- 1Dementia Research Centre, UCL Queen Square Institute of Neurology, University College London, London, United Kingdom
- 2Preventive Neurology Unit, Wolfson Institute of Preventive Medicine, Queen Mary University of London, London, United Kingdom
Our awareness of time, specifically of longer intervals spanning hours, days, months, and years, is critical for ensuring our sense of self-continuity. Disrupted time awareness over such intervals is a clinical feature in a number of frontotemporal dementia syndromes and Alzheimer's disease, but has not been studied and compared systematically in these diseases. We used a semi-structured caregiver survey to capture time-related behavioral alterations in 71 patients representing all major sporadic and genetic syndromes of frontotemporal dementia, in comparison to 28 patients with typical Alzheimer's disease and nine with logopenic aphasia, and 32 healthy older individuals. Survey items pertained to apparent difficulties ordering past personal events or estimating time intervals between events, temporal rigidity and clockwatching, and propensity to relive past events. We used a logistic regression model including diagnosis, age, gender, and disease severity as regressors to compare the proportions of individuals exhibiting each temporal awareness symptom between diagnostic groups. Gray matter associations of altered time awareness were assessed using voxel-based morphometry. All patient groups were significantly more prone to exhibit temporal awareness symptoms than healthy older individuals. Clinical syndromic signatures were identified. While patients with typical and logopenic Alzheimer's disease most frequently exhibited disturbed event ordering or interval estimation, patients with semantic dementia were most prone to temporal rigidity and clockwatching and those with behavioral variant frontotemporal dementia commonly exhibited all these temporal symptoms as well as a propensity to relive past events. On voxel-based morphometry, the tendency to relive past events was associated with relative preservation of a distributed left-sided temporo-parietal gray matter network including hippocampus. These findings reveal a rich and complex picture of disturbed temporal awareness in major dementia syndromes, with stratification of frontotemporal dementia syndromes from Alzheimer's disease. This is the first study to assess symptoms of altered temporal awareness across frontotemporal dementia syndromes and provides a motivation for future work directed to the development of validated clinical questionnaires, analysis of underlying neurobiological mechanisms and design of interventions.
Introduction
Our capacity to experience and calibrate the passage of time anchors us in the flux of sensory experience and allows us to track external events, conduct our daily affairs and most fundamentally, maintain a sense of self-continuity from past to future (1–3). Our awareness of time is not dictated simply by the clock: it is a complex and elastic, subjective psychological construct, encompassing multiple, hierarchically embedded scales, ranging from fractions of a second to an entire lifetime (4–6). Brief time intervals are more directly accessible to laboratory analysis and accordingly, most neuropsychological data on time perception and awareness relate to shorter timescales (7–9). However, many of our psychologically salient experiences unfold over longer timespans that are challenging to study experimentally.
Distributed cortical and subcortical brain networks—including prefrontal and insular cortices (2, 5, 10–14), parietal cortex (15–17), hippocampus (3, 18–20), and basal ganglia (21–23)—have been associated with temporal encoding at different time scales and mediating different kinds of temporal computations (4, 6, 24, 25). Normal temporal awareness across timescales and in particular, integration of external clock time with internal bodily or “subjective” time is likely to depend on interactions between large-scale neural networks: for example, fronto-striatal time-keeping circuitry (26–29) operating in concert with the so-called “default mode” temporo-parietal network that mediates self-awareness and self-projection (12, 25, 30).
This neural network paradigm provides a rationale for anticipating and understanding alterations of subjective temporal awareness accompanying neurodegenerative pathologies. These pathologies characteristically and selectively target the distributed neural networks implicated in temporal processing in the healthy brain (31–35). It follows that neurodegenerative disorders are likely to have overlapping but separable phenotypes of abnormal temporal awareness, arising from the profiles of network involvement they produce. From a neurobiological perspective, better definition of temporal processing mechanisms in these diseases might provide novel insights into their pathophysiology. However, the clinical features and brain substrates of altered temporal awareness in major dementias such as Alzheimer's disease (AD) and in particular, frontotemporal dementia (FTD), have not been systematically assessed and compared.
Several questionnaires and interview protocols have been developed to look at subjective time awareness in both healthy and clinical populations (36–39). Notably, the Autobiographical Interview (40) has been useful in demonstrating impaired retrieval of past autobiographical memories (41, 42), and diminished ability to project into the future (43) in patients diagnosed with AD. Distortions of subjective time estimation (44–46), confusion about temporal ordering and reduced self-projection in time (46–49) have also been described in AD. Deficits of temporal processing in AD are not simply due to deteriorating episodic memory for the details of events, but rather the sequencing of those events in relation to one another, suggesting a more fundamental disorder of the mental timeline.
Temporal processing abnormalities have also been observed in FTD. This is a clinically and pathologically heterogeneous group of diseases (31), comprising three canonical clinico-anatomical syndromes: behavioral variant FTD (bvFTD; led by impaired socio-emotional signal processing and reactivity, with atrophy predominantly of prefrontal and insular cortices and their connections) and the language-led syndromes of semantic dementia (SD; led by degradation of vocabulary and conceptual knowledge with selective left anterior temporal lobe atrophy) and progressive non-fluent aphasia (PNFA; led by speech and language output failure with predominant left-sided peri-Sylvian atrophy). Impaired perception and generation of temporal intervals and patterns over short time intervals attributed to a deranged internal “clock” mechanism have been described in bvFTD and PNFA (50, 51). Over longer timescales, difficulties with prospection and retrospection have been documented in bvFTD and SD (49, 52–56). There are currently no validated instruments to assess clockwatching and more general inflexibility or obsessionality around time, although these are frequently associated with both bvFTD and SD (31, 57, 58). Impaired awareness of time and associated disruptions of socio-emotional behaviors in these diseases potentially take a substantial toll on patient well-being and care burden and therefore constitute a significant clinical issue.
Here we addressed the issue of temporal awareness in FTD in a cohort of patients representing all canonical syndromes of FTD (bvFTD, SD, and PNFA), in relation to healthy older individuals and a cohort of patients with typical amnestic AD and its major, language-led variant phenotype, logopenic aphasia (LPA). We surveyed temporal behavioral alterations which we hypothesized on clinical grounds to be particularly pertinent to the target syndromes and to the timeframes of daily life and autobiographical experience. We did not set out in this first study to characterize temporal symptoms in detail. Rather, our principal objective here was to survey the kinds of altered temporal awareness that occur in FTD, to estimate the relative proportions of patients with different FTD syndromes exhibiting symptoms of altered temporal awareness and to explore possible differences with respect to typical and amnestic AD. We assessed structural neuroanatomical correlates of altered temporal awareness using voxel-based morphometry (VBM) of patients' brain magnetic resonance images.
Based on clinical observations, we hypothesized that patients with AD syndromes (both the typical amnestic and the language-led phenotypes) would exhibit particularly prominent disturbances of temporal interval estimation and event ordering. In contrast, we hypothesized that patients with FTD syndromes (especially bvFTD and SD) would particularly exhibit reduced temporal flexibility and clockwatching. Based on available neuroimaging evidence in the healthy brain (2, 4, 5, 11, 13, 15–17, 20, 25, 28, 59–61), we further hypothesized that alterations of temporal awareness would have neural network correlates, reflecting the relative degree of involvement of posterior temporo-parietal cortices and hippocampus (engaged in temporal sequencing) vs. prefrontal and antero-mesial temporal cortices (engaged in temporal scheduling, appraisal, and valuation).
