- 1Movement Disorders Clinic, Department of Neurosciences, Bambino Gesù Children's Hospital, Rome, Italy
- 2Department of Neuroscience, University of Rome Tor Vergata, Rome, Italy
Tourette syndrome (TS) is a neurodevelopmental disorder characterized by motor and vocal tics. Co-occurrence of attention-deficit/hyperactivity disorder (ADHD) or obsessive–compulsive disorder (OCD) is very frequent in the pediatric population as well as the presence of an impairment of the executive functions. The aim of our study was to investigate motor timing, that is, the temporal organization of motor behavior, in a pediatric population of Tourette patients. Thirty-seven Tourette patients (divided in 22 “pure” Tourette patients and 15 with ADHD) were compared with 22 healthy age- and gender-matched subjects. All subjects underwent a neuropsychiatric screening and were tested for their planning and decision-making abilities by using a standardized test, such as Tower of London (ToL). Two experimental paradigms were adopted: finger-tapping test (FTT), a free motor tapping task, and synchronization–continuation task. An accuracy index was calculated as measure of ability of synchronization. We found that “pure” TS as well as TS+ADHD showed lower scores in the FTT for the dominant and non-dominant hands than controls. Moreover, in the synchronization and continuation test, we observed an overall lack of accuracy in both TS groups in the continuation phase for 2,000 ms (supra-second interval), interestingly, with opposite direction of accuracy index. Thus, “pure” TS patients were classified as “behind the beat,” whereas, TS+ADHD as “ahead of the beat.” The performance in the finger tapping was inversely correlated to ToL total scores and execution time, whereas we did not find any correlation with the accuracy index of the synchronization and continuation test. In conclusion, here, we explored motor timing ability in a childhood cohort of Tourette patients, confirming that patients exhibit an impaired temporal control of motor behavior and these findings may be explained by the common underlying neurobiology of TS and motor timing.
Introduction
Tourette syndrome (TS) is a childhood-onset neurodevelopmental movement disorder clinically characterized by the presence of multiple motor tics and one or more phonic/vocal tics that last for more than 1 year (1). The age at onset of TS ranges from 2 to 21 years, and the mean age is 5–7 years, with males suffering more than females in a ratio of 3–4:1 (2). Pure TS patients, referring to patients with TS without any other comorbid conditions, are relatively uncommon (3); attention-deficit/hyperactivity disorder (ADHD) or obsessive–compulsive disorder (OCD) is commonly associated (4), but other several clinical and subclinical conditions, such as explosive outbursts, conduct problems, anxiety, self-injurious behavior, and depression, could run the clinical course of the disease (5–7). Moreover, cognitive functions, and in particular, executive ones, including inhibition/attention, working memory, planning ability, and problem solving, have been reported to be impaired in TS (8), although with conflicting results (9, 10), probably because it is difficult to distinguish the role of comorbid conditions in this framework.
Time is an intriguing ability of humans and deserves several adaptive and behavioral responses to changing environment (11). Time processing is a multifaceted decoding ability of the brain depending on several factors including time intervals, reproduction of intervals, as well as estimation of a duration. Nevertheless, basic cognitive functions, such as working memory, attention, and decision making, deeply modulate and regulate time coding. Thus, although ultimate neural mechanisms are far to be completely elucidated, theories, widely accepted, on the psychological and anatomical components of interval timing are based on the neurobiological model of an “internal clock” (12), which consists of an internal pacemaker connected via a decision mechanism to previously important duration codes held in reference memory. Functional imaging studies in humans and lesional studies in animals pointed out the role of basal ganglia nuclei and cortico-striato-thalamo-cortical (CSTC) circuitry (13), as well as it seems that the model crucially depends on the striatal integration of oscillating cortical activity (14).
Despite its multifactorial and unknown etiology, recent studies suggest that a dysfunction of the CSTC circuits in TS leads to disinhibition and other dysfunctions in executive functioning (15, 16). Noteworthy, basal ganglia connection to the prefrontal cortex could be the neurobiological basis of impaired motor and non-motor inhibitory control, one of the key futures of TS as recently Morand-Bealieu et al. argued in a comprehensive meta-analysis (17). Distortion in motor and perceptual timing is present in many neurological and psychiatric conditions (18–21). Temporal processing has been studied also in movement disorders in both hypokinetic conditions, such as Parkinson's disease (22), as well as hyperkinetic disorders, including Huntington disease, essential tremor, and dystonia (23, 24). Findings clearly suggest that the basal ganglia network is involved in explicit motor and perceptual timing and implicit timing as well. TS has been investigated mainly for perceptual aspects of timing (23, 25, 26), meaning the ability to estimate temporal intervals measured by task of duration discrimination, duration estimation, and duration reproduction (27). Data show a reduction of accuracy on time reproduction tasks for supra-second intervals, with performance variability influenced by dopamine D2 receptor antagonists (23, 28). On the contrary, few studies have been conducted in children with TS elucidating mechanisms of motor timing. Motor timing, referring to the temporal organization of motor behavior, is a pivotal functional domain influencing the efficiency and the correctness to the context of any motor output (29). Recently, motor timing skills were investigated by Martino et al. in an adult cohort (29) and in 2011 by Avanzino et al. in a pediatric cohort (30). Nevertheless, in healthy infants, the existence of a primitive “sense” of time that changes and develops throughout childhood is well known (31), making difficult studying time in children but, likewise, even more appealing.
The ability to suppress tics, as well as to uncouple the premonitory urge sensation and tics, together with the possibility to train this capacity with appropriate techniques, reveals that motor timing plays an underestimated role in TS evaluation and management.
The aim of our study was to investigate motor timing processing in a cohort of children affected by TS with and without comorbidity compared with a healthy group of controls.
