- 1Department of Neurology, Center of Cerebrovascular Diseases, West China Hospital of Sichuan University, Chengdu, China
- 2Department of Neurology, The Third People's Hospital of Chengdu, The Affiliated Hospital of Southwest Jiaotong University, Chengdu, China
Studies suggest that microRNAs that regulate expression of matrix metalloproteinase (MMP)-9 may be involved in hemorrhagic transformation (HT) after cardioembolic stroke, so we examined whether such microRNAs could predict HT in acute cardioembolic stroke patients. Blood samples were prospectively collected from patients who later experienced HT (n = 29) or did not (n = 29), and the samples were assayed for eight microRNAs identified as related to MMP-9 based on three microRNA databases. Expression levels of these microRNAs were analyzed by quantitative real-time polymerase chain reaction (qRT-PCR) in 28 of the 58 patients, 14 of whom suffered HT and 14 of whom did not. Four differentially expressed miRNAs were identified: hsa-miR-21-5p, hsa-miR-206, hsa-miR-491-5p, and hsa-miR-3123. Subsequent qRT-PCR analysis of these four miRNAs across all 58 patients showed that levels of miR-21-5p, miR-206, and miR-3123 were significantly higher in patients with HT than in those without HT, while expression of miR-491-5p was similar between the two groups. The area under the receiver operating characteristic curve for predicting HT was 0.677 (95% CI 0.535–0.818) for miR-21-5p, 0.687 (95% CI 0.543–0.830) for miR-206, and 0.661 (95% CI 0.512–0.810) for miR-3123. Our results suggest that these three microRNAs may be prognostic markers for HT after cardioembolic stroke, which should be verified by future studies with large samples.
Introduction
Hemorrhagic transformation (HT) occurs in some ischemic stroke patients. HT can occur naturally following ischemic infarction or be triggered by therapeutic interventions (1, 2). The reported incidence of spontaneous HT in Western countries ranges from 10 to 40% and in the Chinese population from 23 to 43% (3–6). Cardioembolic strokes, such as those caused by atrial fibrillation, are associated with an increased risk of HT and their prognosis could be worsened by HT (7, 8). The fear of HT and its associated complications hinder treatments for cardioembolic stroke, including reperfusion therapy, early anticoagulation, and dual-antiplatelet agents (9). Although thrombolysis for acute stroke and anticoagulation for stroke patients with atrial fibrillation are recommended by national health guidelines in many countries including China (10, 11), only 2–5% of Chinese patients with acute ischemic stroke receive thrombolysis (12, 13). Among patients who do not receive thrombolysis, the reason in more than one third of cases is concern over the risk of HT (14). Similarly, only 21–37% of stroke patients with atrial fibrillation receive anticoagulation in China (15). About 32% of stroke patients with atrial fibrillation do not receive anticoagulation because of concerns about the risk of intracranial hemorrhage (16). Therefore, finding predictors for identifying patients that are at high risk of HT would improve treatment of cardioembolic stroke.
Matrix metalloproteinase-9 (MMP-9) participates in multiple physiological and pathological processes in the central nervous system. MMP-9 is an enzyme that digests type IV collagen, laminin, and fibronectin, which are major components of the basal lamina in cerebral blood vessels (17). Animal and human studies have demonstrated that increased MMP-9 expression plays an important role in blood-brain barrier (BBB) leakage and is significantly increased in patients with HT after ischemic stroke (18). Systematic review of previous studies concluded that MMP-9 independently predicts HT in acute stroke patients after the use of recombinant tissue plasminogen activator (19). These studies collectively demonstrated that MMP-9 may be involved in HT after acute ischemic stroke, possibly through mechanisms related to BBB disruption.
MicroRNAs (miRNAs) are small, non-coding RNA molecules of 20–24 nucleotides that regulate gene expression at the posttranscriptional level (20). Certain miRNAs regulate MMP-9 expression during stroke (21). Furthermore, some miRNAs that are highly expressed in brain tissue can similarly be detected in peripheral blood (22). These results suggest that circulating miRNAs may serve as clinical biomarkers for stroke.
In the current study, we hypothesized that some miRNAs that are related to MMP-9 would be prognostic biomarkers for predicting HT following cardioembolic stroke. Therefore, we tested the potential association between these miRNAs and HT after cardioembolic stroke in a patient sample.
Materials and Methods
Patient Selection
A total of 58 cardioembolic stroke patients admitted within 7 days of stroke onset were randomly selected from the Department of Neurology, West China Hospital of Sichuan University (Chengdu, China) between September 2013 and January 2017. Among these patients, 29 patients (66 ± 13 years old; 72.4% female) later experienced HT and the other 29 patients (67 ± 14 years old; 79.3% female) did not. No significant differences were found between two groups regarding age, gender, risk factors, and anti-thrombotic medications used before admission. The datasets supporting the findings of the current study are available from the corresponding author upon reasonable request.