Methods
Participants
Seventy-one patients with FTD (34 bvFTD, 17 SD, 20 PNFA), twenty-eight patients with a typical memory-led syndrome of AD (hereafter, AD) and nine patients with LPA were recruited via a specialist cognitive disorders clinic. Thirty-two age-matched healthy individuals with no history of neurological or active psychiatric illness were recruited via the departmental research database. All patients fulfilled consensus diagnostic criteria for the relevant syndromic diagnosis (62–64) and all had clinically mild to moderate severity disease. Genetic screening revealed pathogenic mutations in twenty-two cases (eight C9orf72, all bvFTD; seven MAPT, 6 bvFTD and 1 SD; seven GRN, 4 bvFTD and 3 PNFA). Brain MRI was consistent with the syndromic diagnosis in all patients and none had evidence of significant cerebrovascular burden. Cerebrospinal fluid profiles of tau and beta-amyloid1−42 were available for twelve patients with typical AD and six patients with LPA; each was consistent with underlying Alzheimer's pathology, based on local reference ranges (total tau/beta-amyloid1−42 ratio >0.8). In all patients, the syndromic diagnosis was further corroborated by a comprehensive general neuropsychological assessment. We recorded the use of medications (antidepressants and neuroleptics) that could potentially affect time perception among the participant groups (65, 66). Clinical, demographic, and neuropsychological characteristics of all participant groups are summarized in Table 1.
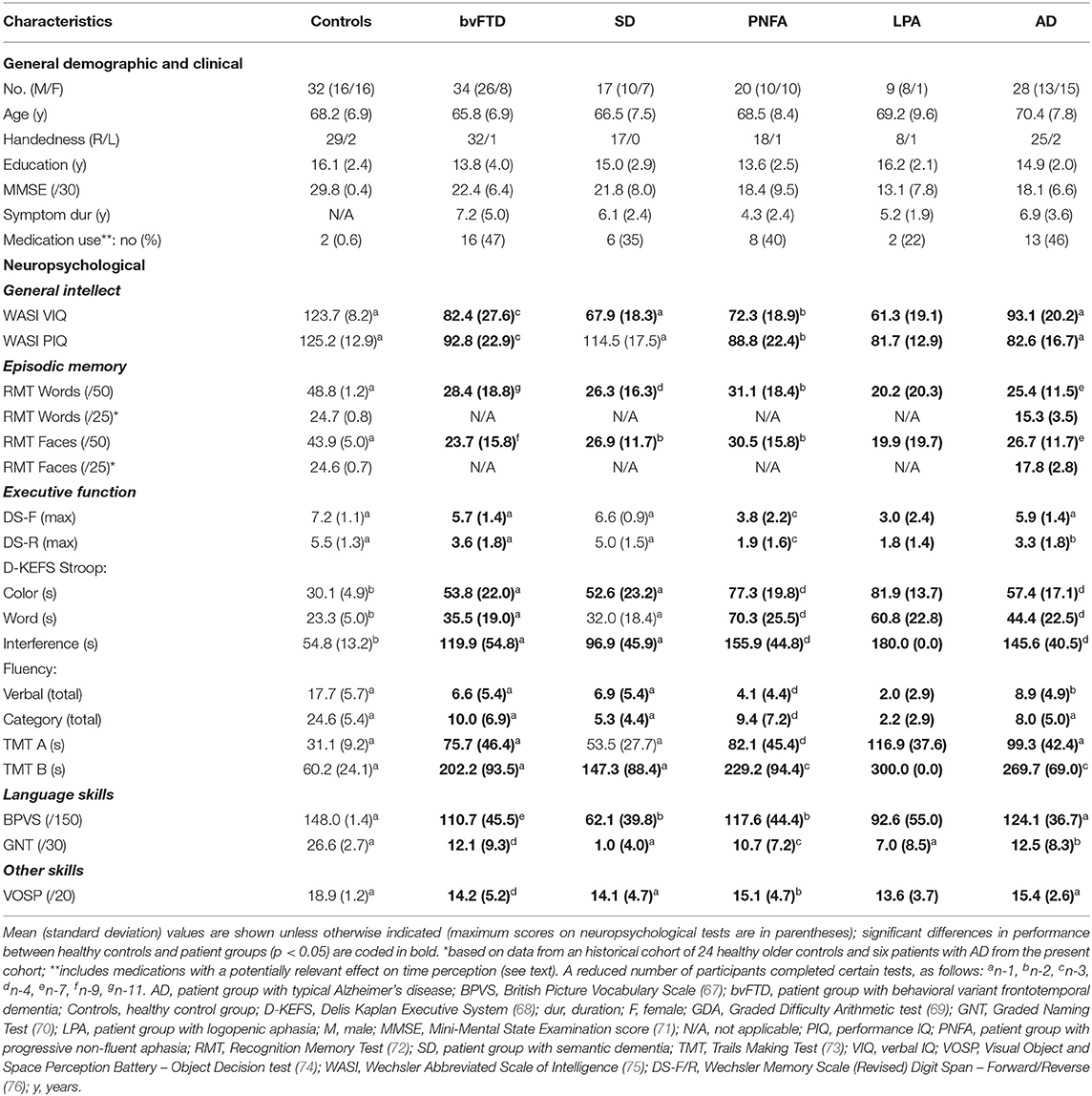
Table 1. General demographic, clinical and neuropsychological characteristics of participant groups.
All participants gave informed consent for their involvement in the study. Ethical approval was granted by the University College London and National Hospital for Neurology and Neurosurgery Joint Research Ethics Committees in accordance with Declaration of Helsinki.
Assessment of Temporal Awareness
We surveyed the presence of behavioral symptoms suggesting an alteration of subjective temporal awareness (Table 2). We sampled temporal behavioral symptoms that we felt were likely to be pertinent based on our accumulated clinical experience of the target syndromes. These symptoms comprised: apparent confusion about the temporal ordering of experienced past personal events and/or how long ago such events occurred or will occur in future (i.e., difficulty estimating the interval separating the present from the past/prospective event); reduced temporal flexibility (temporal rigidity, exemplified by high valuation of punctuality, and discomfort if schedules were disturbed) and/or clockwatching (looking at their watch or asking for the time very often); and an increased tendency to re-live personal events from the past (as indicated, for example, by a conversational preoccupation with such events). The survey was completed by healthy controls and by each patient's primary caregiver; involvement of caregivers (either the patient's spouse or child) was intended to maximize understanding, communication, and accuracy of symptom reporting, since people with dementia (particularly FTD syndromes) often have limited insight into their own illness. For each of the sampled symptoms of altered temporal awareness, survey respondents were asked to indicate whether or not prominent changes (i.e., evident most days) had occurred. Caregivers were asked to compare patients' current behavior with their behavior premorbidly while healthy controls were asked whether they felt there had been any changes in their own behavior, referenced in each case to the situation 10 years previously: this interval reflects the typical duration of clinical symptoms in the target diseases plus some allowance for any prodromal changes. In addition, respondents were given the opportunity to make free comments, to provide further details about temporal behavioral alterations.
Analysis of Clinical and Behavioral Data
Clinical and behavioral data were analyzed using Stata version 14.0 software (StataCorp, College Station, TX, USA). Participant groups were compared using a one-way ANOVA for continuous variables satisfying normality criteria, or the non-parametric equivalent Kruskal-Wallis test if this was not the case. Post-hoc tests of non-parametric continuous variables were performed using Dunn's test. For categorical variables, we used the chi-square test, or Fisher's exact test when expected counts were small.
