson's disease after subthalamic deep brain stimulation: de novo cases and 3-year follow-up. J Neurol Neurosurg Psychiatr. (2015) 86:562–4. doi: 10.1136/jnnp-2013-307214
142. Pallanti S, Bernardi S, Raglione LM, Marini P, Ammannati F, Sorbi S, et al. Complex repetitive behavior: punding after bilateral subthalamic nucleus stimulation in Parkinson's disease. Parkinsonism Relat Disord. (2010) 16:376–80. doi: 10.1016/j.parkreldis.2010.02.011
143. Hälbig TD, Tse W, Frisina PG, Baker BR, Hollander E, Shapiro H, et al. Subthalamic deep brain stimulation and impulse control in Parkinson's disease. Eur J Neurol. (2009) 16:493–7. doi: 10.1111/j.1468-1331.2008.02509.x
144. Doshi P, Bhargava P. Hypersexuality following subthalamic nucleus stimulation for Parkinson's disease. Neurol India. (2008) 56:474–6. doi: 10.4103/0028-3886.44830
145. Ulla M, Thobois S, Llorca P-M, Derost P, Lemaire J-J, Chereau-Boudet I, et al. Contact dependent reproducible hypomania induced by deep brain stimulation in Parkinson's disease: clinical, anatomical and functional imaging study. J Neurol Neurosurg Psychiatr. (2011) 82:607–14. doi: 10.1136/jnnp.2009.199323
146. Smeding HMM, Goudriaan AE, Foncke EMJ, Schuurman PR, Speelman JD, Schmand B. Pathological gambling after bilateral subthalamic nucleus stimulation in Parkinson disease. J Neurol Neurosurg Psychiatr. (2007) 78:517–9. doi: 10.1136/jnnp.2006.102061
147. Funkiewiez A, Ardouin C, Krack P, Fraix V, Van Blercom N, Xie J, et al. Acute psychotropic effects of bilateral subthalamic nucleus stimulation and levodopa in Parkinson's disease. Mov Disord. (2003) 18:524–30. doi: 10.1002/mds.10441
148. Romito LM, Raja M, Daniele A, Contarino MF, Bentivoglio AR, Barbier A, et al. Transient mania with hypersexuality after surgery for high frequency stimulation of the subthalamic nucleus in Parkinson's disease. Mov Disord. (2002) 17:1371–4. doi: 10.1002/mds.10265
149. Sensi M, Eleopra R, Cavallo MA, Sette E, Milani P, Quatrale R, et al. Explosive-aggressive behavior related to bilateral subthalamic stimulation. Parkinsonism Relat Disord. (2004) 10:247–51. doi: 10.1016/j.parkreldis.2004.01.007
150. Castrioto A, Lhommée E, Moro E, Krack P. Mood and behavioural effects of subthalamic stimulation in Parkinson's disease. Lancet Neurol. (2014) 13:287–305. doi: 10.1016/S1474-4422(13)70294-1
151. Mallet L, Schüpbach M, N'Diaye K, Remy P, Bardinet E, Czernecki V, et al. Stimulation of subterritories of the subthalamic nucleus reveals its role in the integration of the emotional and motor aspects of behavior. Proc Natl Acad Sci USA. (2007) 104:10661–6. doi: 10.1073/pnas.0610849104
152. Lhommée E, Klinger H, Thobois S, Schmitt E, Ardouin C, Bichon A, et al. Subthalamic stimulation in Parkinson's disease: restoring the balance of motivated behaviours. Brain. (2012) 135:1463–77. doi: 10.1093/brain/aws078
153. Eusebio A, Witjas T, Cohen J, Fluchère F, Jouve E, Regis J, et al. Subthalamic nucleus stimulation and compulsive use of dopaminergic medication in Parkinson's disease. J Neurol Neurosurg Psychiatr. (2013) 84:868–74. doi: 10.1136/jnnp-2012-302387
154. Cilia R, Siri C, Canesi M, Zecchinelli AL, De Gaspari D, Natuzzi F, et al. Dopamine dysregulation syndrome in Parkinson's disease: from clinical and neuropsychological characterisation to management and long-term outcome. J Neurol Neurosurg Psychiatr. (2014) 85:311–8. doi: 10.1136/jnnp-2012-303988
155. Angeli A, Mencacci NE, Duran R, Aviles-Olmos I, Kefalopoulou Z, Candelario J, et al. Genotype and phenotype in Parkinson's disease: lessons in heterogeneity from deep brain stimulation. Mov Disord. (2013) 28:1370–5. doi: 10.1002/mds.25535
156. Contarino MF, Daniele A, Sibilia AH, Romito LMA, Bentivoglio AR, Gainotti G, et al. Cognitive outcome 5 years after bilateral chronic stimulation of subthalamic nucleus in patients with Parkinson's disease. J Neurol Neurosurg Psychiatr. (2007) 78:248–52. doi: 10.1136/jnnp.2005.086660
157. Witt K, Granert O, Daniels C, Volkmann J, Falk D, van Eimeren T, et al. Relation of lead trajectory and electrode position to neuropsychological outcomes of subthalamic neurostimulation in Parkinson's disease: results from a randomized trial. Brain. (2013) 136:2109–19. doi: 10.1093/brain/awt151
158. Okun MS, Fernandez HH, Wu SS, Kirsch-Darrow L, Bowers D, Bova F, et al. Cognition and mood in Parkinson's disease in subthalamic nucleus versus globus pallidus interna deep brain stimulation: the COMPARE trial. Ann Neurol. (2009) 65:586–95. doi: 10.1002/ana.21596
159. Fasano A, Romito LM, Daniele A, Piano C, Zinno M, Bentivoglio AR, et al. Motor and cognitive outcome in patients with Parkinson's disease 8 years after subthalamic implants. Brain. (2010) 133:2664–76. doi: 10.1093/brain/awq221
160. Parsons TD, Rogers SA, Braaten AJ, Woods SP, Tröster AI. Cognitive sequelae of subthalamic nucleus deep brain stimulation in Parkinson's disease: a meta-analysis. Lancet Neurol. (2006) 5:578–88. doi: 10.1016/S1474-4422(06)70475-6