All patients received a clinical diagnosis of stroke according to World Health Organization criteria (23), and this diagnosis was confirmed by computed tomography (CT) or magnetic resonance imaging (MRI). Cardioembolic etiology was identified according to the Trial of ORG 10172 in Acute Stroke Treatment (TOAST) classification (24). Only Han Chinese adults over 18 years of age were included in this study. Patients were excluded if they (1) had intracerebral hemorrhage (ICH) before or at admission; (2) had recent head injuries, hematological disorders, severe renal or liver dysfunction, malignancies, systemic infection, or large-artery stenosis due to atherosclerosis or arteritis; (3) received immunosuppressive therapies before or during admission; (4) received thrombolytic therapies after stroke onset; or (5) were unable to take care of themselves for any reason prior to stroke onset.
Baseline Data Collection
We recorded baseline clinical and demographic information, including age; sex; ethnicity; stroke severity assessed by the National Institutes of Health Stroke Scale (NIHSS) on admission (25); pre-existing risk factors, including history of hypertension, diabetes mellitus, hyperlipidemia, atrial fibrillation, coronary artery disease, ICH, ischemic stroke or transient ischemic attack, current smoking, and alcohol consumption; anti-thrombotic medications used before admission, such as antiplatelet agents and anticoagulants; date of stroke symptom onset; and time of blood sampling and neuroimaging. All patients in our study underwent CT within 24 h on arrival at the emergency department, which is the point of entry to our comprehensive stroke center at West China Hospital (26). Patients received another CT or MRI again between 2 days and 2 weeks after stroke onset or within 2 days after worsening of the patient's condition. Any changes in symptoms or signs were recorded. CT and MRI were evaluated by a neuroradiologist blinded to patient details, including clinical characteristics of the stroke. HT was identified when hemorrhage within the acute ischemic lesion was not detected by the initial CT but was later confirmed by a second CT or MRI (27).
Blood Sampling
Venipuncture was prospectively performed in all inpatients within 24 h after arrival in the emergency department, when none of the 58 patients was known to have HT. Venous blood was collected in EDTA-containing tubes and was then sent to the laboratory within 30 min of collection. After centrifugation (3,000 rpm for 15 min at 4°C), plasma was collected and immediately frozen at −80°C until further use.
Selection and Assessment of Candidate MMP-9-related MiRNAs
TargetScan version 6.2 (www.targetscan.org), miRanda August 2010 release (www.microrna.org/microrna/home.do) and miRDB version 4.0 (www.mirdb.org) were used to predict miRNAs acting upstream of the human MMP9 gene in silico. A literature review was also performed in PubMed to identify candidate miRNA regulators of MMP-9 expression.
Extraction of miRNAs, Reverse Transcription and qRT-PCR
Plasma fractions enriched for miRNAs were isolated from plasma samples using the miRcute miRNA Isolation Kit (Tiangen, Beijing, China). Before this isolation step, all plasma samples were spiked with ath-miR156a (2.5 μl, 1 μM), which shows no sequence homology to human miRNAs and therefore served as a reference RNA. Fractions enriched in miRNA were eluted in 30 μl, and a 3 μl aliquot was treated with gDNA Eraser (Takara, Japan) to eliminate potential contaminating genomic DNA, then reverse-transcribed using the PrimeScript RT reagent Kit (Takara). Sangon (Shanghai, China) designed miRNA-specific stem-loop primers for reverse transcription (Supplementary Table).
Quantitative real-time polymerase chain reaction (qRT-PCR) was performed using a C1000 Touch Thermal Cycler (CFX96 Real-Time System, Bio-Rad, Hercules, CA, USA) with SsoFast EvaGreen Surpermix (Bio-Rad). Forward and reverse primers for each miRNA in qRT-PCR were obtained from Sangon (Supplementary Table). The resulting solubility curve and cycle threshold (Ct) were evaluated via CFX Manager software (Bio-Rad), and relative expression (fold difference) of candidate miRNAs with respect to the average level in patients who did not suffer HT (NHT) was calculated using the 2−ΔΔCt method according to the formula: fold difference = 2−[(Ct Candidate−Ct miR156a)−(Mean Ct CandidateNHT−Mean Ct miR156aNHT)].
Statistical Analyses
Categorical variables were reported as percentages. Continuous variables were reported as mean ± standard deviation or median (interquartile range [IQR]). Data normality was examined using the Shapiro–Wilk test. Results for categorical variables were compared between groups using Fisher exact tests. Results for continuous variables were compared using the Welch t-tests or Mann–Whitney U-tests. Differences were considered significant when p < 0.05 (two-tailed). All statistical analyses were performed using R version 3.4.4 (www.R-project.org).