Survey data were analyzed to determine the prevalence of changes in temporal awareness for each participant group. Temporal awareness symptoms were coded as 1 (present) or 0 (absent). Because for three of the five symptom items no healthy control participant exhibited the symptom, we did not build a logistic regression model to compare the prevalence of alterations on that item for all patient groups vs. the healthy control group. Instead, we performed a two-tailed Fisher's exact test for each item and corrected for multiple comparisons using the Benjamini-Yekutieli procedure (77). For each temporal awareness symptom, once we had established an overall disease effect for that symptom, we used a logistic regression model to compare the log odds of exhibiting that symptom (as the dependent variable) between patient groups. We specified a dummy variable for diagnosis (our main variable of interest) taking the AD group as the reference and included age, gender and Mini-Mental State Examination (MMSE) score as covariates, to take into account of potentially confounding effects from these factors. MMSE score here served as an index of overall disease severity; although there is no single, principled index of severity for FTD syndromes (and all candidate severity measures are to some extent problematic and potentially confounded by linguistic and other considerations), the MMSE is a simple, widely used index that can be applied across participant groups. We also built logistic regression models to assess possible correlations between temporal awareness symptoms. Finally, we looked for any associations between altered temporal awareness and general clinical covariates (age, gender, years of education, MMSE score, relevant medication use) using the Student's t-test or the Wilcoxon rank sum test for continuous variables, and the chi-square test for categorical variables. A statistical significance threshold p < 0.05 was accepted for all tests.
Brain Image Acquisition and Analysis
Volumetric brain MRI data from 91 patients were entered into the VBM analysis; scans were unavailable for 14 patients (three bvFTD, one PNFA, two LPA, eight AD) and a further three (two bvFTD, one PNFA) were inadequate on technical grounds. For each patient, a sagittal 3D magnetization-prepared rapid-gradient echo T1-weighted volumetric brain MR sequence (TE/TR/TI 2.9/2200/900 ms, dimensions 256 × 256 × 208, voxel volume of 1.1 × 1.1 × 1.1 mm) was acquired on a Siemens Prisma 3T MRI scanner using a 32-channel phased array head-coil. Pre-processing of brain images was performed using the New Segment and Diffeomorphic Anatomical Registration Through Exponentiated Lie Algebra (DARTEL) toolboxes in SPM12 (www.fil.ion.ucl.ac.uk/spm/software/spm12/), following an optimized protocol (78). Normalization, segmentation, and modulation of gray and white matter images were carried out using default parameter settings. Gray matter images were subsequently smoothed using a 6 mm full width-at-half-maximum Gaussian kernel. For each patient, total intracranial volume was calculated by combining gray matter, white matter, cerebrospinal fluid volumes after segmentation of these tissue types. A study-specific template brain image was created by warping all native space whole-brain images to the final DARTEL template and calculating the average of the warped brain images.
Across the combined patient cohort, we ran a full factorial model to assess associations of regional gray matter volume with each temporal symptom. The model incorporated the five temporal symptom items (coded as 0/1 for absence/presence of that symptom, respectively), diagnosis as a five-level factor, as well as age, total intracranial volume, and MMSE score as nuisance covariates. Negative (inverse) associations with regional gray matter (i.e., associations with gray matter atrophy) were assessed for every symptom item; positive gray matter associations were additionally assessed for symptoms of temporal rigidity, clockwatching, and re-living the past, since these phenomena are likely a priori to require at least partially preserved temporal processing mechanisms (58). Statistical parametric maps were generated using an initial threshold p < 0.001 and evaluated at peak voxel statistical significance level p < 0.05, after family-wise error (FWE) correction for multiple voxel-wise comparisons, separately within individual pre-specified neuroanatomical regions of interest. These regions were selected a priori based on functional neuroanatomical substrates of subjective time awareness identified in the healthy brain comprising anterior temporal lobe (the anterior parts of the superior, middle, inferior temporal, and fusiform gyri, and the temporal pole) (27, 79–81), insular cortex (2, 5, 13, 82), parietal cortex (inferior and superior parietal lobules, precuneus, and posterior cingulate cortex) (14–17, 83) and hippocampus (3, 19, 20). Regions were defined for the right and left hemispheres using the Harvard-Oxford Brain Atlas (http://fsl.fmrib.ox.ax.uk/fsl/fslwiki/Atlases). The regions used are presented in Figure S1.
Results
General Clinical and Neuropsychological Data
Participant groups (see Table 1) did not differ in age [F(5,134) = 1.31; p = 0.2619], nor handedness (p = 0.885); but differed significantly in gender balance [X2(5,140) = 11.204; p = 0.047] and years of education [X2(5,138) = 15.212; p = 0.0095]; the absolute difference was small, of the order of two years). Syndromic groups did not differ significantly in clinical illness duration [X2(4,103) = 8.43; p = 0.077]. However, there was a significant difference in MMSE scores [F (4,103) = 3.66; p = 0.0078], attributable in a post hoc analysis to significantly higher scores in the bvFTD group compared with the LPA group (p = 0.012).
Temporal Awareness Symptom Data
Data on the prevalence of temporal awareness alterations for all participant groups are summarized in Table 3. Examples of caregiver comments about patients' behavioral alterations are provided in Table S1. The logistic regression analysis comparing syndromic groups is presented in Table 4. Logistic regression analysis probing temporal symptom correlations are also presented in Table S2. Prevalence data for altered temporal awareness in individual patients with genetic mutations causing FTD are summarized in Table S3.
Raw prevalence data (Table 3) indicated that alterations of temporal awareness were frequent in all syndromic groups but experienced by only a small minority of healthy controls. Overall, patients with probable AD pathology (AD and LPA) most frequently exhibited confusion ordering past events or difficulty estimating intervals between events; while patients with FTD pathology (bvFTD, SD, PNFA) were most frequently prone to temporal rigidity, clockwatching and/or a tendency to re-live past events. Certain temporal symptoms were especially salient in particular syndromic groups (present in over half the cases in that group): event ordering confusion and/or difficulty estimating intervals between events in bvFTD, LPA, and AD; temporal rigidity and clockwatching in SD; and a tendency to re-live past events in bvFTD. Difficulties with interval estimation was significantly correlated with temporal rigidity, whereas no significant associations were found between these symptoms and a tendency to re-live past events (Table S2).
Compared to healthy controls, the patient cohort overall had a significantly higher prevalence of confusion about ordering events in time and difficulty estimating intervals between events (both p < 0.0001). In the logistic regression analysis comparing syndromic groups, there was a main effect of diagnosis for both confusion of temporal order [X2(4, 103) = 20.70, p = 0.0004] and interval estimation difficulties [X2(4, 103) = 16.29, p = 0.0026]. Such disturbances were significantly more prevalent in the AD group than the SD group [temporal ordering: odds ratio (OR) = 0.06, 95% confidence interval (CI) 0.01–0.35; temporal estimation: OR = 0.06, CI 0.01–0.31] and the PNFA group (temporal ordering: OR = 0.06, CI 0.01–0.30; temporal estimation: OR = 0.12, CI 0.03–0.49).
Compared to healthy controls, the patient cohort overall also had a significantly higher prevalence of increased temporal rigidity and clockwatching (both p < 0.001). There was a main effect of diagnosis for clockwatching [X2(4, 103) = 10.57, p = 0.0318] and temporal rigidity [X2(4, 103) = 12.98, p = 0.0114]. Clockwatching was significantly more prevalent in the bvFTD and SD groups than the AD group (bvFTD: OR = 4.44, CI 1.21–16.35; SD: OR = 8.98, CI 2.09–38.58). Temporal rigidity was significant more prevalent in the bvFTD, SD, and PNFA groups than the AD group (bvFTD: OR = 5.50, CI 1.24–24.40; SD: OR = 17.33, CI 3.30–91.09; PNFA: OR = 5.29, CI 1.11–25.14).
Compared to healthy controls, patient groups overall were significantly more likely to re-live past events (p < 0.001). However, while this symptom was more prevalent in the bvFTD and SD groups, the logistic regression analysis showed no significant main effect of diagnosis [X2(4,108) = 5.78, p = 0.22], precluding further comparisons between disease groups.