Materials and Methods
Subjects
Thirty-seven patients with TS diagnosed according to the Diagnostic and Statistical Manual of Mental Disorders (DSM)-5 criteria were prospectively recruited from the outpatient Movement Disorder Clinic of Bambino Gesù Children's Hospital of Rome. Inclusion criteria were (2) a defined diagnosis of TS, (3) age between 7 and 17 years old, and (4) no other neurological or general comorbidities. All TS patients were drug free from the previous 6 months before the study. Age- and sex-matched 26 healthy controls were enrolled by using a school-based recruitment call. Four of 26 healthy controls were excluded from the analysis because the neuropsychiatric screening resulted positive. The ethnicity of the entire sample was all Caucasian. Among the 37 TS patients, 15 subjects met the criteria of DSM-5 for ADHD; the remaining 22 subjects, on the other hand, had no DSM-5 diagnosis of ADHD. We consequently sub-grouped TS patients in two separate groups: TS+ADHD (n = 15) and “pure” TS (n = 22).
Procedure
Participants were tested individually in a quiet room in the Movement Disorder Clinic of the Department of Neuroscience; tests administration was performed with clinical feasibility to avoid fatigue, with frequent breaks and chats with the children along the evaluation, in order to make them feel more comfortable and increase the focus on the activities. Each session entirely lasted from 2 to 3 h. For the computer-based tests, we used a 17-inch laptop for the presentation of stimuli and recording the responses by the participants. The LCD screen had a resolution of 1,440 × 900 pixels and a refresh rate of 60 Hz. A standard Italian keyboard was used as response keys. The background luminance of the screen was a mid-level gray measured at a viewing distance of 65 cm.
Measures Neuropsychiatric Screening
All subjects underwent a structured neuropsychological evaluation. They were tested by either a neuropsychologist or a child neuropsychiatrist. We performed a screening for neuropsychiatric comorbidities using parent report questionnaire to assess ADHD, obsessions and compulsions disorder, anxiety disorder, mood disorder, and conduct disorder (Child Behavior Checklist [CBCL] and Conners' Parent Rating Scale [CPRS]). Children's Yale–Brown Obsessive–Compulsive Scale (CY-BOCS) was used for obsessions and compulsions severity score. Furthermore, a structural neuropsychological evaluation including (2) a non-verbal cognitive test assessing fluid intelligence (Raven's Progressive Matrices) and (3) a spatial problem-solving and planning task (Tower of London [ToL] test) was performed. All TS patients were tested for tics severity and impairment using a questionnaire interview, the Yale Global Tic Severity Scale (YGTSS). For a detailed description of the tests, see Supplementary Materials.
Motor Timing Assessment
To assess motor timing, we used the finger-tapping test (FTT) and the sensorimotor test (SM), performed using the Inquisit 5.0 software (Millisecond®) downloaded on a laptop computer. Inquisit is a software widely used for experimental psychology (see www.millisecond.com for the latest research papers with Inquisit); in the Millisecond® website, a test library is provided with downloadable tests (and scripts) including those used for this study. For the SM tones at 500 Hz and 70 dB, sound pressure level (SPL) (duration 50 ms) were delivered through headphones. Subjects performed a single hand task.
Finger-Tapping Test
We used a script by Katja Borchert of Millisecond Software® (https://www.millisecond.com/download/library/v6/fingertapping/fingertapping/fingertapping.manual), adapted from the original Finger Tapping Oscillation Test part of Halstead's test battery of 1947 and later modified (32, 33). The FTT is a self-directed motor-speed test: participants have to tap with the index finger of both the dominant hand (DH) and the non-dominant hand (n-DH) as often they can within 10 s. Participants run through a mandatory number of 5 rounds (=blocks) of 10 s each. If the scores of these first 5 rounds were within 5 taps of each other, the final score was the mean of the number of taps of these 5 rounds (expressed as number of taps/10 s). On the other hand, if the scores of these 5 rounds were not within 5 taps of each other, an additional block was run, until 5 scores could be found that were within a 5-point range. The final score was the mean of these 5 scores for both the DH and the non-DH. The maximum number of rounds was 10. If no 5 scores could be found that are within a 5-point range from each other, the final score was the mean of all 10 tapping scores. After each round of testing, participants received feedback (number of taps) and got at least a 10-s rest period. After every 3 testing rounds, this resting period was increased to 60 s. We measured the final score for the DH and the final score for the non-DH.
Sensorimotor Synchronization Test
We used a script available from Millisecond Software® Library (https://www.millisecond.com/download/library/v6/timeestimation/pacedmotortiming/pacedmotortiming.manual) implementing a Paced Motor Timing procedure described by Wittmann et al. (19). In the SM test, the participant needed to synchronize responses with a series of 20 pacer signals (beeps, 500 Hz, 50 ms durations) for two stimuli, i.e., 1000 and 2000 ms (intertone intervals). The experimental procedure consisted of two different conditions (Figure 1): (a) in Condition A (synchronization subtest), the pacer signal was played for all test trials, and the participants had to synchronize their responses (spacebar press on the keyboard) with a steady series of the pacer signals. (b) In Condition B (continuation subtest), the pacer signals were played for the first 10 tones at the beginning of the trial; after 10 tones, the beat stopped, and the participants were asked to continue tapping (spacebar keyboard press) at the same rhythm until the end without the pacer signal. The continuation tapping was performed for 20 taps. The two conditions were tested in blocked format with Condition A running first. Stimuli were sampled randomly within each condition. Variables considered in the analysis were: tap intervals (TI) expressed as mean (±SD) of time intervals in ms between taps as measure of tapping speed for both Condition A and Condition B. For Condition A, we also considered the invalid responses (IRs) as the number of motor reactions (responses after 120 ms from the onset of tone) per trial as a further measure of synchronization ability. For Condition B, we considered TI expressed as mean (±SD) of time intervals in ms between taps. Additionally, for both Conditions A and B, we calculated an accuracy index (Δi) as follows: subjective time (TI)/t where subjective time was the TI produced experimentally by the subject, and t is the objective time, i.e., the base interval set (1000 or 2000 ms; Δi1000 and Δi2000, respectively). This index provided the directionality of the tapping performance, being >0 if the subject was behind the beat and <0 if the subject is ahead of the beat; moreover, it was a direct measure of the magnitude of the error in reproducing the corresponding time interval. To complete the two subtests, it takes approximately 6 min. Δi was used in all statistical analysis performed.