Receiver operating characteristic (ROC) curves were constructed to assess the sensitivity and specificity of MMP-9-related miRNAs for predicting HT after cardioembolic stroke. The prognostic values of specific miRNAs were assessed based on the area under the curve (AUC). For further analyses, partial AUCs (pAUCs) were generated for the specificity range of 80–100%, meaning that a negative blood test should correctly identify at least 8 of 10 patients as having low risk of HT.
Results
A three-step approach was adopted to identify whether MMP-9-related miRNAs were prognostic biomarkers for predicting HT following cardioembolic stroke. First, only the miRNA-mRNA pairs simultaneously predicted by at least two of three databases (see Methods) were considered as possible miRNA-mRNA pairs (Supplementary Figure). This step identified x candidate miRNAs upstream of the MMP9 gene: hsa-miR-183-5p, hsa-miR-204-5p, hsa-miR-206, hsa-miR-491-5p, hsa-miR-211-5p, hsa-miR-3123, and hsa-miR-3145-5p. We also included hsa-miR-21-5p, a well-known miRNA that has been linked to MMP-9 (28).
Second, a pilot study was performed to screen levels of the 8 candidate miRNAs in 28 of the 58 patients, comprising 14 who suffered HT and 14 who did not (Table 1). This study showed that levels of circulating miR-21-5p (p = 0.009), miR-206 (p = 0.037), and miR-3123 (p = 0.036) were significantly higher in HT patients (Figure 1). In contrast, levels of miR-491-5p, miR-183-5p, miR-211-5p, miR-3145-5p, and miR-204-5p did not differ significantly between the two patient groups. The remaining miRNA, miR-491-5p, showed a trend toward statistical significance between groups: the median 2−ΔΔCt value was 18.38 (0.66–161.49) in HT vs. 0.35 (0.02–10.12) without HT (p = 0.0594). Therefore, it was included in further investigation.
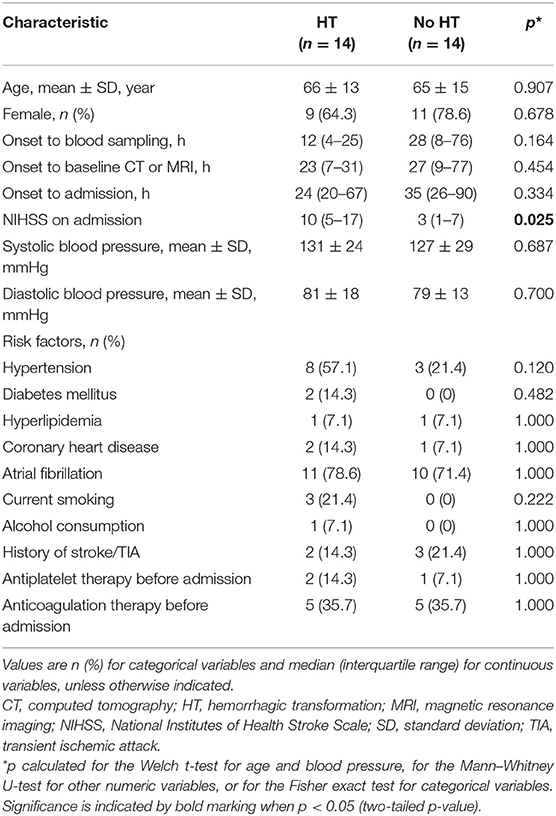
Table 1. Demographic and clinical characteristics of patients with cardioembolic stroke used to test miRNAs predicted in silico to affect MMP-9 expression.
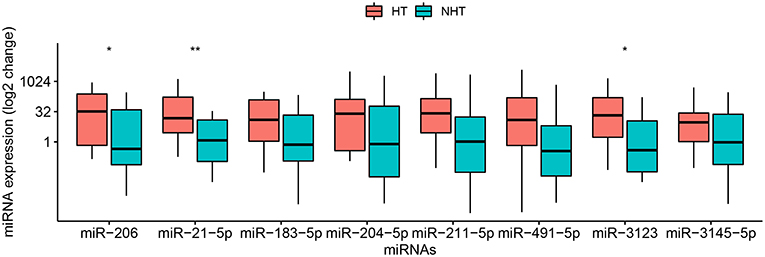
Figure 1. Relative levels of 8 miRNAs in plasma of 28 patients with cardioembolic stroke. Patients were stratified into 14 who experienced hemorrhagic transformation (HT) and 14 who did not (NHT). Relative miRNA levels were measured by qRT-PCR and calculated using the 2−ΔΔCt method. Results are presented in the log2 scale in the boxplot, where the upper limit of the box is 75th percentile, lower limit is 25th percentile, and the lines inside the box indicate the median. The Mann–Whitney U-test was used for statistical analysis. *p < 0.05; **p < 0.01.