Across the patient cohort, symptoms of disturbed past event ordering, or interval estimation were significantly associated with MMSE score (OR = 0.92, CI 0.86–0.98 and OR = 0.91, 95% CI 0.86–0.97, respectively). No other significant associations between developing temporal awareness symptoms and general patient characteristics (gender, age, education, or relevant medication use) were identified.
Considering the small subgroup of patients with genetic mutations (Table S3), symptoms of altered time awareness were generally frequent with all major mutations causing FTD. However, temporal rigidity was particularly associated with MAPT mutations, contrasting with its low prevalence in association with C9orf72 and GRN mutations; no patients with GRN mutations were reported to have exhibited disturbances of temporal event ordering or interval estimation.
Neuroanatomical Associations of Altered Time Awareness
Significant gray matter associations of altered time awareness across the patient cohort are summarized in Table 5, all thresholded at pFWE < 0.05 within pre-specified anatomical regions of interest; statistical parametric maps are presented in Figure 1. Across the combined patient cohort, increased tendency to re-live past events was associated with relatively preserved gray matter in a distributed left-sided network including anterior middle temporal gyrus and superior temporal sulcus, hippocampus, posterior cingulate, and superior parietal cortices. No other neuroanatomical associations of altered temporal awareness were identified.
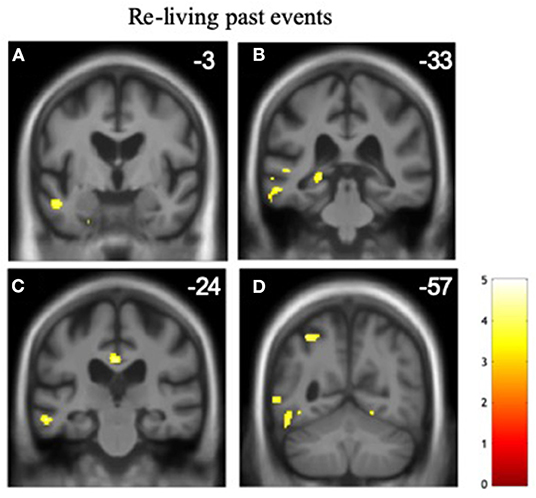
Figure 1. Gray matter associations of altered temporal awareness in the patient cohort. Statistical parametric maps show regional gray matter volume positively associated with the propensity to re-live past events across all syndromic groups (see text and Tables 2, 3 for details). For display purposes, maps have been rendered on coronal sections of the group mean template T1-weighted MR brain image and thresholded at p < 0.001, uncorrected for multiple voxel-wise comparisons over the whole brain volume (areas significant at pFWE < 0.05 after correction for multiple comparisons within pre-specified neuroanatomical regions of interest are summarized in Table 5); the y-coordinate (mm) in MNI space of the plane of each section is indicated. The color bar codes voxel-wise T scores. The left hemisphere is shown on the left in all sections. The sections traverse the following key structures: (A) anterior middle temporal gyrus/superior temporal sulcus. (B) posterior hippocampus. (C) posterior cingulate cortex. (D) superior parietal lobule.
Discussion
Here we have demonstrated alterations of multiple dimensions of long-duration, subjective time awareness in canonical syndromes of FTD, referenced to syndromes of AD as well as healthy older individuals. Abnormalities of time awareness were exhibited by over half of patients with bvFTD and SD as well as typical amnestic AD and LPA, and by around a third of patients with PNFA. However, the pattern of abnormalities was not uniform over the patient cohort: syndromic groups showed separable (albeit overlapping) profiles of altered time awareness, in line with our prior clinical hypotheses. Patients with typical AD and LPA had particularly salient difficulties with ordering past events and placing events in time, without significant changes in other aspects of temporal behavior sampled here; the pattern of temporal symptoms was much more heterogeneous in the FTD cohort. The SD group had prominent temporal rigidity and clockwatching, while the bvFTD group exhibited predominant abnormalities of past event ordering and re-living past events. Profiles of altered temporal awareness differed significantly between AD and FTD disease groups when directly compared and although there was an overall correlation of abnormal past event ordering and interval estimation with worsening disease severity, syndromic profiles of altered temporal awareness were evident after taking background clinical characteristics (age, gender, MMSE score) into account.
Informed by previous work in the healthy brain (84–87), we propose a tentative synthesis of these findings in terms of different “domains” of temporal awareness. Symptoms of abnormal event ordering or interval estimation might both plausibly reflect a disturbed mental timeline, while temporal rigidity and clockwatching reflect related aspects of mental timekeeping. The latter might be further related to the “Godot syndrome”, a phenomenon previously described in AD, and which refers to anxiety surrounding upcoming events (88, 89). Our findings corroborate previous reports of obsessional clockwatching in SD and bvFTD (31, 58) as well as time estimation difficulties in AD (46) but further illustrate that alterations of temporal awareness transcend canonical syndromic boundaries. Across syndromic groups, patients presenting with temporal rigidity, or clockwatching were less likely to also exhibit difficulty ordering past events and vice versa, but no association was found between those symptoms and a tendency to re-live past events. This is in line with the hypothesis that the propensity to re-live past events might constitute a partly compensatory phenomenon in the face of impoverished mental timeline, somewhat analogous to the normal phenomenon of “nostalgia” (77, 90).
Across the combined patient cohort, a tendency to re-live past events was associated with relative preservation of a distributed left-sided temporal, parietal, and hippocampal network. This is in line with an emerging picture of temporal processing and subjective temporal awareness derived from studies of the healthy brain. In particular, the parietal cortex has been implicated in reconstructing sequences of spatio-temporal events and their temporal ordering (15, 16, 91, 92). In conjunction with hippocampus as well as insular and prefrontal cortices, the parietal lobes participate in a distributed neural network that accesses and manipulates the mental timeline according to salience and behavioral context (82, 93, 94). This network further overlaps core elements of the default mode network which integrates information about current bodily states and memories with incoming sensory traffic. Moreover, the hippocampus is critical for initial encoding of events and their embedding in emotional context (3, 19, 20, 95), and the anterior temporal lobe is likely to be important for the semantic integration of autobiographical events (96). The integrity (or partial integrity) of these brain areas might plausibly support a tendency to re-live past autobiographical events, in line with previous evidence in neurodegenerative syndromes (97–99).
The lack of gray matter associations of other symptoms of altered temporal awareness here is, at first sight, a little surprising. However, there are several factors that might potentially account for these results. Firstly, the neurobiological status of the temporal awareness “symptoms” we considered here are different between symptom categories. Re-living of past events depends to some extent on a neuroanatomical substrate that is at least partly preserved (since temporal events have to be represented and accessed in order to be re-lived). It is therefore plausible that this substrate should be identified across syndromic groups, since it reflects the architecture of the healthy brain. On the other hand, we sampled other temporal symptoms that directly reflect the impact of neurodegenerative brain damage, which will have varied between syndromes and is therefore less likely to have a common neuroanatomical association across the patient cohort. In addition, it is possible that the neuroanatomical substrates of these other temporal symptoms are extensively distributed, widely variable between individuals or alternatively, highly convergent between symptom categories: any of these scenarios would have made neuroanatomical associations less liable to be identified using the VBM model we employed here. It is further plausible that at least some symptoms may arise from network connectivity changes that are not captured using VBM. Finally, the binarised symptom classification here may well have reduced scope to detect gray matter associations that might have been evident with a continuously distributed variable (e.g., a symptom severity score). It should be kept in mind that these factors are, to some extent, limitations imposed by the VBM technique itself (which is essentially a correlational methodology) rather than specific to temporal processing per se.