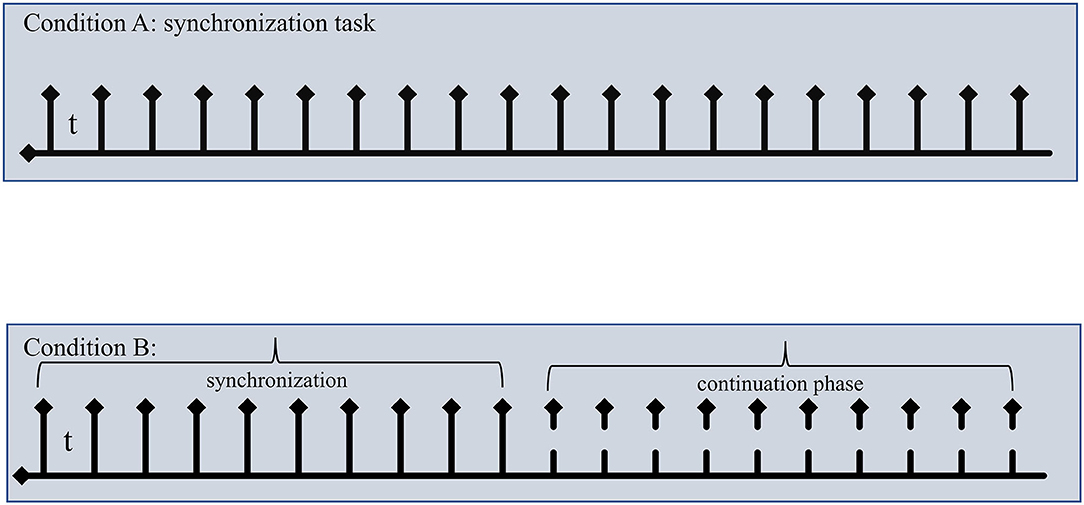
Figure 1. A schematic representation of sensorimotor synchronization task. Top: In Condition A, subjects were asked to tap in synchrony to “beep. Bottom: in Condition B, subjects tapped in synchrony for the first 10 taps, then beeps stopped, and subjects continued to tap at same tempo in the so-called continuation phase (the last 10 taps). The last 10 taps for both conditions were used in the analysis. t: intertone interval 1000 ms or 2000 ms; solid arrow indicated taps in the synchronization phase; dashed arrows indicated when beep stopped.
Statistical Analysis
Results are reported as mean ± SD. Normal distribution was assessed by Shapiro–Wilk test and Levine test for normality. Differences between means were assessed using unpaired two-tailed t test (where appropriate) and analysis of covariance (ANCOVA) (considering “age” as covariate) with Bonferroni post-hoc test for comparison between the groups, at 95% CI. For finger-tapping results, we used a two-way ANOVA, in which GROUP was analyzed as between-subjects factor, and HAND (DH vs. n-DH) as within-subjects factor. Post-hoc comparisons were performed using the Bonferroni post-hoc test. Linear least-square regression and generalized linear model (GLM) analyses were used in the selection of predictors. GLM estimation was performed by stepwise method with 95% CI. Significance was assigned for p < 0.05. All analyses were performed using SYSTAT software version 13.0 for Windows.
Results
Demographic Characteristics
Twenty-two pediatric healthy controls and 37 TS patients were included in the analysis. In the patients' group, 60% had a diagnosis of TS without any comorbid condition (pure TS, n = 22) and 40% with ADHD comorbidity (TS+ADHD, n = 15). Male to female ratio was 3:1 in both groups. All patients and healthy controls did not take any medication. Participants' ages range between 8 and 14 years old, and no statistical significance was found between the groups (ANOVA-F[2,56] = 0.85; p = 0.43). IQ levels were 108.3 ± 13.4 in the “pure” TS group, 110.1 ± 12.1 in the TS+ADHD group, and 114.5 ± 11.7 in the control group, showing no statistical significance between the groups (ANCOVA-F[2,55] = 1.77; p = 0.39, corrected for age). Total mean score of the YGTSS rating scale for all TS was 41.3 ± 15.4 (maximum score 100). The two TS groups did not differ for YGTSS scores (40.9 ± 14.3 vs. 41.9 ± 17.4 for “pure” TS and TS+ADHD, respectively; t = 0.182, df 35; p = 0.85, 95% CI). Total mean score of the CY-BOCS rating scale for all TS patients was 8.6 ± 1.3 (maximum score 40); particularly, the range of severity of patients with obsessions and compulsions was subclinical in 32/37 (86.5%), mild in 2/37 (5.4%), moderate in 1/37 (2.7%), and severe in 2/37 (5.4%). CBCL and CPRS scores showed statistical differences between “pure” TS and TS+ADHD in several domains including those related to ADHD comorbidity. Table 1 resumes all clinical characteristics of the population in the study.
Motor Timing
Finger-Tapping Test
In the FTT, DH as well as non-DH were tested. There was a statistical significance difference in mean number of taps (number of taps/trial) performed with DH between the three groups (F[2,55] = 6.849; p = 0.002). In particular, we found a lower mean number of taps in both the Tourette groups, “pure” TS and TS+ADHD, respectively, 56.29 ± 5.78 (SD) (p = 0.03 post-hoc Bonferroni test) and 54.94 ± 7.03 (SD) (p < 0.01 post-hoc test) vs. controls, 63.28 ± 8.42 (SD). No statistical difference was found between “pure” TS and TS+ADHD. In non-DH trials, the “pure” TS group differed statistically from controls (respectively, 48.62 ± 13.31 vs. 55.81 ± 7.99; p = 0.043 post-hoc Bonferroni test), whereas no difference was found between controls and TS+ADHD (48.97 ± 8.70 vs. 55.81 ± 7.99). Considering in the model “HAND” as factor within each level (GROUP), we found that both controls and “pure” TS patients showed a statistically significant reduction with non-DH compared with DH (DH vs. non-DH, p < 0.01 post-hoc Bonferroni test). We did not find any difference in TS+ADHD between DH and non-DH.