161. Haegelen C, García-Lorenzo D, Le Jeune F, Péron J, Gibaud B, Riffaud L, et al. SPECT and PET analysis of subthalamic stimulation in Parkinson's disease: analysis using a manual segmentation. J Neurol. (2010) 257:375–82. doi: 10.1007/s00415-009-5327-8
162. Kalbe E, Calabrese P, Kohn N, Hilker R, Riedel O, Wittchen H-U, et al. Screening for cognitive deficits in Parkinson's disease with the Parkinson neuropsychometric dementia assessment (PANDA) instrument. Parkinsonism Relat Disord. (2008) 14:93–101. doi: 10.1016/j.parkreldis.2007.06.008
163. Weaver FM, Follett KA, Stern M, Luo P, Harris CL, Hur K, et al. Randomized trial of deep brain stimulation for Parkinson disease: thirty-six-month outcomes. Neurology. (2012) 79:55–65. doi: 10.1212/WNL.0b013e31825dcdc1
164. Volkmann J, Daniels C, Witt K. Neuropsychiatric effects of subthalamic neurostimulation in Parkinson disease. Nat Rev Neurol. (2010) 6:487–98. doi: 10.1038/nrneurol.2010.111
165. Volkmann J, Fogel W, Krack P. Postoperatives neurologisches management bei stimulation des nucleus subthalamicus. Aktuelle Neurologie. (2008) 27:S23–39. doi: 10.1055/s-2007-1017592
166. Fasano A, Herzog J, Seifert E, Stolze H, Falk D, Reese R, et al. Modulation of gait coordination by subthalamic stimulation improves freezing of gait. Mov Disord. (2011) 26:844–51. doi: 10.1002/mds.23583
167. Eitan R, Shamir RR, Linetsky E, Rosenbluh O, Moshel S, Ben-Hur T, et al. Asymmetric right/left encoding of emotions in the human subthalamic nucleus. Front Syst Neurosci. (2013) 7:69. doi: 10.3389/fnsys.2013.00069
168. Grant PF, Lowery MM. Effect of dispersive conductivity and permittivity in volume conductor models of deep brain stimulation. IEEE Trans Bio Med Eng. (2010) 57:2386–93. doi: 10.1109/TBME.2010.2055054
169. Gabriel S, Lau RW, Gabriel C. The dielectric properties of biological tissues: III. Parametric models for the dielectric spectrum of tissues. Phys Med Biol. (1996) 41:2271–93.
170. Foster KR, Schwan HP. Dielectric properties of tissues and biological materials: a critical review. Crit Rev Biomed Eng. (1989) 17:25–104.
171. Latikka J, Kuurne T, Eskola H. Conductivity of living intracranial tissues. Phys Med Biol. (2001) 46:1611–6. doi: 10.1088/0031-9155/46/6/302
172. Baumann CR, Imbach LL, Baumann-Vogel H, Uhl M, Sarnthein J, Sürücü O. Interleaving deep brain stimulation for a patient with both Parkinson's disease and essential tremor. Mov Disord. (2012) 27:1700–1. doi: 10.1002/mds.25221
173. Wojtecki L, Vesper J, Schnitzler A. Interleaving programming of subthalamic deep brain stimulation to reduce side effects with good motor outcome in a patient with Parkinson's disease. Parkinsonism Relat Disord. (2011) 17:293–4. doi: 10.1016/j.parkreldis.2010.12.005
174. Herzog J, Pinsker M, Wasner M, Steigerwald F, Wailke S, Deuschl G, et al. Stimulation of subthalamic fibre tracts reduces dyskinesias in STN-DBS. Mov Disord. (2007) 22:679–84. doi: 10.1002/mds.21387
175. Burrows AM, Ravin PD, Novak P, Peters MLB, Dessureau B, Swearer J, et al. Limbic and motor function comparison of deep brain stimulation of the zona incerta and subthalamic nucleus. Neurosurgery. (2012) 70:125–30; discussion: 130–1. doi: 10.1227/NEU.0b013e318232fdac
176. Ricciardi L, Morgante L, Epifanio A, Zibetti M, Lanotte M, Lopiano L, et al. Stimulation of the subthalamic area modulating movement and behavior. Parkinsonism Relat Disord. (2014) 20:1298–300. doi: 10.1016/j.parkreldis.2014.07.013
177. Ramirez-Zamora A, Kahn M, Campbell J, DeLaCruz P, Pilitsis JG. Interleaved programming of subthalamic deep brain stimulation to avoid adverse effects and preserve motor benefit in Parkinson's disease. J Neurol. (2015) 262:578–84. doi: 10.1007/s00415-014-7605-3
178. Kern DS, Picillo M, Thompson JA, Sammartino F, di Biase L, Munhoz RP, et al. Interleaving stimulation in Parkinson's disease, tremor, and dystonia. Stereotact Funct Neurosurg. (2019) 96:379–91. doi: 10.1159/000494983
179. Hariz M, Blomstedt P, Zrinzo L. Future of brain stimulation: new targets, new indications, new technology. Mov Disord. (2013) 28:1784–92. doi: 10.1002/mds.25665
180. Stefani A, Peppe A, Pierantozzi M, Galati S, Moschella V, Stanzione P, et al. Multi-target strategy for Parkinsonian patients: the role of deep brain stimulation in the centromedian-parafascicularis complex. Brain Res Bull. (2009) 78:113–8. doi: 10.1016/j.brainresbull.2008.08.007
181. Almeida L, Rawal PV, Ditty B, Smelser BL, Huang H, Okun MS, et al. Deep brain stimulation battery longevity: comparison of monopolar versus bipolar stimulation modes. Move Dis Clin Pract. (2016) 3:359–66. doi: 10.1002/mdc3.12285
182. Bouthour W, Wegrzyk J, Momjian S, Péron J, Fleury V, Tomkova Chaoui E, et al. Short pulse width in subthalamic stimulation in Parkinson's disease: a randomized, double-blind study. Mov Disord. (2017) 33:169–73. doi: 10.1002/mds.27265