Furthermore, cellular and molecular as well as macro-anatomical factors are likely to influence temporal processing and subjective temporal awareness in neurodegenerative pathologies (100). The small case numbers here preclude firm conclusions concerning the temporal awareness profiles of particular genetic mutations. However, it is noteworthy that temporal rigidity and clockwatching were not reported in patients with GRN mutations, whereas these were salient symptoms in patients with MAPT mutations. It may be relevant that MAPT mutations target the antero-mesial temporal lobes relatively selectively (101) while GRN mutations frequently target parietal cortex (102). These issues will only be resolved by further neuroanatomical work addressing functional as well as structural network connectivity.
From a clinical perspective, our findings endorse the long-held bedside impression of temporal obsessionality in SD and bvFTD, while further corroborating reports of disordered temporal estimation in AD. Quantification of changes within the temporal symptom categories we have foregrounded here would help in planning, implementing and evaluating new behavioral interventions designed to help patients orient and navigate in time and to reduce patient and caregiver distress incurred by abnormal temporal reactivity. The overall correlation of mental timeline abnormalities with advancing disease noted here accords with previous suggestions that clockwatching behavior may be restricted to the earlier stages of SD (58). Our observations here could motivate further work to develop quantifiable cognitive tests of temporal awareness. Such tests might, in future studies, yield novel functional biomarkers that index the integrity of temporal processing mechanisms in neurodegenerative syndromes.
This study has several limitations that should inform future work. Most fundamentally, there is a need to further corroborate the results in larger patient cohorts and ideally with histopathological and molecular correlation. It would be of considerable interest to compare the profiles of altered temporal awareness exhibited by patients with FTD and AD directly with other neurodegenerative pathologies, for example Lewy body disease, which also target brain systems implicated in temporal perception. It will also be relevant to study these profiles longitudinally: the phenomenology of neurodegenerative syndromes is dynamic and multiphasic, while both in patients and healthy individuals, subjective temporal awareness may be modulated by key life events (such as retirement from work). The categories of temporal symptoms assessed in this first study were intentionally broad and qualitative, designed to capture a diverse range of phenomena. However, these symptom categories should be unpacked in further studies to quantify the frequency and severity of symptoms that patients experience and to capture the nature of their difficulties more precisely. For example, “difficulty” estimating the temporal intervals between events could mean simply that patients fail to take account of lapsed time or rather that they express unreasonable estimates of the relevant intervals. In turn, these processes might plausibly be affected differentially in different syndromes and diseases (in particular, AD vs. FTD). Particularly with regard to symptoms suggesting disturbances of the mental timeline, it will be crucial to define how such disturbances relate to deficits of episodic memory and the detail with which particular events and their spatio-temporal context are encoded. It will also be important to acquire patients' own reports of time awareness, ideally in parallel with caregivers' perspectives. Certain aspects of temporal awareness (for example, capacity to envisage the future) are intrinsically difficult to capture from second-person questionnaires but potentially highly illuminating in particular neurodegenerative syndromes [such as bvFTD and SD (53, 55)]. Our findings provide a prima facie case for the future design and validation of temporal symptom scales relevant to a broad range of neurodegenerative diseases.
Understanding the neural mechanisms of altered temporal awareness associated with these neurodegenerative pathologies will require a detailed assessment of temporal perception (in particular the psychophysical correlates of interval and pattern processing), consideration of accompanying behavioral phenotypes [since altered emotional reactivity is very likely to impact on temporal behaviors (7)], and functional neuroimaging techniques that can capture dynamic interactions and connectivity between brain network elements. In this regard, magnetoencephalography would be a particularly attractive modality, by virtue of its high temporal resolution and capacity to track changes in cortical laminar physiology. The last would offer the exciting prospect of relating complex temporal behavioral phenotypes to dysfunction at the level of tissue microcircuits and synapses, which is likely to be apposite in light of emerging evidence that neural mechanisms of temporal awareness span scales ranging from the cellular to the macroscopic (4, 5). Indeed, this is arguably a compelling motivation for developing true “temporal biomarkers,” since indices of universal brain processes (such as temporal processing) are likely to prevail across pathologies and disease stages.
This preliminary study calls attention to the significance of temporal awareness symptoms and pertinent neural network substrates across major dementia syndromes. The findings provide a rationale for a more systematic analysis of subjective time in neurodegenerative pathologies, with a view to developing validated clinical assessment tools, understanding underlying neurobiological mechanisms and designing management interventions.
Data Availability Statement
The datasets used and/or analyzed during the present study are available from the corresponding author on reasonable request.
Ethics Statement
The studies involving human participants were reviewed and approved by University College London and National Hospital for Neurology and Neurosurgery Joint Research Ethics Committees. The patients/participants provided their written informed consent to participate in this study.
Author Contributions
CM and JW were responsible for the conception and design of the study. CM and HS carried out symptom survey data collection. LR, CG, KM, EB, and JR were responsible for recruitment, neuropsychology, and imaging data acquisition. M-CR-K was responsible for the analysis of the survey data and M-CR-K, CM, CH, RB, and JA for the voxel-based morphometry analysis. M-CR-K, CM, and JW were responsible for the interpretation of the data. M-CR-K and JW were responsible for drafting the manuscript. M-CR-K, CM, CH, and JW for revisiting it critically for important intellectual content. All authors read and approved the final manuscript.
Funding
The Dementia Research Center was supported by Alzheimer's Research UK, Brain Research UK and the Wolfson Foundation. This work was funded by the Alzheimer's Society, Alzheimer's Research UK, the National Institute for Health Research University College London Hospitals Biomedical Research Center, and the UCL Leonard Wolfson Center for Experimental Neurology. Individual authors were supported by the Wellcome Trust (4-year Ph.D. Studentship in Neuroscience 102129/B/13/Z to M-CR-K; 091673/Z/10/Z to JW), the Wolfson Foundation (Clinical Research Fellowship previously to CM), Bart's Charity (MGU0366 to CM), the Medical Research Council (Ph.D. studentship to RB and previously to CH; MRC Clinician Scientist Fellowship MR/M008525/1 to JR), Alzheimer's Society (Ph.D. studentship AS-PhD-2015-005 to KM), Brain Research UK (4-year Ph.D. Studentship to EB), and Action on Hearing Loss (Dunhill Medical Trust Pauline Ashley Fellowship to CH).
Conflict of Interest
The authors declare that the research was conducted in the absence of any commercial or financial relationships that could be construed as a potential conflict of interest.
Acknowledgments
The authors are grateful to Dr. Jennifer Nicholas for her statistical advice, to Dr. Guillaume Flandin for his advice on choosing the appropriate model for the VBM analysis, and to all participants for their involvement in the study.
Supplementary Material
The Supplementary Material for this article can be found online at: https://www.frontiersin.org/articles/10.3389/fneur.2020.00291/full#supplementary-material
References
1. Buhusi CV, Oprisan SA, Buhusi M. Biological and cognitive frameworks for a mental timeline. Front Neurosci. (2018) 12:377. doi: 10.3389/fnins.2018.00377
2. Craig ADB. How do you feel — now? The anterior insula and human awareness. Nat Rev Neurosci. (2009) 10:59–70. doi: 10.1038/nrn2555
3. Eichenbaum H. On the integration of space, time, and memory. Neuron. (2017) 95:1007–18. doi: 10.1016/j.neuron.2017.06.036
4. Paton JJ, Buonomano DV. The neural basis of timing: distributed mechanisms for diverse functions. Neuron. (2018) 98:687–705. doi: 10.1016/j.neuron.2018.03.045
5. Wittmann M. The inner experience of time. Phil Trans R Soc B. (2009) 364:1955–67. doi: 10.1098/rstb.2009.0003
6. Wiener M, Matell MS, Coslett HB. Multiple mechanisms for temporal processing. Front Integr Neurosc. (2011) 5:31. doi: 10.3389/fnint.2011.00031
7. Droit-Volet S, Meck WH. How emotions colour our perception of time. Trends Cogn Sci. (2007) 11:504–13. doi: 10.1016/j.tics.2007.09.008
8. Timm J, Schonwiesner M, SanMiguel I, Schroger E. Sensation of agency and perception of temporal order. Conscious Cogn. (2014) 23:42–52. doi: 10.1016/j.concog.2013.11.002
9. van Wassenhove V, Wittmann M, Craig AD, Paulus MP. Psychological and neural mechanisms of subjective time dilation. Front Neurosci. (2011) 5:56. doi: 10.3389/fnins.2011.00056
10. Rubia K, Smith A. The neural correlates of cognitive time management: a review. Acta Neurolbiol Exp. (2004) 64:329–40.