Sensorimotor Test
In the SM task, we tested the ability of subjects to synchronize their taps with pacer signals with or without played beep (Conditions A and B, see Materials and Methods section for details). An accuracy index (Δi) was calculated as described in the Materials and Methods section to directly assess the accuracy of synchronization along with the directionality (earlier or later) of tapping. Additionally, in Condition A, we considered IRs as adjunctive measures of synchronization. In Condition A (paced signals) for the interval (t) tested, 1,000 ms, all groups did not differ in a statistical manner. Δi1,000 values were 1.04 ± 0.04 for controls, 1.02 ± 0.05 for “pure” TS, and 1.02 ± 0.06 for TS+ADHD, indicating a good synchronization accuracy (Δi = 1). Mean number of IRs in each group per trial (reactions) confirmed the data. For the interval t, 2,000 ms, we did not find any statistical difference between the groups (F[2,55] = 0.65; p = 0.52). Mean number of IRs confirmed the lack of difference between the groups. Table 2 shows the results expressed as TI for each group. In Condition B (unpaced signals), Δi1,000 did not differ significantly between the three groups (F[2,55] = 0.20; p = 0.55). Conversely, in the task for 2,000 ms, both TS groups showed a statistical difference compared with controls (Δi2,000 = 1.01 ± 0.12; F[2,55] = 14.2; p < 0.001), adjusted for age; in particular, “pure” TS showed a Δi2,000 value >1 (1.21 ± 0.25; p = 0.035 vs. controls; Bonferroni post-hoc test 95% CI), indicating a direction of synchronization “behind the beat,” whereas TS+ADHD' Δi2,000 was <1 (0.84 ± 0.22; p = 0.035 vs. controls; Bonferroni post-hoc test 95% CI), placing these patients significantly “ahead of the beat.” A statistical significance was found comparing “pure” TS and TS+ADHD (p < 0.001). Data are summarized in Table 2, Figure 2.
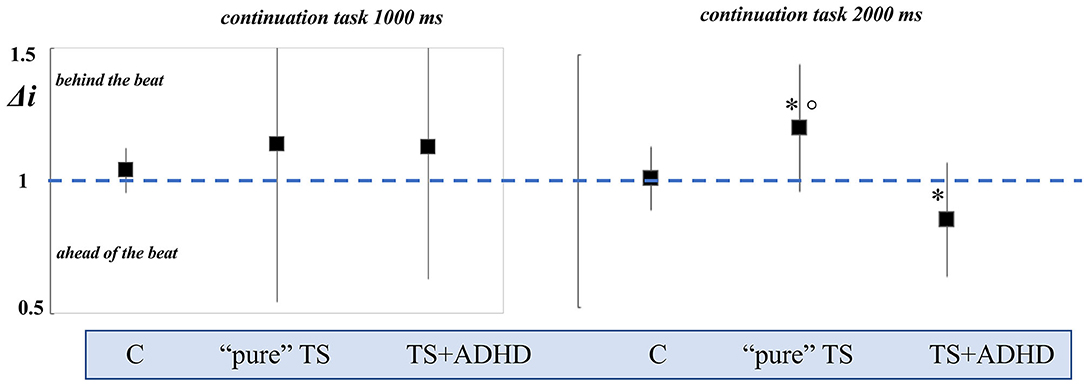
Figure 2. Representation of accuracy index (Δi) in Condition B (synchronization and continuation task) for t = 1,000 ms and for t = 2,000 ms. The Δi value is 1 in the iso-synchrony performance (dashed blue line); values >1 or <1 indicate the direction of continuation tapping, “behind the beat” or” ahead of the beat,” respectively. “Pure” TS and TS+ADHD patients showed a lack of accuracy in the continuation task for 2,000 ms, with opposite directions. “Pure” TS: Tourette syndrome only; TS+ADHD: Tourette syndrome plus; C: controls; *p < 0.05 Bonferroni post-hoc test with 95% CI vs. controls; °p < 0.001 Bonferroni post-hoc test with 95% CI “pure” TS vs. TS+ADHD.
Planning and Decision-Making Abilities
We measured planning and decision-making abilities using the standardized ToL test. Results are summarized in Table 3. Briefly, we considered the total score achieved, the mean solution time, the mean execution time, and the time of the first move (as measure of impulsivity). “Pure” TS and TS+ADHD differed from controls (p < 0.001) for the total score, with no effect of age in the model. As regards the total execution time, we found a statistical difference between the three groups. Post-hoc analysis showed a statistical significance between TS+ADHD and controls (p < 0.001), whereas no differences were found between “pure” TS and controls. Additionally, TS+ADHD patients showed statistically lower mean solution time values than controls (p = 0.045). No differences were found in the time of first move between the groups.
Correlation Analysis
Comparing the finger tapping scores with the IQ and tics severity (YGTSS), we did not find any correlation (p > 0.05), suggesting that tic severity did not impair per se motor performance. IQ and YGTSS did not correlate also with accuracy index for sensorimotor continuation test (Δi2,000). We addressed the interplay between motor timing and ToL (planning and decision-making abilities). We used a GLM to statistically test this hypothesis. In the first model, we assigned as dependent variable the “mean of finger tapping” (DH) of FTT and as independent variables “groups” (controls, “pure” TS, and TS+ADHD), the mean execution time, the total score, and interactions between them (group × mean execution time; group × total score). We found that FTT scores were statistically correlated to group as expected, whereas in the “group × mean execution time” interaction, finger tapping scores and execution time were inversely related (p < 0.001). In the second model, we set as dependent variable the Δi2,000 and as independent factors the mean execution time, the total score, and interactions between them. We did not find any significant correlation.
Discussion
Here, we adopted two well-established experimental paradigms (32, 33), such as FTT and SM (synchronization and continuation), to study motor timing in two groups of TS patients, “pure” TS and TS+ADHD. While in free FTT, subjects tap in a freely chosen rhythm, in the synchronization and continuation task, the accuracy requires motor and perceptual timing fluctuation to replicate externally presented rhythm and memory-driven timing to continue tapping in the absence of the auditory cue (19).