Third, the three miRNAs that were significantly different between HT and non-HT patients, along with miR-491-5p, were analyzed in all 58 patients, whose baseline characteristics are shown in Table 2. Half these patients developed HT subsequently. The median age for all 58 patients was 70.5 (55.3–78.0) years, and the interval between onset and hospitalization was 27 (12–72) h. The interval between stroke onset and blood collection was 16 (6–48) h. Among the characteristics that we assessed, only NIHSS score on admission differed significantly between patients who developed HT or not: the score was higher among those with HT [11 (6–14) vs. 4 (3–14), p = 0.015]. Of the four candidate miRNA markers, three were expressed at significantly higher levels among patients with HT than among those without HT (Figure 2). The comparisons of 2−ΔΔCt values between the two groups were as follows: miR-21-5p, 4.17 (1.27–33.95) vs. 0.59 (0.04–9.21) (p = 0.021); miR-206, 5.29 (1.42–74.26) vs. 0.33 (0.03–37.66) (p = 0.016); and miR-3123, 7.54 (3.07–49.62) vs. 0.29 (0.01–46.50) (p = 0.036). In contrast, miR-491-5p level was similar between patients who suffered HT or not [9.74 (1.12–56.27) vs. 0.26 (0.01–79.72), p = 0.109].
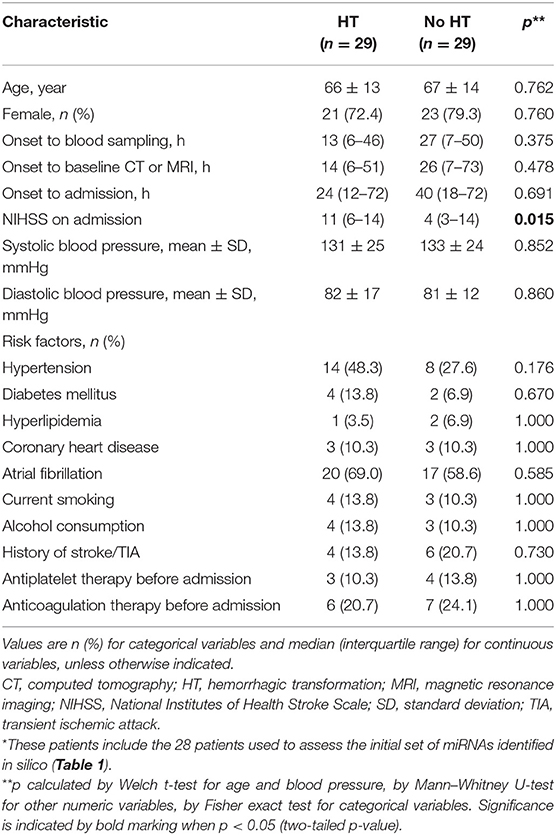
Table 2. Demographic and clinical characteristics of all 58 patients used to validate the candidate miRNAs predicted in silico to regulate MMP-9 expression*.
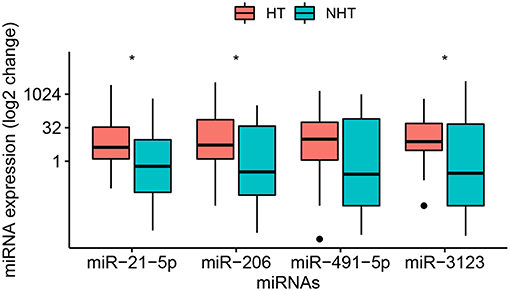
Figure 2. Relative levels of miR-21-5p, -206, -491-5p, and -3123 in plasma of the entire sample of 58 patients with cardioembolic stroke. Data were obtained and displayed as described in Figure 1, except that points indicate values falling outside 80% of the data. The Mann–Whitney U-test was used for statistical analysis. *p < 0.05.
The prognostic value of circulating miR-21-5p, miR-206, and miR-3123 for HT after cardioembolic stroke was then assessed using ROC curves and the pROC package in R (29). The AUC of miR-21-5p was 0.677 (95% CI 0.535–0.818); miR-206, 0.687 (95% CI 0.543–0.830); miR-3123, 0.661 (95% CI 0.512–0.810). Threshold values for predicting HT in the 58 patients were defined based on the upper 80% of the ROC curves (Figure 3 and Table 3). The miR-21-5p showed 31.0% sensitivity and 89.7% specificity with a threshold above a 2−ΔΔCt value of 26.90, and miR-206 and miR-3123 also showed low respective sensitivities of 28.6 and 24.1%. The pAUC for miR-21-5p was 0.042 (95% CI 0.013–0.080); miR-206, 0.034 (95% CI 0.007–0.076); and miR-3123, 0.020 (95% CI 0–0.059).