11. Lewis PA, Miall RC. A right hemispheric prefrontal system for cognitive time measurement. Behav Proc. (2006) 71:226–34. doi: 10.1016/j.beproc.2005.12.009
12. Morillon B, Kell CA, Giraud AL. Three stages and four neural systems in time estimation. J Neurosci. (2009) 29:14803–11. doi: 10.1523/JNEUROSCI.3222-09.2009
13. Wittmann M, Simmons AN, Aron JL, Paulus MP. Accumulation of neural activity in the posterior insula encodes the passage of time. Neuropsychologia. (2010) 48:3110–20. doi: 10.1016/j.neuropsychologia.2010.06.023
14. Gonneaud J, Rauchs G, Groussard M, Landeau B, Mezenge F, de La Sayette V, et al. How do we process event-based and time-based intentions in the brain? an fMRI study of prospective memory in healthy individuals. Hum Brain Mapp. (2014) 35:3066–82. doi: 10.1002/hbm.22385
15. Franklin MS, Jonides J. Order and magnitude share a common representation in parietal cortex. J Cogn Neurosci. (2009) 21:2114–20. doi: 10.1162/jocn.2008.21181
16. Hayashi MJ, Kanai R, Tanabe HC, Yoshida Y, Carlson S, Walsh V, et al. Interaction of numerosity and time in prefrontal and parietal cortex. J Neurosci. (2013) 33:883–93. doi: 10.1523/JNEUROSCI.6257-11.2013
17. Kanayet FJ, Mattarella-Micke A, Kohler PJ, Norcia AM, McCandliss BD, McClelland JL. Distinct representations of magnitude and spatial position within parietal cortex during number-space mapping. J Cogn Neurosci. (2018) 30:200–18. doi: 10.1162/jocn_a_01199
18. O'Keefe J. A review of the hippocampal place cells. Prog Neurobiol. (1979) 13:419–39. doi: 10.1016/0301-0082(79)90005-4
19. Eichenbaum H. Time cells in the hippocampus: a new dimension for mapping memories. Nat Rev Neurosci. (2014) 15:732–44. doi: 10.1038/nrn3827
20. Lisman J, Buzsaki G, Eichenbaum H, Nadel L, Ranganath C, Redish AD. Viewpoints: how the hippocampus contributes to memory, navigation and cognition. Nat Neurosci. (2017) 20:1434–47. doi: 10.1038/nn.4661
21. Ferrandez AM, Hugueville L, Lehericy S, Poline JB, Marsault C, Pouthas V. Basal ganglia and supplementary motor area subtend duration perception: an fMRI study. Neuroimage. (2003) 19:1532–44. doi: 10.1016/S1053-8119(03)00159-9
22. Mello GBM, Soares S, Paton JJ. A scalable population code for time in the striatum. Curr Biol. (2015) 25:1113–22. doi: 10.1016/j.cub.2015.02.036
23. Soares S, Atallah BV, Paton JJ. Midbrain dopamine neurons control judgment of time. Science. (2016) 354:1273–7. doi: 10.1126/science.aah5234
24. Buhusi CV, Meck WH. What makes us tick? functional and neural mechanisms of interval timing. Nat Rev Neurosci. (2005) 6:755–65. doi: 10.1038/nrn1764
25. Wiener M, Turkeltaub P, Coslett HB. The image of time: a voxel-wise meta-analysis. NeuroImage. (2010) 49:1728–40. doi: 10.1016/j.neuroimage.2009.09.064
26. Matell MS, Meck WH. Cortico-striatal circuits and interval timing: coincidence detection of oscillatory processes. Cogn Brain Res.(2004) 21:139–70. doi: 10.1016/j.cogbrainres.2004.06.012
27. Stevens MC, Kiehl KA, Pearlson G, Calhoun VD. Functional neural circuits for mental timekeeping. Hum Brain Mapp. (2007) 28:394–408. doi: 10.1002/hbm.20285
28. Jin DZ, Fujii N, Graybiel AM. Neural representation of time in cortico-basal ganglia circuits. Proc Natl Acad Sci USA. (2009) 106:19156–61. doi: 10.1073/pnas.0909881106
29. Harrington DL, Zimbelman JL, Hinton SC, Rao SM. Neural modulation of temporal encoding, maintenance, and decision processes. Cereb Cortex. (2010) 20:1274–85. doi: 10.1093/cercor/bhp194
30. Buckner RL, Andrews-Hanna JR, Schacter DL. The brain's default network anatomy, function, and relevance to disease. Ann N Y Acad Sci. (2008) 1124:1–38. doi: 10.1196/annals.1440.011
31. Warren JD, Rohrer JD, Rossor MN. Clinical review. Frontotemporal dementia. BMJ. (2013) 347:f4827. doi: 10.1136/bmj.f4827
32. Warren JD, Rohrer JD, Schott JM, Fox NC, Hardy J, Rossor MN. Molecular nexopathies: a new paradigm of neurodegenerative disease. Trends Neurosci. (2013) 36:561–9. doi: 10.1016/j.tins.2013.06.007
33. Warren JD, Fletcher PD, Golden HL. The paradox of syndromic diversity in Alzheimer disease. Nat Rev Neurol. (2012) 8:451–64. doi: 10.1038/nrneurol.2012.135
34. Zhou J, Gennatas ED, Kramer JH, Miller BL, Seeley WW. Predicting regional neurodegeneration from the healthy brain functional connectome. Neuron. (2012) 73:1216–27. doi: 10.1016/j.neuron.2012.03.004
35. Seeley WW, Crawford RK, Zhou J, Miller BL, Greicius MD. Neurodegenerative diseases target large-scale human brain networks. Neuron. (2009) 62:42–52. doi: 10.1016/j.neuron.2009.03.024
36. Wallace M. Future time perspective in schizophrenia. J Abnorm Soc Psychol. (1956) 52:240–5. doi: 10.1037/h0039899
37. Zimbardo PG, Boyd JN. Putting time in perspective: a valid, reliable individual-differences metric. J Personality Soc Psychol. (1999) 77:1271–88. doi: 10.1037/0022-3514.77.6.1271
38. Wittmann M, Lehnhoff S. Age effects in perception of time. Psychol Rep. (2005) 97:921–35. doi: 10.2466/pr0.97.3.921-935
39. Crisci C, Caccavale M, Trojano L. Aging and the subjective experience of time. Aging clin Exp Res. (2016) 28:327–32. doi: 10.1007/s40520-015-0403-7
40. Levine B, Svoboda E, Hay JF, Winocur G, Moscovitch M. Aging and autobiographical memory: dissociating episodic from semantic retrieval. Psychol Aging. (2002) 17:677–89. doi: 10.1037/0882-7974.17.4.677
41. Irish M, Hornberger M, El Wahsh S, Lam BYK, Lah S, Miller L, et al. Grey and white matter correlates of recent and remote autobiographical memory retrieval – insights from the dementias. PLoS ONE. (2014) 9:e113081. doi: 10.1371/journal.pone.0113081
42. Irish M, Hornberger M, Lah S, Miller L, Pengas G, Nestor PJ, et al. Profiles of recent autobiographical memory retrieval in semantic dementia, behavioural-variant frontotemporal dementia, and Alzheimer's disease. Neuropsychologia. (2011) 49:2694–702. doi: 10.1016/j.neuropsychologia.2011.05.017