In the FTT, we found that the control group scored better than both groups of TS patients when using their DH. Conversely, we found that controls scored better than “pure” TS patients also with non-DH, but not than the TS+ADHD group. In addition, controls and “pure” TS scored better with DH than with non-DH, and interestingly, this difference was not found in the TS+ADHD group. These findings deserve more clarification in light of existing data. Fine motor skills have been extensively studied in TS with conflicting results depending on simple or more complex tasks adopted (34–38) as well as on confounding factors, such as age of the experimental cohort, tic severity, and not least comorbidities. The main aim of our study was different; thus, unfortunately, we did not clearly clarify these contradictory results. On one side, we confirm the evidence that an altered organization of motor behavior occurs in TS as well as that motor skills are impaired in TS (both pure or +ADHD) in a single-hand trial; on the other hand, as expected from motor lateralization studies (30), we found a symmetric performance between DH and n-DH only in TS+ADHD and not in pure TS. The symmetry between DH and n-DH in motor performance of TS has been explained by compensatory interhemispheric plasticity mechanisms in TS (30, 39, 40). The apparent inconsistency of our results could be explained by the nature of the task (single for each hand vs. bimanual) and by other factors contributing to the development of symmetry of motor performance, such as the younger age of the cohort (34, 35). Finally, the finding that the TS+ADHD group showed a more symmetrical (poorer) performance with both hands may imply that symmetrical compensatory mechanisms can establish earlier in TS patients with comorbidity than in “pure” TS.
The main result of our study is that obtained in the synchronization and continuation task. “Pure” TS and TS+ADHD showed a poor motor timing organization with opposite performance in the 2,000 ms continuation task (supra-seconds interval), classifying “pure” TS as “behind the beat” and TS+ADHD as “ahead of the beat,” on the basis of their accuracy index. We also found that there is a lack of correlation between the timing accuracy and the tics severity scores (YGTSS), as well as with IQ scores. Martino et al. (29) recently reported motor timing performance in a cohort of adult TS patients, observing a reduced synchronization ability in the continuation condition for 2,000 ms interval. For the first time, our findings replicate this observation in a childhood cohort of “pure” TS. Furthermore, our study improves knowledge on motor timing in TS children, demonstrating that patients with comorbid ADHD scarcely synchronize as “pure” TS but with an opposite direction of accuracy (ahead of the beat) when compared with “pure” TS (see Figure 2).
ADHD comorbidity is considered a confounding factor generating conflicting results in TS studies (41). On the other hand, and strictly from a clinical point of view, ADHD accounts for at least 40% of the total comorbidity of TS patients (3, 42), and data are thought to be underestimated in several cases (43). Moreover, ADHD is one of the major components of clinical worsening of TS during lifespan (4, 44). Motor timing has been extensively studied in ADHD population with some diverging results, probably due to selection of the patients or due to different experimental conditions adopted (45–50). However, in a comprehensive review, Noreika et al. (27) concluded that both children and adults with ADHD tend to show premature responses and poor synchronization for sub-second and supra-second intervals, confirming a highly consistent pattern of motor timing abnormalities.
Motor timing is thought to be controlled by several cortical areas in conjunction with the basal ganglia and cerebellum, constituting the fronto-striato-cerebellar network (51). Additionally, functional MRI (fMRI) studies showed that sensorimotor synchronization is associated with the activation of cortical areas, such as dorsolateral frontal cortex (DLFC), inferior frontal cortex (IFC), medial frontal cortex (MFC), and supplementary motor area (SMA) [for a review, see (52)]. Moreover, Wiener et al. (53) found that bilateral anterior cingulate cortex (ACC), right SMA, dorsolateral prefrontal cortex (DLPFC), and inferior parietal cortex (IPC) were more involved in supra-second motor timing. After all, SMA plays a critical role in tics pathophysiology: SMA is strongly involved in tic generation in TS and also tic regulation during voluntary action (54–56). Ganos et al. (56) proposed that in TS, the right SMA seemed to act as a global inhibition mechanism in TS and was used to simultaneously stop tics and voluntary actions. Thus, SMA–striato-thalamo-cortical loop dysfunction could explain in “pure TS” patients their accuracy “behind the beat” in continuation tasks, as proposed also by Martino et al. (29). On the other hand, the opposite direction of accuracy index in TS+ADHD patients compared with pure TS leads to that other mechanisms occur. In TS+ADHD patients, it could be imagined that pre-frontal areas, such as ACC and DLPFC, may mediate the anticipatory performance for a lack of inhibitory control (impulsiveness) (49). Thus, our findings confirm once more that it is important to consider all endophenotypes in studying a puzzling complex syndrome as Tourette.
In addition, we tested patients (and controls) for their planning and decision-making abilities by performing a standardized task, such as ToL test, as measure of their “executive” function. We found that the pure TS and TS+ADHD groups compared with the control group showed lower scores in the total score, whereas the total execution time was lower in the TS+ADHD group. Planning skills refer to the capacity to organize cognitive and motor behavior in order to perform different steps needed to reach a goal (57). Termine et al. (58) showed in a small cohort of children that both pure TS and TS+ADHD patients show various impairments during the ToL test. Interestingly, we found an inverse correlation between finger tapping scores for DH and the total score and the mean execution time of the ToL test. These findings suggest, once more, that an impairment of “planning skills” in TS contributes to an impairment of the organization of motor behavior in free tapping.
However, we did not find any correlation between continuation ability and ToL performance, suggesting that not all organization of motor timing is dependent from planning ability, and this is true for both groups independently from ADHD co-occurrence.
Finally, some limitations of the study need to be addressed. First, note the small sample size of our cohort and, in particular, of the TS+ADHD group. Second, as a pure ADHD group is lacking, our results cannot be readily transferred to ADHD patients. Finally, although all our efforts have been made to minimize confounding factors, we cannot exclude intrinsic (due to referral) bias in the selection of patients.
In conclusion, we demonstrated that children with TS show an impaired motor timing organization in the free tapping and in the continuation task (tempo synchronization). Moreover, findings in TS+ADHD confirm that, at least in part, TS groups differ, signifying that ADHD co-occurrence should be considered in all studies involving TS to not exclude possible confounding effect.