183. Reich MM, Steigerwald F, Sawalhe AD, Reese R, Gunalan K, Johannes S, et al. Short pulse width widens the therapeutic window of subthalamic neurostimulation. Ann Clin Transl Neurol. (2015) 2:427–32. doi: 10.1002/acn3.168
184. Holsheimer J, Demeulemeester H, Nuttin B, de Sutter P. Identification of the target neuronal elements in electrical deep brain stimulation. Eur J Neurosci. (2000) 12:4573–7. doi: 10.1111/j.1460-9568.2000.01306.x
185. Garcia L, D'Alessandro G, Bioulac B, Hammond C. High-frequency stimulation in Parkinson's disease: more or less? Trends Neurosci. (2005) 28:209–16. doi: 10.1016/j.tins.2005.02.005
186. Garcia L, D'Alessandro G, Fernagut P-O, Bioulac B, Hammond C. Impact of high-frequency stimulation parameters on the pattern of discharge of subthalamic neurons. J Neurophysiol. (2005) 94:3662–9. doi: 10.1152/jn.00496.2005
187. Ferraye MU, Debû B, Fraix V, Goetz L, Ardouin C, Yelnik J, et al. Effects of pedunculopontine nucleus area stimulation on gait disorders in Parkinson's disease. Brain. (2010) 133:205–14. doi: 10.1093/brain/awp229
188. Vercruysse S, Vandenberghe W, Münks L, Nuttin B, Devos H, Nieuwboer A. Effects of deep brain stimulation of the subthalamic nucleus on freezing of gait in Parkinson's disease: a prospective controlled study. J Neurol Neurosurg Psychiatr. (2014) 85:871–7. doi: 10.1136/jnnp-2013-306336
189. Schlenstedt C, Shalash A, Muthuraman M, Falk D, Witt K, Deuschl G. Effect of high-frequency subthalamic neurostimulation on gait and freezing of gait in Parkinson's disease: a systematic review and meta-analysis. Eur J Neurol. (2017) 24:18–26. doi: 10.1111/ene.13167
190. Xie T, Kang UJ, Warnke P. Effect of stimulation frequency on immediate freezing of gait in newly activated STN DBS in Parkinson's disease. J Neurol Neurosurg Psychiatr. (2012) 83:1015–7. doi: 10.1136/jnnp-2011-302091
191. Ramdhani RA, Patel A, Swope D, Kopell BH. Early use of 60 Hz frequency subthalamic stimulation in Parkinson's disease: a case series and review. Neuromodulation. (2015) 18:664–69. doi: 10.1111/ner.12288
192. Fietzek UM, Zwosta J, Schroeteler FE, Ziegler K, Ceballos-Baumann AO. Levodopa changes the severity of freezing in Parkinson's disease. Parkinsonism Relat Disord. (2013) 19:894–6. doi: 10.1016/j.parkreldis.2013.04.004
193. Chan CS, Guzman JN, Ilijic E, Mercer JN, Rick C, Tkatch T, et al. “Rejuvenation” protects neurons in mouse models of Parkinson's disease. Nature. (2007) 447:1081–6. doi: 10.1038/nature05865
194. Moreau C, Delval A, Defebvre L, Dujardin K, Duhamel A, Petyt G, et al. Methylphenidate for gait hypokinesia and freezing in patients with Parkinson's disease undergoing subthalamic stimulation: a multicentre, parallel, randomised, placebo-controlled trial. Lancet Neurol. (2012) 11:589–96. doi: 10.1016/S1474-4422(12)70106-0
195. Moreau C, Defebvre L, Destee A, Bleuse S, Clement F, Blatt JL, et al. STN-DBS frequency effects on freezing of gait in advanced Parkinson disease. Neurology. (2008) 71:80–4. doi: 10.1212/01.wnl.0000303972.16279.46
196. Moreau C, Pennel-Ployart O, Pinto S, Plachez A, Annic A, Viallet F, et al. Modulation of dysarthropneumophonia by low-frequency STN DBS in advanced Parkinson's disease. Mov Disord. (2011) 26:659–63. doi: 10.1002/mds.23538
197. Xie T, Vigil J, MacCracken E, Gasparaitis A, Young J, Kang W, et al. Low-frequency stimulation of STN-DBS reduces aspiration and freezing of gait in patients with PD. Neurology. (2015) 84:415–20. doi: 10.1212/WNL.0000000000001184
198. Khoo HM, Kishima H, Hosomi K, Maruo T, Tani N, Oshino S, et al. Low-frequency subthalamic nucleus stimulation in Parkinson's disease: a randomized clinical trial. Mov Disord. (2014) 29:270–4. doi: 10.1002/mds.25810
199. Brozova H, Barnaure I, Alterman RL, Tagliati M. STN-DBS frequency effects on freezing of gait in advanced Parkinson disease. Neurology. (2009) 72:770; author reply: 770–1. doi: 10.1212/01.wnl.0000339385.187472.7d
200. Phibbs FT, Arbogast PG, Davis TL. 60-Hz frequency effect on gait in Parkinson's disease with subthalamic nucleus deep brain stimulation. Neuromodulation. (2014) 17:717–20; discussion: 720. doi: 10.1111/ner.12131
201. Sidiropoulos C, Walsh R, Meaney C, Poon YY, Fallis M, Moro E. Low-frequency subthalamic nucleus deep brain stimulation for axial symptoms in advanced Parkinson's disease. J Neurol. (2013) 260:2306–11. doi: 10.1007/s00415-013-6983-2
202. Vallabhajosula S, Haq IU, Hwynn N, Oyama G, Okun M, Tillman MD, et al. Low-frequency versus high-frequency subthalamic nucleus deep brain stimulation on postural control and gait in Parkinson's disease: a quantitative study. Brain Stimul. (2015) 8:64–75. doi: 10.1016/j.brs.2014.10.011