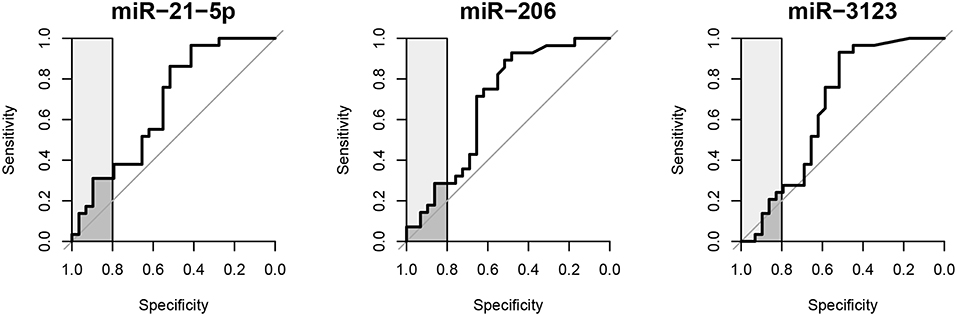
Figure 3. Receiver operating characteristic curves of miR-21-5p, miR-206, and miR-3123 for predicting HT following cardioembolic stroke. Light gray boxes highlight the top 20% of specificity. Dark gray boxes correspond to the partial area under the curve.
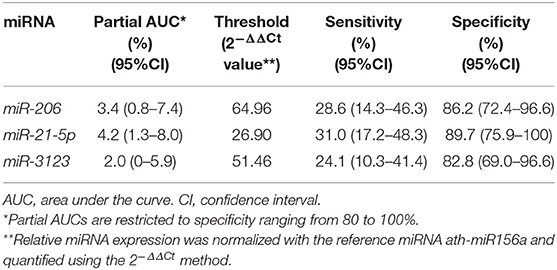
Table 3. Partial AUCs, sensitivities and specificities for miR-21-5p, miR-206, and miR-3123 in receiver operating characteristic analysis.
Discussion
In this case-control study, analysis by qRT-PCR suggested that expression of miR-21-5p, miR-206, and miR-3123 in plasma increases before HT occurs in cardioembolic stroke patients. Consistent with this idea, we found preliminary evidence that plasma levels of miR-21-5p, miR-206, and miR-3123 can predict HT after cardioembolic stroke.
Following acute ischemic stroke, there are numerous pathological processes that can cause BBB disruptions and theoretically increase the risk of HT, including apoptosis, changes in metabolism, and neuro-inflammation. Mounting evidence indicates that some miRNAs participate in these processes, making them potential markers of brain tissue injury after stroke onset (30). Compared with RNA molecules, circulating miRNAs are relatively stable in serum or plasma because they are enclosed within acid- and base-resistant membrane vesicles (31), allowing their quantitation by qRT-PCR.
Existing evidence shows that miR-21-5p, an miRNA highly expressed in vascular smooth muscle and endothelial cells (32), is related to stroke and cardiovascular disease (33). Serum expression levels of miR-21-5p have been found to correlate positively with serum activity of MMP-9 in acute coronary syndrome (34). In vivo ischemia/reperfusion injury models showed that miR-21-5p reduces cell apoptosis and promotes angiogenesis, thereby protecting tissue from damage (32, 35). A protective role for miR-21-5p is consistent with our observation of elevated levels of this miRNA in our group of patients with HT, who showed higher (worse) NIHSS scores than patients without HT. Interestingly, miR-21-5p did not emerge from our in silico screening. Nevertheless, we chose to include it because MMP-9 is regulated at stages other than transcription (28, 36), and it may regulate two MMP inhibitors (32, 37).
A skeletal muscle-specific member of the MyomiR family, miR-206 has an important role in myogenic differentiation (38). Evidence from an in vivo study indicates that miR-206 inhibits tissue inhibitor of MMP-3 and increases levels of MMP-9 and inflammatory factors (39). Moreover, levels of miR-206 positively correlated with infarct volume in an animal model of embolic stroke (40). These findings, together with our results, suggest that elevated expression of miR-206 after cardioembolic stroke exacerbates neuroinflammation and ischemic damage, thereby justifying further assessment of miR-206 as a strong candidate prognostic marker for HT after stroke.
We further identified a link between miR-3123 and risk of HT in patients with cardioembolic stroke. Although experiments have suggested no relationship between miR-3123 levels and MMP-9 expression (36), our in silico tests predict it as a regulator of this enzyme. Future studies should explore this possibility further.
The prognostic value of miR-21-5p, -206 or -3123 on their own appears to be limited, based on pAUC values, which are restricted to specificity of 80–100%. Nevertheless, if a cardioembolic stroke patient shows high values of any one of these, it may be appropriate to consider him or her at high risk of HT. Future studies should examine whether combinations of these or other miRNAs can provide a more sensitive and specific marker of risk.
In the clinical setting, one potential limitation of qRT-PCR is that it may require longer than is available for timely treatment after stroke onset. Median interval from alteplase infusion to symptomatic ICH was reported to range from 5 to 10 h (2), and the qRT-PCR in our study took more than 5 h. Intravenous alteplase therapy, if indicated, should not be delayed to await the results of plasma miRNA testing (10). For this reason, we excluded from our study patients receiving thrombolytic therapies after stroke onset.