Data Availability Statement
The raw data supporting the conclusions of this article will be made available by the authors, without undue reservation.
Ethics Statement
The studies involving human participants were reviewed and approved by Bambino Gesù Local Ethical Committee. Written informed consent to participate in this study was provided by the participants' legal guardian/next of kin.
Author Contributions
FG, CP, and AC contributed to the conception and design of the study. CP, FG, and LD organized the database. FG and AC performed the statistical analysis. FG wrote the first draft of the manuscript. FG, AC, PC, and FV wrote sections of the manuscript and critically discussed the final version. All authors contributed to manuscript revision, read and approved the submitted version.
Funding
This study was partially funded by Ricerca Corrente of the Italian Ministry of Health.
Conflict of Interest
The authors declare that the research was conducted in the absence of any commercial or financial relationships that could be construed as a potential conflict of interest.
The handling Editor declared a shared affiliation, though no other collaboration, with some of the authors FG, LD, and PC.
The reviewer TS declared a shared affiliation, with no collaboration with some of the authors FG, LD, and PC to the handling Editor.
Supplementary Material
The Supplementary Material for this article can be found online at: https://www.frontiersin.org/articles/10.3389/fneur.2020.552701/full#supplementary-material
Abbreviations
ADHD, attention-deficit/hyperactivity disorder; OCD, obsessive–compulsive disorder; CBCL, Child Behavior Checklist; CPRS, Conners' Parent Rating Scale; CSTC, cortico-striato-thalamo-cortical; FTT, finger-tapping test; IQ, intelligence quotient; SD, standard deviation; SM, sensorimotor test; SMA, supplementary motor area; ToL, Tower of London; TS, Tourette syndrome; YGTSS, Yale Global Tic Severity Scale.
References
1. American Psychiatric Association. Diagnostic and Statistical Manual of Mental Disorders. 5th ed. Washington, DC: American Psychiatric Association (2013). doi: 10.1176/appi.books.9780890425596
2. Robertson MM. The prevalence epidemiology of Gilles de la Tourette syndrome. Part. 2: Tentative explanations for differing prevalence figures in GTS, including the possible effects of psychopathology, aetiology, cultural differences, differing phenotypes. J Psychosom Res. (2008) 65:473–86. doi: 10.1016/j.jpsychores.2008.03.007
3. Freeman RD, Fast DK, Burd L, Kerbeshian J, Robertson MM, Sandor P. An international perspective on Tourette syndrome: selected findings from 3500 individuals in 22 countries. Dev Med Child Neurol. (2000) 42:S0012162200000839. doi: 10.1017/S0012162200000839
4. Rizzo R, Gulisano M, Pellico A, Calì PV, Curatolo P. Tourette syndrome comorbid conditions: a spectrum of different severities complexities. J. Child Neurol. (2014) 29:1383–9. doi: 10.1177/0883073814534317
5. Stafford M, Cavanna AE. Prevalence and clinical correlates of self-injurious behavior in tourette syndrome. Neurosci Biobehav Rev. (2020) 113:299–307. doi: 10.1016/j.neubiorev.2020.03.022
6. Budman CL, Bruun RD, Park KS, Lesser M, Olson M. Explosive outbursts in children with Tourette's disorder. J Am Acad Child Adolesc Psychiatry. (2000) 39:1270–6. doi: 10.1097/00004583-200010000-00014
7. Cavanna AE, Rickards H. The psychopathological spectrum of Gilles de la Tourette syndrome. Neurosci Biobehav Rev. (2013) 37:1008–15. doi: 10.1016/j.neubiorev.2012.10.011
8. Rasmussen C, Soleimani M, Carroll A, Hodlevskyy O. Neuropsychological functioning in children with Tourette syndrome (TS). J Can Acad Child Adolesc Psychiatry. (2009) 18:307–15.
9. Morand-Beaulieu S, Leclerc JB, Valois P, Lavoie ME, O'Connor KP, Gauthier B. A review of the neuropsychological dimensions of tourette syndrome. Brain Sci. (2017) 7:106. doi: 10.3390/brainsci7080106
10. Hovik KT, Plessen KJ, Cavanna AE, Skogli EW, Andersen PN, Øie M. Cognition, emotion and behavior in children with tourette's syndrome and children with ADHD-combined subtype - A two-year follow-up study. PLoS ONE. (2015) 10:e0144874. doi: 10.1371/journal.pone.0144874
11. McAuley JD, Jones MR, Holub S, Johnston HM, Miller NS. The time of our lives: Life span development of timing and event tracking. J Exp Psychol Gen. (2006) 135:348–67. doi: 10.1037/0096-3445.135.3.348
12. Treisman M. Temporal discrimination and the indifference interval. Implications for a model of the “internal clock” Psychol Monogr. (1963) 77:1–31. doi: 10.1037/h0093864
13. Yin HH. Action, time and the basal ganglia. Philos Trans R Soc B Biol Sci. (2014) 369. doi: 10.1098/rstb.2012.0473
14. Matell MS, Meck WH. Cortico-striatal circuits and interval timing: coincidence detection of oscillatory processes. Brain Res Cogn Brain Res. (2004) 21:139–70. doi: 10.1016/j.cogbrainres.2004.06.012
15. Mazzone L, Yu S, Blair C.l., Gunter B, Wang Z, Marsh R, et al. An fMRI study of frontostriatal circuits during the inhibition of eye blinking in persons with tourette syndrome. Am J Psychiatry. (2010) 167:341–9. doi: 10.1176/appi.ajp.2009.08121831