203. Stegemöller EL, Vallabhajosula S, Haq I, Hwynn N, Hass CJ, Okun MS. Selective use of low frequency stimulation in Parkinson's disease based on absence of tremor. NeuroRehabilitation. (2013) 33:305–12. doi: 10.3233/NRE-130960
204. Ricchi V, Zibetti M, Angrisano S, Merola A, Arduino N, Artusi CA, et al. Transient effects of 80 Hz stimulation on gait in STN DBS treated PD patients: a 15 months follow-up study. Brain Stimul. (2012) 5:388–92. doi: 10.1016/j.brs.2011.07.001
205. Xie T, Padmanaban M, Bloom L, MacCracken E, Bertacchi B, Dachman A, et al. Effect of low versus high frequency stimulation on freezing of gait and other axial symptoms in Parkinson patients with bilateral STN DBS: a mini-review. Transl Neurodegener. (2017) 6:13. doi: 10.1186/s40035-017-0083-7
206. Huang H, Watts RL, Montgomery EB. Effects of deep brain stimulation frequency on bradykinesia of Parkinson's disease. Mov Disord. (2014) 29:203–6. doi: 10.1002/mds.25773
207. Wojtecki L, Timmermann L, Jörgens S, Südmeyer M, Maarouf M, Treuer H, et al. Frequency-dependent reciprocal modulation of verbal fluency and motor functions in subthalamic deep brain stimulation. Arch Neurol. (2006) 63:1273–6. doi: 10.1001/archneur.63.9.1273
208. Mazzone P, Lozano A, Stanzione P, Galati S, Scarnati E, Peppe A, et al. Implantation of human pedunculopontine nucleus: a safe and clinically relevant target in Parkinson??s disease. Neuroreport. (2005) 16:1877–81. doi: 10.1097/01.wnr.0000187629.38010.12
209. Golestanirad L, Elahi B, Graham SJ, Das S, Wald LL. Efficacy and safety of Pedunculopontine Nuclei (PPN) deep brain stimulation in the treatment of gait disorders: a meta-analysis of clinical studies. Can J Neurol Sci. (2016) 43:120–6. doi: 10.1017/cjn.2015.318
210. Windels F, Thevathasan W, Silburn P, Sah P. Where and what is the PPN and what is its role in locomotion? Brain. (2015) 138:1133–4. doi: 10.1093/brain/awv059
211. Schrader C, Seehaus F, Capelle HH, Windhagen A, Windhagen H, Krauss JK. Effects of pedunculopontine area and pallidal DBS on gait ignition in Parkinson's disease. Brain Stimul. (2013) 6:856–9. doi: 10.1016/j.brs.2013.05.005
212. Khan S, Mooney L, Plaha P, Javed S, White P, Whone AL, et al. Outcomes from stimulation of the caudal zona incerta and pedunculopontine nucleus in patients with Parkinson's disease. Br J Neurosurg. (2011) 25:273–80. doi: 10.3109/02688697.2010.544790
213. Wang J-W, Zhang Y-Q, Zhang X-H, Wang Y-P, Li J-P, Li Y-J. Deep brain stimulation of pedunculopontine nucleus for postural instability and gait disorder after parkinson disease: a meta-analysis of individual patient data. World Neurosurg. (2017) 102:72–8. doi: 10.1016/j.wneu.2017.02.110
214. Fournier-Gosselin M-P, Lipsman N, Saint-Cyr JA, Hamani C, Lozano AM. Regional anatomy of the pedunculopontine nucleus: relevance for deep brain stimulation. Mov Disord. (2013) 28:1330–6. doi: 10.1002/mds.25620
215. Morita H, Hass CJ, Moro E, Sudhyadhom A, Kumar R, Okun MS. Pedunculopontine nucleus stimulation: where are we now and what needs to be done to move the field forward? Front Neurol. (2014) 5:243. doi: 10.3389/fneur.2014.00243
216. Thevathasan W, Debû B, Aziz T, Bloem BR, Blahak C, Butson C, et al. Pedunculopontine nucleus deep brain stimulation in Parkinson's disease: A clinical review. Mov Disord. (2017) 33:10–20. doi: 10.1002/mds.27098
217. Moro E, Hamani C, Poon Y-Y, Al-Khairallah T, Dostrovsky JO, Hutchison WD, et al. Unilateral pedunculopontine stimulation improves falls in Parkinson's disease. Brain. (2010) 133:215–24. doi: 10.1093/brain/awp261
218. Brosius SN, Gonzalez CL, Shuresh J, Walker HC. Reversible improvement in severe freezing of gait from Parkinson's disease with unilateral interleaved subthalamic brain stimulation. Parkinsonism Relat Disord. (2015) 21:1469–70. doi: 10.1016/j.parkreldis.2015.09.047
219. Koss AM, Alterman RL, Tagliati M, Shils JL. Calculating total electrical energy delivered by deep brain stimulation systems. Ann Neurol. (2005) 58:168; author reply: 168–9. doi: 10.1002/ana.20525
220. Merola A, Zibetti M, Artusi CA, Rizzi L, Angrisano S, Lanotte M, et al. 80 Hz versus 130 Hz subthalamic nucleus deep brain stimulation: effects on involuntary movements. Parkinsonism Relat Disord. (2013) 19:453–6. doi: 10.1016/j.parkreldis.2013.01.006
221. Katayama Y, Oshima H, Kano T, Kobayashi K, Fukaya C, Yamamoto T. Direct effect of subthalamic nucleus stimulation on levodopa-induced peak-dose dyskinesia in patients with Parkinson's disease. Stereotact Funct Neurosurg. (2006) 84:176–9. doi: 10.1159/000094957
222. Herzog J, Pogarell O, Pinsker MO, Kupsch A, Oertel WH, Lindvall O, et al. Deep brain stimulation in Parkinson's disease following fetal nigral transplantation. Mov Disord. (2008) 23:1293–6. doi: 10.1002/mds.21768
223. Maltête D, Derrey S, Chastan N, Debono B, Gérardin E, Fréger P, et al. Microsubthalamotomy: an immediate predictor of long-term subthalamic stimulation efficacy in Parkinson disease. Mov Disord. (2008) 23:1047–50. doi: 10.1002/mds.22054