There are several limitations in our study. This was a case-control study based on small numbers of samples in one hospital. Blood samples were not collected longitudinally, which may be important for understanding changes in miRNA expression after stroke onset. In addition to further investigations of the three miRNAs' role in MMP-9 regulation, neurological deterioration and HT development, future studies should perform longitudinal analysis of a large, preferably multi-center population to develop a complete, time-specific miRNA expression profile for HT prediction in cardioembolic stroke patients. Regardless, our study provides preliminary and intriguing hypothesis-generating findings to lead the way for future advancement in the relationship between miRNA and HT.
Data Availability
The datasets generated for this study are available on request to the corresponding author.
Ethics Statement
This study was reviewed and approved under the ethical project identification code 2015(300) by the Biomedical Ethics Committee of West China Hospital, Sichuan University. Written informed consent was obtained from all patients or their guardians.
Author Contributions
DW and ML conceived the project and designed the study. LZ, YX, XY, and LW recruited patients and acquired clinical data. YX, LZ, JL, and SZ performed the biomarker experiments, then collected, and analyzed data. LZ and YX wrote the manuscript, which DW revised. All authors have read, revised, and approved the final version of the manuscript.
Funding
DW was supported by the National Natural Science Foundation of China (81400964 and 81870923), the National Key Research and Development Program of China (2018YFC1705006 and 2017YFC1308401), Sichuan University (2018SCUH0066), and West China Hospital (2018HXFH041). ML was supported by the National Natural Science Foundation of China (81620108009) and the National Key Research and Development Program of China (2016YFC1300500-505). SZ was supported by the National Natural Science Foundation of China (81500923).
Conflict of Interest Statement
The authors declare that the research was conducted in the absence of any commercial or financial relationships that could be construed as a potential conflict of interest.
Acknowledgments
We would like to thank all patient participants in this study.
Supplementary Material
The Supplementary Material for this article can be found online at: https://www.frontiersin.org/articles/10.3389/fneur.2019.00945/full#supplementary-material
References
2. Yaghi S, Willey JZ, Cucchiara B, Goldstein JN, Gonzales NR, Khatri P, et al. Treatment and outcome of hemorrhagic transformation after intravenous alteplase in acute ischemic stroke: a scientific statement for healthcare professionals from the American Heart Association/American Stroke Association. Stroke. (2017) 48:e343–61. doi: 10.1161/STR.0000000000000152
3. Jickling GC, Liu D, Stamova B, Ander BP, Zhan X, Lu A, et al. Hemorrhagic transformation after ischemic stroke in animals and humans. J Cereb Blood Flow Metab. (2014) 34:185–99. doi: 10.1038/jcbfm.2013.203
4. Tan S, Wang D, Liu M, Zhang S, Wu B, Liu B. Frequency and predictors of spontaneous hemorrhagic transformation in ischemic stroke and its association with prognosis. J Neurol. (2014) 261:905–12. doi: 10.1007/s00415-014-7297-8
5. Yin J, Zeng X, Cheng Y, Wan P, Wang P, Ren S, et al. Risk factors of spontaneous hemorrhagic transformation after cerebral infarction. Chin J Neuromed. (2013) 12:53–56. doi: 10.3760/cma.j.issn.1671-8925.2013.01.012
6. Chen J, Zhang S, Zhao Y, Jin X, Li Y, Huang L. Analysis on the clinical characteristics and related risk factors of patients with hemorrhagic transformation after cerebral infarction. Chin J Epidemiol. (2012) 33:1176–80. doi: 10.3760/cma.j.issn.0254-6450.2012.11.018