16. Jung J, Jackson SR, Parkinson A, Jackson GM. Cognitive control over motor output in Tourette syndrome. Neurosci Biobehav Rev. (2013) 37:1016–25. doi: 10.1016/j.neubiorev.2012.08.009
17. Morand-Beaulieu S, Grot S, Lavoie J, Leclerc JB, Luck D, Lavoie ME. The puzzling question of inhibitory control in Tourette syndrome: a meta-analysis. Neurosci Biobehav Rev. (2017) 80:240–62. doi: 10.1016/j.neubiorev.2017.05.006
18. Allman MJ, Meck WH. Pathophysiological distortions in time perception and timed performance. Brain. (2012) 135:656–77. doi: 10.1093/brain/awr210
19. Wittmann M, Leland DS, Churan J, Paulus MP. Impaired time perception and motor timing in stimulant-dependent subjects. Drug Alcohol Depend. (2007) 90:183–92. doi: 10.1016/j.drugalcdep.2007.03.005
20. Anagnostou E, Mitsikostas DD. Time perception in migraine sufferers: An experimental matched-pairs study. Cephalalgia. (2005) 25:60–7. doi: 10.1111/j.1468-2982.2004.00809.x
21. Wittmann M, Simmons AN, Flagan T, Scott LD, Wackermann J, Paulus MP. Neural substrates of time perception and impulsivity. Brain Res. (2011) 2011:43–58. doi: 10.1016/j.brainres.2011.06.048
22. Zhang J, Nombela C, Wolpe N, Barker RA, Rowe JB. Time on timing: Dissociating premature responding from interval sensitivity in Parkinson's disease. Mov Disord. (2016) 31:1163–72. doi: 10.1002/mds.26631
23. Avanzino L, Pelosin E, Vicario CM, Lagravinese G, Abbruzzese G, Martino D. Time processing and motor control in movement disorders. Front Hum Neurosci. (2016) 10:11–8. doi: 10.3389/fnhum.2016.00631
24. Pedrosa DJ, Nelles C, Maier F, Eggers C, Burghaus L, Fink GR, et al. Time reproduction deficits in essential tremor patients. Mov Disord. (2016) 31:1234–40. doi: 10.1002/mds.26630
25. Vicario CM, Martino D, Spata F, Defazio G, Giacch è R, Martino V, et al. Time processing in children with Tourette's syndrome. Brain Cogn. (2010) 73:28–34. doi: 10.1016/j.bandc.2010.01.008
26. Vicario CM, Gulisano M, Martino D, Rizzo R. Timing recalibration in childhood Tourette syndrome associated with persistent pimozide treatment. J Neuropsychol. (2016) 10:211–22. doi: 10.1111/jnp.12064
27. Noreika V, Falter CM, Rubia K. Timing deficits in attention-deficit/hyperactivity disorder (ADHD): Evidence from neurocognitive and neuroimaging studies. Neuropsychologia. (2013) 51:235–66. doi: 10.1016/j.neuropsychologia.2012.09.036
28. Coull JT, Hwang HJ, Leyton M, Dagher A. Dopamine precursor depletion impairs timing in healthy volunteers by attenuating activity in putamen and supplementary motor area. J Neurosci. (2012) 32:16704–15. doi: 10.1523/JNEUROSCI.1258-12.2012
29. Martino D, Hartmann A, Pelosin E, Lagravinese G, Delorme C, Worbe Y, et al. Motor Timing in Tourette Syndrome: The Effect of Movement Lateralization and Bimanual Coordination. Front Neurol. (2019) 10:385. doi: 10.3389/fneur.2019.00385
30. Avanzino L, Martino D, Bove M, De Grandis E, Tacchino A, Pelosin E, et al. Movement lateralization and bimanual coordination in children with Tourette syndrome. Mov Disord. (2011) 26:2114–8. doi: 10.1002/mds.23839
31. Droit-Volet S. Time perception in children: A neurodevelopmental approach. Neuropsychologia. (2013) 51:220–34. doi: 10.1016/j.neuropsychologia.2012.09.023
32. Shimoyama I, Ninchoji T, Uemura K. The finger-tapping test. A quantitative analysis Arch Neurol. (1990) 47:681–4. doi: 10.1001/archneur.1990.00530060095025
33. Carlier M, Dumont AM, Beau J, Michel F. Hand performance of French children on a finger-tapping test in relation to handedness, sex, and age. Percept Mot Skills. (1993) 76:931–40. doi: 10.2466/pms.1993.76.3.931
34. Georgiou N, Bradshaw JL, Phillips JG, Cunnington R, Rogers M. Functional asymmetries in the movement kinematics of patients with Tourette's syndrome. J Neurol Neurosurg Psychiatry. (1997) 63:188–95. doi: 10.1136/jnnp.63.2.188
35. Roessner V, Wittfoth M, August JM, Rothenberger A, Baudewig J, Dechent P. Finger tapping-related activation differences in treatment-naïve pediatric Tourette syndrome: a comparison of the preferred and non preferred hand. J Child Psychol Psychiatry. (2013) 54:273–9. doi: 10.1111/j.1469-7610.2012.02584.x
36. Buse J, August J, Bock N, Dörfel D, Rothenberger A, Roessner V. Fine motor skills and interhemispheric transfer in treatment-naive male children with Tourette syndrome. Dev Med Child Neurol. (2012) 54:629–35. doi: 10.1111/j.1469-8749.2012.04273.x
37. Bloch MH, Sukhodolsky DG, Leckman JF, Schultz RT. Fine-motor skill deficits in childhood predict adulthood tic severity and global psychosocial functioning in Tourette's syndrome. J Child Psychol Psychiatry. (2006) 47:551–9. doi: 10.1111/j.1469-7610.2005.01561.x
38. Sukhodolsky DG, Landeros-Weisenberger A, Scahill L, Leckman JF, Schultz RT. Neuropsychological functioning in children with Tourette syndrome with and without attention-deficit/hyperactivity disorder. J Am Acad Child Adolesc Psychiatry. (2010) 49:1155–64. doi: 10.1016/j.jaac.2010.08.008
39. Martino D, Delorme C, Pelosin E, Hartmann A, Worbe Y, Avanzino L. Abnormal lateralization of fine motor actions in Tourette syndrome persists into adulthood. PLoS ONE. (2017) 12:e0180812. doi: 10.1371/journal.pone.0180812