43. Addis DR, Sacchetti DC, Ally BA, Budson AE, Schacter DL. Episodic simulation of future events is impaired in mild Alzheimer's disease. Neuropsychologia. (2009) 47:2660–71. doi: 10.1016/j.neuropsychologia.2009.05.018
44. Nichelli P, Venneri A, Molinari M, Tavani F, Grafman J. Precision and accuracy of subjective time estimation in different memory disorders. Cogn Brain Res. (1993) 1:87–93. doi: 10.1016/0926-6410(93)90014-V
45. Rueda AD, Schmitter-Edgecombe M. Time estimation abilities in mild cognitive impairment and Alzheimer's disease. Neuropsychology. (2009) 23:178–88. doi: 10.1037/a0014289
46. El Haj M, Kapogiannis D. Time distortions in Alzheimer's disease: a systematic review and theoretical integration. NPJ Aging Mech Dis. (2016) 2:16016. doi: 10.1038/npjamd.2016.16
47. El Haj M, Moroni C, Samson S, Fasotti L, Allain P. Prospective and retrospective time perception are related to mental time travel: evidence from Alzheimer's disease. Brain Cogn. (2013) 83:45–51. doi: 10.1016/j.bandc.2013.06.008
48. El Haj M, Janssen SMJ, Antoine P. Memory and time: backward and forward telescoping in Alzheimer's disease. Brain Cogn. (2017) 117:65–72. doi: 10.1016/j.bandc.2017.06.005
49. Dermody N, Hornberger M, Piguet O, Hodges JR, Irish M. Prospective memory impairments in Alzheimer's disease and behavioral variant frontotemporal dementia: clinical and neural correlates. J Alzheimers Dis. (2016) 50:425–41. doi: 10.3233/JAD-150871
50. Wiener M, Coslett HB. Disruption of temporal processing in a subject with probable frontotemporal dementia. Neuropsychologia. (2008) 46:1927–39. doi: 10.1016/j.neuropsychologia.2008.01.021
51. Henley SM, Downey LE, Nicholas JM, Kinnunen KM, Golden HL, Buckley A, et al. Degradation of cognitive timing mechanisms in behavioural variant frontotemporal dementia. Neuropsychologia. (2014) 65:88–101. doi: 10.1016/j.neuropsychologia.2014.10.009
52. Irish M, Piolino P. Impaired capacity for prospection in the dementias–theoretical and clinical implications. Br J Clin Psychol. (2016) 55:49–68. doi: 10.1111/bjc.12090
53. Irish M, Eyre N, Dermody N, O'Callaghan C, Hodges JR, Hornberger M, et al. Neural substrates of semantic prospection - evidence from the dementias. Front Behav Neurosci. (2016) 10:96. doi: 10.3389/fnbeh.2016.00096
54. Maguire EA, Kumaran D, Hassabis D, Kopelman MD. Autobiographical memory in semantic dementia: a longitudinal fMRI study. Neuropsychologia. (2010) 48:123–36. doi: 10.1016/j.neuropsychologia.2009.08.020
55. Irish M, Hodges JR, Piguet O. Episodic future thinking is impaired in the behavioural variant of frontotemporal dementia. Cortex. (2013) 49:2377–88. doi: 10.1016/j.cortex.2013.03.002
56. McKinnon MC, Nica EI, Sengdy P, Kovacevic N, Moscovitch M, Freedman M, et al. Autobiographical memory and patterns of brain atrophy in frontotemporal lobar degeneration. J Cogn Neurosci. (2008) 20:1839–53. doi: 10.1162/jocn.2008.20126
57. Sivasathiaseelan H, Marshall CR, Agustus JL, Benhamou E, Bond RL, van Leeuwen JEP, et al. Frontotemporal dementia: a clinical review. Semin Neurol. (2019) 39:251–63. doi: 10.1055/s-0039-1683379
58. Nonaka T, Suto S, Yamakawa M, Shigenobu K, Makimoto K. Quantitative evaluation of changes in the clock-watching behavior of a patient with semantic dementia. Am J Alzheimers Dis Other Demen. (2014) 29:540–7. doi: 10.1177/1533317514523486
59. Coull JT, Vidal F, Nazarian B, Macar F. Functional anatomy of the attentional modulation of time estimation. Science. (2004) 303:1506–8. doi: 10.1126/science.1091573
60. Macar F, Coull JT, Vidal F. The supplementary motor area in motor and perceptual time processing: fMRI studies. Cogn Process. (2006) 7:89–94. doi: 10.1007/s10339-005-0025-7
61. Mita A, Mushiake H, Shima K, Matsuzaka Y, Tanji J. Interval time coding by neurons in the presupplementary and supplementary motor areas. Nat Neurosci. (2009) 12:502–7. doi: 10.1038/nn.2272
62. Dubois B, Feldman HH, Jacova C, Hampel H, Molinuevo JL, Blennow K, et al. Advancing research diagnostic criteria for Alzheimer's disease: the IWG-2 criteria. Lancet Neurol. (2014) 13:614–29. doi: 10.1016/S1474-4422(14)70090-0
63. Rascovsky K, Hodges JR, Knopman D, Mendez MF, Kramer JH, Neuhaus J, et al. Sensitivity of revised diagnostic criteria for the behavioural variant of frontotemporal dementia. Brain. (2011) 134:2456–77. doi: 10.1093/brain/awr179
64. Gorno-Tempini ML, Hillis AE, Weintraub S, Kertesz A, Mendez MF, Cappa SF, et al. Classification of primary progressive aphasia and its variants. Neurology. (2011) 76:1006–14. doi: 10.1212/WNL.0b013e31821103e6
65. Ho MY, Velasquez-Martinez DN, Bradshaw CM, Szabadi E. 5-hydroxytryptamine and interval timing behaviour. Pharmacol Biochem Behav. (2002) 71:773–85. doi: 10.1023/A:1015816827946
66. Tomassini A, Ruge D, Galea JM, Penny W, Bestmann S. The role of dopamine in temporal uncertainty. J Cogn Neurosci. (2016) 28:96–110. doi: 10.1162/jocn_a_00880
68. Delis DC, Kaplan E, Kramer JH. Delis-Kaplan Executive Function System (D-KEFS). Psychological Corporation (2001).
69. Jackson M, Warrington EK. Arithmetic skills in patients with unilateral cerebral lesions. Cortex. (1986) 22:611–20. doi: 10.1016/S0010-9452(86)80020-X
70. McKenna P, Warrington EK. Testing for nominal dysphasia. J Neurol Neurosurg Psychiatry. (1987) 43:781–8. doi: 10.1136/jnnp.43.9.781
71. Folstein M, Folstein S, McHugh P. “Mini-mental state”: a practical method for grading the cognitive state of patients for the clinician. J Psychiatr Res. (1975) 12:189–98. doi: 10.1016/0022-3956(75)90026-6
73. Tombaugh TN. Trail making test A and B: normative data stratified by age and education. Arch Clin Neuropsychol. (2004) 19:203–14. doi: 10.1016/S0887-6177(03)00039-8
74. Warrington EK, James M. The Visual Object And Space Perception Battery. Bury St. Edmunds: Thames Valley Test Company (1991).