224. Singh A, Kammermeier S, Mehrkens J-H, Bötzel K. Movement kinematic after deep brain stimulation associated microlesions. J Neurol Neurosurg Psychiatr. (2012) 83:1022–6. doi: 10.1136/jnnp-2012-302309
225. Witt K, Daniels C, Herzog J, Lorenz D, Volkmann J, Reiff J, et al. Differential effects of L-dopa and subthalamic stimulation on depressive symptoms and hedonic tone in Parkinson's disease. J Neuropsychiatry Clin Neurosci. (2006) 18:397–401. doi: 10.1176/jnp.2006.18.3.397
226. Thobois S, Ardouin C, Lhommée E, Klinger H, Lagrange C, Xie J, et al. Non-motor dopamine withdrawal syndrome after surgery for Parkinson's disease: predictors and underlying mesolimbic denervation. Brain. (2010) 133:1111–27. doi: 10.1093/brain/awq032
227. Czernecki V, Schüpbach M, Yaici S, Lévy R, Bardinet E, Yelnik J, et al. Apathy following subthalamic stimulation in Parkinson disease: a dopamine responsive symptom. Mov Disord. (2008) 23:964–9. doi: 10.1002/mds.21949
228. Russmann H, Ghika J, Combrement P, Villemure J-G, Bogousslavsky J, Burkhard PR, et al. L-dopa-induced dyskinesia improvement after STN-DBS depends upon medication reduction. Neurology. (2004) 63:153–5. doi: 10.1212/01.WNL.0000131910.72829.9D
229. Moro E, Esselink RJA, Benabid A-L, Pollak P. Response to levodopa in parkinsonian patients with bilateral subthalamic nucleus stimulation. Brain. (2002) 125:2408–17. doi: 10.1093/brain/awf249
230. Lempka SF, Miocinovic S, Johnson MD, Vitek JL, McIntyre CC. In vivo impedance spectroscopy of deep brain stimulation electrodes. J Neural Eng. (2009) 6:046001. doi: 10.1088/1741-2560/6/4/046001
231. Beaulieu-Boire I, Fasano A. Current or voltage? Another Shakespearean dilemma. Eur J Neurol. (2015) 22:887–8. doi: 10.1111/ene.12537
232. Maks CB, Butson CR, Walter BL, Vitek JL, McIntyre CC. Deep brain stimulation activation volumes and their association with neurophysiological mapping and therapeutic outcomes. J Neurol Neurosurg Psychiatr. (2009) 80:659–66. doi: 10.1136/jnnp.2007.126219
233. Lempka SF, Johnson MD, Miocinovic S, Vitek JL, McIntyre CC. Current-controlled deep brain stimulation reduces in vivo voltage fluctuations observed during voltage-controlled stimulation. Clin Neurophysiol. (2010) 121:2128–33. doi: 10.1016/j.clinph.2010.04.026
234. Benabid AL, Pollak P, Gao D, Hoffmann D, Limousin P, Gay E, et al. Chronic electrical stimulation of the ventralis intermedius nucleus of the thalamus as a treatment of movement disorders. J Neurosurg. (1996) 84:203–14. doi: 10.3171/jns.1996.84.2.0203
235. Sillay KA, Chen JC, Montgomery EB. Long-term measurement of therapeutic electrode impedance in deep brain stimulation. Neuromodulation. (2010) 13:195–200. doi: 10.1111/j.1525-1403.2010.00275.x
236. Hemm S, Vayssiere N, Mennessier G, Cif L, Zanca M, Ravel P, et al. Evolution of brain impedance in dystonic patients treated by GPI electrical stimulation. Neuromodulation. (2004) 7:67–75. doi: 10.1111/j.1094-7159.2004.04009.x
237. Cheung T, Nuño M, Hoffman M, Katz M, Kilbane C, Alterman R, et al. Longitudinal impedance variability in patients with chronically implanted DBS devices. Brain Stimul. (2013) 6:746–51. doi: 10.1016/j.brs.2013.03.010
238. Ramirez de Noriega F, Eitan R, Marmor O, Lavi A, Linetzky E, Bergman H, et al. Constant current versus constant voltage subthalamic nucleus deep brain stimulation in Parkinson's disease. Stereotact Funct Neurosurg. (2015) 93:114–21. doi: 10.1159/000368443
239. Preda F, Cavandoli C, Lettieri C, Pilleri M, Antonini A, Eleopra R, et al. Switching from constant voltage to constant current in deep brain stimulation: a multicenter experience of mixed implants for movement disorders. Eur J Neurol. (2016) 23:190–5. doi: 10.1111/ene.12835
240. Lettieri C, Rinaldo S, Devigili G, Pisa F, Mucchiut M, Belgrado E, et al. Clinical outcome of deep brain stimulation for dystonia: constant-current or constant-voltage stimulation? A non-randomized study. Eur J Neurol. (2015) 22:919–26. doi: 10.1111/ene.12515
241. Bronstein JM, Tagliati M, McIntyre C, Chen R, Cheung T, Hargreaves EL, et al. The rationale driving the evolution of deep brain stimulation to constant-current devices. Neuromodulation. (2015) 18:85–8; discussion: 88–9. doi: 10.1111/ner.12227
242. Barow E, Neumann W-J, Brücke C, Huebl J, Horn A, Brown P, et al. Deep brain stimulation suppresses pallidal low frequency activity in patients with phasic dystonic movements. Brain. (2014) 137:3012–24. doi: 10.1093/brain/awu258
243. Dowsey-Limousin P. Postoperative management of Vim DBS for tremor. Mov Disord. (2002) 17(Suppl. 3):S208–11. doi: 10.1002/mds.10165
244. Earhart GM, Hong M, Tabbal SD, Perlmutter JS. Effects of thalamic stimulation frequency on intention and postural tremor. Exp Neurol. (2007) 208:257–63. doi: 10.1016/j.expneurol.2007.08.014