7. Lee J-H, Park K-Y, Shin J-H, Cha J-K, Kim H-Y, Kwon J-H, et al. Symptomatic hemorrhagic transformation and its predictors in acute ischemic stroke with atrial fibrillation. Eur Neurol. (2010) 64:193–200. doi: 10.1159/000319048
8. Tu HTH, Campbell BCV, Christensen S, Desmond PM, De Silva DA, Parsons MW, et al. Worse stroke outcome in atrial fibrillation is explained by more severe hypoperfusion, infarct growth, and hemorrhagic transformation. Int J Stroke. (2015) 10:534–40. doi: 10.1111/ijs.12007
9. Ogilvie IM, Newton N, Welner SA, Cowell W, Lip GY. Underuse of oral anticoagulants in atrial fibrillation: a systematic review. Am J Med. (2010) 123:638–45. doi: 10.1016/j.amjmed.2009.11.025
10. Powers WJ, Rabinstein AA, Ackerson T, Adeoye OM, Bambakidis NC, Becker K, et al. 2018 guidelines for the early management of patients with acute ischemic stroke: a guideline for healthcare professionals from the American Heart Association/American Stroke Association. Stroke. (2018) 49:e46–99. doi: 10.1016/j.jvs.2018.04.007
11. Wang Y, Liu M, Pu C. 2014 Chinese guidelines for secondary prevention of ischemic stroke and transient ischemic attack: compiled by the Chinese Society of Neurology, Cerebrovascular Disease Group. Int J Stroke. (2017) 12:302–20. doi: 10.1177/1747493017694391
12. Wang Y, Liao X, Zhao X, Wang DZ, Wang C, Nguyen-Huynh MN, et al. Using recombinant tissue plasminogen activator to treat acute ischemic stroke in China: analysis of the results from the Chinese National Stroke Registry (CNSR). Stroke. (2011) 42:1658–64. doi: 10.1161/STROKEAHA.110.604249
13. Chao A-C, Hsu H-Y, Chung C-P, Liu C-H, Chen C-H, Teng MM-H, et al. Outcomes of thrombolytic therapy for acute ischemic stroke in Chinese patients. Stroke. (2010) 41:885–90. doi: 10.1161/STROKEAHA.109.575605
14. Wang M-D, Yin X-X, Yang T-T, Wang Y, Zhu Y-Y, Zhou Y-F, et al. Chinese neurologists' perspective on intravenous thrombolysis for acute ischemic stroke. Brain Behav. (2018) 8:e00882. doi: 10.1002/brb3.882
15. Chang S-S, Dong J-Z, Ma C-S, Du X, Wu J-H, Tang R-B, et al. Current status and time trends of oral anticoagulation use among Chinese patients with nonvalvular atrial fibrillation. Stroke. (2016) 47:1803–10. doi: 10.1161/STROKEAHA.116.012988
16. Yang X, Li Z, Zhao X, Wang C, Liu L, Wang C, et al. Use of warfarin at discharge among acute ischemic stroke patients with nonvalvular atrial fibrillation in China. Stroke. (2016) 47:464–70. doi: 10.1161/STROKEAHA.115.011833
17. Montaner J, Molina CA, Monasterio J, Abilleira S, Arenillas JF, Ribó M, et al. Matrix metalloproteinase-9 pretreatment level predicts intracranial hemorrhagic complications after thrombolysis in human stroke. Circulation. (2003) 107:598–603. doi: 10.1161/01.CIR.0000046451.38849.90
18. Chaturvedi M, Kaczmarek L. Mmp-9 inhibition: a therapeutic strategy in ischemic stroke. Mol Neurobiol. (2014) 49:563–73. doi: 10.1007/s12035-013-8538-z
19. Ramos-Fernandez M, Bellolio MF, Stead LG. Matrix metalloproteinase-9 as a marker for acute ischemic stroke: a systematic review. J Stroke Cerebrovasc Dis. (2011) 20:47–54. doi: 10.1016/j.jstrokecerebrovasdis.2009.10.008
20. Delaloy C, Liu L, Lee J-A, Su H, Shen F, Yang G-Y, et al. MicroRNA-9 coordinates proliferation and migration of human embryonic stem cell-derived neural progenitors. Cell Stem Cell. (2010) 6:323–35. doi: 10.1016/j.stem.2010.02.015
21. Volny O, Kasickova L, Coufalova D, Cimflova P, Novak J. microRNAs in cerebrovascular disease. Adv Exp Med Biol. (2015) 888:155–95. doi: 10.1007/978-3-319-22671-2_9
22. Jeyaseelan K, Lim KY, Armugam A. MicroRNA expression in the blood and brain of rats subjected to transient focal ischemia by middle cerebral artery occlusion. Stroke. (2008) 39:959–66. doi: 10.1161/STROKEAHA.107.500736
23. Hatano S. Experience from a multicentre stroke register: a preliminary report. Bull World Health Organ. (1976) 54:541.