75. Wechsler D. Wechsler Adult Intelligence Scale-Revised. New York, NY: Psychological Corporation (1981).
76. Wechsler D. Wechsler Memory Scale: Revised. San Antonio, TX: The Psychological Corporation (1987).
77. Benjamini Y, Yekutieli D. The control of the false discovery rate in multiple testing under dependency. Ann Stat. (2001) 29:1165–88. doi: 10.1214/aos/1013699998
78. Ridgway GR, Henley SM, Rohrer JD, Scahill RI, Warren JD, Fox NC. Ten simple rules for reporting voxel-based morphometry studies. Neuroimage. (2008) 40:1429–35. doi: 10.1016/j.neuroimage.2008.01.003
79. Tracy JI, Faro SH, Mohamed FB, Pinsk M, Pinus A. Functional localization of a “Time Keeper” function separate from attentional resources and task strategy. Neuroimage. (2000) 11:228–42. doi: 10.1006/nimg.2000.0535
80. Bueti D, Walsh V, Frith C, Rees G. Different brain circuits underlie motor and perceptual representations of temporal intervals. J Cogn Neurosci. (2008) 20:204–14. doi: 10.1162/jocn.2008.20017
81. Ballotta D, Lui F, Porro CA, Nichelli PF, Benuzzi F. Modulation of neural circuits underlying temporal production by facial expressions of pain. PLoS ONE. (2018) 13:e0193100. doi: 10.1371/journal.pone.0193100
82. Apaydin N, Ustun S, Kale EH, Celikag I, Ozguven HD, Baskak B, et al. Neural mechanisms underlying time perception and reward anticipation. Front Hum Neurosci. (2018) 12:115. doi: 10.3389/fnhum.2018.00115
83. Hayashi MJ, Ditye T, Harada T, Hashiguchi M, Sadato N, Carlson S, et al. Time adaptation shows duration selectivity in the human parietal cortex. PLoS Biol. (2015) 13:e1002262. doi: 10.1371/journal.pbio.1002262
84. Pathman T, Coughlin C, Ghetti S. Space and time in episodic memory: effects of linearity and directionality on memory for spatial location and temporal order in children and adults. PLoS ONE. (2018) 13:e0206999. doi: 10.1371/journal.pone.0206999
85. Brunec IK, Chadwick MJ, Javadi AH, Guo L, Malcolm CP, Spiers HJ. Chronologically organized structure in autobiographical memory search. Front Psychol. (2015) 6:338. doi: 10.3389/fpsyg.2015.00338
86. Bonato M, Zorzi M, Umilta C. When time is space: evidence for a mental time line. Neurosci Biobehav Rev. (2012) 36:2257–73. doi: 10.1016/j.neubiorev.2012.08.007
87. Bernsten D, Jacobsen AS. Involuntary (spontaneous) mental time travel into the past and future. Conscious Cogn. (2008) 17:1093–104. doi: 10.1016/j.concog.2008.03.001
88. Kloszewska I. Incidence and relationship between behavioral and psychological symptoms in Alzheimer's disease. Int J Geriat Psychiatry. (1998) 13:785–92. doi: 10.1002/(sici)1099-1166(1998110)13:11<785::aid-gps872>3.0.co;2-u
89. Reisberg B, Borenstein J, Franssen E, Shulman E, Steinberg G, Ferris SH. Remediable behavioral symptomatology in Alzheimer's disease. Hosp Commun Psychiatry. (1986) 37:1199–201. doi: 10.1176/ps.37.12.1199
90. Ismail S, Christopher G, Dodd E, Wildschut T, Sedikides C, Ingram TA, et al. Psychological and mnemonic benefits of nostalgia for people with dementia. J Alzheimers Dis. (2018) 65:1327–44. doi: 10.3233/JAD-180075
91. Manelis A, Popov V, Paynter C, Walsh M, Wheeler ME, Vogt KM, et al. Cortical networks involved in memory for temporal order. J Cogn Neurosci. (2017) 29:1253–66. doi: 10.1162/jocn_a_01123
92. Lieberman JS, Kyle CT, Schedlbauer A, Stokes J, Elkstrom AD. A tale of two temporal coding strategies: common and dissociable brain regions involved in recency versus associative temporal order retrieval strategies. J Cogn Neurosci. (2017) 29:739–54. doi: 10.1162/jocn_a_01081
93. Murai Y, Yotsumoto Y. Context-dependent neural modulations in the perception of duration. Front Int Neurosci. (2016) 10:12. doi: 10.3389/fnint.2016.00012
94. Andreou LV, Griffiths TD, Chait M. Sensitivity to the temporal structure of rapid sound sequences — an MEG study. Neuroimage. (2015) 110:194–204. doi: 10.1016/j.neuroimage.2015.01.052
95. Çalişkan G, Stork O. Hippocampal network oscillations as mediators of behavioural T metaplasticity: insights from emotional learning. Neurobiol Learn Mem. (2018) 154:37–53. doi: 10.1016/j.nlm.2018.02.022
96. Strikwerda-Brown C, Grilli MD, Andrews-Hanna J, Irish M. “All is not lost”—rethinking the nature of memory and the self in dementia. Ageing Res Rev. (2019) 54:100932. doi: 10.1016/j.arr.2019.100932
97. Irish M, Piguet O, Hodges JR, Hornberger M. Common and unique gray matter correlates of episodic memory dysfunction in frontotemporal dementia and Alzheimer's disease. Hum Brain Mapp. (2014) 35:1422–35. doi: 10.1002/hbm.22263
98. Yamashita K-I, Uehara T, Prawiroharjo P, Yamashita K, Togao O, Hiwatashi A, et al. Functional connectivity change between posterior cingulate cortex and ventral attention network relates to the impairment of orientation for time in Alzheimer's disease patients. Brain Imaging Behav. (2019) 13:154–61. doi: 10.1007/s11682-018-9860-x
99. Serra L, Bozzali M, Fadda L, De Simone MS, Bruschini M, Perri R, et al. The role of hippocampus in the retrieval of autobiographical memories in patients with amnestic mild cognitive impairment due to Alzheimer's disease. J Neuropsychol. (2018) 14:46-68. doi: 10.1111/jnp.12174
100. Wiener M, Lohoff FW, Coslett HB. Double dissociation of dopamine genes and timing in humans. J Cogn Neurosci. (2011) 23:2811–21. doi: 10.1162/jocn.2011.21626
101. Rohrer JD, Lashley T, Schott JM, Warren JE, Mead S, Isaacs AM, et al. Clinical and neuroanatomical signatures of tissue pathology in frontotemporal lobar degeneration. Brain. (2011) 134:2565–81. doi: 10.1093/brain/awr198
Keywords: time perception, clockwatching, Alzheimer's disease, frontotemporal dementia, primary progressive aphasia, semantic dementia, voxel-based morphometry
Citation: Requena-Komuro M-C, Marshall CR, Bond RL, Russell LL, Greaves C, Moore KM, Agustus JL, Benhamou E, Sivasathiaseelan H, Hardy CJD, Rohrer JD and Warren JD (2020) Altered Time Awareness in Dementia. Front. Neurol. 11:291. doi: 10.3389/fneur.2020.00291
Received: 12 December 2019; Accepted: 26 March 2020;
Published: 21 April 2020.
Edited by:
Görsev Yener, Dokuz Eylül University, TurkeyReviewed by:
Alberto Benussi, University of Brescia, ItalyHakan Gurvit, Istanbul University, Turkey
Copyright © 2020 Requena-Komuro, Marshall, Bond, Russell, Greaves, Moore, Agustus, Benhamou, Sivasathiaseelan, Hardy, Rohrer and Warren. This is an open-access article distributed under the terms of the Creative Commons Attribution License (CC BY). The use, distribution or reproduction in other forums is permitted, provided the original author(s) and the copyright owner(s) are credited and that the original publication in this journal is cited, in accordance with accepted academic practice. No use, distribution or reproduction is permitted which does not comply with these terms.
*Correspondence: Maï-Carmen Requena-Komuro, bWFpLWNhcm1lbi5yZXF1ZW5hLWtvbXVyby4xN0B1Y2wuYWMudWs=
†These authors have contributed equally to this work and share first authorship