245. Ushe M, Mink JW, Revilla FJ, Wernle A, Schneider Gibson P, McGee-Minnich L, et al. Effect of stimulation frequency on tremor suppression in essential tremor. Mov Disord. (2004) 19:1163–8. doi: 10.1002/mds.20231
246. Ushe M, Mink JW, Tabbal SD, Hong M, Schneider Gibson P, Rich KM, et al. Postural tremor suppression is dependent on thalamic stimulation frequency. Mov Disord. (2006) 21:1290–2. doi: 10.1002/mds.20926
247. O'Suilleabhain PE, Frawley W, Giller C, Dewey RB. Tremor response to polarity, voltage, pulsewidth and frequency of thalamic stimulation. Neurology. (2003) 60:786–90. doi: 10.1212/01.WNL.0000044156.56643.74
248. Moldovan A-S, Hartmann CJ, Trenado C, Meumertzheim N, Slotty PJ, Vesper J, et al. Less is more - Pulse width dependent therapeutic window in deep brain stimulation for essential tremor. Brain Stimul. (2018) 1:1132–9. doi: 10.1016/j.brs.2018.04.019
249. Groppa S, Herzog J, Falk D, Riedel C, Deuschl G, Volkmann J. Physiological and anatomical decomposition of subthalamic neurostimulation effects in essential tremor. Brain. (2014) 137:109–121. doi: 10.1093/brain/awt304
250. Herzog J, Hamel W, Wenzelburger R, Pötter M, Pinsker MO, Bartussek J, et al. Kinematic analysis of thalamic versus subthalamic neurostimulation in postural and intention tremor. Brain. (2007) 130:1608–25. doi: 10.1093/brain/awm077
251. Fytagoridis A, Sandvik U, Åström M, Bergenheim T, Blomstedt P. Long term follow-up of deep brain stimulation of the caudal zona incerta for essential tremor. J Neurol Neurosurg Psychiatr. (2012) 83:258–62. doi: 10.1136/jnnp-2011-300765
252. Blomstedt P, Sandvik U, Tisch S. Deep brain stimulation in the posterior subthalamic area in the treatment of essential tremor. Mov Disord. (2010) 25:1350–6. doi: 10.1002/mds.22758
253. Plaha P, Javed S, Agombar D, O' Farrell G, Khan S, Whone A, Gill S. Bilateral caudal zona incerta nucleus stimulation for essential tremor: outcome and quality of life. J Neurol Neurosurg Psychiatr. (2011) 82:899–904. doi: 10.1136/jnnp.2010.222992
254. Koller W, Pahwa R, Busenbark K, Hubble J, Wilkinson S, Lang A, et al. High-frequency unilateral thalamic stimulation in the treatment of essential and parkinsonian tremor. Ann Neurol. (1997) 42:292–9. doi: 10.1002/ana.410420304
255. Baizabal-Carvallo JF, Kagnoff MN, Jimenez-Shahed J, Fekete R, Jankovic J. The safety and efficacy of thalamic deep brain stimulation in essential tremor: 10 years and beyond. J Neurol Neurosurg Psychiatr. (2014) 85:567–72. doi: 10.1136/jnnp-2013-304943
256. Barbe MT, Pochmann J, Lewis CJ, Allert N, Wirths J, Visser Vandewalle V, et al. Utilization of predefined stimulation groups by essential tremor patients treated with VIM-DBS. Parkinsonism Relat Disord. (2014) 20:1415–8. doi: 10.1016/j.parkreldis.2014.09.021
257. Mücke D, Becker J, Barbe MT, Meister I, Liebhart L, Roettger TB, et al. The effect of deep brain stimulation on the speech motor system. J Speech Lang Hear Res. (2014) 57:1206–18. doi: 10.1044/2014_;JSLHR-S-13-0155
258. Fasano A, Herzog J, Raethjen J, Rose FEM, Muthuraman M, Volkmann J, et al. Gait ataxia in essential tremor is differentially modulated by thalamic stimulation. Brain. (2010) 133:3635–48. doi: 10.1093/brain/awq267
259. Kronenbuerger M, Konczak J, Ziegler W, Buderath P, Frank B, Coenen VA, et al. Balance and motor speech impairment in essential tremor. Cerebellum. (2009) 8:389–98. doi: 10.1007/s12311-009-0111-y
260. Earhart GM, Clark BR, Tabbal SD, Perlmutter JS. Gait and balance in essential tremor: variable effects of bilateral thalamic stimulation. Mov Disord. (2009) 24:386–91. doi: 10.1002/mds.22356
261. Hopfner F, Deuschl G. Is essential tremor a single entity? Eur J Neurol. (2018) 25:71–82. doi: 10.1111/ene.13454
262. Yamamoto T, Katayama Y, Kano T, Kobayashi K, Oshima H, Fukaya C. Deep brain stimulation for the treatment of parkinsonian, essential, and poststroke tremor: a suitable stimulation method and changes in effective stimulation intensity. J Neurosurg. (2004) 101:201–9. doi: 10.3171/jns.2004.101.2.0201
263. Pilitsis JG, Metman LV, Toleikis JR, Hughes LE, Sani SB, Bakay RAE. Factors involved in long-term efficacy of deep brain stimulation of the thalamus for essential tremor. J Neurosurg. (2008) 109:640–6. doi: 10.3171/JNS/2008/109/10/0640
264. Zhang K, Bhatia S, Oh MY, Cohen D, Angle C, Whiting D. Long-term results of thalamic deep brain stimulation for essential tremor. J Neurosurg. (2010) 112:1271–6. doi: 10.3171/2009.10.JNS09371
265. Barbe MT, Liebhart L, Runge M, Pauls KAM, Wojtecki L, Schnitzler A, et al. Deep brain stimulation in the nucleus ventralis intermedius in patients with essential tremor: habituation of tremor suppression. J Neurol. (2011) 258:434–9. doi: 10.1007/s00415-010-5773-3
266. Hariz G-M, Blomstedt P, Koskinen L-OD. Long-term effect of deep brain stimulation for essential tremor on activities of daily living and health-related quality of life. Acta Neurol Scand. (2008) 118:387–94. doi: 10.1111/j.1600-0404.2008.01065.x