24. Adams HP, Bendixen BH, Kappelle LJ, Biller J, Love BB, Gordon DL, et al. Classification of subtype of acute ischemic stroke. Definitions for use in a multicenter clinical trial. TOAST. Trial of Org 10172 in Acute Stroke Treatment. Stroke. (1993) 24:35–41. doi: 10.1161/01.STR.24.1.35
25. Brott T, Adams HP, Olinger CP, Marler JR, Barsan WG, Biller J, et al. Measurements of acute cerebral infarction: a clinical examination scale. Stroke. (1989) 20:864–70. doi: 10.1161/01.STR.20.7.864
26. Hao Z, Wu B, Lin S, Kong F-Y, Tao W-D, Wang D-R, et al. Association between renal function and clinical outcome in patients with acute stroke. Eur Neurol. (2010) 63:237–42. doi: 10.1159/000285165
27. Paciaroni M, Bandini F, Agnelli G, Tsivgoulis G, Yaghi S, Furie KL, et al. Hemorrhagic transformation in patients with acute ischemic stroke and atrial fibrillation: time to initiation of oral anticoagulant therapy and outcomes. J Am Heart Assoc. (2018) 7:e010133. doi: 10.1161/JAHA.118.010133
28. Abba M, Patil N, Allgayer H. MicroRNAs in the regulation of MMPs and metastasis. Cancers. (2014) 6:625–45. doi: 10.3390/cancers6020625
29. Robin X, Turck N, Hainard A, Tiberti N, Lisacek F, Sanchez J-C, et al. pROC: an open-source package for R and S+ to analyze and compare ROC curves. BMC Bioinformatics. (2011) 12:77. doi: 10.1186/1471-2105-12-77
30. Mirzaei H, Momeni F, Saadatpour L, Sahebkar A, Goodarzi M, Masoudifar A, et al. MicroRNA: relevance to stroke diagnosis, prognosis, and therapy. J Cell Physiol. (2018) 233:856–65. doi: 10.1002/jcp.25787
31. Chen X, Ba Y, Ma L, Cai X, Yin Y, Wang K, et al. Characterization of microRNAs in serum: a novel class of biomarkers for diagnosis of cancer and other diseases. Cell Res. (2008) 18:997. doi: 10.1038/cr.2008.282
32. Hu J, Ni S, Cao Y, Zhang T, Wu T, Yin X, et al. The angiogenic effect of microRNA-21 targeting TIMP3 through the regulation of MMP2 and MMP9. PLoS ONE. (2016) 11:e0149537. doi: 10.1371/journal.pone.0149537
33. Jazbutyte V, Thum T. MicroRNA-21: from cancer to cardiovascular disease. Curr Drug Targets. (2010) 11:926–35. doi: 10.2174/138945010791591403
34. Darabi F, Aghaei M, Movahedian A, Pourmoghadas A, Sarrafzadegan N. The role of serum levels of microRNA-21 and matrix metalloproteinase-9 in patients with acute coronary syndrome. Mol Cell Biochem. (2016) 422:51–60. doi: 10.1007/s11010-016-2805-z
35. Buller B, Liu X, Wang X, Zhang RL, Zhang L, Hozeska-Solgot A, et al. miR-21 protects neurons from ischemic death. FEBS J. (2010) 277:4299–307. doi: 10.1111/j.1742-4658.2010.07818.x
36. Hentati-Kallel M, Jan L, Sébastien Bernard P, Antonicelli F, Trussardi-Régnier A. Histone deacetylases meet microRNA-associated MMP-9 expression regulation in glucocorticoid-sensitive and -resistant cell lines. Int J Oncol. (2017) 50:717–26. doi: 10.3892/ijo.2016.3830
37. Reis ST, Pontes-Junior J, Antunes AA, Dall'Oglio MF, Dip N, Passerotti CC, et al. miR-21 may acts as an oncomir by targeting RECK, a matrix metalloproteinase regulator, in prostate cancer. BMC Urol. (2012) 12:14. doi: 10.1186/1471-2490-12-14
38. McCarthy JJ. MicroRNA-206: The skeletal muscle-specific myomiR. Biochim Biophys Acta. (2008) 1779:682–91. doi: 10.1016/j.bbagrm.2008.03.001
39. Fu X, Zeng L, Liu Z, Ke X, Lei L, Li G. MicroRNA-206 regulates the secretion of inflammatory cytokines and MMP9 expression by targeting TIMP3 in Mycobacterium tuberculosis-infected THP-1 human macrophages. Biochem Biophys Res Commun. (2016) 477:167–73. doi: 10.1016/j.bbrc.2016.06.038
Keywords: microRNA, hemorrhagic transformation, cardioembolic stroke, MMP-9, prediction
Citation: Zheng L, Xiong Y, Liu J, Yang X, Wang L, Zhang S, Liu M and Wang D (2019) MMP-9-Related microRNAs as Prognostic Markers for Hemorrhagic Transformation in Cardioembolic Stroke Patients. Front. Neurol. 10:945. doi: 10.3389/fneur.2019.00945
Received: 13 March 2019; Accepted: 15 August 2019;
Published: 06 September 2019.
Edited by:
Jean-Claude Baron, University of Cambridge, United KingdomReviewed by:
Xiaoying Wang, Tulane University, United StatesNikolaus Plesnila, Institute for Stroke and Dementia Research (ISD), Germany
Copyright © 2019 Zheng, Xiong, Liu, Yang, Wang, Zhang, Liu and Wang. This is an open-access article distributed under the terms of the Creative Commons Attribution License (CC BY). The use, distribution or reproduction in other forums is permitted, provided the original author(s) and the copyright owner(s) are credited and that the original publication in this journal is cited, in accordance with accepted academic practice. No use, distribution or reproduction is permitted which does not comply with these terms.
*Correspondence: Deren Wang, d2FuZ2RlcmVuQHdjaHNjdS5jbg==; Ming Liu, d3lwbG1oQGhvdG1haWwuY29t
†These authors have contributed equally to this work as first authors