40. Cheng B, Braass H, Ganos C, Treszl A, Biermann-Ruben K, Hummel FC, et al. Altered intrahemispheric structural connectivity in Gilles de la Tourette syndrome. Neuroimage Clin. (2013) 4:174–81. doi: 10.1016/j.nicl.2013.11.011
41. Roessner V, Becker A, Banaschewski T, Freeman RD, Rothenberger A. Developmental psychopathology of children and adolescents with Tourette syndrome – impact of ADHD. Eur Child Adolesc Psychiatry. (2007) 16:24–35. doi: 10.1007/s00787-007-1004-6
42. Freeman RD, Tourette Syndrome International Database Consortium. Tic disorders and ADHD answers from a world-wide clinical dataset on Tourette syndrome. Eur Child Adolesc Psychiatry. (2007) 16, 15–23. doi: 10.1007/s00787-007-1003-7
43. Cavanna AE, Critchley HD, Orth M, Stern JS, Young MB, Robertson MM. Dissecting the Gilles de la Tourette spectrum: a factor analytic study on 639 patients. J Neurol Neurosurg Psychiatry. (2011) 82:1320–3. doi: 10.1136/jnnp.2010.225029
44. Groth C, Skov L, Lange T, Debes NM. Predictors of the clinical course of tourette syndrome: a longitudinal study. J Child Neurol. (2019) 34:913–21. doi: 10.1177/0883073819867245
45. Rubia K, Taylor A, Taylor E, Sergeant JA. Synchronization, anticipation and consistency of motor timing in dimensionally defined children with attention deficit hyperactivity disorder. Perceptual and Motor Skills. (1999) 89:1237–58. doi: 10.2466/pms.1999.89.3f.1237
46. Pitcher TM, Piek JP, Barrett NC. Timing and force control in boys with attention deficit hyperactivity disorder:Subtype differences and the effect of comorbid developmental coordination disorder. Human Mov Sci. (2002) 21:919–45 doi: 10.1016/S0167-9457(02)00167-7
47. Ben-Pazi H, Gross-Tsur V, Bergman H, Shalev RS. Abnormal rhythmic motor response in children with attention-deficit-hyperactivity disorder. Dev Med Child Neurol. (2003) 45:743–5. doi: 10.1111/j.1469-8749.2003.tb00883.x
48. Tiffin-Richards MC, Hasselhorn M, Richards ML, Banaschewski T, Rothenberger A. Time reproduction in finger tapping tasks by children with attention-deficit hyperactivity disorder and/or dyslexia. Dyslexia. (2004) 10:299–315. doi: 10.1002/dys.281
49. Rubia K, Halari R, Christakou A, Taylor E. Impulsiveness as a timing disturbance: neurocognitive abnormalities in attention-deficit hyperactivity disorder during temporal processes and normalization with methylphenidate. Philos Trans R Soc Lond B Biol Sci. (2009) 364:1919–31 doi: 10.1098/rstb.2009.0014
50. Zelaznik HN, Vaughn AJ, Green JT, Smith AL, Hoza B, Linnea K. Motor timing deficits in children with attention-deficit/hyperactivity disorder. Hum Mov Sci. (2012) 31:255–65. doi: 10.1016/j.humov.2011.05.003
51. Merchant H, Harrington DL, Meck WH. Neural basis of the perception and estimation of time. Annu Rev Neurosci. (2013) 36:313–36. doi: 10.1146/annurev-neuro-062012-170349
52. Rubia K. The neural correlates of timing functions. In: Myslobodsky JG, editor. Timing the Future: The Case for a Time-Based Prospective Memory. Hackensack, NJ: World Scientific Publishing. (2006). p. 213–38. doi: 10.1142/9789812707123_0009
53. Wiener M, Turkeltaub P, Coslett HB. The image of time: a voxel-wise meta-analysis. Neuroimage. (2010) 49:1728–40 doi: 10.1016/j.neuroimage.2009.09.064
54. Bohlhalter S, Goldfine A, Matteson S, Garraux G, Hanakawa T, Kansaku K, et al. Neural correlates of tic generation in Tourette syndrome: an event-related functional MRI study. Brain. (2006) 129:2029–37. doi: 10.1093/brain/awl050
55. Hampson M, Tokoglu F, King RA, Constable RT, Leckman JF. Brain areas coactivating with motor cortex during chronic motor tics and intentional movements. Biol Psychiatry. (2009) 65:594–9. doi: 10.1016/j.biopsych.2008.11.012
56. Ganos C, Kühn S, Kahl U, Schunke O, Feldheim J, Gerloff C, et al. Action inhibition in Tourette syndrome. Mov Disord. (2014) 29:1532–8. doi: 10.1002/mds.25944
57. Shallice T. Specific impairments of planning. Philos Trans R Soc Lond B Biol Sci. (1982) 298:199–209. doi: 10.1098/rstb.1982.0082
Keywords: Tourette syndrome, ADHD, motor timing, synchronization ability, finger tapping, Tower of London
Citation: Graziola F, Pellorca C, Di Criscio L, Vigevano F, Curatolo P and Capuano A (2020) Impaired Motor Timing in Tourette Syndrome: Results From a Case–Control Study in Children. Front. Neurol. 11:552701. doi: 10.3389/fneur.2020.552701
Received: 17 April 2020; Accepted: 28 September 2020;
Published: 29 October 2020.
Edited by:
Antonio Pisani, University of Pavia, ItalyReviewed by:
Tommaso Schirinzi, University of Rome Tor Vergata, ItalyLaura Avanzino, University of Genoa, Italy
Matteo Bologna, Sapienza University of Rome, Italy
Copyright © 2020 Graziola, Pellorca, Di Criscio, Vigevano, Curatolo and Capuano. This is an open-access article distributed under the terms of the Creative Commons Attribution License (CC BY). The use, distribution or reproduction in other forums is permitted, provided the original author(s) and the copyright owner(s) are credited and that the original publication in this journal is cited, in accordance with accepted academic practice. No use, distribution or reproduction is permitted which does not comply with these terms.
*Correspondence: Alessandro Capuano, YWxlc3NhbmRyby5jYXB1YW5vQG9wYmcubmV0