267. Limousin P, Speelman JD, Gielen F, Janssens M, study collaborators. Multicentre European study of thalamic stimulation in parkinsonian and essential tremor. J Neurol Neurosurg Psychiatr. (1999) 66:289–96. doi: 10.1136/jnnp.66.3.289
268. Koller WC, Lyons KE, Wilkinson SB, Troster AI, Pahwa R. Long-term safety and efficacy of unilateral deep brain stimulation of the thalamus in essential tremor. Mov Disord. (2001) 16:464–8. doi: 10.1136/jnnp.71.5.682
269. Martinez-Ramirez D, Morishita T, Zeilman PR, Peng-Chen Z, Foote KD, Okun MS. Atrophy and other potential factors affecting long term deep brain stimulation response: a case series. PLoS ONE. (2014) 9:e111561. doi: 10.1371/journal.pone.0111561
270. Favilla CG, Ullman D, Wagle Shukla A, Foote KD, Jacobson CE, Okun MS. Worsening essential tremor following deep brain stimulation: disease progression versus tolerance. Brain. (2012) 135:1455–62. doi: 10.1093/brain/aws026
271. Garcia Ruiz P, Muñiz de Igneson J, Lopez Ferro O, Martin C, Magariños Ascone C. Deep brain stimulation holidays in essential tremor. J Neurol. (2001) 248:725–6. doi: 10.1007/s004150170127
272. Eltahawy HA, Saint-Cyr J, Giladi N, Lang AE, Lozano AM. Primary dystonia is more responsive than secondary dystonia to pallidal interventions: outcome after pallidotomy or pallidal deep brain stimulation. Neurosurgery. (2004) 54:613–19; discussion: 619–21. doi: 10.1227/01.NEU.0000108643.94730.21
273. Vidailhet M, Vercueil L, Houeto J-L, Krystkowiak P, Benabid A-L, Cornu P, et al. Bilateral deep-brain stimulation of the globus pallidus in primary generalized dystonia. N Engl J Med. (2005) 352:459–67. doi: 10.1056/NEJMoa042187
274. Vidailhet M, Yelnik J, Lagrange C, Fraix V, Grabli D, Thobois S, et al. Bilateral pallidal deep brain stimulation for the treatment of patients with dystonia-choreoathetosis cerebral palsy: a prospective pilot study. Lancet Neurol. (2009) 8:709–17. doi: 10.1016/S1474-4422(09)70151-6
275. Picillo M, Pellecchia MT, Vitale C, Barone P, Amboni M. Pallidal stimulation in atypical pantothenate kinase-associated neurodegeneration: six-year follow-up. Mov Disord. (2014) 29:276–7. doi: 10.1002/mds.25709
276. Krauss JK, Yianni J, Loher TJ, Aziz TZ. Deep brain stimulation for dystonia. J Clin Neurophysiol. (2004) 21:18–30. doi: 10.1097/00004691-200401000-00004
277. Kupsch A, Benecke R, Müller J, Trottenberg T, Schneider G-H, Poewe W, et al. Pallidal deep-brain stimulation in primary generalized or segmental dystonia. N Engl J Med. (2006) 355:1978–90. doi: 10.1056/NEJMoa063618
278. Grips E, Blahak C, Capelle H-H, Bäzner H, Weigel R, Sedlaczek O, et al. Patterns of reoccurrence of segmental dystonia after discontinuation of deep brain stimulation. J Neurol Neurosurg Psychiatr. (2007) 78:318–20. doi: 10.1136/jnnp.2006.089409
279. Cheung T, Zhang C, Rudolph J, Alterman RL, Tagliati M. Sustained relief of generalized dystonia despite prolonged interruption of deep brain stimulation. Mov Disord. (2013) 28:1431–4. doi: 10.1002/mds.25353
280. Grabli D, Ewenczyk C, Coelho Braga MC, Lagrange C, Fraix V, Cornu P, et al. Interruption of deep brain stimulation of the globus pallidus in primary generalized dystonia. Mov Disord. (2009) 24:2363–9. doi: 10.1002/mds.22827
281. Levin J, Singh A, Feddersen B, Mehrkens J-H, Bötzel K. Onset latency of segmental dystonia after deep brain stimulation cessation: a randomized, double-blind crossover trial. Mov Disord. (2013) 29:944–9. doi: 10.1002/mds.25780
282. Yianni J, Bain PG, Gregory RP, Nandi D, Joint C, Scott RB, et al. Post-operative progress of dystonia patients following globus pallidus internus deep brain stimulation. Eur J Neurol. (2003) 10:239–47. doi: 10.1046/j.1468-1331.2003.00592.x
283. Trottenberg T, Meissner W, Kabus C, Arnold G, Funk T, Einhaupl KM, et al. Neurostimulation of the ventral intermediate thalamic nucleus in inherited myoclonus-dystonia syndrome. Mov Disord. (2001) 16:769–71. doi: 10.1002/mds.1119
284. Kumar R. Methods for programming and patient management with deep brain stimulation of the globus pallidus for the treatment of advanced Parkinson's disease and dystonia. Mov Disord. (2002) 17(Suppl. 3):S198–207. doi: 10.1002/mds.10164
285. Cheung T, Noecker AM, Alterman RL, McIntyre CC, Tagliati M. Defining a therapeutic target for pallidal deep brain stimulation for dystonia. Ann Neurol. (2014) 76:22–30. doi: 10.1002/ana.24187
286. Rozanski VE, da Silva NM, Ahmadi S-A, Mehrkens J, da Silva Cunha J, Houde J-C, et al. The role of the pallidothalamic fibre tracts in deep brain stimulation for dystonia: a diffusion MRI tractography study. Hum Brain Mapp. (2017) 38:1224–32. doi: 10.1002/hbm.23450
287. Tisch S, Zrinzo L, Limousin P, Bhatia KP, Quinn N, Ashkan K, et al. Effect of electrode contact location on clinical efficacy of pallidal deep brain stimulation in primary generalised dystonia. J Neurol Neurosurg Psychiatr. (2007) 78:1314–9. doi: 10.1136/jnnp.2006.109694
288. Kupsch A, Tagliati M, Vidailhet M, Aziz T, Krack P, Moro E, et al. Early postoperative management of DBS in dystonia: programming, response to stimulation, adverse events, medication changes, evaluations, and troubleshooting. Mov Disord. (2011) 26(Suppl. 1):S37–53. doi: 10.1002/mds.23624
289. Kupsch A, Klaffke S, Kühn AA, Meissner W, Arnold G, Schneider G-H, et al. The effects of frequency in pallidal deep brain stimulation for primary dystonia. J Neurol. (2003) 250:1201–5. doi: 10.1007/s00415-003-0179-0
290. Alterman RL, Shils JL, Miravite J, Tagliati M. Lower stimulation frequency can enhance tolerability and efficacy of pallidal deep brain stimulation for dystonia. Mov Disord. (2007) 22:366–8. doi: 10.1002/mds.21274
291. Moro E, Piboolnurak P, Arenovich T, Hung SW, Poon YY, Lozano AM. Pallidal stimulation in cervical dystonia: clinical implications of acute changes in stimulation parameters. Eur J Neurol. (2009) 16:506–12. doi: 10.1111/j.1468-1331.2008.02520.x
292. Coubes P, Cif L, Fertit El H, Hemm S, Vayssiere N, Serrat S, et al. Electrical stimulation of the globus pallidus internus in patients with primary generalized dystonia: long-term results. J Neurosurg. (2004) 101:189–94. doi: 10.3171/jns.2004.101.2.0189
293. Vercueil L, Houeto J-L, Krystkowiak P, Lagrange C, Cassim F, Benazzouz A, et al. Effects of pulse width variations in pallidal stimulation for primary generalized dystonia. J Neurol. (2007) 254:1533–7. doi: 10.1007/s00415-007-0578-8
294. Ostrem JL, Markun LC, Glass GA, Racine CA, Volz MM, Heath SL, et al. Effect of frequency on subthalamic nucleus deep brain stimulation in primary dystonia. Parkinsonism Relat Disord. (2014) 20:432–8. doi: 10.1016/j.parkreldis.2013.12.012
295. Zauber SE, Watson N, Comella CL, Bakay RAE, Metman LV. Stimulation-induced parkinsonism after posteroventral deep brain stimulation of the globus pallidus internus for craniocervical dystonia. J Neurosurg. (2009) 110:229–33. doi: 10.3171/2008.6.17621
296. Berman BD, Starr PA, Marks WJ, Ostrem JL. Induction of bradykinesia with pallidal deep brain stimulation in patients with cranial-cervical dystonia. Stereotact Funct Neurosurg. (2009) 87:37–44. doi: 10.1159/000195718
297. Brecl Jakob G, Pelykh O, Košutzká Z, Pirtošek Z, Trošt M, Ilmberger J, et al. Postural stability under globus pallidus internus stimulation for dystonia. Clin Neurophysiol. (2015) 126:2299–305. doi: 10.1016/j.clinph.2015.01.022
298. Krack P, Pollak P, Limousin P, Hoffmann D, Benazzouz A, Le Bas J-F, et al. Opposite motor effects of pallidal stimulation in Parkinson's disease. Ann Neurol. (1998) 43:180–92. doi: 10.1002/ana.410430208
299. Schrader C, Capelle H-H, Kinfe TM, Blahak C, Bäzner H, Lütjens G, et al. GPi-DBS may induce a hypokinetic gait disorder with freezing of gait in patients with dystonia. Neurology. (2011) 77:483–8. doi: 10.1212/WNL.0b013e318227b19e
300. Blahak C, Capelle H-H, Baezner H, Kinfe TM, Hennerici MG, Krauss JK. Micrographia induced by pallidal DBS for segmental dystonia: a subtle sign of hypokinesia? J Neural Transm. (Vienna). (2011) 118:549–53. doi: 10.1007/s00702-010-0544-y
301. Volkmann J, Wolters A, Kupsch A, Müller J, Kühn AA, Schneider G-H, et al. Pallidal deep brain stimulation in patients with primary generalised or segmental dystonia: 5-year follow-up of a randomised trial. Lancet Neurol. (2012) 11:1029–38. doi: 10.1016/S1474-4422(12)70257-0
Keywords: DBS programming algorithms, subthalamic nucleus, DBS side effects, segmented electrode, short pulse width
Citation: Koeglsperger T, Palleis C, Hell F, Mehrkens JH and Bötzel K (2019) Deep Brain Stimulation Programming for Movement Disorders: Current Concepts and Evidence-Based Strategies. Front. Neurol. 10:410. doi: 10.3389/fneur.2019.00410
Received: 13 December 2018; Accepted: 04 April 2019;
Published: 21 May 2019.
Edited by:
Matteo Bologna, Sapienza University of Rome, ItalyReviewed by:
Angel Sesar, Complejo Hospitalario Universitario de Santiago, SpainAdolfo Ramirez-Zamora, University of Florida Health, United States
Copyright © 2019 Koeglsperger, Palleis, Hell, Mehrkens and Bötzel. This is an open-access article distributed under the terms of the Creative Commons Attribution License (CC BY). The use, distribution or reproduction in other forums is permitted, provided the original author(s) and the copyright owner(s) are credited and that the original publication in this journal is cited, in accordance with accepted academic practice. No use, distribution or reproduction is permitted which does not comply with these terms.
*Correspondence: Thomas Koeglsperger, dGhvbWFzLmtvZWdsc3BlcmdlckBtZWQudW5pLW11ZW5jaGVuLmRl
Kai Bötzel, a2FpLmJvZXR6ZWxAbWVkLnVuaS1tdWVuY2hlbi5kZQ==